- 1Clinical Medicine, School of Medicine, Qinghai University, Xining, China
- 2Department of Respiratory and Critical Diseases, Affiliated Hospital of Qinghai University, Xining, China
- 3Research Center of High-Altitude Medicine, School of Medicine, Qinghai University, Xining, China
- 4Joint Lab of Qinghai-Utah for High Altitude Medicine, School of Medicine, Qinghai University, Xining, China
The coronavirus disease 2019 (COVID-19), caused by severe acute respiratory syndrome coronavirus 2 (SARS-CoV-2), epidemic poses a major global public health threat with more than one million daily new infections and hundreds of deaths. To combat this global pandemic, efficient prevention and management strategies are urgently needed. Together with the main characteristics of COVID-19, impaired coagulation with dysfunctions of the immune response in COVID-19 pathophysiology causes high mortality and morbidity. From recent clinical observations, increased expression of specific types of estrogen appears to protect patients from SARS-CoV-2 infection, thereby, reducing mortality. COVID-19 severity is less common in women than in men, particularly in menopausal women. Furthermore, estrogen levels are negatively correlated with COVID-19 severity and mortality. These findings suggest that estrogen plays a protective role in the pathophysiology of COVID-19. In this review, we discuss the potential roles of estrogen in blocking the SARS-CoV-2 from invading alveolar cells and replicating, and summarize the potential mechanisms of anti-inflammation, immune modulation, reactive oxygen species resistance, anti-thrombosis, vascular dilation, and vascular endothelium protection. Finally, the potential therapeutic effects of estrogen against COVID-19 are reviewed. This review provides insights into the role of estrogen and its use as a potential strategy to reduce the mortality associated with COVID-19, and possibly other viral infections and discusses the possible challenges and pertinent questions.
Introduction
In December 2019, an outbreak of coronavirus disease (COVID-19) caused by severe acute respiratory syndrome coronavirus 2 (SARS-COV-2) was reported in Wuhan, China (Guan et al., 2020; Wang et al., 2020), and has since rapidly spread globally (Young et al., 2020). As of 30 September 2022, over 652 million cases have been reported worldwide with a death toll of more than 6.5 million (WHO, 2022a). The huge spread of COVID-19 has created enormous challenges for global healthcare systems and economies. SARS-CoV-2 is a single-stranded RNA virus surrounded by a capsule (Han et al., 2020). Similar to the Middle East respiratory syndrome coronavirus and severe acute respiratory syndrome coronavirus 1 (SARS-CoV-1), SARS-CoV-2 also begins as an infection of the upper respiratory tract and over time invades the lower respiratory tract, eventually leading to fatal pneumonia (Wu et al., 2021).
SARS-CoV-1 and SARS-CoV-2 share 79.6% homology (Zhou et al., 2020; Stukalov et al., 2021). During the previous SARS-CoV-1 and Middle East respiratory syndrome coronavirus epidemics, estrogen and its analogs were reported to play a protective role against SARS coronavirus infections (Channappanavar et al., 2017; Islam et al., 2021). In addition, animal studies have shown that reducing estradiol or using estrogen receptor antagonists is conducive to severe adult respiratory syndrome coronavirus infection (Channappanavar et al., 2017; Suba, 2020). Throughout the SARS-CoV-2 pandemic, the rate of hospitalization for men remains much higher than that for women (Gomez et al., 2021; Mohamed et al., 2021). Moreover, men have more severe symptoms and higher mortality (Alwani et al., 2021; Ferretti et al., 2022). A recent retrospective study of estrogen therapy in female patients with COVID-19 showed that those over 50 years of age receiving estrogen therapy had a 50% reduction in the risk of death from COVID-19 than those under 35 years of age (Seeland et al., 2020). An in vitro study showed that the viral load of cell infection decreased after treating SARS-CoV-2 infected cells with estrogen (Lemes et al., 2021). New studies have shown that estrogen induces a stronger response to the vaccine (Heriyanto et al., 2021; Uwamino et al., 2022). Moreover, plant flavonoids, known as phytoestrogens, surprisingly affect multiple processes including viral penetration into the host cells, replication of viral nucleic acids, and release of viral particles from cells infected with SARS-CoV-2 (Rahman et al., 2022). These findings suggest the potential protective role of estrogen in patients with COVID-19 (Figure 1). However, the exact role of estrogen in COVID-19 and the associated underlying mechanisms remain nebulous. Here, we summarize the potential roles of estrogen in interfering with the invasion and replication of SARS-CoV-2 virus and discuss the underlying mechanisms as well as the challenges in using estrogen to curtail this global pandemic. Briefly, we have organized this mini review as follows: 1) Overview of estrogen; 2) Estrogen and COVID-19; 3) Deficiencies in estrogen therapy and clinical treatment challenges; and 4) Conclusion and future directions.
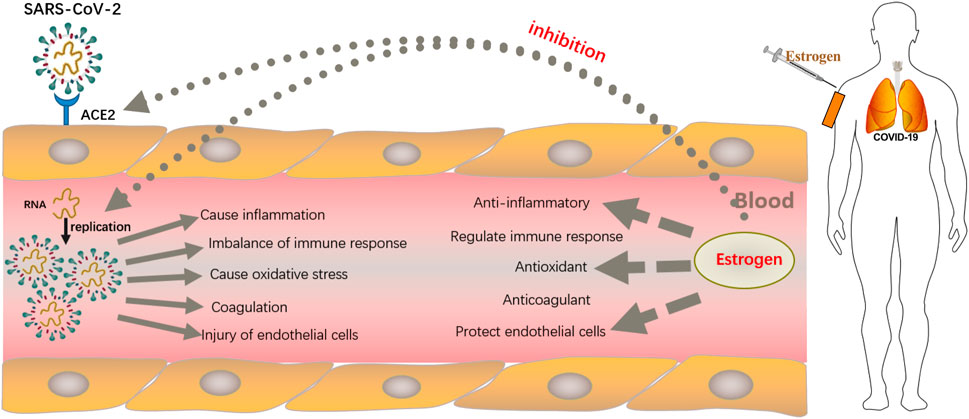
FIGURE 1. Strategies employed by estrogen in resisting SARS-CoV-2 infection. After binding to the angiotensin-converting enzyme 2 (ACE2) receptor on host cells through the spike protein, which lyses and fuses the cell membrane into host type 2 alveolar epithelial cells, SARS-CoV-2 enters the host cell and begins to replicate to produce more viral nucleocapsids, which requires the participation of the main viral protease enzyme. Thereafter, proliferated SARS-CoV-2 causes damage to alveolar macrophages, the tissue microenvironment releases proinflammatory cytokines and chemokines that promote the attraction of macrophages, neutrophils, and T cells, which can lead to uncontrolled inflammation, immune disorders, oxidative stress, damage to endothelial cells, and fatal clots. Estrogen, mostly administered subcutaneously, not only reduces the expression of ACE2 receptors on host type 2 alveolar epithelial cells, thereby, preventing the entry of SARS-COV-2 virus into host cells, but also inhibits viral replication by occupying the active site of the protease required for viral replication. In addition, estrogen has anti-inflammatory, antioxidant, immune cell regulatory, antithrombotic, and endothelial cell protection properties.
Overview of estrogen
Resources and classification
Estrogen is a steroid hormone with lipophilic properties and plays an important role in the growth and development of several organ systems, including the breast, uterus, neuroendocrine, skeletal, and cardiovascular systems (Hamilton et al., 2017). Most of the estrogen in the body is originated from the ovaries, luteum, and placenta of premenopausal women, whereas a small portion comes from non-ovarian organs, such as the adrenal gland, liver, heart, skin, brain, male testicles, and adipose tissue (Barakat et al., 2016; Catenaccio et al., 2016).
Three types of estrogens have been identified in the human body: estrone (E1), 17β-estradiol (E2), and estriol (E3) (Cui et al., 2013), among which E2 has the strongest biological activity (Medzikovic et al., 2019) and is the main and most effective form of estrogen in female blood circulation (Rettberg et al., 2014). E1 is synthesized by the adrenal dehydroepiandrosterone in the adipose tissue and is more predominant after menopause; E2 is the main product of the biosynthetic process and the most effective estrogen before menopause; E3, produced from E1 and formed by 16A hydroxylation, is the weakest, and yet it plays an important role during pregnancy (Lee et al., 2019). E1 is converted into E2 by the action of 17β hydroxylated steroid dehydrogenase 1 (17β-HSD1). Conversely, 17 β-hydroxylated steroid dehydrogenase 2 (17β-HSD2) converts E2 back to E1. This process is reversible; however, the conversion rate from E1 to E2 is slow. E2 also produces different metabolites via different cytochrome P450 enzymes and has been shown to play different biological roles in lung disease (Hood et al., 2016; Docherty et al., 2019).
Interaction of estrogen with cellular receptors
Estrogen can easily pass through the cell membrane and bind to estrogen receptors (ERs), which mediate its physiological effects. Hence, ER is the primary target of the regulatory function of estrogen and affects diseases of multiple organ systems, such as the cardiovascular and skeletal systems (Luo and Kim, 2016).
Most estrogen receptors are classified as ligand-activated transcription factors in the steroid family. To date, two classical ERs, namely nuclear estrogen receptor (nER) and membrane estrogen receptor (mER), have been identified (Fuentes and Silveyra, 2019). There are two subtypes of nERs: ERα and ERβ (Dama et al., 2021). Erα, discovered in 1958 by Elwood Jenson, is diffusely scattered in the testicles, uterus, ovaries, prostate, skeletal muscle, kidney, skin, and at other sites (Russell et al., 2019). ERβ, isolated in 1996 by Kuiper et al. (1996), is widely distributed in the ovary, colon, kidney, brain tissue, as well as in the male reproductive system. Through the regulatory effect of different genes, ERα and ERβ have different effects on the disease state. Interestingly, ERβ not only exerts a protective effect against cardiopulmonary diseases, but also has antifibrotic (Pedram et al., 2010), antihypertrophic (Hajializadeh and Khaksari, 2022), anti-inflammatory (Umar et al., 2011), and vasodilation properties (Mishra et al., 2019). ERβ promotes the production of various angiogenic factors to regulate angiogenic NO and exerts vascular dilatation effects (Iorga et al., 2017). Estrogen can reduce the pressure caused by pulmonary vessels in the pathological state to protect the pulmonary arteries (Lahm et al., 2012). There is evidence that some target cells can respond to estrogen without the involvement of an ER (Thomas & Gustafsson, 2011), which is attributed to the G-protein-coupled estrogen receptors (GPERs) containing G-protein-coupled receptor 30 (GPR30) and ER-X that mediate fast mER-associated estrogen effects (Knowlton and Lee, 2012; Tang et al., 2019). GPR30 is expressed in the adrenal medulla, hippocampus, renal pelvis, cortex, hypothalamus, and ovaries (Xiang et al., 2019). The expression of ER-X is tightly regulated during the developmental stages of the brain, uterus, and fetus. In adults, ER-X is expressed at low levels in normal physiological states, but its expression increases following ischemic injury (Kiyama and Wada-Kiyama, 2015).
