- 1Center of Clinical Pharmacology, The Third Xiangya Hospital, Central South University, Changsha, China
- 2Department of Pharmacy, The Third Xiangya Hospital, Central South University, Changsha, China
- 3Hunan Qianjin Xiangjiang Pharmaceutical Co., Ltd, Zhuzhou, China
- 4Department of Pharmacy, Peking University First Hospital, Beijing, China
Background: Ticagrelor belongs to a new class of P2Y12 receptor inhibitor that has been widely used for antiplatelet therapy. This study aimed to explore the effect of single nucleotide polymorphisms (SNPs) in metabolic enzymes, transporters, and other relevant variants on the pharmacokinetics (PK) of ticagrelor and its active metabolite, AR-C124910XX.
Methods: The study population comprised 68 healthy Chinese volunteers who were enrolled in a ticagrelor bioequivalence clinical trial. The PK profile of ticagrelor was evaluated after orally administering a single 90-mg dose of ticagrelor in tablet form. The plasma concentrations of ticagrelor and AR-C124910XX were determined through liquid chromatography–tandem mass spectrometry. Plasma DNA samples were used to explore the effect of gene polymorphisms on the PK of ticagrelor and AR-C124910XX with whole-exome sequencing.
Results: Female participants had a higher maximum plasma concentration/weight ratio (Cmax/W; p < 0.001) and a shorter half-life (T1/2; p < 0.05) for ticagrelor than their male counterparts. In addition, a higher area under the curve/weight ratio (AUC/W; p < 0.001), and longer T1/2 (p < 0.001) and time to reach the maximum plasma concentration (Tmax; p < 0.001), as well as a lower apparent drug clearance (CL/F; p < 0.001), were observed among healthy volunteers in the fed trial compared to those enrolled in the fasting trial. For AR-C124910XX, higher Cmax/W (p < 0.001) and AUC/W (p < 0.001) but lower CL/F (p < 0.001) and apparent volume of distribution (Vd/F; p < 0.001) were observed among female participants. Healthy volunteers enrolled in the fasting trial exhibited higher Cmax/W (p < 0.001) and AUC/W (p < 0.01), shorter Tmax (p < 0.001), and lower CL/F (p < 0.001) and Vd/F (p < 0.001) than those enrolled in the fed trial. Upon confirmation through multivariate analysis, the CYP4F2 rs2074900 A/A carriers were associated with higher Cmax/W and AUC/W and lower CL/F and Vd/F than the CYP4F2 rs2074900 A/G and G/G carriers.
Conclusion: This study is the first to show that the CYP4F2 rs2074900 SNP had a remarkable effect on ticagrelor PK, which is significant since it adds to the limited pharmacogenetic information on ticagrelor.
Introduction
Cardiovascular diseases are the leading cause of mortality worldwide, and up to 30% of patients die of acute coronary events every year (Rodrigues et al., 2020). Percutaneous coronary intervention is a first-line therapy for patients with acute coronary syndrome (ACS). Guidelines of the American College of Cardiology/American Heart Association recommend the administration of dual antiplatelet therapy (aspirin + a platelet P2Y12 inhibitor) to prevent atherothrombotic complications for 12 months (Capodanno et al., 2018). Ticagrelor is one of the most common P2Y12 inhibitors, which was licensed in 2010 by AstraZeneca (Cambridge, UK). In the PLATO (PLATelet Inhibition and Patients Outcome trial) trial, ticagrelor reduced the total number of cardiovascular events, including initial and subsequent recurrent cardiovascular events (Kohli et al., 2013). In addition, the TICO (Ticagrelor Monotherapy After 3 Months in the Patients Treated With New Generation Sirolimus-Eluting Stent for Acute Coronary Syndrome) clinical trial reported that ticagrelor monotherapy after 3 months of dual antiplatelet therapy significantly reduced the incidence of composite outcomes of major bleeding and cardiovascular events at 12 months for patients with ACS treated with drug-eluting stents (Kim et al., 2019; Kim et al., 2020). The guidelines of the European Society of Cardiology have recommended the administration of ticagrelor therapy for patients at moderate to high ischemic risk with a class of recommendation Ι, level of evidence B (Rodriguez and Mahaffey, 2016).
Unlike clopidogrel, a prodrug that is converted to the active metabolite by hepatic cytochrome P450 enzyme CYP2C19, ticagrelor is an adenine nucleoside analogue that can reversibly target the P2Y12 receptor and block adenosine diphosphate (ADP)-mediated platelet aggregation (Wernly et al., 2020). In addition, it has been reported that the carriers of CYP2C19 loss-of-function alleles are at a higher risk of ischemic events than are non-carriers for clopidogrel (Pereira et al., 2020). However, the genotype of CYP2C19 has no effect on the pharmacokinetics (PK) and the curative effect of ticagrelor (Wallentin et al., 2010). CYP3A4 and CYP3A5 enzymes play a significant role in ticagrelor metabolism, but information regarding the effects of the genetic polymorphisms of metabolic enzymes and transporters on the PK of ticagrelor and its active metabolite, AR-C124910XX, is limited. Data from a clinical trial further corroborated the finding that the CYP3A4*22 allele impaired the elimination and increased the exposure of ticagrelor in healthy Finnish volunteers of Caucasian ethnicity (Holmberg et al., 2019). Furthermore, the CYP3A4*1G allele also increased the clearance of its active metabolite, AR-C124910XX, and had no effect on the PK of ticagrelor in healthy Chinese volunteers (Liu et al., 2018). Moreover, genetic variants of SLCO1B1 rs113681054, SLCO1B1*5 (rs4149056), and CYP3A5*3 (rs776746) were also reported to have no relevance in the PK of ticagrelor in healthy Chinese volunteers (Li et al., 2017a). Platelet endothelial aggregation receptor (PEAR1; location: 1q23.1) plays a vital role in platelet adhesion and platelet aggregation via sustaining αIIbβ3 activation (Duconge et al., 2021). Prior studies have confirmed that PEAR1 triggers PI3K/AKT/PTEN signaling, which leads to megakaryopoiesis and neoangiogenesis (Kauskot et al., 2013). Of note is that PEAR1 polymorphisms attenuate the effect of antiplatelet drugs such as aspirin, clopidogrel, prasugrel, and ticagrelor (Würtz et al., 2014; Li et al., 2017b; Yao et al., 2018; Ansari et al., 2021). Alteration of drug efficacy associated with genetic variants may result from variability in gene-oriented PK; thus, it is essential to explore the relationship between PEAR1 polymorphisms and ticagrelor PK.
