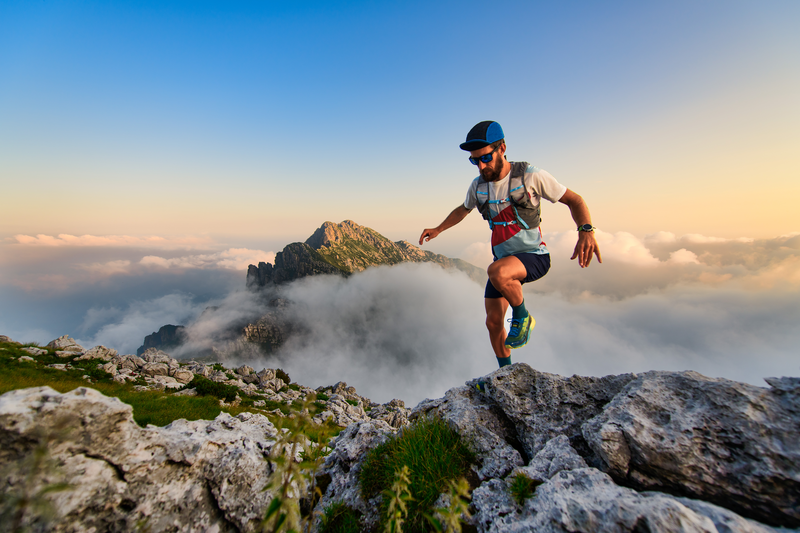
95% of researchers rate our articles as excellent or good
Learn more about the work of our research integrity team to safeguard the quality of each article we publish.
Find out more
SYSTEMATIC REVIEW article
Front. Pharmacol. , 03 November 2022
Sec. Neuropharmacology
Volume 13 - 2022 | https://doi.org/10.3389/fphar.2022.1009169
This article is part of the Research Topic Model Organisms in Neuropharmacology View all 6 articles
Background: Stroke, including ischemic stroke, intracerebral hemorrhage, and subarachnoid hemorrhage (SAH), remains a leading cause of mortality globally. Different stroke subtypes have similar detrimental effects in multiple fields of health. Previous research has shown that metformin plays a neuroprotective role in experimental animal models of stroke; however, a preclinical quantitative analysis on the ability of metformin to treat stroke is still lacking. This meta-analysis evaluates the efficacy of metformin in improving stroke prognosis in rodent models of stroke.
Methods: Relevant preclinical trials were retrieved from PubMed, EMBASE, and the Web of Science. The neurological score (NS), brain water content (BWC), infarct size, rotarod test, TUNEL, neuron quantity, microglia quantity, and p-AMPK levels were compared between a control group and a metformin group using the standardized mean difference (SMD) and corresponding confidence interval (CI). Quality was assessed with SYRCLE’s risk of bias tool.
Results: Fifteen articles published from 2010 to 2022 were included in the meta-analysis. The metformin group had statistically significant differences compared to the control group in the following aspects: NS (SMD −1.45; 95% CI −2.32, −0.58; p = 0.001), BWC (SMD −3.22; 95% CI −4.69, −1.76; p < 0.0001), infarct size (SMD −2.90; 95% CI −3.95, −1.85; p < 0.00001), rotarod test (SMD 2.55; 95% CI 1.87, 3.23; p < 0.00001), TUNEL (SMD -3.63; 95% CI −5.77, −1.48; p = 0.0009), neuron quantity (SMD 3.42; 95% CI 2.51, 4.34; p < 0.00001), microglia quantity (SMD −3.06; 95% CI -4.69, −1.44; p = 0.0002), and p-AMPK levels (SMD 2.92; 95% CI 2.02, 3.82; p < 0.00001). Furthermore, sensitivity analysis and stratified analysis were conducted for heterogeneous outcome indicators.
Conclusion: Overall, metformin treatment improves severe outcomes triggered by stroke. Despite the limitations intrinsic to animal studies, this systematic review may provide a vital reference for future high-quality preclinical trials and clinical use.
Cerebral stroke, a worldwide cerebrovascular disease (CVD) defined as arterial occlusion or rupture, initiates progressive and systemic pathophysiology, and results in functional impairment in multiple domains, such as motor, cognitive, and mental health (Sacco et al., 2013; Gu et al., 2021). Both ischemic and hemorrhagic stroke are characterized by oxidative stress, microglia polarization, neuroinflammation, and cell death, and ultimately lead to brain injury (Aronowski and Zhao, 2011; Hankey, 2014, 2017). People have long searched for effective therapeutic agents that promote recovery in stroke patients (Kuriakose and Xiao, 2020). However, most drugs proven to be therapeutic in preclinical studies have failed in clinical trials (Tao et al., 2020).
