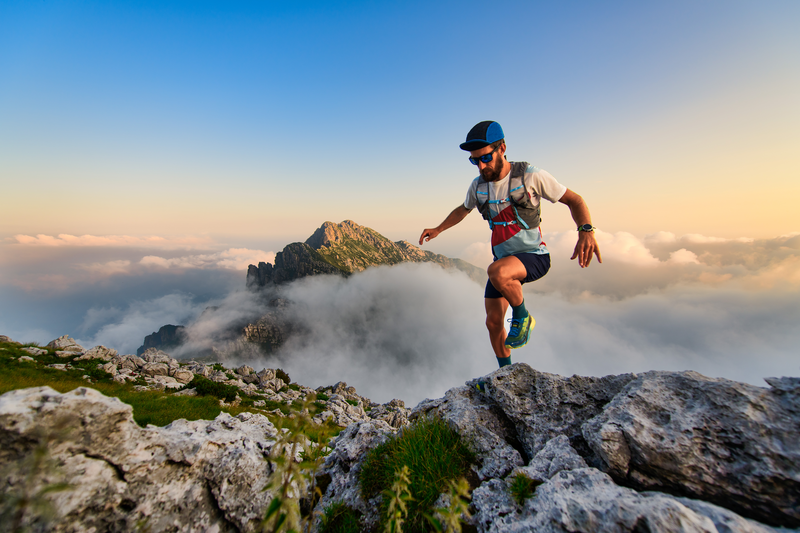
95% of researchers rate our articles as excellent or good
Learn more about the work of our research integrity team to safeguard the quality of each article we publish.
Find out more
REVIEW article
Front. Pharmacol. , 23 September 2022
Sec. Ethnopharmacology
Volume 13 - 2022 | https://doi.org/10.3389/fphar.2022.1014173
This article is part of the Research Topic Bone and Cartilage Diseases – The Role and Potential of Natural Products, Volume II View all 6 articles
Natural alkaloids are polycyclic, nitrogen-containing, and basic compounds obtained from plants. In this review, the advances in bioactive alkaloids with respect to their chemical structures, herbal sources, and effects for the prevention and treatment of osteoporosis are discussed. Anti-osteoporosis alkaloids are classified into six categories based on the chemical structure, namely, isoquinoline alkaloids, quinolizidine alkaloids, piperidine alkaloids, indole alkaloids, pyrrolizidine alkaloids and steroidal alkaloids. They promote mesenchymal stem cells differentiation, improve osteoblast proliferation, stimulate osteoblast autophagy and suppress osteoclast formation. These natural alkaloids can regulate multiple signaling pathways, including interrupting the tumor necrosis factor receptor associated factor 6- receptor activator of nuclear factor kappa B interaction, inhibiting the nuclear factor kappa B pathway in osteoclasts, activating the p38 mitogen-activated protein kinases pathway in osteoblasts, and triggering the wingless and int-1 pathway in mesenchymal stem cells. This review provides evidence and support for novel drug and clinical treatment of osteoporosis using natural alkaloids.
Osteoporosis has become a major global public health problem, which is manifested by reduced bone strength, leading to an increased risk of fracture (Langdahl, 2021). Many different cell types are involved in the bone remodeling, including cells of the osteoblast lineage and those of the osteoclast lineages (Kenkre and Bassett, 2018). Osteoblasts secrete type I collagen, alkaline phosphatase (ALP) and osteocalcin to form osteoid (Kim et al., 2020). Osteoclasts are derived from the monocyte/macrophage hematopoietic precursors and responsible for the bone resorption (Henriksen et al., 2014). Bone destruction promotes the movement of marrow mesenchymal stem cells (MSCs) to new excavated sites, where they differentiate into osteoblasts and enhance the bone formation (Song et al., 2022). Several pathways play a crucial role in the maintenance of bone homeostasis. For example, calcium signaling controls proliferation, differentiation and transcription in bone cells (Kang J. Y. et al., 2020). Osteoprotegerin (OPG) is primarily synthesized in osteoblasts and inhibits osteoclastogenesis by binding to the receptor activator of nuclear factor kappa B ligand (RANKL) (Ono et al., 2020; Yasuda, 2021) and preventing it from binding to its receptor, receptor activator of nuclear factor kappa B (RANK) (Taylan et al., 2019; Xu et al., 2021). Wingless and int-1(Wnt)/β-catenin signaling regulates the survival of osteoblasts and differentiation of MSCs (Stuerznickel et al., 2021). Additionally, several pathways are involved in the apoptosis of bone cells, such as nuclear factor-kappa B (NFκB), mitogen-activated protein kinases (MAPK), and mammalian targe of rapamycin (mTOR) signaling pathways (Wong et al., 2019). Drugs targeting these signaling pathways may be valuable for preventing or treating osteoporosis.
Calcium and vitamin D are the recommended nutrients for patients with osteoporosis (Kahwati et al., 2018). Pharmacological interventions include antiresorptive agents that inhibit bone resorption and anabolic agents that promote bone formation (Seeman and Martin, 2019). Classical drugs include bisphosphonates, denosumab, strontium, teriparatide, calcitonin and estrogen (Martiniakova et al., 2020). However, the long-term use of these drugs is always accompanied by adverse side effects, such as esophagitis for bisphosphonates (Fan and Wang, 2020), cellulitis for denosumab (Zhang N. et al., 2020), nasal congestion for calcitonin (Zhang et al., 2021) and an increased risk of breast cancer for estrogens (Black and Rosen, 2016). More effective treatment methods for osteoporosis are desirable (Lin et al., 2019; Xie et al., 2021).
Alkaloids represent a large class of nitrogen-containing phytochemicals widely present in medicinal plants (Xie et al., 2021) and food (Rasouli et al., 2020). There are more than ten thousand different natural alkaloids, which can be classified according to their carbon skeletal structures (Ziegler and Facchini, 2008). With the continuous development of modern medication, various biopotentials of natural alkaloids have been explored, including antioxidant (Badaoui et al., 2021), antiproliferative (Hu et al., 2018), anti-obesity (Saunders et al., 2018), antidiabetic (Jan et al., 2018), cardiovascular protection (Li et al., 2021a), anti-aging (Asokan et al., 2018), and anti-inflammatory (Hu et al., 2020) effects in experimental trials and clinical applications. At present, natural alkaloids are the mainstream anti-osteoporosis drugs because of their strong efficacy and low toxicity (Ajebli et al., 2021), and some of them have been used clinically. The main groups of anti-osteoporotic alkaloids include isoquinoline, quinolizidine, piperidine, indole, pyrrolizidine and steroidal alkaloids. This review focuses on studies in the field of natural alkaloids in osteoporosis.
Isoquinoline alkaloids are derived from tyrosine or phenylalanine (Supplementary Figure S1), and exhibit various bioactivities, such as antitumor, antibacterial, and anti-inflammatory (Shang et al., 2020). Eight isoquinoline alkaloids possess anti-osteoporotic activity, including berberine, tetrahydropalmatine, boldine, tetrandrine, fangchinoline, sinomenine, cepharanthine, and nitidine.
Berberine and tetrahydropalmatine have been isolated from the genus Coptis (Li et al., 2019) and Corydalis (Ma et al., 2009). Oral administrations of these two compounds inhibit bone loss in many osteoporosis models, such as diabetic mice (Adil et al., 2017; Xie et al., 2018), aged mice (Chen Q. C. et al., 2021), ovariectomized (OVX)-treated rats (He et al., 2017; Zhi et al., 2020) and glucocorticoid-induced mice (Xu et al., 2010) (Table 1). However, berberine undergoes extensive metabolism after oral administration, resulting in low plasma exposure (Fan et al., 2021). Hundreds of berberine derivatives have been prepared and the structure modifications are focused on the C8, C9, C10, C12 and C13 positions. For example, 13-alkyl-substituted berberine showed better anti-inflammation activities than berberine (Zou et al., 2017). The anti-osteoporosis effects and structure-activity relationship of berberine derivatives need to be further clarified.
