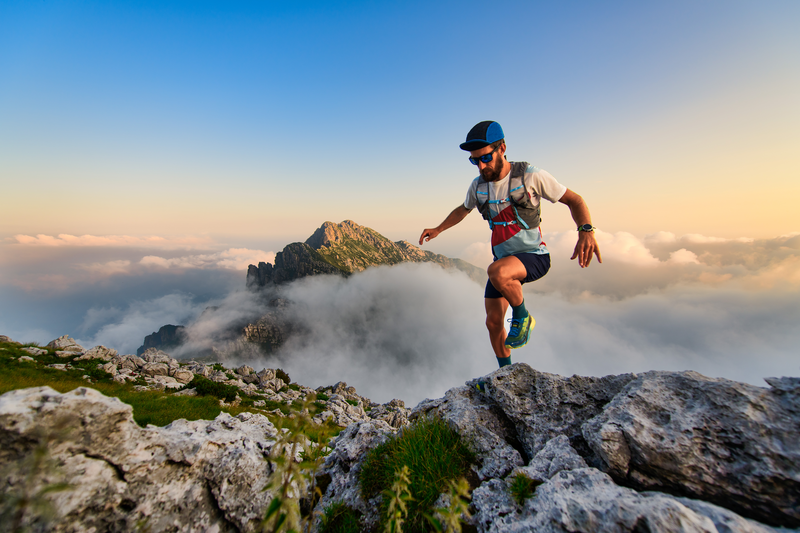
95% of researchers rate our articles as excellent or good
Learn more about the work of our research integrity team to safeguard the quality of each article we publish.
Find out more
SYSTEMATIC REVIEW article
Front. Pharmacol. , 10 January 2023
Sec. Gastrointestinal and Hepatic Pharmacology
Volume 13 - 2022 | https://doi.org/10.3389/fphar.2022.1007654
Background: Parkinson’s disease (PD) is the most common neurodegenerative disease closely related to the immune system, among whose prodromes constipation is a representative symptom. Recent Randomized Controlled Trials (RCTs) have proved that probiotics can be used to effectively treat PD constipation, but the results are inconsistent. We performed a meta-analysis to assess the efficacy and safety of probiotic therapy on Parkinson’s constipation.
Methods: Questions about the research focus were constructed based on the Participants, Intervention, Comparison and Outcomes (PICO) Criteria. We searched electronic databases such as PubMed, Web of Science, EMBASE, Scopus, EBSCO, Cochrane and Google Scholar until March 2022 for eligible literatures. Our primary endpoints were stool frequency, stool consistency, the number of laxatives uses, UPDRS-III scores and adverse events.
Results: 12 eligible studies (n = 818 patients) met the inclusion and endpoint criteria. Meta-analysis results showed that constipation symptoms were improved after probiotic treatment, including an increased stool frequency (WMD = 0.94, 95% CI:0.53 to 1.34; OR = 3.22, 95% CI:1.97–5.29), an improved stool consistency (WMD = 1.46, 95% CI:0.54–2.37), a reduced use of laxatives (WMD = −0.72, 95%CI: −1.04 to−0.41), and also a reduced Parkinson’s UPDRS-III score (WMD = −6.58, 95%CI: −12.02 to −1.14); there was no significant difference in total adverse events (OR = 0.82, 95%CI:0.39–1.72).
Conclusion: Our analysis suggests that probiotics can be used to improve the constipation and motor symptoms for patients with Parkinson’s constipation, possibly by reducing the inflammatory response and improving gut-brain axis neuron function, whose safety also proved to be good.
Parkinson’s disease (PD), which affects more than 1% of the elderly population in the world, is one of the most common neurodegenerative diseases (Nussbaum and Ellis, 2003). Conservative estimates indicate that the number of patients with PD will increase to 12 million worldwide by 2050 (Rocca, 2018). In addition to typical motor symptoms (MSs), PD is often accompanied by non-motor symptoms (NMSs). Many studies have demonstrated that not only the whole course of PD is accompanied by various NMSs, but they even appear before the MSs. This stage is characterized by NMSs, which lacks obvious signs of PD and is known as the prodromal stage of PD (Chaudhuri et al., 2006; Palma and Kaufmann, 2014).
Constipation is the most common prodromal symptom of PD and a predictor of PD onset (Stirpe et al., 2016), which seriously affects patients’ physical and mental health, generating a huge economic burden (Peery et al., 2019). Furthermore, patients with chronic constipation have a higher risk of developing PD (Abbott et al., 2001; Ueki and Otsuka, 2004). Constipation complicates the management of patients with PD, who often seek treatment in gastroenterology departments for reduced bowel movements and changes in their stool characteristics (Barboza et al., 2015; Pedrosa Carrasco et al., 2018).
Unfortunately, there is no effective neuroprotective or disease treatment to stop the progression of PD (Han et al., 2022). Conventional treatments for constipation are also often ineffective for Parkinsonian patients. The pathogenesis of constipation is also intermingled with PD. Measures to improve one of the symptoms of Parkinson’s constipation alone may exacerbate others (Coggrave et al., 2014). For example, anticholinergic drugs used to control PD symptoms can even make constipation worsened (Rodríguez-Ramallo et al., 2021), Constipation can also interfere with the absorption of anti-Parkinson’s drugs such as levodopa (Han et al., 2022). Therefore, it is crucial to identify a new intervention effective for preventing the progression of PD while relieving constipation from the perspective of the prodromal symptoms and risk factors of PD.
The mechanism of constipation in PD mainly involves intestinal neurotransmitter alterations (Wakabayashi et al., 1988; Anderson et al., 2007), intestinal barrier function (Forsyth et al., 2011) and gut microbes (Romano et al., 2021). Gut microbiota modulates the gut-brain axis interaction through the immune system, which is strongly associated with gastrointestinal symptoms that precede MSs, and is consistent with the hypothesis that PD pathology spreads from the gut to the brain (Mulak and Bonaz, 2015; Minato et al., 2017). It has been found in studies that probiotics alleviate constipation symptoms for patients with PD by regulating intestinal microecology (Gershon, 1981; Mulak and Bonaz, 2015). Therefore, the regulation of intestinal microbiota via probiotic supplementation may be a reliable treatment for Parkinson’s constipation. Therefore, to demonstrate the efficacy of probiotics in Parkinson’s constipation, this systematic review and meta-analysis need to be performed.
This meta-analysis conforms to the PRISMA guidelines (Radua, 2021). A variety of databases were used to search published studies, including PubMed, Web of Science, Scopus, Embase, EBSCO, Cochrane and Google Scholar. The last search was performed on 2 March 2022 without being limited by language. The following terms were used to search the studies: “constipation,” “functional constipation,” “dyschezia,” “colonic inertia,” “astriction,” “obstipation,” “coprostasis,” “Parkinson disease,” “Parkinson,” “secondary Parkinson’s disease,” “symptomatic Parkinson’s disease,” “Parkinsonism, symptomatic,” “secondary Parkinsonism,” “secondary vascular Parkinson’s disease,” “atherosclerotic Parkinsonism,” “probiotics,” “probiotic,” “prebiotic” and “prebiotics”. All the titles and abstracts obtained according to the search strategies were independently evaluated by two researchers to screen the studies with those that met the inclusion criteria.
