- 1Institute of Antibiotics, Huashan Hospital, Fudan University, Shanghai, China
- 2Key Laboratory of Clinical Pharmacology of Antibiotics, Shanghai, China
- 3National Health Commission & National Clinical Research Center for Aging and Medicine, Huashan Hospital, Fudan University, Shanghai, China
- 4Department of Biological Medicines & Shanghai Engineering Research Center of Immunotherapeutics, School of Pharmacy, Fudan University, Shanghai, China
- 5Phase I Unit, Huashan Hospital, Fudan University, Shanghai, China
- 6Biomedicine Discovery Institute and Department of Microbiology, Monash University, Melbourne, VIC, Australia
The latest PK/PD findings have demonstrated negligible efficacy of intravenous polymyxins against pulmonary infections. We investigated pharmacokinetic/pharmacodynamic (PK/PD)-based breakpoints of polymyxin B for bloodstream infections and the rationality of the recent withdrawal of polymyxin susceptibility breakpoints by the CLSI. Polymyxin B pharmacokinetic data were obtained from a phase I clinical trial in healthy Chinese subjects and population pharmacokinetic parameters were employed to determine the exposure of polymyxin B at steady state. MICs of 1,431 recent clinical isolates of Pseudomonas aeruginosa, Acinetobacter baumannii and Klebsiella pneumoniae collected from across China were determined. Monte-Carlo simulations were performed for various dosing regimens (0.42–1.5 mg/kg/12 h via 1 or 2-h infusion). The probability of target attainment, PK/PD breakpoints and cumulative fraction of response were determined for each bacterial species. MIC90 of polymyxin B was 1 mg/L for P. aeruginosa and 0.5 mg/L for A. baumannii and K. pneumoniae. With the recommended polymyxin B dose of 1.5–2.5 mg/kg/day, the PK/PD susceptible breakpoints for P. aeruginosa, A. baumannii and K. pneumoniae were 2, 1 and 1 mg/L respectively for bloodstream infection. For Chinese patients, polymyxin B dosing regimens of 0.75–1.5 mg/kg/12 h for P. aeruginosa and 1–1.5 mg/kg/12 h for A. baumannii and K. pneumoniae were appropriate. Breakpoint determination should consider the antimicrobial PK/PD at infection site and delivery route. The recent withdrawal of polymyxin susceptible breakpoint by CLSI primarily based on poor efficacy against lung infections needs to be reconsidered for bloodstream infections.
Introduction
Carbapenem-resistant Gram-negative bacteria are a serious threat to global health. In China, approximately 20–30% of Pseudomonas aeruginosa and Klebsiella spp. and >70% of Acinetobacter spp. are now carbapenem resistant (http://www.chinets.com/). Elsewhere, the World Health Organization has reported high median resistance rates to carbapenems in several bacterial species globally, exemplified by resistance rates in Klebsiella spp. and Acinetobacter spp. isolated from bloodstream infections of ∼20 and ∼60%, respectively (2020; https://www.who.int/glass/resources/publications/early-implementation-report-2020/en/). Although the newly developed β-lactam/β-lactamase inhibitor combinations (such as ceftazidime-avibactam and ceftolozane-tazobactam) have mitigated the situation, none of them is active against metallo-β-lactamase-producing Enterobacterales, P. aeruginosa or carbapenemase-producing A. baumannii (Yahav et al., 2020). Given many of these carbapenem-resistant bacteria remain susceptible to polymyxins (polymyxin B and colistin), this once abandoned class of antibiotics is often the only viable treatment option were revived and being listed on the reserve list of antibiotics by WHO (Jian et al., 2019).
The polymyxins are cyclic lipopeptides naturally produced by the Gram-positive Paenibacillus polymyxa (Velkov et al., 2019). While various polymyxins have been described, only polymyxin B and E (the latter known as colistin) are available for clinical use (Ross et al., 1959; Clifford and Stewart, 1961). The antimicrobial activity is initiated by an electrostatic attraction, causing displacement of divalent cations (Ca2+ and Mg2+) that bridge adjacent LPS molecules and resulting in OM leaflet expansion, disruption of the membrane integrity, and increased membrane permeability (Berglund et al., 2015; Rabanal and Cajal, 2017). Prior to 2020, the Clinical and Laboratory Standards Institute (CLSI) provided susceptible and resistant breakpoints for colistin and polymyxin B (e.g., M100-S29). However, in 2020 the susceptible interpretive category (previously ≤2 mg/L in all cases) was removed (M100-S30). In contrast, the European Committee on Antimicrobial Susceptibility Testing (EUCAST) has maintained the “susceptible” category for colistin (polymyxin B breakpoints are not reported) (Version 10.0, 2020). The decision by the CLSI was based primarily on data suggesting that intravenous polymyxins have limited efficacy for the treatment of lung infections in mice and patients (Rigatto et al., 2013; Cheah et al., 2015; Landersdorfer et al., 2018; Satlin et al., 2020). Indeed, studies in animals clearly show reduced bacterial killing in lung infection models compared to thigh infection models with equivalent parenteral dosage regimens (Cheah et al., 2015; Landersdorfer et al., 2018), and substantially lower concentrations of polymyxin B in epithelial lining fluid (ELF) compared to plasma have been reported following intravenous administration (He et al., 2013). Such data clearly suggests relatively low unbound concentrations are achieved in pulmonary fluids (He et al., 2013). Given that higher polymyxin concentrations can be achieved elsewhere in the body (e.g., blood), the CLSI decision to remove the susceptible category for polymyxins based primarily on lung infection data may not be justified for other infection sites such as the bloodstream.
