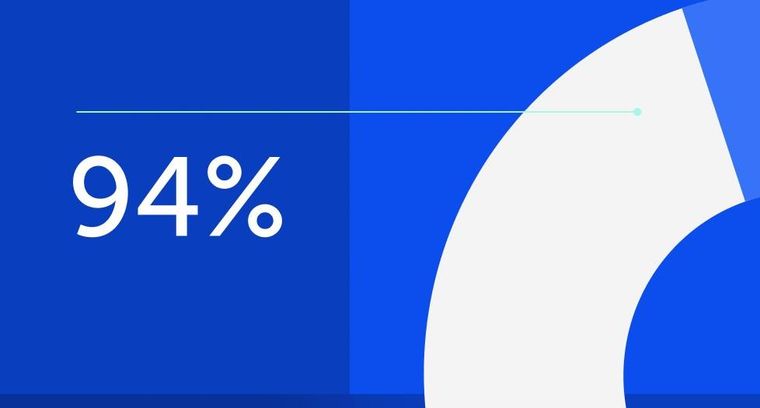
94% of researchers rate our articles as excellent or good
Learn more about the work of our research integrity team to safeguard the quality of each article we publish.
Find out more
ORIGINAL RESEARCH article
Front. Pharmacol., 06 October 2022
Sec. Predictive Toxicology
Volume 13 - 2022 | https://doi.org/10.3389/fphar.2022.1010817
This article is part of the Research TopicToxicity Mechanisms, Exposure, Toxicokinetic and Risk Assessment Aspects of Metals, Toxic for Animals and Humans, Volume IIView all 10 articles
Cadmium (Cd) is a widespread heavy metal known as a toxic environmental pollutant. Cd exposure is threatening due to its bioaccumulation trait in living systems that exceeds 35 years without a beneficial biological role. Acute exposure to high Cd doses was reported to impact adipose tissue (AT) function adversely. The main aim of this study is to investigate the effect of low-dose chronic Cd exposure on the genes involved in adipose tissue (AT) functions. Adult male Sprague-Dawley rats were exposed to a low Cd dose (15 mg/kg B.W./day) for 10 weeks. Then, three AT depots-subcutaneous AT (SUB-AT), abdominal AT (AB-AT), and retroperitoneal AT (REtrop-AT) were excised for Cd accumulation measures and gene expression analysis. Adiponectin and leptin gene expression levels were investigated as markers for adipocytes function and homeostasis. Our results showed that Cd accumulated in all the tested adipose depots, but SUB-AT was found to be the depot to most accumulate Cd. Also, it was exhibited that chronic exposure to low Cd doses altered the gene expression of adipocytokines. The levels of adiponectin and leptin mRNA expression were downregulated in all tested AT-depots after Cd exposure. The significant adverse effect on SUB-AT compared to other depots indicates different responses based on AT depots location toward Cd exposure. Collectively, these results suggest a toxic effect of Cd that influenced adipocyte function.
Environmental pollution is increasing due to the extensive urbanization and expanded industries. Cadmium (Cd) is classified as a toxic environmental pollutant that is listed among the top ten toxic substances by the Agency for Toxic Substances and Disease Registry (ATSDR, 2019). The recent global production of Cd reached 23,000 metric tons, highlighting the current challenge of Cd exposure worldwide (USGS, 2020). The main routes of Cd exposure are inhalation and ingestion of contaminated food and water (Andjelkovic et al., 2019; Fatima et al., 2019). Cadmium pose a toxic risk because of its prolonged biological half-life (10–30 years) with no known beneficial physiological function (Jacobo-Estrada et al., 2017; Rahimzadeh et al., 2017). Cadmium accumulates in different tissues such as liver, kidney, skeletal muscle, pancreas, and also the adipose tissue (Ficková et al., 2003; Lee et al., 2012; Buha et al., 2018).
Adipose tissue (AT) is a key organ that regulates various physiological processes such as lipid metabolism and energy homeostasis. In addition, AT is responsible for releasing factors known as adipokines that regulate appetite, energy expenditure, and fat distribution. Adipokines are involved in metabolic regulation and play an essential role in maintaining systemic functions such as inflammatory and immunological responses, vascular events, reproductive functions, appetite regulation, and insulin sensitivity (Henriques et al., 2019). Additionally, some of these secreted adipokines exert both autocrine and paracrine actions, which mainly affect the processes of AT remodeling, angiogenesis, and adipogenesis (Ordovas and Corella, 2008; Henriques et al., 2019; Attia et al., 2022). The AT secretory status depends on the changes of cellular tissue composition, including alterations in the phenotypes, numbers, and site of adipose tissue depots (Ouchi et al., 2011). There are two types of adipokines- pro-inflammatory such as leptin, monocyte chemoattractant protein 1 (MCP-1), tumor necrosis factor-α (TNF-α), interleukin 6 (IL-6) and anti-inflammatory such as adiponectin and interleukin 10 (IL-10) (Mancuso, 2016; Hui and Feng, 2018; Henriques et al., 2019).
Regarding the adipokines, leptin is an adipokine secreted by AT and was the main reason for adipose tissue recognition as an endocrine organ when first discovered in 1994 (Zhang et al., 1994). Leptin signals have an essential contribution to regulating AT metabolism, appetite, satiety, puberty, fertility, and reproductive function (Fasshauer and Blüher, 2015; Stern et al., 2016). Moreover, leptin can directly increase pro-inflammatory cytokines such as TNF-α and IL-6 in monocytes and enhance the production of chemokines like MCP-1 and IL-8 in macrophages and the lipid mediators PGE2 cysteinyl leukotrienes (Ouchi et al., 2011; Mancuso, 2016). Adiponectin is an adipokine produced exclusively by adipocytes with a high level in the blood that ranges between 3 and 30 μg/ml, and it targets different cell types (Fasshauer and Blüher, 2015; Mancuso, 2016). The metabolic properties of adiponectin are favorable since it is an anti-inflammatory adipokine that can inhibit the activation of nuclear factor kappa-light-chain-enhancer of activated B cells (NF-κB). As a result, it inhibits inflammation, reduces the expression of TNF-α and IL-6, and regulates glucose metabolism and energy homeostasis (Chandrasekar et al., 2008; Novotny et al., 2012; Vasiliauskaité-brooks et al., 2017). Studies showed that a dysregulation in the adipocyte secretion of adiponectin, leptin, resistin, and TNF-α is related to increased risk of type 2 diabetes and arteriosclerosis (Kawakami et al., 2010; Samaras et al., 2010). The crosstalk between the adipokines and cytokines under cadmium exposure were extensively reviewed by Attia et al. (2022).