Studies in line with the following inclusion criteria were enrolled for this research: 1) Participants: clinical studies on patients with a confirmed PD constipation; 2) Intervention: any kind of probiotics can be included, no restrictions on different strains/doses/treatment regimens or the form of medicine, such as tablets, powders, oil suspensions or capsules; 3) Results: indicators related to constipation and Parkinson’s were accurately reported, including stool frequency, stool consistency, the number of laxative uses and UPDRS-III scores. Studies matched to the next criteria were excluded: 1) Combined use of other drugs; 2) Studies on patients with constipation triggered by surgeries, other diseases or medications; 3) Case reports, reviews, clinical experience or trial and review articles; 4) Non-human clinical studies; 5) Similar and repeated studies. Among eligible studies, the definition of the number of stools in this study was: the number of bowel movements per week, allowing the use of laxatives; the Bristol Stool Scale was used as an evaluation standard of stool consistency, with lower scores indicating harder stools.
Two researchers (X.Li and C. Dongmei) extracted the following information separately: data characteristics extracted included study characteristics (first authors, the year of publication and investigation, design, blinding and sample size); participant characteristics (age, sex and proportion of male patients, constipation diagnostic criteria and the duration of disease); intervention characteristics (types of probiotics, the mode of administration and the duration of treatment) and clinical outcomes (stool frequency, stool consistency, the number of laxative uses, UPDRS-III scores and adverse events).
This meta-analysis of data was done using RevMan 5.4.1 software. The X2 test was used to assess statistical heterogeneity, and the degree of heterogeneity was assessed using the I2 statistic. If I2 ≥ 50%, it indicated a high heterogeneity. We used a random-effect model to evaluate variables. If I2 < 50%, we used a fixed-effect model for analysis. We used Cochrane risk-of-bias tool to assess the quality of Randomized Controlled Trials (RCTs), and minors were used to assess that of non-RCTs. Sensitivity analysis studies were performed by sequentially excluding potentially-biased studies, and subgroup analyses were performed for probiotic species. Publication biases were assessed using funnel plots.
Figure 1 illustrates the PRISMA-compliant flowchart, describing the process of inclusion and reasons for the exclusion of references. Initially 1,406 references were searched in the database. After eliminating duplicate references, there were 1,127 references left to be screened by reading the titles and abstracts, of which, 987 references were eliminated because they failed to meet the requirements for inclusion. 108 references were finally identified for a full-text evaluation, 12 were included in the meta-analysis because they met the study eligibility criteria. Finally, nine randomized controlled trials and three single-arm clinical trials were included for meta-analysis (Cassani et al., 2011; Mazza et al., 2015; Cereda et al., 2016; Miliukhina et al., 2017; Xu et al., 2018; Ibrahim et al., 2020; Li and Sun, 2020; Sun, 2020; Wang et al., 2020; Wang-Shaoying et al., 2020; Tan et al., 2021; Yan et al., 2022), including a total of 818 patients published from 2011 to 2022. The studies were carried out in Italy, Malaysia, France, Russia, China and other countries. The types of probiotics or prebiotics used included Lactobacillus, Bifidobacterium, Bacillus and FOS; probiotics were administered in the form of yogurt, tablets, powder and capsules, with the duration of treatment ranging from 2 weeks to 16 weeks. More details are in Table 1.
All RCTs included a probiotic intervention group and a placebo control group. 2 studies were assessed as having a low risk of biases, five had an unclear risk of biases, and two others were assessed as having a high risk of biases because of performance biases (see Figure 2). All the three non-RCTs were assessed for their quality using Minors (a methodological indicator for non-randomized studies) with a score of 16 (see Supplementary Appendix S1).
FIGURE 2. Evaluation of the risk of bias biases in all RCTs. RCTs in all groups were at the risk of biases, a low risk of biases (+), a high risk of biases (-) or an unknown risk of biases (?).
Continuous variables were used in eight studies (Cassani et al., 2011; Mazza et al., 2015; Cereda et al., 2016; Miliukhina et al., 2017; Ibrahim et al., 2020; Li and Sun, 2020; Sun, 2020; Tan et al., 2021) to indicate the number of bowel movements per week (Figure 3). In general, the number of stools per week in the probiotic group increased by 0.94, significantly different from that in the placebo group (MD: 0.94; 95% CI: 0.53 to 1.34), but the findings were heterogeneous (I2 = 89%). According to different species of probiotics, we conducted a subgroup analysis, which showed that the stool frequency significantly increased with both single (MD:0.97; 95% CI:0.13–1.81) and multi strains (MD:0.82; 95% CI:0.50–1.15), especially for the single strains. However, the heterogeneity of the studies included was significant (I2 = 89%). By excluding each study in turn, we performed a sensitivity analysis without finding any significant change in heterogeneity, indicating the stability of the present random-effect model.
FIGURE 3. Forest plot of Stool frequency in the probiotic intervention group versus that of the control group with MD and a 95% CI analysis using a random-effects effect model.
The overall response (treatment effective or non-responsive) to probiotic treatment was assessed in five studies (Cereda et al., 2016; Ibrahim et al., 2020; Sun, 2020; Wang et al., 2020; Tan et al., 2021) using a dichotomous approach (Figure 4), with an endpoint of achieving a mean of ≥3 BMs or an increase of ≥1 BMs per week from baseline. The meta-analysis showed that stool frequency significantly increased after a probiotic intervention, which was significant compared with the placebo group (OR:3.22; 95% CI:1.97 to 5.29; I2 = 0%).
FIGURE 4. Forest plot of Stool frequency in the probiotic intervention group versus that of the control group. Fixed-effects models were used to analyze OR and 95% CI.
Stool consistency was measured in four studies (Cassani et al., 2011; Cereda et al., 2016; Xu et al., 2018; Sun, 2020) (Figure 5), the meta-analysis showed that stool consistency was significantly improved after a probiotic intervention, which was significant compared with the placebo group (MD: 1.46; 95% CI: 0.54–2.37), but the findings were heterogeneous (I2 = 98%), and we did not find significant changes in heterogeneity after a sensitivity analysis by sequentially excluding literatures, which indicated the stability of the present random-effect model.
FIGURE 5. Forest plot of Stool consistency in the probiotic intervention group versus that of the control group, a random-effects model was used to analyze MD and 95% CI.
Changes in the number of daily laxative uses were measured in three studies (Mazza et al., 2015; Cereda et al., 2016; Tan et al., 2021) after treatment with a probiotic intervention (Figure 6). The meta-analysis on the number of daily laxative uses in the probiotic and control group using a fixed-effect model showed a reduction in the number of daily laxative uses in the probiotic group compared to the placebo group (MD: −0.72; 95% CI: −1.04 to −0.41), with no heterogeneity in the study (I2 = 0%).
FIGURE 6. Forest plot of the Number of laxatives used in the probiotic intervention group versus that of the control group, with MD and a 95% CI analysis using a random-effects model.
UPDRS-III scores were measured after a probiotic treatment in three studies (Xu et al., 2018; Ibrahim et al., 2020; Wang-Shaoying et al., 2020) (Figure 7). The meta-analysis on the probiotic and control group using a random-effect model showed a significant reduction of UPDRS-III scores in the probiotic group compared with the placebo group (MD: −6.58; 95% CI: −12.02 to −1.14), there was heterogeneity in the studies (I2 = 77%), and a sensitivity analysis suggested that the Ibrahim A’s study was the source of heterogeneity, which was reduced to 0% after excluding this article.
FIGURE 7. Forest plot of UPDRS-III scores in the probiotic intervention group versus that of the control group, with MD and a 95% CI analysis using a random-effects model.
Seven studies (Cereda et al., 2016; Xu et al., 2018; Ibrahim et al., 2020; Sun, 2020; Wang-Shaoying et al., 2020; Tan et al., 2021; Yan et al., 2022) provided information on adverse events (Figure 8), with 69% (308/448) of patients with PD constipation reporting adverse reactions after probiotic treatment, while 66% (270/410) reported adverse reactions after receiving placebo. Most of the adverse events observed in the study were abdominal distension and abdominal pains (Cereda et al., 2016; Ibrahim et al., 2020; Sun, 2020; Tan et al., 2021; Yan et al., 2022), and no serious adverse events were reported. Reduced white blood cell counts and an elevated glutamate transaminase were reported in two studies (Xu et al., 2018; Wang-Shaoying et al., 2020). However, there was no significant difference in the total number of adverse events between the probiotic and placebo group (OR:0.82; 95% CI:0.39–1.72).