Various historical factors led to colistin being adopted far more widely than polymyxin B. Consequently, most existing polymyxin studies involve colistin and its inactive prodrug, colistin methanesulfonate (CMS) (Tsuji et al., 2019). Recently, however, the use of polymyxin B for treatment of systemic infections has increased, primarily due to better PK characteristics and relatively lower rates of nephrotoxicity than CMS (Aggarwal and Dewan, 2018; Tsuji et al., 2019). Unfortunately, very few clinical studies have utilized polymyxin B (Kvitko et al., 2011; Rigatto et al., 2013; Terayama et al., 2017). Given the circumstances described here, we investigated susceptibility breakpoints for polymyxin B for treatment of bloodstream infections caused by carbapenem-resistant Gram-negative bacteria and the rationality of the recent withdrawal of the ‘susceptible’ category for polymyxins by the CLSI. Clinical breakpoints were determined by epidemiological cut-offs, PK/PD breakpoints and clinical efficacy (Li et al., 2015). To acquire PK/PD breakpoints, we conducted a PK study in healthy Chinese volunteers (Liu et al., 2021) and collected 1,431 isolates of P. aeruginosa, A. baumannii and K. pneumoniae from patients across China for PK/PD analysis. This study will provide useful information of PK/PD breakpoints for polymyxin B in patients and evaluate polymyxin dosing regimens for bloodstream infections caused by different bacterial species.
Materials and Methods
Pharmacokinetics of Polymyxin B
A single-center, randomized, open-label phase I clinical trial of intravenous polymyxin B (0.75 and 1.5 mg/kg) were conducted in healthy Chinese subjects (Liu et al., 2021). The liquid chromatography-tandem mass spectrometry (LC-MS/MS) assay of polymyxin B was employed to determine the concentrations as previously reported (Liu et al., 2020). Non-compartment analysis was employed to calculate the AUC0-inf of polymyxin B in WinNonlin (v8.0, Pharsight, United States), which was linear with dose according to a power model with a 95% confidence interval (0.94, 1.2). Population pharmacokinetic (PPK) analysis was conducted using NONMEM 7.4 (Icon Development Solutions, Ellicott City, MD) with G77 FORTRAN complier and FOCEI algorithm. The base model of polymyxin B was fit into a three-compartment model. The interindividual and residual variabilities were best described by an exponential model and a proportional model, respectively. Age and gender were included in the final PK model (Liu et al., 2021).
Microbiological Information
A total of 1,431 clinical isolates (517 strains of P. aeruginosa, 262 of A. baumannii and 652 of K. pneumoniae) were collected across 2017–2019 from more than 30 teaching hospitals in 23 provinces across China. Polymyxin B and meropenem minimum inhibitory concentrations (MICs) were determined by broth microdilution and interpreted according to the CLSI breakpoints (M100-S30, 2020). Escherichia coli ATCC 25922, E. coli ATCC 35218 and K. pneumoniae ATCC 700603 acted as quality control strains. Statistical analysis was performed using WHONET (version 5.6). The study protocol was approved by the Institutional Review Board of Huashan Hospital, Fudan University (no. 2018-408, no. 2019-460).
Pharmacokinetic/Pharmacodynamic Targets for Polymyxin B Against the Three Gram-Negative Pathogens
The area under the unbound concentration-time curve over 24 h to the MIC ratio (fAUC0–24h/MIC) is the most predictive PK/PD index for polymyxins (Tam et al., 2005; Bergen et al., 2008; Bergen et al., 2010; Cheah et al., 2015; Landersdorfer et al., 2018). PK/PD targets of 1-log10 CFU and 2-log10 CFU reductions in colony forming units (CFU)/thigh were derived from dose-fractionation studies of polymyxin B (K. pneumoniae) and colistin (P. aeruginosa and A. baumannii) in murine thigh infection models (Supplementary Table S1; Cheah et al., 2015; Landersdorfer et al., 2018). Given polymyxin B and colistin have essentially identical in vitro potencies and spectra of antibacterial activity (Gales et al., 2011), the colistin targets were adopted for polymyxin B. An unbound fraction (f) of polymyxin B in plasma of 42% was applied (Sandri et al., 2013).
Probability of Target Attainment, Pharmacokinetic/Pharmacodynamic Breakpoints and Cumulative Fraction of Response
Four thousand data sets were generated with the final PPK model based on the estimated PPK parameters for each dosage regimen ranging from 0.42 to 1.5 mg/kg/12 h (administered via a 1- or 2-h infusion). Mean and SD of AUC0–24,ss were calculated using the simulated AUC0-12,ss. Monte-Carlo simulations for PTA and CFR were performed using MATLAB (Mathworks, United States, version 7.0.1). The add-in macro permitted the MATLAB program to perform Monte-Carlo simulations for 10,000 simulated data sets (AUC0–24,ss ± SD). The upper limit of the MIC range was taken as the PK/PD breakpoint when PTA was >90%. MIC distributions of polymyxin B for each bacterial species were used to determine the CFR, with a CFR of 90% considered effective.