Metformin, derived from the plant Galega officinalis, has been widely used as a first-line treatment for type 2 diabetes (T2D) for over 60 years (Flory and Lipska, 2019). Many non-diabetic studies have reported that metformin delays tumor progression, slows aging, attenuates lung fibrosis, and reduces the risk of cardiovascular events (Rena et al., 2017; Farkhondeh et al., 2021). In recent years, metformin has been shown to exert a neuroprotective effect in middle cerebral artery occlusion (MCAO), ICH, and SAH animal models (Zeng et al., 2019; Lin et al., 2021; Zhang et al., 2022). Nevertheless, the overall efficacy of metformin in stroke prevention and recovery has been difficult to be evaluated due to methodological differences between studies.
To date, no systematic review or meta-analysis has been performed to synthesize the evidence from preclinical studies related to the effects of metformin in stroke or to assess their quality. Therefore, the purpose of this meta-analysis was to evaluate whether metformin could attenuate brain injury caused by stroke.
A search was conducted by following the Preferred Reporting Items for Systematic Reviews and Meta-Analysis (PRISMA) guidelines (Moher et al., 2009). Preclinical trials related to the neuropharmacological efficacy of metformin for stroke in rodent models were retrieved from PubMed, EMBASE, and the Web of Science until 12 May 2022, with the publication language being restricted to English. The search was designed using the terms “[Metformin (Title/Abstract)] AND [Cerebral Hemorrhage (Title/Abstract) OR Ischemic Stroke (Title/Abstract) OR Subarachnoid Hemorrhage (Title/Abstract)],” as shown in Supplementary File S1.
Articles were screened on the basis of the PICOS principle (population, intervention, control, outcome, and study design).
Research articles were included according to the following criteria: 1) stroke animal model, 2) includes a metformin experimental group, 3) includes a control group with placebo, 4) the effectiveness of metformin on stroke could be measured in the animal model, 5) findings were expressed or could be converted to the mean and standard deviation, and 6) other criteria: published in English.
Research articles were excluded according to the following criteria: 1) review articles, letters, and case reports; 2) repeated publications and abstracts without full text; 3) studies that did not report the number of animals in each group; and 4) animals with other diseases.
Data were extracted from a full-text article of each study by two reviewers (Fu and Tang), respectively, and any controversies were resolved by discussion with a third reviewer (Che). The following items were included: 1) the first author and publication year; 2) animal stroke models, including species, gender, age, and modeling; 3) anesthetic drugs and modality; 4) metformin administration time point and route, and metformin initial and total dosage; 5) time and outcome of measurement; and 6) methodological quality score. In the case that a study contained multiple experimental groups with different dosage and assessment times, each experimental group was independently considered as one comparison. The mean value and standard deviation were calculated statistically for continuous variables. GetData Graph Digitizer version 2.25 (http://getdata-graph-digitizer.com/) was used to obtain data from graphs. In addition, we calculated the standard deviation by multiplying the reported standard error of the mean (SEM) by the square root of the group.
SYRCLE’s risk of bias tool was used for quality assessment of all included studies by two independent reviewers (Fu and Tang) (Hooijmans et al., 2014). Disagreements were resolved by discussion with a third reviewer (Che).
Outcomes included NS, BWC, infarct size, rotarod test, TUNEL, neuron quantity, microglia quantity, and p-AMPK levels. Review Manager (RevMan) 5.4 software (Cochrane Library, London, United Kingdom) and STATA 12.0 software (StataCorp, College Station, TX, United States) were used to analyze the data collected from the studies for meta-analysis. The SMD was used to describe the differences in the effect of metformin on stroke between the treatment group and the control group (Zeng et al., 2021). The heterogeneity among included studies was evaluated using the I-squared (I2) statistic. For I2 < 50% and I2 ≥ 50%, the fixed-effects models and the random-effects models were employed, respectively (DerSimonian and Laird, 1986; Vetter, 2019). Sensitivity analysis was used to identify the source of heterogeneity (Zeng et al., 2021). A stratified analysis was conducted to clarify the influence of the methodological differences, including animal model background, anesthetic agents and route, stroke model, metformin treatment time point, and measurement time. Publication bias was checked by a funnel plot, and asymmetry was estimated by Egger’s test and the trim-and-fill method (Egger et al., 1997). p < 0.05 was considered statistically significant.
The flow diagram showing the screening process is presented in Figure 1. A total of 283 articles (19 in PubMed, two in EMBASE, and 262 in the Web of Science) were retrieved. Sixteen duplicate studies were removed. Subsequently, 230 studies were excluded due to lack of relevance. The full text of 27 studies was recorded. Twelve studies included animal models with comorbidities and were thus excluded. Finally, fifteen articles published from 2010 to 2022 were included (Li et al., 2010; Jin et al., 2014; Liu et al., 2014a; Liu et al., 2014b; Sarkaki et al., 2015; Zhu et al., 2015; Deng et al., 2016; Ge et al., 2017; Qi et al., 2017; Zeng et al., 2019; Lin et al., 2021; Zemgulyte et al., 2021; Jin et al., 2022; Liu et al., 2022; and Zhang et al., 2022).
