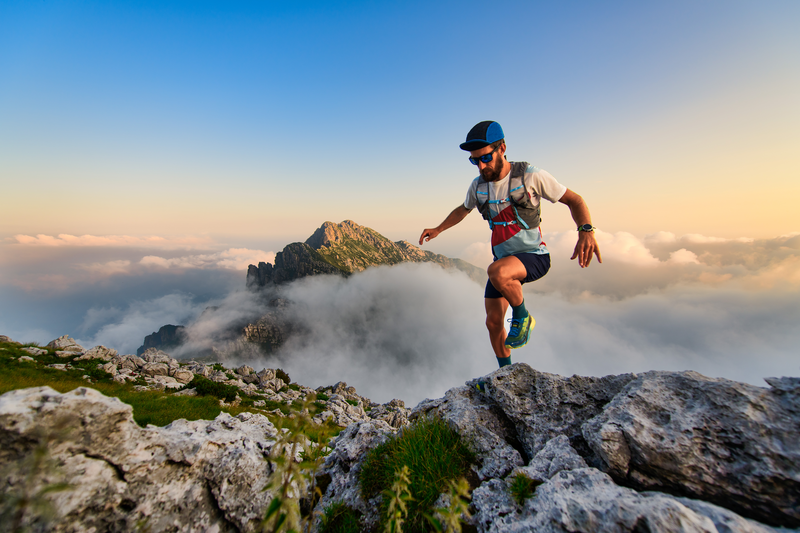
94% of researchers rate our articles as excellent or good
Learn more about the work of our research integrity team to safeguard the quality of each article we publish.
Find out more
REVIEW article
Front. Pharmacol. , 20 January 2022
Sec. Respiratory Pharmacology
Volume 12 - 2021 | https://doi.org/10.3389/fphar.2021.806393
This article is part of the Research Topic Fibrosis in the Respiratory and Digestive Systems View all 50 articles
Empyema, a severe complication of pneumonia, trauma, and surgery is characterized by fibrinopurulent effusions and loculations that can result in lung restriction and resistance to drainage. For decades, efforts have been focused on finding a universal treatment that could be applied to all patients with practice recommendations varying between intrapleural fibrinolytic therapy (IPFT) and surgical drainage. However, despite medical advances, the incidence of empyema has increased, suggesting a gap in our understanding of the pathophysiology of this disease and insufficient crosstalk between clinical practice and preclinical research, which slows the development of innovative, personalized therapies. The recent trend towards less invasive treatments in advanced stage empyema opens new opportunities for pharmacological interventions. Its remarkable efficacy in pediatric empyema makes IPFT the first line treatment. Unfortunately, treatment approaches used in pediatrics cannot be extrapolated to empyema in adults, where there is a high level of failure in IPFT when treating advanced stage disease. The risk of bleeding complications and lack of effective low dose IPFT for patients with contraindications to surgery (up to 30%) promote a debate regarding the choice of fibrinolysin, its dosage and schedule. These challenges, which together with a lack of point of care diagnostics to personalize treatment of empyema, contribute to high (up to 20%) mortality in empyema in adults and should be addressed preclinically using validated animal models. Modern preclinical studies are delivering innovative solutions for evaluation and treatment of empyema in clinical practice: low dose, targeted treatments, novel biomarkers to predict IPFT success or failure, novel delivery methods such as encapsulating fibrinolysin in echogenic liposomal carriers to increase the half-life of plasminogen activator. Translational research focused on understanding the pathophysiological mechanisms that control 1) the transition from acute to advanced-stage, chronic empyema, and 2) differences in outcomes of IPFT between pediatric and adult patients, will identify new molecular targets in empyema. We believe that seamless bidirectional communication between those working at the bedside and the bench would result in novel personalized approaches to improve pharmacological treatment outcomes, thus widening the window for use of IPFT in adult patients with advanced stage empyema.
Empyema is a common, potentially life-threatening infection of the pleural space with several different etiologies (Franklin et al., 2021). The incidence and mortality of empyema appears to be increasing over the past 4 decades despite advances in modern medicine (Finley et al., 2008; Bender et al., 2009; Burgos et al., 2011; Grijalva et al., 2011; Burgos et al., 2013; Bedawi et al., 2018; Godfrey et al., 2019). The estimated sixty-five thousand cases of empyema that occur annually in the United States demonstrate a mortality between 10 and 27%, with greater likelihood of worse outcomes if initial treatment is unsuccessful (Chapman and Davies, 2004; Maskell et al., 2006; Porcel and Light, 2006; Koegelenberg et al., 2008; Ben-Or et al., 2011; Light, 2011; Abu-Daff et al., 2013; Reichert et al., 2017; Shen et al., 2017; Meyer et al., 2018; Semenkovich et al., 2018). Treatment of patients with empyema has been estimated to cost up to half a billion dollars per annum (Taylor and Kozower, 2012). The American Thoracic Society recognizes three sequential stages in the development of empyema (I) early exudative, (II) fibrinopurulent, and (III) organized. Although evacuation of pleural fluid and lung re-expansion can be achieved by thoracentesis in stage I, the increased fibrin deposition in the pleural space observed in stage II may require treatment with intrapleural fibrinolytic therapy (IPFT) or video-assisted thoracoscopic surgery (VATS). Treatment of patients with stage II empyema remains controversial in adults, with pharmacological and surgical approaches being used contingent on practitioner preference and hospital protocols. Stage III, which features a thick pleural rind and trapped lung, often requires open decortication of the lung via thoracotomy (Andrews, 1965; Light, 2011; Søgaard et al., 2014; Reichert et al., 2017; Reichert et al., 2018b; Reichert and Bodner, 2018; Semenkovich et al., 2018; Godfrey et al., 2019). The presence of pleural thickening on chest computed tomography (CT) coupled with fever could indicate severe, organizing empyema (Stefani et al., 2013). Patients with empyema complicated by lung abscess have a higher risk of failure with surgical intervention and a higher rate of mortality (23% compared to 14%) (Huang et al., 2010). Notably, VATS was recently shown to be effective in treatment of stage III empyema in adult patients (Hajjar et al., 2016; Aljehani and Alabkary, 2018; Reichert et al., 2018a; Reichert et al., 2018b; Semenkovich et al., 2018), where thoracotomy with decortication of visceral pleura had previously dominated. Thus, the shift from a more invasive surgical approach for stage III empyema towards VATS becomes feasible. A trend towards less invasive procedures to treat stage II/III empyema in combination with the recently reported equal efficacy of VATS and IPFT (Ohara et al., 2018; Samancilar et al., 2018; Kermenli and Azar, 2021) opens a window of opportunity to expand the use of pharmacological treatment in advanced stage empyema.
IPFT is particularly effective in pediatric patients with empyema (de Benedictis et al., 2000; Thomson et al., 2002; Barbato et al., 2003; Barnes et al., 2005; Sonnappa et al., 2006; Sonnappa and Jaffe, 2007; Bianchini et al., 2010; Stefanutti et al., 2010; Abu-Daff et al., 2013; Israel and Blackmer, 2014; Nie et al., 2014; Coelho et al., 2016; James et al., 2017; Livingston et al., 2017). In sharp contrast to adults, retrospective studies of VATS in pediatric patients with empyema (Avansino et al., 2005; Chibuk et al., 2011; Cohen et al., 2012; Cohen et al., 2013), showed minimal mortality (n = 101) (Chen et al., 2009). While there is still a debate regarding whether VATS is more expensive than IPFT (Sonnappa et al., 2006; Cohen et al., 2008; St Peter et al., 2009) or not (Shah et al., 2010; Derderian et al., 2020), the latter is advocated for by some as the preferred initial treatment of empyema in pediatric patients (Livingston et al., 2016b; Derderian et al., 2020). IPFT in pediatric practice is remarkably effective but still empiric. IPFT with single chain tissue-type plasminogen activator (tPA, Alteplase) was more effective than saline irrigation (Hanson et al., 2015). Meta-analysis (Pacilli and Nataraja, 2018) and multiple studies have demonstrated equivalent efficacy between VATS and IPFT with urokinase (uPA) (Sonnappa et al., 2006; Marhuenda et al., 2014; Sonnappa, 2015; Pacilli and Nataraja, 2018) or tPA (St Peter et al., 2009; Livingston et al., 2016b) in treatment of empyema in pediatric patients. As a result, IPFT is recommended as the first line treatment for empyema in pediatrics (St Peter et al., 2009; Kobr et al., 2010; Stefanutti et al., 2010; Slaats et al., 2019; Baram and Yaldo, 2020; Livingston et al., 2020). Further studies of treatment with tPA (4 mg) alone or with DNase (Dornase; 5 mg) in empyema in pediatric patients (n = 97; sequential injection, 1 h apart) demonstrated that DNase does not improve the efficacy of tPA (Livingston et al., 2017; Livingston et al., 2020). While there are gaps in our understanding, the differences between empyema in adults and children, including a low mortality in the latter favors applying minimally invasive pharmacological treatment as the first option. Preclinical studies may further assist in closing this gap in our knowledge of age-dependent progression of empyema.
Multiple recent clinical trials have demonstrated the efficacy of IPFT in adult patients with empyema (Thommi et al., 2007; Rahman et al., 2011; Thommi et al., 2012; Popowicz et al., 2014a; Popowicz et al., 2014b; Nie et al., 2014; Piccolo et al., 2014; Piccolo et al., 2015; Barthwal et al., 2016; Majid et al., 2016; Mehta et al., 2016; Vial et al., 2016; Bishwakarma et al., 2017; Popowicz et al., 2017; Beckert et al., 2019; Godfrey et al., 2019). However, the strikingly higher mortality in adults does not allow for extrapolation of treatment strategies used in pediatrics and speaks to the imperative for preclinical studies focused on the mechanisms governing development and resolution of empyema in adults. Advanced stage empyema is considered a risk factor for failure of IPFT (Himelman and Callen, 1986); (Moulton et al., 1995; Temes et al., 1996). Patients that present with extensive loculation, lung abscess, and pleural thickening are more likely to fail IPFT (Davies et al., 1999; Thommi et al., 2007; Chen et al., 2016; Khemasuwan et al., 2018) and require surgical intervention (Davies et al., 1999; Maskell et al., 2005; Thommi et al., 2007). On the other hand, a randomized, double blinded trial demonstrated that Alteplase (25 mg/100 ml, 1 h clamp, suction after second hour, daily up to 3 days) was both more effective than placebo (95 and 12%, respectively) and safe (Thommi et al., 2012). While meta-analyses also have revealed the advantages of IPFT over placebo there is still controversy regarding what constitutes optimal therapy (Janda and Swiston, 2012). Thus, in contrast to pediatric practice, there is insufficient data to recommend routine use of IPFT in adults. Notably, a meta-analysis did not find a statistically significant difference in mortality between surgical and non-surgical management of pleural empyema, with VATS potentially associated with a shorter hospital stay (Kwon, 2014; Redden et al., 2017; Chong et al., 2021). High-risk subsets of adults with empyema, such as pregnant patients, often have markedly limited data available regarding the safety and efficacy of IPFT. Systemic fibrinolytics have been used in pregnant patients with life-threatening or potentially debilitating conditions such as ischemic stroke, myocardial infarction, and venous thrombotic events, with no apparent increase in complications from that observed in non-pregnant adults (Leonhardt et al., 2006). The few publications available concur that likewise, IPFT is effective and can be safely used in pregnancy, but should be used on a case-by-case basis given the lack of clinical trials and limited data regarding possible fetal complications (Torbic et al., 2017; Dikensoy et al., 2018; Amariei et al., 2019; Nasralla et al., 2021). Thus, we propose that preclinical studies with appropriate animal models can inform the limits of IPFT and enhance the design of clinical trials aimed to improve the treatment of advanced stage empyema in adults.