Results
Susceptibility and PK Data
Polymyxin B MIC distributions and MIC50/MIC90 values for the 1,431 clinical isolates are shown in Table 1. MICs ranged between 0.125 and >32 mg/L for P. aeruginosa, 0.25 to >32 mg/L for A. baumannii, and 0.25–16 mg/L for K. pneumoniae. Meropenem resistance was detected in 25.9, 85.5 and 37.0% of P. aeruginosa, A. baumannii and K. pneumoniae isolates, respectively. The AUC0–24h,ss achieved with different polymyxin B dosing regimens (0.42–1.5 mg/kg/12 h) and infusion times (1 or 2 h) are shown in Supplementary Table S2. Steady-state exposures (range, 26.2–93.7 mg h/L) increased in proportion to the dose.

TABLE 1. Polymyxin B MIC distributions for P. aeruginosa, A. baumannii and K. pneumoniae.a
Pharmacokinetic/Pharmacodynamic Analysis of Polymyxin B With Different Dosing Regimens
Figures 1–3 and Supplementary Table S3 show the MIC distributions and PTA for each dosing regimen at steady state against strains of each bacterial species with diverse MICs. For P. aeruginosa, the fAUC0–24h/MIC required to achieve 1- and 2-log10 CFU killing (fAUC0–24h/MIC of 10 and 13.5, respectively) were achieved in 100% of cases for MICs ≤0.5 and ≤1 mg/L with the lower-dose regimens (0.42 and 0.75 mg/kg/12 h, respectively). However, with these regimens the PTA decreased dramatically as MICs increased above these values. For an MIC of 2 mg/L, the 1 mg/kg/12 h dosing regimen was effective with a PTA for 1-log10 CFU killing of 99% and the PTA for the 1.25 mg/kg/12 h dosing regimen was 95.1% to achieve 2- log10 CFU killing (Figure 1).
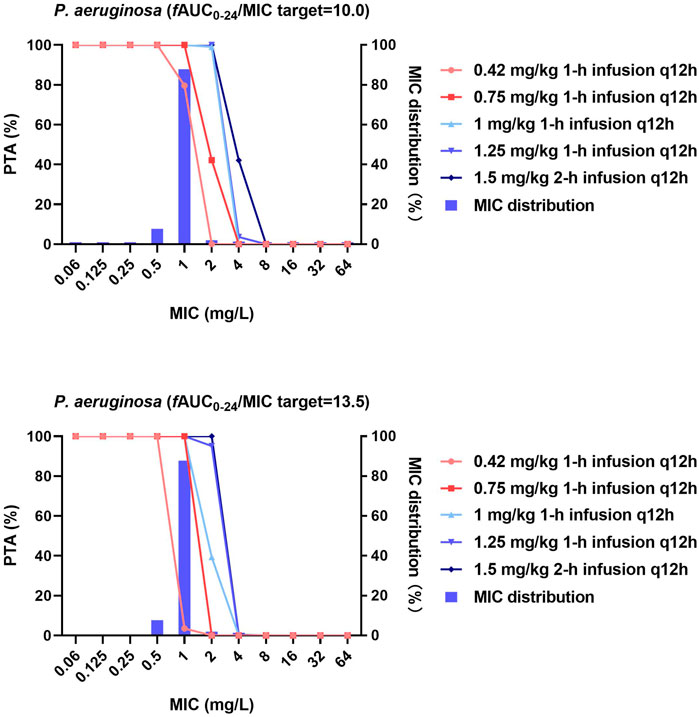
FIGURE 1. MIC distribution and PTA of different polymyxin B dosing regimens against P. aeruginosa. MICs of >32 mg/L are represented by 64 mg/L and PTA was determined using the AUC0–24h at steady state. The fAUC0–24h/MIC targets of 10 and 13.5 represent 1-log10 CFU and 2-log10 CFU reductions, respectively.
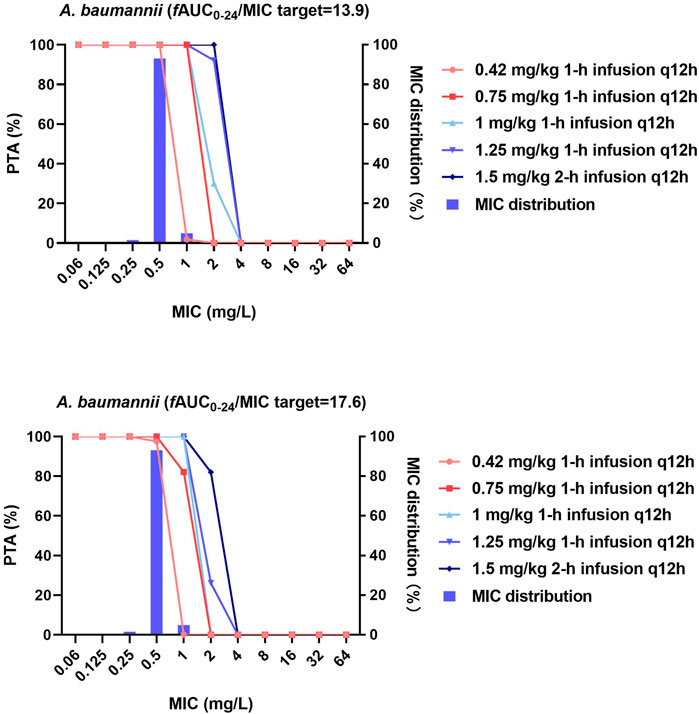
FIGURE 2. MIC distribution and PTA of different dosing regimens against A. baumannii. MICs of >32 mg/L are represented by 64 mg/L; PTA was determined using the AUC0–24h at steady state. The fAUC0–24h/MIC targets of 13.9 and 17.6 represent 1-log10 CFU and 2-log10 CFU reductions, respectively.
