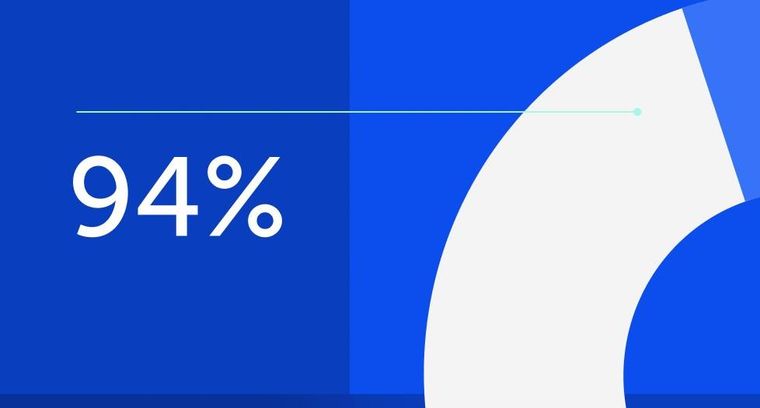
94% of researchers rate our articles as excellent or good
Learn more about the work of our research integrity team to safeguard the quality of each article we publish.
Find out more
ORIGINAL RESEARCH article
Front. Pharmacol., 06 October 2022
Sec. Ethnopharmacology
Volume 13 - 2022 | https://doi.org/10.3389/fphar.2022.1014160
This article is part of the Research TopicThe Application of Network Analysis in EthnopharmacologyView all 13 articles
5-fluorouracil (5-FU) is one of the most widely used chemotherapy drugs for malignant tumors. However, intestinal mucositis caused by 5-FU is a severe dose-limiting toxic effect and even leads to treatment interruption. Isoliquiritigenin (ISL) is one of the main active compounds of licorice, which is a traditional Chinese herbal medicine commonly used in inflammation and gastrointestinal diseases. It is speculated that ISL have protective effects on intestinal mucositis. However, no such studies have been reported. Therefore, to investigate the impact of ISL on 5-Fu-induced intestinal mucositis, a strategy based on network prediction and pharmacological experimental validation was proposed in this study. Firstly, the targets and mechanism of ISL in alleviating 5-Fu-induced gastrointestinal toxicity were predicted by network analysis. And the results were further confirmed by molecular docking. Then, a mouse model of intestinal mucositis was established by intraperitoneal injection of 5-FU (384 μmol/kg) to verify the prediction of network analysis. The network analysis results suggested that PTGS2 (Prostaglandin G/H synthase 2) and NOS2 (Nitric oxide synthase, inducible) might be the critical targets of ISL for reducing the intestinal toxicity of 5-FU. In addition, KEGG and GO enrichment analysis revealed that the HIF-1, TNF, MAPK, IL-17, PI3K-Akt, Ras, NF-kappa B signaling pathway, and biological processes of the inflammatory response, apoptosis regulation, NO production and NF-kappa B transcription factor activity might be involved in the mechanism of ISL against intestinal mucositis. Subsequent animal experiments showed that ISL could reduce the weight loss, leukopenia and mucosal damage caused by 5-FU. Compared with the intestinal mucositis model, the protein expressions of PTGS2, NOS2, TNFα (Tumor necrosis factor-alpha) and NF-κB p65 (nuclear factor kappa-B P65) were decreased after ISL treatment. In conclusion, this study is the fist time to find that ISL can attenuate 5-FU-induced intestinal mucositis in mice. Its anti-mucositis effect may be through regulating TNF/NF-κB pathway and inhibiting inflammatory mediators PTGS2 and NOS2. It will provide a potential candidate for the prevention and treatment of chemotherapy-induced intestinal mucositis.
5-fluorouracil (5-FU) is an analogue of uracil (McQuade et al., 2016). It can be activated into fluorouracil deoxynucleotide (FdUMP) in cells, which disrupts DNA synthesis by suppressing thymidylic acid synthase (Panchenko et al., 2018). 5-FU has been the backbone of therapy for many solid tumors, such as esophageal, breast, stomach and intestinal malignant tumors (McQuade et al., 2016; Zhang et al., 2019).
However, the non-selective cytotoxicity of 5-FU can cause serious side effects, including gastrointestinal dysfunction, mucosal inflammation, cardiotoxicity, and even myelosuppression (Atiq et al., 2019). Mucositis is the most common gastrointestinal toxicity, involving the entire gastrointestinal tract from the mouth to the anus. Especially intestinal mucositis has been regarded as one of the main obstacles to tumor chemotherapy. Intestinal mucositis usually presents with anorexia, nausea, vomiting, diarrhea and so on (Justino et al., 2020). And severe mucositis will develop into ulcers that invade the entire submucosa. This can cause severe pain and even require narcotic analgesics to relieve it (Xing et al., 2015). So that, patients have to reduce the drug dose or discontinue the medication, which may lead to efficacy reduction or treatment failure. However, the clinical treatment of intestinal mucositis is mainly symptomatic support, such as drugs to relieve pain or diarrhea. So far, chemotherapy-induced intestinal mucositis is still lack of effective drugs to target its pathogenesis. Therefore, it is necessary to explore drugs for intestinal mucositis.
Traditional Chinese medicine (TCM) has the advantages of low adverse reactions and stable efficacy. It has been widely used in tumor adjuvant therapy to enhance the efficacy of chemotherapy and reduce toxic effects. Clinical studies have shown that TCM can significantly reduce the incidence of diarrhea and other gastrointestinal symptoms during chemotherapy. Among these TCM, licorice, called Gancao in Chinese, is the most frequently used herbal medicine (Yang et al., 2017). In TCM treatment, licorice is one of the essential Chinese herbal medicines for treating gastrointestinal diseases (Yang et al., 2017).
ISL is one of the most important bioactive compounds in licorice (Tang et al., 2018). Numerous studies have demonstrated that ISL has inhibitory effects on various malignant tumors, such as breast cancer, prostate cancer, colon cancer, lung cancer, Ovary Cancer, cervical cancer and leukemia (Wang et al., 2021). It can also be combined with chemotherapeutic agents to enhance anti-tumor efficacy (Peng et al., 2015). In addition, ISL is an effective anti-inflammatory agent, which can reduce inflammation by inhibiting IL-1β, IL-6, TNFα and other cytokines (Du et al., 2016; Liao et al., 2020). Recent studies have shown that ISL can significantly suppress the dextran sulfate sodium (DSS)-induced colitis-associated tumorigenesis in mice and maintain the integrity of the intestinal mucosal barrier (Zhao et al., 2014; Wu C. H. et al., 2016). Those findings suggest that ISL can protect intestinal mucosa and reduce inflammatory injury. It may be used as an adjunctive agent to relieve 5-FU-induced intestinal mucositis during chemotherapy. Although the antitumor and the synergistic effect of ISL have been widely reported, the protective effect of ISL on intestinal mucositis has not been investigated, which is also one of the major factors affecting cancer treatment.
