- 1Department of Basic Medicine, School of Medicine, Taizhou University, Taizhou, China
- 2Key Laboratory of Pharmaceutics and Bioengineering, School of Medical Technology, Beihua University, Jilin, China
- 3Center of Reproductive Medicine and Center of Prenatal Diagnosis, First Hospital, Jilin University, Changchun, China
Exosomes are nano-sized biological extracellular vesicles transmitting information between cells and constituting a new intercellular communication mode. Exosomes have many advantages as an ideal drug delivery nanocarrier, including good biocompatibility, permeability, low toxicity, and low immunogenicity. Recently, exosomes have been used to deliver chemotherapeutic agents, natural drugs, nucleic acid drugs, and other antitumor drugs to treat many types of tumors. Due to the limited production of exosomes, synthetic exosome-mimics have been developed as an ideal platform for drug delivery. This review summarizes recent advances in the application of exosomes and exosome-mimics delivering therapeutic drugs in treating cancers.
1 Introduction
Malignant tumors are among the leading causes of death worldwide (Mattiuzzi et al., 2019). Current therapeutics, including chemotherapy, radiation, surgical resection, and immunotherapy, remain the most commonly used treatment (Leal and García-Perdomo, 2019). However, many malignant tumors have poor prognoses due to the late diagnosis and lack of effective treatment options. Thus, novel antitumor drugs and treatments are urgently needed to enhance treatment efficacies. Drug delivery systems (DDS) hold great promise in improving cancer treatments (Song et al., 2021). Despite the improved efficacy of reported DDS in treating many types of tumors, high clearance, toxicity to normal tissues, and limited penetrating depth are the main limitations of current nanocarriers for cancer therapy, leading to poor treatment outcomes (Maeda and Khatami, 2018).
Exosomes are nano-sized extracellular vesicles (EVs) ranged from 40 to 100 nm that are secreted by many types of cells (Pegtel and Gould, 2019). Exosomes, apoptotic bodies, and microvesicles are three members of EVs family (Liao et al., 2019). Exosomes, as the smallest EVs, contain various proteins and nucleic acid molecules, essential in transmitting information between cells. Exosomes were first isolated and purified from sheep reticulocytes by Johnstone (Johnstone et al., 1987). Initially, exosomes were thought to be wastes discharged by cells. However, subsequent studies in recent years have shown that exosomes play critical roles in the tumor microenvironment, such as regulating the occurrence and development of tumors (Xiong et al., 2017; Wang et al., 2020). Further investigations have shown that exosomes secreted by tumor cells may have unique properties and could act as biomarkers for tumor diagnosis (Wang et al., 2018). In addition, exosomes exhibited efficient tumor enrichment effects known as high permeability and retention effects (EPR) (Ngoune et al., 2016). Therefore, the appropriate size and unique physiological structure properties of exosomes make them suitable for delivering various reagents for therapeutic applications (Mohammed et al., 2017). In addition, the natural materials-derived biocompatibility, structural stability, good permeability, low toxicity, and immunogenicity make them ideal carriers for drug delivery (Lee et al., 2012; Xin et al., 2012; Lou et al., 2015). Increased studies have indicated the superior effect of drug-loaded exosomes in treating many diseases (Bagheri et al., 2020; Pei et al., 2021).
1.1 Origin of exosomes
Exosomes can be isolated from cell culture supernatants, plasma, serum, and various sources (Muller et al., 2014). The biogenetic processes of exosomes can be divided into four stages: initiation, endocytosis, formation of multivesicular bodies (MVBs), and secretion of exosomes (Figure 1) (Razi and Futter, 2006). The formation of exosomes in MVBs has similarities with lysosomal formation because lysosomal surface proteins such as LAMP and CD63 are also present in exosomes (Johnsen et al., 2014). The production of exosomes is affected by many factors, such as an increase in intracellular Ca2+ (Merendino et al., 2010), amino acids, and intracellular and intercellular pH (Fader and Colombo, 2009). Some specific mechanisms have been proposed to explain the various stages of exosome biogenesis, suggesting that exosomal formation may be a fine-tuning process (Van Niel et al., 2018). However, it is still unclear what triggers exosome biogenesis and secretion.
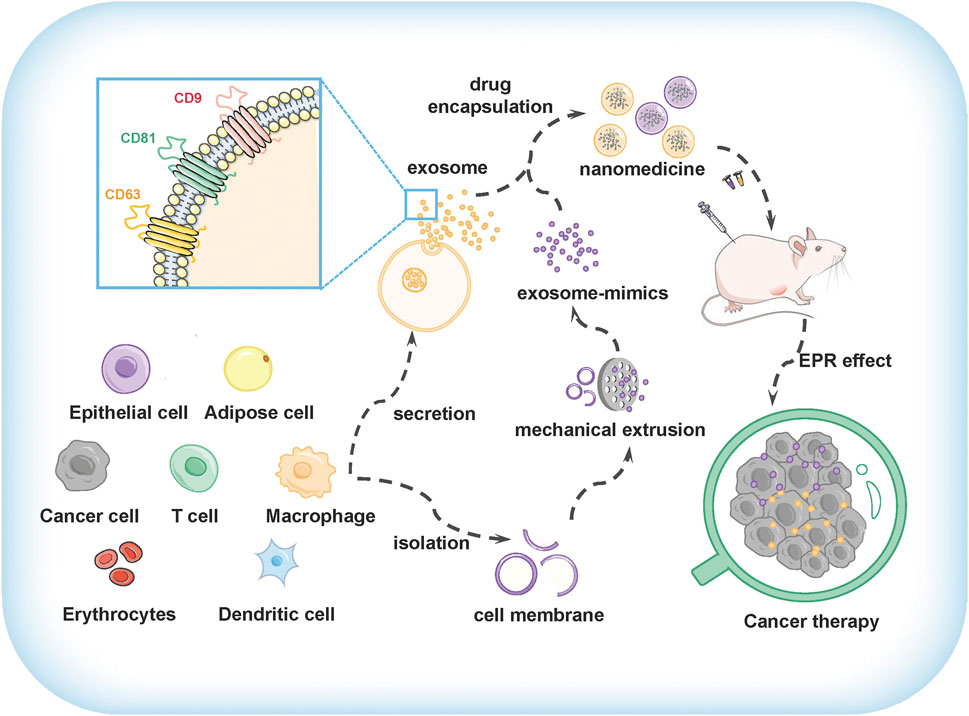
FIGURE 1. The preparation and application of exosomes and exosome-mimics as drug delivery vehicles for cancer therapy.
1.2 The composition of exosomes
Exosomes from various organisms and cell types contain thousands of proteins, lipids, mRNAs, and miRNAs. (Zhuang et al., 2011). The confluence of cholesterol, diglyceride, phospholipid, sphingolipid, and glycerophospholipid is higher in exosomes than in parental cells (Yang et al., 2015). Specific molecules, including targeting and fusion proteins, cytoplasmic enzymes, chaperones, and membrane transport proteins, are enriched in exosomes (Gonzalez-Begne et al., 2009). CD9, CD63, and CD81 proteins are usually considered marker proteins of exosomes (Subra et al., 2010; Mathieu et al., 2021). Exosomes can transfer their functional molecules from one cell to another via cell-to-cell communication (Dourado et al., 2019).