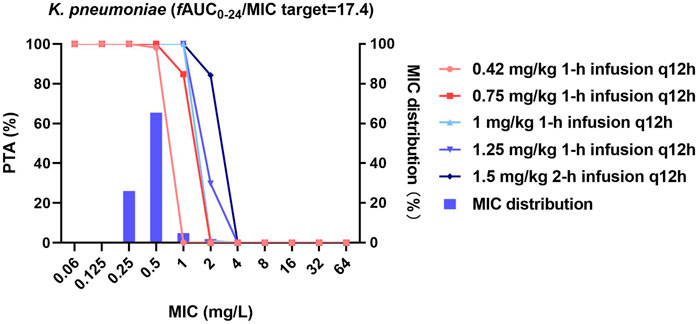
FIGURE 3. MIC distribution and PTA of different dosing regimens against K. pneumoniae. MICs of >32 mg/L are represented by 64 mg/L; PTA was determined using the AUC0–24h at steady state. The fAUC0–24h/MIC target of 17.4 represents a 1-log10 CFU reduction.
Against A. baumannii, both the 0.42 and 0.75 mg/kg/12 h regimens at steady state effectively achieved the fAUC0–24h/MIC target of 17.6 (2-log10 CFU reduction) against strains with MICs ≤0.5 mg/L (PTA = 97.5 and 100%), while the remaining dosing regimens were additionally effective against strains with an MIC ≤1 mg/L (PTA = 100%). No dosing regimen achieved the fAUC0–24h/MIC target of 17.6 when the MIC was ≥2 mg/L.
For K. pneumoniae, 0.42 and 0.75 mg/kg/12 h achieved the fAUC0–24h/MIC target of 17.4 in 98.1 and 100% of cases against strains with MICs ≤0.5 mg/L, respectively. All higher-dose regimens were additionally effective against strains with an MIC of ≤1 mg/L (PTA = 100%, Figure 3).
With the exception of the lowest dose regimen (0.42 mg/kg/12 h) against P. aeruginosa, all dosing regimens achieved a CFR >90% for all three bacterial species (MICs ranging from 0.125 to >32 mg/L; Table 2). The maximum treatable MICs for each bacterial species with the examined dosing regimens are shown in Table 3. Based on the dosing regimens of polymyxin B recommended throughout most of the world (1.5–2.5 mg/kg/day) and the highest fAUC0–24h/MIC target value, the PK/PD susceptibility breakpoints for P. aeruginosa, A. baumannii and K. pneumoniae were 2, 1 and 1 mg/L, respectively, for bloodstream infection. These breakpoints are higher than MIC90 values of current clinical isolates of all three bacterial species in China (Table 1), indicating the potential usefulness of polymyxin B for treatment of bloodstream infections caused by these bacteria.
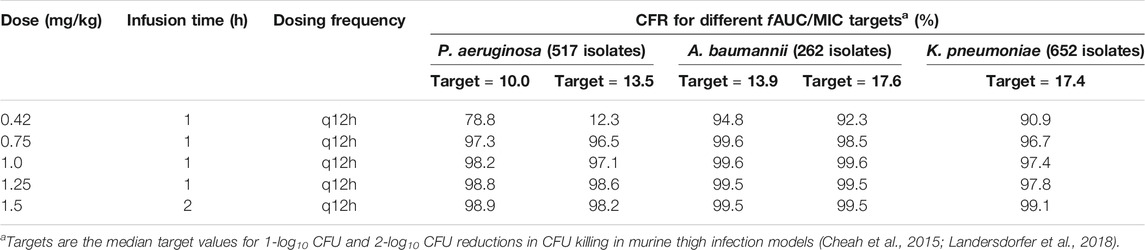
TABLE 2. Cumulative fraction of response (CFR) to different dosing regimens of polymyxin B against P. aeruginosa, A. baumannii and K. pneumoniae.
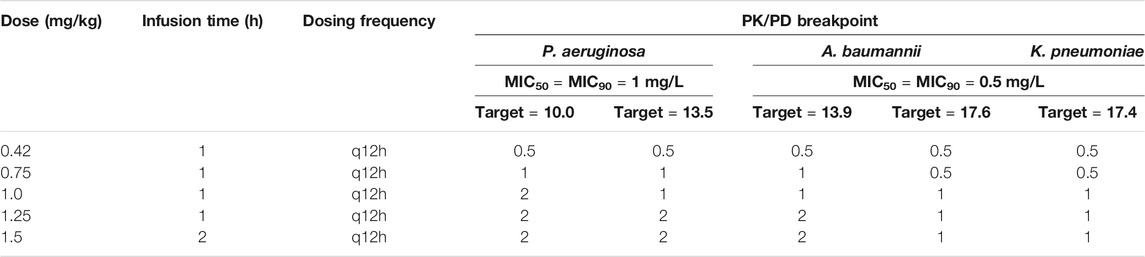
TABLE 3. Susceptible breakpoints for isolates of P. aeruginosa, A. baumannii and K. pneumoniae based on the PK/PD of polymyxin B.
Discussion
Removal of the “susceptible” interpretation category for polymyxins by CLSI was driven primarily by lung infection data (He et al., 2013; Landersdorfer et al., 2018). However, polymyxins exhibit concentration-dependent killing against Gram-negative bacteria and efficacy is highly dependent upon concentrations achieved at the target site (Lee et al., 2019). For bloodstream infections, the average steady-state concentrations (Css,avg) achieved with intravenously administered polymyxin B (0.45–3.38 mg/kg/day) or CMS (2–18 MIU/day) are 2–3 mg/L (polymyxin B and colistin) in critically-ill patients (Sandri et al., 2013; Nation et al., 2017). Thus, polymyxins may still be an appropriate therapeutic choice for bloodstream infections. The recent removal of the breakpoints for polymyxins by CLSI has caused significant uncertainties regarding their efficacy against bloodstream infections caused by the three aforementioned problematic Gram-negative bacteria. Therefore, we collected MIC data in the latest surveillance program in China and evaluated the rationality of the CLSI breakpoint modification for bloodstream infections.
