- 1UFR Sciences de La Nature, Université Nangui Abrogoua, Abidjan, Côte d'Ivoire
- 2Université de Lille, Université de Liège, Université de Picardie Jules Verne, JUNIA, UMRT 1158 BioEcoAgro, Métabolites Spécialisés D’origine Végétale, Lille, France
- 3Univ Lille, CNRS, INSERM, CHU Lille, Institut Pasteur de Lille, U1019-UMR 9017-CIIL-Center for Infection and Immunity of Lille, Lille, France
Millions of people are still infected with hepatitis C virus (HCV) nowadays. Although recent antivirals targeting HCV proteins are very efficient, they are not affordable for many people infected with this virus. Therefore, new and more accessible treatments are needed. Several Ivorian medicinal plants are traditionally used to treat “yellow malaria”, a nosological category including illness characterized by symptomatic jaundice such as hepatitis. Therefore, some of these plants might be active against HCV. An ethnobotanical survey in Côte d’Ivoire allowed us to select such medicinal plants. Those were first extracted with methanol and tested for their anti-HCV activity. The most active ones were further studied to specify their IC50 and to evaluate their toxicity in vitro. Greener solvents were tested to obtain extracts with similar activities. Following a phytochemical screening, tannins of the most active plants were removed before re-testing on HCV. Some of these tannins were identified by UPLC-MS and pure molecules were tested against HCV. Out of the fifteen Ivorian medicinal plants selected for their putative antiviral activities, Carapa procera DC. and Pericopsis laxiflora (Benth. ex Baker) Meeuwen were the most active against HCV (IC50: 0.71 and 0.23 μg/ml respectively) and not toxic for hepatic cells. Their crude extracts were rich in polyphenols, including tannins such as procyanidins A2 which is active against HCV. The same extracts without tannin lost their anti-HCV activity. Replacing methanol by hydro-ethanolic solvent led to tannins-rich extracts with similar antiviral activities, and higher than that of aqueous extracts.
Introduction
Hepatitis C is a major cause of chronic hepatitis often associated with complications, such as liver cirrhosis and hepatocellular carcinoma (Levrero, 2006; Messina et al., 2015). It is estimated that approximately 71.1 million people are infected with hepatitis C virus (HCV) worldwide (Polaris Observatory HCV Collaborators, 2017). The prevalence of HCV infection varies considerably between countries. The adult HCV seroprevalence was estimated at 2.2% in Côte d’Ivoire and can be greater than 10% in some countries like Egypt (Riou et al., 2016). To date, no vaccine is available (Tarr et al., 2015). In the last decade, treatments with direct-acting antiviral (DAA) agents targeting viral proteins have been established. These therapies with high rates of sustained viral response against all HCV genotypes and very few side effects are driven by DAAs that target viral proteins NS3/4A protease, NS5A, and NS5B polymerase (Falade-Nwulia et al., 2017). However, there is a risk of selecting viral variants resistant to DAAs leading to failure of the therapy, which will require new treatments (Pawlotsky, 2016). Moreover, these treatments are very expensive and will benefit only a very small proportion of patients (Graham and Swan, 2015). The use of plant extracts or compounds isolated from plant extracts should render the therapy more accessible to many patients in developing countries. Therefore, the search for such compounds and extracts is still needed to treat the vast majority of patients in the coming decades.
Natural products are largely used for healthcare worldwide, and remain an important source of inspiration for medicinal chemistry nowadays (Newman and Cragg, 2020). Numerous DAAs from natural origins are now described and are able to target different steps of the HCV infectious cycle. Many plant extracts and natural products with such antiviral activities have been reviewed these last years (Patil et al., 2016; Jardim et al., 2018; Seron, 2018). In this context, crude extracts from plants used in traditional medicine are promising sources of antiviral molecules.
In Côte d’Ivoire, several medicinal plants are traditionally used to treat “yellow malaria”, a nosological category including illness characterized by symptomatic jaundice such as hepatitis. Based on an ethnobotanical survey and a bibliographic search, 15 plants were selected in Côte d’Ivoire. They were screened in vitro for their activity on HCV and the two most active ones were selected and further studied. For this antiviral screening, methanol was first used to obtain crude extracts for each plant. However, this toxic solvent can obviously not be used in traditional medicine. In addition, a sustainable approach was carried out. Therefore, methanol was replaced by hydro-ethanolic solvents and leaves were used rather than bark or roots. The composition of obtained extracts was investigated in order to elucidate which types of components might contribute to the anti-HCV activity.
Material and Methods
Chemicals, Culture Medium and Antibodies
Solvents used for extractions were ethanol and methanol (>99% purity; VWR France), and ultrapure water prepared with an Integral 5 Milli-Q system (Merck Millipore). Ethyl acetate (Fisher), acetic acid and formic acid (Carlo Erba) were used for TLC.
Dulbecco’s modified Eagle’s medium (DMEM), Opti-MEM, phosphate buffered saline (PBS), glutamax-I, and fetal bovine serum were purchased from Invitrogen (Carlsbad, CA). 4′,6-Diamidino-2-phenylindole (DAPI) was from Molecular Probes (Thermo Fischer Scientific, Waltham, United States).
Delphinidin chloride was from Extrasynthèse (Lyon, France) and was >96% pure. Boceprevir was kindly provided by Philippe Halfon (Hôpital Européen, Laboratoire Alphabio, Marseille, France). Procyanidins A1, A2, B1, B2 and B3 were >96% pure and from Phytolab (Germany). All these compounds were tested on Huh-7 cells. In addition, several other compounds were used as standards for TLC or UPLC: quercetin and quinin were from Merck (Germany), gallic acid, stigmasterol and glycyrrhetic acid were from Extrasynthése (France), (−)-epicatechin, (+)-catechin, aloin and ellagic acid were from Sigma (Germany), (−)-EGC was from Chromadex (United States), (−)-EGCG was from Selleckchem (United States).
The anti-E1 monoclonal antibody (A4) (Dubuisson et al., 1994) was produced in vitro by using MiniPerm apparatus (Heraeus, Hanau, Germany). Cy3-conjugated goat anti-mouse IgG was from Jackson Immunoresearch (West Grove, PA, United States).
Ethnobotanical Survey
The ethnobotanical survey was conducted in the Bafing region, which is situated in North-West Côte d’Ivoire and corresponds to the Touba department. It covers a total land area of 8,650 km2 and shares boundaries with the Odienné department in the North and the Séguéla and Biankouma departments in the East and the South respectively. In the West, it shares boundaries with the Republic of Guinea. It is one of the most distant Ivorian regions from the capital city Abidjan. Therefore, people are still more dependent on traditional medicine compared to people living closer to cities. In this region, traditional healers could be assigned in at least one of these 3 groups: 1) “Bouêti”: they are plain healers, who know medicinal plants and treat common illnesses; they are the first medical resort in this region; 2) “Kamon” or marabout: they heal with natural products according to the Coran; 3) “Komanti”: they heal with natural products and talismans. Traditional healers could also belong to two of these groups: “Kamon-Bouêti” and “Komanti-Bouêti”. Seven of them (5 “Bouêti”, 1 “Komanti-Bouêti” and 1 “Kamon-Bouêti”) were met three times during one month of non-structured interviews, in the city of Touba, the Mahanan village and the Ouintoulo camp. Religion in Côte d’Ivoire is diverse and the main religions are Islam, Christianity (primarily Roman Catholic) and various indigenous religions. The Survey considered the use of plants against a group of diseases called “yellow malaria”, which is a rough translation for diseases that cause icterus. This nosological category include malaria but also other illness characterized by symptomatic jaundice as hepatitis, obstruction of the bile duct, hemolysis. Traditional healers were asked about the illness they cured with plants, the name of these plants, the part used, how they prepared them and the mode of application.
Plant Material and Extractions
The 15 plants were collected in the wild during the morning. They were then cleaned and air-dried at constant temperature (26°C) for 1–2 weeks at the Nangui Abrogoua University (Abidjan). They were authenticated by a botanist at the Centre National de Floristique (CNF), University of Félix Houphouët Boigny de Cocody (Abidjan), by comparing their morphological characters to a flora. Voucher specimens were deposited in an Herbarium at the CNF. The voucher numbers and the traditional uses of the investigated species are presented in Table 1. Their names have been checked with “The World Flora Online” (http://www.worldfloraonline.org/- accessed October, 2021). Each plant was then powdered and stored in the dark until extractions. For each plant, 20 g of dried powder were mixed with 100 ml methanol for 24 h. After filtration, the grounds were extracted again twice in the same way. The 3 resulting filtrates were combined and dried under vacuum at 40°C.