Since genetic data on the PK profile of ticagrelor are inconsistent and incomprehensive at present, we performed whole-exome sequencing using DNA samples from 68 healthy Chinese volunteers to determine all gene variants potentially related to the PK of ticagrelor and AR-C124910XX. We expect our results to provide a more comprehensive understanding of the relationship between single nucleotide polymorphisms (SNPs) and ticagrelor PK and offer a reference for clinical individual antiplatelet therapy.
Patients and Methods
Study Population
The study population consisted of 68 healthy Chinese volunteers enrolled in a ticagrelor bioequivalence clinical trial. This study complied with the Good Clinical Practice guidelines and the tenets of the Declaration of Helsinki and was approved by the Institutional Review Board of The Third Xiangya Hospital of Central South University (IRB #18031). All participants provided informed consent for participation in the clinical trial and the pharmacogenetic study. The inclusion criteria were: non-smoking healthy men or women, aged 18–55 years, and having a body mass index (BMI) between 19 and 26 kg/m2. In addition, no clinically relevant physical abnormalities were detected in each subject, as revealed through their medical records and detailed physical examination (vital signs, laboratory analysis, and electrocardiography). The exclusion criteria were as follows: a history of drug abuse 1 year prior to the trial, having used any drugs or health products including herbs in the past 2 weeks, having participated in any clinical trial or having used any investigational drug within 3 months, having a history of blood donation or blood loss volume of >200 ml or having accepted transfusion of blood products within 3 months, being pregnant or lactating, and having consumed grapefruit in the previous 48 h.
Study Design
The ticagrelor bioequivalence study included fasting and fed trials. Each trial was a phase I, randomized, open-label, single-center, single-dose, two-product, two-period crossover design to compare the PK bioequivalence of biosimilar drugs and its reference biopharmaceutical Brilinta®. In each period, subjects received a single 90-mg oral dose of a ticagrelor tablet, either the reference or test formulation, after overnight fasting of at least 10 h. The washout period was 7 days. Volunteers took ticagrelor on an empty stomach and fasted for another 4 h in the fasting trial. In contrast, a high-fat meal was consumed within 30 min prior to drug dosing in the fed trial. Furthermore, they were forbidden to carry out vigorous activity and ate unified lunch and dinner after 4 and 10 h of the dose.
Sample Collection and Bioanalysis
Blood samples for PK evaluation were harvested from peripheral veins at 20 min (fasting trial) and 30 min (fed trial) before dosing and at 20 min (fasting trial)/0.5 h (fed trial), at 40 min (fasting trial), at 1 h, 80 min, 100 min, 2 h, 140 min, 160 min, and 3, 3.5, 4, and 4.5 h (fed trial), and at 5, 6, 8, 12, 24, 36, and 48 h after dosing. Approximately 4 ml blood sample was collected in heparinized vacutainer tubes and centrifuged at 2–8°C for 10 min at 1,500 × g within 1 h after sample collection. Plasma samples were vertically stored at −80°C until the plasma concentration of ticagrelor was measured using validated liquid chromatography–tandem mass spectrometry. Human plasma samples were precipitated with acetonitrile (ACN) using ticagrelor-D7 and AR-C124910XX-D7 as internal standards. To be more specific, 40.0 μl of standard curve samples, quality control samples, unknown samples, and blank matrix was transferred into a 96-well polypropylene plate, and 20.0 μl of internal standard working fluid (250 ng/ml in 50% ACN) was added to all samples in addition to the blank sample, in which a 50% ACN solution of 20.0 μl was added. Subsequently, 340 μl of ACN was added to all samples. Separation was carried out on a Waters ACQUITY UPLC HSS T3 (2.1×100 mm, 1.8 μm) column with a mobile phase of NH4Ac-ACN (including 0.1% formic acid) at a flow rate of 0.6 ml/min. Electrospray ionization (ESI) was performed in multiple reaction monitoring (MRM) negative ion mode using target ions at a mass-to-charge ratio (m/z) of 521.3→m/z 361.2 (ticagrelor) and m/z 477.3→m/z 361.2 (AR-C124910XX). The calibration curves of ticagrelor and AR-C124910XX were obtained in ranges of ∼5.00–2000.00 and ∼2.50–1,000.00 ng/ml with good linearity (R2 > 0.99) and an intra- and inter-assay variability of <15%. The lower and upper limits of quantification were 5.00 and 2,000.00 ng/ml for ticagrelor and 2.50 and 1,000 ng/ml for AR-C124910XX, respectively. The accuracy and the intra- and inter-day precision of the different quality control samples were adequate for the method and are indicated in Supplementary Table S1.