In China, the MIC90 of polymyxin B for the three bacterial species were relatively low (1 mg/L for P. aeruginosa and 0.5 mg/L for A. baumannii and K. pneumoniae; Table 1) and the 0.75 mg/kg/12 h regimen was effective against >90% of pathogens. However, the success of this regimen will depend on regional susceptibility data. According to the SENTRY antimicrobial surveillance program for bloodstream infections (data collected from 45 nations including China between 1997 and 2016) (Diekema et al., 2019), the MIC90 of colistin for P. aeruginosa (7,107 isolates), A. baumannii or A. calcoaceticus (3,124 isolates) and Enterobacteriaceae (54,476 isolates) was 2, 2, and >4 mg/L, respectively. Given substantial regional susceptibility differences, effective therapeutic regimens must consider the regional susceptibility of the bacterial species involved.
In a recent Chinese study investigating the clinical efficacy and safety of polymyxin B-based regimens (100–200 mg/day, ≥5 days) for treatment of bloodstream infection caused by extensively-drug resistant Gram-negative bacteria, the clearance rate of microorganisms was 65.2%, overall effectiveness (cure or improvement) 59.0%, and 28-day all-cause mortality 41.0% (Zhao et al., 2020). Importantly, both effectiveness and microbial clearance were significantly higher with higher daily doses of polymyxin B (150 and 200 mg) compared to the lowest daily dose (100 mg). This is in agreement with other clinical data which support the use of high doses of polymyxin B for bloodstream infections (Elias et al., 2010; Cai et al., 2020).
The recommended dose of intravenously administered polymyxin B throughout much of the world is 1.5–2.5 mg/kg/day with a loading dose of 2–2.5 mg/kg (Tsuji et al., 2019). In China, the only brand of polymyxin B currently available has a recommended intravenous dose of 50–100 mg/day (Polymyxin B for injection (Daily Med, 2017), equivalent to 0.83–1.66 mg/kg/day (0.42–0.83 mg/kg/12 h) for a 60 kg patient. Importantly, Lakota et al. (2018) proposed that for pathogens with MICs ≤2 mg/L, the target AUC0–24h,ss for polymyxin B should be 50–100 mg h/L. In our study, the polymyxin B AUC0–24h,ss achieved with the 0.42 and 0.75 mg/kg/12 h dosing regimens fell below this target range (AUC0–24h,ss of 26.2 and 46.9 mg h/L, respectively; Supplementary Table S2), indicating that the recommended dose in China is very likely insufficient. Loading doses have no impact on the exposure at steady state but in favour of the rapid achievement to the effective concentrations at day 1. From our previous population pharmacokinetic analysis, gender and age are covariates in the final model (Liu et al., 2021). However, both of them had no significant effect on the AUC0–24h,ss. If the new CLSI breakpoints (M100-S30, 2020) are employed, the recent MIC data collected in Chinese hospitals showed that most isolates of P. aeruginosa, A. baumannii, and K. pneumoniae (MIC90 values of 1, 0.5, and 0.5 mg/L, respectively) would have intermediate susceptibility to polymyxin B. However, further PK/PD analysis showed that increasing doses could be effective against strains with MICs up to 2 mg/L. Taking decreases in bacterial counts of 1-log10 CFU (for K. pneumoniae) or 2-log10 CFU (for P. aeruginosa and A. baumannii) kill as the target values, dosing regimens of ≥0.75 mg/kg/12 h could be effective against P. aeruginosa with MIC of 1 mg/L; for A. baumannii and K. pneumoniae, all dosing regimens were effective against isolates with MIC ≤0.5 mg/L. One PK/PD study on polymyxin B for bloodstream infections has been conducted though the rationality of the present CLSI breakpoints were not well explained which is a valuable reference in clinical practice (Wu et al., 2021). In addition, the possible effects from variabilities of protein binding and PK/PD targets were not fully investigated. Based on the recommended polymyxin B dose of 1.5–2.5 mg/kg/day, we have proposed here PK/PD susceptibility breakpoints for P. aeruginosa, A. baumannii and K. pneumoniae of 2, 1 and 1 mg/L, respectively. Thus, EUCAST interpretation standards would be recommended in terms of PK/PD. Given strain-to-strain variability in PD targets, we conducted sensitivity analysis using different PK/PD targets (7.4 for P. aeruginosa and A. baumannii and 28.0 for K. pneumoniae). The breakpoints for P. aeruginosa, A. baumannii and K. pneumoniae were 2, 2 and 1 mg/L, respectively. The variable protein binding may affect the PK/PD breakpoints as well. According to the reported protein binding of 78.5–92.4% (Zavascki et al., 2008), the breakpoints were 1, 0.5, 0.5 mg/L assuming protein binding rate was 78.5% and 0.25, 0.25, 0.25 mg/L using the value of 92.4% for P. aeruginosa, A. baumannii and K. pneumoniae. The protein binding of less than 80% is acceptable for treating infections caused by these three bacterial species. Clearly, for all three pathogens polymyxin B would not be recommended for the treatment of bloodstream infections when the pathogen MIC is >2 mg/L.