C. procera and P. laxiflora were also extracted with water-ethanol and water-methanol mixtures (0–100%) as solvent. For each plant, extractions were carried out in 100 ml flasks with 2 g of dried powder and 40 ml of solvent. Flasks were placed for 2 h in a shaking incubator (Multitron II Infors HT) in order to maintain agitation at 160 rpm and temperature at 20°C. These extractions were carried out in triplicates. Final extracts were then centrifuged for 10 min at 10,000 tr. min−1 and 4°C and the supernatants were stored at −18°C in the dark until use. 10 ml of supernatant were used to determine dry mass yields (measurements made in duplicate, each one with 5 ml of supernatant) with an infrared moisture analyzer (Precisa XM60). The remaining volume of supernatant was freeze-dried (freezedryer Heto PowerDry PL9000 Thermo Fisher Scientific) so as to get dehydrated extract for further analysis. Evaporation under vacuum was carried out prior to freeze-drying in the case of supernatants containing ethanol or methanol (rotary evaporator Heidolph instrument, GmbH and Co. KG).
All the dried crude extracts or isolated compounds were resuspended in DMSO before antiviral assays. Plant extracts were dissolved in DMSO to reach a final concentration of 25 mg/ml. Delphinidin and boceprevir were also resuspended in DMSO at a final concentration of 100 mM. Serial dilutions in DMEM cell culture medium were then performed to reach the final concentration mentioned for each experiment.
Phytochemical Screening
Phytochemical screening was performed with thin layer chromatography using pre-coated silica gel plates (Alugram Xtra Sil G/UV254, Machery-Nagel). The solvent system was EtOAc/Acetic acid/Formic acid/H2O (100:11:11:20 v/v). For each extract, 10 µL (5 mg/ml) were spotted on the TLC plate. The same revealers as in Santos et al. (Santos et al., 2018) were used, except for total and condensed tannins, for which ferric chloride (Oliveira et al., 2015) and DMACA (Lea, 1978) (Sigma-Aldrich) were used. Folin-Ciocalteu reagent (VWR chemicals) was also used to reveal all the polyphenols. The following standards were used as controls on TLC plates: quercetin, (+)-catechin, gallic acid, aloin, stigmasterol, glycyrrhetic acid and quinin.
Tannin Removal
To quantify tannins and other phenolics in our extracts, Wiesneth and Jürgenliemk, (2017) that is based on the Folin-Ciocalteu method of the European Pharmacopeia (2.8.14) was adapted. Briefly, 1.5 ml of water was first added to 5 mg of each extract to dissolve them in water bath (95°C) during 30 min. These were centrifuged at 2,500 rpm for 5 min, and the supernatants were the “total phenolic solutions” (TPS). For each TPS, 10 mg hide powder was added to an aliquot of 0.5 ml. These preparations were stirred and further incubated without light for 60 min while shaking. The supernatants after centrifugation (2,500 rpm, 5 min) resulted in the solutions containing phenols without tannins (TPS-T). The removal of tannins was checked with TLC revealed by the DMACA reagent by comparing TPS (blue spots) and TPS-T (no spot).
Polyphenols and Tannins Quantification
For total phenolics and tannins quantification, Wiesneth and Jürgenliemk microplate method was used. Briefly, except for the outer wells filled with water, 20 µL of sample (TPS, TPS-T), or gallic acid (Extrasynthese, France) for calibration curve were added in each well. Then 100 µL of a 1/10th dilution of Folin-Ciocalteu’s reagent was added and shaken without light for four minutes. Finally, 80 µL of a 10.6%-solution of Na2CO3 anhydrous in water was added to the samples. Measurement of absorbance at 690 nm at room temperature was achieved after 90 min using a Spectrostar Nano (BMG Labtech) spectrophotometer.
Extracts Characterization by UPLC-UV-MS
Ultra-High Performance Liquid Chromatography (UPLC) analyses were performed on an Acquity UPLC H-Class system (Waters, Guyancourt, France) coupled with a Diode Array Detector (DAD) and a QDa ESI-Quadrupole Mass Spectrometer. All data were acquired and processed using Empower 3 software. Separation was achieved using an ACQUITY UPLC® BEH phenyl 1.7 µm (2.1 × 100 mm) column (Waters, Milford MA). Gradient elution was performed with (A) 0.1% formic acid in water and (B) 0.1% formic acid in acetonitrile at a flowrate of 0.3 ml/min. The amount of B was increased from 5% for the initial 1 min to 30% at 9 min, and to 100% at 9.5 min. The condition was then kept at 100% B until 10.5 min. Finally, the column was re-equilibrated to 5% B in 0.5 min and maintained for an additional 2 min, resulting in a total run time of 13 min. Column oven and auto-sampler were set at 30°C and 15°C respectively, and 4 µL of each sample (1 mg/ml in MeOH/water) were injected. Analytes were monitored using UV detection (l range: 190–790 nm, resolution: 1.2 nm) and MS-Scan from 100 to 1,000 Da (both in positive and negative mode). Cone voltage was set at 15 V. Probe temperature was 600°C. Capillary voltage was 0.8 kV. The main tannins and anthocyanins were identified based on the retention time of the commercial standards and their mass spectra (Supplementary Table S1).
Antiviral Assays
Cells and Culture Conditions
Huh-7 cells, established from a human hepatocellular carcinoma (Nakabayashi et al., 1982), were grown in DMEM supplemented with glutaMAX-I and 10% fetal bovine serum (complete culture medium) in an incubator at 37°C with 5% CO2.
Virus and Infectivity Assays
A modified JFH1 strain (Japanese Fulminant Hepatitis-1, genotype 2a) containing cell culture adaptive mutations (Delgrange et al., 2007; Goueslain et al., 2010) was used. JFH1 was kindly provided by T Wakita (National Institute of Infectious Diseases, Tokyo, Japan). The viral stocks were produced in Huh-7 cells. Huh-7 cells were infected with a pre-stock of HCVcc in flasks. After 24, 48 and 72 h the supernatants of flasks were collected. The titer of the stock was 5 × 106 focus forming unit (ffu)/mL for JFH1. For infection assay, Huh-7 cells, 6,000/well, seeded in 96-well plate were inoculated with HCVcc at a multiplicity of infection (MOI) of 0.8 during 2 h at 37°C then the inoculum was removed and cells were incubated in complete culture medium for 28 h at 37°C. Compounds were added to cells for 2 h at 37°C in the presence of the virus (inoculation), and for 28 h at 37°C after virus removal (post-inoculation). Cells were fixed with ice-cold methanol and subjected to immunofluorescent detection of viral E1 envelope protein.
Immunofluorescent Detection Assay
HCVcc infected cells grown in 96-well plates were processed for immunofluorescence detection of viral proteins, as previously described (Rouillé et al., 2006). Nuclei were stained with 1 μg/ml of DAPI, and infected cells were detected by immunofluorescent labeling of E1 envelope glycoprotein (HCV), followed by Cy3-conjugated anti-mouse secondary antibody. For quantification of infection, confocal images were recorded with an automated confocal microscope IN Cell Analyzer 6,000 (GE Healthcare Life Sciences) using a ×20 objective with exposure parameters 405/450 nm and 561/610 nm. Six fields per well were recorded. Each image was then processed using the Colombus image analysis software (Perkin Elmer). Nuclei were first segmented and the cytoplasm region was extrapolated based on the DAPI staining. Objects with a specific and predefined size were defined as cells. The ratio of infected cells over total cells represents the infection rate. For each virus, the number of cells per well and MOI were determined in order to have 30–40% of infected cells in control experiments with no inhibitor. Infection rates in DMSO controls were expressed as 100%.
Cytotoxicity Assay
Huh-7 cells were plated in 96-well plates at a density of 6,000 cells/well and then were incubated the next day in 100 μL of culture medium containing increasing concentrations of plant crude extracts for either 24, 48, or 72 h. An MTS [3-(4,5-dimethylthiazol-2-yl)-5-(3-carboxymethoxyphenyl)-2-(4-sulfophenyl)-2H-tetrazolium]-based viability assay (Cell Titer 96 Aqueous non-radioactive cell proliferation assay, Promega) was performed as recommended by the manufacturer. The absorbance of formazan at 490 nm is detected using an ELISA plate reader (ELX 808 Bio-Tek Instruments Inc.). Each measure was performed in triplicate.