1.3 Separation of exosomes
The great potential of exosomes for delivering vehicles prompts the need for high-yield exosomes. Exosomes are usually isolated from cells incubated with serum-free mediums for several days (Gupta et al., 2018; Biadglegne et al., 2021). Although many cells can produce exosomes, the yield of exosomes produced by different cell types is highly variable (Kim et al., 2021). Therefore, selecting the optimal source of cells is crucial for the production of exosomes. Nowadays, the exosomal preparation protocols include differential ultracentrifugation and density gradient centrifugation, sedimentation, capture, and microfluidic separation (Coughlan et al., 2020; Jung et al., 2020). As the most popular protocol, differential ultracentrifugation with a centrifugal force of 200 × g to 100,000 × g removes larger particles and cell debris and finally precipitates exosomes (Thakur et al., 2020).
Due to the unsatisfactory yield, how to increase the production of exosomes is still a significant challenge. Kim et al. found that the mesenchymal stem cells (MSCs) cultured in three-dimensional (3D) spheroids produced a higher level of exosome than that in the traditional two-dimensional (2D) culture (Kim et al., 2018). The changes in exosome production may be attributed to the non-adherent and round MSCs, which means the three-dimensional structure may affect the efficiency of exosome production. Ludwig et al. found that adenosine receptors modulated the production of exosomes originating from tumor cells (Ludwig et al., 2020). Ambattu et al. showed that high-frequency acoustic cell stimulation induced an 8-fold increase in exosome production through irradiation and post-excitation incubation steps (Ambattu et al., 2020). These findings provided new ideas for the preparation of a large number of exosomes. However, obtaining exosomes with high purity and satisfied yield is still a significant problem limiting the application of exosomes in cancer treatment.
2 Drug loading into exosomes
Drug loading into exosomes refers to loading different drugs into purified exosomes’ inner cavities or intramembrane. Exosomes could encapsulate hydrophilic drugs, hydrophobic reagents, and membrane proteins as efficient drug delivery vehicles. In principle, drug-loaded exosomes can be acquired through post-loading and pre-loading of exosomes.
2.1 Post-loading of exosomes
There are several ways to load drugs into isolated exosomes, including co-incubation, electroporation, and ultrasound (Değirmenci et al., 2022). Co-incubation is a commonly used method for drug loading into exosomes, which is simple to operate but has low loading efficiency (Fang and Liang, 2021). Yang and his coworkers prepared a linezolid-loaded exosome using co-incubation at 37°C with a drug loading efficiency of 5% (Yang et al., 2018). Nucleic acid drugs, including DNA, siRNA, miRNA, and others were usually loaded into the inner cavity of exosomes by the electroporation method (Figure 2) (Faruqu et al., 2018; Asadirad et al., 2019). The loading efficiency of nucleic acid drugs into exosomes depends on the molecule weight and the size of exosomes (Lamichhane et al., 2015). Unlike the traditional strategies, Yang et al. designed a cell nanoporation (CNP) biochip, combining exosome purification and drug loading into a device, significantly improving the exosome yield and mRNA loading efficiency (Yang et al., 2020). Improving the drug loading capacity of exosomes is crucial for enhancing the efficacy of cancer treatment.
2.2 Pre-loading of exosomes
Another drug loading method is introducing the drugs into the exosome–derived cells, which is especially important for those drugs that cannot be loaded into purified exosomes, such as the cytosol and transmembrane proteins (Bai et al., 2020). Tian et al. transfected cells with iRGD plasmid to obtain iRGD-decorated exosomes, encapsulating doxorubicin for targeted therapy in breast cancer (Tian et al., 2014). Severic et al. modified exosome-mimics with prostate-specific targeting peptides, which significantly increased the accumulation of exosome-mimics in prostate tumor tissues and reduced their distribution in normal tissues and organs (Severic et al., 2021).
3 Application of exosomes as drug delivery vehicles
3.1 Nucleic acid drugs
Recently, exosomes from tumor cells, adipose stem cells, mesenchymal stem cells and epithelial cells have been used to treat different diseases (Zhang M. et al., 2021; Pan et al., 2021; Rui et al., 2021; Sun et al., 2021; Wang L. et al., 2022). Gene therapy has shown great promise in treating intractable diseases (Suresh et al., 2014). The key to gene therapy is introducing nucleic acid drugs into the targeted cells for long-term gene regulation (Misra, 2013). Despite some promising results, the clinical application of gene therapy is limited by the lack of proper delivery systems (Mendell et al., 2021). In this regard, exosomes have been used to deliver many nucleic acid drugs, including miRNA, siRNA, and mRNA (Aqil et al., 2019; Zhang D. et al., 2021; Huang et al., 2021).
3.1.1 miRNA
miRNA is a non-coding RNA molecule with 20–22 nucleotides in size binding to a partially complementary mRNA sequence, resulting in targeted degradation or translational inhibition (Novina and Sharp, 2004). Increasing evidence has shown that the acquisition or loss of related miRNA function is closely related to tumorigenesis (Farazi et al., 2013; Zhou et al., 2014; Ye et al., 2017; Mo et al., 2018). The inherent ability of exosomes in delivering bio-related molecules is a significant advantage over other delivery platforms (Esposito et al., 2021). For example, exosomes modified with GE11 peptide could deliver let-7a miRNA into the epidermal growth factor receptor (EGFR)-overpressed breast cancer cells (HCC70, HCC 1954, and MCF-7). Enhanced tumor suppression was observed in mice treated with miRNA-loaded exosomes in breast cancer (Ohno et al., 2013). Han et al. developed exosomes delivering miR-567 and found that they could target autophagy-related proteins (ATG5) to reverse trastuzumab resistance in breast cancer cells (Han et al., 2020). Yao et al. used exosomes derived from HEK-293T cells to deliver miR-204-5p for cancer treatment. The results showed that exosomal miR-204-5p could significantly inhibit the proliferation of cancer cells and increase their sensitivity to chemotherapeutic agents (Yao et al., 2020).
3.1.2 siRNA
Tumor cells can overcome the immune attack from the host immune system through the immunosuppressive tumor microenvironment (Chew et al., 2012). Cancer immunotherapy results largely depend on the continuous activation and expansion of tumor-specific T cells, especially the tumor-infiltrating cytotoxic T lymphocytes (De Sanctis et al., 2022). Pei et al. used cRGD-modified exosomes to deliver siFGL1 and siTGF-β1, significantly increasing the number of tumor-infiltrating T lymphocytes (Pei et al., 2021). Galectin-9 is a β-galactoside-binding lectin, and its blockade could induce the antitumor immune response (Moar and Tandon, 2021). Galectin-9 was highly expressed in tumor tissues of patients with pancreatic ductal adenocarcinoma (PDAC) (Seifert et al., 2020). Zhou et al. developed exosomes modified with oxaliplatin prodrug to deliver galectin-9 siRNA to PDAC tissues, which induced immunogenic cell death (ICD) of tumor cells and showed significant therapeutic effects on PDAC (Zhou et al., 2021). Shtam et al. prepared exosomes to deliver RAD51-siRNA and promoted the massive reproductive cell death of recipient cancer cells (Shtam et al., 2013). KRAS is a signaling protein that drives pancreatic cancer formation mutations (Diehl et al., 2021). Exosomes delivering siRNA targeting the oncogene protein Kras (KrasG12D) demonstrated unprecedented tumor regression and promising potential for targeting pancreatic cancer (Zorde Khvalevsky et al., 2013; Kamerkar et al., 2017).
3.1.3 mRNA
Many studies have shown that exosomes isolated from many tumor cells contained tumor cell-specific mRNA, and exosomes delivering mRNA has attracted considerable attention for cancer treatment (Gutkin et al., 2016). Mizrak et al. reported that mRNA-loaded exosomes could efficiently deliver mRNA and show a combination therapy effect with other anti-cancer drugs (Mizrak et al., 2013).