PK Analysis
PK parameters were calculated using a non-compartmental approach with Phoenix® WinNonlin 8.2 (Cetara USA, Inc., Princeton, NJ, USA). The maximum plasma concentration (Cmax) and the time to reach the maximum plasma concentration (Tmax) were both obtained directly from the concentration–time curves. Linear trapezoidal integration was used to calculate the area under the curve (AUC) between the predose (t = 0 h) and the final (t = 48 h) time points, namely, AUC0–t. The remaining AUC from 48 h to infinity (AUCt–∞) was obtained by dividing the last measured concentration (Ct) by the constant of elimination (ke). The terminal rate constant (ke) was calculated by linear regression of the log-linear part of the concentration–time curve. The AUC between 0 and ∞ (AUC0–∞) was calculated as AUC0–t + AUCt–∞. Apparent drug clearance (CL/F) was calculated as the dose divided by the AUC0–∞ and weight (W). Similarly, the apparent volume of distribution (Vd/F) was calculated as CL/F divided by ke. Half-life (T1/2) was estimated to be 0.693/ke.
DNA Extraction and Whole-Exome Sequencing
Genomic DNA was extracted using a Wizard® Genomic DNA Purification Kit (Promega, Madison, WI, USA) in accordance with the manufacturer’s instructions. In total, qualified genomic DNA (3 μg) from each sample was considered for initial whole-exome sequencing, from which the genomic regions of interest were captured using the Agilent SureSelectXT Target Enrichment Kit for Illumina multiplex sequencing at Capital Bio Technology Co., Ltd (Beijing, China). Ultimately, a total of 35 variants in 10 genes were included on the basis of the identified SNPs of metabolic enzymes (CYP/UGT enzymes), transporters (ABCB1or SLCO1B1), and other relevant variants (PEAR1). The included variants were the following: CYP4F2 rs2074900; CYP2C9 rs17847029, rs1057911, and rs9332245; CYP2B6 rs2279342, rs35930845, rs3745276, rs434606, and rs56156262; CYP2C19*2 (rs4244285), rs12769205, and rs3758580; CYP3A4*18 (rs28371759); CYP3A5 rs15524, rs4646453, and rs6977165; UGT2B7 rs141257984, rs28365062, rs7438284, rs5013211, and rs4257713; PEAR1 rs77235035, rs735953, and rs189160314; SLCO1B1 rs4149032, rs4149034, rs2306283, rs4149057, rs2291075, and rs2291076; and ABCB1 rs2235048, rs2235047, rs1045642, rs2235013, and rs2235033.
Statistical Analysis
R (version 4.0.2) was used for statistical analysis. The variables AUC and Cmax were divided by the weight (AUC/W and Cmax/W, respectively) to correct the impact of weight. All PK parameters were log-transformed to normalize distributions. Initially, a univariate analysis was performed, where the mean of the PK parameters was compared in accordance with categorical variables (e.g., sex, food effect, and SNPs). A t-test (two groups) or an analysis of variance (ANOVA) followed by post-hoc Bonferroni correction (more than two groups) was conducted to evaluate the associations between the categorical variables and PK parameters. A p-value <0.05 was considered significant. Afterward, multivariate analysis was performed using the multiple linear regression model. As dependent variables, all PK parameters were analyzed; as independent variables, only variables with p < 0.05 in the univariate analysis were included. Bonferroni correction for multiple comparisons was performed. The significant p-value of <0.05 was divided by the number of variables introduced in multivariate analysis. All genotyped variants were analyzed using the chi-square test with the HardyWeinberg package to confirm whether the gene distribution complied with the Hardy–Weinberg equilibrium.
Results
Demographic Characteristics
The study population (N = 68) comprised 45 (66%) men and 23 (34%) women. Women had significantly lower weight and height (p < 0.0001) compared with men, with similar age and BMI (Table 1). In addition, the age, weight, height, and BMI were comparable between the fasting and fed trials.
Pharmacokinetics
For ticagrelor, a significantly higher Cmax/W (p < 0.001) and a shorter T1/2 (p < 0.05) were observed among women than among men (Table 2). In addition, healthy volunteers enrolled in the fed trial had higher values of AUC/W (p < 0.001), T1/2 (p < 0.001), and Tmax (p < 0.001), as well as lower CL/F (p < 0.001), than those enrolled in the fasting trial. For AR-C124910XX, the Cmax/W (p < 0.001) and AUC/W (p < 0.001) values were higher and the CL/F (p < 0.001) and Vd/F (p < 0.001) values were lower in women than in men. Moreover, higher Cmax/W (p < 0.001) and AUC/W (p < 0.01) and a shorter Tmax (p < 0.001) and lower CL/F (p < 0.001) and Vd/F (p < 0.001) were observed in healthy volunteers enrolled in the fasting trial than those enrolled in the fed trial.
All included SNPs were in Hardy–Weinberg equilibrium. Genetic polymorphism was associated with ticagrelor pharmacokinetic variability. Table 3 shows the associations between SNPs and ticagrelor PK with statistical significance. On univariate analysis, the Cmax/W (p < 0.05) and AUC/W (p < 0.05) were higher and the CL/F (p < 0.05) and Vd/F (p < 0.05) were lower among CYP4F2 rs2074900 A/A carriers after post-hoc Bonferroni correction than in CYP4F2 rs2074900 A/G and G/G carriers. On multivariate analysis, since sex and food intake were significantly related to PK variability, they were included to rule out the impact of confounding factors. The level of significance was set at p = 0.0167 (p < 0.05 divided by 3, the number of variables introduced in the multivariate analysis). After confirmation through multivariate analysis, the Cmax/W and AUC/W values were lower and the CL/F and Vd/F values were higher among CYP4F2 rs2074900 A/G and G/G carriers than among CYP4F2 rs2074900 A/A carriers (unstandardized β coefficients = −0.182, −0.168, 0.150, and 0.115; p < 0.01 for all; R2 = 0.27, 0.296, 0.269, and 0.139). Moreover, the AUC/W values were lower among PEAR1 rs77235035 A/A carriers than among A/C carriers (PANOVA < 0.05), but not among C/C carriers; hence, this SNP was excluded from multivariate analysis (Table 3). Regarding AR-C124910X, no associations were observed between SNPs and their PK parameters. Non-significant associations between SNPs and ticagrelor/AR-C124910XX PK are shown in Tables 4 and 5.