FIGURE 1. PRISMA flow diagram for review and selection process of studies included in meta-analysis of metformin in rodent models of stroke.
The detailed characteristics of the included studies are presented in Table 1. All studies used adult male animals. The animal models were established in C57/BL6 mice (n = 5), Sprague–Dawley (SD) rats (n = 5), Wistar rats (n = 2), and CD-1 mice (n = 3). Anesthetic drugs included chloral hydrate (n = 5), sodium pentobarbital (n = 5), isoflurane (n = 3), and ketamine (n = 2). In addition to inhaling isoflurane, chloral hydrate, sodium pentobarbital, and ketamine were all administered by intraperitoneal injection (i.p.). Furthermore, MCAO (n = 11), ICH (n = 2), and SAH (n = 2) were chosen stroke models. The timing of metformin administration was divided into before MCAO (n = 3), after MCAO (n = 8), after SAH (n = 2), after ICH (n = 1), and before and after ICH (n = 1). Animals were subjected to i.p. (n = 10) and intragastric administration (i.g.) (n = 5) of metformin. The initial dosage of metformin was 10–200 mg/kg, and the most frequent dosage was 200 mg/kg (n = 6). The total dosage of metformin was 10–2,800 mg/kg.
The details of study quality are presented in Table 2. High and low scores represent high quality and low quality in the methodology, respectively. Most of the included studies scored between 2 to 8. All studies described analogous baseline characteristics, selecting outcome recording and free of counting sample sizes. Eleven studies reported the stochastic distribution. Two studies described the distribution concealment and blinding methods. Three studies described the blinded evaluation of results. In addition, there were seven studies that reported random collection of outcome measures, and there were three studies with other analysis bias.
Metformin positively affected NS outcomes by an SMD of −1.45 (95% CI: −2.32, −0.58; p = 0.001, 10 studies, 26 comparisons; Figure 2A), with statistically significant heterogeneity (I2 = 89%; p < 0.00001). Treatment with metformin reduced BWC by an SMD of −3.22 (95% CI: −4.69, −1.76; p < 0.0001, 6 studies; Figure 2B), with statistically significant heterogeneity (I2 = 69%; p = 0.007). Metformin also reduced the infarct size by an SMD of −2.90 (95% CI: −3.95, −1.85; p < 0.00001, 8 studies, 13 comparisons; Figure 2C), with statistically significant heterogeneity (I2 = 69%; p = 0.0001). Furthermore, metformin improved rotarod test results by an SMD of 2.55 (95% CI: 1.87, 3.23; p < 0.00001, 4 studies, 12 comparisons; Figure 2D), with statistically significant heterogeneity (I2 = 61%; p = 0.003). Metformin administration decreased TUNEL-positive cells by an SMD of -3.63 (95% CI: 5.77, −1.48; p = 0.0009, 5 studies; Figure 3A), with statistically significant heterogeneity (I2 = 74%; p = 0.004). Therefore, we further performed stratified analysis based on the animal model background, anesthetic drugs and route, stroke subtype, metformin administration time point and route, outcome measurement time for NS, BWC, infarct size, rotarod test, and TUNEL.
FIGURE 2. (A) Forest plot analyzing the effect of metformin treatment of NS. (B) Forest plot analyzing the effect of metformin treatment of the brain water content. (C) Forest plot analyzing the effect of metformin treatment of the infarct size. (D) Forest plot analyzing the effect of metformin treatment of the rotarod test.
FIGURE 3. (A)Forest plot analyzing the effect of metformin treatment of TUNEL. (B) Forest plot analyzing the effect of metformin treatment of the neuron quantity. (C) Forest plot analyzing the effect of metformin treatment of the microglia quantity. (D) Forest plot analyzing the effect of metformin treatment of p-AMPK.
Metformin treatment increased the neuron quantity by an SMD of 3.42 (95% CI: 2.51, 4.34; p < 0.00001, 6 studies; Figure 3B), with a low heterogeneity (I2 = 0%; p = 0.47). Metformin treatment reduced the microglia quantity by an SMD of −3.06 (95% CI: 4.69, -1.44; p = 0.0002, 2 studies; Figure 3C), with a low heterogeneity (I2 = 0%; p = 0.79). Metformin increased p-AMPK levels by an SMD of 2.92 (95% CI: 2.02, 3.82; p < 0.00001, 8 studies, 9 comparisons; Figure 3D), with a low heterogeneity (I2 = 40%; p = 0.1). Thus, further stratified analysis was not performed.
For NS, significant differences among subgroups were found based on the animal model background (p = 0.00001), anesthetic drugs (p = 0.0004), anesthetic route (p = 0.0003), stroke subtype (p = 0.01), metformin administration timepoint (p = 0.00001), and outcome measurement time (p = 0.005). Among these, a clear difference was indicated in the therapeutic effect by the anesthetic route and outcome measurement time, and the effect size was greater with inhalation (SMD −3.72, 95% CI: −4.92, −2.53) and with outcomes measured at more than 7 days after treatment (SMD −3.31, 95% CI: −4.74, −1.88) (Table 3).