Xie et al. compared the efficacy and safety of polymyxin B in various patient populations (Xie et al., 2020). Exposure was noticeably influenced by patient’ body weight when doses were calculated in mg/kg. The lower exposures achieved in patients with less body weight such as 50 kg in comparison to patients with body weight of 75–100 kg are more likely to result in treatment failure. This is important to note the recommended dose of polymyxin B in China (50–100 mg/day) is not based on body weight. The lower end of the recommended dosing range in most Western countries (1.5 mg/kg/day) equals to 90 mg/day for a 60 kg patient. Utilizing polymyxin B over 90 mg/kg is essential in terms of PK/PD.
In summary, the determination of breakpoints should be species- and infection-site specific and underpinned by solid PK/PD data. Given the polymyxins remain important last-line antibiotics for the treatment of infections caused by carbapenem-resistant Gram-negative bacteria, appropriate PK/PD considerations must be given when determining their breakpoints and dosage regimens. Adequate doses (i.e., 90–100 mg/day) should be utilized in terms of Chinese recommended dosing range. The revival of CLSI susceptibility breakpoint of polymyxins for bloodstream infections is potential in the future.
Data Availability Statement
The original contributions presented in the study are included in the article/Supplementary Material, further inquiries can be directed to the corresponding authors.
Ethics Statement
The studies involving human participants were reviewed and approved by the Institutional Review Board of Huashan Hospital, Fudan University. Written informed consent from the participants’ legal guardian/next of kin was not required to participate in this study in accordance with the national legislation and the institutional requirements.
Author Contributions
XCB, BP, and JL wrote the manuscript; JZ, FPH, and MQF designed the research; XCB, XFL, and YG performed the research; XCB, XFL, and XL analyzed the data; YCC contributed new reagents/analytical tools.
Funding
This research was supported by the Science and Technology Commission of Shanghai Municipality (grant number 17DZ1910402, 17DZ1910403 and 19411964900); Major Research and Development Project of Innovative Drugs, Ministry of Science and Technology of China (grant number 2017ZX09304005).
Conflict of Interest
The authors declare that the research was conducted in the absence of any commercial or financial relationships that could be construed as a potential conflict of interest.
Publisher’s Note
All claims expressed in this article are solely those of the authors and do not necessarily represent those of their affiliated organizations, or those of the publisher, the editors and the reviewers. Any product that may be evaluated in this article, or claim that may be made by its manufacturer, is not guaranteed or endorsed by the publisher.
Acknowledgments
This work was performed in Huashan Hospital affiliated to Fudan University; the pharmacokinetic study was conducted in the Phase I Unit and the pharmacodynamic data were acquired from the Clinical Microbiology Laboratory, Huashan Hospital.
Supplementary Material
The Supplementary Material for this article can be found online at: https://www.frontiersin.org/articles/10.3389/fphar.2021.785893/full#supplementary-material
References
Aggarwal, R., and Dewan, A. (2018). Comparison of Nephrotoxicity of Colistin with Polymyxin B Administered in Currently Recommended Doses: A Prospective Study. Ann. Clin. Microbiol. Antimicrob. 17 (1), 15. doi:10.1186/s12941-018-0262-0
Bergen, P. J., Bulitta, J. B., Forrest, A., Tsuji, B. T., Li, J., and Nation, R. L. (2010). Pharmacokinetic/pharmacodynamic Investigation of Colistin against Pseudomonas aeruginosa Using an In Vitro Model. Antimicrob. Agents Chemother. 54 (9), 3783–3789. doi:10.1128/AAC.00903-09
Bergen, P. J., Li, J., Nation, R. L., Turnidge, J. D., Coulthard, K., and Milne, R. W. (2008). Comparison of once-, Twice- and Thrice-Daily Dosing of Colistin on Antibacterial Effect and Emergence of Resistance: Studies with Pseudomonas aeruginosa in an In Vitro Pharmacodynamic Model. J. Antimicrob. Chemother. 61 (3), 636–642. doi:10.1093/jac/dkm511
Berglund, N. A., Piggot, T. J., Jefferies, D., Sessions, R. B., Bond, P. J., and Khalid, S. (2015). Interaction of the Antimicrobial Peptide Polymyxin B1 with Both Membranes of E. coli: A Molecular Dynamics Study. Plos Comput. Biol. 11 (4), e1004180. doi:10.1371/journal.pcbi.1004180
Cai, Y., Leck, H., Tan, R. W., Teo, J. Q., Lim, T. P., Lee, W., et al. (2020). Clinical Experience with High-Dose Polymyxin B against Carbapenem-Resistant Gram-Negative Bacterial Infections-A Cohort Study. Antibiotics 9 (8), 451. doi:10.3390/antibiotics9080451
Cheah, S. E., Wang, J., Nguyen, V. T., Turnidge, J. D., Li, J., and Nation, R. L. (2015). New Pharmacokinetic/pharmacodynamic Studies of Systemically Administered Colistin against Pseudomonas aeruginosa and Acinetobacter baumannii in Mouse Thigh and Lung Infection Models: Smaller Response in Lung Infection. J. Antimicrob. Chemother. 70 (12), 3291–3297. doi:10.1093/jac/dkv267
Clifford, H. E., and Stewart, G. T. (1961). Intraventricular Administration of a New Derivative of Polymyxin B in Meningitis Due to Ps. Pyocyanea. Lancet 2 (7195), 177–180. doi:10.1016/s0140-6736(61)90464-0
Daily Med (2017). Polymyxin B for Injection [package Insert]. China: Shanghai First Biochemical Pharmaceutical Co., Ltd.