3.2 Chemotherapy drugs
Chemotherapy remains one of the primary cancer treatments in the clinic. Unfortunately, many chemotherapy drugs are associated with severe adverse events in clinical use (Cassidy and Misset, 2002). Many studies have shown the decreased toxicity of chemotherapeutics -loaded exosomes toward normal tissues for improved cancer therapy (Hadla et al., 2016; Li Y. et al., 2018b). Tian et al. developed iRGD-modified exosomes derived from mouse immature dendritic cells (imDC) to encapsulate doxorubicin for cancer treatment, showing good antitumor efficacy with no toxicity observed (Tian et al., 2014). Wang et al. purified exosomes from M1 type macrophages by ultra-high speed centrifugation and loaded paclitaxel into exosomes by ultrasound in a breast cancer mice model. The paclitaxel-loaded exosomes demonstrated enhanced tumor targeting and inhibited tumor growth compared with free paclitaxel (Wang et al., 2019). Embryonic stem cell-derived exosomes delivering paclitaxel also showed good antitumor efficacy in glioblastoma (Zhu et al., 2019).
Curcumin, a polyphenol enriched in turmeric plants, has a wide range of pharmacological effects, including anti-oxidative stress and inhibition of cell proliferation of malignant tumors. The poor water solubility greatly limited their further applications (Anand et al., 2007). Exosomes derived from cow milk and intestinal epithelial cells could improve the oral bioavailability of curcumin (Carobolante et al., 2020). Moreover, exosomes derived from milk could protect curcumin from metabolism and improve its anti-cancer activity of curcumin (González-Sarrías et al., 2022). Gemcitabine is an effective chemotherapeutic drug for treating pancreatic cancer, but it is often associated with several adverse events in the circulatory system, gastrointestinal tract, and kidneys (Cidon et al., 2018). Li et al. prepared gemcitabine-loaded exosomes, which showed increased tumor accumulation and better tumor inhibition than free gemcitabine (Li et al., 2020). In addition, exosomes have been used to overcome the drug resistance of tumors. Zhang et al. loaded cisplatin into exosomes derived from M1 macrophages of human cord blood (exoCIS) and found that exoCIS could significantly inhibit the growth of cisplatin-resistant ovarian cancer cells (Zhang et al., 2020). Exosome-modified targeting moieties significantly enhanced the antitumor efficacy and reduced the toxicity to normal tissues.
3.3 Others
Recently, exosomes have been used to deliver photosensitizers to tumor tissues for cancer therapy (Zhu et al., 2022). Pan et al. loaded PMA/Au-BSA@Ce6 nanoparticles into urinary exosomes and constructed passion fruit-like exosome nanoparticles, which achieved targeted tumor imaging and photodynamic therapy (Pan et al., 2020). Cao et al. prepared vanadium carbide quantum dots-loaded exosomes, which showed a tumor-killing effect through photothermal therapy (Cao et al., 2019). Fan et al. used DNA hinges to connect quantum dots to exosome surfaces (Exosome-DNA-QDs) and found that exosome-DNA-QDs could be phagocytosed by tumor cells faster than normal cells, suggesting the unique advantage of the exosome-based delivery platform for cancer treatment (Fan et al., 2019).
4 Exosome-mimics
Exosomes have been an ideal platform to deliver various drugs in treating cancers. However, their further application is limited by the low production yield and lack of targeting properties (Yong et al., 2019; Zhang H. et al., 2021b). Therefore, artificially produced nanovesicles, which mimic the structure of exosomes, have received extensive attention in drug delivery (Nie et al., 2019; Fan et al., 2021; Oroojalian et al., 2021). Exosome-mimics, prepared by extruding whole cells or cell membranes through certain-sized filters, have similar structures and characteristics to exosomes (Li et al., 2022). Synthetic exosome-mimics, retaining the natural properties of cells, can be applied to developing novel therapeutic strategies (He et al., 2021). Some studies have shown that exosome-mimics can result in a 100-fold increase in production yield compared with natural exosomes (Jang et al., 2013).
Until now, exosome-mimics prepared from various cell membranes or platelet membranes could be used in developing cancer vaccination and drug delivery systems (Fang et al., 2014; Zhang et al., 2019). The exosome-mimics encapsulating PLGA nanoparticles showed enhanced stability compared with the bare nanoparticles (Kroll et al., 2017; Zhang et al., 2017). The exosome-mimics prepared by the T cell membrane, which retained T cell receptors on the surface, could bind to HIV surface glycoprotein gp120 and inhibit gp120-induced CD4+ T cell damage, suggesting a promising therapeutic agent against HIV infection (Wei et al., 2018b). Exosome-mimics prepared from gastric epithelial cell membranes, macrophage membranes, platelet membranes, and neutrophil membranes have also shown improved efficacies in treating some diseases (Thamphiwatana et al., 2017; Angsantikul et al., 2018; Wei et al., 2018a; Zhang Q. et al., 2018a). Exosome-mimetic nanovesicles have similar abilities as drug delivery systems compared with exosomes (Pisano et al., 2020).
Cancer immunotherapy, which aims to eliminate cancer cells by the host immune system, has attracted significant attention during the past decade (Wang W. et al., 2022b; Peng et al., 2022). Among different cancer immunotherapy strategies, immune checkpoint blockade has shown significant clinical effects in many tumors (Sharpe and Pauken, 2018). Zhang et al. prepared engineered cellular nanovesicles presenting the programmed death-1 (PD-1) receptor, which could selectively bind to programmed death-ligand 1 (PD-L1). The PD-1 nanovesicles induced an antitumor immune response (Zhang X. et al., 2018b). Zha et al. prepared gemcitabine-loaded PD-1 nanovesicles, which significantly suppressed tumor growth in mice, showing the unique advantage of PD-1-decorated exosome-mimics in cancer therapy (Zha et al., 2022). Signal regulatory protein-α (SIRPα) could bind to CD47 molecules on tumors and normal tissues and release a “do not eat me” signal to prevent the phagocytosis of cells (Ring et al., 2017). Rao et al. prepared SIRPα variant-presented extracellular vesicle mimics (SαV-C-NVS), which could disrupt the CD47-SIRPα axis and repolarize TAMs towards the M1 phenotype (Rao et al., 2020). These studies demonstrated the potential of functional exosome-mimics in drug delivery for cancer therapy.
5 Conclusion and perspectives
Exosomes and exosome-mimics have shown great potential in delivering various drugs and nanoparticles for cancer therapy. Exosomes derived from dendritic cells (DCs), mesenchymal stem cells (MSCs), and patient tumor cells have been used to deliver tumor antigens or anti-cancer drugs in some clinical trials (Chen et al., 2020). However, many problems remain to be solved to advance the further application of exosomes. For example, the limited yield of exosomes could not satisfy the therapeutic application in preclinical and clinical studies. Exosome-mimics, prepared from cell membranes on a large scale, showed promising potential in treating some diseases. Moreover, the drug loading efficiency into exosomes, especially for nucleic acid drugs, is relatively low. Developing novel strategies with efficient drug loading is urgently needed (Li S.-P. et al., 2018a). In addition, the unsatisfied transfection efficiency of exosomes dramatically influences the final gene regulation results in recipient cells. Further studies are required to improve the exosomal yield and drug loading efficiency. Exosomes and exosome-mimics have promising prospects as ideal drug delivery systems in cancer therapy.
Author contributions
FY and MW wrote the manuscript. FY designed the figures. XG supervised the study. All authors have read and agreed to the published version of the manuscript.
Funding
This study was supported by the Scientific Research Foundation for Advanced Talents, Taizhou University (No.T20220101026), Science and Technology Program of Taizhou city (22gya03), and the Science and Technology Innovation Project of Jilin Health Commission (2020J023).