Biosynthesis and metabolism of estrogen
Estrogen is produced by endometrial and granulosa cells in the follicles through the synergistic action of follicle-stimulating hormone (FSH) and luteinizing hormone (LH) (Barakat et al., 2016). This is referred to as the two-cell two-gonadotropin theory of estrogen synthesis, also known as the dual-cell theory (Figure 2). First, the endometrial cells of follicles use cholesterol as a raw material under the action of LH and convert it into pregnenolone via cytochrome P450 enzymes (Bassi et al., 2021). Pregnenolone in turn is converted into progesterone through the action of 3β-hydroxysteroid dehydrogenase. Progesterone is then oxidized to dehydroepiandrosterone and androstenedione by 17α-hydroxylase (Manca et al., 2012). Androstenedione is converted to testosterone by 17β-hydroxysteroid dehydrogenase type 3 (HSD17B3), which is expressed almost exclusively in testicular interstitial cells (Yazawa et al., 2020). The resulting androstenedione and testosterone then diffuse into the granulosa cells through the basement membrane (Biegon et al., 2020). Aromatase activity is enhanced by FSH (Cooke et al., 2017). Androstenedione is then converted to estrone and testosterone is converted to estradiol (Fukami and Ogata, 2022). A small proportion of synthetic estrogen enters the follicular cavity, whereas a large proportion enters the bloodstream, thereby regulating the differentiation and growth of target cells, such as the endometrium and breast. Estrogen is metabolized via sulfation, which is a strategy to control its activity. By conjugating with sulfonic acid groups, sulfotransferase converts E1, E2, estrogen precursors, and metabolites (dehydroepiandrosterone and 2-Me) to Sulfated E1, E2, and 2-ME. These inactive sulfated steroids do not activate ERS, and being hydrophilic, they are more easily excreted by the kidneys (Sánchez-Guijo et al., 2016).
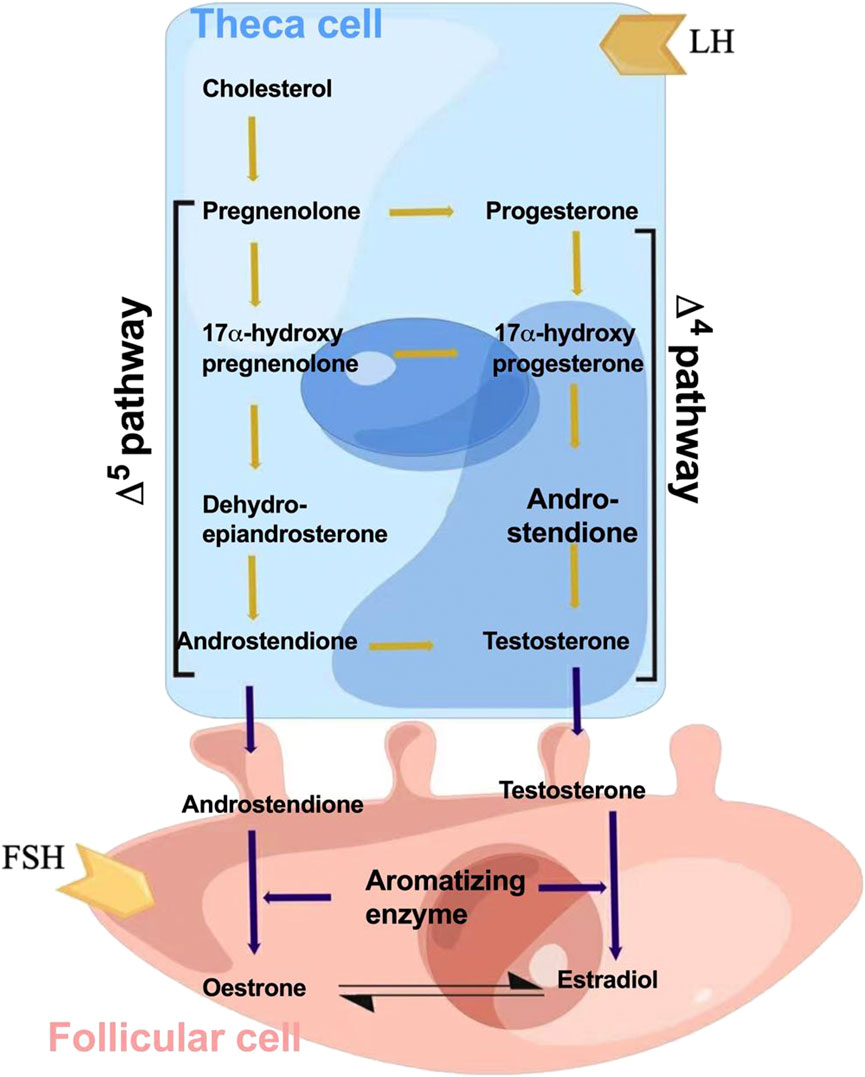
FIGURE 2. Schematic for estrogen synthesis in the ovaries. The dual-cell theory or two-gonadotropin theory of estrogen synthesis explains that estrogen is produced from endometrial and granulosa cells in the follicles through the synergistic action of follicle-stimulating hormone (FSH) and luteinizing hormone (LH). The endometrial cells of follicles use cholesterol as a raw material under the action of the luteinizing hormone and convert it into pregnenolone via cytochrome P450 enzymes. Thereafter, pregnenolone is converted into progesterone through the action of 3β-hydroxysteroid dehydrogenase. Progesterone is then oxidized to dehydroepiandrosterone and androstenedione by 17α-hydroxylase. Androstenedione is converted to testosterone by 17β-hydroxysteroid dehydrogenase type 3 (HSD17B3). The resulting androstenedione and testosterone then diffuse into the granulosa cells through the basement membrane. Aromatase activity is enhanced by FSH, and androstenedione is then converted to estrone and testosterone is converted to estradiol as the end products.
Physiological roles of estrogen
Estrogen promotes the development of female secondary sexual characteristics and plays an important role in regulating the structure and function of the female reproductive system (Vasquez, 2018). Estrogen can also promote the development of the uterus; it relaxes the cervical opening during ovulation, promotes hypertrophy of the uterine smooth muscle and contraction of the fallopian tube and cilia swing, and makes vaginal secretions acidic to resist infection, besides being involved in follicular and external genital development in coordination with FSH (Chou and Chen, 2018). Estrogen also has important effects on other systems in the body. For instance, it can promote bone growth and development, thereby increasing the height of adolescent females (Iravani et al., 2017). Moreover, it improves lipid composition, prevents atherosclerosis, and protects the cardiovascular system (Knowlton and Lee, 2012). It also promotes the growth, differentiation, and regeneration of neurons (Brann et al., 2022). High estrogen levels can cause fluid movement between tissues, leading to the retention of sodium and water (Mallareddy et al., 2007). In addition, estrogen plays an important role in the occurrence, development, and prognosis of COVID-19 through its anti-inflammatory effects (Pelekanou et al., 2016); regulation of immune response (Kovats, 2015); antioxidative (Tsialtas et al., 2021), antithrombotic (Lee and Syed, 2022), and vasodilatory properties; and by protecting endothelial cells (Diebel et al., 2021).
Estrogen and COVID-19
Estrogen inhibits the replication of SARS-CoV-2
SARS-CoV-2 consists of four structural proteins—the spike, membrane, envelope, and nucleocapsid proteins. Of these, the spike protein protrudes from the virus surface and plays an important role in its attachment to host cells and in the penetration of cell membranes (Ke et al., 2020). SARS-CoV-2 binds to the angiotensin-converting enzyme 2 (ACE2) receptor on host cells through the spike protein, which lyses and fuses the cell membrane with host type 2 alveolar epithelial cells (Chambers et al., 2020; Jackson et al., 2022). SARS-CoV-2 then enters the host cell and begins to replicate to produce more viral nucleocapsids, which requires the participation of the main viral protease enzyme (Sun et al., 2022).
ACE2 is widely expressed in human cells, including alveolar and small intestinal epithelial cells, vascular endothelial cells, and smooth muscle cells (Kočar et al., 2021). Estrogen can affect the function and activity of SARS-CoV-2 (Mateus et al., 2022). It can reduce the expression of ACE2 receptors on host type 2 alveolar epithelial cells, downregulate the reactivity and number of ACE2 receptors (Seeland et al., 2020), reduce the binding of SARS-CoV-2 to ACE2 receptors, and prevent the entry of SARS-CoV-2 into host cells (Baristaite and Gurwitz, 2022). Furthermore, estrogen strongly interacts with the active site of the main protease required for viral replication, as a competitive inhibitor, thereby preventing SARS-COV-2 replication in host cells by occupying the active site of the protease enzyme (Mansouri et al., 2022). In addition, estrogen mitigates common mechanisms of injury by reducing the expression of ACE2 receptor and excessive inflammation, protecting endothelial and mitochondrial functions, insulin sensitivity, clotting cascade, immune response, cerebrovascular flow, and cognition (Saavedra, 2021). These properties of estrogen have already been employed in the treatment of inflammation, age-related changes, neurodegeneration, and metabolic disorders in many organs, including the brain and lungs (Saavedra, 2021).
