- 1Department of Clinical Pharmacy, Shanghai General Hospital, Shanghai Jiaotong University School of Medicine, Shanghai, China
- 2Department of Health Statistics, Naval Medical University, Shanghai, China
- 3Department of Pharmacy, Shanghai Fourth People’s Hospital Affiliated to Tongji University School of Medicine, Shanghai, China
Background: The beneficial effects of colchicine on cardiovascular disease have been widely reported in recent studies. Previous research demonstrated that colchicine has a certain protective effect on ischemic myocardium and has the potential to treat myocardial ischemia reperfusion injury (MIRI). However, the potential targets and pharmacological mechanism of colchicine to treat MIRI has not been reported.
Methods: In this study, we used network pharmacology and experimental verification to investigate the pharmacological mechanisms of colchicine for the treatment of MIRI. Potential targets of colchicine and MIRI related genes were screened from public databases. The mechanism of colchicine in the treatment of MIRI was determined by protein-protein interaction (PPI), gene ontology (GO) and Kyoto Encyclopedia of Genes and Genomes (KEGG) pathway enrichment analysis. Additionally, we evaluated the effect of colchicine on H9C2 cell activity using CCK-8 assays, observed the effect of colchicine on H9C2 cell apoptosis via flow cytometry, and further verified the expression of key targets after colchicine treated by Western blot.
Results: A total of 626 target genes for colchicine and 1549 MIRI disease targets were obtained. 138 overlapping genes were determined as potential targets of colchicine in treating MIRI. the PPI network analysis demonstrated that the targets linked to MIRI were ALB, TNF, ACTB, AKT1, IL6, TP53, IL1B, CASP3 and these targets showed nice affinity with colchicine in molecular docking experiments. The results of GO analysis and KEGG pathway enrichment demonstrated that the anti-MIRI effect of colchicine involves in apoptotic signaling pathway. Further tests suggested that colchicine can protect H9C2 cell from Hypoxia/Reoxygenation (H/R) injury through anti-apoptotic effects. Western blot results demonstrated that colchicine can inhibited MIRI induced apoptosis of H9C2 cell by enhancing the decreased levels of Caspase-3 in myocardial injure model induced by H/R and activating the PI3K/AKT/eNOS pathway.
Conclusions: we performed network pharmacology and experimental evaluation to reveal the pharmacological mechanism of colchicine against MIRI. The results from this study could provide a theoretical basis for the development and clinical application of colchicine.
Introduction
Acute Myocardial Infarction is the necrosis of myocardial tissue caused by acute or persistent ischemia and hypoxia of coronary arteries, which seriously threatens the lives of patients (Castro-Dominguez et al., 2018; Gulati et al., 2020). The most effective treatment for reducing the area of myocardial infarction is reperfusion timely (Bajaj et al., 2015; Toldo and Abbate, 2018). However, after restoring blood supply to the damaged myocardial tissue, many patients suffered more severe damage to the heart structure, more disordered energy metabolism of myocardial cells and even some irreversible damage such as re-enlargement of myocardial infarction area, life-threatening arrhythmia and so forth, therefore, we called it myocardial ischemia reperfusion injury (MIRI) (Buja, 2005; Marchant et al., 2012). Studies have revealed that the physiological mechanisms of MIRI are apoptosis, calcium overload, oxidative stress, autophagy, inflammation, endoplasmic reticulum stress, mitochondrial disorders, etc (Walters et al., 2012; Munzel et al., 2017). Previous research found that cardiomyocyte apoptosis is the core factor of MIRI, and about 50% of MIRI is related with cardiomyocyte apoptosis (Buja, 2005). Myocardial ischemia triggers the process of cardiomyocyte apoptosis, and reperfusion further aggravates the degree of this process, which would result in malignant arrhythmia, heart failure and even sudden cardiac death (Nagai et al., 2010; Fujiwara et al., 2019). Myocardial ischemia and hypoxia can induce the expression of a variety of apoptosis-related factors and inflammatory factors, which could promote abnormal apoptosis of myocardial cells (Yao et al., 2020). As an important factor affecting the progression of disease, the imbalance of myocardial cell apoptosis is also an important regulatory pathway for drugs to play a therapeutic role. Unfortunately, at present, there are few effective medicines for the treatment of MIRI in clinical practice.
Colchicine, which is a zhuophenone alkaloid extracted from the bulb of Colchicum autumnale, has extensive anti-inflammatory, immunosuppressive and strong anti-fibrosis effects, it is the first-line drug for the treatment of acute gout attack (Downing, 2000; Terkeltaub, 2009; Roubille et al., 2013). A retrospective study suggested that the use of colchicine in gout patients can reduce the incidence of acute myocardial infarction (Crittenden et al., 2012). Studies have reported that STEMI patients with chest pain less than 12 h were randomly treated with colchicine or placebo for 5 days after percutaneous coronary intervention (PCI), the CK-MB level in the colchicine group was declined and the area of infarction decreased significantly (Deftereos et al., 2015). The COLCOT study have demonstrated that colchicine can reduce the risk of recurring myocardial ischemic in patients after acute myocardial infarction and it has good safety in low dose (Tardif et al., 2019). What’s more, the earlier you start using colchicine after acute myocardial infarction, the greater the benefit (Bouabdallaoui et al., 2020). Above all, colchicine has a certain protective effect on ischemic myocardium and has the potential to treat MIRI, but its mechanism is still unclear.
Network pharmacology is developed based on the theories of systems biology, network biology, multidirectional pharmacology and omics. It reveals the interaction mechanism between the body and disease from the aspects of protein, molecule and gene, and provides a basis for the development and mechanism research of multi-target drugs (Hopkins, 2007; Huang et al., 2014; Zhang et al., 2017). This study screened the potential targets and functional characteristics of colchicine in the treatment of MIRI through network pharmacology. The colchicine multi-target network was constructed, and the key targets were enriched. In addition, the key targets were identified by experimental verification to further confirm the effect of colchicine on related signal pathways. The process of network pharmacology analysis is shown in Figure 1. The main purpose of this study is to explore the therapeutic targets and key signal pathways of colchicine in treatment of MIRI, and provide potential strategies for disease treatment.
Material and Methods
Prediction of Potential Targets of Colchicine
The 2D molecular structure and PubChem CID of Colchicine were obtained from PubChem (https://pubchem.ncbi.nlm.nih.gov/, update time:2021-02-04), which is the world’s largest database of free access to chemical information. Swiss Target Prediction (http://www.swisstargetprediction.ch, update time:2019) (Daina et al., 2019), Comparative Toxicogenomics database (CTD, http://ctdbase.org/about/, update time: 2021-09-02) (Davis et al., 2019), BATMAN-TCM database (http://bionet.ncpsb.org.cn/batman-tcm/, update time: 2020-07-29) (Liu et al., 2016) and PharmMapper (http://lilab-ecust.cn/pharmmapper/, update time: 2017) (Wang et al., 2017) were used to obtain the targets name, gene name, UniProt ID and FIT score of colchicine. Then the names of target genes were converted into the official symbols using UniProt database (http://www.uniprot.org/, update time: 2021-04) (UniProt, 2019) and types was selected to “Homo sapiens”.