Over the past decade, cost effective (Asciak et al., 2019) IPFT with a combination of tPA and deoxyribonuclease (DNase) (Dornase), as demonstrated in the Multicenter Intrapleural Sepsis Trial 2 (MIST2) (Rahman et al., 2011), has been broadly embraced as IPFT for empyema (Abu-Daff et al., 2013; Popowicz et al., 2014a; Popowicz et al., 2014b; Israel and Blackmer, 2014; Piccolo et al., 2014; Piccolo et al., 2015; Majid et al., 2016; Bishwakarma et al., 2017; Majid et al., 2017; Popowicz et al., 2017; Innabi et al., 2018; Kheir et al., 2018; Fitzgerald et al., 2019; Fitzgerald et al., 2021). A recent expert consensus statement (Chaddha et al., 2021) described the level of severity of empyema that should be treated with tPA/DNase injected concurrently, appropriate dosing (10/5 mg, respectively), dwelling time, dose schedule (twice a day), and an algorithm for IPFT, thus re-establishing a universal “one for all” treatment approach to empyema based on the MIST2 protocol. On the other hand, there have been multiple calls for optimization of the MIST2 strategy (Chan et al., 2018) by adjusting the doses of tPA and DNase (Innabi et al., 2018; Hart et al., 2019), dosing schedule (Majid et al., 2016; Mehta et al., 2016; Majid et al., 2017), and the use of plasminogen activators other than tPA (Alemán et al., 2015; Beckert et al., 2019; Bédat et al., 2019), which may indicate growing concerns regarding MIST2 protocol-based IPFT among clinicians. Dose de-escalation studies in patients in Australia were designed to determine a “minimal effective dose” of the drug in humans, with the goal of decreasing side effects such as bleeding (Popowicz et al., 2017). Other approaches were assessed in an attempt to increase the safety and efficacy of the MIST2 (Rahman et al., 2011) protocol, including increasing the dose of DNase with a concurrent decrease in tPA (Innabi et al., 2018), increasing dosing intervals (once a day Alteplase/DNase for 3 days, n = 55; 92.7% success) (Mehta et al., 2016), the use of tPA alone (Majid et al., 2016), and the administration of Alteplase and DNase concurrently (Majid et al., 2017). A 5-year analysis of complex pleural fluid collections (n = 103; 82 empyema) treated with Alteplase alone (6 mg daily) demonstrated the high efficacy of this treatment (need for surgery 7.3%, mortality 17.1%) (Heimes et al., 2017). A second 5-year observational study reported 83% efficacy for Alteplase in complicated pleural effusions (CPE) and 62.7% in empyema (n = 88 and 14, respectively) (Barthwal et al., 2016). Alteplase alone in doses between 10 and 100 mg daily (n = 120) was successful in 85% of empyema cases (Thommi et al., 2007). However, even high doses of Alteplase often failed in advanced stage empyema (Thommi et al., 2007). These reports indicate uncertainty in the clinical field regarding the optimal treatment of empyema in adults, invalidating the current concept that one dose can treat everyone and supporting further studies on a personalized approach to pharmacological management of empyema in adults. Thus, preclinical studies with validated models of advanced stage empyema could answer several fundamental questions: 1) which stages of empyema can best be treated with IPFT, 2) what the relationship between the “minimal effective dose” and disease severity is, and 3) is there one “minimal effective dose” that can be used for all patients with empyema or should the dose and dosing schedule be personalized for each patient.
Bleeding and treatment failure necessitating surgical intervention are the two major adverse events that have been reported when Alteplase IPFT is used alone or in combination with DNase (Froudarakis et al., 2008; Rahman et al., 2011; Piccolo et al., 2014; Alemán et al., 2015; Majid et al., 2016; Mehta et al., 2016; Jiang et al., 2020). Notably, the frequency of bleeding complications differs between clinical centers that are experienced with the MIST2 protocol (0–5.4%) (Rahman et al., 2011; Piccolo et al., 2014; Majid et al., 2016; Mehta et al., 2016), and those still trying to adopt it (up to 12–16%) (Alemán et al., 2015; Jiang et al., 2020), likely indicating the importance of procedural experience rather than the effect of dose of tPA (Skeete et al., 2004; Thommi et al., 2007). In contrast to the MIST2 protocol, treating patients (n = 48) with urokinase (two-chain, 100,000 IU daily for up to 6 days) demonstrated similar efficacy (97%) without bleeding complications (Alemán et al., 2015). Interestingly, urokinase appears to be more effective than tPA in patients with non-purulent complicated parapneumonic pleural effusions (Nie et al., 2014). Additionally, treatment with urokinase is associated with a lower likelihood of subsequent surgery when compared to IPFT with either tPA or streptokinase (Nie et al., 2014). Finally, direct comparison between tPA/DNase and urokinase has shown equal efficacy, albeit with a lower incidence of hemothorax with the latter (Bédat et al., 2019). Recent comparisons of IPFT with placebo have found that, while IPFT decreased the need for surgical intervention and overall failure of pleural fluid drainage, treatment with Alteplase versus urokinase was associated with a higher risk of bleeding (Altmann et al., 2019). The authors highlight the importance of considering disease severity when comparing different studies (Altmann et al., 2019). These observations agree with the results of a recent phase 1 trial using single chain urokinase (scuPA) (Beckert et al., 2019). It is not clear why urokinase and Alteplase demonstrate different efficacy in empyema in adults, while the former is successful in pediatric practice. Therefore, translational studies may help to understand whether urokinase is superior to tPA alone (Idell et al., 2007; Komissarov et al., 2013; Komissarov et al., 2016) or in combination with DNase, or if dose and choice of plasminogen activator should be personalized. Thus, clinical trials can be best informed by preclinical testing to define approaches to selecting treatment strategies that would account for disease severity as well as overall patient health and comorbidities. Targeting the mechanisms of a serpin, plasminogen activator inhibitor 1 (PAI-1), with monoclonal antibodies (mAbs) or a small Docking Site Peptide (DSP) resulted in an up to eight fold decrease in the effective bolus dose of plasminogen activator in rabbit models of chemically induced pleural injury and acute streptococcal empyema (Florova et al., 2015; Florova et al., 2018; Florova et al., 2021) without an increase in bleeding. Thus, PAI-1-targeted fibrinolytic therapy (PAI-1-TFT) aiming to support effective intrapleural fibrinolysis with low doses of tPA or uPA could become a novel approach to increasing the efficacy of treatment and decreasing the risk of bleeding complications in patients with empyema. Further preclinical studies of novel adjuncts and adjunct combinations for PAI-1-TFT would result in a novel, low-dose, well-tolerated treatment tractable in clinical settings.
While patient mortality is negligible, the rate of IPFT failure in pediatric empyema remains around 15% (Sonnappa et al., 2006; St Peter et al., 2009; Livingston et al., 2016b; Long et al., 2016; Derderian et al., 2020). Defining reliable molecular predictors of treatment failure (Arnold et al., 2012020; Derderian et al., 2020; Khemasuwan et al., 2018; Livingston et al., 2016a) would therefore likely increase the efficacy of IPFT as a therapy for empyema in pediatrics. The striking difference in the efficacy of IPFT in adult and pediatric patients with empyema abrogates the possibility of applying successful pediatric treatments to adults. Moreover, biomarkers to identify patients in whom IPFT is likely to fail may not be equally successful in both populations. While clinicians acknowledge the importance of rapid diagnosis and treatment selection in advanced stage empyema, to the best of our knowledge there is currently no assay or procedure capable of predicting the likelihood of successful IPFT. Rapid diagnosis and staging of empyema and the choice of the most effective treatment could be critical for successful outcomes (Petrakis et al., 2010; Stefani et al., 2013; Reichert et al., 2017; Reichert and Bodner, 2018; Semenkovich et al., 2018). Prolonged delays between diagnosis and treatment may result in exacerbation of the empyema and necessitate more invasive treatment (Stefani et al., 2013).
IPFT activates endogenous plasminogen and is less effective in patients with plasminogen deficiency due to low expression, degradation, or imbalance in inhibitors. A hereditary or inflammation-induced plasminogen deficiency or overexpression of serpins (Mingers et al., 1999; Mehta and Shapiro, 2008; Martin-Fernandez et al., 2016; Prabhudesai et al., 2017; Saes et al., 2019; Tenbrock et al., 2019; Hangul et al., 2021) could affect the rate of fibrinolysis and thus outcomes of IPFT. Notably, while modern diagnostic approaches can evaluate the severity of empyema, they cannot assess the patient’s fibrinolytic system and determine whether IPFT could be a tractable approach. Unfortunately, neither Light’s Criteria (Light et al., 1972; Light, 1995; Light, 2013) nor current imaging diagnostic modalities–ultrasonography (Ramnath et al., 1998; Gates et al., 2004; Calder and Owens, 2009; Rahman et al., 2010) and chest CT (Kearney et al., 2000; Calder and Owens, 2009), can define optimal treatment and predict therapeutic outcomes (Kearney et al., 2000; Calder and Owens, 2009). Recent attempts to use artificial intelligence to predict the results of IPFT are still in an early stage (Khemasuwan et al., 2018; Ost, 2018; Khemasuwan et al., 2020). A RAPID score (Rahman et al., 2014) was developed to identify high-risk adults with empyema based on the degree of Renal impairment, Age exceeding 70 years, presence of Purulent pleural fluid, hospital-acquired Infection, and insufficient Diet (low albumin). Interestingly, patients with non-purulent pleural effusions had increased morbidity using this scoring system. This system can be used to stratify patient populations for comparability in clinical trial testing of different forms of IPFT or other interventions. A recent validation study demonstrated that the 3-months mortality increases from 2.3 to 29.3% with an increase in RAPID score from 0 to 2 to 5–7 (Corcoran et al., 2020). However, while high RAPID score is associated with higher 3-months mortality in adults (Corcoran et al., 2020; Touray et al., 1962018; White et al., 2015), it is not applicable for pediatric patients and does not identify patients that may be poor candidates for IPFT in any group. Identifying whether IPFT, VATS or other surgical options are the optimal treatment for a given patient remains challenging (Petrakis et al., 2004). Although a recently proposed algorithm focuses on surgical treatment for stage II and III empyema in adults (Reichert et al., 2017), the authors acknowledge a gap in our understanding regarding selection of optimal treatments and a lack of randomized, prospective clinical trials on catheter-directed IPFT versus minimally invasive surgery for such patients. Current biochemical tests and imaging techniques cannot assess the fibrinolytic system of a patient and inform on likely prognosis if they were offered IPFT or surgery, thus calling for the development of novel approaches to assist in treatment selection.
A novel biomarker, Fibrinolytic Potential (FP) was identified in preclinical studies and may address this issue (Florova et al., 2015; Komissarov et al., 2016; Idell et al., 2017; Florova et al., 2018; Beckert et al., 2019; Florova et al., 2021). Plasminogen accumulates in the pleural fluid of patients with empyema due to inhibited plasminogen activation. Consequently, fibrinolytic activity (FA0) in sampled pleural fluids increases dramatically with ex vivo supplementation with a plasminogen activator (FAPA), mimicking IPFT. Thus, FP, which is defined as the incremental change in fibrinolytic activity in pleural fluids at presentation induced by introducing an exogenous plasminogen activator (FAPA-FA0) accounts for much of the variability caused by individual parameters that affect fibrinolytic activity during IPFT, including levels of expression and degradation of plasminogen, other fibrinolytic proenzymes and enzymes, as well as inhibitors of fibrinolysis other than PAI-1. FP varies in humans by up to two orders of magnitude (Florova et al., 2015; Komissarov et al., 2016; Beckert et al., 2019). Low FP in pleural fluid—for example, due to low levels of expression or significant degradation of plasminogen, would predict IPFT failure in an individual (Beckert et al., 2019) and support surgery as the preferred intervention. FP is equally applicable to both adult and pediatric populations. Thus, the FP of pleural fluid sampled from a patient with empyema could become a prognostic biomarker to assist in informed decision making, with the value of FP associated with the probability of successful IPFT. Preclinical development and validation of a novel FP point-of-care test (POCT) that could differentiate between cohorts of patients that are candidates for IPFT (relatively high FP) and those in need of surgical intervention (low or undetectable FP), offers a paradigm shift in treatment of empyema. We posit that assessing FP can decrease the frequency of IPFT failure, morbidity and mortality, as well as overall cost of treatment for empyema, thereby becoming a critical element that contributes to decision making in personalized treatments. Confirmation awaits clinical trials, but the development of new diagnostics that can assess the suitability of a patient for IPFT is desirable and could potentially optimize therapy for individual patients with empyema.
