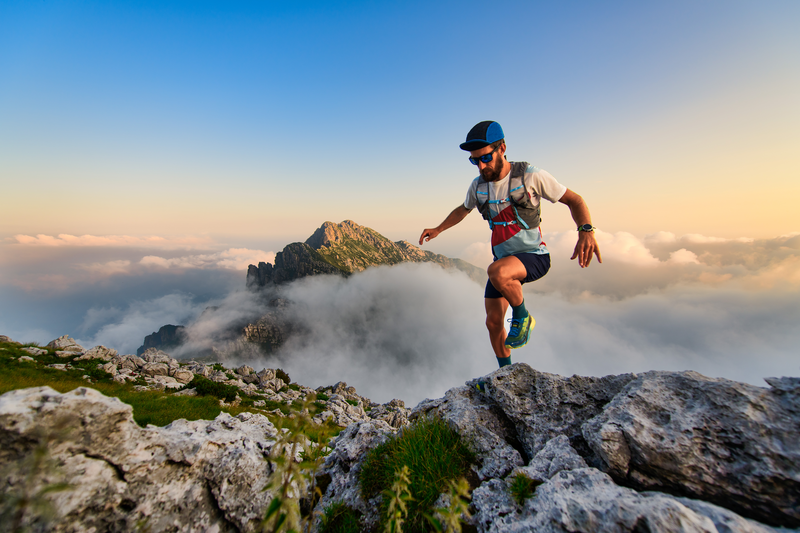
94% of researchers rate our articles as excellent or good
Learn more about the work of our research integrity team to safeguard the quality of each article we publish.
Find out more
ORIGINAL RESEARCH article
Front. Pharmacol. , 09 September 2022
Sec. Ethnopharmacology
Volume 13 - 2022 | https://doi.org/10.3389/fphar.2022.1003264
This article is part of the Research Topic New Plant-Based Therapeutics against Cancer and Associated Complications View all 4 articles
Background: There is abundant ethnopharmacological evidence the uses of regarding Solanum species as antitumor and anticancer agents. Glycoalkaloids are among the molecules with antiproliferative activity reported in these species.
Purpose: To evaluate the anticancer effect of the Solanum glycoalkaloid tomatine in hepatocellular carcinoma (HCC) in vitro (HepG2 cells) and in vivo models.
Methods: The resazurin reduction assay was performed to detect the effect of tomatine on cell viability in human HepG2 cell lines. Programmed cell death was investigated by means of cellular apoptosis assays using Annexin V. The expression of cancer related proteins was detected by Western blotting (WB). Reactive oxygen species (ROS) and calcium were determined by 2,7-dichlorodihydrofluorescein diacetate and Fluo-4, respectively. Intrahepatic HepG2 xenograft mouse model was used to elucidate the effect of tomatine on tumor growth in vivo.
Results and Discussion: Tomatine reduced HepG2 cell viability and induced the early apoptosis phase of cell death, consistently with caspase-3, -7, Bcl-2 family, and P53 proteins activation. Furthermore, tomatine increased intracellular ROS and cytosolic Ca+2 levels. Moreover, the NSG mouse xenograft model showed that treating mice with tomatine inhibited HepG2 tumor growth.
Conclusion: Tomatine inhibits in vitro and in vivo HCC tumorigenesis in part via modulation of p53, Ca+2, and ROS signalling. Thus, the results suggest the potential cancer therapeutic use of tomatine in HCC patients.
Wild species of genus Solanum (Solanaceae) are characterized by the production of several types of glycoalkaloids (GA), among them tomatine. Tomatine has been detected in leaves and tubers of species of Solanum genus, endemic of western South America such as Solanum acaule Bitter., S. curtilobum Juz. and Bukasov, S. demissum Lindl., S. microdontum Bitter, S. polyadenium Greenm., and S. tarijense Hawkes, as well as only in the leaves of S. chomatophilum Bitter, S. raphanifolium Cárdenas and Hawkes, S. tuberosum ssp. andigena (Juz. and Bukasov) Hawkes (white), S. tuberosum ssp. andigena (Juz. and Bukasov) Hawkes (violet), and S. tubersoum ssp. andigena (Juz. and Bukasov) Hawkes (Distl and Wink, 2009). It also is found in S. brevidens L., S. commersonii Dun., S. etuberosum L., S. jamesii L., and S. pinnatisectum L. (Osman et al., 1978; Gregory et al., 1981; Van Gelder, 1985). There is abundant ethnopharmacological evidence regarding the uses of these species as antitumor and anticancer agents (Kaunda and Zhang, 2019). Tomatine, one of the glycoalkaloids in Solanum species, has beneficial bioactivities, including antibiotic, anti-inflammatory, antioxidant, antimalarial, antifungal, cholesterol-lowering, cardiovascular, and immunological effects (Friedman, 2013).
Recently published evidence suggests that tomatine presents antitumor activity, as demonstrated by several in vitro and in vivo studies (Faria-Silva et al., 2022). For instance, in vitro studies have shown that tomatine is highly effective in inhibiting the growth of human cancer cell lines of the breast (Choi et al., 2012; Shi et al., 2013), colon (Kim et al., 2015; Rudolf and Rudolf, 2016), gastric (Xiuwei et al., 2007; Choi et al., 2012), hepatocellular (Xiuwei et al., 2007; Del Giudice et al., 2015), prostate (Choi et al., 2012; Huang et al., 2015), and ovarian (Wu et al., 2021) among others. At the same time, in vivo experiments indicate carcinomas that tomatine showed great antitumor potential in mice mammary adenocarcinoma (Tomsik et al., 2013), and HL60 xenograft tumor (Chao et al., 2012); in rainbow trout stomach tumors (Friedman et al., 2007); and subcutaneous and orthotopic xenograft tumors of human prostate cancer PC-3 cells (Lee et al., 2013a; Lee et al., 2013b, Lee et al., 2022).
The incidence and mortality of hepatocellular carcinoma (HCC) have increased in North America and several European regions and declined in traditionally high-risk regions, including Japan and parts of China (Kulik and El-Serag, 2019). Specifically, the in vitro cytotoxic effect of tomatine in HepG2 cells produced an inhibition with an IC50 of 43 μg/ml (Friedman, 2013). Correspondingly, tomatine, in a range of 0.1–100 μg/ml, has proven to be a potent dose-dependent inhibitor, reducing the growth of liver cancer HepG2 cell lines from 46.3 to 89.2%. Also, 1 μg/ml of tomatine showed better anticarcinogenic activity against human liver cancer cells than doxorubicin (Lee et al., 2004). Moreover, cytotoxicity analysis of α-tomatine showed that it induced a significant cytotoxic effect on both normal liver cells and liver cancer cells (Del Giudice et al., 2015). In turn, in vivo analysis support confirm the health-promoting effects of tomatine in animal models (Friedman, 2013). For instance, long-term experiments in rainbow trout showed that administration of tomatine (2000 ppm) and dibenzo [a,l]pyrene (DBP) (224 ppm) reduced incidence of liver tumors the induce by DBP (Friedman et al., 2007).