Acquisition of MIRI Related Genes
The therapeutic targets for MIRI were collected from the Online Mendelian Inheritance in Man (OMIM, http://www.omim.org/, update time: 2021-09-02) (Amberger et al., 2015), Therapeutic Target database (TTD, https://db.idrblab. org/ttd/, update time: 2021-09-29) (Wang et al., 2020) and GeneCards (https://www.genecards.org/, update time: 2021-09-13) (Fishilevich et al., 2016). The MIRI was used as the searching keyword and the putative targets of colchicine and the known therapeutic targets on MIRI amalgamated.
Protein-Protein Interaction (PPI) Networks Construction
The STRING database is a database of known and predicted protein-protein interaction. The potential targets of colchicine in the treatment of MIRI were put into STRING V11.5 (https://string-db.org/cgi/input.pl, update time: 2021) (Szklarczyk et al., 2017) to build the PPI network interaction. Cytoscape V3.8.2 (http://www.cytoscape.org/, update time: 2020-10-29) (Shannon et al., 2003) were used to construct and visualize the PPI network. The topological properties of PPI network were examined by 'Network analyzer' a cytoscape plugin app. Nodes in the network are connected by edges if there are regulatory relations. The parameters of “Degree”, “Average Shortest Path Length”, “Betweenness Centrality”, “Closeness Centrality”, “Clustering Coefficient” were estimated, and the value of “Degree” was used as a reference for the importance of the target.
Gene Ontology (GO) and Kyoto Encyclopedia of Genes and Genomes (KEGG) Pathway Enrichment Analysis
Metascape (http://metascape.org/gp/index.html, update time: 2021-10-18) (Zhou et al., 2019) was used to conducted GO enrichment analysis and KEGG pathway enrichment analysis. The operation is simply to copy and paste the core genes in the Metascape’s gene list and then select the species of Homo sapiens. In the Enrichment section of the analysis page, KEGG Pathways, GO Molecular Functions, GO Biological Processes and GO Cellular Components were selected for enrichment analysis of KEGG Pathways, GO Molecular Functions, GO Biological Processes and GO Cellular Components respectively. The visualization of the important enrichment results of GO and KEGG pathways was implemented by “ggplot2” package of R version 4.0.2 software (update time: 2020-06-22).
Molecular Docking
The 3D structure of the target protein was downloaded from the PDB database (https://www.rcsb.org/). The target protein is processed by removing ligand and water motifs and adding hydrogen using AutoDockTools-1.5.6 (update time: 2014-09-17) (Trott and Olson, 2010). The protein receptors and ligand were converted to PDBQT format. Finally, AutoDock vina software will be used for molecular docking, and the lowest free energy model is selected for visual analysis by using PyMOL. The docking score is carried out for each docking. The smaller the docking score, the better the docking result. Docking score < -6 indicates good binding affinity.
Experimental Chemicals and Reagents
Fetal bovine serum (FBS), phosphate buffered saline (PBS), Krebs-Ringer Bicarbonate buffer, penicillin-streptomycin, trypsin-EDTA and dulbecco modified eagle medium (DMEM) were purchased from GIBCO (Grand Island, NY, United States). Protease inhibitor, RIPA Lysis buffer and BCA protein assay kit were obtained from Beyotime Biotechnology (Shanghai, China). Cell Counting Kit-8 (CCK8) detection kit was obtained from the BBI Life Sciences company (Shanghai, China). Annexin V-FITC/PI apoptosis kits were purchased from YEASEN biological technology company (Shanghai, China). Polyvinylidene fluoride (PVDF) membrane was purchased from Millipore company, (United States). Enhanced chemiluminescence kit (ECL) reagent was obtained from ThermoFisher Scientific, Ltd, (United States). Colchicine and LY294002 were obtained from MedChemExpress company (Shanghai, China) with the purity over 98%. Primary antibodies against Phospho-PI3K, Phospho-AKT, Phospho-eNOS, caspase3, GAPDH and antibodies against PI3K, AKT, eNOS, c-Caspase3 were purchased from ZEN-BIOSCIENCE company (Chendu, China).
H9C2 Myocardial Cell Culture and Treatments
H9C2 cells were purchased from the National Collection of Authenticated Cell Cultures (Shanghai, China) and cultured in DMEM containing 10% FBS and 1% penicillin/streptomycin in CO2 incubator at 37°C and 95% relative humidity.
The H9C2 cells were pretreated with colchicine (0–5 μM) for 24 h, then the drug-containing medium was removed from the colchicine-treated cells and replaced by Krebs–Ringer Bicarbonate buffer, and cells were put into a modular incubator chamber saturated with 99.99% N2 and cultured in a normal incubator for the indicated time (0–16 h). Then the cells were subjected to reoxygenation by changing the DMEM back, and cultured under normoxia (21% O2) for 2 h. The H9C2 cells Hypoxia/Reoxygenation (H/R) model were established.
Detection of Cell Viability
CCK-8 assays were carried out to detect the effect of colchicine on H9C2 cell activity. Briefly, the H9C2 cells were seeded into 96-well plates at the density of 3 × 103 cells in culture medium. After processing the cells as previously described, 10 μL of CCK-8 was then added to each well and placed the 96-well plate in the incubator for 1 h. The absorbance values of each well were determined spectrophotometrically at 450 nm using a microplate reader (Bio-Tek, United States). Each experiment was repeated 5 times.
Detection of Apoptosis
H9C2 cell apoptosis was detected by flow cytometry (Beckman Coulter Inc, Atlanta, Georgia, United States). The cells were trypsinized using 0.25% trypsin. The H9C2 cells in suspension (1 × 106 cells) were extracted and washed using phosphate-buffered saline. The cells were resuscitated with 100 μL of binding buffer, mixed with 5 μL of Annexin V-FITC and 10 μL of PI, and incubated at room temperature in dark for 15 min. The samples were assessed using flow cytometer analysis. Each experiment was repeated 3 times.
Western Blot Analysis
To determine anti-MIRI effects of colchicine through caspase3 and PI3K/AKT/eNOS signaling, protein phosphorylation was measured by western blotting assay. Briefly, H9C2-cell lysates were prepared in RIPA Lysis buffer after drug administration. The protein concentrations were analyzed by the BCA protein assay kit. Protein extracts were separated by 8–15% SDS-PAGE according to different molecular weights, and then transferred onto PVDF membrane. The membranes were blocked with 5% nonfat dry milk in TBST for 1 h at room temperature, and were then incubated overnight at 4°C with the following primary antibodies: p-PI3K (1:1,000), p-Akt (1:1,000), p-eNOS (1:1,000), Caspase-3 (1:1,000) and GAPDH (1:1,000). The membranes were then incubated for 1 h with goat anti-rabbit IgG-HRP (1:5,000) as the secondary antibody. Antibody-bound protein was detected by ECL reagent. GAPDH served as the internal control. ImageJ Java 1.8.0 software (update time: 2021-09-28) (NIH, United States) was used to analyze the imaging map and calculate the ratios of PI3K, AKT, eNOS and Cascapse3 proteins in each group.