Surgery, whether VATS or pleural decortication, in advanced stage empyema (Bouros et al., 2002; Petrakis et al., 2004; Petrakis et al., 2010; Di Napoli et al., 2014; Majeed et al., 2021) could be considered a universal treatment option. VATS is a less invasive surgical approach to treat empyema in both adult and pediatric patients (Chambers et al., 2010; Petrakis et al., 2010; Scarci et al., 2011). Notably, the success rate (60–100%) and mortality (0–13%) of VATS for the treatment of stage II/III empyema has not changed over the last 20 years (Cassina et al., 1999). While studies of different approaches to managing parapneumonic effusions published 20 years ago indicated that surgery is associated with the lowest mortality (2%), followed by IPFT and VATS (4.5 and 5%, respectively) (Colice et al., 2000), they did not mention a dramatic difference between candidates for surgery and patients that have contraindications. There was a significant difference in the age between cohorts of patients that were treated surgically and those who were not. Multiple studies demonstrate that the age of patients could be considered an integral factor associated with contraindications to surgery, which is also related to mortality rates. Retrospective analysis of 4,424 patients (1987–2004) has shown a 2.8% annual rise in the incidence of empyema, with the fraction of patients requiring surgery increasing from 42.4 to 58.4%. Thirty-day mortality was higher in patients that were not treated surgically (16.6 versus 5.4%), and patients treated surgically were on average 10 years younger and with a lower comorbidity index (Farjah et al., 2007). For example, the incidence of empyema in Denmark (1997–2011; n = 6,874 (Søgaard et al., 2014)) increased by 26% for all patients, and by 87.3% for those older than 80 years. In that same timeframe, the mortality was 1.2% in 15 to 39-year-old patients and 20.2% in those older than 80 years of age. Thus, advanced age and comorbidities remain prognostic factors, and empyema continues to be a serious concern. Recent analysis of records of patients with empyema from the New York Inpatient Database (2009–2014; n = 4,095) that underwent chest tube drainage (n = 1,563), VATS and drainage (n = 1,313), and open decortication and drainage (n = 1,219) (Semenkovich et al., 2018), revealed higher mortality and readmission rates in the first group, suggesting that some of these patients would benefit from surgical intervention. However, the chest tube only cohort was significantly older than patients that underwent surgery (average 64 versus 56 and 57 years old; p < 0.001). Moreover, these patients had significantly higher (p < 0.001) rates of serious comorbidities such as congestive heart failure, septicemia, shock, coagulopathy, chronic anemia, cancer, as well as issues with pulmonary circulation, cardiac valve defects, peripheral vascular disease, chronic pulmonary disease, and renal disease. Thus, poorer outcomes in the first group were expected. While surgery is recommended when treating advanced stage empyema, there are several issues that affect morbidity and mortality when using a surgical approach. First, not every patient is fit for even minimally invasive surgery and thus, they undergo “non-surgical drainage” of pleural fluid. An example of such management could be found in the retrospective analysis of the survival of patients that underwent surgical and non-surgical approaches. The median age of patients referred to thoracoscopic surgery (n = 417) was almost a decade younger than those provided with non-surgical drainage (n = 185), 55.9 and 65.3 years, respectively (p < 0.001). Also, the non-surgical group had higher rates of comorbidities such as malignancy, malnutrition, liver cirrhosis and culture-positive pleural fluid (p ≤ 0.013). These indices clearly reflect the difference between patients with empyema that can and cannot be managed surgically, and could contribute to the higher mortality observed in the latter group (24.9 versus 7.5%; p = 0.001) (Chen et al., 2014). For patients with both advanced age and comorbidities who may be unwilling to undergo surgical intervention due to increased risks or contraindications, chest tube drainage remains the only option. This cohort of patients would benefit the most from the development of novel, well-tolerated, less invasive treatment approaches.
A small (n = 8) study of non-surgically treated patients with advanced stage empyema and a non-expandable lung, that is likewise associated with failure of IPFT (Huggins et al., 2018), reported success with slow (average 73.6 days) chest tube drainage (Biswas et al., 2016). Use of VATS without intubation (Kermenli et al., 2021), irrigation with saline, which was shown more effective than drainage alone (Hooper et al., 2015), and the use of an “extended MIST2 protocol” (tPA 10mg/DNase 5mg, twice daily; >6 doses; n = 20) in patients unable to receive surgery (McClune et al., 20162016) were used to address this problem. Notably, the “extended MIST2 protocol” demonstrated an increase in bleeding events (10% compared to 2.5%), and resulted in an improved protocol that requires further evaluation (Gilbert and Gorden, 2017). These clinical studies strongly call for translational research guiding the development of effective, less invasive treatments for patients with advanced stage empyema, who are unable to receive VATS or thoracoscopic surgery. Patients that decline or are unfit for surgical intervention could potentially benefit from treatment with low dose PAI-1-TFT, which allows for a decrease in the dose of plasminogen activator by almost an order of magnitude (Florova et al., 2015; Florova et al., 2018; Florova et al., 2021) or treatment with innovative encapsulated plasminogen activators in combination with ultrasound, which is successfully used in circulation (Bader et al., 2015; Klegerman et al., 2016; Klegerman, 2017; Miao et al., 2018). Any improvement in non-surgical drainage by decreasing the dose of the plasminogen activator, increasing the rate of intrapleural fibrinolysis, or increasing the half-life of plasminogen activating and fibrinolytic activities could positively affect the survival of this patient population. Even small improvements in the current treatment of advanced stage empyema could result increased efficacy and decreased mortality in this patient cohort.
Successful transition from bedside to bench heavily relies on the availability of validated animal models that recapitulate the disease in humans. The absence of such a model could result in unexpected adverse events or even treatment failure in human patients. While adequate models are critical for the development and testing of innovative treatments, there is a dearth of animal models that closely recapitulate advanced stage empyema in humans, indicating a gap in translational science that restricts our understanding of intrapleural fibrinolysis in advanced stage empyema. While mouse models of infectious pleural injury (Hsieh et al., 2008; Wilkosz et al., 2011; Tucker et al., 2016) are good for mechanistic studies of empyema in mice (Rovina et al., 2013; Lapidot et al., 2015; Tucker et al., 2016; Boren et al., 2017; Kamata et al., 2017; Tucker et al., 2019) they often lack correlation with human disease (Kanellakis et al., 2019) and are limited, as mouse fibrinolytic enzymes and inhibitors are structurally different from humans. Furthermore, murine fibrin structure differs significantly from that in humans (Pretorius et al., 2007; Jo et al., 2009; Dewilde et al., 2010). Rabbit models more closely recapitulate pleural injury and intrapleural fibrinolysis in humans (Idell et al., 2009; Komissarov et al., 2016; Kunz et al., 2004; Light et al., 1782000; Sasse et al., 1999; Sasse et al., 1997; Teixeira et al., 2000) and were used to develop the treatments assessed in the MIST2 clinical trial (Dikensoy et al., 2006; Zhu et al., 2006), which resulted in the current Alteplase/DNase protocol (Rahman et al., 2011). Streptococcus pneumoniae-induced pleural injury in rabbits (Komissarov et al., 2016) closely recapitulates the time course and major features of empyema observed in humans, including advanced stage disease, where standard IPFT becomes less effective. Moreover, the similarity of rabbit fibrin structure and intrapleural fibrinolytic system to humans (Pretorius et al., 2007) allows for testing of therapeutics that are used in clinical practice or intended for future clinical trials. Multiple loculations of fluid in the pleural space and pleural thickening with increasing severity of injury seen in rabbits are similar to what is observed during stage II and III empyema (Davies et al., 1999; Khemasuwan et al., 2018) in patients. Empyema in this rabbit model transitions from an acute to an advanced stage if untreated, and there is a notable decrease in the efficacy of IPFT in advanced stage empyema, as has likewise been observed in humans (Himelman and Callen, 1986; Temes et al., 1996). The decrease in the rate of intrapleural fibrinolysis in advanced stage empyema noted by clinicians (Thommi et al., 2007; Thommi et al., 2012; Thommi et al., 2014) contributes to the failure of IPFT. Compensating by increasing the dose of tPA increases the risk of bleeding complications. Over the last decade, the major mechanisms of intrapleural fibrinolysis identified in rabbit models of chemical and infectious pleural injury were also observed in humans (Komissarov et al., 2009; Komissarov et al., 2011; Karandashova et al., 2013; Komissarov et al., 2013; Florova et al., 2015; Komissarov et al., 2016; Florova et al., 2018; Idell and Rahman, 2018; Komissarov et al., 2018; Beckert et al., 2019). This resulted in identification and validation of PAI-1 as a molecular target (Karandashova et al., 2013) and development of PAI-1-TFT, which allows for an up to 8-fold decrease in the dose of plasminogen activator (Florova et al., 2015; Florova et al., 2018; Florova et al., 2021), as well as FP, a novel biomarker for assessing the likelihood of failure of IPFT in an individual patient (Florova et al., 2015; Komissarov et al., 2016; Idell et al., 2017; Florova et al., 2018; Beckert et al., 2019; Florova et al., 2021).
The available literature indicates that at present, there is no one universal treatment for empyema in adult patients and opens new avenues for translational studies using validated animal models. The limits of IPFT could be determined using an appropriate animal model of chronic, advanced stage versus acute, early stage empyema with therapeutics such as human tPA, uPA, and DNase, which are already used in clinical practice. Newer options, such as low dose PAI-1-TFT or plasminogen activators encapsulated in liposomal carriers could likewise be tested in preclinical studies in order to identify and develop innovative treatments for empyema and expanding treatment to patients, who currently are not candidates for IPFT. Varying the plasminogen activator dose, dosing frequency and schedule, as well as exploring novel adjuncts that could increase efficacy, could inform the design of more personalized approaches to the treatment of advanced stage empyema in humans. We believe that it could be beneficial to use biomarker analyses of pleural fluid to develop POC tests that inform on which treatment is the most beneficial for a given patient. Simultaneously, low-dose, well-tolerated treatments for advanced stage empyema could expand the cohort of patients, including those that are of advanced age and with multiple comorbidities, that could benefit from IPFT. Preclinical development and testing of new methods of predicting patient prognosis after intervention and targeted treatments hold promise to improve outcomes in patients with organizing pleural injury with failed drainage and poor responses to currently available therapy.
SK and AAK conceived literature analysis and designed the manuscript. GF and SI revised the manuscript.
This work was supported by NIH grants R01HL130402 and R01HL152059.
AK and GF are supported by NIH and serve as co-investigators on research involving intellectual property licensed to Lung Therapeutics, Inc. (LTI) and have conflict of interest management plans at The University of Texas Health Science Center at Tyler (UTHSCT). SI is likewise supported by NIH and serves as a member of the Board of Directors, Founder and Chief Scientific Officer of LTI. SI has an equity position in the company, as does the University of Texas Horizon Fund and UTHSCT. He has a conflict of interest plan acknowledging and managing these declared conflicts of interest through UTHSC. He is an inventor on a patent USPTO # 7332469 held by the UT Board of Regents and licensed to LTI and served on the Safety Review Committee. SI also serves as a consultant for Monopar Therapeutics. AK, GF and SI are inventors on patent USPTO # 10, 175, 255 B2.