Boldine, an aporphine alkaloid from the genus Peumus (O'Brien et al., 2006), protects against OVX-induced osteoporosis by downregulating bone resorption without affecting bone formation (Chen et al., 2018). Tetrandrine (Luan et al., 2020) and fangchinoline (Merarchi et al., 2018), two benzylisoquinoline alkaloids derived from Stephania tetrandra S. More, exhibit notable therapeutic effects in OVX (Zhou L. et al., 2020; Zhong et al., 2020), sciatic-neurectomized (Takahashi et al., 2012), titanium particle (Liu Z. et al., 2020), and prednisolone-induced models (Zhu et al., 2019). Fangchinoline differs from tetrandrine in one side chain of its isoquinoline ring. This minor structural difference leads to a considerable difference in their anti-inflammatory effects. Specifically, tetrandrine had good suppression on murine interleukin-5 and human interleukin-6, but fangchinoline only on human interleukin-6 (Choi et al., 2000). Nevertheless, the differences of their anti-osteoporosis effects remain unclear.
Sinomenine is an emetine isoquinoline alkaloids derived from Sinomenium acutum (Thunb.) Rehder and E.H.Wilson, which attenuated bone loss in a Mycobacterium tuberculosis H37Ra-induced model (Li et al., 2013). Lycorine, a phenanthridine alkaloids from the Amaryllidaceae family (Roy et al., 2018), can protect against OVX-induced and wear particle-induced osteolysis (Chen et al., 2015). Cepharanthine from Stephania abyssinica (quart.-dill. and A. Rich.) Walp. (Bailly, 2019) and nitidine from Zanthoxylum nitidum (Roxb.) DC. (Khan et al., 2018) could prevent bone loss in OVX-induced model by reducing osteoclast differentiation (Liu et al., 2016; Zhou et al., 2018).
Piperidine alkaloids possess a characteristic saturated heterocyclic ring structure (Green et al., 2012). Piperine is isolated from the Piperaceae family (Haq et al., 2021), and alleviates bone resorption in RANKL-induced RAW 264.7 (Deepak et al., 2015). Arecoline from Areca catechu L. (Volgin et al., 2019) reduced osteoclastic tartrate-resistant acid phosphatase (TRAP) activity and differentiation of macrophage colony-stimulating factor (M-CSF)/RANKL-induced bone marrow monocytes (BMMs) and lipopolysaccharide (LPS)-induced mice (Liu F. L. et al., 2020).
Quinolizidine alkaloids are chemically divided into matrine-type and aloperine-type alkaloids (Wang et al., 2019; Li Y. et al., 2020). Matrine and oxymatrine belong to the matrine-type and are derived from Sophora flavescens Aiton (Wang et al., 2019). Matrine suppresses osteoclastogenesis in OVX mice (Chen et al., 2017). Oxymatrine attenuates osteoclast formation in RANKL-induced BMMs and OVX-induced mice (Jiang et al., 2021). Neither of them has an effect on bone formation, indicating that they improve osteoporosis mainly by inhibiting osteoclastogenesis. Aloperine is found in the genus Sophora (Zhou H. et al., 2020). It attenuates osteoporosis in OVX-treated mice by inhibiting osteoclastogenesis (Hu et al., 2021). Cytisine is isolated from the Leguminosae family and can attenuate bone loss in OVX-treated mice (Qian et al., 2020).
Indole alkaloids originate from condensation of tryptophan and a terpene moiety (Matsuura et al., 2014). Three anti-osteoporotic compounds have been identified in this class. Vindoline from Catharanthus roseus (L.) G. Don, and rutaecarpine from Acronychia acronychioides (F.Muell.) Hartley (Tian et al., 2019) have inhibitory effects on osteoclast differentiation from BMMs and mature osteoclastic bone resorption (Zhan et al., 2020; Li et al., 2021b). Notably, harmine from Peganum harmala L. (Zhang et al., 2020a; Huang et al., 2021) prevented bone loss by enhancing type H vessel formation and decreasing fat cells in the femora of OVX-induced mice (Huang et al., 2018). The structure-activity relationship analysis showed that the C3–C4 double bond and 7-methoxy group of harmine are crucial for its suppression on osteoclast differentiation (Yonezawa et al., 2011).
Pyrrolizidine alkaloids are structurally characterized by a 1-azabicyclo azaoctane or aza-bridged pentalene skeleton (Robertson and Stevens, 2014). These alkaloids are secondary metabolites of the genus Senecio, Crotalaria, Echium, and Eupatorim (Brugnerotto et al., 2021). Neotuberostemonine, a pyrrolizidine alkaloid derived from Stemona tuberosa Lour., can inhibit osteoclastogenesis induced by RANKL (Yun et al., 2019). Stachydrine is the major bioactive component of Leonurus japonicus Houtt. (Cheng et al., 2020). It inhibited bone resorption in RANKL-induced osteoclasts, OVX-treated mice (Chen M. et al., 2021), and LPS-induced mice (Meng et al., 2019).
Steroidal alkaloids have a basic steroidal skeleton with a nitrogen atom incorporated as an integral part of the compound (Jiang et al., 2016). They are generally found in the glycoalkaloid form (Dey et al., 2019) and exert various pharmacological properties such as anti-inflammatory, anti-cancer, anti-microbial, and analgesic activities (Jiang et al., 2016). Tomatidine is abundant in the skin of unrip green tomatoes (Bailly, 2021) and can be used to treat osteoporosis. It can attenuate IL-1β-induced degradation of collagen-II by inhibiting NFκB and MAPK signaling in articular chondrocytes and osteoarthritic rats. Tomatidine also improves OVX-induced osteoporosis by suppressing osteoclastogenesis (Hu et al., 2019).
RANKL is the key regulator of osteoclast differentiation and activity (Kim et al., 2020). It is a TNF related ligand generated on the surface membrane of osteoblasts (Epsley et al., 2021) that functions as an agonistic ligand for the receptor (RANK) (Nagy and Penninger, 2015). It has been proved that RANKL-knockout mice were protected from bone loss during arthritis, suggesting that osteoclastic bone resorption under inflammatory conditions is dependent on RANKL-RANK signaling (Tsukasaki et al., 2017). Previous reports showed that the treatment of several natural alkaloids can inhibit the RANKL synthesis or interrupt the interaction between RANKL and RANK in vitro and in vivo, such as sinomenine (Li et al., 2013; Zhou et al., 2017), arecoline (Liu F. L. et al., 2020), and oxymatrine (Jiang et al., 2018; Jiang et al., 2021). In osteoclasts, RANKL-RANK signaling stimulation activates tumor necrosis factor receptor-associated factor 6 (TRAF6) (Park et al., 2017), thereby upregulating NFκB (Walsh et al., 2015) and MAPK (Shi and Sun, 2018), and expression of c-Fos (Takayanagi, 2005). These factors initially induce NFATc1, which is the master regulator of osteoclastogenic genes (Oh et al., 2021). During osteoclast differentiation, TRAF6 is a dominant adaptor of RANK that assembles signaling proteins that direct osteoclast-specific gene expression resulting in differentiation and activation (Ikeda and Takeshita, 2016). Thus, the excessive interaction of RANK with TRAF6 contributes to the elevated resorption activity of osteoclasts during the development of osteoporosis. Tetrahydropalmatine decreases osteoclastogenesis by blocking the RANK-TRAF6 interaction (Zhi et al., 2020) in BMMs and RAW264.7 cells. Tomatidine (Hu et al., 2019) and neotuberostemonine block TRAF6 and NFκB activation and impair the formation of F-actin ring structure in RANKL-induced osteoclasts (Yun et al., 2019). Cytisine attenuates bone loss by inhibiting the v-akt murine thymoma viral oncogene homolog 1 (AKT)-NFATC1 pathway and RANKL-induced association of RANK-TRAF6 in OVX-treated mice (Qian et al., 2020).