Statistical Analysis
All the data are expressed as the mean ± SD. The statistical analysis was performed with SPSS version 21.0 (update time: 2020) (IBM Corporation, Armonk, NY). For multiple comparisons, one-way analysis of variance (ANOVA) was performed, and comparisons between two groups were analyzed using an LSD test. A value of p < 0.05 was regarded as statistically significant.
Ethics Statements
This study is not animal study, human study and have no identifiable human images/data.
Results
Active Components and Potential Targets of Colchicine on MIRI
The molecular formula of colchicine is C22H25NO6, and its molecular structure is shown in Figure 2. We searched the PharmMapper, Swiss Target Prediction, CTD, and BATMAN-TCM database, and a total of 626 target genes for colchicine were obtained by UniProt gene annotation simultaneously (Supplementary Table S1). For MIRI, we collected 1,549 disease targets through OMIM, GeneCards, and TTD (Supplementary Table S2). By matching the targets of colchicine with MIRI related targets, 227 cross-genes were derived, which were potential targets of colchicine in treating MIRI (Figure 3).
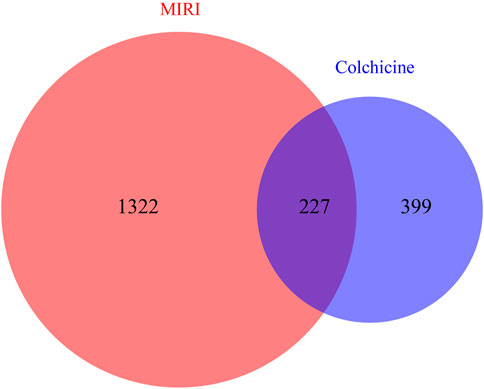
FIGURE 3. Venn diagrams showing the number of shared and unique targets by Colchicine and MIRI were represented. A total of 227 targets of colchicine with therapeutic potential for MIRI were identified.
Conversion of Target Proteins Into Network and Analysis
We uploaded a total of 227 cross-genes into the STRING database for PPI analysis. Then we imported the results from STRING database into Cytoscape to estimate the topological properties of PPI network. From Supplementary Figure S1, a total of 227 nodes and 4,709 edges were acquired. We selected 56 core target genes based on the Q3 of node degree (61), included ALB, TNF, ACTB, AKT1, IL6, TP53, IL1B, CASP3, et al. Figure 4 showed the topological properties results of these 56 core target genes with no isolated nodes. Nodes represented proteins, and each edge represented the interaction relationship between proteins. The more lines, the greater the correlation degree. The average clustering coefficient is 0.4931, average degree is 89.6250, average shortest path length is between 1.3097∼1.7655, and betweenness centrality is between 0.0013∼0.0747. According to our results, it is suggested that ALB, TNF, ACTB, AKT1, IL6, TP53, IL1B, CASP3, may be the key targets of colchicine in the treatment of MIRI.
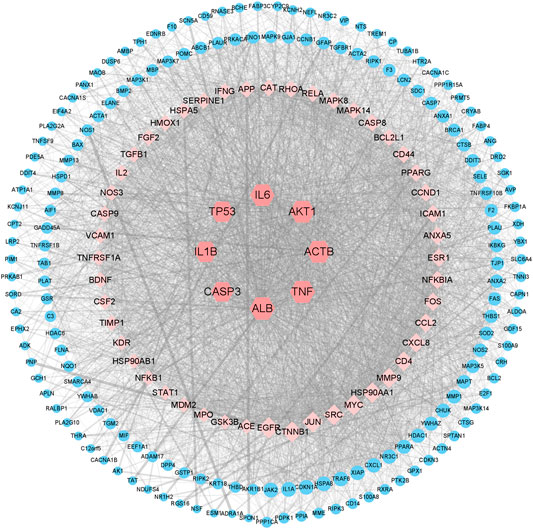
FIGURE 4. Network pharmacology revealed the targets characteristics of colchicine with therapeutic potential for MIRI. A protein network of known targets of colchicine with therapeutic potential for MIRI was generated by Cytoscape.
Gene Ontology Enrichment Analysis
We imported the selected potential 56 core target genes into the Metascape for GO analysis. A total of 98 biological processes were enrichment, such as response to lipopolysaccharide, apoptotic signaling pathway, response to inorganic substance, and et al. A total of 14 cellular component were enrichment, such as membrane raft, vesicle lumen, focal adhesion, and et al. A total of 23 molecular function were enrichment, such as protein kinase binding, cytokine receptor binding, RNA polymerase II-specific DNA-binding transcription factor binding, and et al. (Figure 5A).
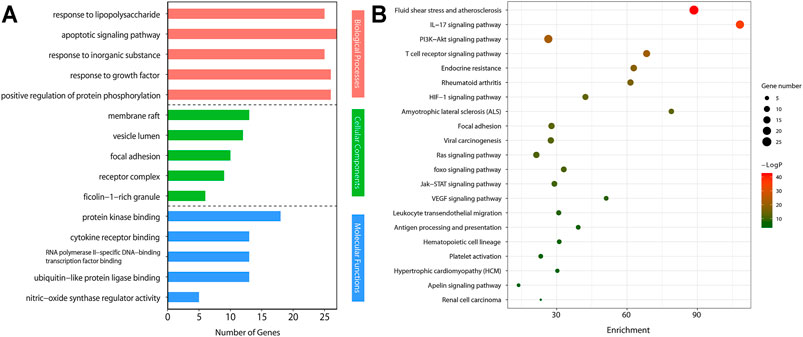
FIGURE 5. Gene Ontology (GO) analyses and Kyoto Encyclopedia of Genes and Genomes (KEGG) pathways by Metascape.(A) GO enrichment analysis of target genes. The number of GO entries in the functional categories of biological process (BP), cell composition (CC), and molecular function (MF) (p < 0.05).(B) KEGG pathways of target genes (p < 0.05).
KEGG Pathway Enrichment Analysis
We conducted KEGG pathway enrichment analysis on potential 56 core target genes using Metascape and screened out 21 pathways. Figure 5B showed these 21 pathways, such as Fluid shear stress and atherosclerosis, IL-17 signaling pathway, PI3K-Akt signaling pathway, T cell receptor signaling pathway, and et al. As shown in Figure 5B, colchicine may play an anti-MIRI role by regulating cascade reactions of multiple signaling pathways. Interestingly, consistent with the results of the previous GO functional annotation, the KEGG pathway also enriched the apoptotic pathways. It is known that apoptosis plays an important role in MIRI injury of myocardial cells. Therefore, it is suggesting that colchicine may protect ischemic myocardium by inhibiting apoptosis in myocardial cells. Based on the enriched pathway of apoptosis, the PI3K/AKT signaling pathway (https://www.kegg.jp/kegg/) was obtained through further screening in the apoptotic mechanism (Figure 6).