FIGURE 8. Forest plot of adverse effects in the probiotic intervention group versus that of the control group, with OR and a 95% CI analyzed analysis using a fixed-effects model.
Funnel plots were used to qualitatively assess publication biases, which were overall symmetrical (Figure 9). No evidence of an obvious asymmetry was shown. Therefore, the results of this meta-analysis were considered stable and reliable.
Our study has demonstrated that probiotics may alleviate Parkinson’s MSs while treating Parkinson’s constipation, in terms of constipation symptoms and UPDRS-III scores. This study provides evidences for the efficacy and safety of probiotic supplementation in the treatment of Parkinson’s constipation as well as a new thinking on the prevention of PD's pathological development. Stool frequency, stool consistency and the number of laxatives uses are all key variables for the evaluation of constipation treatment (Saad et al., 2010). We found that stool frequency and stool consistency increased after probiotic administration, while the number of laxatives uses decreased, which were consistent with the findings of previous studies (Huang and Hu, 2017; Zhang et al., 2020). Notably, it was found in this study that the UPDRS-III scores also decreased significantly after probiotic administration (MD: −6.58; 95% CI: −12.02 to −1.14). The UPDRS-III score is a representative variable representing the severity of MSs among patients with PD, suggesting that the motor function of patients with PD is also improved after an oral probiotic treatment. It is a novel finding relative to previous studies (Xiang et al., 2022), which indicates that through probiotic therapy, constipation symptoms can not only be relieved, but also may be effective in preventing the progression of PD.
Considering that the number of probiotic species greatly influences the therapeutic effect, we performed a subgroup analysis (see Figure 3). It was found that single strains promoted a greater increase in stool frequency compared to multiple strains (MD:0.94; 95% CI:0.53–1.34). Notably, although many studies have been focused on comparing the efficacy of multiple strains with that of a single strain, the results are quite different (Chapman et al., 2013; Wilkins and Sequoia, 2017). A study showed that in most cases, multi-strain probiotics were less effective than single-strain ones (McFarland, 2021). Stronger and more in-depth research is needed in this area.
Our study has demonstrated that probiotics may alleviate Parkinson’s MSs while treating Parkinson’s constipation, in terms of constipation symptoms and UPDRS-III scores.
A typical pathological feature of PD is the aggregation of alpha-synuclein (α-syn) within the central nervous system (CNS). As α-syn progresses, the main symptoms of PD gradually appear, including premotor symptoms, sleep and motor disturbances and cognitive as well as emotional problems (Braak et al., 2003; Fahn, 2003). However, α-syn also begins in the submucosa from the enteric nervous system (ENS), and then travels retrogradely through the gut-brain axis to the CNS, thus causing MSs of PD. Constipation is one of the most common NMSs of PD, with a prevalence of 24.6%–63% among patients with PD (Stocchi and Torti, 2017). This is mainly due to the dysfunction of colonic motility in patients with PD, and many factors may contribute to alterations in colonic transit (Figure 10), including the following factors.
Usually, gastrointestinal motility disorders in PD are mainly caused by ENS and vagal abnormalities (Quigley, 1996). The large number of sensory and motor neurons contained in the ENS control the contraction of the gastrointestinal tract (GIT) muscles and the movement of transmucosal fluid (Furness, 2012). The changes in intestinal neurons and neurotransmitters play an important role in the pathogenesis of PD constipation (Wakabayashi et al., 1988; Anderson et al., 2007). The number of neurons decreases with the accumulation of α-syn in ENS, which may lead to the dysregulation of intestinal fluid secretion and neurotransmitter function, thus further impeding intestinal motility and promoting constipation (Lebouvier et al., 2010). In addition, the aggregation of α-syn causes vagal atrophy, which affects the role of vagus nerves in promoting digestive enzymes, hormone secretion and smooth muscle bowel motility meanwhile also inhibiting gastrointestinal motility, leading to the development of constipation (Del Tredici and Braak, 2016).
PD is associated with the inflammation of GIT, and a significant increase has been found in studies on the expression of pro-inflammatory cytokines in colonic specimens from patients with PD (Devos et al., 2013). A reduced level of short-chain fatty acids (SCFAs) in the intestine of patients with PD leads to an impaired intestinal barrier and an accelerated ENS neuroinflammation (Hirayama and Ohno, 2021). At the same time, the induced inflammatory mediators promote the infiltration of neutrophils and macrophages into the smooth muscle layer as well as the production of nitric oxide (NO), resulting in a weakened contraction of intestinal smooth muscles while promoting the development of constipation (Türler et al., 2006).
Patients with PD are prone to intestinal flora dysbiosis, Unger et al. found that the bacterial phylum Bacteroidetes and the bacterial family Prevotellaceae were reduced, meanwhile Enterobacteriaceae was more abundant in fecal samples from patients with PD (Unger et al., 2016). The dysbiosis of intestinal microecology leads to a significant decrease in the concentration of SCFAs, the products of bacterial fermentation in intestines, which affects the development of constipation by influencing the energy supply of colonocytes (Kaiko et al., 2016), the intestinal barrier (Kelly et al., 2015), the inflammatory response (Inan et al., 2000) and neuronal functions (Vicentini et al., 2021). Probiotics affect intestinal luminal pH, mucosal absorption and secretion as well as colonic motility by altering the intestinal microenvironment (Quigley and Spiller, 2016).
Anti-PD drug use is another cause of constipation, and a significant increase has been found in studies on the incidence of constipation among patients with PD treated with levodopa, possibly due to the delayed gastric emptying triggered by levodopa (Pagano et al., 2015; Bestetti et al., 2017). Other anti-PD drugs, such as ropinirole, bromocriptine and piribedil, can promote an increased incidence of constipation (Li et al., 2017). In the later stages of disease progression, patients with PD move slowly, who are even bedridden for long periods of time, resulting in a low colon motility and symptoms including a prolonged defecation as well as difficulties in passing stools. In addition, the dehydration that older patients with PD are prone to causes the release of pressin and aldosterone, which leads to an excessive absorption of water and salt in the colons, resulting in constipation (Read et al., 1995; Thiyagalingam et al., 2021).
The regulation of intestinal flora plays an important role in the development and treatment of PD constipation. Intestinal flora consists of a variety of bacteria in the GIT that live in symbiosis with the human host. Most species of gut flora belong to phyla Firmicutes, including Clostridium coccoides, Clostridium leptin and lactobacillus, as well as the Bacteroides phylum, including Bacteroides and Prevotella (Qin et al., 2010). With the development of macrogenomics, the association between alterations in gut flora and disease states is becoming clearer. Clinical studies have also shown that the number of probiotics in the stool of patients with constipation is significantly reduced. Supplementing probiotics is an effective way to relieve constipation and intestinal flora imbalance (Dimidi et al., 2017). In another randomized controlled trial, stool specimens from elderly patients were examined before and after probiotic administration, through which a significant increase in B. breve, B. longum and B. adolescentis was observed after a probiotic intervention (Kondo et al., 2013). This provides an additional rationale for treating constipation with probiotics through the modulation of intestinal microbiota. Additionally, gut microbes can influence brain functions through the gut because of the bidirectional communication in the gut-brain axis, which affects the progression of PD. The gut-brain axis consists of the entire gut microbiota, ENS, parasympathetic and sympathetic nervous systems, CNS, neuroendocrine connections, humoral pathways, cytokines, neuropeptides as well as signaling molecules (O'Mahony et al., 2015), which is a bidirectional neuroendocrine system that communicates between GIT and CNS. In the gut, which is in contact with the gut microbiota and the brain as well as isolated by the blood-brain barrier (BBB), microbiota acts on the CNS through many hormones and metabolites secreted by the intestinal epithelial cells (IECs) to achieve microbial effect on the gut-brain axis. We hypothesized that probiotics affected PD constipation through gut-brain axis, which were summarized as follows in three ways (Figure 11).