Although tomatine exerts antitumor functions on hepatic tumor cells, the molecular and biological mechanisms are not well elucidated so far. Also, the tomatine in vivo antitumor activity has not yet been addressed in mammalian models. This study aims to 1) determine whether tomatine induces HCC cell death 2) elucidate the involved molecular pathways that may explain the antiproliferative activity and, 3) assess is inhibited whether HCC cell tumor growth in a xenograft animal model.
Using HepG2 cells as the HCC model, the results indicated that tomatine induced inhibition of cell proliferation and promoted apoptosis mainly via regulation of apoptotic mediators and p53 activation. These results were along with the increased intracellular reactive oxygen species (ROS) and cytosolic Ca+2. Also, tomatine reduced HepG2 tumor growth in a mice xenograft model, suggesting this agent’s potential use in anticancer therapies.
Tomatine (purity >98%; CAS: 17406-45-0) was purchased from Sigma Chemical Co. (St. Louis, MO, United States). Doxorubicin hydrochloride (CAS: 25316-40-9), ionomycin (CAS: 56092-81-0), resazurin (7-hydroxy-10-oxidophenoxazin-10-ium-3-one; CAS: 550-82-3) and 2,7-dichlorodihydrofluorescein diacetate (DCFH-DA; CAS: 4091-99-0) were purchased from Sigma-Aldrich (Santiago, Chile). The Fluo-3AM (CAS: 121714-22-5) was obtained from Biovision (San Francisco, CA). All other reagents were of analytical grade and were purchased from Sigma (United States) and Merck (Germany).
The human hepatocellular carcinoma HepG2 cell line (American Type Culture Collection HB-8065) was cultured with Dulbecco’s Modified Eagle Medium (DMEM) supplemented with 10% fetal bovine serum (FBS) (Gibco, NY, United States) and antibiotic-antimycotic mixture (Gibco, NY, United States) at 37°C in a humidified 5% CO2 incubator.
HepG2 viability was determined using the resazurin assay as previously described (Riss et al., 2017). Briefly, exponentially growing cells were seeded in 96 well plates in a density of 5 × 103 cells/well and incubated with 100 ml per well culture media overnight. Then, culture media was replaced with 100 ml fresh media containing tomatine treatments at indicated concentrations. After 24 h of treatment, 20 ml resazurin at a concentration of 0.15 mg/ml in PBS was added to each of the wells and then incubated at 37°C for an additional 4 h. Next, the absorbance signal was quickly measured at 570/600 nm (excitation/emission wavelengths).
To determine the capacity of tomatine to induce cellular apoptosis, the activity of the key effector caspases 3 and 7 by a Caspase-Glo 3/7 assay (Promega Madison, WI, United States) was determined. Cells were seeded at a density of 2000 cells/well in 96-well white-walled plates. The next day, cells were treated with 5 µM of tomatine. Cleaved caspase 3/7 activity was assessed after 4 and 8 h. Caspase-Glo reagent was added into the wells, carefully mixed, and incubated in the dark for 90 min at room temperature. Absorbance and luminescence signals were measured using a microplate reader (Tecan infinite® M200 Pro, Männedorf, Switzerland). Each treatment was read in at least four replicates.
Furthermore, Apoptosis was evaluated through flow cytometry analysis by detecting extracellular membrane phosphatidylserine expression on early apoptotic cells using “Alexa Fluor 488 Annexin V/Dead Cell Apoptosis” Kit; the protocol was carried out as described by the manufacturer’s instructions (Invitrogen, Carlsbad, CA, United States). Cells were analyzed in a flow cytometer model BD FACS Canto II (BD Biosciences, United States), and 10,000 events were analyzed by sample.
The western blot method used was previously described by our research group (Echeverría et al., 2015). For a detailed list of antibodies used, see Supplementary Table S1.
The immunofluorescence assay was conducted as previously reported by our research group (Echeverría et al., 2015). The antibodies’ specific details are described in Supplementary Table S2. A microscopy EVOS® FLoid® cell (Life Technologies, Carls-bad, CA, United States) was used to record and analyze immune-stained cells.
HepG2 cells with or without treatment were subjected to a DCFH-DA assay to determine the intracellular ROS levels. The generated intracellular DCF fluorescence was quantified using a fluorescence plate reader (Tecan infinite® M200pro). Intracellular ROS were expressed as relative fluorescence units (RFU).
Plated HepG2 cells were mounted in a perfusion chamber on the stage of an inverted microscope (Olympus IX-81, UPLFLN 40XO 40 x/1.3 oil-immersion objective), different tomatine concentrations (1-30 µM) were added with a continuous gravity-fed perfusion system. Fluorescence was collected using an sCMO-based imaging system (pco.edge 4.2) running µManager software (Edelstein et al., 2010). Cells were incubated with Fluo-4 (Molecular Probes, 1 μM) as described in (Moreno et al., 2015), and thoroughly washed with an external solution (mM): 100 NaCl, 5 KCl, 2 CaCl2, 1 MgCl2, 90 sorbitol, 5 glucose, and 10 HEPES (pH 7.4 adjusted with Tris-Base). Fluo-4 loaded cells were excited at 480 nm, and the fluorescence emission at 510 nm was collected and recorded at 2 Hz. At the end of each experiment, ionomycin (1 µM) was added to determine the cell viability (not shown). Signals were recorded, and the background intensity was subtracted using equivalent regions of interest (ROI) outside the cell. Fluo-4 results are expressed as normalized fluorescence (F/F0).
Immunodeficient NSG (The Jackson Laboratory, Bar Harbor, ME, United States) mice received autoclaved chow and acidified water and ad libitum were bred and housed in individually ventilated cages (NexGenTM, Allentown) under specific pathogen-free conditions and 12:12 photoperiod (less than 325 lux conditions) in The In vivo Tumor Biology Research Facility of the Centro Oncológico at the Universidad Católica del Maule (UCM). Animal health was monitored at least three times per week until the experiment ended, following a UCM-approved specific tumor burden scoring system. All procedures were performed in Class II A2 Biological Safety Cabinet. All animal experiments were approved by the Institutional Animal Care and Use Committee of the UCM folio: 2018-03-C, date: 28 June 2018.
5 × 106 HepG2 cells in 100 µL in PBS were injected subcutaneously into the dorsal flank of 5 weeks old NOD scid gamma (NSG) mice under isoflurane anesthesia. All mice were weighed weekly, and tumor growth was monitored by a digital caliper to determine the tumor volume using the formula: (length x width2)/2 (Carlsson et al., 1983). Once tumors reached 80-100 mm3, mice were randomly assigned to different experimental groups (n = 6) and treated by intraperitoneal injection (100 µL) as follows: 5 and 20 mg/kg tomatine, according to previous reports (Lee et al., 2013b; Fan et al., 2014; Ma et al., 2015), and vehicle solution (PBS) twice a week. Mice were monitored daily during the treatment, and weight and tumor volume were determined twice a week.
Statistical values were evaluated by one-way analysis of variance (ANOVA), followed by Dunnett’s multiple comparisons tests and were expressed as mean ± standard error of the mean (SEM). Statistical analysis was performed using GraphPad Prism 8 (GraphPad Software, San Diego, California, United States). p < 0.05 was considered statistically significant.