Immunoresistance and immunomodulation of estrogen against SARS-CoV-2
SARS-COV-2 induces apoptotic cell death in infected alveolar epithelial cells, which in turn causes the leakage of blood vessels in alveolar cells, initially triggering local inflammation, and subsequently the recruitment of circulatory immune cells into the infected lungs to confront the extracellular enveloped virus and ultimately destroy the virus-infected cells (Cao, 2020; Shi et al., 2020). Virus-laden lung cells also stimulate surrounding inflammatory cells (e.g., macrophages, mast cells, and dendritic cells), thereby producing many inflammatory mediators, including interleukins (IL) -12, -8, -6, -120, and -1, along with tumor necrosis factor-alpha (TNF-α), macrophage inflammatory protein 1α, interferon-β, monocyte chemotaxis protein, and interferon-γ (Qin et al., 2020). The release of a large number of inflammatory mediators activates the vascular and leukocyte responses of the host, resulting in the recruitment and infiltration of several leukocytes, which further exacerbates the inflammatory response. An excessive inflammatory response results in damage to the lung tissue, diffuse alveolar damage, and alveolar hemorrhage (Ackermann et al., 2020; Pernazza et al., 2020), leading to acute respiratory distress syndrome (Xu et al., 2020).
In addition, the effect of SARS-CoV-2 on the mechanism of cellular stress activation in immunoactive cells is important. SARS-CoV-2 induces polyclonal activation and lymphocyte apoptosis, pathological activation of macrophages, and immunosuppression (Xiong et al., 2020), resulting in a sharp reduction in the number of lymphocytes (mainly T cells) and cytokine storms. Lymphocytes are white blood cells that play a key role in adaptive immunity, enabling the immune system to recognize and remember antigens; they are the core components of the immune system that mainly comprise B and T lymphocytes. The B lymphocytes participate in humoral immunity and secrete antibodies. The T lymphocytes are involved in cellular immunity in the body. Cytokine storms can trigger an inflammatory environment, closely associated with severe tissue damage (Huang et al., 2020). Acute lymphocyte depletion and cytokine storm-induced excessive immune response can cause dysregulation of the immune response in patients with SARS-CoV-2 infection and can ultimately lead to septic shock, acute respiratory distress syndrome, and/or multiple organ failure, thereby increasing mortality (Jamal et al., 2021).
Estrogen is an important factor in inhibiting inflammation and in regulating immunity in COVID-19 (Arnold et al., 2022; Li et al., 2022). It is involved in regulating the development of inflammation and immune responses (Aksoy et al., 2014; Kowsar et al., 2014). The expression of proinflammatory and anti-inflammatory cytokines correlates with estrogen levels—low estrogen levels contribute to the production and regulation of proinflammatory cytokines, whereas high estrogen levels fight inflammation by inhibiting the production of inflammatory cytokines, such as IL-6, IL-1β, and TNF-α (Aksoy et al., 2014; Wang et al., 2021). Estrogen also reduces the risk of COVID-19 by downregulating inflammatory markers, such as chemokines and cell adhesion molecules (Pelekanou et al., 2016).
The role of anti-reactive oxygen species in COVID-19
The human body constantly generates oxygen free radicals during normal metabolism, of which approximately 95% are reactive oxygen species (ROS), including superoxide anions (O2-), hydrogen peroxide (H2O2), hydroxyl free radicals (-OH), and peroxynitrite (ONOO-) (Galaris et al., 2019). Under optimum conditions, the body’s oxidative and antioxidant defense systems maintain a dynamic balance. Pathological damage occurs when there is an imbalance between antioxidant and oxidative effects, which is known as oxidative stress (OS) (Pignatelli et al., 2018). Generally, the excessive production of ROS and the imbalance of antioxidant mechanisms are critical to viral invasion and replication (Khomich et al., 2018). Infections with SARS-CoV-2 induce OS by producing large quantities of ROS, which is the primary cause of severe local and systemic tissue damage in COVID-19. Excessive ROS can increase the formation of neutrophil extracellular traps and inhibit the protective effects of the human immune system, that is, killing T cells involved in cellular immunity and preventing the specific immune response to SARS-CoV-2 from taking effect (Schönrich et al., 2020). Elevated levels of OS and reduced antioxidant capacity are associated with increased disease severity in hospitalized COVID-19 patients. Therefore, the use of antioxidants could be an effective treatment strategy for COVID-19 (Karkhanei et al., 2021).
Estrogen exerts an antioxidant effect on COVID-19 patients. In an animal study, rats with low estrogen levels had higher OS than those with high estrogen levels (Barp et al., 2002). Another in vivo study indicated that young women had lower biochemical markers of OS than their men counterparts (Kander, Cui, & Liu, 2017). In addition, clinical and experimental data indicate that women have stronger antioxidant capacity than that in men (Reckelhoff et al., 2019; Kim, 2022). Multiple studies have suggested that estrogen restrains vascular OS by regulating ROS production, thereby expediting the clearance of ROS and decreasing local ROS production (Arias-Loza et al., 2013). Other studies investigating the effect of estrogen on OS have shown that estrogen can reduce serum lipid peroxides and upregulate the body’s antioxidant status (Palmisano et al., 2018). Estrogen increases the levels of binding proteins produced by the liver, restores sodium balance, and distributes lipids by decreasing the levels of low-density lipoproteins and elevating the levels of high-density lipoproteins (HDL) (Ko and Kim, 2020). HDL levels have also been proven beneficial for COVID-19 prognosis (G. Wang et al., 2021).
Estrogen as an anticoagulant
Abnormal coagulation is one of the prominent features of severe COVID-19. Severe endothelial injury and extensive thrombosis with microangiopathy have been observed in the lungs of COVID-19 patients (Ackermann et al., 2020) due to the extensive activation of the coagulation system in patients infected with SARS-COV-2, which increases the risk of blood clotting (Tang et al., 2020) and thrombosis. A large-scale cadaver lung autopsy in northern Italy revealed platelet-fibrin thrombosis in the small pulmonary arteries, suggesting that thrombosis, following SARS-COV-2 infection, plays a critical role in the death of COVID-19 patients (Carsana et al., 2020). Acute pulmonary embolism caused by venous thrombosis (Danzi et al., 2020; Zuckier et al., 2020) is life threatening in COVID-19 patients.
SARS-CoV-2 cells can stimulate surrounding inflammatory cells to release a large number of cytokines and chemokines to recruit many white blood cells (such as neutrophils and monocytes), which can enhance intravascular coagulation and thrombosis by activating the coagulation system and inhibiting the anticoagulant mechanism (Levi and van der Poll, 2017; Zuckier et al., 2020). Neutrophils infiltrate and activate host cells, producing a large number of toxic tissue mediators, such as ROS, to damage the host cells, and activate monocytes to express tissue factors (TF) that can stimulate the coagulation cascade and thrombin generation (Teuwen et al., 2020). Some cytokines, for instance, TNF-α and IL-6, are effective activators of TF-dependent coagulation cascades (Kerr et al., 2001). Moreover, anticoagulant pathways associated with endothelial cells, especially the protein C system, are also susceptible to damage by inflammatory cytokines such as IL-1β and TNF-α (Levi and van der Poll, 2017). Endothelial cells respond to the activation of IL-1β and TNF-α by expressing p-selectin, von Willebrand factor, and fibrinogen to promote coagulation, leading to the binding of a large number of platelets, which release VEGF, thereby enhancing the expression of TF in endothelial cells and further irritating the coagulation cascade (Teuwen et al., 2020). In other words, these events are conducive to increased fibrin production, which induces the deposition of blood clots in the microvascular system (Levi and van der Poll, 2017).
The effectiveness of estrogen as an anticoagulant depends on its administration. In a study on the effects of estrogen on postmenopausal women undergoing hysterectomy, those who received estrogen therapy alone had an increased risk of venous thromboembolism (VTE) compared with those in an oral placebo group (Anderson et al., 2004). However, some observational studies revealed that the risk of VTE in patients administered estrogen via subcutaneous injection was much lower than that in the orally administered estrogen group (Scarabin et al., 2003; Laliberté et al., 2018). The higher risk of VTE associated with oral estrogen is attributed to its effects on the liver, leading to an increase in some hepatogenic coagulation factors (Elger et al., 2017). The reason for the lower risk of VTE when estrogen is administered via the subcutaneous route is that estrogen can alter the number and function of platelets. Fox and Chambers (2006) observed significant reductions in platelet counts in ovariectomized mice treated with E2 (10 or 100 μg/kg per day, subcutaneously) for 10 days (Fox and Chambers, 2006). Valéra et al. (2012) also observed that the number of platelets in ovariectomized mice was reduced to varying degrees after treatment with E2 (200 μg/kg per day, subcutaneously) for 3 weeks (Valéra et al., 2012). An animal study showed that chronic subcutaneous injection of E2 reduced platelet reactivity and prolonged tail hemorrhage in mice (Valéra et al., 2017). Long-term high physiological levels of E2 in mice significantly reduced the reactivity of platelets in vivo and in vitro and the risk of thrombosis in mice (Valéra et al., 2015). E2 therapy has been shown to modulate the expression of platelet proteins, including β1 tubulin and several other proteins, which affect the production and activation of platelets. Estradiol can also inhibit platelet aggregation in vitro by promoting the excretion or reuptake of Ca2+ (Breithaupt-Faloppa et al., 2020).
Furthermore, estrogen reduces the expression of plasminogen activator inhibitor TYPE 1 and enhances the activity of tissue plasminogen through both non-receptor-mediated and receptor-mediated mechanisms of antioxidant activity, suggesting that estrogen has a beneficial effect on fibrinolysis (Yoon et al., 2020). Estradiol also protects endothelial function by activating endothelial nitric oxide (NO) synthase and increasing NO production (Park et al., 2021). Intact endothelial cells can maintain blood in blood vessels by inhibiting platelet adhesion and anticoagulant effects (Gu et al., 2017).
Estrogen protects vascular endothelium and causes dilation of blood vessels
The vascular endothelium is an active paracrine, endocrine, and autocrine organ, essential for regulating vascular tone and maintaining vascular homeostasis (Flammer et al., 2012). Endothelial dysfunction is a major determinant of microvascular dysfunction; when the endothelial function is compromised, and vascular endothelial barrier function declines, the vascular permeability increases, and the endothelial cells secrete a variety of bioactive substances, which can cause vasoconstriction and subsequent ischemia of organs and tissue edema related to inflammation and blood coagulation (Joffre et al., 2020), causing further damage to the body.