For BWC, there was no significant difference in the estimated effect size among the animal model background (p = 0.24), anesthetic route (p = 0.09), or metformin administration timepoint (p = 0.08). Significant differences among subgroups were based on anesthetic drugs (p = 0.008) and the stroke subtype (p = 0.04) (Table 4).
For infarct size, no significant difference in the estimated effect size was observed among the anesthetic drugs (p = 0.27), anesthetic route (p = 1.00), and metformin administration time point (p = 0.27). Significant differences among subgroups were found based on the animal model background (p = 0.03) and outcome measurement time (p = 0.02). Among them, there was a clear difference in the therapeutic effect by the outcome measurement time. The effect size was greater in groups with outcomes measured at more than 7 days (SMD −5.29, 95% CI: −7.39, −3.19) (Table 5).
For rotarod tests, no significant differences in the estimated effect size among the anesthetic route (p = 0.57) and outcome measurement time (p = 0.47) were observed. Significant differences among subgroups were found based on the animal model background (p = 0.0001), anesthetic drugs (p = 0.0001), stroke subtype (p = 0.007), and metformin administration time point (p = 0.007). Among these, there was a clear difference in the therapeutic effect by the animal model background, stroke subtype, and metformin administration time point. The effect size was greater in C57/BL6 mice (SMD 3.92, 95% CI 3.00, 4.84), the ICH model (SMD 4.27, 95% CI 2.87, 5.66), and before and after ICH (SMD 4.27, 95% CI 2.87, 5.66) (Table 6).
For TUNEL, no significant difference was seen in the estimated effect size among the animal model background (p = 0.07) and anesthetic route (p = 0.3). Significant differences among subgroups were found with stratification by anesthetic drugs (p = 0.02), stroke subtype (p = 0.03), and metformin administration time point (p = 0.03) (Table 7).
The robustness of our results was evaluated through a sensitivity analysis. The NS, BWC, infarct size, rotarod test, TUNEL, neuron quantity, microglia quantity, and p-AMPK levels were not significantly affected by any study for the pooled SMD (Figures 4A–H).
FIGURE 4. (A)Sensitivity analysis of metformin treatment of NS. (B) Sensitivity analysis of metformin treatment of the brain water content. (C) Sensitivity analysis of metformin treatment of the infarct size. (D) Sensitivity analysis of metformin treatment of the rotarod test. (E) Sensitivity analysis of metformin treatment of TUNEL. (F) Sensitivity analysis of metformin treatment of the neuron quantity. (G) Sensitivity analysis of metformin treatment of the microglia quantity. (H) Sensitivity analysis of metformin treatment of p-AMPK.
The publication bias analysis for outcome measures was performed with more than ten included articles. Conspicuous publication bias for the NS, infarct size, and rotarod test were suggested by visual inspection of the funnel plot (Figures 5A–C). Egger’s test confirmed the existence of publication bias in the NS (p = 0.036), infarct size % (p = 0.000), and rotarod test (p = 0.000). In this situation, the trim-and-fill analysis for NS, infarct size %, and rotarod test was conducted to estimate the results for the missing studies and recalculate effect estimates. The results of NS (SMD −1.451; 95% CI −2.321, −0.581; p = 0.000) and infarct size (SMD −2.901; 95% CI −3.951, −1.851; p = 0.000) were consistent, indicating no “missing” studies (Figures 5D,E). The result of the rotarod test (SMD 2.069; 95% CI 1.366, 2.772; p = 0.000) indicated that it had four “missing” studies (Figure 5F).
FIGURE 5. (A) Funnel plots for metformin treatment of NS. (B) Trim-and-fill analysis of metformin treatment of NS. (C) Funnel plots for metformin treatment of the infarct size. (D) Trim-and-fill analysis of metformin treatment of the infarct size. (E) Funnel plots for metformin treatment of the rotarod test. (F) Trim-and-fill analysis of metformin treatment of the rotarod test.
To the best of our knowledge, no meta-analysis has been conducted to evaluate the neuropharmacological efficacy of metformin for stroke in rodents. The present study demonstrated that metformin has a neuropharmacological effect on stroke in animals. Overall, metformin significantly decreased BWC, infarct size, positive-TUNEL cells, and microglia quantity while increasing the neuron quantity and p-AMPK levels. Furthermore, metformin improved the NS and rotarod test. Collectively, the results of this meta-analysis of preclinical studies suggest that metformin may have a potential value as a therapeutic agent that protects against the detrimental effects of clinical stroke in patients.