Conflict of interest
The authors declare that the research was conducted in the absence of any commercial or financial relationships that could be construed as a potential conflict of interest.
Publisher’s note
All claims expressed in this article are solely those of the authors and do not necessarily represent those of their affiliated organizations, or those of the publisher, the editors and the reviewers. Any product that may be evaluated in this article, or claim that may be made by its manufacturer, is not guaranteed or endorsed by the publisher.
References
Ambattu, L. A., Ramesan, S., Dekiwadia, C., Hanssen, E., Li, H., and Yeo, L. Y. (2020). High frequency acoustic cell stimulation promotes exosome generation regulated by a calcium-dependent mechanism. Commun. Biol. 3 (1), 553. doi:10.1038/s42003-020-01277-6
Anand, P., Kunnumakkara, A. B., Newman, R. A., and Aggarwal, B. B. (2007). Bioavailability of curcumin: Problems and promises. Mol. Pharm. 4 (6), 807–818. doi:10.1021/mp700113r
Angsantikul, P., Thamphiwatana, S., Zhang, Q., Spiekermann, K., Zhuang, J., Fang, R. H., et al. (2018). Coating nanoparticles with gastric epithelial cell membrane for targeted antibiotic delivery against Helicobacter pylori infection. Adv. Ther. (Weinh.) 1 (2), 1800016. doi:10.1002/adtp.201800016
Aqil, F., Munagala, R., Jeyabalan, J., Agrawal, A. K., Kyakulaga, A.-H., Wilcher, S. A., et al. (2019). Milk exosomes-Natural nanoparticles for siRNA delivery. Cancer Lett. 449, 186–195. doi:10.1016/j.canlet.2019.02.011
Asadirad, A., Hashemi, S. M., Baghaei, K., Ghanbarian, H., Mortaz, E., Zali, M. R., et al. (2019). Phenotypical and functional evaluation of dendritic cells after exosomal delivery of miRNA-155. Life Sci. 219, 152–162. doi:10.1016/j.lfs.2019.01.005
Bagheri, E., Abnous, K., Farzad, S. A., Taghdisi, S. M., Ramezani, M., and Alibolandi, M. (2020). Targeted doxorubicin-loaded mesenchymal stem cells-derived exosomes as a versatile platform for fighting against colorectal cancer. Life Sci. 261, 118369. doi:10.1016/j.lfs.2020.118369
Bai, J., Duan, J., Liu, R., Du, Y., Luo, Q., Cui, Y., et al. (2020). Engineered targeting tLyp-1 exosomes as gene therapy vectors for efficient delivery of siRNA into lung cancer cells. Asian J. Pharm. Sci. 15 (4), 461–471. doi:10.1016/j.ajps.2019.04.002
Biadglegne, F., Rademacher, P., Sulbaran, D., Jaimes, Y. G., König, B., Rodloff, A. C., et al. (2021). Exosomes in serum-free cultures of THP-1 macrophages infected with Mycobacterium tuberculosis. Mol. Med. Rep. 24 (5), 815. doi:10.3892/mmr.2021.12455
Cao, Y., Wu, T., Zhang, K., Meng, X., Dai, W., Wang, D., et al. (2019). Engineered exosome-mediated near-infrared-II region V2C quantum dot delivery for nucleus-target low-temperature photothermal therapy. ACS Nano 13 (2), 1499–1510. doi:10.1021/acsnano.8b07224
Carobolante, G., Mantaj, J., Ferrari, E., and Vllasaliu, D. (2020). Cow milk and intestinal epithelial cell-derived extracellular vesicles as systems for enhancing oral drug delivery. Pharmaceutics 12 (3), 226. doi:10.3390/pharmaceutics12030226
Cassidy, J., and Misset, J.-L. (2002). Oxaliplatin-related side effects: Characteristics and management. Semin. Oncol. 29 (15), 11–20. doi:10.1053/sonc.2002.35524
Chen, Y.-S., Lin, E.-Y., Chiou, T.-W., and Harn, H.-J. (2020). Exosomes in clinical trial and their production in compliance with good manufacturing practice. Tzu-Chi Med. J. 32 (2), 113–120. doi:10.4103/tcmj.tcmj_182_19
Chew, V., Toh, H. C., and Abastado, J. P. (2012). Immune microenvironment in tumor progression: Characteristics and challenges for therapy. J. Oncol. 2012, 608406. doi:10.1155/2012/608406
Cidon, E. U., Martinez, P. A., and Hickish, T. (2018). Gemcitabine-induced haemolytic uremic syndrome, although infrequent, can it be prevented: A case report and review of literature. World J. Clin. Cases 6 (12), 531–537. doi:10.12998/wjcc.v6.i12.531
Coughlan, C., Bruce, K. D., Burgy, O., Boyd, T. D., Michel, C. R., Garcia-Perez, J. E., et al. (2020). Exosome isolation by ultracentrifugation and precipitation and techniques for downstream analyses. Curr. Protoc. Cell Biol. 88 (1), e110. doi:10.1002/cpcb.110
De Sanctis, F., Lamolinara, A., Boschi, F., Musiu, C., Caligola, S., Trovato, R., et al. (2022). Interrupting the nitrosative stress fuels tumor-specific cytotoxic T lymphocytes in pancreatic cancer. J. Immunother. Cancer 10 (1), e003549. doi:10.1136/jitc-2021-003549
Değirmenci, N. S., Uslu, M., Kırbaş, O. K., Şahin, F., and Uçar, E. Ö. (2022). Lapatinib loaded exosomes as a drug delivery system in breast cancer. J. Drug Deliv. Sci. Tec. 75, 103584. doi:10.1016/j.jddst.2022.103584
Diehl, J. N., Klomp, J. E., Snare, K. R., Hibshman, P. S., Blake, D. R., Kaiser, Z. D., et al. (2021). The KRAS-regulated kinome identifies WEE1 and ERK coinhibition as a potential therapeutic strategy in KRAS-mutant pancreatic cancer. J. Biol. Chem. 297 (5), 101335. doi:10.1016/j.jbc.2021.101335
Dourado, M. R., Korvala, J., Åström, P., De Oliveira, C. E., Cervigne, N. K., Mofatto, L. S., et al. (2019). Extracellular vesicles derived from cancer-associated fibroblasts induce the migration and invasion of oral squamous cell carcinoma. J. Extracell. Vesicles 8 (1), 1578525. doi:10.1080/20013078.2019.1578525
Esposito, C. L., Quintavalle, C., Ingenito, F., Rotoli, D., Roscigno, G., Nuzzo, S., et al. (2021). Identification of a novel RNA aptamer that selectively targets breast cancer exosomes. Mol. Ther. Nucleic Acids 23, 982–994. doi:10.1016/j.omtn.2021.01.012
Fader, C. M., and Colombo, M. I. (2009). Autophagy and multivesicular bodies: Two closely related partners. Cell Death Differ. 16 (1), 70–78. doi:10.1038/cdd.2008.168
Fan, Y., Cui, Y., Hao, W., Chen, M., Liu, Q., Wang, Y., et al. (2021). Carrier-free highly drug-loaded biomimetic nanosuspensions encapsulated by cancer cell membrane based on homology and active targeting for the treatment of glioma. Bioact. Mat. 6 (12), 4402–4414. doi:10.1016/j.bioactmat.2021.04.027