The remaining author declares that the research was conducted in the absence of any commercial or financial relationships that could be construed as a potential conflict of interest.
All claims expressed in this article are solely those of the authors and do not necessarily represent those of their affiliated organizations, or those of the publisher, the editors and the reviewers. Any product that may be evaluated in this article, or claim that may be made by its manufacturer, is not guaranteed or endorsed by the publisher.
Abu-Daff, S., Maziak, D. E., Alshehab, D., Threader, J., Ivanovic, J., Deslaurier, V., et al. (2013). Intrapleural Fibrinolytic Therapy (IPFT) in Loculated Pleural Effusions-Aanalysis of Predictors for Failure of Therapy and Bleeding: a Cohort Study. BMJ Open 3, e001887. doi:10.1136/bmjopen-2012-001887
Alemán, C., Porcel, J. M., Alegre, J., Ruiz, E., Bielsa, S., Andreu, J., et al. (2015). Intrapleural Fibrinolysis with Urokinase versus Alteplase in Complicated Parapneumonic Pleural Effusions and Empyemas: A Prospective Randomized Study. Lung 193, 993–1000. doi:10.1007/s00408-015-9807-6
Aljehani, Y., and Alabkary, S. (2018). Single Incision VATS Decortication for 3rd Stage Empyema. Clin. Case Rep. 6, 2144–2146. doi:10.1002/ccr3.1743
Altmann, E. S., Crossingham, I., Wilson, S., and Davies, H. R. (2019). Intra-pleural Fibrinolytic Therapy versus Placebo, or a Different Fibrinolytic Agent, in the Treatment of Adult Parapneumonic Effusions and Empyema. Cochrane Database Syst. Rev. 2019. doi:10.1002/14651858.cd002312.pub4
Amariei, D. E., Tran, B. C., Yao, R., Britt, E. M., Holden, V. K., Sachdeva, A., et al. (2019). Intrapleural Alteplase and Dornase in a Pregnant Woman with Complicated Parapneumonic Effusion. Ann. Thorac. Surg. 108, e293–e294. doi:10.1016/j.athoracsur.2019.03.023
Andrews, N. C. (1965). The Surgical Treatment of Chronic Empyema. Dis. Chest 47, 533–538. doi:10.1378/chest.47.5.533
Arnold, D. T., Hamilton, F. W., Elvers, K. T., Frankland, S. W., Zahan-Evans, N., Patole, S., et al. (2012). Pleural Fluid suPAR Levels Predict the Need for Invasive Management in Parapneumonic Effusions. Am. J. Respir. Crit. Care Med. 201, 1545–1553. doi:10.1164/rccm.201911-2169OC
Asciak, R., Hassan, M., Mercer, R. M., Hallifax, R. J., Wrightson, J. M., Psallidas, I., et al. (2019). Prospective Analysis of the Predictive Value of Sonographic Pleural Fluid Echogenicity for the Diagnosis of Exudative Effusion. Respiration 97, 451–456. doi:10.1159/000496153
Avansino, J. R., Goldman, B., Sawin, R. S., and Flum, D. R. (2005). Primary Operative versus Nonoperative Therapy for Pediatric Empyema: a Meta-Analysis. Pediatrics 115, 1652–1659. doi:10.1542/peds.2004-1405
Bader, K. B., Bouchoux, G., Peng, T., Klegerman, M. E., McPherson, D. D., and Holland, C. K. (2015). Thrombolytic Efficacy and Enzymatic Activity of rt-PA-loaded Echogenic Liposomes. J. Thromb. Thrombolysis 40, 144–155. doi:10.1007/s11239-015-1204-8
Baram, A., and Yaldo, F. (2020). Pediatric Thoracic Empyema-Outcomes of Intrapleural Thrombolytics: Ten Years of Experience. Glob. Pediatr. Health 7, 2333794X20928200. doi:10.1177/2333794X20928200
Barbato, A., Panizzolo, C., Monciotti, C., Marcucci, F., Stefanutti, G., and Gamba, P. G. (2003). Use of Urokinase in Childhood Pleural Empyema. Pediatr. Pulmonol 35, 50–55. doi:10.1002/ppul.10212
Barnes, N. P., Hull, J., and Thomson, A. H. (2005). Medical Management of Parapneumonic Pleural Disease. Pediatr. Pulmonol 39, 127–134. doi:10.1002/ppul.20127
Barthwal, M. S., Marwah, V., Chopra, M., Garg, Y., Tyagi, R., Kishore, K., et al. (2016). A Five-Year Study of Intrapleural Fibrinolytic Therapy in Loculated Pleural Collections. Indian J. Chest Dis. Allied Sci. 58, 17–20.
Beckert, L., Brockway, B., Simpson, G., Southcott, A. M., Lee, Y. C. G., Rahman, N., et al. (2019). Phase 1 Trial of Intrapleural LTI-01; Single Chain Urokinase in Complicated Parapneumonic Effusions or Empyema. JCI Insight 5, e127470. doi:10.1172/jci.insight.127470
Bédat, B., Plojoux, J., Noel, J., Morel, A., Worley, J., Triponez, F., et al. (2019). Comparison of Intrapleural Use of Urokinase and Tissue Plasminogen activator/DNAse in Pleural Infection. ERJ Open Res. 5, 00084. doi:10.1183/23120541.00084-2019
Bedawi, E. O., Hassan, M., and Rahman, N. M. (2018). Recent Developments in the Management of Pleural Infection: A Comprehensive Review. Clin. Respir. J. 12, 2309–2320. doi:10.1111/crj.12941
Ben-Or, S., Feins, R. H., Veeramachaneni, N. K., and Haithcock, B. E. (2011). Effectiveness and Risks Associated with Intrapleural Alteplase by Means of Tube Thoracostomy. Ann. Thorac. Surg. 91, 860–864. doi:10.1016/j.athoracsur.2010.10.082
Bender, J. M., Ampofo, K., Sheng, X., Pavia, A. T., Cannon-Albright, L., and Byington, C. L. (2009). Parapneumonic Empyema Deaths during Past century, Utah. Emerg. Infect. Dis. 15, 44–48. doi:10.3201/eid1501.080618
Bianchini, M. A., Ceccarelli, P. L., Repetto, P., Durante, V., Biondini, D., Bergamini, B., et al. (2010). Once-daily Intrapleural Urokinase Treatment of Complicated Parapneumonic Effusion in Pediatric Patients. Turk J. Pediatr. 52, 274–277.
Bishwakarma, R., Shah, S., Frank, L., Zhang, W., Sharma, G., and Nishi, S. P. (2017). Mixing it up: Coadministration of tPA/DNase in Complicated Parapneumonic Pleural Effusions and Empyema. J. Bronchology Interv. Pulmonol 24, 40–47. doi:10.1097/LBR.0000000000000334
Biswas, A., Jantz, M. A., Penley, A. M., and Mehta, H. J. (2016). Management of Chronic Empyema with Unexpandable Lung in Poor Surgical Risk Patients Using an Empyema Tube. Lung India 33, 267–271. doi:10.4103/0970-2113.180802
Boren, J., Shryock, G., Fergis, A., Jeffers, A., Owens, S., Qin, W., et al. (2017). Inhibition of Glycogen Synthase Kinase 3β Blocks Mesomesenchymal Transition and Attenuates Streptococcus Pneumonia-Mediated Pleural Injury in Mice. Am. J. Pathol. 187, 2461–2472. doi:10.1016/j.ajpath.2017.07.007
Bouros, D., Antoniou, K. M., Chalkiadakis, G., Drositis, J., Petrakis, I., and Siafakas, N. (2002). The Role of Video-Assisted Thoracoscopic Surgery in the Treatment of Parapneumonic Empyema after the Failure of Fibrinolytics. Surg. Endosc. 16, 151–154. doi:10.1007/s00464-001-9028-3
Burgos, J., Falcó, V., and Pahissa, A. (2013). The Increasing Incidence of Empyema. Curr. Opin. Pulm. Med. 19, 350–356. doi:10.1097/MCP.0b013e3283606ab5
Burgos, J., Lujan, M., Falcó, V., Sánchez, A., Puig, M., Borrego, A., et al. (2011). The Spectrum of Pneumococcal Empyema in Adults in the Early 21st century. Clin. Infect. Dis. 53, 254–261. doi:10.1093/cid/cir354
Calder, A., and Owens, C. M. (2009). Imaging of Parapneumonic Pleural Effusions and Empyema in Children. Pediatr. Radiol. 39, 527–537. doi:10.1007/s00247-008-1133-1
Cassina, P. C., Hauser, M., Hillejan, L., Greschuchna, D., and Stamatis, G. (1999). Video-assisted Thoracoscopy in the Treatment of Pleural Empyema: Stage-Based Management and Outcome. J. Thorac. Cardiovasc. Surg. 117, 234–238. doi:10.1016/S0022-5223(99)70417-4
Chaddha, U., Agrawal, A., Feller-Kopman, D., Kaul, V., Shojaee, S., Maldonado, F., et al. (2021). Use of Fibrinolytics and Deoxyribonuclease in Adult Patients with Pleural Empyema: a Consensus Statement. Lancet Respir. Med. 9 (9), 1050–1064. doi:10.1016/s2213-2600(20)30533-6
Chambers, A., Routledge, T., Dunning, J., and Scarci, M. (2010). Is Video-Assisted Thoracoscopic Surgical Decortication superior to Open Surgery in the Management of Adults with Primary Empyema? Interact Cardiovasc. Thorac. Surg. 11, 171–177. doi:10.1510/icvts.2010.240408
Chan, K. P., Fitzgerald, D. B., and Lee, Y. C. G. (2018). Emerging Concepts in Pleural Infection. Curr. Opin. Pulm. Med. 24, 367–373. doi:10.1097/MCP.0000000000000487
Chapman, S. J., and Davies, R. J. (2004). Recent Advances in Parapneumonic Effusion and Empyema. Curr. Opin. Pulm. Med. 10, 299–304. doi:10.1097/01.mcp.0000129755.79467.67
Chen, J. S., Huang, K. C., Chen, Y. C., Hsu, H. H., Kuo, S. W., Huang, P. M., et al. (2009). Pediatric Empyema: Outcome Analysis of Thoracoscopic Management. J. Thorac. Cardiovasc. Surg. 137, 1195–1199. doi:10.1016/j.jtcvs.2008.10.031
Chen, K. C., Chen, H. Y., Lin, J. W., Tseng, Y. T., Kuo, S. W., Huang, P. M., et al. (2014). Acute Thoracic Empyema: Clinical Characteristics and Outcome Analysis of Video-Assisted Thoracoscopic Surgery. J. Formos. Med. Assoc. 113, 210–218. doi:10.1016/j.jfma.2013.12.010
Chen, N. Y., Bender, J. M., Bard, J. D., Trost, M. J., and Corden, M. H. (2016). Something Doesn't Smell Right: When a Patient with Empyema Isn't Responding to Guideline-Based Management. Hosp. Pediatr. 6, 702–706. doi:10.1542/hpeds.2015-0274
Chibuk, T., Cohen, E., Robinson, J., Mahant, S., and Hartfield, D. (2011). Paediatric Complicated Pneumonia: Diagnosis and Management of Empyema. Paediatr. Child. Health 16, 425–429. doi:10.1093/pch/16.7.428