Network pharmacology is a new branch of pharmacology based on system biology and computer science. By constructing the molecular network of a biological system, the overall relationship between drug-target-disease can be comprehensively analyzed, and the target and mechanism of the drug on diseases can also be predicted (Zhu et al., 2018). Nowadays, network analysis has been widely used to screen the active ingredients and explore the mechanism of TCM.
In this study, a comprehensive network analysis approach was used to predict the mechanism of ISL on 5-FU-induced gastrointestinal toxicity. And the prediction results were further verified in a mouse model of intestinal mucositis. The flow of this study was shown in Figure 1. This is the first time to explore the effect and mechanism of ISL against intestinal mucositis induced by 5-FU. It may provide reference for clinical treatment and drug development of chemotherapy-induced intestinal mucositis.
The TCMSP database (https://old.tcmsp-e.com/tcmsp.php) (Fan et al., 2022) was employed to predict the pharmacological targets of ISL (ISL target, iT). A total of 31 iTs were collected. And the Bisogenet plug-in in Cytoscape 3.8.0 (Martin et al., 2010) was used to screen out 3,174 target proteins related to ISL targets (related target, rT), thus expanding the range of prediction targets and protein interaction network. The therapeutic targets for the gastrointestinal disease were obtained from the DisGeNET database (http://www.disgenet.org/) (Piñero et al., 2017) and the OMIM database (https://omim.org/) (Amberger et al., 2015). Five diseases associated with gastrointestinal injury were searched in those databases, such as gastritis, enteritis, colitis, inflammatory bowel disease and diarrhea. A total of 2,541 targets (duplicate value removed) associated with gastrointestinal disease (disease target, dT) were collected. Then, the CTD-Comparative database (http://ctdbase.org/) (Aihaiti et al., 2021) was used for the collection of 5-FU gastrointestinal toxic targets (toxic targets of 5-FU, tT). In total, 65 potential tTs were collected.
23 common targets were found among iT, rT, tT and dT, and submitted to the DAVID database (https://david.ncifcrf.gov/) (Aihaiti et al., 2021) to use Gene ontology (GO) analysis and Kyoto Encyclopedia of Genes and Genomes (KEGG) pathway enrichment analysis. The protein interaction relationship of the common targets was obtained by the database STRING (https://www.string-db.org/) (Aihaiti et al., 2021). And the networks were visualized using Cytoscape software (http://www.cytoscape.org/) (Aihaiti et al., 2021).
To verify the critical targets of ISL, a molecular docking of ISL with PTGS2 (Prostaglandin G/H synthase 2) and NOS2 (Nitric oxide synthase, inducible) was performed. And Known inhibitors of PTGS2 (Celecoxib) and NOS2 (N-Iminoethyl-L-lysine dihydrochloride) were used as reference (Alqahtani, 2021; Lawal et al., 2021). The 3D structures of PTGS2 and NOS2 were obtained from the Protein Data Bank (PDB, http://www.rcsb.org/) and then imported into PyMOL (v2.3.0) to remove water molecules. The structure of ISL, Celecoxib and N-Iminoethyl-L-lysine dihydrochloride were downloaded from the website of PubChem (https://pubchem.ncbi.nlm.nih.gov/) and then imported into PyMOL (v2.3.0) and AutoDockTools-1.5.6 software. The target proteins and ligands were prepared for molecular docking by Autodock Tools (ADT) program. Finally, molecular docking analysis was performed using the AutoDock Vina program (Trott and Olson, 2010). The crystal structures used for PTGS2 and NOS2 and the parameter settings for the grid box were provided in the Supplementary Material S1.
ISL (HPLC ≥98%) was purchased from Chengdu Pufei De Biotechnology Co., Ltd. 5-FU (HPLC >99%) was purchased from Dalian Meilun Biotechnology Co., Ltd. ISL was dissolved in saline containing 2% dimethylsulfoxide (DMSO). 5-FU was prepared in pyrogen-free saline.
36 male SPF BLAB/c mice (18–20 g, 6–8 weeks old) were obtained from Chengdu Dossy Experimental Animals Co., Ltd. Mice were raised under standard laboratory conditions at 23 ± 1°C and 55 ± 5% humidity with a 12 h light/dark cycle.
The mice were randomly allocated to six groups (6 mice per group): 1) Vehicle control (2% DMSO in saline); 2) ISL 117 μmol/kg; 3) ISL 234 μmol/kg; 4) Model (5-FU 384 μmol/kg); 5) 5-FU + ISL 117 μmol/kg; 6) 5-FU + ISL 234 μmol/kg. All drugs were injected intraperitoneally (i.p.). Except for the vehicle control and model (5-FU) group, all mice received ISL for 10 days. Mice in model and 5-FU + ISL groups were intraperitoneally injected with 5-FU (384 μmol/kg) every other day. The treatment schedule was shown in Figure 2. All mice were fed and waterad libitum during the entire experiment.
All animal experiments were approved by the Experimental Animal Ethical Committee of Chengdu Medical College, and the experimental protocols were carried out strictly in conformity with the guidance for Animal Experiments in Chengdu Medical College and the Declaration of Helsinki.
Mice were monitored daily for body weight, food intake and diarrhea. Bowen’s score system was used to assess diarrhea severity, and the grade ranged from 0 to 3 based on the consistency of stool: 0, normal stool; 1, the stool is slightly wet or soft, indicating mild diarrhea; 2, the stool is moderately wet with unformed pellets, indicating moderate diarrhea; 3, the stool is watery or loose stool, indicating severe diarrhea (Yeung et al., 2015; Atiq et al., 2019).
24 h after the last administration, the mice were anesthetized by intraperitoneal injection of 2% pentobarbital sodium. Then, 1 ml Peripheral blood from each mouse was collected via orbital blood and placed into an anticoagulant tube containing EDTA. The blood was prepared for a routine blood test, which included the analysis of white blood cell (WBC) count, neutrophil count, lymphocyte count, red blood cell (RBC) count and so on. All mice were then sacrificed immediately. And 1–2 cm of the middle segment of the small intestine and colon was dissected, washed with saline, and then fixed with paraformaldehyde for further analysis.