Results and Discussion
Antiviral Plant Selection
During our ethnobotanical survey, 15 plants were identified as commonly used to treat “yellow malaria” and were thus selected (Table 1). Indeed, this symptom could correspond to icterus, which could be caused by hepatitis. Since HCV is quite common in Côte d’Ivoire, and can cause icterus in some cases, some of these 15 plants might have anti-HCV activity. However, not to mention other important considerations such as cultural aspects in traditional medicine, jaundice can be caused by other hepatitis (either viral or not) or many other diseases. For instance, malaria, which is widespread in Côte d’Ivoire, can cause hemolysis which in turn can lead to icterus. Other symptoms such as anemia can be observed and described in “white malaria” (Traore et al., 2021). In fact, many of the plants screened here for putative antiviral activity have been reported to be used against malaria and/or have shown antiplasmodial activity (Table 1). For instance, leave or bark decoction of Alstonia boonei De Wild. are used to treat malaria by Ehotile people in Côte d’Ivoire (Malan et al., 2015) and also in Nigeria (Ishola et al., 2014) and Cameroon (Tsabang et al., 2012). Anogeisus leiocarpa (DC.) Guill. and Perr. was quoted as one of the most used plant to treat malaria in an ethnobotanical survey in Mali (Diarra et al., 2015). Carapa procera DC. extracts had antiplasmodial activity in vitro (Sarpong et al., 2016), and in a more recent study, they had anti-malaria activity on mice (Koama, 2021). Other plant parts were also reported to be used against malaria or have shown antiplasmodial activity: Combretum collinum Fresen, Momordica charantia L., Paulinia pinnata L., Pericopsis laxiflora (Benth. ex Baker) Meeuwen, Rauvolfia vomitoria Afzel., Sarcocephalus latifolius (Sm.) E.A.Bruce and Terminalia macroptera Guill. and Perr. (Table 1). In our sustainable approach, only plant leaves were used for further studies, although other parts of the plants such as bark were traditionally used in some cases. For instance, the stem bark of Pericopsis laxiflora (Benth. ex Baker) Meeuwen was already reported to be traditionally used in Côte d’Ivoire, and the activity of various extracts were tested in vitro on various strains of Plasmodium (Koffi et al., 2020). The methanolic extract was the most active one. However, in Ghana, it was the decoction of P. laxiflora leave that was used against malaria (Asase et al., 2005). Although a putative antiviral activity may be due to different compounds and in different organs, our antiviral assays were carried out with leave extracts only.
Crude methanolic extracts of the 15 selected plants were prepared and tested in vitro on HCV infection (Figure 1). After removing methanol, the dried extracts were dissolved in DMSO at 25 mg/ml stock solution. Huh-7 cells were inoculated with HCV in the presence of the extracts at 25 μg/ml and infection was quantified using an immunofluorescent assay. DMSO was used as negative control, and delphinidin and boceprevir were used as positive controls. Indeed, delphinidin is a natural product with strong anti-HCV activity (Calland et al., 2015), and boceprevir is a commercial protease inhibitor used to treat hepatitis C. The cell number was also quantified at the end of the experiment to determine the toxicity of the extracts.
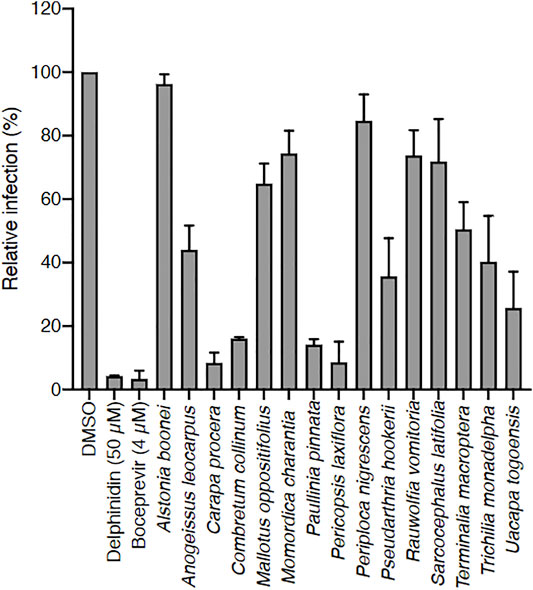
FIGURE 1. Screening of 15 Ivorian medicinal plant crude extracts for their anti-HCV activity. Each plant was extracted with methanol, and dried extracts were dissolved in DMSO (used here as negative control). Huh-7 cells were inoculated with HCV in the presence of crude extracts at 25 μg/ml, or delphinidin at 50 μM, or boceprevir at 4 µM. Infected cells were quantified 30 h post infection by immunofluorescence detection of viral E1 protein. Data are presented relative to untreated control. Data are means ± SEM of 3 experiments performed in triplicates.
Among the 15 plants tested, Carapa procera DC. and Pericopsis laxiflora (Benth. ex Baker) Meeuwen extracts were generally the most active on HCV, with less than 10% infected cells when these were in contact with the corresponding crude extracts (25 μg/ml). In addition, these two extracts were not toxic on hepatic cells at those concentrations with a CC50 of 203.3 μg/ml and 196.6 μg/ml at 24 h, and 93.3 μg/ml and 88.7 μg/ml at 72 h for C. procera and P. laxiflora, respectively (Figures 2A,B). A dose-response concentration experiment was performed to determine the half-median inhibitory concentration IC50. A dose-response inhibitory activity was observed for the two extracts confirming their antiviral capacity against HCV. The IC50 was estimated at 0.71 and 0.23 μg/ml for C. procera and P. laxiflora, respectively (Figures 2C,D).
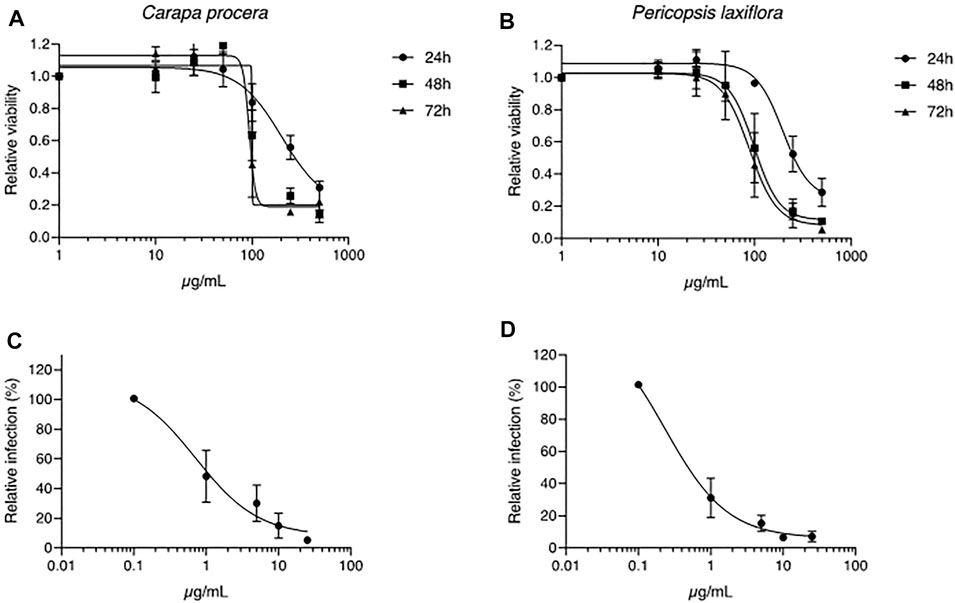
FIGURE 2. Toxicity and antiviral assays of C. procera and P. laxiflora crude extracts. (A, B): Huh-7 cells were incubated with culture medium containing crude extracts at different concentrations. MTS toxicity assay was performed at 24, 48 or 72 h of incubation. (C, D): Huh-7 cells were inoculated with HCV in the presence of crude extracts at different concentrations. Infected cells were quantified 30 h post infection by immunofluorescence detection of viral E1 protein. Data are presented relative to untreated control. Data are means ± SEM of 3 experiments performed in triplicates.
Tannins Are Responsible for the Antiviral Activity of the Selected Plants
The chemical composition of C. procera and P. laxiflora extracts were evaluated by using TLC and conventional reagents to reveal major groups of secondary metabolites. Both extracts were rich in polyphenols, including flavonoids and tannins (Table 2). This is qualitatively consistent with previous results showing that leave extracts of C. procera were rich in polyphenols including anthocyanins and flavonols (Adjé et al., 2019). Polyphenol were also reported in P. laxiflora bark, and to a lesser extend in leave aqueous extracts (Alhaji et al., 2014). However, none of our extracts contained alkaloids, contrary to a previous study revealing some in P. laxiflora leave (Fadipe et al., 2019). This might be due to different extraction protocols.

TABLE 2. Phytochemical screening of the most active plants. Cp: Carapa procera DC.; Pl: Pericopsis laxiflora (Benth. ex Baker) Meeuwen; e: ethanol/water (50:50) eco-extract (crude methanolic extract otherwise); “−T”: after tannins removal; (−) absence or (+) presence of phytochemicals.
Since many tannins have antiviral activity, especially against HCV (Zuo et al., 2007; Calland et al., 2012b; Lin et al., 2013; Ajala et al., 2014; Liu et al., 2015; Chowdhury et al., 2018; Tamura et al., 2019), they were then specifically removed in our two most active extracts (C. procera and P. laxiflora) in order to check whether these “tannin-free” extracts were still active against HCV or not. Results are presented in Figure 3.