Stroke causes secondary pathological changes, including neuroinflammation, brain edema, and neurological function impairment. In addition, stroke decreases p-AMPK levels, which leads to neuronal loss and microglia polarization. The neuroprotective effects of increased p-AMPK have been shown in stroke studies (Lin et al., 2021; Liu et al., 2022; Zhang et al., 2022). Some researchers have found that metformin attenuates brain injury by activating the p-AMPK pathways and decreasing positive-TUNEL cells after stroke. NS, brain edema, and rotarod tests were significantly improved in a metformin treatment group compared with a stroke control group, suggesting that metformin treatment contributes to the recovery of neurological function in animals with stroke through anti-oxidative and anti-inflammation mechanisms (Li et al., 2010; Jin et al., 2014; Liu et al., 2014a; Liu et al., 2014b; Sarkaki et al., 2015; Zhu et al., 2015; Deng et al., 2016; Ge et al., 2017; Qi et al., 2017; Zeng et al., 2019; Lin et al., 2021; Zemgulyte et al., 2021; Jin et al., 2022; Liu et al., 2022; Zhang et al., 2022). Other research studies have reported that metformin could improve brain recovery through regulating microglia polarization (Jin et al., 2014b). These potential improvements in neurological function indicate that metformin could be a promising therapeutic and protective candidate for stroke in the future.
In this meta-analysis, metformin had significant neuropharmacological efficacy in reducing the BWC, infarct size, positive-TUNEL cells, and microglia quantity; in increasing the neuron quantity and p-AMPK levels; and in improving the NS and rotarod test. However, the heterogeneity of the data was statistically significant among NS, BWC, infarct size, rotarod test, and TUNEL. Thus, stratified analysis based on the animal model background, anesthetic drugs, anesthetic route, stroke subtype, metformin administration time point, and outcome measurement time was performed.
Our stratified meta-analysis of NS, infarct size, and rotarod test found that the animal model background was a source of heterogeneity. The researchers selected C57/BL6 mice, SD rats, Wistar rats, and CD-1 mice for the study. Among them, C57/BL6 mice showed the largest effect size. Therefore, C57/BL6 mice may be the best rodent model to be used for the study of stroke.
To date, no systematic review has been conducted to discuss the effects of anesthetic use in rodent models of stroke. Our stratified meta-analysis of NS, BWC, rotarod test, and TUNEL found that anesthetic drugs were a source of heterogeneity. Researchers selected choral hydrate, isoflurane, sodium pentobarbital, and ketamine for this study. Among these, choral hydrate and sodium pentobarbital were chosen most often. Generally, the effect size of choral hydrate was larger than that of sodium pentobarbital, and chloral hydrate protected animals with ischemic stroke against brain infarct and edema, as described previously (Liu et al., 2015). In the present study, choral hydrate was found to be less widely used. In addition, studies have shown that chloral hydrate can have mutagenic and carcinogenic effects in animals (Maud et al., 2014). Thus, we cannot confirm whether choral hydrate is more suitable for the rodent models of stroke. Furthermore, our stratified meta-analysis of NS found that the anesthetic route was a source of heterogeneity, and inhalation has a larger effect size, while the number of studies that used i.p. was greater. Metformin efficacy might be overestimated due to a small number of studies. Therefore, we cannot confirm whether inhalation was the best choice of the anesthetic route. Overall, future studies need to focus on normalizing the selection of the anesthetic drug and route in stroke models.
Our stratified meta-analysis of NS, BWC, rotarod test, and TUNEL found that the source of heterogeneity was related to the stroke subtype. The researchers selected MCAO, ICH, and SAH for analysis, and MCAO was the most used. In addition, NS and BWC, as the primary outcome measures of stroke, had the largest effect sizes when MACO was used. Therefore, metformin treatment may be more appropriate for the MCAO stroke subtype. In the future, more studies are needed to confirm this conclusion.
Our stratified meta-analysis of NS, rotarod test, and TUNEL found that the time point of metformin administration was a source of heterogeneity. The researchers selected before MCAO, after MCAO, after SAH, before and after ICH, and after ICH for analysis. Among these, metformin administration before and after ICH had the largest effect, despite being used in the smallest number of studies. In the clinic, treatment is generally performed after a stroke has occurred. Therefore, future studies are needed to confirm the best time point for metformin administration for stroke treatment.
Our stratified meta-analysis of NS and infarct size found that outcome measurement time was a source of heterogeneity. The analyzed studies selected less than 7 days and more than 7 days as outcome measurement time points for the present analysis. Between the time points, more than 7 days had a larger effect size, although the number of the studies was smaller. Therefore, it is unclear which is the best time to measure outcomes, and more comparisons are needed. In fact, early detection of outcome indicators is favorable for assessing the severity of stroke and starting appropriate treatment. Similarly, long-term monitoring also helps in improving our understanding of the development of stroke, and it provides more references for formulating better treatment plans.