SARS-CoV-2 infects the host by binding to ACE2 receptors expressed by alveolar epithelial cells, which are also widely expressed on vascular endothelial cells (Ferrario et al., 2005; Ackermann et al., 2020). A study of SARS-CoV-2 directly infecting engineered human blood vessels in vitro showed that SARS-CoV-2 could directly infect endothelial cells through ACE2 to alter vascular homeostasis (Monteil et al., 2020). After binding to the vascular endothelium, SARS-CoV-2 downregulated ACE2 receptor reactivity, and number of the receptor by affecting its expression in the vascular endothelium, which is conducive to the progression of pulmonary inflammation and profibrosis caused by local angiotensin II overactivity (Verdecchia et al., 2020). Reduced ACE2 expression can, in turn, indirectly activate the kallikrein—kinin system, ultimately leading to increased vascular permeability (Garvin et al., 2020; Teuwen et al., 2020). Virus-laden lung cells also stimulate the surrounding inflammatory cells to produce large amounts of cytokines, inducing an inflammatory response. Hypercytokinemia and the massive inflammatory response of the host cells can lead to endothelial dysfunction in COVID-19. For example, IL-6 can increase vascular permeability and promote the secretion of inflammatory cytokines by endothelial cells, thereby increasing the release of cytokines (Teuwen et al., 2020) and creating a vicious cycle. Electron microscopy of postmortem patient tissues further confirmed this conclusion, suggesting that SARS-CoV-2 can infect pulmonary endothelial cells and induce endodermatitis (Varga et al., 2020). Virus-infected vascular endothelium leads to extensive endothelial dysfunction associated with apoptosis through the immune-mediated recruitment of immune cells. In addition, the reduction in endothelial NO synthase activity, NO levels, and the release of VEGFs due to ARDS-induced systemic hypoxia have been deemed as pivotal pathogenic processes responsible for endothelial dysfunction after SARS-COV-2 infection (Martini, 2020).
Estrogen exerts a protective effect on endothelial function. Estrogen deficiency reduces NO content and causes an oxidative stress response to OS damage in endothelial cells. In a study on mice, Wassmann et al. (2002) used a selective estrogen receptor modulator, raloxifene, which increased the activity of endothelial NO synthase and the dependence of ER in reducing the release of ROS from blood vessel cells to improve endothelial dysfunction caused by high blood pressure; these vascular effects led to a significant reduction in blood pressure and vascular injury in male spontaneously hypertensive rats. Conversely, high physiological levels of estrogen increase the production of NO and reduce lipid peroxidation in ovariectomized rats (Yazğan et al., 2016). A study on estrogen therapy in healthy postmenopausal women showed that treatment with estrogen can significantly reduce catecholamine levels, mean blood pressure, and LDL levels, and increase HDL levels (Nii et al., 2016; Anagnostis et al., 2020). High HDL levels have also been shown to protect the vascular endothelium (Robert et al., 2021). Estrogen can activate the transcription of NO synthase by binding to the classical E2 receptor or GPER (Iorga et al., 2017), producing the vasodilator, NO, which spreads to vascular smooth muscle cells, activates guanylate cyclase, and increases the levels of cyclic guanosine phosphate (Förstermann et al., 2017). NO plays a direct role in tissue oxygen balance, organ perfusion, vascular remodeling, and metabolic demands by protecting endothelial cells and regulating vascular tension and diameter (Ally et al., 2020).
Deficiencies in estrogen therapy and challenges in clinical treatment
Owing to their numerous biological effects, as shown in Figure 3, estrogen and its analogs are associated with the treatment of a wide range of diseases, including neuroprotective therapies for ischemic stroke, Alzheimer’s disease, and Parkinson’s disease. Furthermore, estrogen remains the most effective treatment for symptomatic relief and for prevention of chronic disease in women with premature menopause due to primary ovarian insufficiency or medical etiology. Estrogen and its metabolites are also protective against pulmonary hypertension (Frump et al., 2021). Tamoxifen, the estrogen analog, has an inhibitory effect on Zika virus replication, which reduces Zika virus infection (Grady et al., 2021). Bristol et al. (2020) found that estrogen can downregulate the expression of early genes (e.g., E6 and E7) by inhibiting the human papillomavirus transcriptional long control region, ultimately attenuating the growth of human papillomavirus-positive epithelial cells (Bristo et al., 2020).
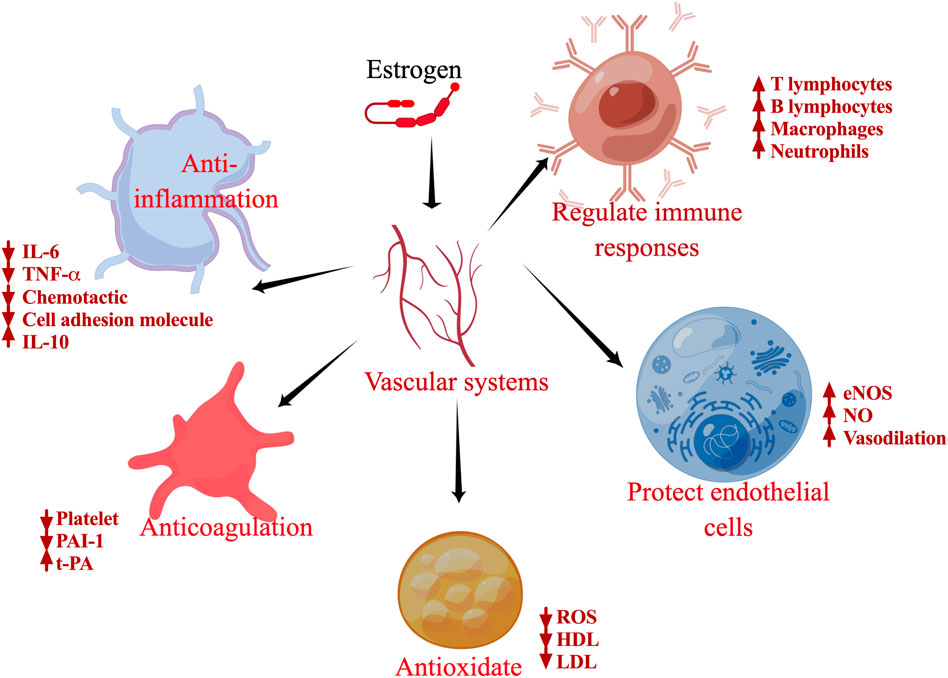
FIGURE 3. Schematic of the biological functions of estrogen. Specific effects of estrogen on inflammation, antioxidants, regulation of immune cells, antithrombosis, and protection of endothelial cells.
The applications of estrogen as an intervention strategy against COVID-19 are summarized in Table 1. Numerous studies have shown that transdermal estrogen therapy reduces the risk of death from COVID-19 in postmenopausal women (Seeland et al., 2020; Costeira et al., 2021; Sund et al., 2022), but for young premenopausal women, the risk of death from COVID-19 is the same regardless of estrogen therapy, probably because they have higher levels of endogenous estradiol (Seeland et al., 2020). A study on men treated with progesterone for COVID-19 showed that they required 3 days less supplemental oxygen and 2.5 days less hospital stay compared with a control group, suggesting a favorable effect of hormone therapy in men (Ghandehari et al., 2021). These findings may be applicable to the treatment of young female patients through personalized treatment strategy based on the level of estrogen in these patients, although the most efficacious estrogen dosage remains to be determined. Oral administration of estrogen is associated with a high risk of VTE, whereas subcutaneous therapy may have a slow onset and low bioavailability. The recently used nanoparticle (NP)-based drug delivery systems for the treatment of COVID-19 might provide a novel approach for estrogen therapy (Rahman et al., 2022; Rauf et al., 2022). However, the effective and efficient integration of estrogen into the NPS needs to be explored further. In addition, data on treatment duration and potential comorbidities are scarce, warranting further investigations.
Conclusion and future directions
COVID-19 has a high morbidity and mortality, which poses a serious burden on healthcare systems and economic development worldwide. Therefore, strategies for the prevention and treatment of COVID-19 are urgently required. The pathophysiological features of COVID-19 include inflammatory storms, immune dysregulation, oxidative stress, thrombus formation in small pulmonary vessels, injury of pulmonary artery endothelial cells, and pulmonary artery spasm, which can lead to acute respiratory distress syndrome, liver dysfunction, multiple organ failure, and eventually, death.
To summarize, estrogen can inhibit the entry of virus particles, mitigate viral replication by reducing ACE2 receptor expression in the host cells, and curtail the activities of main viral proteases. Retrospective and clinical studies have shown that estrogen is associated with improved outcomes in patients with COVID-19 through the modulation of immune responses; anti-inflammatory, antioxidant, and antithrombotic effects; and protection of endothelial cells. Several studies have demonstrated that low estrogen levels are associated with higher morbidity and mortality, which indicates that high estrogen levels have beneficial effects against COVID-19. Pharmacological compounds that can increase the levels of estrogen, such as tamoxifen and progesterone therapy, are potential treatments for patients with COVID-19 that are already in preclinical studies. Notably, many studies related to other viral infections also support the notion that increasing estrogen levels in patients with viral infections may be a viable therapeutic option. However, increasing estrogen levels alone is not apparently enough to restore estrogen function. Therefore, further studies on the composition, structure, and function of estrogen in COVID-19 are required to fully understand the mechanism of estrogen action in COVID-19, and thereby, devise new therapeutic strategies against this disease.
Author contributions
Z-PW wrote the first draft; MH, TJ, and R-LG reviewed the manuscript; ZB outlined and revised the manuscript.
Funding
The West Lighting program of Chinese Academy of Science in 2020 (2019-003); Applied and Basic Science Program, Department of Science and Technology, Qinghai Province, China (2020-ZJ-721).
Conflict of interest
The authors declare that the research was conducted in the absence of any commercial or financial relationships that could be construed as a potential conflict of interest.
Publisher’s note
All claims expressed in this article are solely those of the authors and do not necessarily represent those of their affiliated organizations, or those of the publisher, the editors and the reviewers. Any product that may be evaluated in this article, or claim that may be made by its manufacturer, is not guaranteed or endorsed by the publisher.