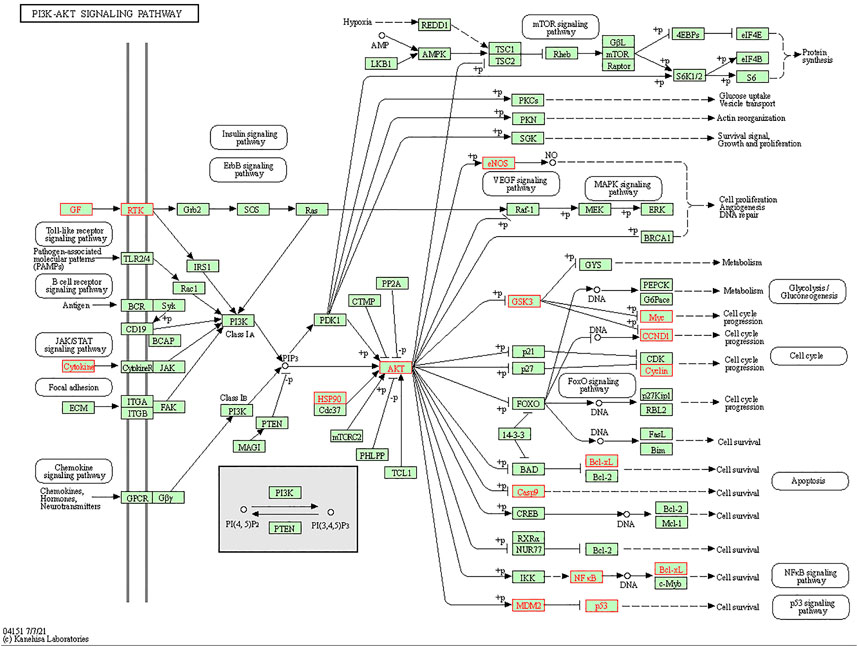
FIGURE 6. Screening of PI3K/AKT signaling pathway in apoptosis. The red marked nodes represent overlapping targets of colchicine and MIRI.
Molecular Docking Analysis
Molecular docking analysis was conducted to evaluate the binding affinity of colchicine with key target proteins. In order to evaluate the biological activity of colchicine, the docking simulation of colchicine and the key target proteins were implemented by molecular docking analysis. As can be seen from Figure 7 and Table 1, the results showed that docking scores of colchicine with ALB, TNF, ACTB, AKT1, IL6, TP53, IL1B and CASP3 ranged from -6.1 to -7.6, and all the hub proteins showed better binding affinity with colchicine. Most of these hub proteins are involved in cell apoptosis.
Result of CCK-8 Cell Viability Assay
To determine the effect of colchicine on H9C2 cells, we used different concentrations of colchicine (0.05, 0.5, 5 μM) to stimulate H9C2 cells and detected the cell viability by CCK8 assay. The cell viability was also measured after incubation with various time of H/R (0, 2, 4, 8, 16 h). From Figure 8, we found that groups with 0.5 μM or 5 μM colchicine have significantly higher cell viability than others hypoxia groups before 8 h, and the results of 5 μM colchicine group were more stable (the SD was smaller). We also observed that there was a significant decrease in cell viability of 0.5 and 5 μM colchicine groups at 16 h. Maybe it’s the toxic effects of colchicine. Therefore, we choose 5 μM as the optimum concentration of colchicine to anti-MIRI at 8 h.
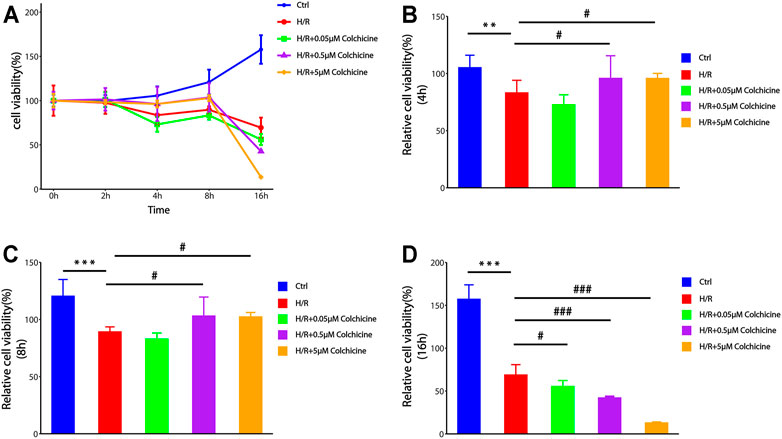
FIGURE 8. The effect of different concentrations of colchicine and different time of H/R-damaged on the viability of H9C2 cell by CCK-8 assays.(A) Optimum concentration of colchicine in appropriate time H/R-damaged was determined by CCK8 assay.(B–D) The effect of different colchicine concentrations on H9C2 cell viability after 4 h/8 h/16 h H/R injury (***p < 0.001, **p < 0.01 compared with control; #p < 0.05, ###p < 0.001 compared with H/R group).
Result of Apoptosis Detection
CCK-8 assay results suggested that colchicine can protect H9C2 cell form H/R injury. To determine whether this anti-MIRI effect was related to apoptosis, flow cytometry was used to detect the apoptosis-inducing effect of colchicine on H9C2 cells and the percentage of apoptotic cells was also detected by Annexin V-FITC and PI double staining. As shown in Figure 9, the apoptosis rate was increased in 6.182%, which is significantly when cells were treated with H/R compared with the controls (1.214%). However, colchicine with 5 μM caused a significant reduction (4.490%) of early apoptotic cells as compared with the H/R only groups. Additionally, LY294002 (PI3K inhibitor) significantly attenuated the effects of colchicine, which can be observed from the apoptotic rate increased to 7.993%. These results suggested that colchicine plays an antiapoptotic role partly on H/R injury of H9C2 cell.
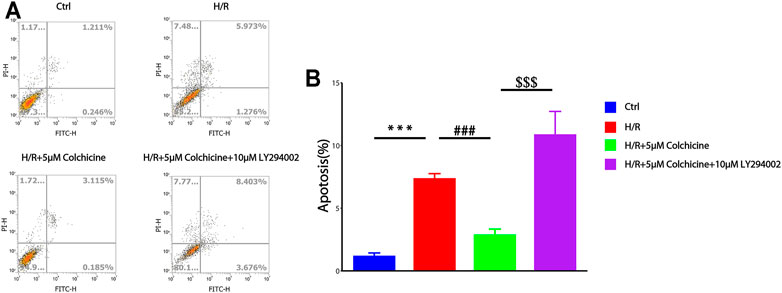
FIGURE 9. Flow cytometry was used to detect the apoptosis-inducing effect of colchicine on H9C2 cells. (A) the apoptotic effects of colchicine was measured through flow cytometry.(B) The quantitative results were analyzed. The data were expressed as mean ± SD (***p < 0.001 compared with control; ###p < 0.001 compared with H/R group; $$$p < 0.001 compared with colchicine treated alone).