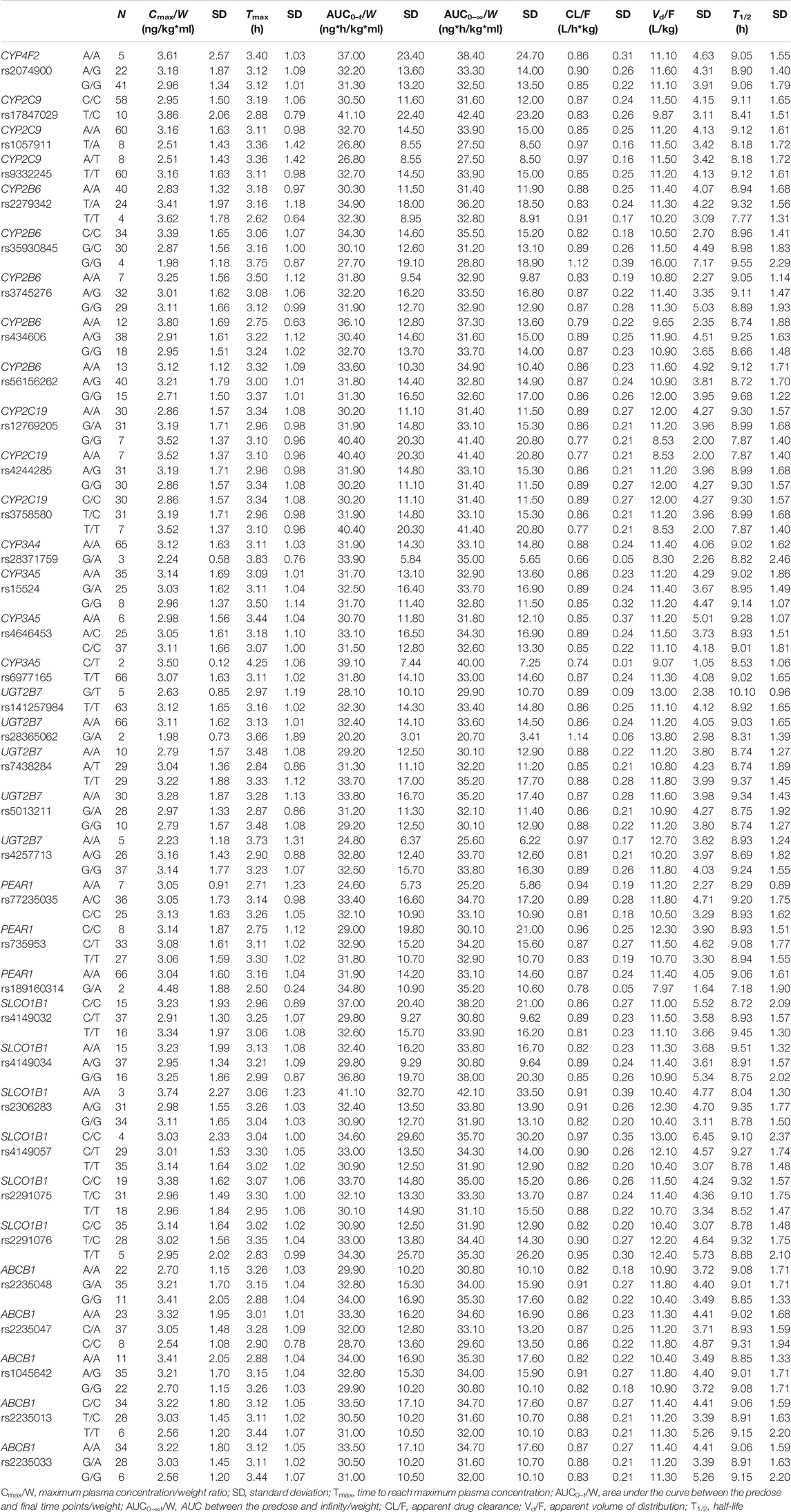
TABLE 5. AR-C124910XX pharmacokinetic parameters based on genotypes without statistical significance
Discussion
Ticagrelor has been considered a new P2Y12 inhibitor and has reportedly overcome clopidogrel resistance. Although ticagrelor non-responders only account for 3% of patients with ACS, the pathological consequences can be devastating (Thomas and Storey, 2016; Laurent et al., 2021). Currently, the US Food and Drug Administration (FDA) reported that ticagrelor does not need dose adjustment for different races. However, owing to the East Asian paradox, East Asian patients with ACS are at a higher risk of major bleeding than ischemic risk compared to Caucasians; hence, a unique antiplatelet strategy needs to be urgently established for East Asian individuals on the basis of reliable clinical and experimental evidence (Kim et al., 2021). This study investigated the impact of gene polymorphisms on the PK of ticagrelor, which could facilitate personalized antiplatelet therapy in East Asian patients with ACS. To the best of our knowledge, this study included the largest number of SNPs that may have an effect on the PK of ticagrelor, as revealed through whole-exome sequencing among healthy Chinese volunteers. Our study is the first to show that CYP4F2 rs2074900 was significantly associated with the PK of ticagrelor.
In this study, women were more likely to exhibit higher values of AUC/W and Cmax/W than men, a result similar to that previously reported for healthy volunteers (Teng et al., 2012). A possible explanation for the increased ticagrelor and AR-C124910XX exposure may be the gender-related physical difference. Since renal clearance plays a minor role in ticagrelor excretion, gender-related differences in renal function are unlikely to be associated with the PK of ticagrelor and AR-C124910XX (Teng et al., 2012). Another reason could be the gender-specific difference in CYP3A activity. Endogenous sex hormones reportedly affect CYP3A activity (Yoon et al., 2021). Ticagrelor is also a P-glycoprotein substrate. A lower P-glycoprotein activity in women than in men could result in a decreased biliary excretion of ticagrelor/AR-C124910XX, leading to a concomitant increase in the blood levels (Wang et al., 2021). However, dose adjustment is considered unnecessary on the basis of gender (Teng, 2015).