The paraformaldehyde-fixed small intestine and colon tissues were embedded in paraffin and sectioned at 3 μm thickness. The paraffin sections were baked at 60°C for 3 h. Then, sections were dewaxed with xylene, and placed in gradient ethanol (absolute ethanol twice for 3 min, 95% ethanol for 3 min, 75% ethanol for 3 min), and finally stained with hematoxylin for 12 min and eosin for 13 s 3-5 complete longitudinal small intestinal villi were selected from each sample for measurement (3 samples per group). The villi height was measured from the top of the villus to the villus-crypt junction using ImageJ software (Atiq et al., 2019).
After dewaxing and rehydration, paraffin sections of colon tissue were placed in sodium citrate buffer and heated by a microwave oven twice for 2–3 min to repair antigens. After washing twice with PBS for 5 min, 5% bovine serum albumin (BSA) was added to block non-special signals for 30 min at 37°C. BSA was then removed, and primary antibodies (1:200, Proteintech Group, Inc, United States) diluted with 1% BSA were added and incubated overnight at 4°C. After washing with PBS, slides were incubated with horseradish peroxidase (HRP)- or Alexa Fluor-secondary antibodies (1:1,000, Proteintech Group, Inc, United States) at room temperature in dark for 1 h. The slides were washed twice with PBS for 5 min, and incubated with diaminobenzidine (DAB) for 3–10 min to develop color, or with DAPI for 10 min to stain nuclei. Finally, the slides were sealed by neutral glue or anti-fluorescence quencher. 3-5 fields were randomly selected for each sample (3 samples per group) and observed under an Olympus BX63 fluorescence microscope (Olympus, Tokyo, Japan) at ×400. And ImageJ analysis software was used to assess the staining results. Protein expression was quantified as mean optical density (MOD = integrated option density/area). (Du et al., 2015; Wang et al., 2022).
Quantitative data were represented as the means ± standard deviations (SD). SPSS and GraphPad Prism were used for statistical analysis and graph drawing, and the differences between groups were analyzed by one-way analysis of variance (ANOVA). p < 0.05 was considered statistically significant.
Based on target prediction via TCMSP, the ISL-target network (Figure 3A) consisted of 31 nodes. To better evaluate the detoxification effect of ISL on 5-FU, rTs interacting with iTs were screened to expand the range of predicted targets, and a total of 3,174 rTs were obtained (Figure 3B). Finally, 23 common targets were obtained from the intersection of tTs, dTs and iTs or rTs. The 23 targets could be considered as potential targets (pT) for ISL to reduce the gastrointestinal toxicity of 5-FU. Among them, PPARG, PTGS2 and NOS2 were the direct targets of ISL (iT) predicted by TCMSP. Moreover, PTGS2 (also known as cyclooxygenases-2, COX-2) and NOS2 (also known as inducible nitric oxidesynthase, iNOS) have been reported to be involved in the occurrence of 5-FU-caused intestinal mucositis (Atiq et al., 2019; Yan et al., 2020; Huang et al., 2022). Therefore, PTGS2 and NOS2 could be considered as critical targets of ISL against 5-FU-induced gastrointestinal toxicity.
FIGURE 3. Targets of ISL against 5-FU gastrointestinal toxicity predicted by network analysis. (A) The ISL-target network. The node in green color is ISL, and the nodes in red color are candidate targets of ISL. (B) Protein interaction network of ISL target (iT) and its related target (rT). The nodes in red color are iTs, and the nodes in blue color are rTs. (C) Protein interaction network of potential targets (pT) of ISL for detoxification. The nodes in red color are pTs for ISL against the gastrointestinal toxicity of 5-FU, the nodes in dark red color are the critical targets for the detoxification of ISL.
As shown in Figure 4A, a network of ISL-potential targets-5-FU-gastrointestinal disease network (ISL-pT-5FU-D) was constructed to reflect the relationship between ISL (green nodes), 5-FU (blue nodes), gastrointestinal diseases (orange nodes) and their common targets (red nodes). We further investigated the correlation degree of each node and sequenced them with the node size (Shao, 2021). More information about the nodes was provided in the Supplementary Materials S2.
FIGURE 4. Network analysis on the mechanism of ISL against 5-FU-induced gastrointestinal toxicity. (A) Network of ISL, potential targets, 5-FU, and gastrointestinal diseases (ISL-pT-5FU-D Network). The node in blue color is 5-FU, the nodes in red color are potential targets, the nodes in orange color are gastrointestinal diseases, and the node in green color is ISL. (B) KEGG pathway enrichment analysis of potential targets. The pathways related to inflammation and gastrointestinal diseases with p values less than 0.05 are shown. (C) GO enrichment analysis of potential targets: cellular components, molecular functions, and biological processes associated with the detoxification effect of ISL on 5-FU. The top 8 with lower p-value are shown.
Next, a bioinformatics analysis of potential targets was performed. As shown in Figures 4B,C, the alleviating effect of ISL on 5-FU-induced gastrointestinal toxicity might be related to biological processes such as inflammatory response, apoptosis regulation, NO production and NF-kappa B transcription factor activity. And the molecular mechanism might involve multiple signaling pathways, such as HIF-1, VEGF, TNF, MAPK, IL-17, PI3K-Akt, Ras, NF-kappa B, Toll-like receptor pathway. Therefore, network analysis suggested that ISL might alleviate 5-FU-induced gastrointestinal toxicity through multiple targets and pathways, which were mainly involved in regulating inflammation and apoptosis.
To verify the accuracy of the above predictions, a molecular docking analysis of ISL with PTGS2 and NOS2 enzyme was performed. ISL has large binding affinity for PTGS2 and NOS2 protein with binding energies of −9.1 and −8.7 kcal/mol, respectively. Furthermore, ISL showed a higher binding energy with PTGS2 and NOS2 than the known protein inhibitor (Table 1). This suggested that ISL might bind and inhibit PTGS2 and NOS2 protein. In addition, as shown in Figure 5, ISL can interact with NOS2 by two H-bonds with ARG260 and TRP366, and interact with PTGS2 by three H-bonds with TRP387, THR206 and ALA199. ISL was successfully docked with PTGS2 and NOS2 protein.
To assess the severity of gastrointestinal injury caused by 5-FU, mice were monitored daily for body weight, food intake and diarrhea. The results were shown in Figure 6. Compared with the vehicle control, intraperitoneal injection with 5-FU significantly reduced body weight, increased diarrhea, and decreased food intake of mice (p < 0.05). After combined treatment with ISL, the weight loss of mice was slower, and the food intake in mice was increased compared with that of the 5-FU group (p < 0.05). Although no significant difference was observed in diarrhea scores between the 5-FU and 5-FU + ISL groups, the diarrhea of mice in the combination groups showed a decreasing trend.