In addition to RANKL-RANK signaling, inflammatory stimuli (IL-1, IL-6, lipopolysaccharide, TNF-α, etc.) increase osteoclast activation and survival through the NFκB (Zhao et al., 2021) and MAPK pathways (Liao et al., 2021). Indeed, increased levels of inflammatory cytokines are relevant characteristics of primary and secondary osteoporosis (Locantore et al., 2020; Ratajczak et al., 2020). Several chronic inflammatory diseases exhibit a detrimental effect on bone homostasis, such as inflammatory bowel disease (Wu et al., 2020), arthritis (Adami and Saag, 2019) and persistent skin inflammation (Mizutani et al., 2020). For instance, high contents of pro-inflammatory cytokines have been showed to be closely associated with osteoclast-mediated focal bone resorption (Coury et al., 2019). Additionally, postmenopausal women always display a chronic inflammatory phenotype with increased levels of circulating inflammatory factors, which contributes to impaired bone formation during menopause (Fischer et al., 2018). Proinflammatory cytokines are also remarkably elevated during senescence and stimulate osteoclast function during the development of senile osteoporosis (De Martinis et al., 2006). Thus, inflammation may be a pivotal target for the development of effective pharmacological interventions. Berberine and tetrahydropalmatine suppressed bone resorption by decreasing the levels of serum TNF-α and IL-6 in OVX mice (He et al., 2017; Zhi et al., 2020). Berberine inhibits osteoclast activity by suppressing NFκB and AKT signaling (Hu et al., 2008). Tomatidine (Hu et al., 2019), tetrandrine (Zhong et al., 2020) and fangchinoline (Zhou L. et al., 2020) can inhibit osteoclastogenesis and decrease TNF-α, IL-6 and the RANKL/OPG ratio by suppressing NFκB and MAPK signaling. Lycorine protected against osteolysis by inhibiting MAPK signaling in OVX-induced and wear particle-induced models (Chen et al., 2015). Aloperine (Hu et al., 2021) and matrine (Jiang et al., 2021) attenuated inflammation and abrogated RANKL-induced stimulation of the NFκB, AKT and MAPK pathways in OVX mice. Stachydrine inhibits the suppression of NFκB and p38 MAPK signaling pathways in RANKL-induced osteoclasts, OVX-treated mice (Chen M. et al., 2021) and LPS-induced mice (Meng et al., 2019).
NFATc1 is a dominant membrane of the NFAT transcription factor family, which is vital in the immune system during osteoporosis (Gu et al., 2020). Nuclear translocation of NFATc1 promotes microphthalmia-associated transcription factor (MITF) expression (Yang et al., 2019), autoactivates the Nfatc1 gene, and stimulates the expression of osteoclast-specific genes by collaborating with other transcription factors (Lorenzo, 2017; Chen et al., 2019). The Nfatc1 gene has been shown to be methylated in over 30% of postmenopausal women, and the Fos gene is methylated in 17% of them (Kalkan and Tosun, 2020). Tetrahydropalmatine (Zhi et al., 2020), oxymatrine (Jiang et al., 2021) and cepharanthine (Zhou et al., 2018) reduce osteoclast differentiation by inhibiting NFATc1 activation in OVX-induced models. Harmine (Yonezawa et al., 2011), sinomenine (Zhang Y. et al., 2019) and piperine (Deepak et al., 2015) inhibit the c-Fos/NFATc1 pathway in RANKL-induced RAW 264.7 macrophages. Oxymatrine (Jiang et al., 2021) and arecoline (Liu F. L. et al., 2020) suppressed the c-Fos/NFATc1 pathway in M-CSF/RANKL-induced BMMs and LPS-induced mice.
RANKL induces the oscillatory changes in the intracellular calcium contents, resulting in the calcium/calcineurin-dependent dephosphorylation, NFATc1 activation, and osteoclastic differentiation (Kang M. A. et al., 2020). Tetrandrine treatment can decrease calcium oscillation in RANKL-induced BMMs (Zhong et al., 2020). LPS exposure enhances calcium entry in osteoclasts by stimulating the expression of RNAKL (Zeng et al., 2020). Sinomenine treatment decreased intracellular calcium in LPS-induced osteoclasts (He et al., 2016).
Osteoblasts are responsible for bone formation and the creation of a physical framework (Hojo and Ohba, 2020). Runt-related transcription factor 2 (Runx2) is an essential transcription factor for osteoblast differentiation and bound to an osteoblast-specific cis-regulatory element in the promoter region of the Bglap gene (Komori, 2018). Among alkaloids, sinomenine activates the AKT/Runx2 pathway in osteoblasts (Zhang B. et al., 2019). MAPK activation of Runx2 occurs both in vivo and in vitro (An et al., 2016). In fact, p38 MAPK phosphorylation is enhanced during osteoblast differentiation, whereas inhibition of this pathway impairs pre-osteoblast differentiation and decreases osteoblast markers (Kang J. Y. et al., 2020). Berberine treatment promotes osteoblast differentiation by upregulating the expression of phosphorylated p38 MAPK (Lee et al., 2008). In addition, as a stress-responsive mechanism, autophagy safeguards osteoblasts against oxidative or inflammatory stress by degrading damaged organelles (Li X. et al., 2020). Fangchinoline protects against prednisolone-induced osteoporosis by inducing autophagy and inhibiting apoptosis in osteoblasts (Zhu et al., 2019). Thus, alkaloids could provide a therapeutic effect on bone formation through activation of the p38 MAPK pathway and autophagy in osteoblasts.
Osteoblasts are derived from MSCs. As a common progenitor, lineage commitment of MSCs to osteoblasts plays an important role in maintaining bone homeostasis (Chen et al., 2016). Numerous studies have provided strong evidence for the role of Wnt signaling in facilitating osteogenic differentiation and inhibiting adipogenic differentiation of MSCs (Han et al., 2019). Berberine can promote osteogenic differentiation of MSCs by increasing the transcriptional activity of β-catenin/T-cell factor (Tao et al., 2016).
Pharmacological studies of natural alkaloids on the efficacy and mechanisms of osteoporosis treatment have made remarkable progress. Based on the information collected in this study, the natural alkaloids exert anti-osteoporotic effects mainly by inhibiting bone resorption (Figure 1). Alkaloids reduce osteoclastogenesis through the RANKL-dependent pathways, including TRAF6, NFκB, MAPK, and NFATc1 signaling pathways. The RANKL-independent mechanisms behind the anti-osteoporosis effects of alkaloids remain elusive. Moreover, three main pathways are involved in the osteogenesis promotion effects of the alkaloids. The screening for alkaloids with osteogenic effects should be further carried out. Importantly, the structure-activity relationship investigations need to be performed to provide more evidence for drug development in osteoporosis.
Acquisition of data (BL and PX), analysis of data (JZ, XD, QY, and ZH), conception and drafting of the work (NW).
This work was supported by the National Natural Science Foundation of China (81973447), Zhejiang Provincial Natural Science Foundation of China (LY21H280001), Zhejiang Science and Technology Project (2021C03047), China Postdoctoral Science Foundation (2020M681364) and Zhejiang Provincial Medicine Foundation (2021ZQ018, 2021ZYYFC8, 2022ZX002).
The authors declare that the research was conducted in the absence of any commercial or financial relationships that could be construed as a potential conflict of interest.
All claims expressed in this article are solely those of the authors and do not necessarily represent those of their affiliated organizations, or those of the publisher, the editors and the reviewers. Any product that may be evaluated in this article, or claim that may be made by its manufacturer, is not guaranteed or endorsed by the publisher.