Intestinal flora can directly stimulate electrical signals in the ENS and the dorsal motor nucleus of vagus (DMV) by propagating signals through the vagus nerves to affect the brain center (Clarke et al., 2014), thus reducing the accumulation of α-syn and alleviating the motor deficits of patients with PD, including tremors, muscle rigidity, bradykinesia and an impaired gait (Sampson et al., 2016). Various probiotics release SCFAs by digesting fibers in the gut (Macfarlane and Macfarlane, 2012; Han et al., 2022), and SCFAs affect the energy supply for CNS cell metabolism through the brain-gut axis. e.g., butyrate and propionate are transported across the BBB into the CNS via monocarboxylate transporters (mCTs), which are then taken up through mCTs on glial cells and neurons (Pellerin, 2005). In addition, SCFAs enable signaling through G-protein-coupled free fatty acid receptors FFAR2 and FFAR3 on the intestinal epitheliums, initiate gluconeogenesis, act on the vagus nerves and DMV, affect CNS transmission processes as well as facilitate the cognitive recovery of patients with PD (Delaere et al., 2012) (Lacassagne and Kessler, 2000; Lal et al., 2001).
The accumulation of reactive oxygen species (ROS) activates neuroinflammation in microglia, which is one of the main factors for the development of PD (Franceschi and Campisi, 2014), because the high energy demand of brain neurons leads to a high sensitivity of them to ROS-induced oxidative damage. Microbiota effectively inhibits ROS accumulation, either directly or by stimulating the production of large amounts of gamma-aminobutyricacid (GABA) through many neural metabolites in the intestinal epithelia, such as Lactobacillus and Bifidobacterium strains, meanwhile promoting 5-hydroxytryptamine (5-HT) production by regulating GABA concentration. The expression of 5-HT is also important for neurological functions of the brain, which is expressed in both the ENS and CNS. SCFAs can modulate intestinal endocrine 5-HT action in the ENS (Evans et al., 2013), increasing intestinal motility to relieve constipation and further affecting α-syn misfolding in the brain-gut axis. Most (>90%) of a body’s serotonin is produced in the EC cells of the intestines (Gershon, 2013). SCFA induces the action of EC cells to express tryptophan 6-hydroxylase 1, so as to regulate 5-HT signaling (Nankova et al., 2014).
The ability of intestinal bacteria to produce ghrelin makes it a promising therapeutic target for PD. Gut hormones such as peptide YY(PYY) and cholecystokinin, produced by EC cells under the influence of microbiota, interact with ghrelin signaling to reduce ROS accumulation, protect mitochondrial integrity and therefore promote an anti-apoptotic environment meanwhile protecting against neuronal functions (Liu et al., 2009). EC cells also produce a number of neuroactive factors, including tryptophan (Trp) and histidine (His), which are neurotransmitters and neuroactive molecules that enter the circulation and cross the BBB, affecting CNS signaling. Intestinal flora can regulate the plasma concentration of Trp, a precursor of essential amino acids and pentraxin as well as a key neurotransmitter in the ENS and CNS, further stimulating the production of gut hormones in the CNS, such as gastrin and indole-3-propionic acid (IPA), which exert neuroprotective effect on the CNS. Trp and tyrosine hydroxylase gene expression regulates the synthesis of neurotransmitters such as Dopamine (DA) and 5-HT (Nankova et al., 2014), which is also very important in the progression of PD.
The gut microbiota acts as a virtual endocrine organ that produces and regulates the metabolic capacity of a wide range of compounds producing a series of endocrine signaling molecules, which affect many biochemical pathways in the systems and brain, in turn affecting the functions of distal organs and systems. For example, short-chain fatty acids are produced during carbohydrate metabolism, such as butyrate and propionate, which provide an important source of nutrition and regulate host digestion. SCFAs can readily enter the circulation from the gut and are transported across the BBB via monocarboxylate transporters (mCTs). Butyric acid, a metabolite of intestinal flora, inhibits histone deacetylase (HDAC) activity and induces forkhead box o (FOXO) to cause cell deaths through the transcriptional regulation of apoptotic genes, and its induction of autophagy genes has been shown as a key mechanism for neurological function protection (Xu et al., 2011). In addition, Parkinson’s α-Syn aggregation is reduced in a FOXO-dependent manner with butyrate, which has a direct neuroprotective potential (Koh et al., 2012; Beharry et al., 2014).
Ferulic acid (FA) is another key class of molecules produced directly by microbiota that can effectively inhibit the production and activity of ROS and is considered as a powerful scavenger of ROS (Trombino et al., 2013), which may be achieved by regulating peroxiredoxin reductase (PRX) and thioredoxin (Trx) (Patenaude et al., 2005). PRX and TRX are ubiquitous antioxidant proteins that regulate cell proliferation and apoptosis while providing neuroprotection (Sung et al., 2014). In addition, FA also inhibits the GSK3 β pathway by upregulating Akt signaling, thus preventing the expression of CRMP-2 and Casp3, thereby inhibiting apoptosis (Gim et al., 2013). Another part of gut microbiota involved in the regulation of the brain-gut axis is the stimulation of the HPA axis, during which the release of cortisol can suppress inflammatory responses and influence brain plasticity development as well as subsequent biological systems (Westfall et al., 2017).
A reason for the probiotic treatment of PD constipation is to reduce the degenerative loss of dopaminergic neurons by reducing the inflammatory response through the modulation of immune function. An inflammatory environment has been shown to increase α-Syn aggregation, which may further activate microglia upon contact, encouraging a feed-forward cascade that leads to more α-Syn aggregation and propagation as well as disease development. After intestinal microecological disorders, intestinal permeability increases, which induces the relevant immune response of pro-inflammatory cells. Inflammation is reduced with probiotics by promoting the balance of the intestinal microecological structure and regulating inflammatory cytokines through the MAPK and NF-kB pathway. It has been found in studies (Borzabadi et al., 2018; Perez Visñuk et al., 2020) that probiotics can be used to increase the production of anti-inflammatory factors and reduce the expression of inflammatory factors for patients with PD.
In addition, the GIT mucosal epithelial tissues contain a large number of antigen-presenting localized innate immune cells, such as macrophages and dendritic cells. Through such localization, immune cells are put in close proximity to the gut microbiota, invading pathogens and antigens that breach the protective epithelial barrier, allowing for an effective immunological communication between the external environment and the systemic immune system. Microbe-associated molecular patterns (MAMPs) on the surface of the microbiota such as lipoteichoicacid (LTA) and surface layer protein A (SlpA) directly stimulate receptors on immune cells, such as toll-like receptors (TLR) and intercellular cell adhesion molecule (ICAM) on dendritic cells (DCs). Anti-inflammatory responses are also propagated during this interaction through the upregulation of anti-inflammatory factors (IL-10 and IL-4) (Lavasani et al., 2010), which also inhibits pro-inflammatory cytokines (TNF-α, IL-1β and IL-6). In addition, some microbiota also directly inhibits pro-inflammatory cytokines, such as the effect of Bacillus animalis on IL-6. Histamine is a biogenic monoamine released from Lactobacillus, which can be involved in the regulation of immune cells meanwhile acting as a neurotransmitter in the brain. Histamine induces anti-inflammatory responses through its receptor H4R by activating several signaling factors including JAK-STAT, MAPK/ERK and PI3K, ultimately leading to the release of anti-inflammatory cytokines, the regulation of dendritic cell functions and the recruitment of T regulatory cells to the sites of acute inflammation (Schneider et al., 2002; Morgan et al., 2007).