FIGURE 6. Effect of ISL on body weight, diarrhea, and daily food intake in mice with or without 5-FU intraperitoneal injection. (A) Changes in daily body weights. (B) Changes in daily diarrhea. (C) Mean daily food intake. Data are presented as mean ± S.D. (n = 6 in each group). #p < 0.05 and ##p < 0.01 vs. control group; *p < 0.05 and **p < 0.01 vs. 5-FU group.
Furthermore, the blood routine indexes were detected to evaluate the effect of ISL on hematotoxicity. As shown in Table 2, the counts of WBC, lymphocytes, neutrophils and RBC were significantly decreased in the 5-FU group compared with the vehicle control group (p < 0.05). And compared to the 5-FU group, the WBC, lymphocytes, and neutrophils indexes in the 5-FU + 234 μmol/kg ISL combination group were significantly increased (p < 0.05). Thus, these results suggested that ISL could alleviate gastrointestinal toxicity symptoms and leukopenia caused by 5-FU.
As shown in Figure 7, no histomorphological changes were observed in the small intestine and colon of the vehicle control mice, with clear intestinal tissue structure, neatly arranged villi, normal epithelial cell morphology, and densely arranged intestinal glands. In the ISL groups, the mucosal structure of mice remained intact. The intestinal mucosa of mice in the 5-FU group was the most seriously damaged, with loose cell arrangement and obvious inflammatory cell infiltration. And the villi heights were significantly lower than that of the vehicle control (p < 0.01). Compared with the 5-FU group, the mice in the 5-FU + ISL combination groups exhibited less mucosal damage, less inflammatory cell infiltration, and greater villi height (p < 0.01). It indicated the protective effect of ISL on 5-FU-induced intestinal mucosal damage.
FIGURE 7. Effect of ISL on intestinal histopathological damage in mice. (A)Hematoxylin and eosin (H&E) staining of small intestine and colon. All images are displayed at ×200 magnification. Inflammatory cell infiltration (black arrows) is observed in the 5-FU group. (B) Length of small intestinal villi. Data are presented as mean ± S.D. (n = 3 in each group). **p < 0.01.
To verify the molecular mechanism of ISL against 5-FU-induced intestinal mucositis, the expression of PTGS2, NOS2, TNFα, and NF-κB p65 in colon tissues were detected. As shown in Figure 8, compared with the vehicle control, 5-FU treatment significantly increased the expression of PTGS2, NOS2, TNFα and NF-κB p65 (p < 0.05). These protein levels were obviously decreased in the colon tissues of mice in the 5-FU + ISL combination groups compared to the 5-FU group. And the decrease in the 5-FU+234 μmol/kg ISL group was highly significant (p < 0.05). The results suggested that the alleviating effect of ISL on 5-FU-induced intestinal mucositis might be related to the inhibition of PTGS2, NOS2, TNFα and NF-κB p65, which was consistent with our network pharmacological predictions.
FIGURE 8. Immunohistochemistry and immunofluorescence analysis for PTGS2, NOS2, TNFα and NF-κB p65. MOD, mean optical density. All images are displayed at ×400 magnification. Data are presented as mean ± SD. (n = 3 in each group). *p < 0.05 and **p < 0.01.
Currently, 5-FU is a routine treatment for a variety of malignant tumors. It is also known to cause severe gastrointestinal toxicity, such as intestinal mucositis. In intestinal mucositis, 5-FU can induce cell apoptosis, force intestinal mucosa to atrophy, damage the intestinal villi, and reduce the absorption of intestinal contents and water, thus causing dyspepsia, anorexia, abdominal pain and diarrhea (Yan et al., 2020). These serious toxic effects of 5-FU will reduce patients’ treatment compliance, and even lead to the interruption of chemotherapy (Kato et al., 2015; Xing et al., 2015). Therefore, it is necessary to explore novel drugs to prevent and treat intestinal mucositis caused by 5-FU.
Recent studies have shown that inflammatory cytokines IL-1β, IL-6, TNFα, and NF-κB are significantly increased in 5-FU-induced intestinal mucositis in mice (Ali et al., 2019; Atiq et al., 2019). 5-FU can induce the production of reactive oxygen species (ROS), promote DNA damage and then activate NF-κB (Chang et al., 2012; Oliveira et al., 2021). Next, NF-κB activation leads to the release of inflammatory mediators, including TNFα, COX-2, iNOS, IL-1β, and so on (Kim et al., 2009; Abdelhamid et al., 2020). TNFα, in turn, activates NF-κB. This positive feedback mechanism results in signal amplification and increased production of inflammatory mediators, which eventually destroy the intestinal mucosal barrier and lead to the formation of mucosal ulcers (Huang et al., 2022; Shi et al., 2022).
In this study, a mouse model of intestinal mucositis was established by intraperitoneal injection of 5-FU. Consistent with the previous studies, administration of 5-FU caused severe diarrhea, weight loss and anorexia in mice, which were the main symptoms of intestinal mucositis. (Soares et al., 2013; Zhang et al., 2019). And histopathological changes were also demonstrated, such as shortening of villi, inflammatory cell infiltration and loss of mucosal structure. And the expressions of NOS2, TNFα, NF-κB P65 and PTGS2 in colon tissues were significantly increased. It suggested that these inflammatory mediators and their signaling pathways might be implicated in the occurrence of intestinal mucositis.
ISL, a natural chalcone flavonoid, has been shown to exhibit good pharmacokinetic properties and a variety of biological activities (Wang et al., 2021), including anti-tumor, anti-inflammatory, immunomodulatory, gastrointestinal protection and so on (Wu M. et al., 2016; Zhao et al., 2019; Liao et al., 2020). In particular, its high antitumor effiacy has attracted extensive attention in recent years. Numerous studies have found that ISL can inhibit the proliferation and induce apoptosis of various cancer cells (Peng et al., 2015; Wang et al., 2021). At the same time, its cytotoxic effect shows selectivity, which can significantly inhibit the viability of tumor cells but almost no cytotoxicity to normal cells (Wu C. H. et al., 2016). ISL also significantly suppresses the growth and metastasis of various tumors in mouse models (Peng et al., 2015; Wang et al., 2021). In addition, ISL has synergistic antitumor effect with chemotherapies. For example, Zhang et al. reported that ISL synergistically sensitized the cytotoxic effect of gemcitabine and 5-FU on pancreatic cancer cells (Zhang et al., 2022). Consistently, Jin et al. demonstrated that ISL potentiated the apoptotic effects of 5-FU on HCT-116 cells (Jin et al., 2018). Overall, both in vitro and in vivo studies have demonstrated the potential of ISL for cancer treatment and adjuvant chemotherapy.