White adipose tissue (WAT) is considered the main site of metabolic dysregulation in several metabolic diseases (Henriques et al., 2019). There are two main subtypes of WAT: the subcutaneous and the visceral. The latter also can be further subdivided into omental, mesenteric, perirenal, and peritoneal fat depots (Choe et al., 2016). Subcutaneous AT is located in the innermost layers of the skin and has a primary function of energy storage (Badimon and Cubedo, 2017; Henriques et al., 2019; Kahn et al., 2019). Moreover, subcutaneous AT is responsible for thermal insulation and providing a protective cushion against mechanical damage (Choe et al., 2016; Chait and den Hartigh, 2020). On the other hand, the visceral AT is located in the internal organs and is known for its high metabolic response (Badimon and Cubedo, 2017; Kahn et al., 2019). Both subcutaneous AT and visceral AT have different metabolic functions and different adipokine expression profiles.
Adipose tissue is a potential target for Cd accumulation. Recent study reported that Cd accumulates in AT of the human body with an average concentration of 12.6 µg/kg (Egger et al., 2019). Moreover, results reported by Echeverría et al. (2019) showed that the mean Cd concentration in AT of breast and waist regions was 32 and 42 µg/kg, respectively. The authors correlated this accumulation of Cd in AT with several parameters such as age, smoking, the types of food consumed, and body mass index. Data collected earlier from animal studies showed similar results. Kawakami et al. (2010) reported a correlation between Cd dose increments and elevation of Cd concentration in AT of male SIc: ICR mice. The risk of Cd accumulation in AT includes disrupting its capability to accommodate the surplus energy and produce the required adipokines for its endocrine function. Consequently, this may affect systemic homeostasis since AT occupies a large part of the whole body. Of note, few studies investigated the direct effect of Cd on AT function. Thus, this study aims to investigate the effect of chronic exposure to low dose of Cd on AT, where the pattern of adipocyte secretion will be evaluated to assess the white adipose tissue function.
The study design was established by Al-Naemi and Das (2020), and approved by the Institutional Animal Care and Use Committee (Approval# QU-IACUC 038/2017) Briefly, adult male Sprague-Dawley (SD) rats (8 weeks old) were divided into two groups, control (C) and cadmium-treated (Cd-T). The control group received standard chow and normal drinking water while cadmium treated group received standard chow and cadmium in drinking water with dose of 15 mg Cd/kg body weight as CdCl2 (BDH Chemicals, England) for 10 weeks, ad libitum. During the study, the health status of the animals were observed and recorded. After 10 weeks, the animals were anesthetized, sacrificed and adipose tissue depots: subcutaneous and visceral (abdominal, and retroperitoneal) were collected as indicated in Figure 1, frozen in liquid nitrogen and stored in the repository at −80°C. The administered dose was chosen to represent the human equivalent dose (HED) of 2.4 mg/kg and similar to the reported intake in epidemiological studies (Reagan-Shaw et al., 2008, Bernhoft, 2013; Chunhabundit, 2016; Ghosh et al., 2018).
FIGURE 1. Locations of the collected adipose tissues (Created with BioRender.com).
Sample preparation was adapted as previously published by Ishak et al. (2015). Harvested adipose tissue samples were weighed and digested in 69% of trace metal analysis grade nitric acid (VWR International) overnight at room temperature. Following digestion, samples were incubated at 60°C for 1 h, allowed to cool, and incubated in hydrogen peroxide at 60°C for 1 h. Then, samples were diluted to a final volume of 10 ml with deionized water and filtered using a 0.2 µm injection filter (GE Healthcare Life Sciences, United Kingdom) to remove any debris. Digested samples were analysed for cadmium quantification by Inductively Coupled Plasma Optical Emission Spectrometry (ICP-OES, Model: Optima 7300 DV) performed by Central Laboratories Unit, Qatar University. The limit of detection for the instrument is 0.0001 µg/g. Concentrations are represented as µg/g of tissue.
The effect of Cd on WAT was evaluated by gene expression assay. Adipose tissue previously stored at −80°C were homogenized using both liquid nitrogen and probe sonicator (QSonica 500), then total RNA was extracted from adipose tissue using TRIzol™ LS Reagent (ThermoFisher Scientific, United States; 10296010). Slight modifications were followed at the washing step, where the RNA pellet was resuspended in ice cold 75% ethanol and kept at −20°C overnight. Then, the sample was washed three times to enhance the RNA purity. Total RNA was quantified using nanophototmeter (Implen; P330). A known amount of RNA (150 ng) was reverse transcribed into cDNA using the high capacity cDNA transcription kit (Applied Biosystems, Lithuania) following the manufacturer’s instructions. Final volume of the reaction was 20 µl and stored at −20°C until the performance of Real Time-PCR gene expression assays. RT-PCR was performed using diluted cDNA (1:3) and TaqMan® Fast Advanced Master Mix (Applied Biosystems, United States) for six targets as following: adiponectin (Rn00595250_m1), leptin (Rn00565158_m1), MCP-1 (Rn00580555_m1), IL-6 (Rn01410330_m1), IL-10 (Rn01483988_g1), TNF-α (Rn01525859_g1), GAPDH (Rn01775763_g1). GAPDH was assigned as the endogenous control gene. The amplification was carried out in QuantStudio 6 flex system (Applied biosystem™). The relative quantity of gene expression was calculated using 2−∆∆Ct method. Results are presented as fold change (log2) versus the mean values of the control samples normalized against the endogenous gene.