In addition, with the probiotic production of SCFAs, the expression of occludin and Claudin-5 can be regulated, which can protect the BBB. Changes in the intestinal flora lead to alterations in barrier immune function meanwhile affecting the nervous systems including the ENS and EGCS. Inflammatory responses trigger microglia maturation, affecting ENS functions, the intestinal motility and α-synaptic nuclear protein misfolding (Forsyth et al., 2011; De Vadder et al., 2014). Probiotics can be used to further delay the pathological progression of PD by strengthening the intestinal barrier function, reducing immune and inflammatory responses as well as improving ENS functions by regulating EGCS homeostasis and affecting α-synaptic nuclear protein misfolding.
We used a rigorous approach to reduce publication biases in this meta-analysis. Two investigators performed literature inclusion and data extraction separately, and where a significant heterogeneity was revealed through data analysis, we used a random-effect model to reduce the possibility of overestimating the treatment effect. In addition, subgroup analysis was used for the number of probiotic species to assess treatment effect; data on adverse events was extracted to supplement probiotic safety in PD constipation, and funnel plots proved to be overall symmetric.
However, this study has some limitations. First of all, most of each study included was conducted in different countries; therefore, different genetic constitutions as well as cultural and environmental factors (eating habits, etc.) might have contributed to the heterogeneity of the studies. Secondly, the heterogeneity of some of the meta-analyses was significant, implying differences in those studies. This might be due to the different types and doses of probiotics in the studies, as well as other factors, such as physical condition and eating habits, etc. Thirdly, the number of existing studies on RCTs is limited, and the sample size of some studies is small, which affect the rigorness of the research results to a certain extent. However, due to the diversity of probiotic species and strain specificity, the efficacy and effect of various types of probiotics on constipation may be inconsistent, and further studies on the effect of different strains, doses as well as treatment durations of probiotics on constipation are needed.
For subsequent studies, we have the following suggestions. First of all, bigger sample sizes and more adequate pilot studies using standardized measurements should be accumulated to obtain fuller evidences. Secondly, more subgroup studies should be added, such as different strains and doses of probiotics.
It is found in this meta-analysis that probiotics can not only be used to treat Parkinson’s constipation symptoms, but also alleviate the MSs for patients with PD. Probiotics influence the pathological development of PD by modulating brain-gut axis functions and immune responses. However, the current randomized controlled trial study still has some limitations, thus further RCTs are warranted.
The original contributions presented in the study are included in the article/Supplementary Material, further inquiries can be directed to the corresponding author.
LX conceived the study. LX and DC extracted and analyzed the data. LX, DC, and XZ interpreted the data. The manuscript was written by LX and critically revised by CC. All authors contributed to the article and approved the submitted version.
The authors declare that the research was conducted in the absence of any commercial or financial relationships that could be construed as a potential conflict of interest.
All claims expressed in this article are solely those of the authors and do not necessarily represent those of their affiliated organizations, or those of the publisher, the editors and the reviewers. Any product that may be evaluated in this article, or claim that may be made by its manufacturer, is not guaranteed or endorsed by the publisher.
The Supplementary Material for this article can be found online at: https://www.frontiersin.org/articles/10.3389/fphar.2022.1007654/full#supplementary-material
Abbott, R. D., Petrovitch, H., White, L. R., Masaki, K. H., Tanner, C. M., Curb, J. D., et al. (2001). Frequency of bowel movements and the future risk of Parkinson's disease. Neurology 57 (3), 456–462. doi:10.1212/wnl.57.3.456
Anderson, G., Noorian, A. R., Taylor, G., Anitha, M., Bernhard, D., Srinivasan, S., et al. (2007). Loss of enteric dopaminergic neurons and associated changes in colon motility in an MPTP mouse model of Parkinson's disease. Exp. Neurol. 207 (1), 4–12. doi:10.1016/j.expneurol.2007.05.010
Barboza, J. L., Okun, M. S., and Moshiree, B. (2015). The treatment of gastroparesis, constipation and small intestinal bacterial overgrowth syndrome in patients with Parkinson's disease. Expert Opin. Pharmacother. 16 (16), 2449–2464. doi:10.1517/14656566.2015.1086747
Beharry, A. W., Sandesara, P. B., Roberts, B. M., Ferreira, L. F., Senf, S. M., and Judge, A. R. (2014). HDAC1 activates FoxO and is both sufficient and required for skeletal muscle atrophy. J. Cell Sci. 127, 1441–1453. doi:10.1242/jcs.136390
Bestetti, A., Capozza, A., Lacerenza, M., Manfredi, L., and Mancini, F. (2017). Delayed gastric emptying in advanced Parkinson disease: Correlation with therapeutic doses. Clin. Nucl. Med. 42 (2), 83–87. doi:10.1097/rlu.0000000000001470
Borzabadi, S., Oryan, S., Eidi, A., Aghadavod, E., Daneshvar Kakhaki, R., Tamtaji, O. R., et al. (2018). The effects of probiotic supplementation on gene expression related to inflammation, insulin and lipid in patients with Parkinson's disease: A randomized, double-blind, PlaceboControlled trial. Arch. Iran. Med. 21 (7), 289–295.
Braak, H., Del Tredici, K., Rüb, U., de Vos, R. A., Jansen Steur, E. N., and Braak, E. (2003). Staging of brain pathology related to sporadic Parkinson's disease. Neurobiol. Aging 24 (2), 197–211. doi:10.1016/s0197-4580(02)00065-9
Cassani, E., Privitera, G., Pezzoli, G., Pusani, C., Madio, C., Iorio, L., et al. (2011). Use of probiotics for the treatment of constipation in Parkinson's disease patients. Minerva Gastroenterol. Dietol. 57 (2), 117–121.
Cereda, E., Pacchetti, C., Bolliri, C., Cassani, E., Iorio, L., Pusani, C., et al. (2016). Double-blind, placebo-controlled trial of a fermented milk containing multiple probiotic strains and prebiotic fiber for constipation associated with Parkinson's disease. Mov. Disord. 31, S99.