In addition, the anti-inflammatory effect of ISL has also been widely reported. Studies have shown that ISL can attenuate the inflammatory injury induced by LPS or carrageenan. Its anti-inflammatory effect is through inhibiting NF-κB and then causing iNOS, TNFα, COX-2, IL-6 and other inflammatory cytokines to down-regulation (Kim et al., 2008; Kim et al., 2008; Tang et al., 2018; Liao et al., 2020). Furthermore, ISL can protect mouse gastrointestinal tracts, and suppress DSS/AOM-induced colon inflammation-related tumorigenesis by down regulating PGE2 and IL-6 (Zhao et al., 2014; Wu M. et al., 2016). These findings suggest that ISL is a promising anti-inflammatory and intestinal protective agent. However, the effect of ISL on intestinal mucositis remains unknown.
Therefore, we observed the alleviating effect of ISL on 5-FU-induced intestinal mucositis in this study. The results showed that ISL could significantly reduce intestinal mucosal damage and its symptoms caused by 5-FU in mice. In addition, leukopenia, one of the main toxic effects of 5-FU, was also observed to be attenuated by ISL. These results reveal for the first time that ISL has good potential for alleviating 5-FU-induced gastrointestinal toxicity in adjuvant tumor therapy, and its mechanism is worthy of further investigation.
Network pharmacological analysis is a new approach to predict the potential pharmacological effects and mechanisms of TCM and its monomers. In this study, network analysis was used to predict the therapeutic targets of ISL on 5-FU-induced intestinal mucositis. And the results showed that PTGS2 and NOS2 might be the critical targets of ISL for reducing intestinal mucositis. In addition, KEGG and GO enrichment analysis indicated that the anti-intestinal mucositis mechanism of ISL was mainly related to inflammatory and apoptosis-related signaling pathways and biological processes, such as HIF-1 pathway, TNF pathway, PI3K-Akt pathway, NF-κB pathway, NO production, NF-κB transcription factor activity, etc.
As we know, TNF is the critical signaling pathway in regulating inflammatory responses (Chu, 2013). TNF family cytokines can promote the expression of inflammation-related genes mainly by activating the NF-κB pathway. (Hayden and Ghosh, 2011; Gilmore and Wolenski, 2012). NF-κB plays a critical role in the transcription of multiple inflammatory mediators. It is usually inactive in the form of p50/P65 dimer binding to its inhibitor kappaB (IκB) (Oeckinghaus et al., 2011). NF-κB can be activated by a variety of intracellular and extracellular stimuli, such as viruses, oxygen free radicals, and various cytokines (Lawrence, 2009; Oeckinghaus and Ghosh, 2009). Among them, TNF family cytokines are considered to be the best-characterized inducers of NF-κB (Ciuffa et al., 2017). Upon stimulation, IκB kinase is activated, resulting in the degradation of IκB protein and the release of NF-κB dimers. NF-κB dimers are then transferred into the nucleus to facilitate transcription of the target genes, such as iNOS, TNFα, COX-2 and IL-1β (Surh et al., 2001; Kim et al., 2009; Abdelhamid et al., 2020; Yu et al., 2020). Some of these inflammatory factors can bind to cell membrane receptors and reactivate NF-κB signaling (Figure 9).
Previous studies have suggested that TNF/NF-κB pathway and its downstream inflammatory mediators may be effective targets for alleviating chemotherapy-induced mucositis. For example, Yuan et al. reported that inhibiting NF-κB activity could reduce inflammation and mucosal damage caused by 5-FU in rats (Yuan et al., 2015). Ribeiro et al. demonstrated that down-regulation of NF-κB p65 and TNF attenuated 5-FU-induced oral mucositis (Ribeiro et al., 2017). Similarly, Barbosa et al. reported that suppressing inflammatory mediators, such as TNFα, IL-1 β and iNOS, could reduce oral mucositis (Barbosa et al., 2019). Moreover, Chang et al. found that COX-2 activity was increased in 5-FU-induced mucositis (Chang et al., 2012). In this study, we examined the effect of ISL on 5-FU-induced expression of TNFα, NF-κB, PTGS2 and NOS2 in mice with intestinal mucositis for the first time. PTGS2 (COX-2) and NOS2 (iNOS) were the critical targets of ISL predicted by network analysis. TNFα and NF-κB p65 were the key nodes in the TNF/NF-κB inflammatory pathway. The results showed that ISL significantly reduced the 5-FU-induced expression of TNFα, NF-κB, PTGS2 and NOS2 in colon tissues. It is consistent with the target prediction and KEGG pathway enrichment results of network pharmacological analysis (Figure 9).
In conclusion, the network analysis and pharmacological experimental verification show that ISL can ameliorate 5-FU-induced intestinal mucositis. Its mechanism may be related to the negative regulation of the TNF/NF-κB pathway, and the inhibition of inflammatory mediators PTGS2 and NOS2. Our study may provide a potential drug candidate for 5-FU-induced intestinal mucositis. Moreover, This study is the first to show that ISL alleviates the gastrointestinal toxicity of 5-FU, which will provide a new reference for the research and development of ISL. However, this study also has limitations, such as the mechanism of ISL against intestinal mucositis has not been thoroughly explored. And further in vivo and in vitro experiments will be considered in our future studies.
The raw data supporting the conclusions of this article will be made available by the authors, without undue reservation.
The animal study was reviewed and approved by the Experimental Animal Welfare Ethics Committee of Chengdu Medical College.
YW and SL contributed to conception and design of the study. QM designed and directed the network pharmacology research strategy. Y-FL, F-LL, S-ST, YY, SW, and T-YJ implemented the methods of the study. YW supervised and directed the execution of the research. Y-FL, F-LL, S-ST completed the collation and statistical analysis of the experimental data and wrote the first draft of the manuscript. YW, SL, and J-WH wrote the final manuscript. All authors contributed to manuscript revision, read, and approved the submitted version.
This study was supported by the Natural Science Foundation of Science and Technology Department of Sichuan Province (2022NSFSC1440, 2021JDRC0156), the National College Students’ innovation and entrepreneurship training program (201813705028), Sichuan Province College Students’ Innovation and Entrepreneurship Training Program (S201913705090, 202113705021).