The Supplementary Material for this article can be found online at: https://www.frontiersin.org/articles/10.3389/fphar.2022.1014173/full#supplementary-material
AKT, v-akt murine thymoma viral oncogene homolog 1; ALP, alkaline phosphatase; BMMs, bone marrow monocytes cells; CTX-1, type I collagen C-terminal peptide; ERK, extracellular signal-regulated kinases; JNK, c-Jun NH2-terminal kinase; IL-1α, interleukin-1α; IL-1β, interleukin-1β; IL-6, interleukin-6; LPS, lipopolysaccharide; MAPK, mitogen-activated protein kinases; M-CSF, macrophage colony-stimulating factor; MITF, microphthalmia-associated transcription factor; MSCs, marrow mesenchymal stem cells; NFATc1, nuclear factor of activated T cells c1; NFκB, nuclear factor kappa B; OPG, osteoprotegerin; OVX, ovariectomized; RANK, receptor activator of nuclear factor kappa B; RANKL, receptor activator of nuclear factor kappa B ligand; Runx2, runt-related transcription factor 2; TRACP5b, tartrate-resistant acid phosphatase 5b; TNF, tumor necrosis factor; TRAF6, tumor necrosis factor receptor associated factor 6; TRAP, tartrate-resistant acid phosphatase; Wnt, wingless and int-1.
Adami, G., and Saag, K. G. (2019). Osteoporosis pathophysiology, epidemiology, and screening in rheumatoid arthritis. Curr. Rheumatol. Rep. 21, 34. doi:10.1007/s11926-019-0836-7
Adil, M., Mansoori, M. N., Singh, D., Kandhare, A. D., and Sharma, M. (2017). Pioglitazone-induced bone loss in diabetic rats and its amelioration by berberine: A portrait of molecular crosstalk. Biomed. Pharmacother. 94, 1010–1019. doi:10.1016/j.biopha.2017.08.001
Ajebli, M., Khan, H., and Eddouks, M. (2021). Natural alkaloids and diabetes mellitus: A review. Endocr. Metab. Immune Disord. Drug Targets 21, 111–130. doi:10.2174/1871530320666200821124817
An, J., Yang, H., Zhang, Q., Liu, C., Zhao, J., Zhang, L., et al. (2016). Natural products for treatment of osteoporosis: The effects and mechanisms on promoting osteoblast-mediated bone formation. Life Sci. 147, 46–58. doi:10.1016/j.lfs.2016.01.024
Asokan, S. M., Mariappan, R., Muthusamy, S., and Velmurugan, B. K. (2018). Pharmacological benefits of neferine - a comprehensive review. Life Sci. 199, 60–70. doi:10.1016/j.lfs.2018.02.032
Badaoui, M. I., Alabdul Magid, A., Benkhaled, M., Bensouici, C., Harakat, D., Voutquenne-Nazabadioko, L., et al. (2021). Pyrroloquinolone a, a new alkaloid and other phytochemicals from Atractylis cancellata L. with antioxidant and anticholinesterase activities. Nat. Prod. Res. 35, 2997–3003. doi:10.1080/14786419.2019.1682575
Bailly, C. (2019). Cepharanthine: An update of its mode of action, pharmacological properties and medical applications. Phytomedicine 62, 152956. doi:10.1016/j.phymed.2019.152956
Bailly, C. (2021). The steroidal alkaloids alpha-tomatine and tomatidine: Panorama of their mode of action and pharmacological properties. Steroids 176, 108933. doi:10.1016/j.steroids.2021.108933
Black, D. M., and Rosen, C. J. (2016). Clinical practice. Postmenopausal osteoporosis. N. Engl. J. Med. 374, 254–262. doi:10.1056/NEJMcp1513724
Brugnerotto, P., Seraglio, S. K. T., Schulz, M., Gonzaga, L. V., Fett, R., and Costa, A. C. O. (2021). Pyrrolizidine alkaloids and beehive products: A review. Food Chem. 342, 128384. doi:10.1016/j.foodchem.2020.128384
Chen, K., Lv, Z., Cheng, P., Zhu, W., Liang, S., Yang, Q., et al. (2018). Boldine ameliorates estrogen deficiency-induced bone loss via inhibiting bone resorption. Front. Pharmacol. 9, 1046. doi:10.3389/fphar.2018.01046
Chen, K., Qiu, P., Yuan, Y., Zheng, L., He, J., Wang, C., et al. (2019). Pseurotin a inhibits osteoclastogenesis and prevents ovariectomized-induced bone loss by suppressing reactive oxygen species. Theranostics 9, 1634–1650. doi:10.7150/thno.30206
Chen, M., Yang, D., Hu, X., Jiang, G., Li, T., Ouyang, Z., et al. (2021a). Stachydrine hydrochloride inhibits osteoclastogenesis by regulating the NF-kappa B and p38 signaling pathways to alleviate postmenopausal osteoporosis. Biochem. Biophys. Res. Commun. 542, 1–8. doi:10.1016/j.bbrc.2021.01.012
Chen, Q. C., Pu, Y. L., Bi, J., and Zhang, Y. (2021b). Protective effects of berberine on senile osteoporosis in mice. J. Bone Min. Metab. 39, 748–756. doi:10.1007/s00774-021-01225-2
Chen, Q., Shou, P., Zheng, C., Jiang, M., Cao, G., Yang, Q., et al. (2016). Fate decision of mesenchymal stem cells: Adipocytes or osteoblasts? Cell Death Differ. 23, 1128–1139. doi:10.1038/cdd.2015.168
Chen, S., Jin, G., Huang, K. M., Ma, J. J., Wang, Q., Ma, Y., et al. (2015). Lycorine suppresses RANKL-induced osteoclastogenesis in vitro and prevents ovariectomy-induced osteoporosis and titanium particle-induced osteolysis in vivo. Sci. Rep. 5, 12853. doi:10.1038/srep12853
Chen, X., Zhi, X., Pan, P., Cui, J., Cao, L., Weng, W., et al. (2017). Matrine prevents bone loss in ovariectomized mice by inhibiting RANKL-induced osteoclastogenesis. Faseb J. 31, 4855–4865. doi:10.1096/fj.201700316R
Cheng, F., Zhou, Y., Wang, M., Guo, C., Cao, Z., Zhang, R., et al. (2020). A review of pharmacological and pharmacokinetic properties of stachydrine. Pharmacol. Res. 155, 104755. doi:10.1016/j.phrs.2020.104755
Choi, H. S., Kim, H. S., Min, K. R., Kim, Y., Lim, H. K., Chang, Y. K., et al. (2000). Anti-inflammatory effects of fangchinoline and tetrandrine. J. Ethnopharmacol. 69, 173–179. doi:10.1016/s0378-8741(99)00141-5
Coury, F., Peyruchaud, O., and Machuca-Gayet, I. (2019). Osteoimmunology of bone loss in inflammatory rheumatic diseases. Front. Immunol. 10, 679. doi:10.3389/fimmu.2019.00679
De Martinis, M., Di Benedetto, M. C., Mengoli, L. P., and Ginaldi, L. (2006). Senile osteoporosis: Is it an immune-mediated disease? Inflamm. Res. 55, 399–404. doi:10.1007/s00011-006-6034-x
Deepak, V., Kruger, M. C., Joubert, A., and Coetzee, M. (2015). Piperine alleviates osteoclast formation through the p38/c-Fos/NFATc1 signaling axis. Biofactors 41, 403–413. doi:10.1002/biof.1241
Dey, P., Kundu, A., Chakraborty, H. J., Kar, B., Choi, W. S., Lee, B. M., et al. (2019). Therapeutic value of steroidal alkaloids in cancer: Current trends and future perspectives. Int. J. Cancer 145, 1731–1744. doi:10.1002/ijc.31965