Gene expression results were generated using 2−∆∆Ct method. Experiments were conducted in duplicate. The values are presented as means ± SEM. Data was analyzed by Kruskal-Wallis test, one-way and two-way ANOVA followed by Tukey’s multiple comparison test. Statistical analysis was performed using GraphPad Prism version 9.3 (GraphPad Software, San Diego, California United States, www.graphpad.com). p-value < 0.05 is considered a significant value.
Body weight, water, and chow intake of control and Cd-T rats were recorded weekly for 10 weeks (Table 1). Initially, no significant differences were observed in the body weight. However, at week 10 statistically significant difference was observed in the mean body weight between the groups (p-value < 0.001). The weekly water intake in both groups remained stable with slight non-significant fluctuations during the study period, with a significantly reduced intake in the Cd-T group (p-value < 0.001). The Cd-T group had a reduced initial water intake of about 52% relative to the control. At the end of the study, the difference in water intake of the Cd-T group was 11 percent lesser than the control. The initial mean chow intake of the Cd-T group was about 15% lesser than the control group, whereas the final mean chow intake of the Cd-T group was found to be like the control group. The differences in weekly chow intake were found to be statistically significant from week 1 until week 10 (p-value < 0.05).
The concentration of Cd in the adipose depots was determined by Inductively Coupled Plasma-Optical Emission Spectrometry (ICP-OES). The three adipose depots collected from Cd-T rats exhibited Cd accumulation (Table 2). The increasing order of Cd accumulation was found to be in REtrop-AT < AB-AT < SUB-AT. The differences in the mean Cd concentrations, considering each depot, was found to be highly significant in SUB-AT (p-value < 0.01) compared to AB-AT and REtrop-AT.
Adipose tissue samples from control and Cd-T rats were analyzed for gene expression to explore the effect of Cd on AT. In Figure 2, the expression patterns of adipokines (adiponectin and leptin) and chemokine (MCP-1) are illustrated in the three fat depots. Subcutaneous adipose tissue exhibited a pattern of downregulated expression for both adiponectin and leptin. Similarly, adiponectin and leptin gene expression were decreased in REtrop-AT and AB-AT. However, the fold change for both adiponectin and leptin in SUB-AT was significant by more than two folds compared to REtrop-AT and AB-AT. For chemokine MCP-1, a downregulation trend is observed in both REtrop-AT and SUB-AT with a significant value (−3.8-fold) in the latter. Conversely, a trend of up-regulation of MCP-1 was observed in AB-AT.
FIGURE 2. Effect of Cd treatment on the expression level of adiponectin (ADIPO.), leptin (LEP.) and monocyte chemoattractant protein 1 (MCP-1) in three different adipose depots of male Sprague-Dawley rats after 10 weeks Cd treatment. Gene expression results were generated using 2−∆∆Ct method and the mean fold change (log2) values of the targets mRNA expression were normalized to control samples and the endogenous gene. (significance: more than 2-fold change) and Two-way ANOVA was performed using GraphPad Prism version 9. The significant difference is represented by **p < 0.01, ***p < 0.001, (n = 6).
After observing differences in MCP-1 among the depots, we investigated the relationship between the expression level of MCP-1 and the inflammatory mediators in each depot under the condition of Cd exposure (Figure 3). Results showed an upregulation trend of TNF-α in AB-AT with no significant fold change. Similar results were observed in REtrop-AT. In contrast, in SUB-AT, TNF-α expression level showed a downregulation trend with no significant fold change. Both IL-6 and IL-10 were downregulated in all AT depots despite varying levels of change of MCP-1. Also, IL-6 was below the detection limit in SUB-AT. Put together, within each AT depot, there was no significant difference between the expression level of MCP-1 and the inflammatory mediators (TNF-α, IL-6, and IL-10). However, when comparing the effect of Cd exposure on the inflammatory mediators between depots (Figure 4), a significant difference was found between SUB-AT and other depots for TNF-α and IL-10 with a p-value < 0.001.
FIGURE 3. The expression level of MCP-1and the inflammatory mediators in three different adipose depots of male Sprague-Dawley rats. (A) Subcutaneous adipose tissue (SUB-AT), (B) Abdominal adipose tissue (AB-AT), (C) Retroperitoneal adipose tissue (REtrop-AT). Gene expression results were generated using 2−∆∆Ct method. The mean fold change (log2) values of the targets mRNA expression were normalized to control samples and the endogenous gene. significance: more than 2 fold change, compared to the control. (n = 6). nd, not detected.
FIGURE 4. The comparison between the three adipose depots in term of the expression level of the inflammatory mediators. (black) Subcutaneous adipose tissue (SUB-AT), (dotted) Abdominal adipose tissue (AB-AT), (diagonal lined) Retroperitoneal adipose tissue (REtrop-AT). Gene expression results were generated using 2−∆∆Ct method and the mean fold change (log2) values of the targets mRNA expression were normalized to control samples and the endogenous gene. Two-way ANOVA and Tukey’s post hoc test was performed using GraphPad Prism version 9, (n = 6). ***p < 0.001. nd, not detected.