Abbreviations
COVID-19, coronavirus disease 2019; E2, 17β-Estradiol; E1, estrone; ERs, estrogen receptors; nER, nuclear estrogen receptor; mER, membrane estrogen receptor; ACE2, angiotensin-converting enzyme 2; TNF-α, tumor necrosis factor-α; IL, interleukins; ROS, reactive oxygen species; OS, oxidative stress; VTE, venous thromboembolism; NO, nitric oxide; HDL, high-density lipoprotein.
References
Ackermann, M., Verleden, S. E., Kuehnel, M., Haverich, A., Welte, T., Laenger, F., et al. (2020). Pulmonary vascular endothelialitis, thrombosis, and angiogenesis in Covid-19. N. Engl. J. Med. 383 (2), 120–128. doi:10.1056/NEJMoa2015432
Aksoy, A. N., Toker, A., Celık, M., Aksoy, M., Halıcı, Z., and Aksoy, H. (2014). The effect of progesterone on systemic inflammation and oxidative stress in the rat model of sepsis. Indian J. Pharmacol. 46 (6), 622–626. doi:10.4103/0253-7613.144922
Ally, A., Powell, I., Ally, M. M., Chaitoff, K., and Nauli, S. M. (2020). Role of neuronal nitric oxide synthase on cardiovascular functions in physiological and pathophysiological states. Nitric Oxide 102, 52–73. doi:10.1016/j.niox.2020.06.004
Alwani, M., Yassin, A., Al-Zoubi, R. M., Aboumarzouk, O. M., Nettleship, J., Kelly, D., et al. (2021). Sex-based differences in severity and mortality in COVID-19. Rev. Med. Virol. 31 (6), e2223. doi:10.1002/rmv.2223
Anagnostis, P., Bitzer, J., Cano, A., Ceausu, I., Chedraui, P., Durmusoglu, F., et al. (2020). Menopause symptom management in women with dyslipidemias: An EMAS clinical guide. Maturitas 135, 82–88. doi:10.1016/j.maturitas.2020.03.007
Anderson, G. L., Limacher, M., Assaf, A. R., Bassford, T., Beresford, S. A., Black, H., et al. (2004). Effects of conjugated equine estrogen in postmenopausal women with hysterectomy: The women's health initiative randomized controlled trial. JAMA 291 (14), 1701–1712. doi:10.1001/jama.291.14.1701
Arias-Loza, P. A., Muehlfelder, M., and Pelzer, T. (2013). Estrogen and estrogen receptors in cardiovascular oxidative stress. Pflugers Arch. 465 (5), 739–746. doi:10.1007/s00424-013-1247-7
Arnold, C. G., Libby, A., Vest, A., Hopkinson, A., and Monte, A. A. (2022). Immune mechanisms associated with sex-based differences in severe COVID-19 clinical outcomes. Biol. Sex. Differ. 13 (1), 7. doi:10.1186/s13293-022-00417-3
Barakat, R., Oakley, O., Kim, H., Jin, J., and Ko, C. J. (2016). Extra-gonadal sites of estrogen biosynthesis and function. BMB Rep. 49 (9), 488–496. doi:10.5483/bmbrep.2016.49.9.141
Baristaite, G., and Gurwitz, D. (2022). Estradiol reduces ACE2 and TMPRSS2 mRNA levels in A549 human lung epithelial cells. Drug Dev. Res. 83 (4), 961–966. doi:10.1002/ddr.21923
Barp, J., Araújo, A. S., Fernandes, T. R., Rigatto, K. V., Llesuy, S., Belló-Klein, A., et al. (2002). Myocardial antioxidant and oxidative stress changes due to sex hormones. Braz. J. Med. Biol. Res. 35 (9), 1075–1081. doi:10.1590/s0100-879x2002000900008
Bassi, G., Sidhu, S. K., and Mishra, S. (2021). The expanding role of mitochondria, autophagy and lipophagy in steroidogenesis. Cells 10 (8), 1851. doi:10.3390/cells10081851
Biegon, A., Alia-Klein, N., Alexoff, D. L., Fowler, J. S., Kim, S. W., Logan, J., et al. (2020). Relationship of estrogen synthesis capacity in the brain with obesity and self-control in men and women. Proc. Natl. Acad. Sci. U. S. A. 117 (37), 22962–22966. doi:10.1073/pnas.2006117117
Brann, D. W., Lu, Y., Wang, J., Zhang, Q., Thakkar, R., Sareddy, G. R., et al. (2022). Brain-derived estrogen and neural function. Neurosci. Biobehav. Rev. 132, 793–817. doi:10.1016/j.neubiorev.2021.11.014
Breithaupt-Faloppa, A. C., Correia, C. J., Prado, C. M., Stilhano, R. S., Ureshino, R. P., et al. (2020). 17β-Estradiol, a potential ally to alleviate SARS-CoV-2 infection. Clin. (Sao Paulo) 75, e1980. doi:10.6061/clinics/2020/e1980
Bristol, M. L., James, C. D., Wang, X., Fontan, C. T., and Morgan, I. M. (2020). Estrogen attenuates the growth of human papillomavirus-positive epithelial cells. mSphere 5 (2), e00049. doi:10.1128/mSphere.00049-20
Cao, X. (2020). COVID-19: Immunopathology and its implications for therapy. Nat. Rev. Immunol. 20 (5), 269–270. doi:10.1038/s41577-020-0308-3
Carsana, L., Sonzogni, A., Nasr, A., Rossi, R. S., Pellegrinelli, A., Zerbi, P., et al. (2020). Pulmonary post-mortem findings in a series of COVID-19 cases from northern Italy: A two-centre descriptive study. Lancet. Infect. Dis. 20 (10), 1135–1140. doi:10.1016/s1473-3099(20)30434-5
Catenaccio, E., Mu, W., and Lipton, M. L. (2016). Estrogen- and progesterone-mediated structural neuroplasticity in women: Evidence from neuroimaging. Brain Struct. Funct. 221 (8), 3845–3867. doi:10.1007/s00429-016-1197-x
Chambers, J. P., Yu, J., Valdes, J. J., and Arulanandam, B. P. (2020). SARS-CoV-2, early entry events. J. Pathog. 2020, 9238696. doi:10.1155/2020/9238696
Channappanavar, R., Fett, C., Mack, M., Ten Eyck, P. P., Meyerholz, D. K., and Perlman, S. (2017). Sex-based differences in susceptibility to severe acute respiratory syndrome coronavirus infection. J. Immunol. 198 (10), 4046–4053. doi:10.4049/jimmunol.1601896
Chou, C. H., and Chen, M. J. (2018). The effect of steroid hormones on ovarian follicle development. Vitam. Horm. 107, 155–175. doi:10.1016/bs.vh.2018.01.013
Cooke, P. S., Nanjappa, M. K., Ko, C., Prins, G. S., and Hess, R. A. (2017). Estrogens in male physiology. Physiol. Rev. 97 (3), 995–1043. doi:10.1152/physrev.00018.2016
Costeira, R., Lee, K. A., Murray, B., Christiansen, C., Castillo-Fernandez, J., Ni Lochlainn, M., et al. (2021). Estrogen and COVID-19 symptoms: Associations in women from the COVID symptom study. PLoS One 16 (9), e0257051. doi:10.1371/journal.pone.0257051
Cui, J., Shen, Y., and Li, R. (2013). Estrogen synthesis and signaling pathways during aging: From periphery to brain. Trends Mol. Med. 19 (3), 197–209. doi:10.1016/j.molmed.2012.12.007
Dama, A., Baggio, C., Boscaro, C., Albiero, M., and Cignarella, A. (2021). Estrogen receptor functions and pathways at the vascular immune interface. Int. J. Mol. Sci. 22 (8), 4254. doi:10.3390/ijms22084254
Danzi, G. B., Loffi, M., Galeazzi, G., and Gherbesi, E. (2020). Acute pulmonary embolism and COVID-19 pneumonia: A random association? Eur. Heart J. 41 (19), 1858. doi:10.1093/eurheartj/ehaa254
Diebel, L. N., Wheaton, M., and Liberati, D. M. (2021). The protective role of estrogen on endothelial and glycocalyx barriers after shock conditions: A microfluidic study. Surgery 169 (3), 678–685. doi:10.1016/j.surg.2020.08.006
Docherty, C. K., Nilsen, M., and MacLean, M. R. (2019). Influence of 2-methoxyestradiol and sex on hypoxia-induced pulmonary hypertension and hypoxia-inducible factor-1-α. J. Am. Heart Assoc. 8 (5), e011628. doi:10.1161/jaha.118.011628
Elger, W., Wyrwa, R., Ahmed, G., Meece, F., Nair, H. B., Santhamma, B., et al. (2017). Estradiol prodrugs (EP) for efficient oral estrogen treatment and abolished effects on estrogen modulated liver functions. J. Steroid Biochem. Mol. Biol. 165, 305–311. doi:10.1016/j.jsbmb.2016.07.008
Ferrario, C. M., Jessup, J., Chappell, M. C., Averill, D. B., Brosnihan, K. B., Tallant, E. A., et al. (2005). Effect of angiotensin-converting enzyme inhibition and angiotensin II receptor blockers on cardiac angiotensin-converting enzyme 2. Circulation 111 (20), 2605–2610. doi:10.1161/circulationaha.104.510461
Ferretti, V. V., Klersy, C., Bruno, R., Cutti, S., and Nappi, R. E. (2022). Men with COVID-19 die. Women survive. Maturitas 158, 34–36. doi:10.1016/j.maturitas.2021.11.014
Flammer, A. J., Anderson, T., Celermajer, D. S., Creager, M. A., Deanfield, J., Ganz, P., et al. (2012). The assessment of endothelial function: From research into clinical practice. Circulation 126 (6), 753–767. doi:10.1161/circulationaha.112.093245
Förstermann, U., Xia, N., and Li, H. (2017). Roles of vascular oxidative stress and nitric oxide in the pathogenesis of atherosclerosis. Circ. Res. 120 (4), 713–735. doi:10.1161/circresaha.116.309326
Fox, S. W., and Chambers, T. J. (2006). The effect of oestrogen on megakaryocyte differentiation and platelet counts in vivo. Int. J. Cardiol. 109 (3), 359–366. doi:10.1016/j.ijcard.2005.06.037