Chapman, C. M., Gibson, G. R., Todd, S., and Rowland, I. (2013). Comparative in vitro inhibition of urinary tract pathogens by single- and multi-strain probiotics. Eur. J. Nutr. 52 (6), 1669–1677. doi:10.1007/s00394-013-0501-2
Chaudhuri, K. R., Martinez-Martin, P., Schapira, A. H., Stocchi, F., Sethi, K., Odin, P., et al. (2006). International multicenter pilot study of the first comprehensive self-completed nonmotor symptoms questionnaire for Parkinson's disease: The NMSQuest study. Mov. Disord. 21 (7), 916–923. doi:10.1002/mds.20844
Clarke, G., Stilling, R. M., Kennedy, P. J., Stanton, C., Cryan, J. F., and Dinan, T. G. (2014). Minireview: Gut microbiota: The neglected endocrine organ. Mol. Endocrinol. 28 (8), 1221–1238. doi:10.1210/me.2014-1108
Coggrave, M., Norton, C., and Cody, J. D. (2014). Management of faecal incontinence and constipation in adults with central neurological diseases. Cochrane Database Syst. Rev. 1, Cd002115. doi:10.1002/14651858.CD002115.pub5
De Vadder, F., Kovatcheva-Datchary, P., Goncalves, D., Vinera, J., Zitoun, C., Duchampt, A., et al. (2014). Microbiota-generated metabolites promote metabolic benefits via gut-brain neural circuits. Cell 156 (1-2), 84–96. doi:10.1016/j.cell.2013.12.016
Del Tredici, K., and Braak, H. (2016). Review: Sporadic Parkinson's disease: Development and distribution of α-synuclein pathology. Neuropathol. Appl. Neurobiol. 42 (1), 33–50. doi:10.1111/nan.12298
Delaere, F., Duchampt, A., Mounien, L., Seyer, P., Duraffourd, C., Zitoun, C., et al. (2012). The role of sodium-coupled glucose co-transporter 3 in the satiety effect of portal glucose sensing. Mol. Metab. 2 (1), 47–53. doi:10.1016/j.molmet.2012.11.003
Devos, D., Lebouvier, T., Lardeux, B., Biraud, M., Rouaud, T., Pouclet, H., et al. (2013). Colonic inflammation in Parkinson's disease. Neurobiol. Dis. 50, 42–48. doi:10.1016/j.nbd.2012.09.007
Dimidi, E., Christodoulides, S., Scott, S. M., and Whelan, K. (2017). Mechanisms of action of probiotics and the gastrointestinal microbiota on gut motility and constipation. Adv. Nutr. 8 (3), 484–494. doi:10.3945/an.116.014407
Evans, J. M., Morris, L. S., and Marchesi, J. R. (2013). The gut microbiome: The role of a virtual organ in the endocrinology of the host. J. Endocrinol. 218 (3), R37–R47. doi:10.1530/joe-13-0131
Fahn, S. (2003). Description of Parkinson's disease as a clinical syndrome. Ann. N. Y. Acad. Sci. 991, 1–14. doi:10.1111/j.1749-6632.2003.tb07458.x
Forsyth, C. B., Shannon, K. M., Kordower, J. H., Voigt, R. M., Shaikh, M., Jaglin, J. A., et al. (2011). Increased intestinal permeability correlates with sigmoid mucosa alpha-synuclein staining and endotoxin exposure markers in early Parkinson's disease. PLoS One 6 (12), e28032. doi:10.1371/journal.pone.0028032
Franceschi, C., and Campisi, J. (2014). Chronic inflammation (inflammaging) and its potential contribution to age-associated diseases. J. Gerontol. A Biol. Sci. Med. Sci. 69, S4–S9. doi:10.1093/gerona/glu057
Furness, J. B. (2012). The enteric nervous system and neurogastroenterology. Nat. Rev. Gastroenterol. Hepatol. 9 (5), 286–294. doi:10.1038/nrgastro.2012.32
Gershon, M. D. (2013). 5-Hydroxytryptamine (serotonin) in the gastrointestinal tract. Curr. Opin. Endocrinol. Diabetes Obes. 20 (1), 14–21. doi:10.1097/MED.0b013e32835bc703
Gershon, M. D. (1981). The enteric nervous system. Annu. Rev. Neurosci. 4, 227–272. doi:10.1146/annurev.ne.04.030181.001303
Gim, S. A., Sung, J. H., Shah, F. A., Kim, M. O., and Koh, P. O. (2013). Ferulic acid regulates the AKT/GSK-3β/CRMP-2 signaling pathway in a middle cerebral artery occlusion animal model. Lab. Anim. Res. 29 (2), 63–69. doi:10.5625/lar.2013.29.2.63
Han, M. N., Finkelstein, D. I., McQuade, R. M., and Diwakarla, S. (2022). Gastrointestinal dysfunction in Parkinson's disease: Current and potential therapeutics. J. Pers. Med. 12 (2), 144. doi:10.3390/jpm12020144
Hirayama, M., and Ohno, K. (2021). Parkinson's disease and gut microbiota. Ann. Nutr. Metab. 77, 28–35. doi:10.1159/000518147
Huang, R., and Hu, J. (2017). Positive effect of probiotics on constipation in children: A systematic review and meta-analysis of six randomized controlled trials. Front. Cell Infect. Microbiol. 7, 153. doi:10.3389/fcimb.2017.00153
Ibrahim, A., Raja Ali, R. A., Abdul Manaf, M. R., Ahmad, N., Tajurruddin, F. W., Qin, W. Z., et al. (2020). Multi-strain probiotics (hexbio) containing mcp bcmc strains improved constipation and gut motility in Parkinson’s disease: A randomised controlled trial. PLoS ONE 15, e0244680. doi:10.1371/journal.pone.0244680
Inan, M. S., Rasoulpour, R. J., Yin, L., Hubbard, A. K., Rosenberg, D. W., and Giardina, C. (2000). The luminal short-chain fatty acid butyrate modulates NF-kappaB activity in a human colonic epithelial cell line. Gastroenterology 118 (4), 724–734. doi:10.1016/s0016-5085(00)70142-9
Kaiko, G. E., Ryu, S. H., Koues, O. I., Collins, P. L., Solnica-Krezel, L., Pearce, E. J., et al. (2016). The colonic crypt protects stem cells from microbiota-derived metabolites. Cell 165 (7), 1137–1720. doi:10.1016/j.cell.2016.10.034
Kelly, C. J., Zheng, L., Campbell, E. L., Saeedi, B., Scholz, C. C., Bayless, A. J., et al. (2015). Crosstalk between microbiota-derived short-chain fatty acids and intestinal epithelial HIF augments tissue barrier function. Cell Host Microbe 17 (5), 662–671. doi:10.1016/j.chom.2015.03.005
Koh, H., Kim, H., Kim, M. J., Park, J., Lee, H. J., and Chung, J. (2012). Silent information regulator 2 (Sir2) and Forkhead box O (FOXO) complement mitochondrial dysfunction and dopaminergic neuron loss in Drosophila PTEN-induced kinase 1 (PINK1) null mutant. J. Biol. Chem. 287 (16), 12750–12758. doi:10.1074/jbc.M111.337907
Kondo, J., Xiao, J. Z., Shirahata, A., Baba, M., Abe, A., Ogawa, K., et al. (2013). Modulatory effects of Bifidobacterium longum BB536 on defecation in elderly patients receiving enteral feeding. World J. Gastroenterol. 19 (14), 2162–2170. doi:10.3748/wjg.v19.i14.2162
Lacassagne, O., and Kessler, J. P. (2000). Cellular and subcellular distribution of the amino-3-hydroxy-5-methyl-4-isoxazole propionate receptor subunit GluR2 in the rat dorsal vagal complex. Neuroscience 99 (3), 557–563. doi:10.1016/s0306-4522(00)00204-9
Lal, S., Kirkup, A. J., Brunsden, A. M., Thompson, D. G., and Grundy, D. (2001). Vagal afferent responses to fatty acids of different chain length in the rat. Am. J. Physiol. Gastrointest. Liver Physiol. 281 (4), G907–G915. doi:10.1152/ajpgi.2001.281.4.G907
Lavasani, S., Dzhambazov, B., Nouri, M., Fåk, F., Buske, S., Molin, G., et al. (2010). A novel probiotic mixture exerts a therapeutic effect on experimental autoimmune encephalomyelitis mediated by IL-10 producing regulatory T cells. PLoS One 5 (2), e9009. doi:10.1371/journal.pone.0009009
Lebouvier, T., Neunlist, M., Bruley des Varannes, S., Coron, E., Drouard, A., N'Guyen, J. M., et al. (2010). Colonic biopsies to assess the neuropathology of Parkinson's disease and its relationship with symptoms. PLoS One 5 (9), e12728. doi:10.1371/journal.pone.0012728