The accumulative properties of Cd in living systems were found to impose adverse effects on tissues and influence their functions (Kumar and Sharma, 2019; Genchi et al., 2020). In this study, the effect of Cd on adipose tissue was investigated in three different depots of adult male SD rats. Our results showed that Cd exposure caused a significant decrease in rats’ body weight. The reduction of the body weight agrees with former studies that recorded Cd exposure caused a downregulation in the body weight of murine (Kawakami et al., 2010; Prabhu et al., 2020). The reduction of the body weight in the Cd-T group is suggestive of either losing adipocytes or dysregulated adipogenesis. Additionally, our results showed a downregulation of adiponectin and leptin mRNA expression levels in all AT depots. This downregulation of adiponectin and leptin mRNA expression level agrees with a previous finding, reporting that acute Cd exposure significantly decreased the mRNA expression level of adiponectin in mice (Kawakami et al., 2010). Another study conducted by Kawakami et al. (2013) using metallothionein-null mice reported that Cd exposure reduced the expression level of leptin and adiponectin in a dose-dependent manner. In our experimental model, we administered chronic low dose exposure of Cd which resulted in a decline in body weight and the expression level of adiponectin and leptin in Cd-T SD rats. The added value of the current work comes from the experimental design that was established to be realistic and proportional to the population exposure in real life (Das et al., 2021).
Under physiological conditions, the mature differentiated adipocytes produce adiponectin and leptin. Therefore, changes in the production of these adipokines are used as markers to assess changes in adipocytes maturation and functions. Conventionally, the expression patterns of adipokines differ between adipose depots (Lee et al., 2013). Subcutaneous adipose tissue expresses leptin and adiponectin more than visceral adipose tissue (Samaras et al., 2010; Lee et al., 2013; Mazaki-Tovi et al., 2016). This aligns with our results where the expression level of adiponectin and leptin is greater in SUB-AT than AB-AT and REtrop-AT.
However, Cd exposure caused a decrease in the gene expression of adiponectin and leptin and was significantly downregulated by more than two folds in SUB-AT (Figure 2). These changes in the mRNA expression pattern of adiponectin and leptin can be linked with the disruption of adipogenesis including adipocytes maturation. Reaching the maturation stage and achieving the healthy expansion for adipocytes are regulated by critical factors such as peroxisome proliferator-activator receptor gamma (PPARγ) and CCAAT/enhancer-binding protein alpha (C/EBPα). A previous study reported that Cd adversely affects the differentiation of preadipocytes by downregulating the expression level of PPARγ and C/EBPα in 3T3-L1 adipocytes (Lee et al., 2012). This also accords with studies by Kawakami et al. (2010) and Kawakami et al. (2013) where results showed that Cd exposure altered the expression level of the critical regulators of adipogenic differentiation, PPARγ and C/EBPα in mice models. Alterations of adiponectin and leptin expression levels indicate the abnormal adipocytes which could be attributed to disrupted maturation process.
The level of Cd accumulation differs in the three adipose depots. The Cd accumulation results (Table 2) showed that the SUB-AT has the highest amount of Cd among other depots, elucidating its vital role as a sink for storage and buffering of Cd. These accumulated Cd levels resulted in a significant downregulation of adiponectin, leptin and MCP-1 expression levels in SUB-AT compared to other depots (Figure 2). According to literature, MCP-1 production and the macrophages’ infiltration are enhanced by leptin which is a pro-inflammatory adipokine (Mancuso, 2016). However, in the current work, leptin is downregulated, which could explain the downregulated trend of MCP-1. Moreover, leptin and MCP-1 expression levels are correlated with adiposity (Bruun et al., 2005; De Victoria et al., 2009). Thus, the reduced body weight could negatively affect their expression level. A prior study investigated the inflammatory infiltration patterns in different AT depots and reported that visceral AT had more macrophages than subcutaneous AT (Jonas et al., 2015). Moreover, visceral AT is associated with inflammatory events. This could explain the upregulated trend of MCP-1 and TNF-α in AB-AT of our study (Figure 3).
The link between the abnormal adipocytes and the induction of inflammation, such as in the case of obesity, is extensively reported (Rull et al., 2010; Greevenbroek et al., 2016). This link is characterized by the presence of activated macrophages and inflammatory cytokines such as TNF-α and IL-6. These cytokines mediate the inflammatory response and are produced by macrophages. Moreover, MCP-1 is responsible for the recruitment of monocytes/macrophages and the induction of inflammatory cytokines in the states of AT abnormality. Therefore, inflammatory cytokines can be used as markers for the activity of macrophages. Additionally, macrophages’ function is determined by macrophages’ quantity, activation state, and metabolic phenotypes (Li et al., 2020). Under pathological conditions, adipose tissue macrophages (ATM) can exhibit mixed phenotypes in response to the local environment (Wang et al., 2021). Furthermore, studies found that preadipocytes have the ability to differentiate into macrophages which highlights the plasticity of AT under different conditions (Charrière et al., 2003; Thomas and Apovian, 2017). Based on the literature, Cd adversely affects the differentiation capacity into macrophages and interferes with the immune cells’ development (Wang et al., 2021). As mentioned earlier, Cd can disrupt the differentiation of preadipocytes through diminishing the transcription factor PPARγ. Another vital role of PPARγ is promoting the differentiation of macrophages into the M2 phenotype (Thomas and Apovian, 2017). Adipose tissue-derived MCP-1 was found to be associated with resident macrophages content, stromal vascular cells, and AT location under both conditions (Bruun et al., 2005). Our results show a trend of downregulation of both IL-6 and IL-10 in all AT depots (Figure 4). However, TNF-α showed a trend of upregulation in AB-AT and REtrop-AT but not in SUB-AT (Figure 4). Thus, the downregulation of both anti-inflammatory and pro-inflammatory mediators further supports the hypothesis of impaired macrophages. Likewise, the impaired differentiation capacity of preadipocytes could explain the downregulation of MCP-1 and the inflammatory cytokines.