The authors declare that the research was conducted in the absence of any commercial or financial relationships that could be construed as a potential conflict of interest.
All claims expressed in this article are solely those of the authors and do not necessarily represent those of their affiliated organizations, or those of the publisher, the editors and the reviewers. Any product that may be evaluated in this article, or claim that may be made by its manufacturer, is not guaranteed or endorsed by the publisher.
The Supplementary Material for this article can be found online at: https://www.frontiersin.org/articles/10.3389/fphar.2022.1014160/full#supplementary-material
Abdelhamid, A. M., Elsheakh, A. R., Abdelaziz, R. R., and Suddek, G. M. (2020). Empagliflozin ameliorates ethanol-induced liver injury by modulating NF-κB/Nrf-2/PPAR-γ interplay in mice. Life Sci. 256, 117908. doi:10.1016/j.lfs.2020.117908
Aihaiti, Y., Song Cai, Y., Tuerhong, X., Ni Yang, Y., Ma, Y., Shi Zheng, H., et al. (2021). Therapeutic effects of naringin in rheumatoid arthritis: Network pharmacology and experimental validation. Front. Pharmacol. 12, 672054. doi:10.3389/fphar.2021.672054
Ali, J., Khan, A. U., Shah, F. A., Ali, H., Islam, S. U., Kim, Y. S., et al. (2019). Mucoprotective effects of Saikosaponin-A in 5-fluorouracil-induced intestinal mucositis in mice model. Life Sci. 239, 116888. doi:10.1016/j.lfs.2019.116888
Alqahtani, Y. S. (2021). Bioactive stigmastadienone from isodon rugosus as potential anticholinesterase, α-glucosidase and COX/LOX inhibitor: In-vitro and molecular docking studies. Steroids 172, 108857. doi:10.1016/j.steroids.2021.108857
Amberger, J. S., Bocchini, C. A., Schiettecatte, F., Scott, A. F., and Hamosh, A. (2015). OMIM.org: Online Mendelian Inheritance in Man (OMIM®), an online catalog of human genes and genetic disorders. Nucleic Acids Res. 43, D789–D798. doi:10.1093/nar/gku1205
Atiq, A., Shal, B., Naveed, M., Khan, A., Ali, J., Zeeshan, S., et al. (2019). Diadzein ameliorates 5-fluorouracil-induced intestinal mucositis by suppressing oxidative stress and inflammatory mediators in rodents. Eur. J. Pharmacol. 843, 292–306. doi:10.1016/j.ejphar.2018.12.014
Barbosa, S., Pereira, V., Wong, D., Santana, A., Lucetti, L. T., Carvalho, L. L., et al. (2019). Amifostine reduces inflammation and protects against 5-fluorouracil-induced oral mucositis and hyposalivation. Braz. J. Med. Biol. Res. = Revista brasileira de pesquisas medicas e Biol. 52 (3), e8251. doi:10.1590/1414-431X20188251
Chang, C. T., Ho, T. Y., Lin, H., Liang, J. A., Huang, H. C., Li, C. C., et al. (2012). 5-Fluorouracil induced intestinal mucositis via nuclear factor-κB activation by transcriptomic analysis and in vivo bioluminescence imaging. PloS one 7 (3), e31808. doi:10.1371/journal.pone.0031808
Chu, W. M. (2013). Tumor necrosis factor. Cancer Lett. 328 (2), 222–225. doi:10.1016/j.canlet.2012.10.014
Ciuffa, R., Caron, E., Leitner, A., Uliana, F., Gstaiger, M., and Aebersold, R. (2017). Contribution of mass spectrometry-based proteomics to the understanding of TNF-α signaling. J. Proteome Res. 16 (1), 14–33. doi:10.1021/acs.jproteome.6b00728
Du, F., Gesang, Q., Cao, J., Qian, M., Ma, L., Wu, D., et al. (2016). Isoliquiritigenin attenuates atherogenesis in apolipoprotein E-deficient mice. Int. J. Mol. Sci. 17 (11), 1932. doi:10.3390/ijms17111932
Du, H., Shi, H., Chen, D., Zhou, Y., and Che, G. (2015). Cross-talk between endothelial and tumor cells via basic fibroblast growth factor and vascular endothelial growth factor signaling promotes lung cancer growth and angiogenesis. Oncol. Lett. 9 (3), 1089–1094. doi:10.3892/ol.2015.2881
Fan, Z., Chen, J., Yang, Q., and He, J. (2022). Network pharmacology and experimental validation to reveal the pharmacological mechanisms of chongcaoyishen decoction against chronic kidney disease. Front. Mol. Biosci. 9, 847812. doi:10.3389/fmolb.2022.847812
Gilmore, T. D., and Wolenski, F. S. (2012). NF-κB: Where did it come from and why? Immunol. Rev. 246 (1), 14–35. doi:10.1111/j.1600-065X.2012.01096.x
Hayden, M. S., and Ghosh, S. (2011). NF-κB in immunobiology. Cell Res. 21 (2), 223–244. doi:10.1038/cr.2011.13
Huang, R., Ai, G., Zhong, L., Mai, L., Chen, J. N., Liu, Y., et al. (2022). Protective effects of oxyberberine in 5-fluorouracil-induced intestinal mucositis in the mice model. Evid. Based. Complement. Altern. Med. 2022, 1238358. doi:10.1155/2022/1238358
Jin, H., Seo, G. S., and Lee, S. H. (2018). Isoliquiritigenin-mediated p62/SQSTM1 induction regulates apoptotic potential through attenuation of caspase-8 activation in colorectal cancer cells. Eur. J. Pharmacol. 841, 90–97. doi:10.1016/j.ejphar.2018.10.015
Justino, P., Franco, A. X., Pontier-Bres, R., Monteiro, C., Barbosa, A., Souza, M., et al. (2020). Modulation of 5-fluorouracil activation of toll-like/MyD88/NF-κB/MAPK pathway by Saccharomyces boulardii CNCM I-745 probiotic. Cytokine 125, 154791. doi:10.1016/j.cyto.2019.154791