Li, B. D., Bi, Z. Y., Liu, J. F., Si, W. J., Shi, Q. Q., Xue, L. P., et al. (2017). Adverse effects produced by different drugs used in the treatment of Parkinson's disease: A mixed treatment comparison. CNS Neurosci. Ther. 23 (10), 827–842. doi:10.1111/cns.12727
Li, F., and Sun, S.-n. (2020). Effects of Bifidobacterium lactis on improving anxiety and depressive symptoms in patients with Parkinson's disease. J. Shenyang Pharm. Univ. 37 (04), 344–349. doi:10.14066/j.cnki.cn21-1349/r.2020.04.009
Liu, Y., Chen, L., Xu, X., Vicaut, E., and Sercombe, R. (2009). Both ischemic preconditioning and ghrelin administration protect hippocampus from ischemia/reperfusion and upregulate uncoupling protein-2. BMC Physiol. 9, 17. doi:10.1186/1472-6793-9-17
Macfarlane, G. T., and Macfarlane, S. (2012). Bacteria, colonic fermentation, and gastrointestinal health. J. AOAC Int. 95 (1), 50–60. doi:10.5740/jaoacint.sge_macfarlane
Mazza, S., Imondi, C., Rao, M., Sorpresi, F., Di Sarno, G., Stocchi, F., et al. (2015). Aleman in treatment of constipation in Parkinson's disease. Neurol. Sci. 36 (2), S216–S217. doi:10.1007/s10072-015-2346-3
McFarland, L. V. (2021). Efficacy of single-strain probiotics versus multi-strain mixtures: Systematic review of strain and disease specificity. Dig. Dis. Sci. 66 (3), 694–704. doi:10.1007/s10620-020-06244-z
Miliukhina, I., Ermolenko, E., Ivanova, A., Kotyleva, M., and Suvorov, A. (2017). Probiotic correction of intestinal dysbiosis in Parkinson's disease. Mov. Disord. 32, 68–69. doi:10.1002/mds.27087
Minato, T., Maeda, T., Fujisawa, Y., Tsuji, H., Nomoto, K., Ohno, K., et al. (2017). Progression of Parkinson's disease is associated with gut dysbiosis: Two-year follow-up study. PLoS One 12 (11), e0187307. doi:10.1371/journal.pone.0187307
Morgan, R. K., McAllister, B., Cross, L., Green, D. S., Kornfeld, H., Center, D. M., et al. (2007). Histamine 4 receptor activation induces recruitment of FoxP3+ T cells and inhibits allergic asthma in a murine model. J. Immunol. 178 (12), 8081–8089. doi:10.4049/jimmunol.178.12.8081
Mulak, A., and Bonaz, B. (2015). Brain-gut-microbiota axis in Parkinson's disease. World J. Gastroenterol. 21 (37), 10609–10620. doi:10.3748/wjg.v21.i37.10609
Nankova, B. B., Agarwal, R., MacFabe, D. F., and La Gamma, E. F. (2014). Enteric bacterial metabolites propionic and butyric acid modulate gene expression, including CREB-dependent catecholaminergic neurotransmission, in PC12 cells-possible relevance to autism spectrum disorders. PLoS One 9 (8), e103740. doi:10.1371/journal.pone.0103740
Nussbaum, R. L., and Ellis, C. E. (2003). Alzheimer's disease and Parkinson's disease. N. Engl. J. Med. 348 (14), 1356–1364. doi:10.1056/NEJM2003ra020003
O'Mahony, S. M., Clarke, G., Borre, Y. E., Dinan, T. G., and Cryan, J. F. (2015). Serotonin, tryptophan metabolism and the brain-gut-microbiome axis. Behav. Brain Res. 277, 32–48. doi:10.1016/j.bbr.2014.07.027
Pagano, G., Tan, E. E., Haider, J. M., Bautista, A., and Tagliati, M. (2015). Constipation is reduced by beta-blockers and increased by dopaminergic medications in Parkinson's disease. Park. Relat. Disord. 21 (2), 120–125. doi:10.1016/j.parkreldis.2014.11.015
Palma, J. A., and Kaufmann, H. (2014). Autonomic disorders predicting Parkinson's disease. Park. Relat. Disord. 20, S94–S98. doi:10.1016/s1353-8020(13)70024-5
Patenaude, A., Murthy, M. R., and Mirault, M. E. (2005). Emerging roles of thioredoxin cycle enzymes in the central nervous system. Cell Mol. Life Sci. 62 (10), 1063–1080. doi:10.1007/s00018-005-4541-5
Pedrosa Carrasco, A. J., Timmermann, L., and Pedrosa, D. J. (2018). Management of constipation in patients with Parkinson's disease. NPJ Park. Dis. 4, 6. doi:10.1038/s41531-018-0042-8
Peery, A. F., Crockett, S. D., Murphy, C. C., Lund, J. L., Dellon, E. S., Williams, J. L., et al. (2019). Burden and cost of gastrointestinal, liver, and pancreatic diseases in the United States: Update 2018. Gastroenterology 156 (1), 254–272. e211. doi:10.1053/j.gastro.2018.08.063
Pellerin, L. (2005). How astrocytes feed hungry neurons. Mol. Neurobiol. 32 (1), 59–72. doi:10.1385/mn:32:1:059
Perez Visñuk, D., Savoy de Giori, G., LeBlanc, J. G., and de Moreno de LeBlanc, A. (2020). Neuroprotective effects associated with immune modulation by selected lactic acid bacteria in a Parkinson's disease model. Nutrition 79-80, 110995. doi:10.1016/j.nut.2020.110995
Qin, J., Li, R., Raes, J., Arumugam, M., Burgdorf, K. S., Manichanh, C., et al. (2010). A human gut microbial gene catalogue established by metagenomic sequencing. Nature 464 (7285), 59–65. doi:10.1038/nature08821
Quigley, E. M. (1996). Gastrointestinal dysfunction in Parkinson's disease. Semin. Neurol. 16 (3), 245–250. doi:10.1055/s-2008-1040981
Quigley, E. M., and Spiller, R. C. (2016). Constipation and the microbiome: Lumen versus mucosa. Gastroenterology 150 (2), 300–303. doi:10.1053/j.gastro.2015.12.023
Radua, J. (2021). Prisma 2020 - an updated checklist for systematic reviews and meta-analyses. Neurosci. Biobehav Rev. 124, 324–325. doi:10.1016/j.neubiorev.2021.02.016
Read, N. W., Celik, A. F., and Katsinelos, P. (1995). Constipation and incontinence in the elderly. J. Clin. Gastroenterol. 20 (1), 61–70. doi:10.1097/00004836-199501000-00016
Rocca, W. A. (2018). The burden of Parkinson's disease: A worldwide perspective. Lancet Neurol. 17 (11), 928–929. doi:10.1016/s1474-4422(18)30355-7
Rodríguez-Ramallo, H., Báez-Gutiérrez, N., Prado-Mel, E., Alfaro-Lara, E. R., Santos-Ramos, B., and Sánchez-Fidalgo, S. (2021). Association between anticholinergic burden and constipation: A systematic review. Healthc. (Basel) 9 (5), 581. doi:10.3390/healthcare9050581
Romano, S., Savva, G. M., Bedarf, J. R., Charles, I. G., Hildebrand, F., and Narbad, A. (2021). Meta-analysis of the Parkinson's disease gut microbiome suggests alterations linked to intestinal inflammation. NPJ Park. Dis. 7 (1), 27. doi:10.1038/s41531-021-00156-z
Saad, R. J., Rao, S. S., Koch, K. L., Kuo, B., Parkman, H. P., McCallum, R. W., et al. (2010). Do stool form and frequency correlate with whole-gut and colonic transit? Results from a multicenter study in constipated individuals and healthy controls. Am. J. Gastroenterol. 105 (2), 403–411. doi:10.1038/ajg.2009.612
Sampson, T. R., Debelius, J. W., Thron, T., Janssen, S., Shastri, G. G., Ilhan, Z. E., et al. (2016). Gut microbiota regulate motor deficits and neuroinflammation in a model of Parkinson's disease. Cell 167 (6), 1469–1480. e1412. doi:10.1016/j.cell.2016.11.018
Schneider, E., Rolli-Derkinderen, M., Arock, M., and Dy, M. (2002). Trends in histamine research: New functions during immune responses and hematopoiesis. Trends Immunol. 23 (5), 255–263. doi:10.1016/s1471-4906(02)02215-9
Stirpe, P., Hoffman, M., Badiali, D., and Colosimo, C. (2016). Constipation: An emerging risk factor for Parkinson's disease? Eur. J. Neurol. 23 (11), 1606–1613. doi:10.1111/ene.13082
Stocchi, F., and Torti, M. (2017). Constipation in Parkinson's disease. Int. Rev. Neurobiol. 134, 811–826. doi:10.1016/bs.irn.2017.06.003
Sun, H. R. (2020). Clinical efficacy of Bifidobacterium triple viable bacteria tablets combined with mosapride in the treatment of Parkinson's constipation. Tibet. Med. 41 (06), 92–94.