According to the literature, former studies showed that Cd significantly reduced the phagocytic activity and decreased the inflammatory responses of murine macrophages in a dose-dependent manner (Loose et al., 1978; Jin et al., 2016). Moreover, a subtoxic Cd dose (10 µM) was found to inhibit the expression level of both IL-10 and IL-6 in murine macrophages (Riemschneider et al., 2015). Furthermore, Cox et al. (2016) proposed that Cd can induce immune dysfunction in macrophages and confirmed it with the lipopolysaccharide treatment after Cd exposure to find that macrophages’ transcription and cytokine release abilities were disrupted (Cox et al., 2016). A single-cell transcriptomic study reported that chronic Cd exposure induces phenotypic alterations in the immune system and reduces the number of monocytes when comparing the circulating immune cells with the plasma Cd level (Lu et al., 2021). Taken together with the current work, chronic Cd exposure disrupts the function of immune cells, especially macrophages.
Former studies that investigated the macrophages infiltration patterns in different AT depots reported that in normal-weight and obese conditions, visceral AT was found to have more macrophages than subcutaneous AT (Jonas et al., 2015). Subcutaneous AT acts as a metabolic sink that stores excess free fatty acids and glycerol in the form of triglycerides. Visceral AT accumulates when the capacity of SUB-AT is exceeded due to chronic stress (Ibrahim, 2010). Also, visceral AT is associated with inflammatory events. This could explain the upregulation trend of MCP-1 and TNF-α in AB-AT of our study. Although the MCP-1 expression level was found to be disrupted, the inflammatory markers were found to be downregulated which suggests that chronic exposure to low-dose Cd is not an inflammatory promotor. It is possible that Cd exposure negatively affected SUB-AT but not to the level that causes significant lipid accumulation in visceral AT.
On the other hand, Kawakami et al. (2013) reported an increment in the number of macrophages and elevation of the mRNA expression level of MCP-1 in the MT-null mice model in a dose-dependent manner. A recent study reported that acute exposure of Cd has a pro-inflammatory effect on human adipocytes (Gasser et al., 2022). An additional study reported acute Cd exposure caused an upregulation of MCP-1 mRNA expression levels in glioblastoma cell lines (Kasemsuk et al., 2020). Moreover, chronic low dose exposure to Cd was found to induce inflammatory infiltration in hepatocytes with an upregulation of MCP-1 mRNA expression level in the pubertal mice model (Li et al., 2021). However, as mentioned earlier, our study followed a different experimental design, including different conditions, tissues, and models which explain the different outcomes regarding MCP-1. This was evident in an in vitro study comparing mouse and rat macrophage cell lines which reported that mouse macrophage cell lines were more sensitive to Cd exposure than rat macrophages cell lines (García-Mendoza et al., 2019). Despite the dose, acute exposure of Cd caused an upregulation of MCP-1 mRNA expression level within the first 24h, but the longer time of Cd exposure caused downregulation of MCP-1 expression level for two rats strains (Harstad, 2002). This suggests a different response based on the duration of Cd exposure. Thus, the downregulation of MCP-1 after 10 weeks of exposure to low Cd dose in the current study is the response of ATs of the SD model to the chronic Cd exposure treatment. Of note, the expression of TNF-α was not significant, which may indicate that low-dose Cd is not a pro-inflammatory factor in AT. This is in accordance with previous studies reporting that the pro-inflammatory cytokines were downregulated in the condition of low-dose Cd (Låg et al., 2010; Messner and Bernhard, 2010).
In conclusion, the current work reported that chronic low-dose Cd exposure leads to accumulation of cadmium in AT depots and the most accumulated amounts were detected in SUB-AT. This altered the gene expression profile of adiponectin and leptin which resulted in its downregulation. The dysregulation of these vital adipokines indicates a toxic effect of Cd that influences adipocyte functions. To the best of our knowledge, the present study is the first to investigate the effect of chronic low-dose Cd on different white adipose depots. Further investigation is required to study the effect of chronic low-dose Cd on the protein level to explore whether Cd induces posttranscriptional alterations that change the functionality of AT proteins. In addition, a histopathological study is required to study the structural changes of each depot. The outcomes of these experiments could further elucidate the role that Cd plays through adipocytes in developing various metabolic complications.
The original contributions presented in the study are included in the article/supplementary materials, further inquiries can be directed to the corresponding author.
The animal study was reviewed and approved by the Institutional Animal Care and Use Committee, Qatar University.
SA: Conceptualization, writing—original draft, investigation, formal analysis, data curation, visualization. SD: Investigation, formal analysis, data curation, writing—review and editing, visualization. KV: Review, editing, methodology, supervision. HA-N: Conceptualization, methodology, data curation, writing—review and editing, visualization, funding acquisition, supervision.
This study was funded by the internal Qatar University grants QUCP-CAS-BES-15/16, and QUST-2-LARC-2020-1.
The assistance for resources provided by Dr. Muralitharan Shanmugakonar was greatly appreciated.
The authors declare that the research was conducted in the absence of any commercial or financial relationships that could be construed as a potential conflict of interest.
All claims expressed in this article are solely those of the authors and do not necessarily represent those of their affiliated organizations, or those of the publisher, the editors and the reviewers. Any product that may be evaluated in this article, or claim that may be made by its manufacturer, is not guaranteed or endorsed by the publisher.
Al-Naemi, H. A., and Das, S. C. (2020). Cadmium-induced endothelial dysfunction mediated by asymmetric dimethylarginine. Environ. Sci. Pollut. Res. 27, 16246–16253. doi:10.1007/s11356-020-08116-5
Andjelkovic, M., Buha Djordjevic, A., Antonijevic, E., Antonijevic, B., Stanic, M., Kotur-Stevuljevic, J., et al. (2019). Toxic effect of acute cadmium and lead exposure in rat blood, liver, and kidney. Int. J. Environ. Res. Public Health 16 (2), 274. doi:10.3390/ijerph16020274
ATSDR (2019). ATSDR’s substance priority list. Available at: https://www.atsdr.cdc.gov/spl/#.