Result of Western Blot Analysis
PI3K/AKT is one of the main signal transduction pathways involved in cell proliferation and apoptosis, and eNOS is a downstream of PI3K/AKT which related to the anti-MIRI effect of colchicine base on the result of GO enrichment and KEGG enrichment (Zhang et al., 2019). To further study the mechanisms underlying the antiapoptotic effects of colchicine and the effects of colchicine on PI3K/AKT/eNOS signaling pathway, we detected the apoptosis-related protein caspase3 and the key proteins of PI3K, AKT, eNOS in the signaling pathway. From Figure 10, it could be seen that after H/R injury in H9C2 cell, phosphorylation levels of PI3K, AKT, and eNOS proteins were significantly decreased compared with those in the control group (p < 0.001). Compared with the H/R group, 5 μM colchicine observably increased the phosphorylation levels of PI3K, AKT, and eNOS (p < 0.01). Simultaneously, c-Caspase3 was significantly increased expression in H/R treated H9C2 cell (p < 0.001), however, markedly reduced by 5 μM colchicine treatment (p < 0.01). Moreover, the use of LY294002 (PI3K inhibitor) significantly prevented the effects of colchicine (p < 0.001), which can be shown from the protein expression of p-PI3K, p-AKT, p-eNOS and c-Caspase-3. Our results demonstrated that colchicine can inhibited MIRI induced apoptosis of H9C2 cell by activating the PI3K/AKT/eNOS pathway.
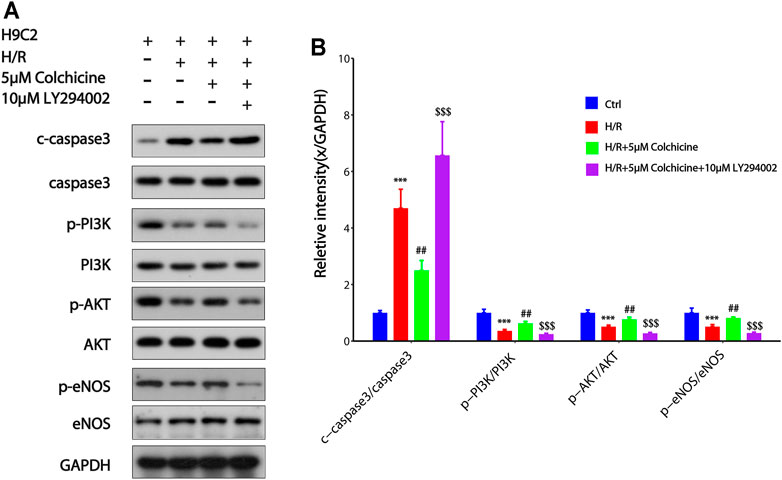
FIGURE 10. Colchicine inhibited MIRI-induced apoptosis of H9C2 by regulating the PI3K/AKT/eNOS pathway.(A) Protein expression of p-PI3K, p-AKT, p-eNOS, and Caspase-3 were detected by western blot analysis;(B) Protein content was quantified using densitometric analysis with GAPDH as internal control. The values of control group were set as one. Data were expressed as mean ± SD (***p < 0.001 compared with control; ##p < 0.01 compared with H/R group; $$$p < 0.001 compared with colchicine treated alone).
Discussion
MIRI is a common pathophysiological injury in ischemic heart disease, shock, extracorporeal circulation, cardiovascular surgery and other diseases or treatments. It causes damage to tissues, organs, etc. through an excessive cascade reaction (Gao et al., 2012). Moreover, together with the injury caused by ischemia, it determines the final region of myocardial infarction and the process of ventricular remodeling, and eventually develops into heart failure (Herr et al., 2020). At present, endogenous protective methods are widely used to reduce reperfusion injury and improve the prognosis of patients with acute myocardial infarction, for example, ischemic preconditioning, ischemic post-conditioning and pharmacological post-conditioning (Wu et al., 2018). Nevertheless, there are few effective drugs for the treatment of MIRI clinically. Therefore, finding a resultful drug to treat MIRI makes sense (Bellis et al., 2020). Network pharmacology is an emerging discipline based on systems biology, which is used to analyze biological system networks to select specific signal nodes, so that the potential interaction between drugs and targets can be accurately determined (Barabasi et al., 2011; Zhao et al., 2016). For these reasons, there is no doubt that network pharmacology is one of the efficacious strategies for discovering new drugs (Hopkins, 2008). This study discovered the potential therapeutic targets and pathways of colchicine through network pharmacology, and explored the mechanism of colchicine on MIRI. Our works provide new ideas for understanding the pharmacological mechanisms of drugs from the perspective of the network.
Colchicine has various biological activities such as anti-inflammatory, anti-hepatic fibrosis, and enhance the effects of sympathomimetic drugs and so on (Leung et al., 2015). The beneficial effects of colchicine on cardiovascular disease have been widely reported in recent studies (Roubille et al., 2014; Martinez et al., 2018; Akodad et al., 2020). Previous reports had shown that colchicine has a certain protective effect on ischemic myocardium, which can reduce the coronary plaque and the area of myocardial infarction in patients with myocardial infarction by inhibiting inflammation response (Nidorf et al., 2019; Nidorf et al., 2020). However, the potential regulatory targets and pharmacological mechanisms of colchicine in MIRI injury remain unclear. In this study, 227 overlapping genes related to colchicine were identified by connecting targets of colchicine with targets of disease. the PPI network analysis demonstrated that the targets linked to MIRI were ALB, TNF, ACTB, AKT1, IL6, TP53, IL1B, CASP3 and these targets showed nice affinity with colchicine in molecular docking experiments, we found that most of the hub targets are involved in external or internal apoptotic pathways. The results of GO analysis and KEGG pathway enrichment demonstrated that the anti-MIRI effect of colchicine involves in apoptotic signaling pathway and the PI3K/AKT signaling pathway was obtained through further screening in the apoptotic mechanism. Previous studies have extensively reported the beneficial effects of inhibition of cardiomyocyte apoptosis on MIRI (Tao et al., 2011; Konstantinidis et al., 2012). However, the effect of colchicine on cardiomyocyte apoptosis induced by MIRI has not been reported. Therefore, network pharmacology can help us understand the mechanism of colchicine in the treatment of MIRI from a general view and lays a good foundation for further discussion of the anti-MIRI pharmacological target and molecular mechanism of colchicine.
Based on KEGG pathway enrichment and the result of molecular docking in the core targets, we chose the key proteins of PI3K/AKT/eNOS signaling pathway and the apoptosis-related protein Caspase-3 for validation the mechanisms underlying the anti-MIRI effects of colchicine. The PI3K/Akt signal pathway can regulate the migration, proliferation, differentiation, apoptosis and metabolism of various types of cells, which is connected to cardiomyocyte apoptosis (Brand et al., 2019; Cui et al., 2020). PI3K is activated and generates phosphatidylinositol 3,4,5-trisphosphate, which induces the phosphorylation of AKT (p-AKT), p-AKT then activates endothelial nitric oxide synthase (eNOS) and produces NO, NO produced by eNOS activation has been shown to play an important role in the process of MIRI (Cohen et al., 2006; Ying et al., 2015). CASP3, as one of the most concerned proteins in caspase family, can catalyze the specific cleavage of a variety of key cell proteins, which is one of the most critical apoptotic protease in the process of apoptosis (Miura, 2011). Activation of caspase-3 is a common ultimate signal in response to factors that induce apoptosis, such as plays an important role in the apoptosis of cardiomyocytes (Gundapaneni et al., 2017).