Epsley, S., Tadros, S., Farid, A., Kargilis, D., Mehta, S., and Rajapakse, C. S. (2021). The effect of inflammation on bone. Front. Physiol. 11, 511799. doi:10.3389/fphys.2020.511799
Fan, Q., and Wang, J. (2020). The efficacy and safety of bisphosphonates for osteoporosis in women older than 65 years: A meta-analysis. Curr. Pharm. Des. 26, 4022–4030. doi:10.2174/1381612826666200423092602
Fan, T., Yang, Y., Zeng, Q., Wang, X., Wei, W., Guo, X., et al. (2021). Structure-activity relationship and biological evaluation of berberine derivatives as PCSK9 down-regulating agents. Bioorg. Chem. 113, 104994. doi:10.1016/j.bioorg.2021.104994
Fischer, V., Kalbitz, M., Mueller-Graf, F., Gebhard, F., Ignatius, A., Liedert, A., et al. (2018). Influence of menopause on inflammatory cytokines during murine and human bone fracture healing. Int. J. Mol. Sci. 19, 2070. doi:10.3390/ijms19072070
Green, B. T., Lee, S. T., Panter, K. E., and Brown, D. R. (2012). Piperidine alkaloids: Human and food animal teratogens. Food Chem. Toxicol. 50, 2049–2055. doi:10.1016/j.fct.2012.03.049
Gu, W., Wang, Z., Sun, Z., Bao, Z., Zhang, L., Geng, Y., et al. (2020). Role of NFATc1 in the bone-vascular axis calcification paradox. J. Cardiovasc. Pharmacol. 75, 200–207. doi:10.1097/fjc.0000000000000788
Han, L., Wang, B., Wang, R., Gong, S., Chen, G., and Xu, W. (2019). The shift in the balance between osteoblastogenesis and adipogenesis of mesenchymal stem cells mediated by glucocorticoid receptor. Stem Cell Res. Ther. 10, 377. doi:10.1186/s13287-019-1498-0
Haq, I. U., Imran, M., Nadeem, M., Tufail, T., Gondal, T. A., and Mubarak, M. S. (2021). Piperine: A review of its biological effects. Phytother. Res. 35, 680–700. doi:10.1002/ptr.6855
He, L. G., Duan, H., Li, X. L., Wang, S., Zhang, Y. Y., Lei, L. S., et al. (2016). Sinomenine down-regulates TLR4/TRAF6 expression and attenuates lipopolysaccharide-induced osteoclastogenesis and osteolysis. Eur. J. Pharmacol. 779, 66–79. doi:10.1016/j.ejphar.2016.03.014
He, X. F., Zhang, L., Zhang, C. H., Zhao, C. R., Li, H., Zhang, L. F., et al. (2017). Berberine alleviates oxidative stress in rats with osteoporosis through receptor activator of NF-kB/receptor activator of NF-kB ligand/osteoprotegerin (RANK/RANKL/OPG) pathway. Bosn. J. Basic Med. Sci. 17, 295–301. doi:10.17305/bjbms.2017.2596
Henriksen, K., Karsdal, M. A., and Martin, T. J. (2014). Osteoclast-derived coupling factors in bone remodeling. Calcif. Tissue Int. 94, 88–97. doi:10.1007/s00223-013-9741-7
Hojo, H., and Ohba, S. (2020). Gene regulatory landscape in osteoblast differentiation. Bone 137, 115458. doi:10.1016/j.bone.2020.115458
Hu, B., Sun, X., Yang, Y., Ying, Z., Meng, J., Zhou, C., et al. (2019). Tomatidine suppresses osteoclastogenesis and mitigates estrogen deficiency-induced bone mass loss by modulating TRAF6-mediated signaling. Faseb J. 33, 2574–2586. doi:10.1096/fj.201800920R
Hu, J. P., Nishishita, K., Sakai, E., Yoshida, H., Kato, Y., Tsukuba, T., et al. (2008). Berberine inhibits RANKL-induced osteoclast formation and survival through suppressing the NF-kappaB and Akt pathways. Eur. J. Pharmacol. 580, 70–79. doi:10.1016/j.ejphar.2007.11.013
Hu, R., Chen, L., Chen, X., Xie, Z., Xia, C., and Chen, Y. (2021). Aloperine improves osteoporosis in ovariectomized mice by inhibiting RANKL-induced NF-kappa B, ERK and JNK approaches. Int. Immunopharmacol. 97, 107720. doi:10.1016/j.intimp.2021.107720
Hu, X., Li, D., Chu, C., Li, X., Wang, X., Jia, Y., et al. (2018). Antiproliferative effects of alkaloid evodiamine and its derivatives. Int. J. Mol. Sci. 19, 3403. doi:10.3390/ijms19113403
Hu, Y., Yang, H., Ding, X., Liu, J., Wang, X., Hu, L., et al. (2020). Anti-inflammatory octahydroindolizine alkaloid enantiomers from Dendrobium crepidatum. Bioorg. Chem. 100, 103809. doi:10.1016/j.bioorg.2020.103809
Huang, J., Li, Y. Y., Xia, K., Wang, Y. Y., Chen, C. Y., Chen, M. L., et al. (2021). Harmine targets inhibitor of DNA binding-2 and activator protein-1 to promote preosteoclast PDGF-BB production. J. Cell. Mol. Med. 25, 5525–5533. doi:10.1111/jcmm.16562
Huang, J., Yin, H., Rao, S. S., Xie, P. L., Cao, X., Rao, T., et al. (2018). Harmine enhances type H vessel formation and prevents bone loss in ovariectomized mice. Theranostics 8, 2435–2446. doi:10.7150/thno.22144
Ikeda, K., and Takeshita, S. (2016). The role of osteoclast differentiation and function in skeletal homeostasis. J. Biochem. 159, 1–8. doi:10.1093/jb/mvv112
Jan, N. U., Ali, A., Ahmad, B., Iqbal, N., Adhikari, A., Inayat Ur, R., et al. (2018). Evaluation of antidiabetic potential of steroidal alkaloid of Sarcococca saligna. Biomed. Pharmacother. 100, 461–466. doi:10.1016/j.biopha.2018.01.008
Jiang, C., Ma, Q., Wang, S., Shen, Y., Qin, A., Fan, S., et al. (2021). Oxymatrine attenuates osteoclastogenesis via modulation of ROS-mediated SREBP2 signaling and counteracts ovariectomy-induced osteoporosis. Front. Cell Dev. Biol. 9, 684007. doi:10.3389/fcell.2021.684007
Jiang, Q., Chen, M., Cheng, K., Yu, P., Wei, X., and Shi, Z. (2016). Therapeutic potential of steroidal alkaloids in cancer and other diseases. Med. Res. Rev. 36, 119–143. doi:10.1002/med.21346
Jiang, Y., Sang, W., Wang, C., Lu, H., Zhang, T., Wang, Z., et al. (2018). Oxymatrine exerts protective effects on osteoarthritis via modulating chondrocyte homoeostasis and suppressing osteoclastogenesis. J. Cell. Mol. Med. 22, 3941–3954. doi:10.1111/jcmm.13674
Kahwati, L. C., Weber, R. P., Pan, H. L., Gourlay, M., Leblanc, E., Coker-Schwimmer, M., et al. (2018). Vitamin D, calcium, or combined supplementation for the primary prevention of fractures in community-dwelling adults evidence report and systematic review for the US preventive services task force. Jama-j. Am. Med. Assoc. 319, 1600–1612. doi:10.1001/jama.2017.21640
Kalkan, R., and Tosun, O. (2020). Do we use methylation of NFATC1 and FOS genes as a biomarker for postmenopausal osteoporosis? Balk. J. Med. Genet. 23, 35–40. doi:10.2478/bjmg-2020-0021
Kang, J. Y., Kang, N., Yang, Y. M., Hong, J. H., and Shin, D. M. (2020a). The role of Ca2+-NFATc1 signaling and its modulation on osteoclastogenesis. Int. J. Mol. Sci. 21, 3646. doi:10.3390/ijms21103646