Attia, S. M., Varadharajan, K., Shanmugakonar, M., Das, S. C., and Al-Naemi, H. A. (2022). Cadmium: An emerging role in adipose tissue dysfunction. Expo. Heal. 14, 171–183. doi:10.1007/s12403-021-00427-3
Badimon, L., and Cubedo, J. (2017). Adipose tissue depots and inflammation: Effects on plasticity and resident mesenchymal stem cell function. Cardiovasc. Res. 113, 1064–1073. doi:10.1093/cvr/cvx096
Bernhoft, R. A. (2013). Cadmium toxicity and treatment. Sci. World J. 2013, 7, doi:10.1155/2013/394652
Bruun, J. M., Lihn, A. S., Pedersen, S. B., and Richelsen, B. (2005). Monocyte chemoattractant protein-1 release is higher in visceral than subcutaneous human adipose tissue (AT): implication of macrophages resident in the AT. J. Clin. Endocrinol. Metab. 90, 2282–2289. doi:10.1210/jc.2004-1696
Buha, A., Matovic, V., Antonijevic, B., Bulat, Z., Curcic, M., Renieri, E. A., et al. (2018). Overview of cadmium thyroid disrupting effects and mechanisms. Int. J. Mol. Sci. 19 (5), 1501. doi:10.3390/ijms19051501
Chait, A., and den Hartigh, L. J. (2020). Adipose tissue distribution, inflammation and its metabolic consequences, including diabetes and cardiovascular disease. Front. Cardiovasc. Med. 7. doi:10.3389/fcvm.2020.00022
Chandrasekar, B., Boylston, W. H., Venkatachalam, K., Webster, N. J. G., Prabhu, S. D., and Valente, A. J. (2008). Adiponectin blocks interleukin-18-mediated endothelial cell death via APPL1-dependent AMP-activated protein kinase (AMPK) activation and IKK/NF-κB/PTEN suppression. J. Biol. Chem. 283, 24889–24898. doi:10.1074/jbc.M804236200
Charrière, G., Cousin, B., Arnaud, E., André, M., Bacou, F., Pénicaud, L., et al. (2003). Preadipocyte conversion to macrophage. J. Biol. Chem. 278, 9850–9855. doi:10.1074/jbc.M210811200
Choe, S. S., Huh, J. Y., Hwang, I. J., Kim, J. I., and Kim, J. B. (2016). Adipose tissue remodeling: Its role in energy metabolism and metabolic disorders. Front. Endocrinol. (Lausanne) 7, 1–16. doi:10.3389/fendo.2016.00030
Chunhabundit, R. (2016). Cadmium exposure and potential health risk from foods in contaminated area, Thailand. Toxicol. Res. 32, 65–72. doi:10.5487/TR.2016.32.1.065
Cox, J. N., Rahman, M. A., Bao, S., Liu, M., Wheeler, S. E., and Knoell, D. L. (2016). Cadmium attenuates the macrophage response to LPS through inhibition of the NF-κB pathway. Am. J. Physiol. Lung Cell. Mol. Physiol. 311, L754–L765. doi:10.1152/ajplung.00022.2016
Das, S. C., Varadharajan, K., Shanmugakonar, M., and Al-Naemi, H. A. (2021). Chronic cadmium exposure alters cardiac matrix metalloproteinases in the heart of sprague-dawley rat. Front. Pharmacol. 12, 663048. doi:10.3389/fphar.2021.663048
De Victoria, E. O. M., Xu, X., Koska, J., Francisco, A. M., Scalise, M., Ferrante, A. W., et al. (2009). Macrophage content in subcutaneous adipose tissue: Associations with adiposity, age, inflammatory markers, and whole-body insulin action in healthy pima Indians. Diabetes 58, 385–393. doi:10.2337/db08-0536
Echeverría, R., Vrhovnik, P., Salcedo-Bellido, I., Iribarne-Durán, L. M., Fiket, Ž., Dolenec, M., et al. (2019). Levels and determinants of adipose tissue cadmium concentrations in an adult cohort from southern Spain. Sci. Total Environ. 670, 1028–1036. doi:10.1016/j.scitotenv.2019.03.114
Egger, A. E., Grabmann, G., Gollmann-Tepeköylü, C., Pechriggl, E. J., Artner, C., Türkcan, A., et al. (2019). Chemical imaging and assessment of cadmium distribution in the human body. Metallomics 11, 2010. doi:10.1039/C9MT00178F
Fasshauer, M., and Blüher, M. (2015). Adipokines in health and disease. Trends Pharmacol. Sci. 36, 461–470. doi:10.1016/j.tips.2015.04.014
Fatima, G., Raza, A. M., Hadi, N., Nigam, N., and Mahdi, A. A. (2019). Cadmium in human diseases: It’s more than just a mere metal. Indian J. Clin. biochem. 34, 371–378. doi:10.1007/s12291-019-00839-8
Ficková, M., Eybl, V., Kotyzová, D., Mičková, V., Möstbök, S., and Brtko, J. (2003). Long lasting cadmium intake is associated with reduction of insulin receptors in rat adipocytes. BioMetals 16, 561–566. doi:10.1023/a:1023485130767
García‐Mendoza, D., Han, B., Berg, H. J. H. J., and Brink, N. W. (2019). Cell‐specific immune‐modulation of cadmium on murine macrophages and mast cell lines in vitro. J. Appl. Toxicol. 39, 992–1001. doi:10.1002/jat.3788
Gasser, M., Lenglet, S., Bararpour, N., Sajic, T., Wiskott, K., Augsburger, M., et al. (2022). Cadmium acute exposure induces metabolic and transcriptomic perturbations in human mature adipocytes. Toxicology 470, 153153. doi:10.1016/j.tox.2022.153153
Genchi, G., Sinicropi, M. S., Lauria, G., Carocci, A., and Catalano, A. (2020). The effects of cadmium toxicity. Int. J. Environ. Res. Public Health 17, 3782. doi:10.3390/ijerph17113782
Ghosh, K., N, I., and Indra, N. (2018). Cadmium treatment induces echinocytosis, DNA damage, inflammation, and apoptosis in cardiac tissue of albino Wistar rats. Environ. Toxicol. Pharmacol. 59, 43–52. doi:10.1016/J.ETAP.2018.02.009
Greevenbroek, M. M. J., Schalkwijk, C. G., and Stehouwer, C. D. A. (2016). Dysfunctional adipose tissue and low-grade inflammation in the management of the metabolic syndrome. Curr. Pract. Future AdvancesF1000Research 5, 2515. doi:10.12688/f1000research.8971.1
Harstad, E. B. (2002). Analysis of strain difference in sensitivity to cadmium-induced hepatotoxicity in fischer 344 and sprague-dawley rats. Toxicol. Sci. 67, 329–340. doi:10.1093/toxsci/67.2.329
Henriques, F., Bedard, H., and Luiz Batista Júnior, M. (2019). Adipose tissue - an update, Adipose Tissue Inflamm. Metabolic Disord., 13. doi:10.5772/intechopen.88631
Hui, H. X., and Feng, T. (2018). Adipose tissue as an endocrine organ. Vienna: Intechopen, 13. doi:10.5772/57353
Ibrahim, M. M. (2010). Subcutaneous and visceral adipose tissue: Structural and functional differences. Obes. Rev. 11, 11–18. doi:10.1111/j.1467-789X.2009.00623.x
Ishak, I., Rosli, F. D., Mohamed, J., and Mohd Ismail, M. F. (2015). Comparison of digestion methods for the determination of trace elements and heavy metals in human hair and nails. Malays. J. Med. Sci. 22, 11–20. Available at: https://pubmed.ncbi.nlm.nih.gov/28223880.