Fan, Z., Xiao, K., Lin, J., Liao, Y., and Huang, X. (2019). Functionalized DNA enables programming exosomes/vesicles for tumor imaging and therapy. Small 15 (47), e1903761. doi:10.1002/smll.201903761
Fang, J., and Liang, W. (2021). ASCs-derived exosomes loaded with vitamin A and quercetin inhibit rapid senescence-like response after acute liver injury. Biochem. Biophys. Res. Commun. 572, 125–130. doi:10.1016/j.bbrc.2021.07.059
Fang, R. H., Hu, C. M., Luk, B. T., Gao, W., Copp, J. A., Tai, Y., et al. (2014). Cancer cell membrane-coated nanoparticles for anti-cancer vaccination and drug delivery. Nano Lett. 14 (4), 2181–2188. doi:10.1021/nl500618u
Farazi, T. A., Hoell, J. I., Morozov, P., and Tuschl, T. (2013). MicroRNAs in human cancer. Adv. Exp. Med. Biol. 774, 1–20. doi:10.1007/978-94-007-5590-1_1
Faruqu, F. N., Xu, L., and Al-Jamal, K. T. (2018). Preparation of exosomes for siRNA delivery to cancer cells. J. Vis. Exp. 142, e58814. doi:10.3791/58814
Gonzalez-Begne, M., Lu, B., Han, X., Hagen, F. K., Hand, A. R., Melvin, J. E., et al. (2009). Proteomic analysis of human parotid gland exosomes by multidimensional protein identification technology (MudPIT). J. Proteome Res. 8 (3), 1304–1314. doi:10.1021/pr800658c
González-Sarrías, A., Iglesias-Aguirre, C. E., Cortés-Martín, A., Vallejo, F., Cattivelli, A., del Pozo-Acebo, L., et al. (2022). Milk-derived exosomes as nanocarriers to deliver curcumin and resveratrol in breast tissue and enhance their anticancer activity. Int. J. Mol. Sci. 23 (5), 2860. doi:10.3390/ijms23052860
Gupta, S., Rawat, S., Arora, V., Kottarath, S. K., Dinda, A. K., Vaishnav, P. K., et al. (2018). An improvised one-step sucrose cushion ultracentrifugation method for exosome isolation from culture supernatants of mesenchymal stem cells. Stem Cell Res. Ther. 9 (1), 180. doi:10.1186/s13287-018-0923-0
Gutkin, A., Uziel, O., Beery, E., Nordenberg, J., Pinchasi, M., Goldvaser, H., et al. (2016). Tumor cells derived exosomes contain hTERT mRNA and transform nonmalignant fibroblasts into telomerase positive cells. Oncotarget 7 (37), 59173–59188. doi:10.18632/oncotarget.10384
Hadla, M., Palazzolo, S., Corona, G., Caligiuri, I., Canzonieri, V., Toffoli, G., et al. (2016). Exosomes increase the therapeutic index of doxorubicin in breast and ovarian cancer mouse models. Nanomedicine 11 (18), 2431–2441. doi:10.2217/nnm-2016-0154
Han, M., Hu, J., Lu, P., Cao, H., Yu, C., Li, X., et al. (2020). Exosome-transmitted miR-567 reverses trastuzumab resistance by inhibiting ATG5 in breast cancer. Cell Death Dis. 11 (1), 43. doi:10.1038/s41419-020-2250-5
He, W., Mei, Q., Li, J., Zhai, Y., Chen, Y., Wang, R., et al. (2021). Preferential targeting cerebral ischemic lesions with cancer cell-inspired nanovehicle for ischemic stroke treatment. Nano Lett. 21 (7), 3033–3043. doi:10.1021/acs.nanolett.1c00231
Huang, W., Qu, M., Li, L., Liu, T., Lin, M., and Yu, X. (2021). SiRNA in MSC-derived exosomes silences CTGF gene for locomotor recovery in spinal cord injury rats. Stem Cell Res. Ther. 12 (1), 334. doi:10.1186/s13287-021-02401-x
Jang, S. C., Kim, O. Y., Yoon, C. M., Choi, D.-S., Roh, T.-Y., Park, J., et al. (2013). Bioinspired exosome-mimetic nanovesicles for targeted delivery of chemotherapeutics to malignant tumors. ACS Nano 7 (9), 7698–7710. doi:10.1021/nn402232g
Johnsen, K. B., Gudbergsson, J. M., Skov, M. N., Pilgaard, L., Moos, T., and Duroux, M. (2014). A comprehensive overview of exosomes as drug delivery vehicles - endogenous nanocarriers for targeted cancer therapy. Biochim. Biophys. Acta 1846 (1), 75–87. doi:10.1016/j.bbcan.2014.04.005
Johnstone, R. M., Adam, M., Hammond, J., Orr, L., and Turbide, C. (1987). Vesicle formation during reticulocyte maturation. Association of plasma membrane activities with released vesicles (exosomes). J. Biol. Chem. 262 (19), 9412–9420. doi:10.1016/s0021-9258(18)48095-7
Jung, H. H., Kim, J.-Y., Lim, J. E., and Im, Y.-H. (2020). Cytokine profiling in serum-derived exosomes isolated by different methods. Sci. Rep. 10 (1), 14069. doi:10.1038/s41598-020-70584-z
Kamerkar, S., LeBleu, V. S., Sugimoto, H., Yang, S., Ruivo, C. F., Melo, S. A., et al. (2017). Exosomes facilitate therapeutic targeting of oncogenic KRAS in pancreatic cancer. Nature 546 (7659), 498–503. doi:10.1038/nature22341
Kim, J., Song, Y., Park, C. H., and Choi, C. (2021). Platform technologies and human cell lines for the production of therapeutic exosomes. Extracell. Vesicles Circ. Nucleic Acids 2 (1), 3–17. doi:10.20517/evcna.2020.01
Kim, M., Yun, H.-W., Park, D. Y., Choi, B. H., and Min, B.-H. (2018). Three-dimensional spheroid culture increases exosome secretion from mesenchymal stem cells. Tissue Eng. Regen. Med. 15 (4), 427–436. doi:10.1007/s13770-018-0139-5
Kroll, A. V., Fang, R. H., Jiang, Y., Zhou, J., Wei, X., Yu, C. L., et al. (2017). Nanoparticulate delivery of cancer cell membrane elicits multiantigenic antitumor immunity. Adv. Mat. 29 (47), 1703969. doi:10.1002/adma.201703969
Lamichhane, T. N., Raiker, R. S., and Jay, S. M. (2015). Exogenous DNA loading into extracellular vesicles via electroporation is size-dependent and enables limited gene delivery. Mol. Pharm. 12 (10), 3650–3657. doi:10.1021/acs.molpharmaceut.5b00364
Leal, F., and García-Perdomo, H. A. (2019). Effectiveness of platinum-based chemotherapy in patients with metastatic prostate cancer: Systematic review and meta-analysis. Clin. Genitourin. Cancer 17 (3), e627–e644. doi:10.1016/j.clgc.2019.03.008
Lee, Y., El Andaloussi, S., and Wood, M. J. (2012). Exosomes and microvesicles: Extracellular vesicles for genetic information transfer and gene therapy. Hum. Mol. Genet. 21 (R1), R125–R134. doi:10.1093/hmg/dds317
Li, H., Wang, X., Guo, X., Wan, Q., Teng, Y., and Liu, J. (2022). Development of rapamycin-encapsulated exosome-mimetic nanoparticles-in-PLGA microspheres for treatment of hemangiomas. Biomed. Pharmacother. 148, 112737. doi:10.1016/j.biopha.2022.112737