The treatment of HepG2 cells with increased tomatine concentrations provoked a dose-dependent inhibition of cell proliferation, as determined by the resazurin assay. Significant inhibition of cell viability was noticed at all tested concentrations after 24 h of tomatine treatment. An IC50 of around 3.6 ± 1.2 µM for tomatine treatment was obtained (Figure 1) compared to the IC50 value of 1.7 ± 1.2 µM of positive control Doxorubicin (DOX) (Supplementary Figure S1).
FIGURE 1. Concentration-response curve of tomatine in HepG2 cells after 24 h incubation as determined with resazurin. Data shown are mean ± SEM of 3 independent experiments.
Resistance to apoptosis is one of the hallmarks exhibited by cancer cells, and one of the crucial objectives in cancer treatment is to overcome this resistance via the generation of new drugs to activate the cell death program and involved signaling (Kuno et al., 2012; Ramirez-Tagle et al., 2016). The treatment of HepG2 cells with different tomatine concentrations for 24 h significantly triggered apoptosis, reaching 25.1% at 30 µM of the compound, as shown in Figure 2.
FIGURE 2. (A) Tomatine-induced apoptosis in HepG2 cells as assayed by flow cytometry. HepG2 cells were treated with tomatine (1–30 μM) for 24 h. The cells were then harvested and stained with Annexin V and PI, and flow cytometric analysis was performed to analyze the apoptosis. (B) Summary of the apoptosis data in histogram form. *p < 0.05 vs. the untreated (UT) group.
Later, it was determined whether tomatine can activate caspases, key cysteine proteases family that regulates apoptosis (Green and Llambi, 2015; Nirmala and Lopus, 2019). Immunofluorescence analysis of cells treated with 5 µM of tomatine for 4 and 8 h increased caspase-3 along with inhibition of the antiapoptotic Bcl-2 protein expression (Figure 3A). Similarly, tomatine strongly induced caspases 3/7 activation (Figure 3B). The increase of caspase activation was also confirmed by western blot analysis revealing the induction, by 5 µM of tomatine treatment, of the procaspase-3 (35 kDa) proteolytic-dependent cleavage to the active caspase-3 (17 kDa) form (Figure 3C) and noticeable between 2 and 8 h of treatment.
FIGURE 3. Protein expression of caspase-3, Bcl-2, and activity of caspase 3/7 in HepG2 cells. Cells were treated with 5 μM of tomatine for 4 and 8 h, then immunofluorescence analysis, luminescence, and western blot were performed as described in materials and methods. (A) Cells were fixed and immunostained with anti-tubulin antibody (green), anti-caspase-3, or anti-Bcl-2 (red), and cell nuclei were counterstained with DAPI reagent (blue). Tubulin was used to control expression. The bar scale represents 20 μm. (B) Caspase Glo Assay results are expressed as a normalized activity from untreated (UT) control cells. (C) Western blot analysis of caspase 3. Data shown are mean ± SEM of 3 independent experiments.
The effect of tomatine on the expression of apoptosis-related proteins such as family-Bcl-2 proteins like Bcl-2 and Bax was evaluated. The Bcl-2, Bcl-XL, Cytochrome-c (Cyt-c), and Bax proteins are associated with antiapoptotic and pro-apoptotic functions. As shown in Figures 4A,B, tomatine treatment at 5 μM significantly decreased Bcl-2 expression in contrast to the levels of the control cells at 4 and 8 h; similarly, treatment with tomatine significantly decreased Bcl-XL expression after 8 h (Figures 4C,D). Conversely, Bax protein expression increased significantly at 2, 4, and 8 h after treatment with tomatine (Figures 4E,F). Densitometric analysis of tomatine revealed a significant increase of Bax/Bcl-2 and Bax/Bcl-xl ratio at 2-8 h (Figures 4G,H). Next, it was determined the role of apoptotic protease-activating factor 1 (Apaf-1), a key molecule for the activation of caspase-9 in the apoptosome. Apaf-1 protein expression, increased significantly at 1 and 2 h after treatment with tomatine (Figures 5A,B).
FIGURE 4. Western blot analysis for Bcl-2, Bcl-XL, and Bax proteins in HepG2 cells. (A–F) Cells were treated with tomatine (5 μM), and protein expression was analyzed. (A,C,and E) representative images from western blot experiments performed for the detection of proteins. (B,D,and F) Densitometric analyses of the experiments are shown in (A,C,and E) respectively. (G,H) the data were presented in the bar graphs as Bax/Bcl-2 and Bcl-XL ratio. Protein levels were normalized against tubulin and caspase-3. Data are expressed relative to the UT (untreated) condition. Statistical differences were assessed by a one-way ANOVA (Kruskal-Wallis) followed by Dunn’s post hoc test. *p < 0.05; **p < 0.01; ***p < 0.001 vs. untreated (UT) group.
FIGURE 5. Western blot analysis for APAF-1, Cyt-C, and P53 proteins in HepG2 cells. (A–F) Cells were treated with Tomatine (5 μM), and protein expression was analyzed. (A,C, and E) representative images from western blot experiments performed for the detection of proteins. (B,D, and F) Densitometric analyses of the experiments are shown in (A,C, and E) respectively. Protein levels were normalized against tubulin, and caspase-3 and data are expressed relative to the untreated (UT) condition. Statistical differences were assessed by a one-way ANOVA (Kruskal-Wallis) followed by Dunn’s post hoc test. *p < 0.05; **p < 0.01; ***p < 0.001 vs. UT group.
Subsequently, its was investigated whether tomatine promotes leakage of the mitochondrial intermembrane content such as Cyt-c. Western blot analysis showed that Cyt-c release significantly in HepG2 cells treated with 5 μM tomatine after increase 1 until 4 h (Figures 5C,D). Finally, to determine whether Bcl-2 and Bcl-XL downregulation depend on the p53 pathway, tomatine’s effect on the p53 activation was examined. Tomatine treatment led to a time-dependent induction of phosphorylated P53 (p-P53) (Figures 5E,F), with p-P53 protein being upregulated in tomatine-treated cells within 30 min until 2 h of treatment, while after 4 and 8 h of treatment no significant increase was observed. These results indicate that tomatine-induced apoptosis was mediated via a p53-dependent pathway, consistent with studies in other glycoalkaloids (Ding et al., 2013).
ROS generation has been implicated as an early event in apoptosis. Then, it was determined whether tomatine induced ROS in HepG2 cells. In cells incubated with 1-30 μM of tomatine 4 h before fluorescence analysis (Figure 6), a rapid and significant generation of ROS was detected at 5 μM concentration of tomatine, reaching a maximum of 30 μM with a value 250% higher than that in control cells. Pre-incubation with NAC 5 mM prevented the increase of ROS in HepG2 cells. CuSO4 used as a positive control.
FIGURE 6. Effects of tomatine on HepG2 cells intracellular ROS generation. Cells were exposed to tomatine at 1-30 μM for 4 h and co-treated with NAC. CuSo4 is used as a positive control. Stained cells with DCFDA and analyzed by a fluorescence plate reader. Data are expressed as the mean ± SEM from three independent experiments, each performed in triplicate. A one-way ANOVA (Kruskal–Wallis) assessed statistical differences, followed by Dunn’s post hoc test. *p < 0.05; **p < 0.01; ****p < 0.001 vs. Untreated (UT) group. The bar scale represents 20 μm.