Kato, S., Hayashi, S., Kitahara, Y., Nagasawa, K., Aono, H., Shibata, J., et al. (2015). Saireito (TJ-114), a Japanese traditional herbal medicine, reduces 5-fluorouracil-induced intestinal mucositis in mice by inhibiting cytokine-mediated apoptosis in intestinal crypt cells. PloS one 10 (1), e0116213. doi:10.1371/journal.pone.0116213
Kim, J. Y., Park, S. J., Yun, K. J., Cho, Y. W., Park, H. J., and Lee, K. T. (2008). Isoliquiritigenin isolated from the roots of Glycyrrhiza uralensis inhibits LPS-induced iNOS and COX-2 expression via the attenuation of NF-kappaB in RAW 264.7 macrophages. Eur. J. Pharmacol. 584 (1), 175–184. doi:10.1016/j.ejphar.2008.01.032
Kim, Y. H., Kim, D. H., Lim, H., Baek, D. Y., Shin, H. K., and Kim, J. K. (2009). The anti-inflammatory effects of methylsulfonylmethane on lipopolysaccharide-induced inflammatory responses in murine macrophages. Biol. Pharm. Bull. 32 (4), 651–656. doi:10.1248/bpb.32.651
Lawal, B., Lee, C. Y., Mokgautsi, N., Sumitra, M. R., Khedkar, H., Wu, A., et al. (2021). mTOR/EGFR/iNOS/MAP2K1/FGFR/TGFB1 are druggable candidates for N-(2, 4-difluorophenyl)-2', 4'-difluoro-4-hydroxybiphenyl-3-carboxamide (NSC765598), with consequent anticancer implications. Front. Oncol. 11, 656738. doi:10.3389/fonc.2021.656738
Lawrence, T. (2009). The nuclear factor NF-kappaB pathway in inflammation. Cold Spring Harb. Perspect. Biol. 1 (6), a001651. doi:10.1101/cshperspect.a001651
Liao, Y., Tan, R. Z., Li, J. C., Liu, T. T., Zhong, X., Yan, Y., et al. (2020). Isoliquiritigenin attenuates UUO-induced renal inflammation and fibrosis by inhibiting mincle/syk/NF-kappa B signaling pathway. Drug Des. devel. Ther. 14, 1455–1468. doi:10.2147/DDDT.S243420
Martin, A., Ochagavia, M. E., Rabasa, L. C., Miranda, J., Fernandez-de-Cossio, J., and Bringas, R. (2010). BisoGenet: A new tool for gene network building, visualization and analysis. BMC Bioinforma. 11, 91. doi:10.1186/1471-2105-11-91
McQuade, R. M., Stojanovska, V., Donald, E., Abalo, R., Bornstein, J. C., and Nurgali, K. (2016). Gastrointestinal dysfunction and enteric neurotoxicity following treatment with anticancer chemotherapeutic agent 5-fluorouracil. Neurogastroenterol. Motil. 28 (12), 1861–1875. doi:10.1111/nmo.12890
Oeckinghaus, A., and Ghosh, S. (2009). The NF-kappaB family of transcription factors and its regulation. Cold Spring Harb. Perspect. Biol. 1 (4), a000034. doi:10.1101/cshperspect.a000034
Oeckinghaus, A., Hayden, M. S., and Ghosh, S. (2011). Crosstalk in NF-κB signaling pathways. Nat. Immunol. 12 (8), 695–708. doi:10.1038/ni.2065
Oliveira, M., de Araújo, A. A., Ribeiro, S. B., de Sales Mota, P., Marques, V. B., da Silva Martins Rebouças, C., et al. (2021). Losartan improves intestinal mucositis induced by 5-fluorouracil in mice. Sci. Rep. 11 (1), 23241. doi:10.1038/s41598-021-01969-x
Panchenko, A. V., Fedoros, E. I., Pigarev, S. E., Maydin, M. A., Gubareva, E. A., Yurova, M. N., et al. (2018). Effect of the polyphenol composition BP-C3 on haematological and intestinal indicators of 5-fluorouracil toxicity in mice. Exp. Ther. Med. 15 (3), 3124–3132. doi:10.3892/etm.2018.5782
Peng, F., Du, Q., Peng, C., Wang, N., Tang, H., Xie, X., et al. (2015). A review: The pharmacology of Isoliquiritigenin. Phytother. Res. 29 (7), 969–977. doi:10.1002/ptr.5348
Piñero, J., Bravo, À., Queralt-Rosinach, N., Gutiérrez-Sacristán, A., Deu-Pons, J., Centeno, E., et al. (2017). DisGeNET: A comprehensive platform integrating information on human disease-associated genes and variants. Nucleic Acids Res. 45 (D1), D833–D839. doi:10.1093/nar/gkw943
Ribeiro, S. B., de Araújo, A. A., Araújo Júnior, R. F., Brito, G., Leitão, R. C., Barbosa, M. M., et al. (2017). Protective effect of dexamethasone on 5-FU-induced oral mucositis in hamsters. PloS one 12 (10), e0186511. doi:10.1371/journal.pone.0186511
Shao, L. (2021). Network pharmacology evaluation method guidance-draft. World J. Tradit. Chin. Med. 7 (01), 148–166+146154. doi:10.4103/wjtcm.wjtcm_11_21
Shi, P., Zhao, T., Wang, W., Peng, F., Wang, T., Jia, Y., et al. (2022). Protective effect of homogeneous polysaccharides of Wuguchong (HPW) on intestinal mucositis induced by 5-fluorouracil in mice. Nutr. Metab. 19 (1), 36. doi:10.1186/s12986-022-00669-1
Soares, P. M., Mota, J. M., Souza, E. P., Justino, P. F., Franco, A. X., Cunha, F. Q., et al. (2013). Inflammatory intestinal damage induced by 5-fluorouracil requires IL-4. Cytokine 61 (1), 46–49. doi:10.1016/j.cyto.2012.10.003
Surh, Y. J., Chun, K. S., Cha, H. H., Han, S. S., Keum, Y. S., Park, K. K., et al. (2001). Molecular mechanisms underlying chemopreventive activities of anti-inflammatory phytochemicals: Down-regulation of COX-2 and iNOS through suppression of NF-kappa B activation. Mutat. Res. 480-481, 243–268. doi:10.1016/s0027-5107(01)00183-x
Tang, Y., Wang, C., Wang, Y., Zhang, J., Wang, F., Li, L., et al. (2018). Isoliquiritigenin attenuates LPS-induced AKI by suppression of inflammation involving NF-κB pathway. Am. J. Transl. Res. 10 (12), 4141–4151.