Kang, M. A., Lee, J., and Park, S. H. (2020b). Cannabidiol induces osteoblast differentiation via angiopoietin1 and p38 MAPK. Environ. Toxicol. 35, 1318–1325. doi:10.1002/tox.22996
Kenkre, J. S., and Bassett, J. H. D. (2018). The bone remodelling cycle. Ann. Clin. Biochem. 55, 308–327. doi:10.1177/0004563218759371
Khan, H., Ben Hadda, T., and Touzani, R. (2018). Diverse therapeutic potential of nitidine, a comprehensive review. Curr. Drug Metab. 19, 986–991. doi:10.2174/1389200219666180628165643
Kim, J. M., Lin, C., Stavre, Z., Greenblatt, M. B., and Shim, J.-H. (2020). Osteoblast-osteoclast communication and bone homeostasis. Cells 9, 2073. doi:10.3390/cells9092073
Komori, T. (2018). Runx2, an inducer of osteoblast and chondrocyte differentiation. Histochem. Cell Biol. 149, 313–323. doi:10.1007/s00418-018-1640-6
Langdahl, B. L. (2021). Overview of treatment approaches to osteoporosis. Br. J. Pharmacol. 178, 1891–1906. doi:10.1111/bph.15024
Lee, H. W., Suh, J. H., Kim, H.-N., Kim, A. Y., Park, S. Y., Shin, C. S., et al. (2008). Berberine promotes osteoblast differentiation by Runx2 activation with p38 MAPK. J. Bone Min. Res. 23, 1227–1237. doi:10.1359/jbmr.080325
Li, C. L., Tan, L. H., Wang, Y. F., Luo, C. D., Chen, H. B., Lu, Q., et al. (2019). Comparison of anti-inflammatory effects of berberine, and its natural oxidative and reduced derivatives from Rhizoma Coptidis in vitro and in vivo. Phytomedicine 52, 272–283. doi:10.1016/j.phymed.2018.09.228
Li, X., He, L., Hu, Y., Duan, H., Li, X., Tan, S., et al. (2013). Sinomenine suppresses osteoclast formation and mycobacterium tuberculosis H37Ra-induced bone loss by modulating RANKL signaling pathways. Plos One 8, e74274. doi:10.1371/journal.pone.0074274
Li, X., Xu, J., Dai, B., Wang, X., Guo, Q., and Qin, L. (2020a). Targeting autophagy in osteoporosis: From pathophysiology to potential therapy. Ageing Res. Rev. 62, 101098. doi:10.1016/j.arr.2020.101098
Li, Y., Feng, Y. F., Liu, X. T., Li, Y. C., Zhu, H. M., Sun, M. R., et al. (2021a). Songorine promotes cardiac mitochondrial biogenesis via Nrf2 induction during sepsis. Redox Biol. 38, 101771. doi:10.1016/j.redox.2020.101771
Li, Y., Han, X., Wang, W., Jiang, X., Han, J., Zhang, J., et al. (2021b). Rutaecarpine exerted anti-osteroporosis. Acta Pharm. Sin. 56, 511–519. doi:10.16438/j.0513-4870.2020-1616
Li, Y., Wang, G., Liu, J., and Ouyang, L. (2020b). Quinolizidine alkaloids derivatives from Sophora alopecuroides linn: Bioactivities, structure-activity relationships and preliminary molecular mechanisms. Eur. J. Med. Chem. 188, 111972. doi:10.1016/j.ejmech.2019.111972
Liao, S., Feng, W., Liu, Y., Wang, Z., Ding, X., Song, F., et al. (2021). Inhibitory effects of biochanin A on titanium particle-induced osteoclast activation and inflammatory bone resorption via NF-kappa B and MAPK pathways. J. Cell. Physiol. 236, 1432–1444. doi:10.1002/jcp.29948
Lin, K. M., Lu, C. L., Hung, K. C., Wu, P. C., Pan, C. F., Wu, C. J., et al. (2019). The paradoxical role of uric acid in osteoporosis. Nutrients 11, 2111. doi:10.3390/nu11092111
Liu, F. L., Chen, C. L., Lai, C. C., Lee, C. C., and Chang, D. M. (2020a). Arecoline suppresses RANKL-induced osteoclast differentiation in vitro and attenuates LPS-induced bone loss in vivo. Phytomedicine 69, 153195. doi:10.1016/j.phymed.2020.153195
Liu, Q., Wang, T., Zhou, L., Song, F., Qin, A., Feng, H. T., et al. (2016). Nitidine chloride prevents OVX-induced bone loss via suppressing NFATc1-mediated osteoclast differentiation. Sci. Rep. 6, 36662. doi:10.1038/srep36662
Liu, Z., Li, Y., Guo, F., Zhang, C., Song, G., Yang, J., et al. (2020b). Tetrandrine inhibits titanium particle-induced inflammatory osteolysis through the nuclear factor-kappa B pathway. Mediat. Inflamm. 2020, 1926947. doi:10.1155/2020/1926947
Locantore, P., Del Gatto, V., Gelli, S., Paragliola, R. M., and Pontecorvi, A. (2020). The interplay between Immune system and microbiota in osteoporosis. Mediat. Inflamm. 2020, 3686749. doi:10.1155/2020/3686749
Lorenzo, J. (2017). The many ways of osteoclast activation. J. Clin. Invest. 127, 2530–2532. doi:10.1172/jci94606
Luan, F., He, X., and Zeng, N. (2020). Tetrandrine: A review of its anticancer potentials, clinical settings, pharmacokinetics and drug delivery systems. J. Pharm. Pharmacol. 72, 1491–1512. doi:10.1111/jphp.13339
Ma, H., Wang, Y., Guo, T., He, Z., Chang, X., and Pu, X. (2009). Simultaneous determination of tetrahydropalmatine, protopine, and palmatine in rat plasma by LC-ESI-MS and its application to a pharmacokinetic study. J. Pharm. Biomed. Anal. 49, 440–446. doi:10.1016/j.jpba.2008.11.019
Martiniakova, M., Babikova, M., and Omelka, R. (2020). Pharmacological agents and natural compounds: Available treatments for osteoporosis. J. Physiol. Pharmacol. 71, 307–320. doi:10.26402/jpp.2020.3.01
Matsuura, H. N., Rau, M. R., and Fett-Neto, A. G. (2014). Oxidative stress and production of bioactive monoterpene indole alkaloids: Biotechnological implications. Biotechnol. Lett. 36, 191–200. doi:10.1007/s10529-013-1348-6
Meng, J., Zhou, C., Zhang, W., Wang, W., He, B., Hu, B., et al. (2019). Stachydrine prevents LPS-induced bone loss by inhibiting osteoclastogenesis via NF-kappa B and Akt signalling. J. Cell. Mol. Med. 23, 6730–6743. doi:10.1111/jcmm.14551
Merarchi, M., Sethi, G., Fan, L., Mishra, S., Arfuso, F., and Ahn, K. S. (2018). Molecular targets modulated by fangchinoline in tumor cells and preclinical models. Molecules 23, 2538. doi:10.3390/molecules23102538
Mizutani, K., Isono, K., Matsushima, Y., Okada, K., Umaoka, A., Iida, S., et al. (2020). Inflammatory skin-derived cytokines accelerate osteoporosis in mice with persistent skin inflammation. Int. J. Mol. Sci. 21, 3620. doi:10.3390/ijms21103620
Nagy, V., and Penninger, J. M. (2015). The RANKL-RANK story. Gerontology 61, 534–542. doi:10.1159/000371845
O'brien, P., Carrasco-Pozo, C., and Speisky, H. (2006). Boldine and its antioxidant or health-promoting properties. Chem. Biol. Interact. 159, 1–17. doi:10.1016/j.cbi.2005.09.002
Oh, S. Y., Kang, N., Kang, J. Y., Kim, K. W., Choi, J. H., Yang, Y. M., et al. (2021). Sestrin2 regulates osteoclastogenesis via the p62-TRAF6 interaction. Front. Cell Dev. Biol. 9, 646803. doi:10.3389/fcell.2021.646803