Jacobo-Estrada, T., Santoyo-Sánchez, M., Thévenod, F., and Barbier, O. (2017). Cadmium handling, toxicity and molecular targets involved during pregnancy: Lessons from experimental models. Int. J. Mol. Sci. 18, 1590. doi:10.3390/ijms18071590
Jin, Y., Liu, L., Zhang, S., He, R., Wu, Y., Chen, G., et al. (2016). Cadmium exposure to murine macrophages decreases their inflammatory responses and increases their oxidative stress. Chemosphere 144, 168–175. doi:10.1016/j.chemosphere.2015.08.084
Jonas, M. I., Kurylowicz, A., Bartoszewicz, Z., Lisik, W., Jonas, M., Wierzbicki, Z., et al. (2015). Interleukins 6 and 15 levels are higher in subcutaneous adipose tissue, but obesity is associated with their increased content in visceral fat depots. Int. J. Mol. Sci. 16, 25817–25830. doi:10.3390/ijms161025817
Kahn, C. R., Wang, G., and Lee, K. Y. (2019). Altered adipose tissue and adipocyte function in the pathogenesis of metabolic syndrome. J. Clin. Invest. 129, 3990–4000. doi:10.1172/JCI129187
Kasemsuk, T., Phuagkhaopong, S., Yubolphan, R., Rungreangplangkool, N., and Vivithanaporn, P. (2020). Cadmium induces CCL2 production in glioblastoma cells via activation of MAPK, PI3K, and PKC pathways. J. Immunotoxicol. 17, 186–193. doi:10.1080/1547691X.2020.1829211
Kawakami, T., Sugimoto, H., Furuichi, R., Kadota, Y., Inoue, M., Setsu, K., et al. (2010). Cadmium reduces adipocyte size and expression levels of adiponectin and Peg1/Mest in adipose tissue. Toxicology 267, 20–26. doi:10.1016/j.tox.2009.07.022
Kawakami, T., Nishiyama, K., Kadota, Y., Sato, M., Inoue, M., and Suzuki, S. (2013). Cadmium modulates adipocyte functions in metallothionein-null mice. Toxicol. Appl. Pharmacol. 272, 625–636. doi:10.1016/j.taap.2013.07.015
Kumar, S., and Sharma, A. (2019). Cadmium toxicity: Effects on human reproduction and fertility. Rev. Environ. Health 34, 327–338. doi:10.1515/reveh-2019-0016
Låg, M., Rodionov, D., Øvrevik, J., Bakke, O., Schwarze, P. E., and Refsnes, M. (2010). Cadmium-induced inflammatory responses in cells relevant for lung toxicity: Expression and release of cytokines in fibroblasts, epithelial cells and macrophages. Toxicol. Lett. 193, 252–260. doi:10.1016/j.toxlet.2010.01.015
Lee, E., Moon, J. Y., and Yoo, B. S. (2012). Cadmium inhibits the differentiation of 3T3-L1 preadipocyte through the C/EBPα and PPARγ pathways. Drug Chem. Toxicol. 35, 225–231. doi:10.3109/01480545.2011.591401
Lee, M., Wu, Y., and Fried, S. K. (2013). Adipose tissue heterogeneity: Implication of depot differences in adipose tissue for obesity complications. Mol. Asp. Med. 34, 1–11. doi:10.1016/j.mam.2012.10.001
Li, Y., Yun, K., and Mu, R. (2020). A Review on the biology and properties of adipose tissue macrophages involved in adipose tissue physiological and pathophysiological processes. Lipids Health Dis. 19, 164. doi:10.1186/s12944-020-01342-3
Li, X., Li, H., Cai, D., Li, P., Jin, J., Jiang, X., et al. (2021). Chronic oral exposure to cadmium causes liver inflammation by NLRP3 inflammasome activation in pubertal mice. Food Chem. Toxicol. 148, 111944. doi:10.1016/j.fct.2020.111944
Loose, L. D., Silkworth, J. B., and Simpson, D. W. (1978). Influence of cadmium on the phagocytic and microbicidal activity of murine peritoneal macrophages, pulmonary alveolar macrophages, and polymorphonuclear neutrophils. Infect. Immun. 22, 378–381. doi:10.1128/iai.22.2.378-381.1978
Lu, Y., Wu, J., Gu, W., Huang, Z., Shu, Z., Huang, M., et al. (2021). Single-cell transcriptomics uncovers phenotypic alterations in the monocytes in a Chinese population with chronic cadmium exposure. Ecotoxicol. Environ. Saf. 211, 111881. doi:10.1016/j.ecoenv.2020.111881
Mancuso, P. (2016). The role of adipokines in chronic inflammation. ImmunoTargets Ther. 5, 47. doi:10.2147/ITT.S73223
Mazaki-Tovi, M., Bolin, S. R., and Schenck, P. A. (2016). Differential secretion of adipokines from subcutaneous and visceral adipose tissue in healthy dogs: Association with body condition and response to troglitazone. Vet. J. 216, 136–141. doi:10.1016/j.tvjl.2016.08.002
Messner, B., and Bernhard, D. (2010). Cadmium and cardiovascular diseases: Cell biology, pathophysiology, and epidemiological relevance. BioMetals 23, 811–822. doi:10.1007/s10534-010-9314-4