Furthermore, food showed considerable effects on the PK of ticagrelor in our study. On consuming a standard high-fat breakfast, the AUC/W values were found to be higher than those in the fasting condition, which was consistent with the FDA guidelines and with the results of a previous study (Wang et al., 2021). The FDA reported that a high-fat meal could result in a 21% increase in AUC. Nonetheless, the FDA suggests that ticagrelor can be taken with or without food, in accordance with the label. A possible explanation was that food had a minimal clinically significant effect, and this difference did not translate into changes in the pharmacodynamic (PD) profile of ticagrelor (Schilling et al., 2020).
Interestingly, we found that CYP4F2 rs2074900 A/A carriers had higher Cmax/W and AUC/W values and lower CL/F and Vd/F values than A/G and G/G carriers, implying that A/A carriers may experience a better therapeutic effect or unexpected bleeding events. CYP4F2 belongs to the CYP4 subfamily of enzymes and is primarily expressed in the human liver and kidney. CYP4F2 catalyzes the ω-hydroxylation of arachidonic acid to 20-HETE, which is a potent vasoconstrictor (Hirani et al., 2008; Zordoky and El-Kadi, 2010). In patients with ACS, CYP4F2 polymorphisms have significantly affected antiplatelet therapy, and CYP4F2 rs3093135 T/T carriers reportedly had a higher antiplatelet effect of ticagrelor than A/A and A/T carriers (Kupstyte et al., 2015; Tatarunas et al., 2020). The rs2074900 G>A mutation frequency is up to 22% in the East Asian population, as reported in the PharmGKB database (https://www.pharmgkb.org/page/dpwg), which is concurrent with our results; hence, it is worth further exploring whether CYP4F2 rs2074900 polymorphisms impact the PK and PD of ticagrelor in patients with ACS.
Previous studies have suggested that PEAR1 rs12041331 A/A homozygote carriers showed significantly increased inhibition of platelet aggregation (IPA) than did G allele carriers (Li et al., 2017b; Alhazzani et al., 2021). In general, there is a close affinity between the SNPs of PEAR1 and platelet function. Furthermore, the PEAR1 SNPs also influence the prognosis of patients with ACS (Stimpfle et al., 2018). The PEAR1 rs77235035 C>A mutation frequency is 35.52% in the Asian population, according to the PharmGKB database, and this is the first study to report the effect of PEAR1 variants on the PK of ticagrelor. In this study, the PEAR1 rs77235035 A/A carriers exhibited lower AUC/W values than did A/C carriers, but not the C/C carriers. Thus, this finding could be spurious. We highly recommend verifying the association between PEAR1 SNPs and ticagrelor in a large sample size.
CYP3A4 and CYP3A5 are the primary enzymes that convert ticagrelor to AC-R124910XX, the former playing a dominant role in the process. Three studies have reported the effect of CYP3A on the PK of ticagrelor in healthy volunteers. A previous study reported that CYP3A4*1G allele carriers had greater AR-C124910XX AUC0–t, AUC0–∞, and Cmax and longer T1/2 than *1*1 carriers in healthy Chinese subjects (Liu et al., 2017). Nevertheless, another study reported that CYP3A4*1G was not related to the PK of ticagrelor and AR-C123410XX (Li et al., 2017a). Moreover, in Caucasian individuals, CYP3A4*22 carriers showed a higher exposure of ticagrelor than did non-carriers (Holmberg et al., 2019). In our study, the rs28371759 SNP was the only identified variant of CYP3A4. Nevertheless, the CYP3A4*18 (rs28371759) variant was not associated with ticagrelor and AR-C124910XX PK. Most studies primarily explored the potential interactions between ticagrelor and CYP3A4 inducers or inhibitors. Hence, further studies are required to investigate the effect of CYP3A4/5 polymorphisms on the PK of ticagrelor.
CYP2C19 is a key clopidogrel-metabolizing enzyme, and there is a strong association between CYP2P19*2 polymorphism and clopidogrel resistance in patients with coronary heart disease (CHD) (Sun et al., 2020). CYP2C19*2 and CYP2C19∗3 appeared to be the most common alleles in the Chinese population. A previous study reported that CYP2C19*3 G/G carriers had a higher Tmax than G/A carriers after a single dose of ticagrelor (Zhu et al., 2019). In our study, CYP2C19*2 had no effect on the PK of ticagrelor. Ticagrelor is a putative P-glycoprotein substrate that could be encoded by ABCB1. As an efflux transporter, P-glycoprotein significantly affects drug disposition since it is expressed in intestinal mucosal epithelial cells, hepatocytes, and capillary endothelial cells (Zhu et al., 2019; Ji et al., 2021). It is reasonable to speculate that ABCB1 polymorphisms are associated with the plasma exposure of ticagrelor. Nevertheless, concurrent with a previous study, the observed ABCB1 variants, such as rs2235048, rs2235047, rs1045642, rs2235013, and rs2235033, were not associated with the PK of ticagrelor (Teng, 2015). In addition, the remaining CYP2C9, CYP2B6, UGT2B7, and SLCO1B1 polymorphisms had no effect on the PK of ticagrelor and its metabolite AR-C124910XX, which is consistent with previous findings (Zhou et al., 2011; Zhu et al., 2019).