Li, S.-P., Lin, Z.-X., Jiang, X.-Y., and Yu, X.-Y. (2018a). Exosomal cargo-loading and synthetic exosome-mimics as potential therapeutic tools. Acta Pharmacol. Sin. 39 (4), 542–551. doi:10.1038/aps.2017.178
Li, Y.-J., Wu, J.-Y., Wang, J.-M., Hu, X.-B., Cai, J.-X., and Xiang, D.-X. (2020). Gemcitabine loaded autologous exosomes for effective and safe chemotherapy of pancreatic cancer. Acta Biomater. 101, 519–530. doi:10.1016/j.actbio.2019.10.022
Li, Y., Gao, Y., Gong, C., Wang, Z., Xia, Q., Gu, F., et al. (2018b). A33 antibody-functionalized exosomes for targeted delivery of doxorubicin against colorectal cancer. Nanomedicine 14 (7), 1973–1985. doi:10.1016/j.nano.2018.05.020
Liao, W., Du, Y., Zhang, C., Pan, F., Yao, Y., Zhang, T., et al. (2019). Exosomes: The next generation of endogenous nanomaterials for advanced drug delivery and therapy. Acta Biomater. 86, 1–14. doi:10.1016/j.actbio.2018.12.045
Lou, G., Song, X., Yang, F., Wu, S., Wang, J., Chen, Z., et al. (2015). Exosomes derived from miR-122-modified adipose tissue-derived MSCs increase chemosensitivity of hepatocellular carcinoma. J. Hematol. Oncol. 8, 122. doi:10.1186/s13045-015-0220-7
Ludwig, N., Azambuja, J. H., Rao, A., Gillespie, D. G., Jackson, E. K., and Whiteside, T. L. J. P. S. (2020). Adenosine receptors regulate exosome production. Purinergic Signal. 16 (2), 231–240. doi:10.1007/s11302-020-09700-7
Maeda, H., and Khatami, M. (2018). Analyses of repeated failures in cancer therapy for solid tumors: Poor tumor-selective drug delivery, low therapeutic efficacy and unsustainable costs. Clin. Transl. Med. 7 (1), 11. doi:10.1186/s40169-018-0185-6
Mathieu, M., Névo, N., Jouve, M., Valenzuela, J. I., Maurin, M., Verweij, F. J., et al. (2021). Specificities of exosome versus small ectosome secretion revealed by live intracellular tracking of CD63 and CD9. Nat. Commun. 12 (1), 4389. doi:10.1038/s41467-021-24384-2
Mattiuzzi, C., and Lippi, G. (2019). Current cancer epidemiology. J. Epidemiol. Glob. Health 9 (4), 217–222. doi:10.2991/jegh.k.191008.001
Mendell, J. R., Al-Zaidy, S. A., Rodino-Klapac, L. R., Goodspeed, K., Gray, S. J., Kay, C. N., et al. (2021). Current clinical applications of in vivo gene therapy with AAVs. Mol. Ther. 29 (2), 464–488. doi:10.1016/j.ymthe.2020.12.007
Merendino, A. M., Bucchieri, F., Campanella, C., Marciano, V., Ribbene, A., David, S., et al. (2010). Hsp60 is actively secreted by human tumor cells. PLoS One 5 (2), e9247. doi:10.1371/journal.pone.0009247
Misra, S. (2013). Human gene therapy: A brief overview of the genetic revolution. J. Assoc. Physicians India 61 (2), 127–133. doi:10.0000/PMID24471251
Mizrak, A., Bolukbasi, M. F., Ozdener, G. B., Brenner, G. J., Madlener, S., Erkan, E. P., et al. (2013). Genetically engineered microvesicles carrying suicide mRNA/protein inhibit schwannoma tumor growth. Mol. Ther. 21 (1), 101–108. doi:10.1038/mt.2012.161
Mo, L. J., Song, M., Huang, Q. H., Guan, H., Liu, X. D., Xie, D. F., et al. (2018). Exosome-packaged miR-1246 contributes to bystander DNA damage by targeting LIG4. Br. J. Cancer 119 (4), 492–502. doi:10.1038/s41416-018-0192-9
Moar, P., and Tandon, R. (2021). Galectin-9 as a biomarker of disease severity. Cell. Immunol. 361, 104287. doi:10.1016/j.cellimm.2021.104287
Mohammed, H. R., Bayraktar, E., Gouda, K. H., Abd-Ellah, M. F., Amero, P., Chavez-Reyes, A., et al. (2017). Exosomes: From garbage bins to promising therapeutic targets. Int. J. Mol. Sci. 18 (3), 538. doi:10.3390/ijms18030538
Muller, L., Hong, C.-S., Stolz, D. B., Watkins, S. C., and Whiteside, T. L. (2014). Isolation of biologically-active exosomes from human plasma. J. Immunol. Methods 411, 55–65. doi:10.1016/j.jim.2014.06.007
Ngoune, R., Peters, A., von Elverfeldt, D., Winkler, K., and Pütz, G. (2016). Accumulating nanoparticles by EPR: A route of no return. J. Control. Release 238, 58–70. doi:10.1016/j.jconrel.2016.07.028
Nie, D., Dai, Z., Li, J., Yang, Y., Xi, Z., Wang, J., et al. (2019). Cancer-cell-membrane-coated nanoparticles with a yolk–shell structure augment cancer chemotherapy. Nano Lett. 20 (2), 936–946. doi:10.1021/acs.nanolett.9b03817
Novina, C. D., and Sharp, P. A. (2004). The RNAi revolution. Nature 430 (6996), 161–164. doi:10.1038/430161a
Ohno, S.-i., Takanashi, M., Sudo, K., Ueda, S., Ishikawa, A., Matsuyama, N., et al. (2013). Systemically injected exosomes targeted to EGFR deliver antitumor MicroRNA to breast cancer cells. Mol. Ther. 21 (1), 185–191. doi:10.1038/mt.2012.180
Oroojalian, F., Beygi, M., Baradaran, B., Mokhtarzadeh, A., and Shahbazi, M. A. (2021). Immune cell Membrane-Coated biomimetic nanoparticles for targeted cancer therapy. Small 17 (12), 2006484. doi:10.1002/smll.202006484
Pan, S., Pei, L., Zhang, A., Zhang, Y., Zhang, C., Huang, M., et al. (2020). Passion fruit-like exosome-PMA/Au-BSA@ Ce6 nanovehicles for real-time fluorescence imaging and enhanced targeted photodynamic therapy with deep penetration and superior retention behavior in tumor. Biomaterials 230, 119606. doi:10.1016/j.biomaterials.2019.119606
Pan, Y., Lin, Y., and Mi, C. (2021). Cisplatin-resistant osteosarcoma cell-derived exosomes confer cisplatin resistance to recipient cells in an exosomal circ_103801-dependent manner. Cell Biol. Int. 45 (4), 858–868. doi:10.1002/cbin.11532
Pegtel, D. M., and Gould, S. J. (2019). Exosomes. Annu. Rev. Biochem. 88, 487–514. doi:10.1146/annurev-biochem-013118-111902
Pei, X., Zhang, X., Zhang, L., Yuan, M., Sun, L., Yu, F., et al. (2021). Targeted exosomes for co-delivery of siFGL1 and siTGF-β1 trigger combined cancer immunotherapy by remodeling immunosuppressive tumor microenvironment. Chem. Eng. J. 421, 129774. doi:10.1016/j.cej.2021.129774
Peng, B., Nguyen, T. M., Jayasinghe, M. K., Gao, C., Pham, T. T., Vu, L. T., et al. (2022). Robust delivery of RIG-I agonists using extracellular vesicles for anti-cancer immunotherapy. J. Extracell. Vesicles 11 (4), e12187. doi:10.1002/jev2.12187
Pisano, S., Pierini, I., Gu, J., Gazze, A., Francis, L. W., Gonzalez, D., et al. (2020). Immune (cell) derived exosome mimetics (IDEM) as a treatment for ovarian cancer. Front. Cell Dev. Biol. 8, 553576. doi:10.3389/fcell.2020.553576