Our present data provide ample evidence to support the protective role of colchicine in MIRI injury through reducing apoptosis. In this study, we found that colchicine could systematically and significantly inhibit the apoptosis cells from H/R injure. Additionally, LY294002 (PI3K inhibitor) significantly attenuated the effects of colchicine. Our results demonstrated that colchicine markedly enhanced the decreased levels of Caspase-3 in myocardial injure model induced by H/R. Then, Western blot results further confirmed that colchicine inhibited H/R induced cardiomyocyte apoptosis by regulating the PI3K/AKT/eNOS pathway. After PI3K/AKT/eNOS signal pathway was specifically blocked (LY294002 group), cardiomyocyte apoptosis induced by ischemia-reperfusion was aggravated. This further suggested that colchicine plays a protective role against MIRI through the apoptosis pathway. R. Singhal et al. found that the activation of PI3K/AKT/eNOS pathway induced by colchicine could reduce apoptosis, inflammation and fibrosis in heart failure cardiomyocytes, which may in part contribute to the improvement of cardiac function (Singhal et al., 2014). This result is consistent with the PI3K/AKT/eNOS signaling pathway and key targets related to apoptosis obtained in our study.
Conclusion
In summary, we performed network pharmacology and experimental evaluation to reveal the pharmacological mechanism of colchicine against MIRI. Moreover, a further in-vitro validation experiment illustrated that colchicine substantially inhibited apoptosis of myocardial cell via regulating PI3K/Akt/eNOS signaling pathway and inhibiting the expression of pro-apoptotic protein Caspase-3. Our findings may guide further pharmacological investigations on the therapeutic effects of colchicine on MIRI and the discovery of new drugs for MIRI treatment. However, this study still has some limitations. Network pharmacology approaches await further improvements, and it is necessary to conduct in vivo experimental study to verify the efficacy and mechanism of colchicine in the treatment of MIRI in our future research.
Data Availability Statement
The original contributions presented in the study are included in the article/Supplementary Material, further inquiries can be directed to the corresponding authors.
Author Contributions
YT and GF designed the study. YT, CS, YQ was responsible for data analysis and interpretation and drafting of the manuscript. SW, HP, MC and XY were involved in data acquisition and experimentation. YL was responsible for the data curation and the revision of the manuscript. GF was responsible for funding acquisition and project administration.
Funding
This work is financially supported by grants from the National Natural Science Foundation of China (81973289), the National Natural Science Foundation of China (81902395).
Conflict of Interest
The authors declare that the research was conducted in the absence of any commercial or financial relationships that could be construed as a potential conflict of interest.
Publisher’s Note
All claims expressed in this article are solely those of the authors and do not necessarily represent those of their affiliated organizations, or those of the publisher, the editors and the reviewers. Any product that may be evaluated in this article, or claim that may be made by its manufacturer, is not guaranteed or endorsed by the publisher.
Supplementary Material
The Supplementary Material for this article can be found online at: https://www.frontiersin.org/articles/10.3389/fphar.2021.804030/full#supplementary-material
References
Akodad, M., Sicard, P., Fauconnier, J., and Roubille, F. (2020). Colchicine and Myocardial Infarction: A Review. Arch. Cardiovasc. Dis. 113 (10), 652–659. doi:10.1016/j.acvd.2020.04.007
Amberger, J. S., Bocchini, C. A., Schiettecatte, F., Scott, A. F., and Hamosh, A. (2015). OMIM.org: Online Mendelian Inheritance in Man (OMIM®), an Online Catalog of Human Genes and Genetic Disorders. Nucleic Acids Res. 43, D789–D798. doi:10.1093/nar/gku1205
Bajaj, A., Sethi, A., Rathor, P., Suppogu, N., and Sethi, A. (2015). Acute Complications of Myocardial Infarction in the Current Era: Diagnosis and Management. J. Investig. Med. 63 (7), 844–855. doi:10.1097/JIM.0000000000000232
Barabási, A. L., Gulbahce, N., and Loscalzo, J. (2011). Network Medicine: a Network-Based Approach to Human Disease. Nat. Rev. Genet. 12 (1), 56–68. doi:10.1038/nrg2918
Bellis, A., Mauro, C., Barbato, E., Di Gioia, G., Sorriento, D., Trimarco, B., et al. (2020). The Rationale of Neprilysin Inhibition in Prevention of Myocardial Ischemia-Reperfusion Injury during ST-Elevation Myocardial Infarction. Cells 9 (9), 2134. doi:10.3390/cells9092134
Bouabdallaoui, N., Tardif, J. C., Waters, D. D., Pinto, F. J., Maggioni, A. P., Diaz, R., et al. (2020). Time-to-treatment Initiation of Colchicine and Cardiovascular Outcomes after Myocardial Infarction in the Colchicine Cardiovascular Outcomes Trial (COLCOT). Eur. Heart J. 41 (42), 4092–4099. doi:10.1093/eurheartj/ehaa659
Brand, C. S., Lighthouse, J. K., and Trembley, M. A. (2019). Protective Transcriptional Mechanisms in Cardiomyocytes and Cardiac Fibroblasts. J. Mol. Cel Cardiol 132, 1–12. doi:10.1016/j.yjmcc.2019.04.023
Buja, L. M. (2005). Myocardial Ischemia and Reperfusion Injury. Cardiovasc. Pathol. 14 (4), 170–175. doi:10.1016/j.carpath.2005.03.006
Castro-Dominguez, Y., Dharmarajan, K., and McNamara, R. L. (2018). Predicting Death after Acute Myocardial Infarction. Trends Cardiovasc. Med. 28 (2), 102–109. doi:10.1016/j.tcm.2017.07.011
Cohen, M. V., Yang, X. M., and Downey, J. M. (2006). Nitric Oxide Is a Preconditioning Mimetic and Cardioprotectant and Is the Basis of many Available Infarct-Sparing Strategies. Cardiovasc. Res. 70 (2), 231–239. doi:10.1016/j.cardiores.2005.10.021