Figures 7A,B illustrate the intracellular calcium recorded in HepG2 cells treated with 5 or 30 µM of tomatine. As shown, even 5 µM of tomatine induces a transient intracellular calcium increase within the first 15 min; moreover, the maximal recorded fluorescent signal does not change much with increasing doses (Figure 7C). However, cells exposed to increasing doses of tomatine (Figure 7D) show a faster response lag time (defined as the time where the temporal derivative of the fluorescence exceeds 10% of its maximum value). These results suggest that in HepG2 cells, intracellular stores are the origin of the observed intracellular calcium increase as previously shown in neuroblastomas (da Silva et al., 2017).
FIGURE 7. Time courses for normalized fluorescence in HepG2 cells loaded with Fluo4 and treated with 5 µM (A) or 30 µM (B) tomatine. Grey lines correspond to [Ca2+]i changes in individual cells (40 cells, 6 independent experiments for 5 μM; 27 cells, 5 independent experiments for 30 µM). White circles correspond to the mean trace of [Ca2+] signals. Bar graphs show the summary of maximal normalized fluorescence (C) or response lag time (D) obtained from experiments as shown in (A) and (B) data are shown as mean ± sem. A one-way ANOVA (Kruskal–Wallis) assessed statistical differences, followed by Dunn’s post hoc test. *p < 0.05; **p < 0.01; ****p < 0.001 vs. Untreated (UT) group.
To examine the effect of tomatine on tumor growth in vivo, HepG2 cell subcutaneous tumors grown in mice were challenged. In these experiments, tumors were allowed to establish for 1 week before challenge 3 times per week for 3 weeks with tomatine (5 or 20 mg/kg/mouse) and vehicle solution. Figure 8A shows that 5 and 20 mg/kg tomatine were equally efficient at retarding the growth of HepG2 cell subcutaneous mouse tumors. Specifically, 3 weeks after the commencement of the drug challenge, the relative tumor volume in-vehicle control compared to mice treated with 5 and 20 mg/kg tomatine was about half compared to untreated animals (Figure 8B). Besides, tomatine treatment did not provoke changes in the body weight of mice (Figure 8C).
FIGURE 8. HepG2 tumor growth was inhibited by tomatine treatment in a xenograft mouse model. (A) Representative images of tumors from each group treatment in hepatoma xenograft tumor growth. (B) Tumor volumes of HepG2 xenografts during the 3-weeks treatment period for tomatine and saline control. *p < 0.05 for tomatine vs. saline control group (n = 6 each). (C) Representative body weight curve of mice bearing HepG2 xenografts during the 3-weeks treatment period with tomatine. Statistical differences were assessed by a one-way ANOVA (Kruskal–Wallis) followed by Dunn’s post hoc test. *p < 0.05 vs. untreated (UT) group.
Tomatine has demonstrated promising anticancer function on solid tumors (Lee et al., 2004; Ramirez-Tagle et al., 2016). Still, the underlying biological and molecular mechanisms have not been well elucidated yet. The results of this investigation indicated that it inhibited the viability of the HCC cancer cell line HepG2 in vitro in a dose-dependent fashion (Figure 1). In this sense, the results agree with had been reported in several other cancer cell lines (Friedman et al., 2009; Huang et al., 2015). The inhibition of cell proliferation was accompanied by apoptotic induction, partly due to caspase-3 activation, Bcl2 downregulation, and increased activation of the tumor suppressor protein p53. Specifically, P53 downregulates Bcl2 expression at the transcriptional level by interaction with its promoter in the regulatory binding sequence (Wu et al., 2001).
Besides the induction of cell cycle arrest, tomatine can also inhibit tumor cell proliferation via induction of apoptosis (Chao et al., 2012; Kim et al., 2015). ROS have a crucial role in induced apoptosis (Simon et al., 2000; Redza-Dutordoir and Averill-Bates, 2016). Increases in intracellular ROS promote the mitochondrial permeability transition pore (mPTP) opening, thus promoting transitory permeabilization of the mitochondrial inner membrane, mainly contributing to apoptosis activation. Indeed, the extrinsic and intrinsic apoptosis pathways load the mPTP (Kinnally et al., 2011). The treatment with tomatine for 4 h significantly increased intracellular ROS levels (Figure 6) consistently with the notion of ROS as an early response mechanism during apoptosis induction (Merad-Boudia et al., 1998; Ni et al., 2008).
Furthermore, the integrity of the outer mitochondrial membrane depends on the balance of the anti- and pro-apoptotic Bcl-2 family proteins expression (Raisova et al., 2001; Breckenridge and Xue, 2004). Tomatine downregulated the antiapoptotic Bcl-2 and Bcl-xl protein expression while upregulating Bax and Bad expression, both playing a role as pro-apoptotic factors. Therefore, tomatine disrupted the antiapoptotic-proapoptotic balance in favor of cell death. Indeed, Bax/Bcl-2 and Bax/Bcl-xl ratios were significantly increased after 4-8 h of tomatine treatment, which may enhance cells’ susceptibility to death signals and mitochondrial function in response to the treatment (Raisova et al., 2001). In addition, the activation of Bax due to tomatine treatment may indicate that apoptosis induction can be mediated by the endoplasmic reticulum (ER) stress pathway in HepG2 cells. Besides, one of the characteristics of many antitumoral agents is their capacity to increase ROS levels causing an oxidative stress activation of apoptosis (Lau et al., 2008). Drugs that induce oxidative stress result in lipid peroxidation and antioxidant defenses reduction, such as reduced glutathione levels, superoxide dismutase, catalase, and thioredoxin expressions, which highly contribute to the apoptosis induction program (Yang et al., 2008). Moreover, for the expression of cell death programs in mammalian cells, the mitochondrial-dependent apoptotic pathway is critical in chemotherapy to kill cancer cells (Polčic and Mentel, 2020). In this sense, a Bax/Bcl-2 ratio increase is critical in the apoptosis process because it stimulates the release of cytochrome c (Cyt-c) from mitochondria into the cytosol (Kirkin et al., 2004). Cyt-c interacts with Apaf-1 to form the apoptosome–deoxyadenosine triphosphate-dependent complex, and the apoptosis program is initiated with the activation of caspase-3 and caspase-9 (Zhang, 2019). Consistently, on HepG2 cells, tomatine provoked a dysregulation of Bax/Bcl-2 ratio, increased Cyt-c cytoplasmic release and apaf-1 expression alongside caspase-3 and caspase-7 activation.
P53 plays a key role in ROS-promoted DNA damage response and apoptosis induction (Maillet and Pervaiz, 2012). Hence, in HepG2 cells, tomatine promoted p53 activation and Bcl-2 reduction with subsequent activation of mitochondrial downstream molecular pathways such as caspase-7 and caspase-3 activation. These results agree with previous investigations demonstrating that ROS may induce DNA damage along with p53 activation in tumoral development (Maillet and Pervaiz, 2012; Budanov, 2014; Li et al., 2020).