Frump, A. L., Albrecht, M., Yakubov, B., Breuils-Bonnet, S., Nadeau, V., Tremblay, E., et al. (2021). 17β-Estradiol and estrogen receptor α protect right ventricular function in pulmonary hypertension via BMPR2 and apelin. J. Clin. Invest. 131 (6), 129433. doi:10.1172/jci129433
Fuentes, N., and Silveyra, P. (2019). Estrogen receptor signaling mechanisms. Adv. Protein Chem. Struct. Biol. 116, 135–170. doi:10.1016/bs.apcsb.2019.01.001
Fukami, M., and Ogata, T. (2022). Congenital disorders of estrogen biosynthesis and action. Best. Pract. Res. Clin. Endocrinol. Metab. 36 (1), 101580. doi:10.1016/j.beem.2021.101580
Galaris, D., Barbouti, A., and Pantopoulos, K. (2019). Iron homeostasis and oxidative stress: An intimate relationship. Biochim. Biophys. Acta. Mol. Cell Res. 1866 (12), 118535. doi:10.1016/j.bbamcr.2019.118535
Garvin, M. R., Alvarez, C., Miller, J. I., Prates, E. T., Walker, A. M., Amos, B. K., et al. (2020). A mechanistic model and therapeutic interventions for COVID-19 involving a RAS-mediated bradykinin storm. Elife 9, e59177. doi:10.7554/eLife.59177
Ghandehari, S., Matusov, Y., Pepkowitz, S., Stein, D., Kaderi, T., Narayanan, D., et al. (2021). Progesterone in addition to standard of care vs standard of care alone in the treatment of men hospitalized with moderate to severe COVID-19: A randomized, controlled pilot trial. Chest 160 (1), 74–84. doi:10.1016/j.chest.2021.02.024
Gomez, J. M. D., Du-Fay-de-Lavallaz, J. M., Fugar, S., Sarau, A., Simmons, J. A., Clark, B., et al. (2021). Sex differences in COVID-19 hospitalization and mortality. J. Womens Health (Larchmt) 30 (5), 646–653. doi:10.1089/jwh.2020.8948
Grady, S. F., Pinto, A. K., Hassert, M., D'Angelo, J. A., Brien, J. D., and Arnatt, C. K. (2021). Selective estrogen receptor modulator, tamoxifen, inhibits Zika virus infection. J. Med. Virol. 93 (11), 6155–6162. doi:10.1002/jmv.27230
Gu, H., Chen, X., Liu, X., Zhan, W., Lyu, Z., Yu, Q., et al. (2017). A hemocompatible polyurethane surface having dual fibrinolytic and nitric oxide generating functions. J. Mat. Chem. B 5 (5), 980–987. doi:10.1039/c6tb02735k
Guan, W. J., Liang, W. H., Zhao, Y., Liang, H. R., Chen, Z. S., Li, Y. M., et al. (2020). Comorbidity and its impact on 1590 patients with COVID-19 in China: A nationwide analysis. Eur. Respir. J. 55 (5), 2000547. doi:10.1183/13993003.00547-2020
Hajializadeh, Z., and Khaksari, M. (2022). The protective effects of 17-β estradiol and SIRT1 against cardiac hypertrophy: A review. Heart fail. Rev. 27 (2), 725–738. doi:10.1007/s10741-021-10171-0
Hamilton, K. J., Hewitt, S. C., Arao, Y., and Korach, K. S. (2017). Estrogen hormone biology. Curr. Top. Dev. Biol. 125, 109–146. doi:10.1016/bs.ctdb.2016.12.005
Han, Q., Lin, Q., Jin, S., and You, L. (2020). Coronavirus 2019-nCoV: A brief perspective from the front line. J. Infect. 80 (4), 373–377. doi:10.1016/j.jinf.2020.02.010
Heriyanto, R. S., Kurniawan, A., Wijovi, F., Halim, D. A., Jodhinata, C., Marcella, E., et al. (2021). The role of COVID-19 survivor status and gender towards neutralizing antibody titers 1, 2, 3 months after Sinovac vaccine administration on clinical-year medical students in Indonesia. Int. J. Infect. Dis. 113, 336–338. doi:10.1016/j.ijid.2021.10.009
Hood, K. Y., Montezano, A. C., Harvey, A. P., Nilsen, M., MacLean, M. R., and Touyz, R. M. (2016). Nicotinamide adenine dinucleotide phosphate oxidase-mediated redox signaling and vascular remodeling by 16α-hydroxyestrone in human pulmonary artery cells: Implications in pulmonary arterial hypertension. Hypertension 68 (3), 796–808. doi:10.1161/hypertensionaha.116.07668
Huang, C., Wang, Y., Li, X., Ren, L., Zhao, J., Hu, Y., et al. (2020). Clinical features of patients infected with 2019 novel coronavirus in Wuhan, China. Lancet 395 (10223), 497–506. doi:10.1016/s0140-6736(20)30183-5
Iorga, A., Cunningham, C. M., Moazeni, S., Ruffenach, G., Umar, S., and Eghbali, M. (2017). The protective role of estrogen and estrogen receptors in cardiovascular disease and the controversial use of estrogen therapy. Biol. Sex. Differ. 8 (1), 33. doi:10.1186/s13293-017-0152-8
Iravani, M., Lagerquist, M., Ohlsson, C., and Sävendahl, L. (2017). Regulation of bone growth via ligand-specific activation of estrogen receptor alpha. J. Endocrinol. 232 (3), 403–410. doi:10.1530/joe-16-0263
Islam, F., Bibi, S., Meem, A. F. K., Islam, M. M., Rahaman, M. S., Bepary, S., et al. (2021). Natural bioactive molecules: An alternative approach to the treatment and control of COVID-19. Int. J. Mol. Sci. 22 (23), 12638. doi:10.3390/ijms222312638
Jackson, C. B., Farzan, M., Chen, B., and Choe, H. (2022). Mechanisms of SARS-CoV-2 entry into cells. Nat. Rev. Mol. Cell Biol. 23 (1), 3–20. doi:10.1038/s41580-021-00418-x
Jamal, M., Bangash, H. I., Habiba, M., Lei, Y., Xie, T., Sun, J., et al. (2021). Immune dysregulation and system pathology in COVID-19. Virulence 12 (1), 918–936. doi:10.1080/21505594.2021.1898790
Joffre, J., Hellman, J., Ince, C., and Ait-Oufella, H. (2020). Endothelial responses in sepsis. Am. J. Respir. Crit. Care Med. 202 (3), 361–370. doi:10.1164/rccm.201910-1911TR
Kander, M. C., Cui, Y., and Liu, Z. (2017). Gender difference in oxidative stress: A new look at the mechanisms for cardiovascular diseases. J. Cell. Mol. Med. 21 (5), 1024–1032. doi:10.1111/jcmm.13038
Karkhanei, B., Talebi Ghane, E., and Mehri, F. (2021). Evaluation of oxidative stress level: Total antioxidant capacity, total oxidant status and glutathione activity in patients with COVID-19. New Microbes New Infect. 42, 100897. doi:10.1016/j.nmni.2021.100897
Ke, Z., Oton, J., Qu, K., Cortese, M., Zila, V., McKeane, L., et al. (2020). Structures and distributions of SARS-CoV-2 spike proteins on intact virions. Nature 588 (7838), 498–502. doi:10.1038/s41586-020-2665-2
Kerr, R., Stirling, D., and Ludlam, C. A. (2001). Interleukin 6 and haemostasis. Br. J. Haematol. 115 (1), 3–12. doi:10.1046/j.1365-2141.2001.03061.x
Khomich, O. A., Kochetkov, S. N., Bartosch, B., and Ivanov, A. V. (2018). Redox biology of respiratory viral infections. Viruses 10 (8), E392. doi:10.3390/v10080392
Kim, S. Y. (2022). Oxidative stress and gender disparity in cancer. Free Radic. Res. 56 (1), 90–105. doi:10.1080/10715762.2022.2038789
Kiyama, R., and Wada-Kiyama, Y. (2015). Estrogenic endocrine disruptors: Molecular mechanisms of action. Environ. Int. 83, 11–40. doi:10.1016/j.envint.2015.05.012
Knowlton, A. A., and Lee, A. R. (2012). Estrogen and the cardiovascular system. Pharmacol. Ther. 135 (1), 54–70. doi:10.1016/j.pharmthera.2012.03.007
Ko, S. H., and Kim, H. S. (2020). Menopause-associated lipid metabolic disorders and foods beneficial for postmenopausal women. Nutrients 12 (1), E202. doi:10.3390/nu12010202
Kočar, E., Režen, T., and Rozman, D. (2021). Cholesterol, lipoproteins, and COVID-19: Basic concepts and clinical applications. Biochim. Biophys. Acta. Mol. Cell Biol. Lipids 1866 (2), 158849. doi:10.1016/j.bbalip.2020.158849
Kovats, S. (2015). Estrogen receptors regulate innate immune cells and signaling pathways. Cell. Immunol. 294 (2), 63–69. doi:10.1016/j.cellimm.2015.01.018
Kowsar, R., Hambruch, N., Marey, M. A., Liu, J., Shimizu, T., Pfarrer, C., et al. (2014). Evidence for a novel, local acute-phase response in the bovine oviduct: Progesterone and lipopolysaccharide up-regulate alpha 1-acid-glycoprotein expression in epithelial cells in vitro. Mol. Reprod. Dev. 81 (9), 861–870. doi:10.1002/mrd.22355
Kuiper, G. G., Enmark, E., Pelto-Huikko, M., Nilsson, S., and Gustafsson, J. A. (1996). Cloning of a novel receptor expressed in rat prostate and ovary. Proc. Natl. Acad. Sci. U. S. A. 93 (12), 5925–5930. doi:10.1073/pnas.93.12.5925
Lahm, T., Albrecht, M., Fisher, A. J., Selej, M., Patel, N. G., Brown, J. A., et al. (2012). 17β-Estradiol attenuates hypoxic pulmonary hypertension via estrogen receptor-mediated effects. Am. J. Respir. Crit. Care Med. 185 (9), 965–980. doi:10.1164/rccm.201107-1293OC
Laliberté, F., Dea, K., Duh, M. S., Kahler, K. H., Rolli, M., and Lefebvre, P. (2018). Does the route of administration for estrogen hormone therapy impact the risk of venous thromboembolism? Estradiol transdermal system versus oral estrogen-only hormone therapy. Menopause 25 (11), 1297–1305. doi:10.1097/gme.0000000000001232