Limitations
There are still some limitations in our study. Firstly, the PD of ticagrelor was not evaluated, especially platelet aggregation; hence, the identified SNPs that affected the PK of ticagrelor in this study remain to be further confirmed in patients with ACS. Secondly, the sample size was not large enough to identify all gene variants associated with the PK of ticagrelor. However, our study included the largest number of candidate SNPs for the analysis of their relationship with the PK of ticagrelor. In addition, as the bioequivalence study design excluded confounding factors, the results are relatively reliable.
Conclusion
This study included the largest number of candidate SNPs in analyzing the association between gene variants and the PK of ticagrelor and AR-C124910XX using whole-exome sequencing. This study is the first to report that CYP4F2 rs2074900 has a remarkable impact on ticagrelor PK variability. In addition, sex and food also impacted the PK profiles of ticagrelor and AR-C124910XX. Further studies are warranted to confirm our findings.
Data Availability Statement
The data presented in the study are deposited in the Sequences Read Archive database at the NCBI under accession number PRJNA798857.
Ethics Statement
The studies involving human participants were reviewed and approved by Institutional Review Board of The Third Xiangya Hospital of Central South University. The patients/participants provided written informed consent to participate in this study. Written informed consent was obtained from the individual(s) for the publication of any potentially identifiable images or data included in this article.
Author Contributions
SN, KC, and GY conceptualized the study. SN, KC, CG, and QP designed the methodology. CG, QP, and CZ performed validation. CZ, GY, and JH conducted formal Analysis. XZ, RX, XH, and GY contributed to resources. LY and HY curated the data. SN and KC wrote the original draft. GY and JH supervised the study. GY, JH, and XZ helped with funding acquisition. All authors contributed to manuscript revision, read, and approved the submitted version.
Funding
The research was funded by the National Scientific Foundation of China (no. 81803639), Hunan Provincial Natural Science Foundation of China (no. 2020JJ5852), the Key Research and Development Project of Hunan Province (2020SK 2010), and the National Science and Technology Major Projects for “Major New Drugs Innovation and Development” of China (no. 2017ZX09101001).
Conflict of Interest
Authors LY and HY were employed by Hunan Qianjin Xiangjiang Pharmaceutical Co., Ltd. The remaining authors declare that the research was conducted in the absence of any commercial or financial relationships that could be construed as a potential conflict of interest.
Publisher’s Note
All claims expressed in this article are solely those of the authors and do not necessarily represent those of their affiliated organizations, or those of the publisher, the editors and the reviewers. Any product that may be evaluated in this article, or claim that may be made by its manufacturer, is not guaranteed or endorsed by the publisher.
Acknowledgments
We thank all the staff of the Research Center for Clinical Trials of The Third Xiangya Hospital of Central South University. Moreover, we are grateful to all participants for providing blood samples.
Supplementary Material
The Supplementary Material for this article can be found online at: https://www.frontiersin.org/articles/10.3389/fphar.2021.797278/full#supplementary-material.
References
Alhazzani, A., Venkatachalapathy, P., Padhilahouse, S., Sellappan, M., Munisamy, M., Sekaran, M., et al. (2021). Biomarkers for Antiplatelet Therapies in Acute Ischemic Stroke: A Clinical Review. Front. Neurol. 12, 667234. doi:10.3389/fneur.2021.667234
Ansari, N., Najafi, S., Shahrabi, S., and Saki, N. (2021). PEAR1 Polymorphisms as a Prognostic Factor in Hemostasis and Cardiovascular Diseases. J. Thromb. Thrombolysis 51 (1), 89–95. doi:10.1007/s11239-020-02149-w
Capodanno, D., Alfonso, F., Levine, G. N., Valgimigli, M., and Angiolillo, D. J. (2018). ACC/AHA versus ESC Guidelines on Dual Antiplatelet Therapy: JACC Guideline Comparison. J. Am. Coll. Cardiol. 72 (23 Pt A), 2915–2931. doi:10.1016/j.jacc.2018.09.057
Duconge, J., Santiago, E., Hernandez-Suarez, D. F., Moneró, M., López-Reyes, A., Rosario, M., et al. (2021). Pharmacogenomic Polygenic Risk Score for Clopidogrel Responsiveness Among Caribbean Hispanics: A Candidate Gene Approach. Clin. Transl Sci. 14 (6), 2254–2266. doi:10.1111/cts.13124
Hirani, V., Yarovoy, A., Kozeska, A., Magnusson, R. P., and Lasker, J. M. (2008). Expression of CYP4F2 in Human Liver and Kidney: Assessment Using Targeted Peptide Antibodies. Arch. Biochem. Biophys. 478 (1), 59–68. doi:10.1016/j.abb.2008.06.025