Sung, J. H., Gim, S. A., and Koh, P. O. (2014). Ferulic acid attenuates the cerebral ischemic injury-induced decrease in peroxiredoxin-2 and thioredoxin expression. Neurosci. Lett. 566, 88–92. doi:10.1016/j.neulet.2014.02.040
Tan, A. H., Lim, S.-Y., Chong, K. K., A Manap, M. A. A., Hor, J. W., Lim, J. L., et al. (2021). Probiotics for constipation in Parkinson disease: A randomized placebo-controlled study. Neurology 96 (5), e772–e782. doi:10.1212/WNL.0000000000010998
Thiyagalingam, S., Kulinski, A. E., Thorsteinsdottir, B., Shindelar, K. L., and Takahashi, P. Y. (2021). Dysphagia in older adults. Mayo Clin. Proc. 96 (2), 488–497. doi:10.1016/j.mayocp.2020.08.001
Trombino, S., Cassano, R., Ferrarelli, T., Barone, E., Picci, N., and Mancuso, C. (2013). Trans-ferulic acid-based solid lipid nanoparticles and their antioxidant effect in rat brain microsomes. Colloids Surf. B Biointerfaces 109, 273–279. doi:10.1016/j.colsurfb.2013.04.005
Türler, A., Kalff, J. C., Moore, B. A., Hoffman, R. A., Billiar, T. R., Simmons, R. L., et al. (2006). Leukocyte-derived inducible nitric oxide synthase mediates murine postoperative ileus. Ann. Surg. 244 (2), 220–229. doi:10.1097/01.sla.0000229963.37544.59
Ueki, A., and Otsuka, M. (2004). Life style risks of Parkinson's disease: Association between decreased water intake and constipation. J. Neurol. 251, vII18–23. doi:10.1007/s00415-004-1706-3
Unger, M. M., Spiegel, J., Dillmann, K. U., Grundmann, D., Philippeit, H., Bürmann, J., et al. (2016). Short chain fatty acids and gut microbiota differ between patients with Parkinson's disease and age-matched controls. Park. Relat. Disord. 32, 66–72. doi:10.1016/j.parkreldis.2016.08.019
Vicentini, F. A., Keenan, C. M., Wallace, L. E., Woods, C., Cavin, J. B., Flockton, A. R., et al. (2021). Intestinal microbiota shapes gut physiology and regulates enteric neurons and glia. Microbiome 9 (1), 210. doi:10.1186/s40168-021-01165-z
Wakabayashi, K., Takahashi, H., Takeda, S., Ohama, E., and Ikuta, F. (1988). Parkinson's disease: The presence of lewy bodies in auerbach's and meissner's plexuses. Acta Neuropathol. 76 (3), 217–221. doi:10.1007/bf00687767
Wang, S.-y., Wang, L.-d., Cao, Y.-j., Li, J.-b., Zhao, C., Liu, C.-q., et al. (2020). The triple live bacteria preparation regulates intestinal flora and improves constipation, anxiety and sleep in patients with Parkinson's disease. J. Clin. Pathology 40 (11), 2919–2924.
Wang-Shaoying, , Wang-Iidi, , Cao-Yujiao, , Li-Jinbin, , Can, Z., Cuiqin, L., et al. (2020). The triple viable preparation regulates the intestinal flora to improve the constipation, anxiety and sleep of the patients with Parkinson's disease. J J. Clin. Pathological Res. 40 (11), 2919–2924.
Westfall, S., Lomis, N., Kahouli, I., Dia, S. Y., Singh, S. P., and Prakash, S. (2017). Microbiome, probiotics and neurodegenerative diseases: Deciphering the gut brain axis. Cell Mol. Life Sci. 74 (20), 3769–3787. doi:10.1007/s00018-017-2550-9
Wilkins, T., and Sequoia, J. (2017). Probiotics for gastrointestinal conditions: A summary of the evidence. Am. Fam. Physician 96 (3), 170–178.
Xiang, S., Ji, J. L., Li, S., Cao, X. P., Xu, W., Tan, L., et al. (2022). Efficacy and safety of probiotics for the treatment of alzheimer's disease, mild cognitive impairment, and Parkinson's disease: A systematic review and meta-analysis. Front. Aging Neurosci. 14, 730036. doi:10.3389/fnagi.2022.730036
Xu, L., Zhao, Y., Mao, L., and Zhang, X.-h. (2018). Effects of early intestinal flora intervention on constipation symptoms and the efficacy of dopasehydrazine in patients with Parkinson's disease. J. Pharm. Pract. 36 (06), 547–550.
Xu, P., Das, M., Reilly, J., and Davis, R. J. (2011). JNK regulates FoxO-dependent autophagy in neurons. Genes Dev. 25 (4), 310–322. doi:10.1101/gad.1984311
Yan, T., Shi, J., and Li, X. (2022). Clinical efficacy of probiotics in the treatment of constipation in Parkinson patients. Minerva Gastroenterol. (Torino) 68, 369–371. doi:10.23736/s2724-5985.21.03078-3
Keywords: probiotics, Parkinson's disease, constipation, inflammation, gut-brain axis, meta-analysis
Citation: Xie L, Chen D, Zhu X and Cheng C (2023) Efficacy and safety of probiotics in Parkinson’s constipation: A systematic review and meta-analysis. Front. Pharmacol. 13:1007654. doi: 10.3389/fphar.2022.1007654
Received: 02 August 2022; Accepted: 21 December 2022;
Published: 10 January 2023.
Edited by:
Raquel Abalo, Rey Juan Carlos University, SpainReviewed by:
Ali Chaari, Weill Cornell Medicine- Qatar, QatarCopyright © 2023 Xie, Chen, Zhu and Cheng. This is an open-access article distributed under the terms of the Creative Commons Attribution License (CC BY). The use, distribution or reproduction in other forums is permitted, provided the original author(s) and the copyright owner(s) are credited and that the original publication in this journal is cited, in accordance with accepted academic practice. No use, distribution or reproduction is permitted which does not comply with these terms.
*Correspondence: Cisong Cheng, Y2NzdGNtQDE2My5jb21jY3M=
Disclaimer: All claims expressed in this article are solely those of the authors and do not necessarily represent those of their affiliated organizations, or those of the publisher, the editors and the reviewers. Any product that may be evaluated in this article or claim that may be made by its manufacturer is not guaranteed or endorsed by the publisher.
Research integrity at Frontiers
Learn more about the work of our research integrity team to safeguard the quality of each article we publish.