Lee, C., Kim, J., and Jung, Y. (2019). Potential therapeutic application of estrogen in gender disparity of nonalcoholic fatty liver disease/nonalcoholic steatohepatitis. Cells 8 (10), E1259. doi:10.3390/cells8101259
Lee, A., and Syed, Y. Y. (2022). Estetrol/Drospirenone: A Review in Oral Contraception. Drugs 82 (10), 1117–1125. doi:10.1007/s40265-022-01738-8
Lemes, R. M. R., Costa, A. J., Bartolomeo, C. S., Bassani, T. B., Nishino, M. S., Pereira, G., et al. (2021). 17β-estradiol reduces SARS-CoV-2 infection in vitro. Physiol. Rep. 9 (2), e14707. doi:10.14814/phy2.14707
Levi, M., and van der Poll, T. (2017). Coagulation and sepsis. Thromb. Res. 149, 38–44. doi:10.1016/j.thromres.2016.11.007
Li, F., Boon, A. C. M., Michelson, A. P., Foraker, R. E., Zhan, M., and Payne, P. R. O. (2022). Estrogen hormone is an essential sex factor inhibiting inflammation and immune response in COVID-19. Sci. Rep. 12 (1), 9462. doi:10.1038/s41598-022-13585-4
Luo, T., and Kim, J. K. (2016). The role of estrogen and estrogen receptors on cardiomyocytes: An overview. Can. J. Cardiol. 32 (8), 1017–1025. doi:10.1016/j.cjca.2015.10.021
Mallareddy, M., Hanes, V., and White, W. B. (2007). Drospirenone, a new progestogen, for postmenopausal women with hypertension. Drugs Aging 24 (6), 453–466. doi:10.2165/00002512-200724060-00002
Manca, P., Caria, M. A., Blasi, J., Martín-Satué, M., and Mameli, O. (2012). Cytochrome P450 17α-hydroxylase/C(17, 20)-lyase immunoreactivity and molecular expression in the cerebellar nuclei of adult male rats. J. Chem. Neuroanat. 45 (1-2), 18–25. doi:10.1016/j.jchemneu.2012.07.002
Mansouri, A., Kowsar, R., Zakariazadeh, M., Hakimi, H., and Miyamoto, A. (2022). The impact of calcitriol and estradiol on the SARS-CoV-2 biological activity: A molecular modeling approach. Sci. Rep. 12 (1), 717. doi:10.1038/s41598-022-04778-y
Martini, R. (2020). The compelling arguments for the need of microvascular investigation in COVID-19 critical patients. Clin. Hemorheol. Microcirc. 75 (1), 27–34. doi:10.3233/ch-200895
Mateus, D., Sebastião, A. I., Carrascal, M. A., Carmo, A. D., Matos, A. M., and Cruz, M. T. (2022). Crosstalk between estrogen, dendritic cells, and SARS-CoV-2 infection. Rev. Med. Virol. 32 (3), e2290. doi:10.1002/rmv.2290
Medzikovic, L., Aryan, L., and Eghbali, M. (2019). Connecting sex differences, estrogen signaling, and microRNAs in cardiac fibrosis. J. Mol. Med. Berl. 97 (10), 1385–1398. doi:10.1007/s00109-019-01833-6
Mishra, J. S., Te Riele, G. M., Qi, Q. R., Lechuga, T. J., Gopalakrishnan, K., Chen, D. B., et al. (2019). Estrogen receptor-β mediates estradiol-induced pregnancy-specific uterine artery endothelial cell angiotensin type-2 receptor expression. Hypertension 74 (4), 967–974. doi:10.1161/hypertensionaha.119.13429
Mohamed, M. S., Moulin, T. C., and Schiöth, H. B. (2021). Sex differences in COVID-19: The role of androgens in disease severity and progression. Endocrine 71 (1), 3–8. doi:10.1007/s12020-020-02536-6
Monteil, V., Kwon, H., Prado, P., Hagelkrüys, A., Wimmer, R. A., Stahl, M., et al. (2020). Inhibition of SARS-CoV-2 infections in engineered human tissues using clinical-grade soluble human ACE2. Cell 181 (4), 905–913. doi:10.1016/j.cell.2020.04.004
Nii, S., Shinohara, K., Matsushita, H., Noguchi, Y., Watanabe, K., and Wakatsuki, A. (2016). Hepatic effects of estrogen on plasma distribution of small dense low-density lipoprotein and free radical production in postmenopausal women. J. Atheroscler. Thromb. 23 (7), 810–818. doi:10.5551/jat.33175
Palmisano, B. T., Zhu, L., Eckel, R. H., and Stafford, J. M. (2018). Sex differences in lipid and lipoprotein metabolism. Mol. Metab. 15, 45–55. doi:10.1016/j.molmet.2018.05.008
Park, J. S., Lee, G. H., Jin, S. W., Pham, T. H., Thai, T. N., Kim, J. Y., et al. (2021). G protein-coupled estrogen receptor regulates the KLF2-dependent eNOS expression by activating of Ca(2+) and EGFR signaling pathway in human endothelial cells. Biochem. Pharmacol. 192, 114721. doi:10.1016/j.bcp.2021.114721
Pedram, A., Razandi, M., O'Mahony, F., Lubahn, D., and Levin, E. R. (2010). Estrogen receptor-beta prevents cardiac fibrosis. Mol. Endocrinol. 24 (11), 2152–2165. doi:10.1210/me.2010-0154
Pelekanou, V., Kampa, M., Kiagiadaki, F., Deli, A., Theodoropoulos, P., Agrogiannis, G., et al. (2016). Estrogen anti-inflammatory activity on human monocytes is mediated through cross-talk between estrogen receptor ERα36 and GPR30/GPER1. J. Leukoc. Biol. 99 (2), 333–347. doi:10.1189/jlb.3A0914-430RR
Pernazza, A., Mancini, M., Rullo, E., Bassi, M., De Giacomo, T., Rocca, C. D., et al. (2020). Early histologic findings of pulmonary SARS-CoV-2 infection detected in a surgical specimen. Virchows Arch. 477 (5), 743–748. doi:10.1007/s00428-020-02829-1
Pignatelli, P., Menichelli, D., Pastori, D., and Violi, F. (2018). Oxidative stress and cardiovascular disease: New insights. Kardiol. Pol. 76 (4), 713–722. doi:10.5603/KP.a2018.0071
Qin, C., Zhou, L., Hu, Z., Zhang, S., Yang, S., Tao, Y., et al. (2020). Dysregulation of immune response in patients with coronavirus 2019 (COVID-19) in Wuhan, China. Clin. Infect. Dis. 71 (15), 762–768. doi:10.1093/cid/ciaa248
Rahman, M. M., Ahmed, M., Islam, M. T., Khan, M. R., Sultana, S., Maeesa, S. K., et al. (2022). Nanotechnology-based approaches and investigational therapeutics against COVID-19. Curr. Pharm. Des. 28 (12), 948–968. doi:10.2174/1381612827666210701150315
Rahman, M. M., Islam, M. R., Shohag, S., Hossain, M. E., Shah, M., Shuvo, S. K., et al. (2022). Multifaceted role of natural sources for COVID-19 pandemic as marine drugs. Environ. Sci. Pollut. Res. Int. 29 (31), 46527–46550. doi:10.1007/s11356-022-20328-5
Rauf, A., Abu-Izneid, T., Khalil, A. A., Hafeez, N., Olatunde, A., Rahman, M., et al. (2022). Nanoparticles in clinical trials of COVID-19: An update. Int. J. Surg. 104, 106818. doi:10.1016/j.ijsu.2022.106818
Reckelhoff, J. F., Romero, D. G., and Yanes Cardozo, L. L. (2019). Sex, oxidative stress, and hypertension: Insights from animal models. Physiol. (Bethesda) 34 (3), 178–188. doi:10.1152/physiol.00035.2018
Rettberg, J. R., Yao, J., and Brinton, R. D. (2014). Estrogen: A master regulator of bioenergetic systems in the brain and body. Front. Neuroendocrinol. 35 (1), 8–30. doi:10.1016/j.yfrne.2013.08.001
Robert, J., Osto, E., and von Eckardstein, A. (2021). The endothelium is both a target and a barrier of HDL's protective functions. Cells 10 (5), 1041. doi:10.3390/cells10051041
Russell, J. K., Jones, C. K., and Newhouse, P. A. (2019). The role of estrogen in brain and cognitive aging. Neurotherapeutics 16 (3), 649–665. doi:10.1007/s13311-019-00766-9
Saavedra, J. M. (2021). Angiotensin receptor blockers are not just for hypertension anymore. Physiol. (Bethesda) 36 (3), 160–173. doi:10.1152/physiol.00036.2020
Sánchez-Guijo, A., Neunzig, J., Gerber, A., Oji, V., Hartmann, M. F., Schuppe, H. C., et al. (2016). Role of steroid sulfatase in steroid homeostasis and characterization of the sulfated steroid pathway: Evidence from steroid sulfatase deficiency. Mol. Cell. Endocrinol. 437, 142–153. doi:10.1016/j.mce.2016.08.019
Scarabin, P. Y., Oger, E., and Plu-Bureau, G. (2003). Differential association of oral and transdermal oestrogen-replacement therapy with venous thromboembolism risk. Lancet 362 (9382), 428–432. doi:10.1016/s0140-6736(03)14066-4
Schönrich, G., Raftery, M. J., and Samstag, Y. (2020). Devilishly radical NETwork in COVID-19: Oxidative stress, neutrophil extracellular traps (NETs), and T cell suppression. Adv. Biol. Regul. 77, 100741. doi:10.1016/j.jbior.2020.100741
Seeland, U., Coluzzi, F., Simmaco, M., Mura, C., Bourne, P. E., Heiland, M., et al. (2020). Evidence for treatment with estradiol for women with SARS-CoV-2 infection. BMC Med. 18 (1), 369. doi:10.1186/s12916-020-01851-z
Shi, Y., Wang, Y., Shao, C., Huang, J., Gan, J., Huang, X., et al. (2020). COVID-19 infection: The perspectives on immune responses. Cell Death Differ. 27 (5), 1451–1454. doi:10.1038/s41418-020-0530-3
Stukalov, A., Girault, V., Grass, V., Karayel, O., Bergant, V., Urban, C., et al. (2021). Multilevel proteomics reveals host perturbations by SARS-CoV-2 and SARS-CoV. Nature 594 (7862), 246–252. doi:10.1038/s41586-021-03493-4