Calcium signaling is involved in various cellular functions, including cell proliferation and apoptotic cell death (Berridge et al., 2003). Furthermore, the disruption of intracellular calcium homeostasis can induce ER stress and, consequently, cellular apoptosis (Wang et al., 2013; Monteith et al., 2017). Our results show that tomatine induces a transient increase of intracellular calcium within 15 min. Increasing doses of tomatine reduce the response time instead of augmenting the amount of calcium change, suggesting that internal calcium stores are involved in the mechanism of action tomatine. This observation agrees with previous reports that show, in SH-SY5Y cells, that this alkaloid induces an increase in the basal level of calcium after 1 h of incubation, probably because of ER stress (da Silva et al., 2017), the kinetics of calcium increase also suggests that the increase of calcium could be one of the first steps in the observed tomatine-induced apoptosis insert paragraph. Other glycoalkaloids have been studied for their ability to induce apoptosis; a group of them are present in Solanum melongena L. fruit peels have promising anticancer activity against HCC (Maha M Salama, 2013), but there is no evidence on the mechanism by which tomatine induces apoptosis in HCC. ROS and calcium together can initiate apoptosis signals in various cell types and are crucial candidates to initiate apoptosis in HCC by tomatine treatment (Wang et al., 2003; Maha M Salama, 2013).
Besides, tomatine also demonstrated in vivo inhibitory capacity of tumor HepG2 cell growth. The IP injection of 5 and 20 mg/kg body weight of tomatine significantly reduced tumor sizes. The concentrations of the antitumor compound seem not toxic as no major-organs abnormalities and non-loss of the animals’ body weight were produced (Figure 8). Thus, the results suggest that tomatine can be safely used in tumor animal models at the experimental conditions used in this study and consistently with the agent’s concentrations used in previous investigations (Chao et al., 2012; Lee et al., 2013b; Kim et al., 2015). Therefore, tomatine demonstrated its potential use as a chemopreventive and chemotherapeutic agent for future clinical strategies in cancer patients.
Interestingly, the tomatine’s antiproliferative and promoting cell death activities can be explained by its capacity to downregulate several intracellular signals associated with these events. Tomatine inhibits ERK, PI3K/AKT, and NF-κB pathways that can be implicated in inhibiting HepG2 cell proliferation, apoptotic induction, and in vivo tumor growth (Shih et al., 2009; Lee et al., 2013b; Bailly, 2021). Moreover, recently the epidermal growth factor receptor (EGFR) has been indicated as a direct tomatine molecular target acting as receptor tyrosine kinase inhibitor by a molecular docking into ATP binding sites of EGFR. In this sense, HepG2 cells express EGFR and depend on the EGF/EGFR signal transduction for proliferation and metastatic capabilities (Huang et al., 2014; Chen et al., 2021). Nevertheless, further experimental analyses are necessary to determine the potential contribution of EGFR inhibition in the capacity of tomatine to inhibit in vitro and in vivo tumoral functions of HepG2 cells.
Tomatine inhibits cancer cell proliferation and in vivo tumor growth of cancer cells, such as prostate (Lee et al., 2013a), solid Ehrlich tumor (Tomsik et al., 2013), and HepG2 (this study), and in several cancer cell lines in vitro as well (Xiuwei et al., 2007; Choi et al., 2012; Shi et al., 2013; Del Giudice et al., 2015; Huang et al., 2015; Kim et al., 2015; Rudolf and Rudolf, 2016). This study may have limitations because it was conducted with only one cell line. Nevertheless, we expect to confirm our results with other HCC and non-tumorigenic hepatic cell lines in further experiments, which may increase the impact of our findings.
Despite the mentioned limitation, this study contributes to a better understanding of the molecular and biological mechanisms of tomatine implicated in its antitumoral functions. Next, we propose a simplified model indicating the involved mechanisms in tomatine inhibition of HCC (Figure 9).
On the safe use in humans, several studies have shown that tomatine is not toxic when consumed orally in moderate amounts (Friedman et al., 2007). For example, Peruvians consume a tomato variant very rich in tomatine (0.5–5 mg/g dry weight) without presenting visible signs of toxicity (Rick et al., 1994). In mice, LD50 values of 1000, 500, 25–33.5 and 18 mg/kg body weight have been reported for α-tomatine by subcutaneous, oral, intraperitoneal and intravenous routes, respectively (Wilson et al., 1961; Friedman, 2002). All these data contribute to the evidence for the safe therapeutic use of tomatine in humans.
In conclusion, tomatine potently inhibited the HCC HepG2 cell line viability by inducing cellular apoptosis, which involved the increased intracellular ROS and calcium, p53 activation along with Bcl-2 downregulation, and mitochondrial-dependent caspase cascade activation. Also, this glycoalkaloid agent inhibited in vivo HepG2 tumor growth in a SCID mouse xenograft model. Together, the current investigation results indicate that tomatine is an excellent therapeutic candidate for treating human HCC.
The original contributions presented in the study are included in the article/Supplementary Material, further inquiries can be directed to the corresponding authors.
The animal study was reviewed and approved by Institutional Animal Care and Use Committee of the Universidad Católica Del Maule.
AM and FS performed in vitro experiments. MN and DV performed the intracellular calcium experiments. RP-C and RV-V performed in vivo experiments. CS, JS, and OF-D provided data curation. CE and JE designed, supervised the work, and prepared the manuscript. All authors have read and agreed to the published version of the manuscript.
We gratefully acknowledge funding from Comisión Nacional de Investigación Científica y Tecnológica—CONICYT PAI/ACADEMIA No. 79160109, Fondo Nacional de Desarrollo Científico y Tecnológico - FONDECYT postdoctoral fellowship No. 3130327 and Dirección de Investigación en Ciencia y Tecnología- Universidad de Santiago de Chile (DICYT-USACH) postdoctoral project No. 022041EM and regular Project No. 022141EM (JE). This work was supported by research grants from Fondo Nacional de Desarrollo Científico y Tecnológico—FONDECYT No. 11170840 (CE), No. 1201039 (FS) and Vicerrectoría de investigación de la Universidad de Chile, grant #ENL24/19 (DV). The Millennium Nucleus of Ion Channels–Associated Diseases - MiNICAD) is a Millennium Nucleus supported by the Iniciativa Científica Milenio of the Ministry of Economy, Development, and Tourism (Chile) (DV and FS). Agencia Nacional de Investigación y Desarrollo (ANID)-Millennium Science Initiative Program-ICN09_016/ICN 2021_045: Millennium Institute on Immunology and Immunotherapy [ICN09_016/ICN 2021_045; former P09/016-F] (FS). The Millennium Nucleus of Ion Channel-Associated Diseases is a Millennium Nucleus of the Millennium Scientific Initiative, National Agency of Research and Development (ANID), Ministry of Science, Technology, Knowledge and Innovation, Chile [NCN19_168] (DV and FS).
The authors declare that the research was conducted in the absence of any commercial or financial relationships that could be construed as a potential conflict of interest.
All claims expressed in this article are solely those of the authors and do not necessarily represent those of their affiliated organizations, or those of the publisher, the editors and the reviewers. Any product that may be evaluated in this article, or claim that may be made by its manufacturer, is not guaranteed or endorsed by the publisher.