Diekema, D. J., Hsueh, P. R., Mendes, R. E., Pfaller, M. A., Rolston, K. V., Sader, H. S., et al. (2019). The Microbiology of Bloodstream Infection: 20-year Trends from the SENTRY Antimicrobial Surveillance Program. Antimicrob. Agents Chemother. 63 (7), e00355–00319. doi:10.1128/AAC.00355-19
Elias, L. S., Konzen, D., Krebs, J. M., and Zavascki, A. P. (2010). The Impact of Polymyxin B Dosage on In-Hospital Mortality of Patients Treated with This Antibiotic. J. Antimicrob. Chemother. 65 (10), 2231–2237. doi:10.1093/jac/dkq285
Gales, A. C., Jones, R. N., and Sader, H. S. (2011). Contemporary Activity of Colistin and Polymyxin B Against a Worldwide Collection of Gram-Negative Pathogens: Results from the SENTRY Antimicrobial Surveillance Program (2006-09). J. Antimicrob. Chemother. 66 (9), 2070–2074. doi:10.1093/jac/dkr239
He, J., Abdelraouf, K., Ledesma, K. R., Chow, D. S., and Tam, V. H. (2013). Pharmacokinetics and Efficacy of Liposomal Polymyxin B in a Murine Pneumonia Model. Int. J. Antimicrob. Agents 42 (6), 559–564. doi:10.1016/j.ijantimicag.2013.07.009
Jian, L., Nation, R., and Kaye, K. (2019). Polymyxin Antibiotics: From Laboratory Bench to Bedside. Switzerland: Springer Nature.
Kvitko, C. H., Rigatto, M. H., Moro, A. L., and Zavascki, A. P. (2011). Polymyxin B versus Other Antimicrobials for the Treatment of Pseudomonas aeruginosa Bacteraemia. J. Antimicrob. Chemother. 66 (1), 175–179. doi:10.1093/jac/dkq390
Lakota, E. A., Landersdorfer, C. B., Nation, R. L., Li, J., Kaye, K. S., Rao, G. G., et al. (2018). Personalizing Polymyxin B Dosing Using an Adaptive Feedback Control Algorithm. Antimicrob. Agents Chemother. 62 (7), e00483–00418. doi:10.1128/AAC.00483-18
Landersdorfer, C. B., Wang, J., Wirth, V., Chen, K., Kaye, K. S., Tsuji, B. T., et al. (2018). Pharmacokinetics/pharmacodynamics of Systemically Administered Polymyxin B against Klebsiella pneumoniae in Mouse Thigh and Lung Infection Models. J. Antimicrob. Chemother. 73 (2), 462–468. doi:10.1093/jac/dkx409
Lee, W., Cai, Y., Lim, T. P., Teo, J., Chua, S. C., and Kwa, A. L. (2019). In Vitro pharmacodynamics and PK/PD in Animals. Adv. Exp. Med. Biol. 1145, 105–116. doi:10.1007/978-3-030-16373-0_8
Li, Y., Zheng, B., Lv, Y., Wei, M., and Shan, A. (2015). Chinese Experts' Consensus on Protocol of Breakpoints Setting of New Antibacterial Agents for Clinical Trial. Chin. J. Clin. Pharmacol. 31 (11), 1069–1076.
Liu, X., Chen, Y., Yang, H., Li, J., Yu, J., Yu, Z., et al. (2021). Acute Toxicity is a Dose-Limiting Factor for Intravenous Polymyxin B: A Safety and Pharmacokinetic Study in Healthy Chinese Subjects. J. Infect. 82 (2), 207–215. doi:10.1016/j.jinf.2021.01.006
Liu, X., Yu, Z., Wang, Y., Wu, H., Bian, X., Li, X., et al. (2020). Therapeutic Drug Monitoring of Polymyxin B by LC-MS/MS in Plasma and Urine. Bioanalysis 12 (12), 845–855. doi:10.4155/bio-2020-0051
Nation, R. L., Garonzik, S. M., Thamlikitkul, V., Giamarellos-Bourboulis, E. J., Forrest, A., Paterson, D. L., et al. (2017). Dosing Guidance for Intravenous Colistin in Critically-Ill Patients. Clin. Infect. Dis. 64 (5), 565–571. doi:10.1093/cid/ciw839
Rabanal, F., and Cajal, Y. (2017). Recent Advances and Perspectives in the Design and Development of Polymyxins. Nat. Prod. Rep. 34 (7), 886–908. doi:10.1039/c7np00023e
Rigatto, M. H., Ribeiro, V. B., Konzen, D., and Zavascki, A. P. (2013). Comparison of Polymyxin B with Other Antimicrobials in the Treatment of Ventilator-Associated Pneumonia and Tracheobronchitis Caused by Pseudomonas aeruginosa or Acinetobacter baumannii. Infection 41 (2), 321–328. doi:10.1007/s15010-012-0349-z
Ross, S., Puig, J. R., and Zaremba, E. A. (1959). Colistin: Some Preliminary Laboratory and Clinical Observations in Specific Gastroenteritis in Infants and Children. Antibiot. Annu. 7, 89–100.