Trott, O., and Olson, A. J. (2010). AutoDock Vina: Improving the speed and accuracy of docking with a new scoring function, efficient optimization, and multithreading. J. Comput. Chem. 31 (2), 455–461. doi:10.1002/jcc.21334
Wang, K. L., Yu, Y. C., and Hsia, S. M. (2021). Perspectives on the role of Isoliquiritigenin in cancer. Cancers 13 (1), 115. doi:10.3390/cancers13010115
Wang, R. X., Wu, L., Chen, S. F., Li, Z. Y., Zhao, M. H., and Chen, M. (2022). Renal expression of annexin A1 is associated with the severity of renal injury in antineutrophil cytoplasmic autoantibody-associated vasculitis. Front. Med. 9, 769813. doi:10.3389/fmed.2022.769813
Wu, C. H., Chen, H. Y., Wang, C. W., Shieh, T. M., Huang, T. C., Lin, L. C., et al. (2016a). Isoliquiritigenin induces apoptosis and autophagy and inhibits endometrial cancer growth in mice. Oncotarget 7 (45), 73432–73447. doi:10.18632/oncotarget.12369
Wu, M., Wu, Y., Deng, B., Li, J., Cao, H., Qu, Y., et al. (2016b). Isoliquiritigenin decreases the incidence of colitis-associated colorectal cancer by modulating the intestinal microbiota. Oncotarget 7 (51), 85318–85331. doi:10.18632/oncotarget.13347
Xing, F., Chen, C., Wang, C., and Lou, J. (2015). Explore the function of gancao xiexin decoction in the prevention and treatment of 5-fu related mucositis. J. Liaoning Univ. Traditional Chin. Med. 17 (09), 125–127. doi:10.13194/j.issn.1673-842x.2015.09.043
Yan, X. X., Li, H. L., Zhang, Y. T., Wu, S. Y., Lu, H. L., Yu, X. L., et al. (2020). A new recombinant MS-superoxide dismutase alleviates 5-fluorouracil-induced intestinal mucositis in mice. Acta Pharmacol. Sin. 41 (3), 348–357. doi:10.1038/s41401-019-0295-8
Yang, R., Yuan, B. C., Ma, Y. S., Zhou, S., and Liu, Y. (2017). The anti-inflammatory activity of licorice, a widely used Chinese herb. Pharm. Biol. 55 (1), 5–18. doi:10.1080/13880209.2016.1225775
Yeung, C. Y., Chan, W. T., Jiang, C. B., Cheng, M. L., Liu, C. Y., Chang, S. W., et al. (2015). Amelioration of chemotherapy-induced intestinal mucositis by orally administered probiotics in a mouse model. PloS one 10 (9), e0138746. doi:10.1371/journal.pone.0138746
Yu, H., Lin, L., Zhang, Z., Zhang, H., and Hu, H. (2020). Targeting NF-κB pathway for the therapy of diseases: Mechanism and clinical study. Signal Transduct. Target. Ther. 5 (1), 209. doi:10.1038/s41392-020-00312-6
Yuan, K. T., Yu, H. L., Feng, W. D., Chong, P., Yang, T., Xue, C. L., et al. (2015). Bifidobacterium infantis has a beneficial effect on 5-fluorouracil-induced intestinal mucositis in rats. Benef. Microbes 6 (1), 113–118. doi:10.3920/BM2013.0095
Zhang, L., Jin, Y., Peng, J., Chen, W., Lisha, L., and Lin, J. (2019). Qingjie Fuzheng Granule attenuates 5-fluorouracil-induced intestinal mucosal damage. Biomed. Pharmacother. = Biomedecine Pharmacother. 118, 109223. doi:10.1016/j.biopha.2019.109223
Zhang, Z., Chen, W. Q., Zhang, S. Q., Bai, J. X., Liu, B., Yung, K. K., et al. (2022). Isoliquiritigenin inhibits pancreatic cancer progression through blockade of p38 MAPK-regulated autophagy. Phytomedicine. 106, 154406. Advance online publication. doi:10.1016/j.phymed.2022.154406
Zhao, H., Zhang, X., Chen, X., Li, Y., Ke, Z., Tang, T., et al. (2014). Isoliquiritigenin, a flavonoid from licorice, blocks M2 macrophage polarization in colitis-associated tumorigenesis through downregulating PGE2 and IL-6. Toxicol. Appl. Pharmacol. 279 (3), 311–321. doi:10.1016/j.taap.2014.07.001
Zhao, T. T., Xu, Y. Q., Hu, H. M., Gong, H. B., and Zhu, H. L. (2019). Isoliquiritigenin (ISL) and its formulations: Potential antitumor agents. Curr. Med. Chem. 26 (37), 6786–6796. doi:10.2174/0929867325666181112091700
Zhu, B., Zhang, W., Lu, Y., Hu, S., Gao, R., Sun, Z., et al. (2018). Network pharmacology-based identification of protective mechanism of Panax Notoginseng Saponins on aspirin induced gastrointestinal injury. Biomed. Pharmacother. = Biomedecine Pharmacother. 105, 159–166. doi:10.1016/j.biopha.2018.04.054
Keywords: 5-fluorouracil, intestinal mucositis, Isoliquiritigenin, inflammatory mediator, network analysis
Citation: Liao Y-f, Luo F-l, Tang S-s, Huang J-w, Yang Y, Wang S, Jiang T-y, Man Q, Liu S and Wu Y-y (2022) Network analysis and experimental pharmacology study explore the protective effects of Isoliquiritigenin on 5-fluorouracil-Induced intestinal mucositis. Front. Pharmacol. 13:1014160. doi: 10.3389/fphar.2022.1014160
Received: 08 August 2022; Accepted: 20 September 2022;
Published: 06 October 2022.
Edited by:
Ren-You Gan, Agency for Science, Technology and Research, SingaporeReviewed by:
Ming Niu, Fifth Medical Center of the PLA General Hospital, ChinaCopyright © 2022 Liao, Luo, Tang, Huang, Yang, Wang, Jiang, Man, Liu and Wu. This is an open-access article distributed under the terms of the Creative Commons Attribution License (CC BY). The use, distribution or reproduction in other forums is permitted, provided the original author(s) and the copyright owner(s) are credited and that the original publication in this journal is cited, in accordance with accepted academic practice. No use, distribution or reproduction is permitted which does not comply with these terms.
*Correspondence: Yi-ying Wu, d3V5aXlpbmdAY21jLmVkdS5jbg==; Qiong Man, bWFucWlvbmdAY21jLmVkdS5jbg==; Sha Liu, MTAyMDE1MDA1QGNtYy5lZHUuY24=
†These authors have contributed equally to this work and share first authorship
Disclaimer: All claims expressed in this article are solely those of the authors and do not necessarily represent those of their affiliated organizations, or those of the publisher, the editors and the reviewers. Any product that may be evaluated in this article or claim that may be made by its manufacturer is not guaranteed or endorsed by the publisher.
Research integrity at Frontiers
Learn more about the work of our research integrity team to safeguard the quality of each article we publish.