Holmberg, M. T., Tornio, A., Paile-Hyvärinen, M., Tarkiainen, E. K., Neuvonen, M., Neuvonen, P. J., et al. (2019). CYP3A4*22 Impairs the Elimination of Ticagrelor, but Has No Significant Effect on the Bioactivation of Clopidogrel or Prasugrel. Clin. Pharmacol. Ther. 105 (2), 448–457. doi:10.1002/cpt.1177
Ji, J. Z., Li, Y. F., Jiang, L. P., Tai, T., Ge, P. X., Mi, Q. Y., et al. (2021). P-glycoprotein Deficiency Enhances Metabolic Activation of and Platelet Response to Clopidogrel through Marked Up-Regulation of Cyp3a11 in Mice: Direct Evidence for the Interplay between P-Glycoprotein and Cyp3a. Biochem. Pharmacol. 183, 114313. doi:10.1016/j.bcp.2020.114313
Kauskot, A., Vandenbriele, C., Louwette, S., Gijsbers, R., Tousseyn, T., Freson, K., et al. (2013). PEAR1 Attenuates Megakaryopoiesis via Control of the PI3K/PTEN Pathway. Blood 121 (26), 5208–5217. doi:10.1182/blood-2012-10-462887
Kim, B. K., Hong, S. J., Cho, Y. H., Yun, K. H., Kim, Y. H., Suh, Y., et al. (2020). Effect of Ticagrelor Monotherapy vs Ticagrelor with Aspirin on Major Bleeding and Cardiovascular Events in Patients with Acute Coronary Syndrome: The TICO Randomized Clinical Trial. JAMA 323 (23), 2407–2416. doi:10.1001/jama.2020.7580
Kim, C., Hong, S. J., Shin, D. H., Kim, B. K., Ahn, C. M., Kim, J. S., et al. (2019). Randomized Evaluation of Ticagrelor Monotherapy after 3-month Dual-Antiplatelet Therapy in Patients with Acute Coronary Syndrome Treated with New-Generation Sirolimus-Eluting Stents: TICO Trial Rationale and Design. Am. Heart J. 212, 45–52. doi:10.1016/j.ahj.2019.02.015
Kim, H. K., Tantry, U. S., Smith, S. C., Jeong, M. H., Park, S. J., Kim, M. H., et al. (2021). The East Asian Paradox: An Updated Position Statement on the Challenges to the Current Antithrombotic Strategy in Patients with Cardiovascular Disease. Thromb. Haemost. 121 (4), 422–432. doi:10.1055/s-0040-1718729
Kohli, P., Wallentin, L., Reyes, E., Horrow, J., Husted, S., Angiolillo, D. J., et al. (2013). Reduction in First and Recurrent Cardiovascular Events with Ticagrelor Compared with Clopidogrel in the PLATO Study. Circulation 127 (6), 673–680. doi:10.1161/CIRCULATIONAHA.112.124248
Kupstyte, N., Zaliunas, R., Tatarunas, V., Skipskis, V., Zaliaduonyte-Peksiene, D., Grabauskyte, I., et al. (2015). Effect of Clinical Factors and Gene Polymorphism of CYP2C19*2, *17 and CYP4F2*3 on Early Stent Thrombosis. Pharmacogenomics 16 (3), 181–189. doi:10.2217/pgs.14.165
Laurent, D., Dodd, W. S., Small, C., Gooch, M. R., Ghosh, R., Goutnik, M., et al. (2021). Ticagrelor Resistance: a Case Series and Algorithm for Management of Non-responders. J. Neurointerv Surg. 14 (2), 179–183. doi:10.1136/neurintsurg-2021-017638
Li, M., Hu, Y., Li, H., Wen, Z., Hu, X., Zhang, D., et al. (2017a). No Effect of SLCO1B1 and CYP3A4/5 Polymorphisms on the Pharmacokinetics and Pharmacodynamics of Ticagrelor in Healthy Chinese Male Subjects. Biol. Pharm. Bull. 40 (1), 88–96. doi:10.1248/bpb.b16-00686
Li, M., Hu, Y., Wen, Z., Li, H., Hu, X., Zhang, Y., et al. (2017b). Association of PEAR1 Rs12041331 Polymorphism and Pharmacodynamics of Ticagrelor in Healthy Chinese Volunteers. Xenobiotica 47 (12), 1130–1138. doi:10.1080/00498254.2016.1271962
Liu, S., Shi, X., Tian, X., Zhang, X., Sun, Z., and Miao, L. (2017). Effect of CYP3A4∗1G and CYP3A5∗3 Polymorphisms on Pharmacokinetics and Pharmacodynamics of Ticagrelor in Healthy Chinese Subjects. Front. Pharmacol. 8, 176. doi:10.3389/fphar.2017.00176
Liu, S., Xue, L., Shi, X., Sun, Z., Zhu, Z., Zhang, X., et al. (2018). Population Pharmacokinetics and Pharmacodynamics of Ticagrelor and AR-C124910XX in Chinese Healthy Male Subjects. Eur. J. Clin. Pharmacol. 74 (6), 745–754. doi:10.1007/s00228-018-2427-3
Pereira, N. L., Farkouh, M. E., So, D., Lennon, R., Geller, N., Mathew, V., et al. (2020). Effect of Genotype-Guided Oral P2Y12 Inhibitor Selection vs Conventional Clopidogrel Therapy on Ischemic Outcomes after Percutaneous Coronary Intervention: The TAILOR-PCI Randomized Clinical Trial. JAMA 324 (8), 761–771. doi:10.1001/jama.2020.12443
Rodrigues, B. S., Alves, M., Duarte, G. S., Costa, J., Pinto, F. J., and Caldeira, D. (2021). The Impact of Influenza Vaccination in Patients with Cardiovascular Disease: An Overview of Systematic Reviews. Trends Cardiovasc. Med. 31, 315–320. doi:10.1016/j.tcm.2020.06.003
Rodriguez, F., and Mahaffey, K. W. (2016). Management of Patients with NSTE-ACS: A Comparison of the Recent AHA/ACC and ESC Guidelines. J. Am. Coll. Cardiol. 68 (3), 313–321. doi:10.1016/j.jacc.2016.03.599
Schilling, U., Dingemanse, J., and Ufer, M. (2020). Pharmacokinetics and Pharmacodynamics of Approved and Investigational P2Y12 Receptor Antagonists. Clin. Pharmacokinet. 59 (5), 545–566. doi:10.1007/s40262-020-00864-4