Crittenden, D. B., Lehmann, R. A., Schneck, L., Keenan, R. T., Shah, B., Greenberg, J. D., et al. (2012). Colchicine Use Is Associated with Decreased Prevalence of Myocardial Infarction in Patients with Gout. J. Rheumatol. 39 (7), 1458–1464. doi:10.3899/jrheum.111533
Cui, Z. H., Zhang, X. J., Shang, H. Q., Wang, X., and Rong, D. (2020). Glutamine Protects Myocardial Ischemia-Reperfusion Injury in Rats through the PI3K/Akt Signaling Pathway. Eur. Rev. Med. Pharmacol. Sci. 24 (1), 444–451. doi:10.26355/eurrev_202001_19944
Daina, A., Michielin, O., and Zoete, V. (2019). SwissTargetPrediction: Updated Data and New Features for Efficient Prediction of Protein Targets of Small Molecules. Nucleic Acids Res. 47 (W1), W357–W364. doi:10.1093/nar/gkz382
Davis, A. P., Grondin, C. J., Johnson, R. J., Sciaky, D., McMorran, R., Wiegers, J., et al. (2019). The Comparative Toxicogenomics Database: Update 2019. Nucleic Acids Res. 47 (D1), D948–D954. doi:10.1093/nar/gky868
Deftereos, S., Giannopoulos, G., Angelidis, C., Alexopoulos, N., Filippatos, G., Papoutsidakis, N., et al. (2015). Anti-Inflammatory Treatment with Colchicine in Acute Myocardial Infarction: A Pilot Study. Circulation 132 (15), 1395–1403. doi:10.1161/CIRCULATIONAHA.115.017611
Downing, K. H. (2000). Structural Basis for the Interaction of Tubulin with Proteins and Drugs that Affect Microtubule Dynamics. Annu. Rev. Cel Dev Biol 16, 89–111. doi:10.1146/annurev.cellbio.16.1.89
Fishilevich, S., Zimmerman, S., Kohn, A., Iny Stein, T., Olender, T., Kolker, E., et al. (2016). Genic Insights from Integrated Human Proteomics in GeneCards. Database 2016, baw030. doi:10.1093/database/baw030
Fujiwara, M., Matoba, T., Koga, J. I., Okahara, A., Funamoto, D., Nakano, K., et al. (2019). Nanoparticle Incorporating Toll-like Receptor 4 Inhibitor Attenuates Myocardial Ischaemia-Reperfusion Injury by Inhibiting Monocyte-Mediated Inflammation in Mice. Cardiovasc. Res. 115 (7), 1244–1255. doi:10.1093/cvr/cvz066
Gao, H., Kiesewetter, D. O., Zhang, X., Huang, X., Guo, N., Lang, L., et al. (2012). PET of Glucagonlike Peptide Receptor Upregulation after Myocardial Ischemia or Reperfusion Injury. J. Nucl. Med. 53 (12), 1960–1968. doi:10.2967/jnumed.112.109413
Gulati, R., Behfar, A., Narula, J., Kanwar, A., Lerman, A., Cooper, L., et al. (2020). Acute Myocardial Infarction in Young Individuals. Mayo Clin. Proc. 95 (1), 136–156. doi:10.1016/j.mayocp.2019.05.001
Gundapaneni, K. K., Shyamala, N., Galimudi, R. K., Kupsal, K., Gantala, S. R., Padala, C., et al. (2017). Polymorphic Variants of Caspase Genes (8 & 3) in the Risk Prediction of Coronary Artery Disease. Gene 627, 278–283. doi:10.1016/j.gene.2017.06.035
Herr, D. J., Singh, T., Dhammu, T., and Menick, D. R. (2020). Regulation of Metabolism by Mitochondrial Enzyme Acetylation in Cardiac Ischemia-Reperfusion Injury. Biochim. Biophys. Acta Mol. Basis Dis. 1866 (6), 165728. doi:10.1016/j.bbadis.2020.165728
Hopkins, A. L. (2007). Network Pharmacology. Nat. Biotechnol. 25 (10), 1110–1111. doi:10.1038/nbt1007-1110
Hopkins, A. L. (2008). Network Pharmacology: the Next Paradigm in Drug Discovery. Nat. Chem. Biol. 4 (11), 682–690. doi:10.1038/nchembio.118
Huang, C., Zheng, C., Li, Y., Wang, Y., Lu, A., and Yang, L. (2014). Systems Pharmacology in Drug Discovery and Therapeutic Insight for Herbal Medicines. Brief Bioinform 15 (5), 710–733. doi:10.1093/bib/bbt035
Konstantinidis, K., Whelan, R. S., and Kitsis, R. N. (2012). Mechanisms of Cell Death in Heart Disease. Arterioscler Thromb. Vasc. Biol. 32 (7), 1552–1562. doi:10.1161/ATVBAHA.111.224915
Leung, Y. Y., Yao Hui, L. L., and Kraus, V. B. (2015). Colchicine--Update on Mechanisms of Action and Therapeutic Uses. Semin. Arthritis Rheum. 45 (3), 341–350. doi:10.1016/j.semarthrit.2015.06.013
Liu, Z., Guo, F., Wang, Y., Li, C., Zhang, X., Li, H., et al. (2016). BATMAN-TCM: a Bioinformatics Analysis Tool for Molecular mechANism of Traditional Chinese Medicine. Sci. Rep. 6, 21146. doi:10.1038/srep21146
Marchant, D. J., Boyd, J. H., Lin, D. C., Granville, D. J., Garmaroudi, F. S., and McManus, B. M. (2012). Inflammation in Myocardial Diseases. Circ. Res. 110 (1), 126–144. doi:10.1161/CIRCRESAHA.111.243170
Martínez, G. J., Celermajer, D. S., and Patel, S. (2018). The NLRP3 Inflammasome and the Emerging Role of Colchicine to Inhibit Atherosclerosis-Associated Inflammation. Atherosclerosis 269, 262–271. doi:10.1016/j.atherosclerosis.2017.12.027
Miura, M. (2011). Apoptotic and Non-apoptotic Caspase Functions in Neural Development. Neurochem. Res. 36 (7), 1253–1260. doi:10.1007/s11064-010-0341-x
Münzel, T., Camici, G. G., Maack, C., Bonetti, N. R., Fuster, V., and Kovacic, J. C. (2017). Impact of Oxidative Stress on the Heart and Vasculature: Part 2 of a 3-Part Series. J. Am. Coll. Cardiol. 70 (2), 212–229. doi:10.1016/j.jacc.2017.05.035
Nagai, T., Anzai, T., Kaneko, H., Anzai, A., Mano, Y., Nagatomo, Y., et al. (2010). Impact of Systemic Acidosis on the Development of Malignant Ventricular Arrhythmias after Reperfusion Therapy for ST-Elevation Myocardial Infarction. Circ. J. 74 (9), 1808–1814. doi:10.1253/circj.cj-10-0229
Nidorf, S. M., Fiolet, A. T. L., Eikelboom, J. W., Schut, A., Opstal, T. S. J., Bax, W. A., et al. (2019). The Effect of Low-Dose Colchicine in Patients with Stable Coronary Artery Disease: The LoDoCo2 Trial Rationale, Design, and Baseline Characteristics. Am. Heart J. 218, 46–56. doi:10.1016/j.ahj.2019.09.011
Nidorf, S. M., Fiolet, A. T. L., Mosterd, A., Eikelboom, J. W., Schut, A., Opstal, T. S. J., et al. (2020). Colchicine in Patients with Chronic Coronary Disease. N. Engl. J. Med. 383 (19), 1838–1847. doi:10.1056/NEJMoa2021372