The Supplementary Material for this article can be found online at: https://www.frontiersin.org/articles/10.3389/fphar.2022.1003264/full#supplementary-material
Apaf-1, apoptotic protease-activating factor 1; Bax, BCL2 Associated X; Bcl-2, B-cell CLL/lymphoma-2; Cyt-c, Cytochrome c; DBP, dibenzo[a,l]pyrene; DCFH-DA, 2,7-Dichlorodihydrofluorescein diacetate; DMEM, Dulbecco’s modified Eagle Medium; ER, endoplasmic-reticulum; FBS, fetal bovine serum; HCC, hepatocellular carcinoma; IVC, individually ventilated cages; NSG, NOD scid gamma; PBS, Phosphate-buffered saline; ROS, reactive oxygen species; RFU, relative fluorescence unity’s; sCMO, stationary camera with moving objects.
Bailly, C. (2021). The steroidal alkaloids α-tomatine and tomatidine: Panorama of their mode of action and pharmacological properties. Steroids 176, 108933. doi:10.1016/j.steroids.2021.108933
Berridge, M. J., Bootman, M. D., and Roderick, H. L. (2003). Calcium signalling: Dynamics, homeostasis and remodelling. Nat. Rev. Mol. Cell Biol. 4, 517–529. doi:10.1038/nrm1155
Breckenridge, D. G., and Xue, D. (2004). Regulation of mitochondrial membrane permeabilization by BCL-2 family proteins and caspases. Curr. Opin. Cell Biol. 16, 647–652. doi:10.1016/j.ceb.2004.09.009
Budanov, A. V. (2014). The role of tumor suppressor p53 in the antioxidant defense and metabolism. Subcell. Biochem. 85, 337–358. doi:10.1007/978-94-017-9211-0_18
Carlsson, G., Gullberg, B., and Hafström, L. (1983). Estimation of liver tumor volume using different formulas---An experimental study in rats. J. Cancer Res. Clin. Oncol. 105, 20–23. doi:10.1007/BF00391826
Chao, M.-W., Chen, C.-H., Chang, Y.-L., Teng, C.-M., and Pan, S.-L. (2012). α-Tomatine-mediated anti-cancer activity in vitro and in vivo through cell cycle-and caspase-independent pathways. PLoS One 7, e44093. doi:10.1371/journal.pone.0044093
Chen, H., Wu, X., Zhou, H., He, Z., Li, H., and Wang, Q. (2021). Epidermal growth factor upregulates the expression of A20 in hepatic cells via the MEK1/MSK1/p-p65 (Ser276) signaling pathway. Am. J. Transl. Res. 13, 708–718.
Choi, S. H., Ahn, J.-B., Kozukue, N., Kim, H.-J., Nishitani, Y., Zhang, L., et al. (2012). Structure–activity relationships of α-β1-γ-and δ-tomatine and tomatidine against human breast (MDA-MB-231), gastric (KATO-III), and prostate (PC3) cancer cells. J. Agric. Food Chem. 60, 3891–3899. doi:10.1021/jf3003027
da Silva, D. C., Andrade, P. B., Valentao, P., and Pereira, D. M. (2017). Neurotoxicity of the steroidal alkaloids tomatine and tomatidine is RIP1 kinase-and caspase-independent and involves the eIF2α branch of the endoplasmic reticulum. J. Steroid Biochem. Mol. Biol. 171, 178–186. doi:10.1016/j.jsbmb.2017.03.009
Del Giudice, R., Raiola, A., Tenore, G. C., Frusciante, L., Barone, A., Monti, D. M., et al. (2015). Antioxidant bioactive compounds in tomato fruits at different ripening stages and their effects on normal and cancer cells. J. Funct. Foods 18, 83–94. doi:10.1016/j.jff.2015.06.060
Ding, X., Zhu, F., Yang, Y., and Li, M. (2013). Purification, antitumor activity in vitro of steroidal glycoalkaloids from black nightshade (Solanum nigrum L). Food Chem. 141, 1181–1186. doi:10.1016/j.foodchem.2013.03.062
Distl, M., and Wink, M. (2009). Identification and quantification of steroidal alkaloids from wild tuber-bearing Solanum species by HPLC and LC-ESI-MS. Potato Res. 52, 79–104. doi:10.1007/s11540-008-9123-0
Echeverría, C., Montorfano, I., Cabello-Verrugio, C., Armisén, R., Varela, D., and Simon, F. (2015). Suppression of transient receptor potential melastatin 4 expression promotes conversion of endothelial cells into fibroblasts via transforming growth factor/activin receptor-like kinase 5 pathway. J. Hypertens. 33, 981–992. doi:10.1097/HJH.0000000000000496
Edelstein, A., Amodaj, N., Hoover, K., Vale, R., and Stuurman, N. (2010). Computer control of microscopes using µManager. Curr. Protoc. Mol. Biol. 92, Unit14.20. doi:10.1002/0471142727.mb1420s92
Fan, C., Zheng, W., Fu, X., Li, X., Wong, Y.-S., and Chen, T. (2014). Strategy to enhance the therapeutic effect of doxorubicin in human hepatocellular carcinoma by selenocystine, a synergistic agent that regulates the ROS-mediated signaling. Oncotarget 5, 2853–2863. doi:10.18632/oncotarget.1854
Faria-Silva, C., de Sousa, M., Carvalheiro, M. C., Simões, P., and Simões, S. (2022). Alpha-tomatine and the two sides of the same coin: An anti-nutritional glycoalkaloid with potential in human health. Food Chem. 391, 133261. doi:10.1016/j.foodchem.2022.133261
Friedman, M. (2013). Anticarcinogenic, cardioprotective, and other health benefits of tomato compounds lycopene, α-tomatine, and tomatidine in pure form and in fresh and processed tomatoes. J. Agric. Food Chem. 61, 9534–9550. doi:10.1021/jf402654e
Friedman, M., Levin, C. E., Lee, S.-U., Kim, H.-J., Lee, I.-S., Byun, J.-O., et al. (2009). Tomatine-containing green tomato extracts inhibit growth of human breast, colon, liver, and stomach cancer cells. J. Agric. Food Chem. 57, 5727–5733. doi:10.1021/jf900364j
Friedman, M., McQuistan, T., Hendricks, J. D., Pereira, C., and Bailey, G. S. (2007). Protective effect of dietary tomatine against dibenzo [a, l] pyrene (DBP)‐induced liver and stomach tumors in rainbow trout. Mol. Nutr. Food Res. 51, 1485–1491. doi:10.1002/mnfr.200700176
Friedman, M. (2002). Tomato glycoalkaloids: Role in the plant and in the diet. J. Agric. Food Chem. 50, 5751–5780. doi:10.1021/jf020560c
Green, D. R., and Llambi, F. (2015). Cell death signaling. Cold Spring Harb. Perspect. Biol. 7, a006080. doi:10.1101/cshperspect.a006080
Gregory, P., Sinden, S. L., Osman, S. F., Tingey, W. M., and Chessin, D. A. (1981). Glycoalkaloids of wild, tuber-bearing Solanum species. J. Agric. Food Chem. 29, 1212–1215. doi:10.1021/jf00108a028