Ono, T., Hayashi, M., Sasaki, F., and Nakashima, T. (2020). RANKL biology: Bone metabolism, the immune system, and beyond. Inflamm. Regen. 40, 2. doi:10.1186/s41232-019-0111-3
Park, J. H., Lee, N. K., and Lee, S. Y. (2017). Current understanding of RANK signaling in osteoclast differentiation and maturation. Mol. Cells 40, 706–713. doi:10.14348/molcells.2017.0225
Qian, Z., Zhong, Z., Ni, S., Li, D., Zhang, F., Zhou, Y., et al. (2020). Cytisine attenuates bone loss of ovariectomy mouse by preventing RANKL-induced osteoclastogenesis. J. Cell. Mol. Med. 24, 10112–10127. doi:10.1111/jcmm.15622
Rasouli, H., Yarani, R., Pociot, F., and Popovic-Djordjevic, J. (2020). Anti-diabetic potential of plant alkaloids: Revisiting current findings and future perspectives. Pharmacol. Res. 155, 104723. doi:10.1016/j.phrs.2020.104723
Ratajczak, A. E., Rychter, A. M., Zawada, A., Dobrowolska, A., and Krela-Kazmierczak, I. (2020). Nutrients in the prevention of osteoporosis in patients with inflammatory bowel diseases. Nutrients 12, 1702. doi:10.3390/nu12061702
Robertson, J., and Stevens, K. (2014). Pyrrolizidine alkaloids. Nat. Prod. Rep. 31, 1721–1788. doi:10.1039/c4np00055b
Roy, M., Liang, L., Xiao, X., Feng, P., Ye, M., and Liu, J. (2018). Lycorine: A prospective natural lead for anticancer drug discovery. Biomed. Pharmacother. 107, 615–624. doi:10.1016/j.biopha.2018.07.147
Saunders, K. H., Umashanker, D., Igel, L. I., Kumar, R. B., and Aronne, L. J. (2018). Obesity pharmacotherapy. Med. Clin. North Am. 102, 135–148. doi:10.1016/j.mcna.2017.08.010
Seeman, E., and Martin, T. J. (2019). Antiresorptive and anabolic agents in the prevention and reversal of bone fragility. Nat. Rev. Rheumatol. 15, 225–236. doi:10.1038/s41584-019-0172-3
Shang, X. F., Yang, C. J., Morrisnatschke, S. L., Li, J. C., Yin, X. D., Liu, Y. Q., et al. (2020). Biologically active isoquinoline alkaloids covering 2014-2018. Med. Res. Rev. 40, 2212–2289. doi:10.1002/med.21703
Shi, J.-H., and Sun, S.-C. (2018). Tumor necrosis factor receptor-associated factor regulation of nuclear factor kappa B and mitogen-activated protein kinase pathways. Front. Immunol. 9, 1849. doi:10.3389/fimmu.2018.01849
Song, S., Guo, Y., Yang, Y., and Fu, D. (2022). Advances in pathogenesis and therapeutic strategies for osteoporosis. Pharmacol. Ther. 237, 108168. doi:10.1016/j.pharmthera.2022.108168
Stuerznickel, J., Rolvien, T., Delsmann, A., Butscheidt, S., Barvencik, F., Mundlos, S., et al. (2021). Clinical phenotype and relevance of LRP5 and LRP6 variants in patients with early-onset osteoporosis (EOOP). J. Bone Min. Res. 36, 271–282. doi:10.1002/jbmr.4197
Takahashi, T., Tonami, Y., Tachibana, M., Nomura, M., Shimada, T., Aburada, M., et al. (2012). Tetrandrine prevents bone loss in sciatic-neurectomized mice and inhibits receptor activator of nuclear factor kappa B ligand-induced osteoclast differentiation. Biol. Pharm. Bull. 35, 1765–1774. doi:10.1248/bpb.b12-00445
Takayanagi, H. (2005). Mechanistic insight into osteoclast differentiation in osteoimmunology. J. Mol. Med. 83, 170–179. doi:10.1007/s00109-004-0612-6
Tao, K., Xiao, D., Weng, J., Xiong, A., Kang, B., and Zeng, H. (2016). Berberine promotes bone marrow-derived mesenchymal stem cells osteogenic differentiation via canonical Wnt/β-catenin signaling pathway. Toxicol. Lett. 240, 68–80. doi:10.1016/j.toxlet.2015.10.007
Taylan, A., Birlik, M., Kenar, G., Toprak, B., Gundogdu, B., Gurler, O., et al. (2019). Osteoprotegrin interacts with biomarkers and cytokines that have roles in osteoporosis, skin fibrosis, and vasculopathy in systemic sclerosis: A potential multifaceted relationship between OPG/RANKL/TRAIL and Wnt inhibitors. Mod. Rheumatol. 29, 619–624. doi:10.1080/14397595.2018.1500736
Tian, K.-M., Li, J.-J., and Xu, S.-W. (2019). Rutaecarpine: A promising cardiovascular protective alkaloid from evodia rutaecarpa (Wu Zhu yu). Pharmacol. Res. 141, 541–550. doi:10.1016/j.phrs.2018.12.019
Tsukasaki, M., Hamada, K., Okamoto, K., Nagashima, K., Terashima, A., Komatsu, N., et al. (2017). LOX fails to substitute for RANKL in osteoclastogenesis. J. Bone Min. Res. 32, 434–439. doi:10.1002/jbmr.2990
Volgin, A. D., Bashirzade, A., Arnstislavskaya, T. G., Yakovlev, O. A., Demin, K. A., Ho, Y.-J., et al. (2019). DARK classics in chemical neuroscience: Arecoline. Acs Chem. Neurosci. 10, 2176–2185. doi:10.1021/acschemneuro.8b00711
Walsh, M. C., Lee, J., and Choi, Y. (2015). Tumor necrosis factor receptor- associated factor 6 (TRAF6) regulation of development, function, and homeostasis of the immune system. Immunol. Rev. 266, 72–92. doi:10.1111/imr.12302
Wang, H., Xia, C., Chen, L., Zhao, J., Tao, W., Zhang, X., et al. (2019). Phytochemical information and biological activities of quinolizidine alkaloids in sophora: A comprehensive review. Curr. Drug Targets 20, 1572–1586. doi:10.2174/1389450120666190618125816
Wong, S. K., Chin, K. Y., and Ima-Nirwana, S. (2019). The osteoprotective effects of kaempferol: The evidence from in vivo and in vitro studies. Drug Des. devel. Ther. 13, 3497–3514. doi:10.2147/dddt.S227738
Wu, F., Huang, Y., Hu, J., and Shao, Z. (2020). Mendelian randomization study of inflammatory bowel disease and bone mineral density. BMC Med. 18, 312. doi:10.1186/s12916-020-01778-5
Xie, C. L., Park, K. H., Kang, S. S., Cho, K. M., and Lee, D. H. (2021). Isoflavone-enriched soybean leaves attenuate ovariectomy-induced osteoporosis in rats by anti-inflammatory activity. J. Sci. Food Agric. 101, 1499–1506. doi:10.1002/jsfa.10763
Xie, H., Wang, Q., Zhang, X., Wang, T., Hu, W., Manicum, T., et al. (2018). Possible therapeutic potential of berberine in the treatment of STZ plus HFD-induced diabetic osteoporosis. Biomed. Pharmacother. 108, 280–287. doi:10.1016/j.biopha.2018.08.131
Xu, D., Yang, W., Zhou, C., Liu, Y., and Xu, B. (2010). Preventive effects of berberine on glucocorticoid-induced osteoporosis in rats. Planta Med. 76, 1809–1813. doi:10.1055/s-0030-1250040
Xu, S., Li, S., Liu, X., Tan, K., Zhang, J., Li, K., et al. (2021). Rictor is a novel regulator of TRAF6/TRAF3 in osteoclasts. J. Bone Min. Res. 36, 2053–2064. doi:10.1002/jbmr.4398