Several limitations exist in our study. Although we searched the vast majority of influential databases, there is still a shortage in the retrieved articles due to the fact that only articles published in English were included and some studies with negative results were ignored. Thus, the effect size of metformin may be exaggerated. The quality of this study was reduced due to some degree of heterogeneity across analyzed studies. The animal model background, anesthetic drugs and route, stroke subtype, metformin administration time point, and outcome measurement time may influence the quality. The differences among subgroups may not have been significant due to the lack of sufficient data for statistics, despite performing a stratified analysis. Therefore, more sufficient evidence is needed for statistical analysis in the future. In addition, varied intervention time spans may lead to substantial heterogeneity among the studies. Overall, significant work remains to be done for the clinical translation of metformin treatment for stroke patients.
Although it has been suggested that patients with stroke on treatment with metformin have a better functional outcome in a few clinical research studies, there has been a lack of specific mechanism to prove that metformin could reduce brain damage caused by stroke (Cheng et al., 2014; Westphal et al., 2020; Kersten et al., 2022). In this review, we found metformin played a neuroprotective role through anti-oxidative and anti-inflammation mechanisms after stroke. Although this review was only conducted on preclinical studies, it reminds us that metformin may be one of the drugs used to treat stroke in the future.
Our meta-analysis revealed that metformin treatment plays a neuroprotective role and improves pathological and behavioral outcomes in rodent models of stroke. Although multiple limitations regarding animal study methodology exist, the results of our study may provide an important reference for future preclinical and clinical studies on stroke outcome recovery.
The original contributions presented in the study are included in the article/Supplementary Material; further inquiries can be directed to the corresponding author.
WF and YT were responsible for the study design and conducting the study; ZH was responsible for the language editing and critically revised the article; WF and YT were responsible for the search strategy, selection of study, extraction of data, statistical analysis, and manuscript drafting; JT and YM were responsible for the evaluation of quality; ZH and XC were responsible for the integrity of the data and the accuracy of the data analysis.
This study was supported by a grant from the National Natural Science Foundation of China (NSFC 81870927).
The authors declare that the research was conducted in the absence of any commercial or financial relationships that could be construed as a potential conflict of interest.
All claims expressed in this article are solely those of the authors and do not necessarily represent those of their affiliated organizations, or those of the publisher, the editors, and the reviewers. Any product that may be evaluated in this article, or claim that may be made by its manufacturer, is not guaranteed or endorsed by the publisher.
The Supplementary Material for this article can be found online at: https://www.frontiersin.org/articles/10.3389/fphar.2022.1009169/full#supplementary-material
Aronowski, J., and Zhao, X. (2011). Molecular pathophysiology of cerebral hemorrhage: Secondary brain injury. Stroke 42 (6), 1781–1786. doi:10.1161/STROKEAHA.110.596718
Cheng, Y. Y., Leu, H. B., Chen, T. J., Chen, C. L., Kuo, C. H., Lee, S. D., et al. (2014). Metformin-inclusive therapy reduces the risk of stroke in patients with diabetes: A 4-year follow-up study. J. Stroke Cerebrovasc. Dis. 23 (2), e99–e105. doi:10.1016/j.jstrokecerebrovasdis.2013.09.001
Deng, T., Zheng, Y.-R., Hou, W.-W., Yuan, Y., Shen, Z., Wu, X.-L., et al. (2016). Pre-stroke metformin treatment is neuroprotective involving AMPK reduction. Neurochem. Res. 41 (10), 2719–2727. doi:10.1007/s11064-016-1988-8
DerSimonian, R., and Laird, N. (1986). Meta-analysis in clinical trials. Control. Clin. Trials 7 (3), 177–188. doi:10.1016/0197-2456(86)90046-2
Egger, M., Davey Smith, G., Schneider, M., and Minder, C. (1997). Bias in meta-analysis detected by a simple, graphical test. Bmj 315 (7109), 629–634. doi:10.1136/bmj.315.7109.629