Huang, H., Chen, S., Van Doren, J., Li, D., Farichon, C., He, Y., et al. (2015). α-Tomatine inhibits growth and induces apoptosis in HL-60 human myeloid leukemia cells. Mol. Med. Rep. 11, 4573–4578. doi:10.3892/mmr.2015.3238
Huang, P., Xu, X., Wang, L., Zhu, B., Wang, X., and Xia, J. (2014). The role of EGF ‐ EGFR signalling pathway in hepatocellular carcinoma inflammatory microenvironment. J. Cell. Mol. Med. 18, 218–230. doi:10.1111/jcmm.12153
Kaunda, J. S., and Zhang, Y.-J. (2019). The genus Solanum: An ethnopharmacological, phytochemical and biological properties review. Nat. Prod. Bioprospect. 9, 77–137. doi:10.1007/s13659-019-0201-6
Kim, S. P., Nam, S. H., and Friedman, M. (2015). The tomato glycoalkaloid α-tomatine induces caspase-independent cell death in mouse colon cancer CT-26 cells and transplanted tumors in mice. J. Agric. Food Chem. 63, 1142–1150. doi:10.1021/jf5040288
Kinnally, K. W., Peixoto, P. M., Ryu, S.-Y., and Dejean, L. M. (2011). Is mPTP the gatekeeper for necrosis, apoptosis, or both? Biochim. Biophys. Acta 1813, 616–622. doi:10.1016/j.bbamcr.2010.09.013
Kirkin, V., Joos, S., and Zörnig, M. (2004). The role of Bcl-2 family members in tumorigenesis. Biochim. Biophys. Acta 1644, 229–249. doi:10.1016/j.bbamcr.2003.08.009
Kulik, L., and El-Serag, H. B. (2019). Epidemiology and management of hepatocellular carcinoma. Gastroenterology 156, 477–491. doi:10.1053/j.gastro.2018.08.065
Kuno, T., Tsukamoto, T., Hara, A., and Tanaka, T. (2012). Cancer chemoprevention through the induction of apoptosis by natural compounds. J. Biophys. Chem. 3, 156–173. doi:10.4236/jbpc.2012.32018
Lau, A. T. Y., Wang, Y., and Chiu, J. (2008). Reactive oxygen species: Current knowledge and applications in cancer research and therapeutic. J. Cell. Biochem. 104, 657–667. doi:10.1002/jcb.21655
Lee, K. R., Kozukue, N., Han, J. S., Park, J. H., Chang, E. Y., Baek, E. J., et al. (2004). Glycoalkaloids and metabolites inhibit the growth of human colon (HT29) and liver (HepG2) cancer cells. J. Agric. Food Chem. 52, 2832–2839. doi:10.1021/jf030526d
Lee, S.-T., Wong, P.-F., He, H., Hooper, J. D., and Mustafa, M. R. (2022). Correction: Alpha-Tomatine attenuation of in vivo growth of subcutaneous and orthotopic xenograft tumors of human prostate carcinoma PC-3 cells is accompanied by inactivation of nuclear factor-kappa B signaling. PLoS One 17, e0268234. doi:10.1371/journal.pone.0268234
Lee, S.-T., Wong, P.-F., Hooper, J. D., and Mustafa, M. R. (2013a). Alpha-tomatine synergises with paclitaxel to enhance apoptosis of androgen-independent human prostate cancer PC-3 cells in vitro and in vivo. Phytomedicine 20, 1297–1305. doi:10.1016/j.phymed.2013.07.002
Lee, S. T., Wong, P. F., He, H., Da Hooper, J., and Mustafa, M. R. (2013b). Alpha-tomatine attenuation of in vivo growth of subcutaneous and orthotopic xenograft tumors of human prostate carcinoma PC-3 cells is accompanied by inactivation of nuclear factor-kappa B signaling. PLoS One 8, e57708. doi:10.1371/journal.pone.0057708
Li, Y., Jiang, B., Wang, R., Wang, J., Li, Y., and Bao, Y. (2020). Synergistic effects of tanshinone IIA and andrographolide on the apoptosis of cancer cells via crosstalk between p53 and reactive oxygen species pathways. Pharmacol. Rep. 72, 400–417. doi:10.1007/s43440-019-00006-z
Ma, L., Wang, X., Jia, T., Wei, W., Chua, M.-S., and So, S. (2015). Tankyrase inhibitors attenuate WNT/β-catenin signaling and inhibit growth of hepatocellular carcinoma cells. Oncotarget 6, 25390–25401. doi:10.18632/oncotarget.4455
Maha M Salama, M. M. S. (2013). In vitro and in vivo anticancer activity of the fruit peels of Solanum melongena L. Against hepatocellular carcinoma. J. Carcinog. Mutagen. 4, 149–154. doi:10.4172/2157-2518.1000149
Maillet, A., and Pervaiz, S. (2012). Redox regulation of p53, redox effectors regulated by p53: A subtle balance. Antioxid. Redox Signal. 16, 1285–1294. doi:10.1089/ars.2011.4434
Merad-Boudia, M., Nicole, A., Santiard-Baron, D., Saillé, C., and Ceballos-Picot, I. (1998). Mitochondrial impairment as an early event in the process of apoptosis induced by glutathione depletion in neuronal cells: Relevance to Parkinson’s disease. Biochem. Pharmacol. 56, 645–655. doi:10.1016/S0006-2952(97)00647-3
Monteith, G. R., Prevarskaya, N., and Roberts-Thomson, S. J. (2017). The calcium-cancer signalling nexus. Nat. Rev. Cancer 17, 367–380. doi:10.1038/nrc.2017.18
Moreno, C., Hermosilla, T., Morales, D., Encina, M., Torres-Díaz, L., Díaz, P., et al. (2015). Cavβ2 transcription start site variants modulate calcium handling in newborn rat cardiomyocytes. Pflugers Arch. 467, 2473–2484. doi:10.1007/s00424-015-1723-3
Ni, Y., Gong, X., Lu, M., Chen, H., and Wang, Y. (2008). Mitochondrial ROS burst as an early sign in sarsasapogenin-induced apoptosis in HepG2 cells. Cell Biol. Int. 32, 337–343. doi:10.1016/j.cellbi.2007.12.004
Nirmala, J. G., and Lopus, M. (2019). Cell death mechanisms in eukaryotes. Cell Biol. Toxicol. 36, 145–164. doi:10.1007/s10565-019-09496-2
Osman, S. F., Herb, S. F., Fitzpatrick, T. J., and Schmiediche, P. (1978). Glycoalkaloid composition of wild and cultivated tuber-bearing Solanum species of potential value in potato breeding programs. J. Agric. Food Chem. 26, 1246–1248. doi:10.1021/jf60219a024
Polčic, P., and Mentel, M. (2020). Reconstituting the mammalian apoptotic switch in yeast. Genes 11, 145. doi:10.3390/genes11020145
Raisova, M., Hossini, A. M., Eberle, J., Riebeling, C., Orfanos, C. E., Geilen, C. C., et al. (2001). The Bax/Bcl-2 ratio determines the susceptibility of human melanoma cells to CD95/Fas-mediated apoptosis. J. Invest. Dermatol. 117, 333–340. doi:10.1046/j.0022-202x.2001.01409.x