Rao, L., Wu, L., Liu, Z., Tian, R., Yu, G., Zhou, Z., et al. (2020). Hybrid cellular membrane nanovesicles amplify macrophage immune responses against cancer recurrence and metastasis. Nat. Commun. 11 (1), 4909. doi:10.1038/s41467-020-18626-y
Razi, M., and Futter, C. E. (2006). Distinct roles for Tsg101 and Hrs in multivesicular body formation and inward vesiculation. Mol. Biol. Cell 17 (8), 3469–3483. doi:10.1091/mbc.e05-11-1054
Ring, N. G., Herndler-Brandstetter, D., Weiskopf, K., Shan, L., Volkmer, J. P., George, B. M., et al. (2017). Anti-SIRPα antibody immunotherapy enhances neutrophil and macrophage antitumor activity. Proc. Natl. Acad. Sci. U. S. A. 114 (49), E10578–E10585. doi:10.1073/pnas.1710877114
Rui, K., Hong, Y., Zhu, Q., Shi, X., Xiao, F., Fu, H., et al. (2021). Olfactory ecto-mesenchymal stem cell-derived exosomes ameliorate murine Sjögren’s syndrome by modulating the function of myeloid-derived suppressor cells. Cell. Mol. Immunol. 18 (2), 440–451. doi:10.1038/s41423-020-00587-3
Seifert, A. M., Reiche, C., Heiduk, M., Tannert, A., Meinecke, A.-C., Baier, S., et al. (2020). Detection of pancreatic ductal adenocarcinoma with galectin-9 serum levels. Oncogene 39 (15), 3102–3113. doi:10.1038/s41388-020-1186-7
Severic, M., Ma, G., Pereira, S. G., Ruiz, A., Cheung, C. C., and Al-Jamal, W. T. (2021). Genetically-engineered anti-PSMA exosome mimetics targeting advanced prostate cancer in vitro and in vivo. J. Control. Release 330, 101–110. doi:10.1016/j.jconrel.2020.12.017
Sharpe, A. H., and Pauken, K. E. (2018). The diverse functions of the PD1 inhibitory pathway. Nat. Rev. Immunol. 18 (3), 153–167. doi:10.1038/nri.2017.108
Shtam, T. A., Kovalev, R. A., Varfolomeeva, E. Y., Makarov, E. M., Kil, Y. V., and Filatov, M. V. (2013). Exosomes are natural carriers of exogenous siRNA to human cells in vitro. Cell Commun. Signal. 11, 88. doi:10.1186/1478-811X-11-88
Song, R., Li, T., Ye, J., Sun, F., Hou, B., Saeed, M., et al. (2021). Acidity-Activatable dynamic nanoparticles boosting ferroptotic cell death for immunotherapy of cancer. Adv. Mat. 33 (31), 2101155. doi:10.1002/adma.202101155
Subra, C., Grand, D., Laulagnier, K., Stella, A., Lambeau, G., Paillasse, M., et al. (2010). Exosomes account for vesicle-mediated transcellular transport of activatable phospholipases and prostaglandins. J. Lipid Res. 51 (8), 2105–2120. doi:10.1194/jlr.M003657
Sun, H., Wang, C., Hu, B., Gao, X., Zou, T., Luo, Q., et al. (2021). Exosomal S100A4 derived from highly metastatic hepatocellular carcinoma cells promotes metastasis by activating STAT3. Signal Transduct. Target. Ther. 6 (1), 187. doi:10.1038/s41392-021-00579-3
Suresh, P. S., Venkatesh, T., and Tsutsumi, R. (2014). Gene therapy in endocrine tumors: A comprehensive overview. Endocr. J. 61 (7), 655–665. doi:10.1507/endocrj.ej14-0007
Thakur, A., Sidu, R. K., Zou, H., Alam, M. K., Yang, M., and Lee, Y. (2020). Inhibition of glioma cells’ proliferation by doxorubicin-loaded exosomes via microfluidics. Int. J. Nanomedicine 15, 8331–8343. doi:10.2147/IJN.S263956
Thamphiwatana, S., Angsantikul, P., Escajadillo, T., Zhang, Q., Olson, J., Luk, B. T., et al. (2017). Macrophage-like nanoparticles concurrently absorbing endotoxins and proinflammatory cytokines for sepsis management. Proc. Natl. Acad. Sci. U. S. A. 114 (43), 11488–11493. doi:10.1073/pnas.1714267114
Tian, Y., Li, S., Song, J., Ji, T., Zhu, M., Anderson, G. J., et al. (2014). A doxorubicin delivery platform using engineered natural membrane vesicle exosomes for targeted tumor therapy. Biomaterials 35 (7), 2383–2390. doi:10.1016/j.biomaterials.2013.11.083
Van Niel, G., d'Angelo, G., and Raposo, G. (2018). Shedding light on the cell biology of extracellular vesicles. Nat. Rev. Mol. Cell Biol. 19 (4), 213–228. doi:10.1038/nrm.2017.125
Wang, L., Cheng, W., Zhu, J., Li, W., Li, D., Yang, X., et al. (2022a). Electrospun nanoyarn and exosomes of adipose-derived stem cells for urethral regeneration: Evaluations in vitro and in vivo. Colloids Surf. B Biointerfaces 209, 112218. doi:10.1016/j.colsurfb.2021.112218
Wang, L., He, J., Hu, H., Tu, L., Sun, Z., Liu, Y., et al. (2020). Lung CSC-derived exosomal miR-210-3p contributes to a pro-metastatic phenotype in lung cancer by targeting FGFRL1. J. Cell. Mol. Med. 24 (11), 6324–6339. doi:10.1111/jcmm.15274
Wang, M., Ji, S., Shao, G., Zhang, J., Zhao, K., Wang, Z., et al. (2018). Effect of exosome biomarkers for diagnosis and prognosis of breast cancer patients. Clin. Transl. Oncol. 20 (7), 906–911. doi:10.1007/s12094-017-1805-0
Wang, P., Wang, H., Huang, Q., Peng, C., Yao, L., Chen, H., et al. (2019). Exosomes from M1-polarized macrophages enhance paclitaxel antitumor activity by activating macrophages-mediated inflammation. Theranostics 9 (6), 1714–1727. doi:10.7150/thno.30716
Wang, W., Zhao, J., Hao, C., Hu, S., Chen, C., Cao, Y., et al. (2022b). The development of chiral nanoparticles to target NK cells and CD8+ T cells for cancer immunotherapy. Adv. Mat. 34 (16), 2109354. doi:10.1002/adma.202109354
Wei, X., Ying, M., Dehaini, D., Su, Y., Kroll, A. V., Zhou, J., et al. (2018a). Nanoparticle functionalization with platelet membrane enables multifactored biological targeting and detection of atherosclerosis. ACS Nano 12 (1), 109–116. doi:10.1021/acsnano.7b07720
Wei, X., Zhang, G., Ran, D., Krishnan, N., Fang, R. H., Gao, W., et al. (2018b). T-Cell-Mimicking nanoparticles can neutralize HIV infectivity. Adv. Mat. 30 (45), e1802233. doi:10.1002/adma.201802233
Xin, H., Li, Y., Buller, B., Katakowski, M., Zhang, Y., Wang, X., et al. (2012). Exosome-mediated transfer of miR-133b from multipotent mesenchymal stromal cells to neural cells contributes to neurite outgrowth. Stem Cells 30 (7), 1556–1564. doi:10.1002/stem.1129
Xiong, L., Zhen, S., Yu, Q., and Gong, Z. (2017). HCV-E2 inhibits hepatocellular carcinoma metastasis by stimulating mast cells to secrete exosomal shuttle microRNAs. Oncol. Lett. 14 (2), 2141–2146. doi:10.3892/ol.2017.6433