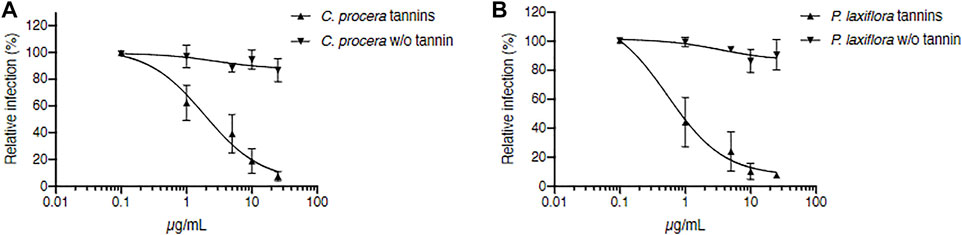
FIGURE 3. Tannins are responsible for C. procera and P. laxiflora activity against HCV. Tannin removal required heating of extracts. C. procera (A) and P. laxiflora (B) methanolic extracts were tested on HCV infection after heating (tannins), and after tannins removal (w/o tannins). Quantification of infection was as described above. Data are presented relative to untreated control and are means ± SEM of 3 experiments performed in triplicates.
The IC50 of crude extracts after heating were 1.89 and 0.53 μg/ml for C. procera and P. laxiflora, respectively. Although slightly higher, these were similar to the IC50 of the same extracts before heating (Figure 2C,D): 0.71 and 0.23 μg/ml for C. procera and P. laxiflora, respectively. Some active molecules might have been lost during this process, either by heat degradation (95°C), or because they were less soluble in water and therefore lost in the pellet (see material and methods section).
After heating and tannins removal (w/o tannin), both extracts lost completely their activity, regardless of their concentration (Figures 3A,B). Therefore, tannins are most likely responsible for C. procera and P. laxiflora activity against HCV in vitro.
Traditional and Green Extractions of C. procera and P. laxiflora Preserve Their Antiviral Activity
Our most active methanolic extracts were from C. procera and P. laxiflora. According to our ethnobotanical survey, C. procera and P. laxiflora leaves are traditionally used in water extracts. However, water may not be the best solvent to extract the active tannins of these plants. Acetone and alcoholic solvents as well as their mixtures with water have been commonly used to extract tannins from various vegetal sources (Wei et al., 2011; Metrouh-Amir et al., 2015). To avoid toxic solvents, water, ethanol and their mixtures were used here since they are considered as eco-friendly and traditionally used solvents. Hence, C. procera was extracted with hydro-alcoholic solvents containing 0–100% ethanol, and total polyphenols and tannins were quantified in these extracts. These results were compared to those obtained using similar water/methanol mixtures as solvents. The effect of these various hydro-alcoholic solvents was first studied on dried mass yields. Figure 4A shows that best yields were obtained with 50% alcohol (ethanol or methanol). The lowest yields were obtained with water or ethanol 100%. Moreover 50% ethanol extraction allowed a better yield than any (hydro-)methanolic extraction.
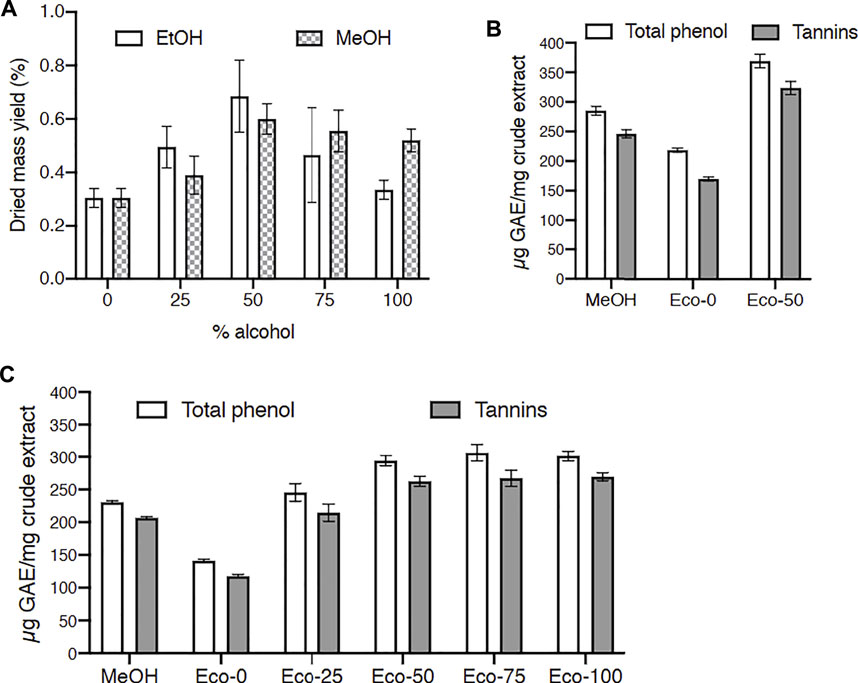
FIGURE 4. Effect of solvents on dried mass, total phenol and tannin yields for Carapa procera DC. and Pericopsis laxifora (Benth. ex Baker) Meeuwen. (A): Dried mass yield for C. procera with hydro-alcoholic solvents containing 0–100% alcohol. (B, C): Total phenol and tannin yields for C. procera (B) and P. laxifora (C); GAE: gallic acid equivalent; Eco: hydroalcoholic eco-extracts with 0–100% ethanol; MeOH: crude methanolic extracts.
Then total polyphenols and tannins were quantified in these extracts (Figures 4B,C). For C. procera eco-extracts (Figure 4B), polyphenols and tannins contents increased when ethanol was added to water for extractions and reached a plateau from 50 to 100% ethanol. Quite similar solvent effect was reported for tannins extraction from Arum maculatum leaves (Farahmandfar et al., 2019). Since tannins are not very hydrophilic, the addition of ethanol in the solvent enhanced their extraction. Polyphenols and tannins contents were higher in these hydro-ethanolic extracts compared to methanolic extract. Similarly, for P. laxiflora (Figure 4C), the polyphenols and tannins contents were higher in the 50:50 hydro-ethanolic extract compared to methanolic and aqueous extracts.
Since the dried mass yields were better with the 50% hydro-alcoholic solvents compared to water or alcohol extractions (Figure 4), the ethanol/water (50:50) extracts were chosen for further antiviral assays. Figure 5 shows that for both C. procera and P. laxiflora, these crude hydro-ethanolic extracts were very active against HCV (IC50 = 1.18 and 2.11 μg/ml, respectively), even after heating (IC50 = 2.69 and 5.92 μg/ml, respectively). These results are similar compared to those of Figures 3A,B, suggesting that some active molecules might have been lost during the heating process before tannin removal. In addition, the ethanol/water (50:50) extract and crude methanolic extracts (Figure 3) had a similar anti-HCV activity, whereas the activity of the aqueous extracts was lower (Supplementary Figure S1). This is consistent with the tannin contents of all these extracts (Figures 4B,C). Moreover, the eco-extracts lost completely their activities after tannins removal, as the methanolic extracts did (Figure 3). Hence, the antiviral activities of C. procera and P. laxiflora are similar in eco-extracts compared to methanolic extracts, and they are also due to tannins. Those tannins are in much lower quantity in aqueous extracts that are less active against HCV. It is important to note that according to our survey, these plants were used in decoction, whereas our aqueous extracts were obtained at 20°C. Therefore, the compositions of these extracts were probably different. In this study our aim was to compare different solvents without heating.
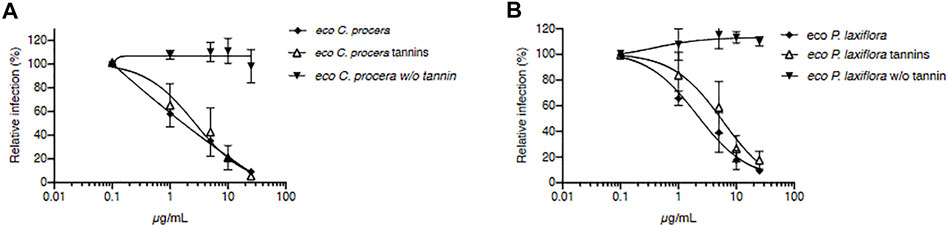
FIGURE 5. Tannin-rich eco-extracts of C. procera and P. laxiflora are active against HCV. C. procera (A) and P. laxiflora (B) eco-extracts (50% ethanol) were tested on HCV infection before tannin removal and before heating (squares), after heating (triangles), and after tannins removal (w/o tannins). Quantification of infection was as described above. Data are presented relative to untreated control. Data are means ± SEM of 3 experiments performed in triplicates.
The obtained results show that very active extracts could be obtained using eco-friendly solvents such as ethanol-water mixtures in a simple extraction and without heating or any further purification steps. Such extraction method respects the principles of the green extraction (Chemat et al., 2019) and could be easily applied for the preparation of antiviral extracts from C. procera and P. laxiflora.