Ramirez-Tagle, R., Escobar, C., Romero, V., Montorfano, I., Armisén, R., Borgna, V., et al. (2016). Chalcone-induced apoptosis through caspase-dependent intrinsic pathways in human hepatocellular carcinoma cells. Int. J. Mol. Sci. 17, 260. doi:10.3390/ijms17020260
Redza-Dutordoir, M., and Averill-Bates, D. A. (2016). Activation of apoptosis signalling pathways by reactive oxygen species. Biochim. Biophys. Acta 1863, 2977–2992. doi:10.1016/j.bbamcr.2016.09.012
Rick, C. M., Uhlig, J. W., and Jones, A. D. (1994). High alpha-tomatine content in ripe fruit of andean Lycopersicon esculentum var. cerasiforme: Developmental and genetic aspects. Proc. Natl. Acad. Sci. U. S. A. 91, 12877–12881. doi:10.1073/pnas.91.26.12877
Riss, T. L., Moravec, R. A., Niles, A. L., and Minor, L. (2017). in Cell viability assays. Editors D. F. Gilbert, and O. Friedrich (New York, NY: Springer New York). doi:10.1007/978-1-4939-6960-9
Rudolf, K., and Rudolf, E. (2016). Antiproliferative effects of α-tomatine are associated with different cell death modalities in human colon cancer cells. J. Funct. Foods 27, 491–502. doi:10.1016/j.jff.2016.10.005
Shi, M.-D., Shih, Y.-W., Lee, Y.-S., Cheng, Y.-F., and Tsai, L.-Y. (2013). Suppression of 12-O-Tetradecanoylphorbol-13-Acetate-Induced MCF-7 breast adenocarcinoma cells invasion/migration by α-tomatine through activating PKCα/ERK/NF-κB-Dependent MMP-2/MMP-9 expressions. Cell biochem. Biophys. 66, 161–174. doi:10.1007/s12013-012-9465-8
Shih, Y.-W., Shieh, J.-M., Wu, P.-F., Lee, Y.-C., Chen, Y.-Z., and Chiang, T.-A. (2009). Alpha-tomatine inactivates PI3K/akt and ERK signaling pathways in human lung adenocarcinoma A549 cells: Effect on metastasis. Food Chem. Toxicol. 47, 1985–1995. doi:10.1016/j.fct.2009.05.011
Simon, H. U., Haj-Yehia, A., and Levi-Schaffer, F. (2000). Role of reactive oxygen species (ROS) in apoptosis induction. Apoptosis 5, 415–418. doi:10.1023/A:1009616228304
Tomsik, P., Micuda, S., Sucha, L., Cermakova, E., Suba, P., Zivny, P., et al. (2013). The anticancer activity of alpha-tomatine against mammary adenocarcinoma in mice. Biomed. Pap. Med. Fac. Univ. Palacky. Olomouc Czech. Repub. 157, 153–161. doi:10.5507/bp.2013.031
Van Gelder, W. M. J. (1985). Determination of the total C27-steroidal alkaloid composition of Solanum species by high-resolution gas chromatography. J. Chromatogr. A 331, 285–293. doi:10.1016/0021-9673(85)80034-0
Wang, C., Liu, C., Niu, L., Wang, L., Hou, L., and Cao, X. (2013). Surfactin-induced apoptosis through ROS-ERS-Ca2+-ERK pathways in HepG2 cells. Cell biochem. Biophys. 67, 1433–1439. doi:10.1007/s12013-013-9676-7
Wang, H., Yang, X., Zhang, Z., and Xu, H. (2003). Both calcium and ROS as common signals mediate Na2SeO 3-induced apoptosis in SW480 human colonic carcinoma cells. J. Inorg. Biochem. 97, 221–230. doi:10.1016/S0162-0134(03)00284-8
Wilson, R. H., Poley, G. W., and DeEds, F. (1961). Some pharmacologic and toxicologic properties of tomatine and its derivatives. Toxicol. Appl. Pharmacol. 3, 39–48. doi:10.1016/0041-008x(61)90006-0
Wu, H., Li, W., Wang, T., Rong, Y., He, Z., Huang, S., et al. (2021). α-Tomatine, a novel early-stage autophagy inhibitor, inhibits autophagy to enhance apoptosis via Beclin-1 in Skov3 cells. Fitoterapia 152, 104911. doi:10.1016/j.fitote.2021.104911
Wu, Y., Mehew, J. W., Heckman, C. A., Arcinas, M., and Boxer, L. M. (2001). Negative regulation of bcl-2 expression by p53 in hematopoietic cells. Oncogene 20, 240–251. doi:10.1038/sj.onc.1204067
Xiuwei, Y., Fuxiang, R., Ruiqing, W., Jun, W., Jun, P., Huiping, P., et al. (2007). Inhibitory effects of 44 alkaloids compounds against growth of human gastric carcinoma cell line BGC and human hepatic carcinoma cell line BEL-7402 in vitro [J]. Mod. Chin. Med. 2.
Yang, H.-L., Chen, S.-C., Chen, C.-S., Wang, S.-Y., and Hseu, Y.-C. (2008). Alpinia pricei rhizome extracts induce apoptosis of human carcinoma KB cells via a mitochondria-dependent apoptotic pathway. Food Chem. Toxicol. 46, 3318–3324. doi:10.1016/j.fct.2008.08.003
Keywords: Solanum glycoalkaloids, tomatine, antitumoral activity, hepatocellular carcinoma, apoptosis, caspase pathways
Citation: Echeverría C, Martin A, Simon F, Salas CO, Nazal M, Varela D, Pérez-Castro RA, Santibanez JF, Valdés-Valdés RO, Forero-Doria O and Echeverría J (2022) In Vivo and in vitro antitumor activity of tomatine in hepatocellular carcinoma. Front. Pharmacol. 13:1003264. doi: 10.3389/fphar.2022.1003264
Received: 26 July 2022; Accepted: 12 August 2022;
Published: 09 September 2022.
Edited by:
Michał Tomczyk, Medical University of Bialystok, PolandReviewed by:
Rajeev K. Singla, West China Hospital, Sichuan University, ChinaCopyright © 2022 Echeverría, Martin, Simon, Salas, Nazal, Varela, Pérez-Castro, Santibanez, Valdés-Valdés, Forero-Doria and Echeverría. This is an open-access article distributed under the terms of the Creative Commons Attribution License (CC BY). The use, distribution or reproduction in other forums is permitted, provided the original author(s) and the copyright owner(s) are credited and that the original publication in this journal is cited, in accordance with accepted academic practice. No use, distribution or reproduction is permitted which does not comply with these terms.
*Correspondence: Cesar Echeverría, Y2VzYXIuZWNoZXZlcnJpYUB1ZGEuY2w=; Javier Echeverría, amF2aWVyLmVjaGV2ZXJyaWFtQHVzYWNoLmNs
Disclaimer: All claims expressed in this article are solely those of the authors and do not necessarily represent those of their affiliated organizations, or those of the publisher, the editors and the reviewers. Any product that may be evaluated in this article or claim that may be made by its manufacturer is not guaranteed or endorsed by the publisher.
Research integrity at Frontiers
Learn more about the work of our research integrity team to safeguard the quality of each article we publish.