Chong, W. H., Hu, K., Saha, B. K., and Chopra, A. (2021). Comparing the Outcomes of Intrapleural Fibrinolytic and DNase Therapy versus Intrapleural Fibrinolytic or DNase Therapy: A Systematic Review and Meta-Analysis. Pulm. Pharmacol. Ther. 71, 102081. doi:10.1016/j.pupt.2021.102081
Coelho, A., Coelho, M., Pereira, J., Lavrador, V., Morais, L., and Carvalho, F. (2016). Fibrinolytics in the Treatment of Complicated Pleural Effusions. Acta Med. Port 29, 711–715. doi:10.20344/amp.7178
Cohen, E., Mahant, S., Dell, S. D., Traubici, J., Ragone, A., Wadhwa, A., et al. (2012). The Long-Term Outcomes of Pediatric Pleural Empyema: a Prospective Study. Arch. Pediatr. Adolesc. Med. 166, 999–1004. doi:10.1001/archpediatrics.2012.1055
Cohen, E., Mahant, S., and Weinstein, M. (2013). Empyema Inchildren. J. Pediatr. Surg. 48, 899. doi:10.1016/j.jpedsurg.2013.01.051
Cohen, E., Weinstein, M., and Fisman, D. N. (2008). Cost-effectiveness of Competing Strategies for the Treatment of Pediatric Empyema. Pediatrics 121, e1250–7. doi:10.1542/peds.2007-1886
Colice, G. L., Curtis, A., Deslauriers, J., Heffner, J., Light, R., Littenberg, B., et al. (2000). Medical and Surgical Treatment of Parapneumonic Effusions. Chest 118, 1158–1171. doi:10.1378/chest.118.4.1158
Corcoran, J. P., Psallidas, I., Gerry, S., Piccolo, F., Koegelenberg, C. F., Saba, T., et al. (2020). Prospective Validation of the RAPID Clinical Risk Prediction Score in Adult Patients with Pleural Infection: the PILOT Study. Eur. Respir. J. 56, 2020. doi:10.1183/13993003.00130-2020
Davies, C. W., Kearney, S. E., Gleeson, F. V., and Davies, R. J. (1999). Predictors of Outcome and Long-Term Survival in Patients with Pleural Infection. Am. J. Respir. Crit. Care Med. 160, 1682–1687. doi:10.1164/ajrccm.160.5.9903002
de Benedictis, F. M., De Giorgi, G., Niccoli, A., Troiani, S., Rizzo, F., and Lemmi, A. (2000). Treatment of Complicated Pleural Effusion with Intracavitary Urokinase in Children. Pediatr. Pulmonol 29, 438–442. doi:10.1002/(sici)1099-0496(200006)29:6<438:aid-ppul5>3.0.co;2-n
Derderian, S. C., Meier, M., Partrick, D. A., Demasellis, G., Reiter, P. D., Annam, A., et al. (2020). Pediatric Empyemas - Has the Pendulum Swung Too Far? J. Pediatr. Surg. 55, 2356–2361. doi:10.1016/j.jpedsurg.2019.12.017
Dewilde, M., Van De, C. B., Compernolle, G., Madsen, J. B., Strelkov, S., Gils, A., et al. (2010). Subtle Structural Differences between Human and Mouse PAI-1 Reveal the Basis for Biochemical Differences. J. Struct. Biol. 171 (1), 95–101. doi:10.1016/j.jsb.2010.03.006
Di Napoli, G., Ronzini, M., and Paradies, G. (2014). VATS: First Step in the Parapneumonic Empyema**. G Chir 35, 146–148. doi:10.11138/gchir/2014.35.5.146
Dikensoy, E., Dikensoy, Ö., and Light, R. W. (2018). Management of Parapneumonic Effusion in Pregnant Women. Tuberk Toraks 66, 64–67. doi:10.5578/tt.66557
Dikensoy, O., Zhu, Z., Na, M. J., Liao, H., Donnelly, E., and Light, R. W. (2006). Intrapleural Heparin or Heparin Combined with Human Recombinant DNase Is Not Effective in the Treatment of Empyema in a Rabbit Model. Respirology 11, 755–760. doi:10.1111/j.1440-1843.2006.00934.x
Farjah, F., Symons, R. G., Krishnadasan, B., Wood, D. E., and Flum, D. R. (2007). Management of Pleural Space Infections: a Population-Based Analysis. J. Thorac. Cardiovasc. Surg. 133, 346–351. doi:10.1016/j.jtcvs.2006.09.038
Finley, C., Clifton, J., Fitzgerald, J. M., and Yee, J. (2008). Empyema: an Increasing Concern in Canada. Can. Respir. J. 15, 85–89. doi:10.1155/2008/975312
Fitzgerald, D. B., Leong, S. L., Budgeon, C. A., Murray, K., Rosenstengal, A., Smith, N. A., et al. (2019). Relationship of Pleural Fluid pH and Glucose: a Multi-centre Study of 2,971 Cases. J. Thorac. Dis. 11, 123–130. doi:10.21037/jtd.2018.12.101
Fitzgerald, D. B., Muruganandan, S., Tsim, S., Ip, H., Asciak, R., Walker, S., et al. (2021). Intrapleural Fibrinolytics and Deoxyribonuclease for Treatment of Indwelling Pleural Catheter-Related Pleural Infection: A Multi-Center Observational Study. Respiration 100, 452–460. doi:10.1159/000514643
Florova, G., Azghani, A., Karandashova, S., Schaefer, C., Koenig, K., Stewart-Evans, K., et al. (2015). Targeting of Plasminogen Activator Inhibitor 1 Improves Fibrinolytic Therapy for Tetracycline-Induced Pleural Injury in Rabbits. Am. J. Respir. Cel Mol Biol 52, 429–437. doi:10.1165/rcmb.2014-0168OC
Florova, G., Azghani, A. O., Karandashova, S., Schaefer, C., Yarovoi, S. V., Declerck, P. J., et al. (2018). Targeting Plasminogen Activator Inhibitor-1 in Tetracycline-Induced Pleural Injury in Rabbits. Am. J. Physiol. Lung Cel Mol Physiol 314, L54–L68. doi:10.1152/ajplung.00579.2016
Florova, G., Girard, R. A., Azghani, A. O., Sarva, K., Buchanan, A., Karandashova, S., et al. (2021). Precision Targeting of the Plasminogen Activator Inhibitor-1 Mechanism Increases Efficacy of Fibrinolytic Therapy in Empyema. Physiol. Rep. 9, e14861. doi:10.14814/phy2.14861
Franklin, J., Talwar, A., Addala, D., Helm, E. J., Benamore, R., Rahman, N. M., et al. (2021). CT Appearances of Pleural Infection: Analysis of the Second Multi-centre Intra-pleural Sepsis Trial (MIST 2) Cohort. Clin. Radiol. 76, 436–442. doi:10.1016/j.crad.2020.12.017
Froudarakis, M. E., Kouliatsis, G., Steiropoulos, P., Anevlavis, S., Pataka, A., Popidou, M., et al. (2008). Recombinant Tissue Plasminogen Activator in the Treatment of Pleural Infections in Adults. Respir. Med. 102, 1694–1700. doi:10.1016/j.rmed.2008.08.012
Gates, R. L., Caniano, D. A., Hayes, J. R., and Arca, M. J. (2004). Does VATS Provide Optimal Treatment of Empyema in Children? A Systematic Review. J. Pediatr. Surg. 39, 381–386. doi:10.1016/j.jpedsurg.2003.11.045
Gilbert, C. R., and Gorden, J. A. (2017). Use of Intrapleural Tissue Plasminogen Activator and Deoxyribonuclease in Pleural Space Infections: an Update on Alternative Regimens. Curr. Opin. Pulm. Med. 23, 371–375. doi:10.1097/MCP.0000000000000387
Godfrey, M. S., Bramley, K. T., and Detterbeck, F. (2019). Medical and Surgical Management of Empyema. Semin. Respir. Crit. Care Med. 40, 361–374. doi:10.1055/s-0039-1694699
Grijalva, C. G., Zhu, Y., Nuorti, J. P., and Griffin, M. R. (2011). Emergence of Parapneumonic Empyema in the USA. Thorax 66, 663–668. doi:10.1136/thx.2010.156406
Hajjar, W. M., Ahmed, I., Al-Nassar, S. A., Alsultan, R. K., Alwgait, W. A., Alkhalaf, H. H., et al. (2016). Video-assisted Thoracoscopic Decortication for the Management of Late Stage Pleural Empyema, Is it Feasible? Ann. Thorac. Med. 11, 71–78. doi:10.4103/1817-1737.165293
Hangul, M., Tuzuner, A. B., Somekh, I., Klein, C., Patiroglu, T., Unal, E., et al. (2021). Type 1 Plasminogen Deficiency with Pulmonary Involvement: Novel Treatment and Novel Mutation. J. Pediatr. Hematol. Oncol. 43, e558–e560. doi:10.1097/MPH.0000000000001951
Hanson, S. J., Havens, P. L., Simpson, P. M., Nugent, M. L., and Wells, R. G. (2015). Intrapleural Alteplase Decreases Parapneumonic Effusion Volume in Children More Than saline Irrigation. Pediatr. Pulmonol 50, 1328–1335. doi:10.1002/ppul.23184
Hart, J. A., Badiei, A., and Lee, Y. C. G. (2019). Successful Management of Pleural Infection with Very Low Dose Intrapleural Tissue Plasminogen Activator/deoxyribonuclease Regime. Respirol Case Rep. 7, e00408. doi:10.1002/rcr2.408
Heimes, J., Copeland, H., Lulla, A., Duldulao, M., Bahjri, K., Zaheer, S., et al. (2017). The Use of Thrombolytics in the Management of Complex Pleural Fluid Collections. J. Thorac. Dis. 9, 1310–1316. doi:10.21037/jtd.2017.04.56
Himelman, R. B., and Callen, P. W. (1986). The Prognostic Value of Loculations in Parapneumonic Pleural Effusions. Chest 90, 852–856. doi:10.1378/chest.90.6.852
Hooper, C. E., Edey, A. J., Wallis, A., Clive, A. O., Morley, A., White, P., et al. (2015). Pleural Irrigation Trial (PIT): a Randomised Controlled Trial of Pleural Irrigation with normal saline versus Standard Care in Patients with Pleural Infection. Eur. Respir. J. 46, 456–463. doi:10.1183/09031936.00147214
Hsieh, Y. C., Tsao, P. N., Chen, C. L., Lin, T. L., Lee, W. S., Shao, P. L., et al. (2008). Establishment of a Young Mouse Model and Identification of an Allelic Variation of zmpB in Complicated Pneumonia Caused by Streptococcus Pneumoniae. Crit. Care Med. 36, 1248–1255. doi:10.1097/CCM.0b013e318169f0c3
Huang, H. C., Chen, H. C., Fang, H. Y., Lin, Y. C., Wu, C. Y., and Cheng, C. Y. (2010). Lung Abscess Predicts the Surgical Outcome in Patients with Pleural Empyema. J. Cardiothorac. Surg. 5, 88. doi:10.1186/1749-8090-5-88
Huggins, J. T., Maldonado, F., Chopra, A., Rahman, N., and Light, R. (2018). Unexpandable Lung from Pleural Disease. Respirology 23, 160–167. doi:10.1111/resp.13199
Idell, S., Azghani, A., Chen, S., Koenig, K., Mazar, A., Kodandapani, L., et al. (2007). Intrapleural Low-Molecular-Weight Urokinase or Tissue Plasminogen Activator versus Single-Chain Urokinase in Tetracycline-Induced Pleural Loculation in Rabbits. Exp. Lung Res. 33, 419–440. doi:10.1080/01902140701703333