Farkhondeh, T., Amirabadizadeh, A., Aramjoo, H., Llorens, S., Roshanravan, B., Saeedi, F., et al. (2021). Impact of metformin on cancer biomarkers in non-diabetic cancer patients: A systematic review and meta-analysis of clinical trials. Curr. Oncol. 28 (2), 1412–1423. doi:10.3390/curroncol28020134
Flory, J., and Lipska, K. (2019). Metformin in 2019. JAMA 321 (19), 1926–1927. doi:10.1001/jama.2019.3805
Ge, X.-H., Zhu, G.-J., Geng, D.-Q., Zhang, H.-Z., He, J.-M., Guo, A.-Z., et al. (2017). Metformin protects the brain against ischemia/reperfusion injury through PI3K/Akt1/JNK3 signaling pathways in rats. Physiol. Behav. 170, 115–123. doi:10.1016/j.physbeh.2016.12.021
Gu, H. Q., Yang, X., Wang, C. J., Zhao, X. Q., Wang, Y. L., Liu, L. P., et al. (2021). Clinical characteristics, management, and in-hospital outcomes in patients with stroke or transient ischemic attack in China. JAMA Netw. Open 4 (8), e2120745. doi:10.1001/jamanetworkopen.2021.20745
Hankey, G. J. (2014). Secondary stroke prevention. Lancet. Neurol. 13 (2), 178–194. doi:10.1016/s1474-4422(13)70255-2
Hooijmans, C., Rovers, M., de Vries, R., Leenaars, M., Ritskes-Hoitinga, M., and Langendam, M. (2014). SYRCLE's risk of bias tool for animal studies. BMC Med. Res. Methodol. 14, 43. doi:10.1186/1471-2288-14-43
Jin, L., Jin, F., Guo, S., Liu, W., Wei, B., Fan, H., et al. (2022). Metformin inhibits NLR family pyrin domain containing 3 (NLRP)-Relevant neuroinflammation via an adenosine-5'-monophosphate-activated protein kinase (AMPK)-Dependent pathway to alleviate early brain injury after subarachnoid hemorrhage in mice. Front. Pharmacol. 13, 796616. doi:10.3389/fphar.2022.796616
Jin, Q., Cheng, J., Liu, Y., Wu, J., Wang, X., Wei, S., et al. (2014). Improvement of functional recovery by chronic metformin treatment is associated with enhanced alternative activation of microglia/macrophages and increased angiogenesis and neurogenesis following experimental stroke. Brain Behav. Immun. 40, 131–142. doi:10.1016/j.bbi.2014.03.003
Kersten, C., Knottnerus, I. L. H., Heijmans, E., Haalboom, M., Zandbergen, A. A. M., and den Hertog, H. M. (2022). Effect of metformin on outcome after acute ischemic stroke in patients with type 2 diabetes mellitus. J. Stroke Cerebrovasc. Dis. 31 (9), 106648. doi:10.1016/j.jstrokecerebrovasdis.2022.106648
Kuriakose, D., and Xiao, Z. (2020). Pathophysiology and treatment of stroke: Present status and future perspectives. Int. J. Mol. Sci. 21 (20), E7609. doi:10.3390/ijms21207609
Li, J., Benashski, S. E., Venna, V. R., and McCullough, L. D. (2010). Effects of metformin in experimental stroke. Stroke 41 (11), 2645–2652. doi:10.1161/strokeaha.110.589697
Lin, C., Yang, K., Zhang, G., and Yu, J. (2021). Metformin ameliorates neuronal necroptosis after intracerebral hemor-rhage by activating AMPK. Curr. Neurovasc. Res. 18 (3), 351–359. doi:10.2174/1567202618666210923150251
Liu, J. H., Feng, D., Zhang, Y. F., Shang, Y., Wu, Y., Li, X. F., et al. (2015). Chloral hydrate preconditioning protects against ischemic stroke via upregulating annexin A1. CNS Neurosci. Ther. 21 (9), 718–726. doi:10.1111/cns.12435
Liu, K., Li, L., Liu, Z., Li, G., Wu, Y., Jiang, X., et al. (2022). Acute administration of metformin protects against neuronal apoptosis induced by cerebral ischemia-reperfusion injury via regulation of the AMPK/CREB/BDNF pathway. Front. Pharmacol. 13, 832611. doi:10.3389/fphar.2022.832611
Liu, Y., Tang, G., Li, Y., Wang, Y., Chen, X., Gu, X., et al. (2014a). Metformin attenuates blood-brain barrier disruption in mice following middle cerebral artery occlusion. J. Neuroinflammation 11, 177. doi:10.1186/s12974-014-0177-4
Liu, Y., Tang, G., Zhang, Z., Wang, Y., and Yang, G.-Y. (2014b). Metformin promotes focal angiogenesis and neurogenesis in mice following middle cerebral artery occlusion. Neurosci. Lett. 579, 46–51. doi:10.1016/j.neulet.2014.07.006
Maud, P., Thavarak, O., Cédrick, L., Michèle, B., Vincent, B., Olivier, P., et al. (2014). Evidence for the use of isoflurane as a replacement for chloral hydrate anesthesia in experimental stroke: An ethical issue. Biomed. Res. Int. 2014, 802539. doi:10.1155/2014/802539
Moher, D., Liberati, A., Tetzlaff, J., and Altman, D. G., and PRISMA Group (2009). Preferred reporting items for systematic reviews and meta-analyses: The PRISMA statement. J. Clin. Epidemiol. 62 (10), 1006–1012. doi:10.1016/j.jclinepi.2009.06.005
Qi, B., Hu, L., Zhu, L., Shang, L., Wang, X., Liu, N., et al. (2017). Metformin attenuates neurological deficit after intracerebral hemorrhage by inhibiting apoptosis, oxidative stress and neuroinflammation in rats. Neurochem. Res. 42 (10), 2912–2920. doi:10.1007/s11064-017-2322-9