Procyanidin A2 and Other Tannins Contribute to the anti-HCV Activity of C. procera and P. laxiflora Extracts
Several extracts were analyzed by UPLC-MS especially for their tannins and anthocyanins that are known to have antiviral activities. For instance, epigallocatechin-3-gallate (EGCG) has been identified to inhibit HCV entry and cell-to-cell transmission (Ciesek et al., 2011; Calland et al., 2015). (−)-epicatechin-3-O-gallate (ECG) were found to inhibit NS3 protease in a study (Zuo et al., 2007), although it exhibited weak antiviral activity on HCV entry in another study (Calland et al., 2012a). Ellagic acid is also known to have anti-HCV activity (Reddy et al., 2014). Procyanidin B1, a dimer of (−)- epicatechin and (+)- catechin with a procyanidin type B structure, extracted from Cinnamomi cortex, could inhibit HCVpp entry in a dose-dependent manner (Li et al., 2010). More recently, a procyanidin with a type A structure was shown to inhibit HCV entry (Fauvelle et al., 2017). Results presented in Table 3 confirmed that all crude extracts contained tannins in various amounts, that were largely reduced—if not completely removed—after treating these samples with hide powder.
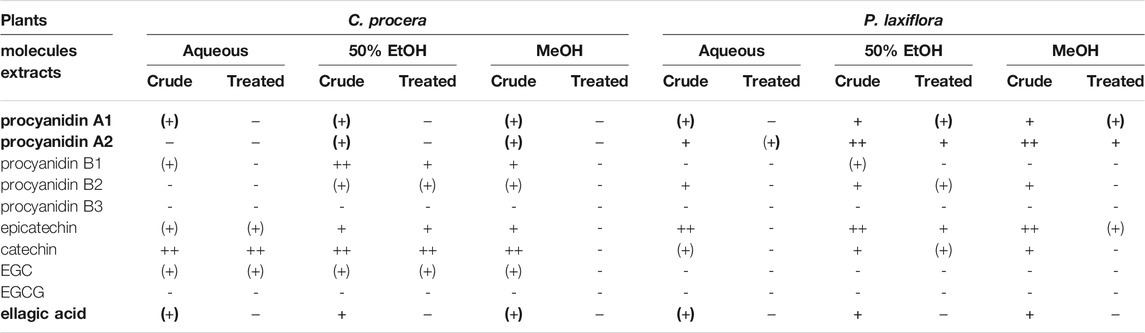
TABLE 3. Tannins and monomers content of C. procera and P. laxiflora extracts before and after tannin removal. For both plants, these molecules were searched in each extract (aqueous, hydro-ethanolic and methanolic) before (crude) and after treatment with hide powder (treated). +: presence; −: absence (no peak detected); (+): trace (peak area just above the noise). Bold: molecules found in all alcoholic (active) crude extracts but not in the treated ones.
Some of these tannins or related molecules (precursors or degradation products) could contribute to the antiviral activity of these extracts. For instance, ellagic acid is present in all crude extracts, but absent after tannin removal. Conversely, other tannins (EGC and EGCG) or monomers (catechin and epicatechin) are either totally absent or present in all extracts of at least one plant, even after tannin removal. Thus, their contribution to the anti-HCV activity is unlikely here. More interestingly, at least traces of procyanidins A1 and A2 are present in most crude extracts, except procyanidin A2 which is absent in the C. procera aqueous extract (Table 3), but these compounds are not detected or in much smaller amounts after removing tannins with hide powder (“treated” extracts). Therefore, these tannins may explain the anti-HCV activity of P. laxiflora extracts at least, and to a lesser extend this of C. procera extracts, where those procyanidins are found in much smaller quantities. On the other hand, the activity of C. procera extracts might be due to tannins such as procyanidin B1, known for having effects on HCV replication (Li et al., 2010), and which is present in higher amounts for these crude extracts compared to those treated with hide powder. Similarly to the other dimers, procyanidin B2 was usually more concentrated in the most active (methanolic and hydro-ethanolic) extracts compared to the other ones (Table 3), and it has already shown antiviral activities on other viruses (Yang et al., 2014; Liu et al., 2018). Therefore, it might also contribute to the anti-HCV activity.
Hence, these four procyanidins were tested against HCV. Their structures are shown in Supplementary Table S2. Figure 6 shows that procyanidin A2 is clearly the most active one, which is consistent with above results (Figures 3, 6; Table 3). For instance, extracts of P. laxiflora are antiviral before tannin removal, but not after. However, procyanidin A2 cannot explain the anti-HCV activity in all extracts. Indeed, this molecule is in much lower (trace) amount in C. procera extracts compared to P. laxiflora extracts, although the former plant has anti-HCV activity as well. Together with ellagic acid, procyanidins A1 and B1 may contribute to the activity of various extracts, although they are less active compared to procyanidin A2. Thus, the antiviral activity of all tested extracts is likely due to several tannins. Those that were found to be active were present in higher amounts in the most active extracts (methanolic and hydro-ethanolic), and also in aqueous extract in lower amount. In addition, they were absent from all these extracts after hide powder treatment. These results are correlated with the anti-HCV activity of these extracts: lower in aqueous extracts compared to alcoholic ones, and null after tannins removal.
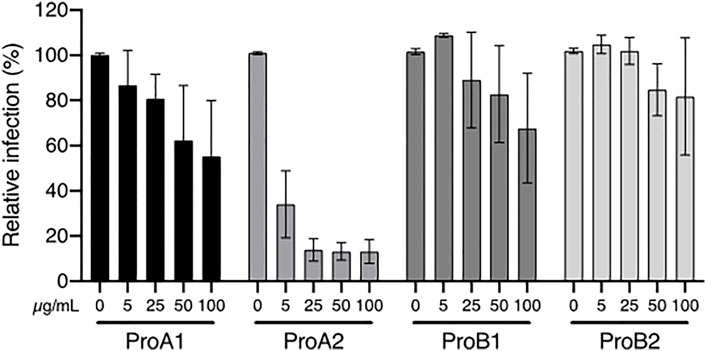
FIGURE 6. Anti-HCV activity of procyanidins. Huh-7 cells were inoculated with HCV in the presence of procyanidin A1 (ProA1), A2 (ProA2), B1 (ProB1), and B2 (ProB2) at 5, 25, 50 and 100 µM. Cells were fixed 30 h post-infection. Quantification of infection was as described above. Data are presented relative to untreated control and are means ± SEM of 3 experiments performed in triplicates.
Some of the tannins were identified and semi-quantified here, but many others were probably present in the same extracts. Indeed, the chromatograms in Supplementary Figure S2 shows that most peaks found in C. procera and P. laxiflora crude extracts can also be seen after tannin removal and therefore probably inactive on HCV. But, for both plants, the baseline is increased between 5- and 10-min elution for crude extracts, whereas it is close to zero after tannin removal. Thus, the difference is probably due to various tannins that were not all identified here and that may contribute to antiviral activity. Nonetheless, procyanidin A2 is one of the major compounds of P. laxiflora eco-extract, and was mostly removed after hide powder treatment (Supplementary Figure S2).
Tannins including procyanidins are generally known to have low oral biodisponibility. However, this depends on numerous factors, such as their degree of polymerization (DP). For example, procyanidins with DP < 4 can be absorbed and distributed in the organism, at least under certain conditions (Zhang et al., 2016). Depending on other factors (e.g. gastric pH, food intake), tannins can be degraded into smaller molecules, and metabolized by the colonic flora, leading to other compounds that may have a biological activity as well (Zhang et al., 2016; Smeriglio et al., 2017). In our survey, P. laxiflora was used in bath, whereas C. procera was also used in tea (Table 1). Therefore, digestion related factors might lead to other active molecules after drinking this plant extract. It would be interesting to test procyanidins and other putative anti-HCV tannins in vivo, before extrapolating any of our data in terms of clinical relevance for treating hepatitis C.
Conclusion
Plants are widely used in Ivorian traditional medicine to treat various diseases. Our ethnobotanical survey revealed that at least 15 plants are used in the Bafing region to treat yellow malaria. Since this nosological category includes illnesses characterized by jaundice, some of these plants may have antiviral activity. Therefore their crude methanolic extracts were screened against HCV in vitro. The two most active ones, Carapa procera DC. and Pericopsis laxiflora (Benth. ex Baker) Meeuwen, were further studied for their activity (IC50: 0.71 and 0.23 μg/ml respectively), toxicity and chemical composition. They were not toxic on hepatic cells and rich in polyphenols including tannins. The latter were removed from these extracts before retesting on HCV, showing that tannins were responsible for antiviral activity. These active tannins could be efficiently extracted with eco-friendly and traditionally used hydro-ethanolic solvents, resulting in similar antiviral activities compared to methanolic extracts, and higher antiviral activity compared to aqueous extracts. Among them, procyanidin A2 was identified in several extracts and the pure molecule was very active in our in vitro antiviral assay. Other tannins most likely contributed to this activity. Further studies should be carried out to discover other active tannins and to investigate extracts activity in vivo.
Data Availability Statement
The raw data supporting the conclusions of this article will be made available by the authors, without undue reservation.