Roubille, F., Busseuil, D., Merlet, N., Kritikou, E. A., Rhéaume, E., and Tardif, J. C. (2014). Investigational Drugs Targeting Cardiac Fibrosis. Expert Rev. Cardiovasc. Ther. 12 (1), 111–125. doi:10.1586/14779072.2013.839942
Roubille, F., Kritikou, E., Busseuil, D., Barrere-Lemaire, S., and Tardif, J. C. (2013). Colchicine: an Old Wine in a New Bottle? Antiinflamm Antiallergy Agents Med. Chem. 12 (1), 14–23. doi:10.2174/1871523011312010004
Shannon, P., Markiel, A., Ozier, O., Baliga, N. S., Wang, J. T., Ramage, D., et al. (2003). Cytoscape: a Software Environment for Integrated Models of Biomolecular Interaction Networks. Genome Res. 13 (11), 2498–2504. doi:10.1101/gr.1239303
Singhal, R., Chang, S. L., Chong, E., Hsiao, Y. W., Liu, S. H., Tsai, Y. N., et al. (2014). Colchicine Suppresses Atrial Fibrillation in Failing Heart. Int. J. Cardiol. 176 (3), 651–660. doi:10.1016/j.ijcard.2014.07.069
Szklarczyk, D., Morris, J. H., Cook, H., Kuhn, M., Wyder, S., Simonovic, M., et al. (2017). The STRING Database in 2017: Quality-Controlled Protein-Protein Association Networks, Made Broadly Accessible. Nucleic Acids Res. 45 (D1), D362–D368. doi:10.1093/nar/gkw937
Tao, J., Zhu, W., Li, Y., Xin, P., Li, J., Liu, M., et al. (2011). Apelin-13 Protects the Heart against Ischemia-Reperfusion Injury through Inhibition of ER-dependent Apoptotic Pathways in a Time-dependent Fashion. Am. J. Physiol. Heart Circ. Physiol. 301 (4), H1471–H1486. doi:10.1152/ajpheart.00097.2011
Tardif, J. C., Kouz, S., Waters, D. D., Bertrand, O. F., Diaz, R., Maggioni, A. P., et al. (2019). Efficacy and Safety of Low-Dose Colchicine after Myocardial Infarction. N. Engl. J. Med. 381 (26), 2497–2505. doi:10.1056/NEJMoa1912388
Terkeltaub, R. A. (2009). Colchicine Update: 2008. Semin. Arthritis Rheum. 38 (6), 411–419. doi:10.1016/j.semarthrit.2008.08.006
Toldo, S., and Abbate, A. (2018). The NLRP3 Inflammasome in Acute Myocardial Infarction. Nat. Rev. Cardiol. 15 (4), 203–214. doi:10.1038/nrcardio.2017.161
Trott, O., and Olson, A. J. (2010). AutoDock Vina: Improving the Speed and Accuracy of Docking with a New Scoring Function, Efficient Optimization, and Multithreading. J. Comput. Chem. 31 (2), 455–461. doi:10.1002/jcc.21334
UniProt, C. (2019). UniProt: a Worldwide Hub of Protein Knowledge. Nucleic Acids Res. 47 (D1), D506–D515. doi:10.1093/nar/gky1049
Walters, A. M., Porter, G. A., and Brookes, P. S. (2012). Mitochondria as a Drug Target in Ischemic Heart Disease and Cardiomyopathy. Circ. Res. 111 (9), 1222–1236. doi:10.1161/CIRCRESAHA.112.265660
Wang, X., Shen, Y., Wang, S., Li, S., Zhang, W., Liu, X., et al. (2017). PharmMapper 2017 Update: a Web Server for Potential Drug Target Identification with a Comprehensive Target Pharmacophore Database. Nucleic Acids Res. 45 (W1), W356–W360. doi:10.1093/nar/gkx374
Wang, Y., Zhang, S., Li, F., Zhou, Y., Zhang, Y., Wang, Z., et al. (2020). Therapeutic Target Database 2020: Enriched Resource for Facilitating Research and Early Development of Targeted Therapeutics. Nucleic Acids Res. 48 (D1), D1031–D1041. doi:10.1093/nar/gkz981
Wu, M. Y., Yiang, G. T., Liao, W. T., Tsai, A. P., Cheng, Y. L., Cheng, P. W., et al. (2018). Current Mechanistic Concepts in Ischemia and Reperfusion Injury. Cell Physiol Biochem 46 (4), 1650–1667. doi:10.1159/000489241
Yao, B., Wan, X., Zheng, X., Zhong, T., Hu, J., Zhou, Y., et al. (2020). Critical Roles of microRNA-141-3p and CHD8 in Hypoxia/reoxygenation-Induced Cardiomyocyte Apoptosis. Cell Biosci 10, 20. doi:10.1186/s13578-020-00384-5
Ying, Z., Xie, X., Chen, M., Yi, K., and Rajagopalan, S. (2015). Alpha-lipoic Acid Activates eNOS through Activation of PI3-kinase/Akt Signaling Pathway. Vascul Pharmacol. 64, 28–35. doi:10.1016/j.vph.2014.11.004
Zhang, X., Huang, L. F., Hua, L., Feng, H. K., and Shen, B. (2019). Resveratrol Protects Myocardial Apoptosis Induced by Ischemia-Reperfusion in Rats with Acute Myocardial Infarction via Blocking P13K/Akt/e-NOS Pathway. Eur. Rev. Med. Pharmacol. Sci. 23 (4), 1789–1796. doi:10.26355/eurrev_201902_17142
Zhang, Y., Wang, Z., and Wang, Y. (2017). Multi-hierarchical Profiling: an Emerging and Quantitative Approach to Characterizing Diverse Biological Networks. Brief Bioinform 18 (1), 57–68. doi:10.1093/bib/bbv112
Zhao, Z., Zhang, Y., Gai, F., and Wang, Y. (2016). Confirming an Integrated Pathology of Diabetes and its Complications by Molecular Biomarker-Target Network Analysis. Mol. Med. Rep. 14 (3), 2213–2221. doi:10.3892/mmr.2016.5478
Keywords: colchicine, myocardial ischemia reperfusion injury, network pharmacology, experimental verification, apoptosis
Citation: Tang Y, Shi C, Qin Y, Wang S, Pan H, Chen M, Yu X, Lou Y and Fan G (2021) Network Pharmacology-Based Investigation and Experimental Exploration of the Antiapoptotic Mechanism of Colchicine on Myocardial Ischemia Reperfusion Injury. Front. Pharmacol. 12:804030. doi: 10.3389/fphar.2021.804030
Received: 28 October 2021; Accepted: 29 November 2021;
Published: 16 December 2021.
Edited by:
Hong Zhang, Shanghai University of Traditional Chinese Medicine, ChinaReviewed by:
Zhang Yuefan, Shanghai University, ChinaJianguo Xing, Xinjiang Institute of Materia Medica, China
Copyright © 2021 Tang, Shi, Qin, Wang, Pan, Chen, Yu, Lou and Fan. This is an open-access article distributed under the terms of the Creative Commons Attribution License (CC BY). The use, distribution or reproduction in other forums is permitted, provided the original author(s) and the copyright owner(s) are credited and that the original publication in this journal is cited, in accordance with accepted academic practice. No use, distribution or reproduction is permitted which does not comply with these terms.
*Correspondence: Yuefen Lou, bG91eXVlZmVuQHNpbmEuY24=; Guorong Fan, Z3VvcmZhbkAxNjMuY29t
†These authors have contributed equally to this work and share first authorship