Suba, Z. (2020). Prevention and therapy of COVID-19 via exogenous estrogen treatment for both male and female patients. J. Pharm. Pharm. Sci. 23 (1), 75–85. doi:10.18433/jpps31069
Sun, Z., Wang, L., Li, X., Fan, C., Xu, J., Shi, Z., et al. (2022). An extended conformation of SARS-CoV-2 main protease reveals allosteric targets. Proc. Natl. Acad. Sci. U. S. A. 119 (15), e2120913119. doi:10.1073/pnas.2120913119
Sund, M., Fonseca-Rodríguez, O., Josefsson, A., Welen, K., and Fors Connolly, A. M. (2022). Association between pharmaceutical modulation of oestrogen in postmenopausal women in Sweden and death due to COVID-19: A cohort study. BMJ Open 12 (2), e053032. doi:10.1136/bmjopen-2021-053032
Tang, N., Li, D., Wang, X., and Sun, Z. (2020). Abnormal coagulation parameters are associated with poor prognosis in patients with novel coronavirus pneumonia. J. Thromb. Haemost. 18 (4), 844–847. doi:10.1111/jth.14768
Tang, Z. R., Zhang, R., Lian, Z. X., Deng, S. L., and Yu, K. (2019). Estrogen-receptor expression and function in female reproductive disease. Cells 8 (10), E1123. doi:10.3390/cells8101123
Teuwen, L. A., Geldhof, V., Pasut, A., and Carmeliet, P. (2020). COVID-19: The vasculature unleashed. Nat. Rev. Immunol. 20 (7), 389–391. doi:10.1038/s41577-020-0343-0
Thomas, C., and Gustafsson, J. (2011). The different roles of ER subtypes in cancer biology and therapy. Nat. Rev. Cancer 11 (8), 597–608. doi:10.1038/nrc3093
Tsialtas, I., Georgantopoulos, A., Karipidou, M. E., Kalousi, F. D., Karra, A. G., Leonidas, D. D., et al. (2021). Anti-apoptotic and antioxidant activities of the mitochondrial estrogen receptor beta in N2A neuroblastoma cells. Int. J. Mol. Sci. 22 (14), 7620. doi:10.3390/ijms22147620
Umar, S., Iorga, A., Matori, H., Nadadur, R. D., Li, J., Maltese, F., et al. (2011). Estrogen rescues preexisting severe pulmonary hypertension in rats. Am. J. Respir. Crit. Care Med. 184 (6), 715–723. doi:10.1164/rccm.201101-0078OC
Uwamino, Y., Kurafuji, T., Sato, Y., Tomita, Y., Shibata, A., Tanabe, A., et al. (2022). Young age, female sex, and presence of systemic adverse reactions are associated with high post-vaccination antibody titer after two doses of BNT162b2 mRNA SARS-CoV-2 vaccination: An observational study of 646 Japanese healthcare workers and University staff. Vaccine 40 (7), 1019–1025. doi:10.1016/j.vaccine.2022.01.002
Valéra, M. C., Gratacap, M. P., Gourdy, P., Lenfant, F., Cabou, C., Toutain, C. E., et al. (2012). Chronic estradiol treatment reduces platelet responses and protects mice from thromboembolism through the hematopoietic estrogen receptor α. Blood 120 (8), 1703–1712. doi:10.1182/blood-2012-01-405498
Valéra, M. C., Noirrit-Esclassan, E., Dupuis, M., Buscato, M., Vinel, A., Guillaume, M., et al. (2017). Effect of chronic estradiol plus progesterone treatment on experimental arterial and venous thrombosis in mouse. PLoS One 12 (5), e0177043. doi:10.1371/journal.pone.0177043
Valéra, M. C., Parant, O., Cenac, C., Arnaud, C., Gallini, A., Hamdi, S., et al. (2015). Platelet adhesion and thrombus formation in whole blood at arterial shear rate at the end of pregnancy. Am. J. Reprod. Immunol. 74 (6), 533–541. doi:10.1111/aji.12433
Varga, Z., Flammer, A. J., Steiger, P., Haberecker, M., Andermatt, R., Zinkernagel, A. S., et al. (2020). Endothelial cell infection and endotheliitis in COVID-19. Lancet 395 (10234), 1417–1418. doi:10.1016/s0140-6736(20)30937-5
Vasquez, Y. M. (2018). Estrogen-regulated transcription: Mammary gland and uterus. Steroids 133, 82–86. doi:10.1016/j.steroids.2017.12.014
Verdecchia, P., Cavallini, C., Spanevello, A., and Angeli, F. (2020). The pivotal link between ACE2 deficiency and SARS-CoV-2 infection. Eur. J. Intern. Med. 76, 14–20. doi:10.1016/j.ejim.2020.04.037
Wang, D., Hu, B., Hu, C., Zhu, F., Liu, X., Zhang, J., et al. (2020). Clinical characteristics of 138 hospitalized patients with 2019 novel coronavirus-infected pneumonia in Wuhan, China. JAMA 323 (11), 1061–1069. doi:10.1001/jama.2020.1585
Wang, G., Deng, J., Li, J., Wu, C., Dong, H., Wu, S., et al. (2021). The role of high-density lipoprotein in COVID-19. Front. Pharmacol. 12, 720283. doi:10.3389/fphar.2021.720283
Wang, J., Hou, Y., Zhang, L., Liu, M., Zhao, J., Zhang, Z., et al. (2021). Estrogen attenuates traumatic brain injury by inhibiting the activation of microglia and astrocyte-mediated neuroinflammatory responses. Mol. Neurobiol. 58 (3), 1052–1061. doi:10.1007/s12035-020-02171-2
Wassmann, S., Laufs, U., Stamenkovic, D., Linz, W., Stasch, J. P., Ahlbory, K., et al. (2002). Raloxifene improves endothelial dysfunction in hypertension by reduced oxidative stress and enhanced nitric oxide production. Circulation 105 (17), 2083–2091. doi:10.1161/01.cir.0000014618.91633.67
Wu, Z., Harrich, D., Li, Z., Hu, D., and Li, D. (2021). The unique features of SARS-CoV-2 transmission: Comparison with SARS-CoV, MERS-CoV and 2009 H1N1 pandemic influenza virus. Rev. Med. Virol. 31 (2), e2171. doi:10.1002/rmv.2171
Xiang, J., Liu, X., Ren, J., Chen, K., Wang, H. L., Miao, Y. Y., et al. (2019). How does estrogen work on autophagy? Autophagy 15 (2), 197–211. doi:10.1080/15548627.2018.1520549
Xiong, Y., Liu, Y., Cao, L., Wang, D., Guo, M., Jiang, A., et al. (2020). Transcriptomic characteristics of bronchoalveolar lavage fluid and peripheral blood mononuclear cells in COVID-19 patients. Emerg. Microbes Infect. 9 (1), 761–770. doi:10.1080/22221751.2020.1747363
Xu, Z., Shi, L., Wang, Y., Zhang, J., Huang, L., Zhang, C., et al. (2020). Pathological findings of COVID-19 associated with acute respiratory distress syndrome. Lancet. Respir. Med. 8 (4), 420–422. doi:10.1016/s2213-2600(20)30076-x
Yazawa, T., Imamichi, Y., Uwada, J., Sekiguchi, T., Mikami, D., Kitano, T., et al. (2020). Evaluation of 17β-hydroxysteroid dehydrogenase activity using androgen receptor-mediated transactivation. J. Steroid Biochem. Mol. Biol. 196, 105493. doi:10.1016/j.jsbmb.2019.105493
Yazğan, B., Yazğan, Y., Övey, İ. S., and Nazıroğlu, M. (2016). Raloxifene and tamoxifen reduce PARP activity, cytokine and oxidative stress levels in the brain and blood of ovariectomized Rats. J. Mol. Neurosci. 60 (2), 214–222. doi:10.1007/s12031-016-0785-9
Yoon, B. K., Kang, Y. H., Oh, W. J., Lee, D. Y., Kim, D. K., Kessel, B., et al. (2020). Effects of 17β-estradiol on the plasminogen activator system in vascular smooth muscle cells treated with lysophophatidylcholine. J. Menopausal Med. 26 (1), 9–17. doi:10.6118/jmm.19005
Young, B. E., Ong, S. W. X., Kalimuddin, S., Low, J. G., Tan, S. Y., Loh, J., et al. (2020). Epidemiologic features and clinical course of patients infected with SARS-CoV-2 in Singapore. JAMA 323 (15), 1488–1494. doi:10.1001/jama.2020.3204
Zhou, F., Yu, T., Du, R., Fan, G., Liu, Y., Liu, Z., et al. (2020). Clinical course and risk factors for mortality of adult inpatients with COVID-19 in wuhan, China: A retrospective cohort study. Lancet 395 (10229), 1054–1062. doi:10.1016/s0140-6736(20)30566-3
Keywords: COVID-19, SARS-CoV-2, estrogen, estrogen therapy, prognosis, epidemic, immune response
Citation: Wang Z-P, Hua M, Jiu T, Ge R-L and Bai Z (2022) Biofunctional roles of estrogen in coronavirus disease 2019: Beyond a steroid hormone. Front. Pharmacol. 13:1003469. doi: 10.3389/fphar.2022.1003469
Received: 28 July 2022; Accepted: 06 October 2022;
Published: 19 October 2022.
Edited by:
Abdur Rauf, University of Swabi, PakistanReviewed by:
Md. Mominur Rahman, Daffodil International University, BangladeshAnees Ahmed Khalil, University of Lahore, Pakistan
Copyright © 2022 Wang, Hua, Jiu, Ge and Bai. This is an open-access article distributed under the terms of the Creative Commons Attribution License (CC BY). The use, distribution or reproduction in other forums is permitted, provided the original author(s) and the copyright owner(s) are credited and that the original publication in this journal is cited, in accordance with accepted academic practice. No use, distribution or reproduction is permitted which does not comply with these terms.
*Correspondence: Zhenzhong Bai, tibetannanka@hotmail.com, baizz@126.com