Author Contributions
MB carried out the ethnobotanical survey, collected the plants, did the crude extractions and phytochemical screening; SB acquired financial support, supervised the phytochemical experiments, wrote the original draft, wrote and reviewed the final manuscript; M-ES carried out antiviral and cytotoxic assays; SM performed UPLC experiments and analysed data; JS carried out tannin removal and polyphenol dosage assays; VR contribute to the phytochemical experiments and the writing of the manuscript; PV carried out eco-extraction experiments; KD acquired financial support, followed the eco-extraction experiments; YR Contribute to antiviral and cytotoxic assays; JD acquired financial support; FT formulated the research goals and aims, acquired financial support, supervised the ethnobotanical survey, guaranteed the botanical identification of plant samples and processing of the voucher specimens, and the respect of biodiversity rights; KS Formulated the research goals and aims, carried out, verified and analysed antiviral and cytotoxic assays, wrote the original draft; SS Formulated the research goals and aims, guaranteed the respect of biodiversity rights, followed the study process, reviewed the final manuscript. All authors have read and agreed to the published version of the manuscript.
Funding
This research was funded by the PEPS funding given by the University of Lille and the CNRS. The authors also thank the Ivorian and French government. MB received financial support by the Ivorian Government. M-ES was a recipient of a Ph.D. Fellowship provided by the French Government.
Conflict of Interest
The authors declare that the research was conducted in the absence of any commercial or financial relationships that could be construed as a potential conflict of interest.
Publisher’s Note
All claims expressed in this article are solely those of the authors and do not necessarily represent those of their affiliated organizations, or those of the publisher, the editors, and the reviewers. Any product that may be evaluated in this article, or claim that may be made by its manufacturer, is not guaranteed or endorsed by the publisher.
Acknowledgments
The authors wish to thank the imaging core facility of the BioImaging Center Lille Nord-de-France for their help with the immunofluorescence analyses.
Supplementary Material
The Supplementary Material for this article can be found online at: https://www.frontiersin.org/articles/10.3389/fphar.2021.789688/full#supplementary-material
Abbreviations
CC50, Half-median Cytotoxic Concentration; DAAs: Direct-Acting Antivirals; DAPI, 4′,6-diamidino-2-phenylindole; DMACA, p-dimethylaminocinnamaldehyde DMEM, Dulbecco’s modified Eagle’s medium; DMSO, Dimethyl sulfoxide; DP, Degree of Polymerization; ECG, (-)-epicatechin-3-O-gallate; EGCG, epigallocatechin-3-gallate; ELISA, Enzyme-Linked Immunosorbent Assay; EtOAc, Ethyl acetate; ffu, focus forming unit; HCV, Hepatitis C virus; HCVcc, HCV cell culture; Huh, Human hepatocyte; IC50: Half-median Inhibitory Concentration; IgG, Immunoglobulin G; JFH1, Japanese Fulminant Hepatitis-1; MOI, Multiplicity of infection; MTS, 3-(4,5-dimethylthiazol-2-yl)-5-(3-carboxymethoxyphenyl)-2-(4-sulfophenyl)-2H-tetrazolium; NS (protease/polymerase): Non Structural; PBS, Phosphate buffered saline; TLC, Thin Layer Chromatography; TPS, Total Phenolic Solution; TPS-T, TPS without tannins; UPLC, Ultra-High Performance Liquid Chromatography; w/o, without.
References
Adamu, H. M., Abayeh, O. J., Agho, M. O., Abdullahi, A. L., Uba, A., Dukku, H. U., et al. (2005). An Ethnobotanical Survey of Bauchi State Herbal Plants and Their Antimicrobial Activity. J. Ethnopharmacol 99, 1–4. doi:10.1016/j.jep.2004.12.025
Adjé, F. A., Koffi, E. N., Koné, K. Y., Meudec, E., Adima, A. A., Lozano, P. R., et al. (2019). Polyphenol Characterization in Red Beverages of Carapa Procera (D.C) Leaf Extracts. Beverages 5, 68. doi:10.3390/beverages5040068
Agbodeka, K., Gbekley, H. E., Karou, S. D., Anani, K., Agbonon, A., Tchacondo, T., et al. (2016). Ethnobotanical Study of Medicinal Plants Used for the Treatment of Malaria in the Plateau Region, Togo. Pharmacognosy Res. 8, S12–S18. doi:10.4103/0974-8490.178646
Ajala, O. S., Jukov, A., and Ma, C. M. (2014). Hepatitis C Virus Inhibitory Hydrolysable Tannins from the Fruits of Terminalia Chebula. Fitoterapia 99, 117–123. doi:10.1016/j.fitote.2014.09.014
Alhaji, U. I., Samuel, N. U., Aminu, M., Chidi, A. V., Umar, Z. U., Umar, U. A., et al. (2014). In Vitro antitrypanosomal Activity, Antioxidant Property and Phytochemical Constituents of Aqueous Extracts of Nine Nigerian Medicinal Plants. Asian Pac. J. Trop. Dis. 4, 348–355. doi:10.1016/S2222-1808(14)60586-7
Asase, A., Oteng-Yeboah, A. A., Odamtten, G. T., and Simmonds, M. S. (2005). Ethnobotanical Study of Some Ghanaian Anti-malarial Plants. J. Ethnopharmacol 99, 273–279. doi:10.1016/j.jep.2005.02.020
Bla, K., Trebissou, J., Bidie, A., Assi, Y., Zihiri-Guede, N., and Djaman, A. (2015). Étude ethnopharmacologique des plantes antipaludiques utilisées chez les Baoulé- N'Gban de Toumodi dans le Centre de la Côte d'Ivoire. J. App. Biosci. 85, 7775. doi:10.4314/jab.v85i1.4
Bremer Christensen, C., Soelberg, J., Stensvold, C. R., and Jäger, A. K. (2015). Activity of Medicinal Plants from Ghana against the Parasitic Gut Protist Blastocystis. J. Ethnopharmacol 174, 569–575. doi:10.1016/j.jep.2015.03.006
Calland, N., Albecka, A., Belouzard, S., Wychowski, C., Duverlie, G., Descamps, V., et al. (2012a). (-)-Epigallocatechin-3-gallate Is a New Inhibitor of Hepatitis C Virus Entry. Hepatology 55, 720–729. doi:10.1002/hep.24803
Calland, N., Dubuisson, J., Rouillé, Y., and Séron, K. (2012b). Hepatitis C Virus and Natural Compounds: a New Antiviral Approach. Viruses 4, 2197–2217. doi:10.3390/v4102197
Calland, N., Sahuc, M. E., Belouzard, S., Pène, V., Bonnafous, P., Mesalam, A. A., et al. (2015). Polyphenols Inhibit Hepatitis C Virus Entry by a New Mechanism of Action. J. Virol. 89, 10053–10063. doi:10.1128/JVI.01473-15
Chemat, F., Abert-Vian, M., Fabiano-Tixier, A. S., Strube, J., Uhlenbrock, L., Gunjevic, V., et al. (2019). Green Extraction of Natural Products. Origins, Current Status, and Future Challenges. Trac Trends Anal. Chem. 118, 248–263. doi:10.1016/j.trac.2019.05.037
Chowdhury, P., Sahuc, M. E., Rouillé, Y., Rivière, C., Bonneau, N., Vandeputte, A., et al. (2018). Theaflavins, Polyphenols of Black tea, Inhibit Entry of Hepatitis C Virus in Cell Culture. PLoS One 13, e0198226. doi:10.1371/journal.pone.0198226
Ciesek, S., von Hahn, T., Colpitts, C. C., Schang, L. M., Friesland, M., Steinmann, J., et al. (2011). The green tea Polyphenol, Epigallocatechin-3-Gallate, Inhibits Hepatitis C Virus Entry. Hepatology 54, 1947–1955. doi:10.1002/hep.24610
Delgrange, D., Pillez, A., Castelain, S., Cocquerel, L., Rouillé, Y., Dubuisson, J., et al. (2007). Robust Production of Infectious Viral Particles in Huh-7 Cells by Introducing Mutations in Hepatitis C Virus Structural Proteins. J. Gen. Virol. 88, 2495–2503. doi:10.1099/vir.0.82872-0
Diarra, N., Klooster, C. V., Togola, A., Diallo, D., Willcox, M., and Jong, Jd. (2015). Ethnobotanical Study of Plants Used against Malaria in Sélingué Subdistrict, Mali. J. Ethnopharmacol 166, 352–360. doi:10.1016/j.jep.2015.02.054
Dubuisson, J., Hsu, H. H., Cheung, R. C., Greenberg, H. B., Russell, D. G., and Rice, C. M. (1994). Formation and Intracellular Localization of Hepatitis C Virus Envelope Glycoprotein Complexes Expressed by Recombinant Vaccinia and Sindbis Viruses. J. Virol. 68, 6147–6160. doi:10.1128/JVI.68.10.6147-6160.1994
Fadipe, L. A., Babayi, H., and Anselm, O. A. (2019). Isolation and In-Vitro Assessment of Two Indole Alkaloids from Pericopsis Laxiflora Leaf Extract for Their Antibacterial Potentials. J. Chem. Soc. Nigeria 44, 7.