Stimpfle, F., Bauer, M., Rath, D., Schaeffeler, E., Schwab, M., Gawaz, M., et al. (2018). Variants of PEAR1 Are Associated with Outcome in Patients with ACS and Stable CAD Undergoing PCI. Front. Pharmacol. 9, 490. doi:10.3389/fphar.2018.00490
Sun, Y., Lu, Q., Tao, X., Cheng, B., and Yang, G. (2020). Cyp2C19*2 Polymorphism Related to Clopidogrel Resistance in Patients with Coronary Heart Disease, Especially in the Asian Population: A Systematic Review and Meta-Analysis. Front. Genet. 11, 576046. doi:10.3389/fgene.2020.576046
Tatarunas, V., Kupstyte-Kristapone, N., Zvikas, V., Jakstas, V., Zaliunas, R., and Lesauskaite, V. (2020). Factors Associated with Platelet Reactivity during Dual Antiplatelet Therapy in Patients with Diabetes after Acute Coronary Syndrome. Sci. Rep. 10 (1), 3175. doi:10.1038/s41598-020-59663-3
Teng, R., Mitchell, P., and Butler, K. (2012). Effect of Age and Gender on Pharmacokinetics and Pharmacodynamics of a Single Ticagrelor Dose in Healthy Individuals. Eur. J. Clin. Pharmacol. 68 (8), 1175–1182. doi:10.1007/s00228-012-1227-4
Teng, R. (2015). Ticagrelor: Pharmacokinetic, Pharmacodynamic and Pharmacogenetic Profile: An Update. Clin. Pharmacokinet. 54 (11), 1125–1138. doi:10.1007/s40262-015-0290-2
Thomas, M. R., and Storey, R. F. (2016). Clinical Significance of Residual Platelet Reactivity in Patients Treated with Platelet P2Y12 Inhibitors. Vascul Pharmacol. 84, 25–27. doi:10.1016/j.vph.2016.05.010
Wallentin, L., James, S., Storey, R. F., Armstrong, M., Barratt, B. J., Horrow, J., et al. (2010). Effect of CYP2C19 and ABCB1 Single Nucleotide Polymorphisms on Outcomes of Treatment with Ticagrelor versus Clopidogrel for Acute Coronary Syndromes: a Genetic Substudy of the PLATO Trial. Lancet 376 (9749), 1320–1328. doi:10.1016/S0140-6736(10)61274-3
Wang, J., Zhang, H., Wang, R., and Cai, Y. (2021). Pharmacokinetics, Bioequivalence and Safety Evaluation of Two Ticagrelor Tablets under Fasting and Fed Conditions in Healthy Chinese Subjects. Drug Des. Devel Ther. 15, 1181–1193. doi:10.2147/DDDT.S297918
Wernly, B., Erlinge, D., Pernow, J., and Zhou, Z. (2021). Ticagrelor: a Cardiometabolic Drug Targeting Erythrocyte-Mediated Purinergic Signaling? Am. J. Physiol. Heart Circ. Physiol. 320, H90–H94. doi:10.1152/ajpheart.00570.2020
Würtz, M., Nissen, P. H., Grove, E. L., Kristensen, S. D., and Hvas, A. M. (2014). Genetic Determinants of On-Aspirin Platelet Reactivity: Focus on the Influence of PEAR1. PLoS One 9 (10), e111816. doi:10.1371/journal.pone.0111816
Yao, Y., Tang, X. F., He, C., Song, Y., Xu, J. J., Meng, X. M., et al. (2018). Effect of PEAR1 Genetic Variants on 1-Year Outcomes in Chinese Patients with Acute Myocardial Infarction after Percutaneous Coronary Intervention. J. Atheroscler. Thromb. 25 (5), 454–459. doi:10.5551/jat.39982
Yoon, S., Jeong, S., Jung, E., Kim, K. S., Jeon, I., Lee, Y., et al. (2021). Effect of CYP3A4 Metabolism on Sex Differences in the Pharmacokinetics and Pharmacodynamics of Zolpidem. Sci. Rep. 11 (1), 19150. doi:10.1038/s41598-021-98689-z
Zhou, D., Andersson, T. B., and Grimm, S. W. (2011). In Vitro evaluation of Potential Drug-Drug Interactions with Ticagrelor: Cytochrome P450 Reaction Phenotyping, Inhibition, Induction, and Differential Kinetics. Drug Metab. Dispos. 39 (4), 703–710. doi:10.1124/dmd.110.037143
Zhu, Q., Zhong, W., Wang, X., Mai, L., He, G., Chen, J., et al. (2019). Pharmacokinetic and Pharmacogenetic Factors Contributing to Platelet Function Recovery after Single Dose of Ticagrelor in Healthy Subjects. Front. Pharmacol. 10, 209. doi:10.3389/fphar.2019.00209
Keywords: ticagrelor, pharmacokinetics, CYP4F2, genetic polymorphisms, single nucleotide polymorphism
Citation: Nie S, Chen K, Guo C, Pei Q, Zou C, Yao L, Yuan H, Zhao X, Xie R, He X, Huang J and Yang G (2022) Effect of CYP4F2 Polymorphisms on Ticagrelor Pharmacokinetics in Healthy Chinese Volunteers. Front. Pharmacol. 12:797278. doi: 10.3389/fphar.2021.797278
Received: 18 October 2021; Accepted: 27 December 2021;
Published: 25 February 2022.
Edited by:
Takeo Nakanishi, Takasaki University of Health and Welfare, JapanReviewed by:
Yan Li, Auckland University of Technology, New ZealandTheodora Katsila, National Hellenic Research Foundation, Greece
Copyright © 2022 Nie, Chen, Guo, Pei, Zou, Yao, Yuan, Zhao, Xie, He, Huang and Yang. This is an open-access article distributed under the terms of the Creative Commons Attribution License (CC BY). The use, distribution or reproduction in other forums is permitted, provided the original author(s) and the copyright owner(s) are credited and that the original publication in this journal is cited, in accordance with accepted academic practice. No use, distribution or reproduction is permitted which does not comply with these terms.
*Correspondence: Guoping Yang, eWdwOTg4MEAxMjYuY29t; Jie Huang, Y2VsbGFodWFuZzE5ODhAMTYzLmNvbQ==
†These authors have contributed equally to this work