Idell, S., Florova, G., Shetty, S., Tucker, T., Idell, R., Koenig, K., et al. (2017). Precision-guided, Personalized Intrapleural Fibrinolytic Therapy for Empyema and Complicated Parapneumonic Pleural Effusions: The Case for the Fibrinolytic Potential. Clin. Pulm. Med. 24, 163–169. doi:10.1097/CPM.0000000000000216
Idell, S., Jun Na, M., Liao, H., Gazar, A. E., Drake, W., Lane, K. B., et al. (2009). Single-chain Urokinase in Empyema Induced by Pasturella Multocida. Exp. Lung Res. 35, 665–681. doi:10.3109/01902140902833277
Idell, S., and Rahman, N. M. (2018). Intrapleural Fibrinolytic Therapy for Empyema and Pleural Loculation: Knowns and Unknowns. Ann. Am. Thorac. Soc. 15, 515–517. doi:10.1513/AnnalsATS.201711-848PS
Innabi, A., Surana, A., Alzghoul, B., and Meena, N. (2018). Rethinking the Doses of Tissue Plasminogen Activator and Deoxyribonuclease Administrated Concurrently for Intrapleural Therapy for Complicated Pleural Effusion and Empyema. Cureus 10, e2214. doi:10.7759/cureus.2214
Israel, E. N., and Blackmer, A. B. (2014). Tissue Plasminogen Activator for the Treatment of Parapneumonic Effusions in Pediatric Patients. Pharmacotherapy 34, 521–532. doi:10.1002/phar.1392
James, C. A., Braswell, L. E., Pezeshkmehr, A. H., Roberson, P. K., Parks, J. A., and Moore, M. B. (2017). Stratifying Fibrinolytic Dosing in Pediatric Parapneumonic Effusion Based on Ultrasound Grade Correlation. Pediatr. Radiol. 47, 89–95. doi:10.1007/s00247-016-3711-y
Janda, S., and Swiston, J. (2012). Intrapleural Fibrinolytic Therapy for Treatment of Adult Parapneumonic Effusions and Empyemas: a Systematic Review and Meta-Analysis. Chest 142, 401–411. doi:10.1378/chest.11-3071
Jiang, C., Xie, M., Cervellione, K., and Thurm, C. (2020). Clinical Efficacy and Bleeding Outcomes of Tissue Plasminogen Activator and Dornase Alfa in Pleural Space Infection with once Daily Concurrent Administration: a Retrospective Cohort Study. BMC Res. Notes 13, 368. doi:10.1186/s13104-020-05210-2
Jo, M., Takimoto, S., Montel, V., and Gonias, S. L. (2009). The Urokinase Receptor Promotes Cancer Metastasis Independently of Urokinase-type Plasminogen Activator in Mice. Am. J. Pathol. 175, 190–200. doi:10.2353/ajpath.2009.081053
Kamata, H., Tsukasaki, Y., Sakai, T., Ikebe, R., Wang, J., Jeffers, A., et al. (2017). KIF5A Transports Collagen Vesicles of Myofibroblasts during Pleural Fibrosis. Sci. Rep. 7, 4556. doi:10.1038/s41598-017-04437-7
Kanellakis, N. I., Wrightson, J. M., Hallifax, R., Bedawi, E. O., Mercer, R., Hassan, M., et al. (2019). Biological Effect of Tissue Plasminogen Activator (t-PA) and DNase Intrapleural Delivery in Pleural Infection Patients. BMJ Open Respir. Res. 6, e000440. doi:10.1136/bmjresp-2019-000440
Karandashova, S., Florova, G., Azghani, A. O., Komissarov, A. A., Koenig, K., Tucker, T. A., et al. (2013). Intrapleural Adenoviral Delivery of Human Plasminogen Activator Inhibitor-1 Exacerbates Tetracycline-Induced Pleural Injury in Rabbits. Am. J. Respir. Cel Mol Biol 48, 44–52. doi:10.1165/rcmb.2012-0183OC
Kearney, S. E., Davies, C. W., Davies, R. J., and Gleeson, F. V. (2000). Computed Tomography and Ultrasound in Parapneumonic Effusions and Empyema. Clin. Radiol. 55, 542–547. doi:10.1053/crad.1999.0480
Kermenli, T., and Azar, C. (2021). Can Intrapleural Alteplase Treatment Be an Alternative to Videothoracoscopic Deloculation and Decortication in Pleural Empyema? Wideochir Inne Tech. Maloinwazyjne 16, 580–586. doi:10.5114/wiitm.2021.103920
Kermenli, T., Azar, C., and Gundogdu, Z. (2021). First Experiences in Non-intubated, Video-Assisted Thoracoscopic Surgery: a Single-centre Study. Kardiochir Torakochirurgia Pol. 18, 15–22. doi:10.5114/kitp.2021.105181
Kheir, F., Cheng, G., Rivera, E., Folch, A., Folch, E., Fernandez-Bussy, S., et al. (2018). Concurrent versus Sequential Intrapleural Instillation of Tissue Plasminogen Activator and Deoxyribonuclease for Pleural Infection. J. Bronchology Interv. Pulmonol 25, 125–131. doi:10.1097/LBR.0000000000000461
Khemasuwan, D., Sorensen, J., and Griffin, D. C. (2018). Predictive Variables for Failure in Administration of Intrapleural Tissue Plasminogen Activator/Deoxyribonuclease in Patients with Complicated Parapneumonic Effusions/Empyema. Chest 154, 550–556. doi:10.1016/j.chest.2018.01.037
Khemasuwan, D., Sorensen, J. S., and Colt, H. G. (2020). Artificial Intelligence in Pulmonary Medicine: Computer Vision, Predictive Model and COVID-19. Eur. Respir. Rev. 29, 2020. doi:10.1183/16000617.0181-2020
Klegerman, M. E., Naji, A. K., Haworth, K. J., Zou, Y., Golunski, E., Peng, T., et al. (2016). Ultrasound-enhanced Bevacizumab Release from Echogenic Liposomes for Inhibition of Atheroma Progression. J. Liposome Res. 26, 47–56. doi:10.3109/08982104.2015.1029494
Klegerman, M. E. (2017). Translational Initiatives in Thrombolytic Therapy. Front. Med. 11, 1–19. doi:10.1007/s11684-017-0497-8
Kobr, J., Pizingerova, K., Sasek, L., Fremuth, J., Siala, K., and Racek, J. (2010). Treatment of Encapsulated Pleural Effusions in Children: a Prospective Trial. Pediatr. Int. 52, 453–458. doi:10.1111/j.1442-200X.2009.03006.x
Koegelenberg, C. F., Diacon, A. H., and Bolliger, C. T. (2008). Parapneumonic Pleural Effusion and Empyema. Respiration 75, 241–250. doi:10.1159/000117172
Komissarov, A. A., Florova, G., Azghani, A., Karandashova, S., Kurdowska, A. K., and Idell, S. (2013). Active α-macroglobulin Is a Reservoir for Urokinase after Fibrinolytic Therapy in Rabbits with Tetracycline-Induced Pleural Injury and in Human Pleural Fluids. Am. J. Physiol. Lung Cel Mol Physiol 305, L682–L692. doi:10.1152/ajplung.00102.2013
Komissarov, A. A., Florova, G., Azghani, A. O., Buchanan, A., Boren, J., Allen, T., et al. (2016). Dose Dependency of Outcomes of Intrapleural Fibrinolytic Therapy in New Rabbit Empyema Models. Am. J. Physiol. Lung Cel Mol Physiol 311, L389–L399. doi:10.1152/ajplung.00171.2016
Komissarov, A. A., Florova, G., and Idell, S. (2011). Effects of Extracellular DNA on Plasminogen Activation and Fibrinolysis. J. Biol. Chem. 286, 41949–41962. doi:10.1074/jbc.M111.301218
Komissarov, A. A., Mazar, A. P., Koenig, K., Kurdowska, A. K., and Idell, S. (2009). Regulation of Intrapleural Fibrinolysis by Urokinase-Alpha-Macroglobulin Complexes in Tetracycline-Induced Pleural Injury in Rabbits. Am. J. Physiol. Lung Cel Mol Physiol 297, L568–L577. doi:10.1152/ajplung.00066.2009
Komissarov, A. A., Rahman, N., Lee, Y. C. G., Florova, G., Shetty, S., Idell, R., et al. (2018). Fibrin Turnover and Pleural Organization: Bench to Bedside. Am. J. Physiol. Lung Cel Mol Physiol 314, L757–L768. doi:10.1152/ajplung.00501.2017
Kunz, C. R., Jadus, M. R., Kukes, G. D., Kramer, F., Nguyen, V. N., and Sasse, S. A. (2004). Intrapleural Injection of Transforming Growth Factor-Beta Antibody Inhibits Pleural Fibrosis in Empyema. Chest 126, 1636–1644. doi:10.1378/chest.126.5.1636
Kwon, Y. S. (2014). Pleural Infection and Empyema. Tuberc. Respir. Dis. (Seoul) 76, 160–162. doi:10.4046/trd.2014.76.4.160
Lapidot, M., Barash, U., Zohar, Y., Geffen, Y., Naroditsky, I., Ilan, N., et al. (2015). Involvement of Heparanase in Empyema: Implication for Novel Therapeutic Approaches. J. Clin. Cel Immunol 6. doi:10.4172/2155-9899.1000290
Leonhardt, G., Gaul, C., Nietsch, H. H., Buerke, M., and Schleussner, E. (2006). Thrombolytic Therapy in Pregnancy. J. Thromb. Thrombolysis 21, 271–276. doi:10.1007/s11239-006-5709-z
Light, R. W. (1995). A New Classification of Parapneumonic Effusions and Empyema. Chest 108, 299–301. doi:10.1378/chest.108.2.299
Light, R. W., MacGregor, M. I., Luchsinger, P. C., and Ball, W. C. (1972). Pleural Effusions: the Diagnostic Separation of Transudates and Exudates. Ann. Intern. Med. 77, 507–513. doi:10.7326/0003-4819-77-4-507
Light, R. W., Nguyen, T., Mulligan, M. E., and Sasse, S. A. (1782). The In Vitro Efficacy of Varidase versus Streptokinase or Urokinase for Liquefying Thick Purulent Exudative Material from Loculated Empyema. Lung 178, 13–18. doi:10.1007/s004080000002
Light, R. W. (2011). Pleural Controversy: Optimal Chest Tube Size for Drainage. Respirology 16, 244–248. doi:10.1111/j.1440-1843.2010.01913.x
Light, R. W. (2013). The Light Criteria: the Beginning and Why They Are Useful 40 Years Later. Clin. Chest Med. 34, 21–26. doi:10.1016/j.ccm.2012.11.006
Livingston, M. H., Cohen, E., Giglia, L., Pirrello, D., Mistry, N., Mahant, S., et al. (2016). Are Some Children with Empyema at Risk for Treatment Failure with Fibrinolytics? A Multicenter Cohort Study. J. Pediatr. Surg. 51, 832–837. doi:10.1016/j.jpedsurg.2016.02.032
Livingston, M. H., Colozza, S., Vogt, K. N., Merritt, N., and Bütter, A. (2016). Making the Transition from Video-Assisted Thoracoscopic Surgery to Chest Tube with Fibrinolytics for Empyema in Children: Any Change in Outcomes? Can. J. Surg. 59, 167–171. doi:10.1503/cjs.014714
Livingston, M. H., Mahant, S., Connolly, B., Maclusky, I., Laberge, S., Giglia, L., et al. (2020). Effectiveness of Intrapleural Tissue Plasminogen Activator and Dornase Alfa vs Tissue Plasminogen Activator Alone in Children with Pleural Empyema: A Randomized Clinical Trial. JAMA Pediatr.