Yang, Y., Chung, M. R., Zhou, S., Gong, X., Xu, H., Hong, Y., et al. (2019). STAT3 controls osteoclast differentiation and bone homeostasis by regulating NFATc1 transcription. J. Biol. Chem. 294, 15395–15407. doi:10.1074/jbc.RA119.010139
Yasuda, H. (2021). Discovery of the RANKL/RANK/OPG system. J. Bone Min. Metab. 39, 2–11. doi:10.1007/s00774-020-01175-1
Yonezawa, T., Hasegawa, S., Asai, M., Ninomiya, T., Sasaki, T., Cha, B. Y., et al. (2011). Harmine, a beta-carboline alkaloid, inhibits osteoclast differentiation and bone resorption in vitro and in vivo. Eur. J. Pharmacol. 650, 511–518. doi:10.1016/j.ejphar.2010.10.048
Yun, J., Lee, K. Y., and Park, B. (2019). Neotuberostemonine inhibits osteoclastogenesis via blockade of NF-kappa B pathway. Biochimie 157, 81–91. doi:10.1016/j.biochi.2018.11.008
Zeng, X., Zhang, Y., Yang, Q., Wang, S., Zou, B., Tan, Y., et al. (2020). Artesunate attenuates LPS-induced osteoclastogenesis by suppressing TLR4/TRAF6 and PLC gamma 1-Ca2+-NFATc1 signaling pathway. Acta Pharmacol. Sin. 41, 229–236. doi:10.1038/s41401-019-0289-6
Zhan, Y., Liang, J., Tian, K., Che, Z., Wang, Z., Yang, X., et al. (2020). Vindoline inhibits RANKL-induced osteoclastogenesis and prevents ovariectomy-induced bone loss in mice. Front. Pharmacol. 10, 1587. doi:10.3389/fphar.2019.01587
Zhang, B., Zhang, H., Luo, H., Yang, C., and Yuan, Y. (2019a). Sinomenine can promote the proliferation and differentiation of osteoblasts by regulating the Akt/Runx2 signaling pathway in MC3T3-E1 cells. Pharmazie 74, 747–750. doi:10.1691/ph.2019.9636
Zhang, L., Li, D., and Yu, S. (2020a). Pharmacological effects of harmine and its derivatives: A review. Arch. Pharm. Res. 43, 1259–1275. doi:10.1007/s12272-020-01283-6
Zhang, L., Li, Y. Y., Wei, F., Liu, H., Wang, Y. S., Zhao, W. M., et al. (2021). Transdermal delivery of salmon calcitonin using a dissolving microneedle array: Characterization, stability, and in vivo pharmacodynamics. Aaps Pharmscitech 22, 1. doi:10.1208/s12249-020-01865-z
Zhang, N., Zhang, Z. K., Yu, Y. Y., Zhuo, Z. J., Zhang, G., and Zhang, B. T. (2020b). Pros and cons of denosumab treatment for osteoporosis and implication for RANKL aptamer therapy. Front. Cell Dev. Biol. 8, 325. doi:10.3389/fcell.2020.00325
Zhang, Y., Zou, B., Tan, Y., Su, J., Wang, Y., Xu, J., et al. (2019b). Sinomenine inhibits osteolysis in breast cancer by reducing IL-8/CXCR1 and c-Fos/NFATc1 signaling. Pharmacol. Res. 142, 140–150. doi:10.1016/j.phrs.2019.02.015
Zhao, X., Lin, S., Li, H., Si, S., and Wang, Z. (2021). Myeloperoxidase controls bone turnover by suppressing osteoclast differentiation through modulating reactive oxygen species level. J. Bone Min. Res. 36, 591–603. doi:10.1002/jbmr.4215
Zhi, X., Wang, L., Chen, H., Fang, C., Cui, J., Hu, Y., et al. (2020). L-tetrahydropalmatine suppresses osteoclastogenesis in vivo and in vitro via blocking RANK-TRAF6 interactions and inhibiting NF-kappa B and MAPK pathways. J. Cell. Mol. Med. 24, 785–798. doi:10.1111/jcmm.14790
Zhong, Z., Qian, Z., Zhang, X., Chen, F., Ni, S., Kang, Z., et al. (2020). Tetrandrine prevents bone loss in ovariectomized mice by inhibiting RANKL-induced osteoclastogenesis. Front. Pharmacol. 10, 1530. doi:10.3389/fphar.2019.01530
Zhou, B., Lu, X., Tang, Z., Liu, D., Zhou, Y., Zeng, P., et al. (2017). Influence of sinomenine upon mesenchymal stem cells in osteoclastogenesis. Biomed. Pharmacother. 90, 835–841. doi:10.1016/j.biopha.2017.03.084
Zhou, C., Meng, J., Yang, Y., Hu, B., Hong, J., Lv, Z., et al. (2018). Cepharanthine prevents estrogen deficiency-induced bone loss by inhibiting bone resorption. Front. Pharmacol. 9, 210. doi:10.3389/fphar.2018.00210
Zhou, H., Li, J., Sun, F., Wang, F., Li, M., Dong, Y., et al. (2020a). A review on recent advances in aloperine research: Pharmacological activities and underlying biological mechanisms. Front. Pharmacol. 11, 538137. doi:10.3389/fphar.2020.538137
Zhou, L., Hong, G., Li, S., Liu, Q., Song, F., Zhao, J., et al. (2020b). Fangchinoline protects against bone loss in OVX mice via inhibiting osteoclast formation, bone resorption and RANKL-induced signaling. Int. J. Biol. Sci. 16, 309–319. doi:10.7150/ijbs.37162
Zhu, W., Ding, W., Shang, X., Zhu, D., and Dai, X. (2019). Fangchinoline promotes autophagy and inhibits apoptosis in osteoporotic rats. Med. Sci. Monit. 25, 324–332. doi:10.12659/msm.912624
Ziegler, J., and Facchini, P. J. (2008). Alkaloid biosynthesis: Metabolism and trafficking. Annu. Rev. Plant Biol. 59, 735–769. doi:10.1146/annurev.arplant.59.032607.092730
Keywords: alkaloids, osteoporosis, osteoblasts, osteoclasts, NFκB, MAPK
Citation: Lin B, Xu P, Zheng J, Deng X, Ye Q, Huang Z and Wang N (2022) Effects and mechanisms of natural alkaloids for prevention and treatment of osteoporosis. Front. Pharmacol. 13:1014173. doi: 10.3389/fphar.2022.1014173
Received: 08 August 2022; Accepted: 31 August 2022;
Published: 23 September 2022.
Edited by:
Longhuo Wu, Gannan Medical University, ChinaReviewed by:
Ting Zheng, Hospital for Special Surgery, United StatesCopyright © 2022 Lin, Xu, Zheng, Deng, Ye, Huang and Wang. This is an open-access article distributed under the terms of the Creative Commons Attribution License (CC BY). The use, distribution or reproduction in other forums is permitted, provided the original author(s) and the copyright owner(s) are credited and that the original publication in this journal is cited, in accordance with accepted academic practice. No use, distribution or reproduction is permitted which does not comply with these terms.
*Correspondence: Nani Wang, d25uODUxMUAxNjMuY29t
Disclaimer: All claims expressed in this article are solely those of the authors and do not necessarily represent those of their affiliated organizations, or those of the publisher, the editors and the reviewers. Any product that may be evaluated in this article or claim that may be made by its manufacturer is not guaranteed or endorsed by the publisher.
Research integrity at Frontiers
Learn more about the work of our research integrity team to safeguard the quality of each article we publish.