Novotny, D., Vaverkova, H., and Karasek, D. (2012). Lipoproteins - role in health and diseases, Adiponectin A Perspective Adipose Tissue Marker Antiinflammatory Antiaterogenic Potencial, 13. Vienna: InTech. doi:10.5772/47754
Ordovas, J. M., and Corella, D. (2008). Metabolic syndrome pathophysiology: The role of adipose tissue. Kidney Int. 74. doi:10.1038/ki.2008.517
Ouchi, N., Parker, J. L., Lugus, J. J., and Walsh, K. (2011). Adipokines in inflammation and metabolic disease. Nat. Rev. Immunol. 11, 85–97. doi:10.1038/nri2921
Prabhu, R., Ribeiro, M., and Kajdacsy-Balla, A. (2020). The toxic effect of environmental cadmium on visceral adipose tissue. FASEB J. 34, 1. doi:10.1096/fasebj.2020.34.s1.06577
Rahimzadeh, M. R., Rahimzadeh, M. R., Kazemi, S., and Moghadamnia, A. A. (2017). Cadmium toxicity and treatment: An update. Casp. J. Intern. Med. 8, 135–145. doi:10.22088/cjim.8.3.135
Reagan-Shaw, S., Nihal, M., and Ahmad, N. (2008). Dose translation from animal to human studies revisited. FASEB J. 22, 659–661. doi:10.1096/fj.07-9574lsf
Riemschneider, S., Herzberg, M., and Lehmann, J. (2015). Subtoxic doses of cadmium modulate inflammatory properties of murine RAW 264.7 macrophages. Biomed. Res. Int. 2015, 295303. doi:10.1155/2015/295303
Rull, A., Camps, J., Alonso-Villaverde, C., and Joven, J. (2010). Insulin resistance, inflammation, and obesity: Role of monocyte chemoattractant protein-1 (orCCL2) in the regulation of metabolism. Mediat. Inflamm. 2010, 1–11. doi:10.1155/2010/326580
Samaras, K., Botelho, N. K., Chisholm, D. J., and Lord, R. V. (2010). Subcutaneous and visceral adipose tissue gene expression of serum adipokines that predict type 2 diabetes. Obesity 18, 884–889. doi:10.1038/oby.2009.443
Stern, J. H., Rutkowski, J. M., and Scherer, P. E. (2016). Adiponectin, leptin, and fatty acids in the maintenance of metabolic homeostasis through adipose tissue crosstalk. Cell Metab. 23, 770–784. doi:10.1016/j.cmet.2016.04.011
Thomas, D., and Apovian, C. (2017). Macrophage functions in lean and obese adipose tissue. Metab. Clin. Exp. 72, 120–143. doi:10.1016/j.metabol.2017.04.005
USGS (2020). Cadmium. Available at: https://pubs.usgs.gov/periodicals/mcs2020/mcs2020-cadmium.pdf.
Vasiliauskaité-brooks, I., Sounier, R., Rochaix, P., and Bellot, G. (2017). Europe PMC Funders Group Structural insights into adiponectin receptors suggest ceramidase activity. Nature, 544, 120–123. doi:10.1038/nature21714.Structural
Wang, Z., Sun, Y., Yao, W., Ba, Q., and Wang, H. (2021). Effects of cadmium exposure on the immune system and immunoregulation. Front. Immunol. 12, 695484. doi:10.3389/fimmu.2021.695484
Keywords: cadmium, heavy metals, environmental pollutant, adipose tissue, adipocytes dysfunction, adipokines
Citation: Attia SM, Das SC, Varadharajan K and Al-Naemi HA (2022) White adipose tissue as a target for cadmium toxicity. Front. Pharmacol. 13:1010817. doi: 10.3389/fphar.2022.1010817
Received: 03 August 2022; Accepted: 20 September 2022;
Published: 06 October 2022.
Edited by:
Alex Boye, University of Cape Coast, GhanaReviewed by:
Ashutosh Kumar, Washington University in St. Louis, United StatesCopyright © 2022 Attia, Das, Varadharajan and Al-Naemi. This is an open-access article distributed under the terms of the Creative Commons Attribution License (CC BY). The use, distribution or reproduction in other forums is permitted, provided the original author(s) and the copyright owner(s) are credited and that the original publication in this journal is cited, in accordance with accepted academic practice. No use, distribution or reproduction is permitted which does not comply with these terms.
*Correspondence: Hamda A. Al-Naemi, aGFsbmFlbWlAcXUuZWR1LnFh
Disclaimer: All claims expressed in this article are solely those of the authors and do not necessarily represent those of their affiliated organizations, or those of the publisher, the editors and the reviewers. Any product that may be evaluated in this article or claim that may be made by its manufacturer is not guaranteed or endorsed by the publisher.
Research integrity at Frontiers
Learn more about the work of our research integrity team to safeguard the quality of each article we publish.