Livingston, M. H., Mahant, S., Ratjen, F., Connolly, B. L., Thorpe, K., Mamdani, M., et al. (2017). Intrapleural Dornase and Tissue Plasminogen Activator in Pediatric Empyema (DTPA): a Study Protocol for a Randomized Controlled Trial. Trials 18293, 293. doi:10.1186/s13063-017-2026-0
Long, A. M., Smith-Williams, J., Mayell, S., Couriel, J., Jones, M. O., and Losty, P. D. (2016). 'Less May Be Best'-Pediatric Parapneumonic Effusion and Empyema Management: Lessons from a UK center. J. Pediatr. Surg. 51, 588–591. doi:10.1016/j.jpedsurg.2015.07.022
Majeed, F. A., Chatha, S. S., Zafar, U., Ali, A., Farhan, N., and Raza, A. (2021). VATS Thoracoscopic Decortication for Empyema Thoracic: A Retrospective Experience and Analysis of 162 Cases. J. Pak Med. Assoc. 71, 502–504. doi:10.47391/JPMA.431
Majid, A., Kheir, F., Folch, A., Fernandez-Bussy, S., Chatterji, S., Maskey, A., et al. (2016). Concurrent Intrapleural Instillation of Tissue Plasminogen Activator and DNase for Pleural Infection. A Single-Center Experience. Ann. Am. Thorac. Soc. 13, 1512–1518. doi:10.1513/AnnalsATS.201602-127OC
Majid, A., Ochoa, S., Chatterji, S., Fernandez-Bussy, S., Kheir, F., Rivera, E., et al. (2017). Safety and Efficacy of Tissue Plasminogen Activator and DNase for Complicated Pleural Effusions Secondary to Abdominal Pathology. Ann. Am. Thorac. Soc. 14, 342–346. doi:10.1513/AnnalsATS.201608-594BC
Marhuenda, C., Barceló, C., Fuentes, I., Guillén, G., Cano, I., López, M., et al. (2014). Urokinase versus VATS for Treatment of Empyema: a Randomized Multicenter Clinical Trial. Pediatrics 134, e1301–7. doi:10.1542/peds.2013-3935
Martin-Fernandez, L., Marco, P., Corrales, I., Pérez, R., Ramírez, L., López, S., et al. (2016). The Unravelling of the Genetic Architecture of Plasminogen Deficiency and its Relation to Thrombotic Disease. Sci. Rep. 6, 39255. doi:10.1038/srep39255
Maskell, N. A., Batt, S., Hedley, E. L., Davies, C. W., Gillespie, S. H., and Davies, R. J. (2006). The Bacteriology of Pleural Infection by Genetic and Standard Methods and its Mortality Significance. Am. J. Respir. Crit. Care Med. 174, 817–823. doi:10.1164/rccm.200601-074OC
Maskell, N. A., Davies, C. W., Nunn, A. J., Hedley, E. L., Gleeson, F. V., Miller, R., et al. (2005). U.K. Controlled Trial of Intrapleural Streptokinase for Pleural Infection. N. Engl. J. Med. 352, 865–874. doi:10.1056/NEJMoa042473
McClune, J. R., Wilshire, C. L., Gorden, J. A., Louie, B. E., Farviar, A. S., Stefanski, M. J., et al. (20162016). Safety and Efficacy of Intrapleural Tissue Plasminogen Activator and DNase during Extended Use in Complicated Pleural Space Infections. Can. Respir. J. 2016, 9796768. doi:10.1155/2016/9796768
Mehta, H. J., Biswas, A., Penley, A. M., Cope, J., Barnes, M., and Jantz, M. A. (2016). Management of Intrapleural Sepsis with once Daily Use of Tissue Plasminogen Activator and Deoxyribonuclease. Respiration 91, 101–106. doi:10.1159/000443334
Mehta, R., and Shapiro, A. D. (2008). Plasminogen Deficiency. Haemophilia 14, 1261–1268. doi:10.1111/j.1365-2516.2008.01825.x
Meyer, C. N., Armbruster, K., Kemp, M., Thomsen, T. R., and Dessau, R. B. (2018). Pleural Infection: a Retrospective Study of Clinical Outcome and the Correlation to Known Etiology, Co-morbidity and Treatment Factors. BMC Pulm. Med. 18, 160. doi:10.1186/s12890-018-0726-1
Miao, Y. F., Peng, T., Moody, M. R., Klegerman, M. E., Aronowski, J., Grotta, J., et al. (2018). Delivery of Xenon-Containing Echogenic Liposomes Inhibits Early Brain Injury Following Subarachnoid Hemorrhage. Sci. Rep. 8, 450. doi:10.1038/s41598-017-18914-6
Mingers, A. M., Philapitsch, A., Zeitler, P., Schuster, V., Schwarz, H. P., and Kreth, H. W. (1999). Human Homozygous Type I Plasminogen Deficiency and Ligneous Conjunctivitis. APMIS 107, 62–72. doi:10.1111/j.1699-0463.1999.tb01527.x
Moulton, J. S., Benkert, R. E., Weisiger, K. H., and Chambers, J. A. (1995). Treatment of Complicated Pleural Fluid Collections with Image-Guided Drainage and Intracavitary Urokinase. Chest 108, 1252–1259. doi:10.1378/chest.108.5.1252
Nasralla, A., Laing, B., and Turner, S. R. (2021). The Use of Fibrinolytic Therapy for Parapneumonic Effusion in Pregnancy: a Case Report and a Review. J. Cardiothorac. Surg. 16, 236. doi:10.1186/s13019-021-01619-3
Nie, W., Liu, Y., Ye, J., Shi, L., Shao, F., Ying, K., et al. (2014). Efficacy of Intrapleural Instillation of Fibrinolytics for Treating Pleural Empyema and Parapneumonic Effusion: a Meta-Analysis of Randomized Control Trials. Clin. Respir. J. 8, 281–291. doi:10.1111/crj.12068
Ohara, G., Iguchi, K., and Satoh, H. (2018). VATS and Intrapleural Fibrinolytic Therapy for Parapneumonic Empyema. Ann. Thorac. Cardiovasc. Surg. 24, 263–264. doi:10.5761/atcs.lte.18-00092
Ost, D. E. (2018). Machine Learning for Treating Complicated Parapneumonic Effusions: The Fundamentals Still Apply. Chest 154, 471–473. doi:10.1016/j.chest.2018.03.042
Pacilli, M., and Nataraja, R. M. (2018). Management of Paediatric Empyema by Video-Assisted Thoracoscopic Surgery (VATS) versus Chest drain with Fibrinolysis: Systematic Review and Meta-Analysis. Paediatr. Respir. Rev.
Petrakis, I. E., Heffner, J. E., and Klein, J. S. (2010). Surgery Should Be the First Line of Treatment for Empyema. Respirology 15, 202–207. doi:10.1111/j.1440-1843.2009.01677.x
Petrakis, I. E., Kogerakis, N. E., Drositis, I. E., Lasithiotakis, K. G., Bouros, D., and Chalkiadakis, G. E. (2004). Video-assisted Thoracoscopic Surgery for Thoracic Empyema: Primarily, or after Fibrinolytic Therapy Failure? Am. J. Surg. 187, 471–474. doi:10.1016/j.amjsurg.2003.12.048
Piccolo, F., Pitman, N., Bhatnagar, R., Popowicz, N., Smith, N. A., Brockway, B., et al. (2014). Intrapleural Tissue Plasminogen Activator and Deoxyribonuclease for Pleural Infection. An Effective and Safe Alternative to Surgery. Ann. Am. Thorac. Soc. 11, 1419–1425. doi:10.1513/AnnalsATS.201407-329OC
Piccolo, F., Popowicz, N., Wong, D., and Lee, Y. C. (2015). Intrapleural Tissue Plasminogen Activator and Deoxyribonuclease Therapy for Pleural Infection. J. Thorac. Dis. 7, 999–1008. doi:10.3978/j.issn.2072-1439.2015.01.30
Popowicz, N., Bintcliffe, O., De Fonseka, D., Blyth, K. G., Smith, N. A., Piccolo, F., et al. (2017). Dose De-escalation of Intrapleural Tissue Plasminogen Activator Therapy for Pleural Infection. The Alteplase Dose Assessment for Pleural Infection Therapy Project. Ann. Am. Thorac. Soc. 14, 929–936. doi:10.1513/AnnalsATS.201609-673OC
Popowicz, N., Nash, M., and Lee, Y. C. (2014). Unintentional Intramuscular Administration of tPA/DNase for Pleural Infection. Respirol Case Rep. 2, 144–146. doi:10.1002/rcr2.80
Popowicz, N., Piccolo, F., Shrestha, R., and Lee, Y. C. (2014). Two Sequential tPA/DNase Courses for Noncommunicating Loculated Collections in Pleural Infection. Respirol Case Rep. 2, 87–89. doi:10.1002/rcr2.58
Porcel, J. M., and Light, R. W. (2006). Diagnostic Approach to Pleural Effusion in Adults. Am. Fam. Physician 73, 1211–1220.