Falade-Nwulia, O., Suarez-Cuervo, C., Nelson, D. R., Fried, M. W., Segal, J. B., and Sulkowski, M. S. (2017). Oral Direct-Acting Agent Therapy for Hepatitis C Virus Infection: A Systematic Review. Ann. Intern. Med. 166, 637–648. doi:10.7326/M16-2575
Farahmandfar, R., Esmaeilzadeh Kenari, R., Asnaashari, M., Shahrampour, D., and Bakhshandeh, T. (2019). Bioactive Compounds, Antioxidant and Antimicrobial Activities of Arum Maculatum Leaves Extracts as Affected by Various Solvents and Extraction Methods. Food Sci. Nutr. 7, 465–475. doi:10.1002/fsn3.815
Fauvelle, C., Lambotin, M., Heydmann, L., Prakash, E., Bhaskaran, S., Vishwaraman, M., et al. (2017). A Cinnamon-Derived Procyanidin Type A Compound Inhibits Hepatitis C Virus Cell Entry. Hepatol. Int. 11, 440–445. doi:10.1007/s12072-017-9809-y
Goueslain, L., Alsaleh, K., Horellou, P., Roingeard, P., Descamps, V., Duverlie, G., et al. (2010). Identification of GBF1 as a Cellular Factor Required for Hepatitis C Virus RNA Replication. J. Virol. 84, 773–787. doi:10.1128/JVI.01190-09
Graham, C. S., and Swan, T. (2015). A Path to Eradication of Hepatitis C in Low- and Middle-Income Countries. Antivir. Res 119, 89–96. doi:10.1016/j.antiviral.2015.01.004
Haidara, M., Bourdy, G., De Tommasi, N., Braca, A., Traore, K., Giani, S., et al. (2016). Medicinal Plants Used in Mali for the Treatment of Malaria and Liver Diseases. Nat. Prod. Commun. 11, 339–352. doi:10.1177/1934578x1601100309
Ishola, I. O., Oreagba, I. A., Adeneye, A. A., Adirije, C., Oshikoya, K. A., and Ogunleye, O. O. (2014). Ethnopharmacological Survey of Herbal Treatment of Malaria in Lagos, Southwest Nigeria. J. Herbal Med. 4, 224–234. doi:10.1016/j.hermed.2014.08.001
Jardim, A. C. G., Shimizu, J. F., Rahal, P., and Harris, M. (2018). Plant-derived Antivirals against Hepatitis C Virus Infection. Virol. J. 15, 34. doi:10.1186/s12985-018-0945-3
Jiofack, T., Ayissi, I., Fokunang, C., Guedje, N., and Kemeuze, V. (2009). Ethnobotany and Phytomedicine of the Upper Nyong valley forest in Cameroon. Afr. J. Pharm. Pharmacol. 3, 144–150.
Kamaraj, C., Kaushik, N. K., Rahuman, A. A., Mohanakrishnan, D., Bagavan, A., Elango, G., et al. (2012). Antimalarial Activities of Medicinal Plants Traditionally Used in the Villages of Dharmapuri Regions of South India. J. Ethnopharmacol 141, 796–802. doi:10.1016/j.jep.2012.03.003
Koama, K., Serge Yerb, R., Roland Med, N. T., Ouedraogo, N., Da, O., Bosco Oued, J., et al. (2021)). In Vivo Antimalarial, Antioxidant Activities and Safety of Carapa Procera DC. (Meliaceae). Pakistan J. Biol. Sci. 24, 571–578. doi:10.3923/pjbs.2021.571.578
Koffi, J. A., Silué, K. D., Tano, D. K., Dable, T. M., and Yavo, W. (2020). Evaluation of Antiplasmodial Activity of Extracts from Endemic Medicinal Plants Used to Treat Malaria in Côte d'Ivoire. Bioimpacts 10, 151–157. doi:10.34172/bi.2020.19
Koné, W. M., Atindehou, K. K., Terreaux, C., Hostettmann, K., Traoré, D., and Dosso, M. (2004). Traditional Medicine in north Côte-d'Ivoire: Screening of 50 Medicinal Plants for Antibacterial Activity. J. Ethnopharmacol 93, 43–49. doi:10.1016/j.jep.2004.03.006
Lea, A. G. (1978). The Phenolics of Ciders: Oligomeric and Polymeric Procyanidins. J. Sci. Food Agric. 29, 471–477. doi:10.1002/jsfa.2740290511
Levrero, M. (2006). Viral Hepatitis and Liver Cancer: the Case of Hepatitis C. Oncogene 25, 3834–3847. doi:10.1038/sj.onc.1209562
Li, S., Kodama, E. N., Inoue, Y., Tani, H., Matsuura, Y., Zhang, J., et al. (2010). Procyanidin B1 Purified from Cinnamomi Cortex Suppresses Hepatitis C Virus Replication. Antivir. Chem. Chemother. 20, 239–248. doi:10.3851/IMP1597
Lin, L. T., Chen, T. Y., Lin, S. C., Chung, C. Y., Lin, T. C., Wang, G. H., et al. (2013). Broad-spectrum Antiviral Activity of Chebulagic Acid and Punicalagin against Viruses that Use Glycosaminoglycans for Entry. BMC Microbiol. 13, 187. doi:10.1186/1471-2180-13-187
Liu, D., Deng, J., Joshi, S., Liu, P., Zhang, C., Yu, Y., et al. (2018). Monomeric Catechin and Dimeric Procyanidin B2 against Human Norovirus Surrogates and Their Physicochemical Interactions. Food Microbiol. 76, 346–353. doi:10.1016/j.fm.2018.06.009
Liu, S., Chen, R., and Hagedorn, C. H. (2015). Tannic Acid Inhibits Hepatitis C Virus Entry into Huh7.5 Cells. PLoS One 10, e0131358. doi:10.1371/journal.pone.0131358
Malan, D. F., Neuba, D. F., and Kouakou, K. L. (2015). Medicinal Plants and Traditional Healing Practices in Ehotile People, Around the Aby Lagoon (Eastern Littoral of Côte d'Ivoire). J. Ethnobiol. Ethnomed 11, 21. doi:10.1186/s13002-015-0004-8
Messina, J. P., Humphreys, I., Flaxman, A., Brown, A., Cooke, G. S., Pybus, O. G., et al. (2015). Global Distribution and Prevalence of Hepatitis C Virus Genotypes. Hepatology 61, 77–87. doi:10.1002/hep.27259
Metrouh-Amir, H., Duarte, C. M. M., and Maiza, F. (2015). Solvent Effect on Total Phenolic Contents, Antioxidant, and Antibacterial Activities of Matricaria Pubescens. Ind. Crops Prod. 67, 249–256. doi:10.1016/j.indcrop.2015.01.049
Nakabayashi, H., Taketa, K., Miyano, K., Yamane, T., and Sato, J. (1982). Growth of Human Hepatoma Cells Lines with Differentiated Functions in Chemically Defined Medium. Cancer Res. 42, 3858–3863.
Neuwinger, H. D. (1996). African Ethnobotany: Poisons and Drugs: Chemistry, Pharmacology, Toxicology. London, New York: Chapman & Hall.