Yang, T., Martin, P., Fogarty, B., Brown, A., Schurman, K., Phipps, R., et al. (2015). Exosome delivered anti-cancer drugs across the blood-brain barrier for brain cancer therapy in Danio rerio. Pharm. Res. 32 (6), 2003–2014. doi:10.1007/s11095-014-1593-y
Yang, X., Shi, G., Guo, J., Wang, C., and He, Y. (2018). Exosome-encapsulated antibiotic against intracellular infections of methicillin-resistant Staphylococcus aureus. Int. J. Nanomedicine 13, 8095–8104. doi:10.2147/IJN.S179380
Yang, Z., Shi, J., Xie, J., Wang, Y., Sun, J., Liu, T., et al. (2020). Large-scale generation of functional mRNA-encapsulating exosomes via cellular nanoporation. Nat. Biomed. Eng. 4 (1), 69–83. doi:10.1038/s41551-019-0485-1
Yao, S., Yin, Y., Jin, G., Li, D., Li, M., Hu, Y., et al. (2020). Exosome-mediated delivery of miR-204-5p inhibits tumor growth and chemoresistance. Cancer Med. 9 (16), 5989–5998. doi:10.1002/cam4.3248
Ye, Y., Li, S. L., Ma, Y. Y., Diao, Y. J., Yang, L., Su, M. Q., et al. (2017). Exosomal miR-141-3p regulates osteoblast activity to promote the osteoblastic metastasis of prostate cancer. Oncotarget 8 (55), 94834–94849. doi:10.18632/oncotarget.22014
Yong, T., Zhang, X., Bie, N., Zhang, H., Zhang, X., Li, F., et al. (2019). Tumor exosome-based nanoparticles are efficient drug carriers for chemotherapy. Nat. Commun. 10 (1), 3838. doi:10.1038/s41467-019-11718-4
Zha, H., Xu, Z., Xu, X., Lu, X., Shi, P., Xiao, Y., et al. (2022). PD-1 cellular nanovesicles carrying gemcitabine to inhibit the proliferation of triple negative breast cancer cell. Pharmaceutics 14 (6), 1263. doi:10.3390/pharmaceutics14061263
Zhang, D., Cai, G., Liu, K., Zhuang, Z., Jia, K., Pei, S., et al. (2021a). Microglia exosomal miRNA-137 attenuates ischemic brain injury through targeting Notch1. Aging 13 (3), 4079–4095. doi:10.18632/aging.202373
Zhang, H., Yan, C., and Wang, Y. (2021b). Exosome-mediated transfer of circHIPK3 promotes trastuzumab chemoresistance in breast cancer. J. Drug Target. 29 (9), 1004–1015. doi:10.1080/1061186X.2021.1906882
Zhang, M., Yu, Q., Tang, W., Wu, Y., Lv, J., Sun, L., et al. (2021c). Epithelial exosomal contactin-1 promotes monocyte-derived dendritic cell–dominant T-cell responses in asthma. J. Allergy Clin. Immunol. 148 (6), 1545–1558. doi:10.1016/j.jaci.2021.04.025
Zhang, Q., Dehaini, D., Zhang, Y., Zhou, J., Chen, X., Zhang, L., et al. (2018a). Neutrophil membrane-coated nanoparticles inhibit synovial inflammation and alleviate joint damage in inflammatory arthritis. Nat. Nanotechnol. 13 (12), 1182–1190. doi:10.1038/s41565-018-0254-4
Zhang, W., Yu, M., Xi, Z., Nie, D., Dai, Z., Wang, J., et al. (2019). Cancer cell membrane-camouflaged nanorods with endoplasmic reticulum targeting for improved antitumor therapy. ACS Appl. Mat. Interfaces 11 (50), 46614–46625. doi:10.1021/acsami.9b18388
Zhang, X., Angsantikul, P., Ying, M., Zhuang, J., Zhang, Q., Wei, X., et al. (2017). Remote loading of small-molecule therapeutics into cholesterol-enriched cell-membrane -derived vesicles. Angew. Chem. Int. Ed. Engl. 56 (45), 14075–14079. doi:10.1002/anie.201707598
Zhang, X., Liu, L., Tang, M., Li, H., Guo, X., and Yang, X. (2020). The effects of umbilical cord-derived macrophage exosomes loaded with cisplatin on the growth and drug resistance of ovarian cancer cells. Drug Dev. Ind. Pharm. 46 (7), 1150–1162. doi:10.1080/03639045.2020.1776320
Zhang, X., Wang, C., Wang, J., Hu, Q., Langworthy, B., Ye, Y., et al. (2018b). PD-1 blockade cellular vesicles for cancer immunotherapy. Adv. Mat. 30 (22), e1707112. doi:10.1002/adma.201707112
Zhou, W., Fong, M. Y., Min, Y., Somlo, G., Liu, L., Palomares, M. R., et al. (2014). Cancer-secreted miR-105 destroys vascular endothelial barriers to promote metastasis. Cancer Cell 25 (4), 501–515. doi:10.1016/j.ccr.2014.03.007
Zhou, W., Zhou, Y., Chen, X., Ning, T., Chen, H., Guo, Q., et al. (2021). Pancreatic cancer-targeting exosomes for enhancing immunotherapy and reprogramming tumor microenvironment. Biomaterials 268, 120546. doi:10.1016/j.biomaterials.2020.120546
Zhu, D., Zhang, T., Li, Y., Huang, C., Suo, M., Xia, L., et al. (2022). Tumor-derived exosomes co-delivering aggregation-induced emission luminogens and proton pump inhibitors for tumor glutamine starvation therapy and enhanced type-I photodynamic therapy. Biomaterials 283, 121462. doi:10.1016/j.biomaterials.2022.121462
Zhu, Q., Ling, X., Yang, Y., Zhang, J., Li, Q., Niu, X., et al. (2019). Embryonic stem cells-derived exosomes endowed with targeting properties as chemotherapeutics delivery vehicles for glioblastoma therapy. Adv. Sci. 6 (6), 1801899. doi:10.1002/advs.201801899
Zhuang, X., Xiang, X., Grizzle, W., Sun, D., Zhang, S., Axtell, R. C., et al. (2011). Treatment of brain inflammatory diseases by delivering exosome encapsulated anti-inflammatory drugs from the nasal region to the brain. Mol. Ther. 19 (10), 1769–1779. doi:10.1038/mt.2011.164
Keywords: exosome, cancer therapy, nanovesicle, drug delivery, tumor targeting
Citation: Yang F, Wang M and Guan X (2022) Exosomes and mimics as novel delivery platform for cancer therapy. Front. Pharmacol. 13:1001417. doi: 10.3389/fphar.2022.1001417
Received: 23 July 2022; Accepted: 30 September 2022;
Published: 12 October 2022.
Edited by:
Sabrina Oliveira, Utrecht University, NetherlandsReviewed by:
Zhifang Zhou, Jiangnan University, ChinaChiara Martinelli, Politecnico di Milano, Italy
Copyright © 2022 Yang, Wang and Guan. This is an open-access article distributed under the terms of the Creative Commons Attribution License (CC BY). The use, distribution or reproduction in other forums is permitted, provided the original author(s) and the copyright owner(s) are credited and that the original publication in this journal is cited, in accordance with accepted academic practice. No use, distribution or reproduction is permitted which does not comply with these terms.
*Correspondence: Xingang Guan, Z3VhbnhnQGNpYWMuYWMuY24=
†These authors have contributed equally to this work