Rena, G., Hardie, D. G., and Pearson, E. R. (2017). The mechanisms of action of metformin. Diabetologia 60 (9), 1577–1585. doi:10.1007/s00125-017-4342-z
Sacco, R. L., Kasner, S. E., Broderick, J. P., Caplan, L. R., Connors, J. J., Culebras, A., et al. (2013). An updated definition of stroke for the 21st century: A statement for healthcare professionals from the American heart association/American stroke association. Stroke 44 (7), 2064–2089. doi:10.1161/STR.0b013e318296aeca
Sarkaki, A., Farbood, Y., Badavi, M., Khalaj, L., Khodagholi, F., and Ashabi, G. (2015). Metformin improves anxiety-like behaviors through AMPK-dependent regulation of autophagy following transient forebrain ischemia. Metab. Brain Dis. 30 (5), 1139–1150. doi:10.1007/s11011-015-9677-x
Tao, T., Liu, M., Chen, M., Luo, Y., Wang, C., Xu, T., et al. (2020). Natural medicine in neuroprotection for ischemic stroke: Challenges and prospective. Pharmacol. Ther. 216, 107695. doi:10.1016/j.pharmthera.2020.107695
Vetter, T. R. (2019). Systematic review and meta-analysis: Sometimes bigger is indeed better. Anesth. Analg. 128 (3), 575–583. doi:10.1213/ane.0000000000004014
Westphal, L. P., Widmer, R., Held, U., Steigmiller, K., Hametner, C., Ringleb, P., et al. (2020). Association of prestroke metformin use, stroke severity, and thrombolysis outcome. Neurology 95 (4), e362–e373. doi:10.1212/wnl.0000000000009951
Zemgulyte, G., Tanaka, S., Hide, I., Sakai, N., Pampuscenko, K., Borutaite, V., et al. (2021). Evaluation of the effectiveness of post-stroke metformin treatment using permanent middle cerebral artery occlusion in rats. Pharmaceuticals 14 (4), 312. doi:10.3390/ph14040312
Zeng, J., Zhu, L., Liu, J., Zhu, T., Xie, Z., Sun, X., et al. (2019). Metformin protects against oxidative stress injury induced by ischemia/reperfusion via regulation of the lncRNA-H19/miR-148a-3p/Rock2 Axis. Oxid. Med. Cell. Longev. 2019, 8768327. doi:10.1155/2019/8768327
Zeng, L., Zhu, Y., Hu, X., Qin, H., Tang, J., Hu, Z., et al. (2021). Efficacy of melatonin in animal models of intracerebral hemorrhage: A systematic review and meta-analysis. Aging (Albany NY) 13 (2), 3010–3030. doi:10.18632/aging.202457
Zhang, Y., Zhang, T., Li, Y., Guo, Y., Liu, B., Tian, Y., et al. (2022). Metformin attenuates early brain injury after subarachnoid hemorrhage in rats via AMPK-dependent mitophagy. Exp. Neurol. 353, 114055. doi:10.1016/j.expneurol.2022.114055
Zhu, X.-C., Jiang, T., Zhang, Q.-Q., Cao, L., Tan, M.-S., Wang, H.-F., et al. (2015). Chronic metformin preconditioning provides neuroprotection via suppression of NF-kappa B-mediated inflammatory pathway in rats with permanent cerebral ischemia. Mol. Neurobiol. 52 (1), 375–385. doi:10.1007/s12035-014-8866-7
Keywords: metformin, stroke, neuropharmacology, animal models, meta-analysis
Citation: Fu W, Tang Y, Che X, Tan J, Ma Y and He Z (2022) Neuropharmacological efficacy of metformin for stroke in rodents: A meta-analysis of preclinical trials. Front. Pharmacol. 13:1009169. doi: 10.3389/fphar.2022.1009169
Received: 01 August 2022; Accepted: 18 October 2022;
Published: 03 November 2022.
Edited by:
Mohd Farooq Shaikh, Monash University, MalaysiaReviewed by:
Marjan Talebi, Shahid Beheshti University of Medical Sciences, IranCopyright © 2022 Fu, Tang, Che, Tan, Ma and He. This is an open-access article distributed under the terms of the Creative Commons Attribution License (CC BY). The use, distribution or reproduction in other forums is permitted, provided the original author(s) and the copyright owner(s) are credited and that the original publication in this journal is cited, in accordance with accepted academic practice. No use, distribution or reproduction is permitted which does not comply with these terms.
*Correspondence: Zhaohui He, Z2Vub19oZUAxNjMuY29t
†These authors have contributed equally to this work and share first authorship
Disclaimer: All claims expressed in this article are solely those of the authors and do not necessarily represent those of their affiliated organizations, or those of the publisher, the editors and the reviewers. Any product that may be evaluated in this article or claim that may be made by its manufacturer is not guaranteed or endorsed by the publisher.
Research integrity at Frontiers
Learn more about the work of our research integrity team to safeguard the quality of each article we publish.