Prabhudesai, A., Shetty, S., Ghosh, K., and Kulkarni, B. (2017). Dysfunctional Fibrinolysis and Cerebral Venous Thrombosis. Blood Cell Mol Dis 65, 51–55. doi:10.1016/j.bcmd.2017.05.007
Pretorius, E., Humphries, P., Ekpo, O. E., Smit, E., and van der Merwe, C. F. (2007). Comparative Ultrastructural Analyses of Mouse, Rabbit, and Human Platelets and Fibrin Networks. Microsc. Res. Tech. 70, 823–827. doi:10.1002/jemt.20482
Rahman, N. M., Kahan, B. C., Miller, R. F., Gleeson, F. V., Nunn, A. J., and Maskell, N. A. (2014). A Clinical Score (RAPID) to Identify Those at Risk for Poor Outcome at Presentation in Patients with Pleural Infection. Chest 145, 848–855. doi:10.1378/chest.13-1558
Rahman, N. M., Maskell, N. A., West, A., Teoh, R., Arnold, A., Mackinlay, C., et al. (2011). Intrapleural Use of Tissue Plasminogen Activator and DNase in Pleural Infection. N. Engl. J. Med. 365, 518–526. doi:10.1056/NEJMoa1012740
Rahman, N. M., Singanayagam, A., Davies, H. E., Wrightson, J. M., Mishra, E. K., Lee, Y. C., et al. (2010). Diagnostic Accuracy, Safety and Utilisation of Respiratory Physician-Delivered Thoracic Ultrasound. Thorax 65, 449–453. doi:10.1136/thx.2009.128496
Ramnath, R. R., Heller, R. M., Ben-Ami, T., Miller, M. A., Campbell, P., Neblett, W. W., et al. (1998). Implications of Early Sonographic Evaluation of Parapneumonic Effusions in Children with Pneumonia. Pediatrics 101, 68–71. doi:10.1542/peds.101.1.68
Redden, M. D., Chin, T. Y., and van Driel, M. L. (2017). Surgical versus Non-surgical Management for Pleural Empyema. Cochrane Database Syst. Rev. 3, CD010651. doi:10.1002/14651858.CD010651.pub2
Reichert, M., and Bodner, J. (2018). Editorial on: Current State of Empyema Management. J. Thorac. Dis. 10, S4043–S4046. doi:10.21037/jtd.2018.10.46
Reichert, M., Hecker, M., Witte, B., Bodner, J., Padberg, W., Weigand, M. A., et al. (2017). Stage-directed Therapy of Pleural Empyema. Langenbecks Arch. Surg. 402, 15–26. doi:10.1007/s00423-016-1498-9
Reichert, M., Pösentrup, B., Hecker, A., Padberg, W., and Bodner, J. (2018). Lung Decortication in Phase III Pleural Empyema by Video-Assisted Thoracoscopic Surgery (VATS)-results of a Learning Curve Study. J. Thorac. Dis. 10, 4311–4320. doi:10.21037/jtd.2018.06.72
Reichert, M., Pösentrup, B., Hecker, A., Schneck, E., Pons-Kühnemann, J., Augustin, F., et al. (2018). Thoracotomy versus Video-Assisted Thoracoscopic Surgery (VATS) in Stage III Empyema-An Analysis of 217 Consecutive Patients. Surg. Endosc. 32, 2664–2675. doi:10.1007/s00464-017-5961-7
Rovina, N., Dima, E., Psallidas, I., Moschos, C., Kollintza, A., and Kalomenidis, I. (2013). Interleukin-18 Is Up-Regulated in Infectious Pleural Effusions. Cytokine 63, 166–171. doi:10.1016/j.cyto.2013.04.017
Saes, J. L., Schols, S. E. M., Betbadal, K. F., van Geffen, M., Verbeek-Knobbe, K., Gupta, S., et al. (2019). Thrombin and Plasmin Generation in Patients with Plasminogen or Plasminogen Activator Inhibitor Type 1 Deficiency. Haemophilia 25, 1073–1082. doi:10.1111/hae.13842
Samancilar, O., Akçam, T. İ., Kaya, S. O., Ozturk, O., Akcay, O., and Ceylan, K. C. (2018). The Efficacy of VATS and Intrapleural Fibrinolytic Therapy in Parapneumonic Empyema Treatment. Ann. Thorac. Cardiovasc. Surg. 24, 19–24. doi:10.5761/atcs.oa.17-00153
Sasse, S., Nguyen, T., Teixeira, L. R., and Light, R. (1999). The Utility of Daily Therapeutic Thoracentesis for the Treatment of Early Empyema. Chest 116, 1703–1708. doi:10.1378/chest.116.6.1703
Sasse, S., Nguyen, T. K., Mulligan, M., Wang, N. S., Mahutte, C. K., and Light, R. W. (1997). The Effects of Early Chest Tube Placement on Empyema Resolution. Chest 111, 1679–1683. doi:10.1378/chest.111.6.1679
Scarci, M., Zahid, I., Billé, A., and Routledge, T. (2011). Is Video-Assisted Thoracoscopic Surgery the Best Treatment for Paediatric Pleural Empyema? Interact Cardiovasc. Thorac. Surg. 13, 70–76. doi:10.1510/icvts.2010.254698
Semenkovich, T. R., Olsen, M. A., Puri, V., Meyers, B. F., and Kozower, B. D. (2018). Current State of Empyema Management. Ann. Thorac. Surg. 105, 1589–1596. doi:10.1016/j.athoracsur.2018.02.027
Shah, S. S., Ten Have, T. R., and Metlay, J. P. (2010). Costs of Treating Children with Complicated Pneumonia: a Comparison of Primary Video-Assisted Thoracoscopic Surgery and Chest Tube Placement. Pediatr. Pulmonol 45, 71–77. doi:10.1002/ppul.21143
Shen, K. R., Bribriesco, A., Crabtree, T., Denlinger, C., Eby, J., Eiken, P., et al. (2017). The American Association for Thoracic Surgery Consensus Guidelines for the Management of Empyema. J. Thorac. Cardiovasc. Surg. 153, e129–e146. doi:10.1016/j.jtcvs.2017.01.030
Skeete, D. A., Rutherford, E. J., Schlidt, S. A., Abrams, J. E., Parker, L. A., and Rich, P. B. (2004). Intrapleural Tissue Plasminogen Activator for Complicated Pleural Effusions. J. Trauma 57, 1178–1183. doi:10.1097/01.ta.0000141879.67441.52
Slaats, M. A., De Dooy, J., Van Hoorenbeeck, K., Van Schil, P. E. Y., Verhulst, S. L., and Hendriks, J. M. H. (2019). A Combined Intrapleural Administration of Dornase Alfa and Tissue Plasminogen Activator Is Safe in Children with Empyema - A Pilot Study. Acta Chir Belg. 1-5, 1–5. doi:10.1080/00015458.2019.1696516
Søgaard, M., Nielsen, R. B., Nørgaard, M., Kornum, J. B., Schønheyder, H. C., and Thomsen, R. W. (2014). Incidence, Length of Stay, and Prognosis of Hospitalized Patients with Pleural Empyema: a 15-year Danish Nationwide Cohort Study. Chest 145, 189–192. doi:10.1378/chest.13-1912
Sonnappa, S., Cohen, G., Owens, C. M., van Doorn, C., Cairns, J., Stanojevic, S., et al. (2006). Comparison of Urokinase and Video-Assisted Thoracoscopic Surgery for Treatment of Childhood Empyema. Am. J. Respir. Crit. Care Med. 174, 221–227. doi:10.1164/rccm.200601-027OC
Sonnappa, S., and Jaffe, A. (2007). Treatment Approaches for Empyema in Children. Paediatr. Respir. Rev. 8, 164–170. doi:10.1016/j.prrv.2007.05.002
Sonnappa, S. (2015). Urokinase and VATS Are Equally Effective for Septated Empyema. J. Pediatr. 166, 1320–1321. doi:10.1016/j.jpeds.2015.02.059
St Peter, S. D., Tsao, K., Spilde, T. L., Keckler, S. J., Harrison, C., Jackson, M. A., et al. (2009). Thoracoscopic Decortication vs Tube Thoracostomy with Fibrinolysis for Empyema in Children: a Prospective, Randomized Trial. J. Pediatr. Surg. 44, 106–111. doi:10.1016/j.jpedsurg.2008.10.018
Stefani, A., Aramini, B., della Casa, G., Ligabue, G., Kaleci, S., Casali, C., et al. (2013). Preoperative Predictors of Successful Surgical Treatment in the Management of Parapneumonic Empyema. Ann. Thorac. Surg. 96, 1812–1819. doi:10.1016/j.athoracsur.2013.06.013
Stefanutti, G., Ghirardo, V., Barbato, A., and Gamba, P. (2010). Evaluation of a Pediatric Protocol of Intrapleural Urokinase for Pleural Empyema: a Prospective Study. Surgery 148, 589–594. doi:10.1016/j.surg.2010.01.010
Taylor, M. D., and Kozower, B. D. (2012). Surgical Spectrum in the Management of Empyemas. Thorac. Surg. Clin. 22, 431–440. doi:10.1016/j.thorsurg.2012.04.007
Teixeira, L. R., Sasse, S. A., Villarino, M. A., Nguyen, T., Mulligan, M. E., and Light, R. W. (2000). Antibiotic Levels in Empyemic Pleural Fluid. Chest 117, 1734–1739. doi:10.1378/chest.117.6.1734
Temes, R. T., Follis, F., Kessler, R. M., Pett, S. B., and Wernly, J. A. (1996). Intrapleural Fibrinolytics in Management of Empyema Thoracis. Chest 110, 102–106. doi:10.1378/chest.110.1.102
Tenbrock, K., Lehmann, S., Schrading, S., and Moran, J. (2019). Replacement Therapy with Glu-Plasminogen for the Treatment of Severe Respiratory and Auditory Complications of Congenital Plasminogen Deficiency. Acta Haematol. 141, 146–147. doi:10.1159/000495336
Thommi, G., Nair, C. K., Aronow, W. S., Shehan, C., Meyers, P., and McLeay, M. (2007). Efficacy and Safety of Intrapleural Instillation of Alteplase in the Management of Complicated Pleural Effusion or Empyema. Am. J. Ther. 14, 341–345. doi:10.1097/01.mjt.0000208275.88120.d1
Thommi, G., Shehan, C. J., and McLeay, M. T. (2014). Fibrinolytics in Parapneumonic Effusions/empyemas. Chest 146, e103–e104. doi:10.1378/chest.14-0706
Thommi, G., Shehan, J. C., Robison, K. L., Christensen, M., Backemeyer, L. A., and McLeay, M. T. (2012). A Double Blind Randomized Cross over Trial Comparing Rate of Decortication and Efficacy of Intrapleural Instillation of Alteplase vs Placebo in Patients with Empyemas and Complicated Parapneumonic Effusions. Respir. Med. 106, 716–723. doi:10.1016/j.rmed.2012.02.005
Thomson, A. H., Hull, J., Kumar, M. R., Wallis, C., and Balfour Lynn, I. M. (2002). Randomised Trial of Intrapleural Urokinase in the Treatment of Childhood Empyema. Thorax 57, 343–347. doi:10.1136/thorax.57.4.343
Torbic, H., Inaty, H., Raja, S., and Choi, H. (2017). Safe Administration of Intrapleural Alteplase during Pregnancy. J. Thorac. Dis. 9, E801–E804. doi:10.21037/jtd.2017.07.114
Touray, S., Sood, R. N., Lindstrom, D., Holdorf, J., Ahmad, S., Knox, D. B., et al. (1962). Risk Stratification in Patients with Complicated Parapneumonic Effusions and Empyema Using the RAPID Score. Lung 196, 623–629. doi:10.1007/s00408-018-0146-2
Tucker, T., Tsukasaki, Y., Sakai, T., Mitsuhashi, S., Komatsu, S., Jeffers, A., et al. (2019). Myocardin Is Involved in Mesothelial-Mesenchymal Transition of Human Pleural Mesothelial Cells. Am. J. Respir. Cel Mol Biol. doi:10.1164/ajrccm-conference.2019.199.1_meetingabstracts.a5348
Tucker, T. A., Jeffers, A., Boren, J., Quaid, B., Owens, S., Koenig, K. B., et al. (2016). Organizing Empyema Induced in Mice by Streptococcus Pneumoniae: Effects of Plasminogen Activator Inhibitor-1 Deficiency. Clin. Transl Med. 5 (17), 17. doi:10.1186/s40169-016-0097-2
Vial, M. R., Ost, D. E., Eapen, G. A., Jimenez, C. A., Morice, R. C., O'Connell, O., et al. (2016). Intrapleural Fibrinolytic Therapy in Patients with Nondraining Indwelling Pleural Catheters. J. Bronchology Interv. Pulmonol 23, 98–105. doi:10.1097/LBR.0000000000000265
White, H. D., Henry, C., Stock, E. M., Arroliga, A. C., and Ghamande, S. (2015). Predicting Long-Term Outcomes in Pleural Infections. RAPID Score for Risk Stratification. Ann. Am. Thorac. Soc. 12, 1310–1316. doi:10.1513/AnnalsATS.201505-272OC
Wilkosz, S., Edwards, L. A., Bielsa, S., Hyams, C., Taylor, A., Davies, R. J., et al. (2011). Characterization of a New Mouse Model of Empyema and the Mechanisms of Pleural Invasion by Streptococcus Pneumoniae. Am. J. Respir. Cel Mol Biol 46 (2), 180–187.
Keywords: empyema, fibrinolytic therapy, preclinical, treatment, animal model
Citation: Karandashova S, Florova G, Idell S and Komissarov AA (2022) From Bedside to the Bench—A Call for Novel Approaches to Prognostic Evaluation and Treatment of Empyema. Front. Pharmacol. 12:806393. doi: 10.3389/fphar.2021.806393
Received: 31 October 2021; Accepted: 31 December 2021;
Published: 20 January 2022.
Edited by:
Jing Qu, Huazhong University of Science and Technology, ChinaCopyright © 2022 Karandashova, Florova, Idell and Komissarov. This is an open-access article distributed under the terms of the Creative Commons Attribution License (CC BY). The use, distribution or reproduction in other forums is permitted, provided the original author(s) and the copyright owner(s) are credited and that the original publication in this journal is cited, in accordance with accepted academic practice. No use, distribution or reproduction is permitted which does not comply with these terms.
*Correspondence: Andrey A. Komissarov, YW5kcmV5LmtvbWlzc2Fyb3ZAdXRoY3QuZWR1
Disclaimer: All claims expressed in this article are solely those of the authors and do not necessarily represent those of their affiliated organizations, or those of the publisher, the editors and the reviewers. Any product that may be evaluated in this article or claim that may be made by its manufacturer is not guaranteed or endorsed by the publisher.
Research integrity at Frontiers
Learn more about the work of our research integrity team to safeguard the quality of each article we publish.