Newman, D. J., and Cragg, G. M. (2020). Natural Products as Sources of New Drugs over the Nearly Four Decades from 01/1981 to 09/2019. J. Nat. Prod. 83, 770–803. doi:10.1021/acs.jnatprod.9b01285
Odugbemi, T. O., Akinsulire, O. R., Aibinu, I. E., and Fabeku, P. O. (2006). Medicinal Plants Useful for Malaria Therapy in Okeigbo, Ondo State, Southwest Nigeria. Afr. J. Tradit Complement. Altern. Med. 4, 191–198. doi:10.4314/ajtcam.v4i2.31207
Okpekon, T., Yolou, S., Gleye, C., Roblot, F., Loiseau, P., Bories, C., et al. (2004). Antiparasitic Activities of Medicinal Plants Used in Ivory Coast. J. Ethnopharmacol 90, 91–97. doi:10.1016/j.jep.2003.09.029
Oliveira, F., Aguiar, P., Ribeiro, G., de, M., Guimaraes, P., Brandao, M., et al. (2015). Antioxidant Activity and Phytochemical Screening of Extracts of Erythroxylum Suberosum A.St.-Hil (Erythroxylaceae). Res. J. Phytochemistry 9, 68–78. doi:10.3923/rjphyto.2015.68.78
Oppong Bekoe, E., Agyare, C., Boakye, Y. D., Baiden, B. M., Asase, A., Sarkodie, J., et al. (2020). Ethnomedicinal Survey and Mutagenic Studies of Plants Used in Accra metropolis, Ghana. J. Ethnopharmacol 248, 112309. doi:10.1016/j.jep.2019.112309
Patil, V. M., Masand, N., and Gupta, S. P. (2016). HCV Inhibitors: Role of Compounds from Botanical Sources. Curr. Top. Med. Chem. 16, 1402–1409. doi:10.2174/1568026616666151120112802
Pawlotsky, J. M. (2016). Hepatitis C Virus Resistance to Direct-Acting Antiviral Drugs in Interferon-free Regimens. Gastroenterology 151, 70–86. doi:10.1053/j.gastro.2016.04.003
Pham, A. T., Dvergsnes, C., Togola, A., Wangensteen, H., Diallo, D., Paulsen, B. S., et al. (2011). Terminalia Macroptera, its Current Medicinal Use and Future Perspectives. J. Ethnopharmacol 137, 1486–1491. doi:10.1016/j.jep.2011.08.029
Polaris Observatory HCV Collaborators (2017). Global Prevalence and Genotype Distribution of Hepatitis C Virus Infection in 2015: a Modelling Study. Lancet Gastroenterol. Hepatol. 2, 161–176. doi:10.1016/S2468-1253(16)30181-9
Reddy, B. U., Mullick, R., Kumar, A., Sudha, G., Srinivasan, N., and Das, S. (2014). Small Molecule Inhibitors of HCV Replication from Pomegranate. Sci. Rep. 4, 5411. doi:10.1038/srep05411
Riou, J., Aït Ahmed, M., Blake, A., Vozlinsky, S., Brichler, S., Eholié, S., et al. (2016). Hepatitis C Virus Seroprevalence in Adults in Africa: a Systematic Review and Meta-Analysis. J. Viral Hepat. 23, 244–255. doi:10.1111/jvh.12481
Rouillé, Y., Helle, F., Delgrange, D., Roingeard, P., Voisset, C., Blanchard, E., et al. (2006). Subcellular Localization of Hepatitis C Virus Structural Proteins in a Cell Culture System that Efficiently Replicates the Virus. J. Virol. 80, 2832–2841. doi:10.1128/JVI.80.6.2832-2841.2006
Rusaati, B. I. W. (2021). A Systematic Review of Antimalarial Medicinal Plants in Democratic Republic of the Congo | BOIS & FORETS DES TROPIQUES. Available at: https://revues.cirad.fr/index.php/BFT/article/view/31882 (Accessed July 19, 2021).
Santos, D. K. D. D. N., de Almeida, V. S., de Araujo, D. R. C., Harand, W., Soares, A. K. A., Moreira, L. R., et al. (2018). Evaluation of Cytotoxic, Immunomodulatory and Antibacterial Activities of Aqueous Extract from Leaves of Conocarpus Erectus Linnaeus (Combretaceae). J. Pharm. Pharmacol. 70, 1092–1101. doi:10.1111/jphp.12930
Sarpong, I. M., Sarpong, F. M., and Amponsah, I. E. (2016). Antiplasmodial Activity of the Leaves and Stem Bark Ofcarapa Procera and Alstonia Boonei. Der Pharmacia Lettre 8, 116–122.
Séron, K., Sahuc, M.-E., and Rouillé, Y. (2018). “Natural Products and Hepatitis C Virus,” in Natural Antimicrobial Agents. Editors J.-M. Mérillon, and C. Rivière, 289–327. doi:10.1007/978-3-319-67045-4_12
Smeriglio, A., Barreca, D., Bellocco, E., and Trombetta, D. (2017). Proanthocyanidins and Hydrolysable Tannins: Occurrence, Dietary Intake and Pharmacological Effects. Br. J. Pharmacol. 174, 1244–1262. doi:10.1111/bph.13630
Stangeland, T., Alele, P. E., Katuura, E., and Lye, K. A. (2011). Plants Used to Treat Malaria in Nyakayojo Sub-county, Western Uganda. J. Ethnopharmacol 137, 154–166. doi:10.1016/j.jep.2011.05.002
Tamura, S., Yang, G. M., Koitabashi, T., Matsuura, Y., Komoda, Y., Kawano, T., et al. (2019). Oenothein B, Dimeric Hydrolysable Tannin Inhibiting HCV Invasion from Oenothera Erythrosepala. J. Nat. Med. 73, 67–75. doi:10.1007/s11418-018-1239-1
Tarr, A. W., Khera, T., Hueging, K., Sheldon, J., Steinmann, E., Pietschmann, T., et al. (2015). Genetic Diversity Underlying the Envelope Glycoproteins of Hepatitis C Virus: Structural and Functional Consequences and the Implications for Vaccine Design. Viruses 7, 3995–4046. doi:10.3390/v7072809
Traore, M. S., Baldé, M. A., Diallo, M. S., Baldé, E. S., Diané, S., Camara, A., et al. (2013). Ethnobotanical Survey on Medicinal Plants Used by Guinean Traditional Healers in the Treatment of Malaria. J. Ethnopharmacol 150, 1145–1153. doi:10.1016/j.jep.2013.10.048
Traore, O., Ouedraogo, A., Compaore, M., Nikiema, K., Zombre, A., Kiendrebeogo, M., et al. (2021). Social Perceptions of Malaria and Diagnostic-Driven Malaria Treatment in Burkina Faso. Heliyon 7, e05553. doi:10.1016/j.heliyon.2020.e05553
Tsabang, N., Fokou, P. V., Tchokouaha, L. R., Noguem, B., Bakarnga-Via, I., Nguepi, M. S., et al. (2012). Ethnopharmacological Survey of Annonaceae Medicinal Plants Used to Treat Malaria in Four Areas of Cameroon. J. Ethnopharmacol 139, 171–180. doi:10.1016/j.jep.2011.10.035
Tshikalange, T. E., Mophuting, B. C., Mahore, J., Winterboer, S., and Lall, N. (2016). An Ethnobotanical Study of Medicinal Plants Used in Villages Under Jongilanga Tribal Council, Mpumalanga, South Africa. Afr. J. Tradit Complement. Altern. Med. 13, 83–89. doi:10.21010/ajtcam.v13i6.13
Wei, S.-D., Chen, R.-Y., Liao, M.-M., Tu, N.-W., Zhou, H.-C., and Lin, Y.-M. (2011). Antioxidant Condensed Tannins from Machilus Pauhoi Leaves. J. Med. Plants Res. 5, 796–804.
Wiesneth, S., and Jürgenliemk, G. (2017). Total Phenolic and Tannins Determination: a Modification of Ph. Eur. 2.8.14 for Higher Throughput. Pharmazie 72, 195–196. doi:10.1691/ph.2017.6911
Yang, Z. F., Bai, L. P., Huang, W. B., Li, X. Z., Zhao, S. S., Zhong, N. S., et al. (2014). Comparison of In Vitro Antiviral Activity of tea Polyphenols against Influenza A and B Viruses and Structure-Activity Relationship Analysis. Fitoterapia 93, 47–53. doi:10.1016/j.fitote.2013.12.011
Zhang, L., Wang, Y., Li, D., Ho, C. T., Li, J., and Wan, X. (2016). The Absorption, Distribution, Metabolism and Excretion of Procyanidins. Food Funct. 7, 1273–1281. doi:10.1039/C5FO01244A
Keywords: antiviral, tannins, traditional medicine, hepatitis C, Côte d'Ivoire, sustainable extractions
Citation: Bamba M, Bordage S, Sahuc M-E, Moureu S, Samaillie J, Roumy V, Vauchel P, Dimitrov K, Rouillé Y, Dubuisson J, Tra Bi FH, Séron K and Sahpaz S (2022) Anti-HCV Tannins From Plants Traditionally Used in West Africa and Extracted With Green Solvents. Front. Pharmacol. 12:789688. doi: 10.3389/fphar.2021.789688
Received: 19 October 2021; Accepted: 16 December 2021;
Published: 28 January 2022.
Edited by:
Matthias F. Melzig, Freie Universität Berlin, GermanyReviewed by:
Anna Karolina Kiss, Medical University of Warsaw, PolandKlaus Peter Latté, Independent researcher, Berlin, Germany
Copyright © 2022 Bamba, Bordage, Sahuc, Moureu, Samaillie, Roumy, Vauchel, Dimitrov, Rouillé, Dubuisson, Tra Bi, Séron and Sahpaz. This is an open-access article distributed under the terms of the Creative Commons Attribution License (CC BY). The use, distribution or reproduction in other forums is permitted, provided the original author(s) and the copyright owner(s) are credited and that the original publication in this journal is cited, in accordance with accepted academic practice. No use, distribution or reproduction is permitted which does not comply with these terms.
*Correspondence: Simon Bordage, c2ltb24uYm9yZGFnZUB1bml2LWxpbGxlLmZy
†These authors have contributed equally to this work and share first authorship
‡These authors have contributed equally to this work and share last authorship