Sandri, A. M., Landersdorfer, C. B., Jacob, J., Boniatti, M. M., Dalarosa, M. G., Falci, D. R., et al. (2013). Population Pharmacokinetics of Intravenous Polymyxin B in Critically Ill Patients: Implications for Selection of Dosage Regimens. Clin. Infect. Dis. 57 (4), 524–531. doi:10.1093/cid/cit334
Satlin, M. J., Lewis, J. S., Weinstein, M. P., Patel, J., Humphries, R. M., Kahlmeter, G., et al. (2020). Clinical and Laboratory Standards Institute and European Committee on Antimicrobial Susceptibility Testing Position Statements on Polymyxin B and Colistin Clinical Breakpoints. Clin. Infect. Dis. 71 (9), e523–529. doi:10.1093/cid/ciaa121
Tam, V. H., Schilling, A. N., Vo, G., Kabbara, S., Kwa, A. L., Wiederhold, N. P., et al. (2005). Pharmacodynamics of Polymyxin B against Pseudomonas aeruginosa. Antimicrob. Agents Chemother. 49 (9), 3624–3630. doi:10.1128/AAC.49.9.3624-3630.2005
Terayama, T., Yamakawa, K., Umemura, Y., Aihara, M., and Fujimi, S. (2017). Polymyxin B Hemoperfusion for Sepsis and Septic Shock: A Systematic Review and Meta-Analysis. Surg. Infect. 18 (3), 225–233. doi:10.1089/sur.2016.168
Tsuji, B. T., Pogue, J. M., Zavascki, A. P., Paul, M., Daikos, G. L., Forrest, A., et al. (2019). International Consensus Guidelines for the Optimal Use of the Polymyxins: Endorsed by the American College of Clinical Pharmacy (ACCP), European Society of Clinical Microbiology and Infectious Diseases (ESCMID), Infectious Diseases Society of America (IDSA), International Society for Anti-infective Pharmacology (ISAP), Society of Critical Care Medicine (SCCM), and Society of Infectious Diseases Pharmacists (SIDP). Pharmacotherapy 39 (1), 10–39. doi:10.1002/phar.2209
Velkov, T., Thompson, P. E., Azad, M. A. K., Roberts, K. D., and Bergen, P. J. (2019). History, Chemistry and Antibacterial Spectrum. Adv. Exp. Med. Biol. 1145, 15–36. doi:10.1007/978-3-030-16373-0_3
World Health Organization (2020). Global Antimicrobial Resistance and Use Surveillance System (GLASS) Report. Available at: https://www.who.int/glass/resources/publications/early-implementation-report-2020/en/ (Accessed October 15, 2020).
Wu, X., Huang, C., Wang, H., Ji, J., Ying, C., and Xiao, Y. (2021). Optimal Empiric Polymyxin B Treatment of Patients Infected with Gram-Negative Organisms Detected Using a Blood Antimicrobial Surveillance Network in China. Drug Des. Devel Ther. 15, 2593–2603. doi:10.2147/DDDT.S313714
Xie, J., Roberts, J. A., Lipman, J., Cai, Y., Wang, H., Zhao, N., et al. (2020). Pharmacokinetic/Pharmacodynamic Adequacy of Polymyxin B against Extensively Drug-Resistant Gram-Negative Bacteria in Critically Ill, General ward and Cystic Fibrosis Patient Populations. Int. J. Antimicrob. Agents 55 (6), 105943. doi:10.1016/j.ijantimicag.2020.105943
Yahav, D., Giske, C. G., Grāmatniece, A., Abodakpi, H., Tam, V. H., and Leibovici, L. (2020). New β-Lactam-β-Lactamase Inhibitor Combinations. Clin. Microbiol. Rev. 34 (1), e00115–20. doi:10.1128/CMR.00115-20
Zavascki, A. P., Goldani, L. Z., Cao, G., Superti, S. V., Lutz, L., Barth, A. L., et al. (2008). Pharmacokinetics of Intravenous Polymyxin B in Critically Ill Patients. Clin. Infect. Dis. 47 (10), 1298–1304. doi:10.1086/592577
Zhao, S., Yan, L., Wang, C., and Peng, M. (2020). Clinical Analysis of Sepsis with Extensively Drug Resistant Gram-Negative Bacteria in Intensive Care Unit Treated with Polymyxin B-Based Combination Therapy. Zhonghua Wei Zhong Bing Ji Jiu Yi Xue 32 (2), 150–154. doi:10.3760/cma.j.cn121430-20200108-00028
Keywords: susceptibility breakpoint, polymyxin B, PK/PD analysis, Monte-Carlo simulation, bloodstream infection
Citation: Bian X, Liu X, Hu F, Feng M, Chen Y, Bergen PJ, Li J, Li X, Guo Y and Zhang J (2022) Pharmacokinetic/Pharmacodynamic Based Breakpoints of Polymyxin B for Bloodstream Infections Caused by Multidrug-Resistant Gram-Negative Pathogens. Front. Pharmacol. 12:785893. doi: 10.3389/fphar.2021.785893
Received: 29 September 2021; Accepted: 01 December 2021;
Published: 04 January 2022.
Edited by:
Wangxue Chen, National Research Council Canada (NRC-CNRC), CanadaReviewed by:
Maytham Hussein, The University of Melbourne, AustraliaHanna Evelina Sidjabat, Griffith University, Australia
Copyright © 2022 Bian, Liu, Hu, Feng, Chen, Bergen, Li, Li, Guo and Zhang. This is an open-access article distributed under the terms of the Creative Commons Attribution License (CC BY). The use, distribution or reproduction in other forums is permitted, provided the original author(s) and the copyright owner(s) are credited and that the original publication in this journal is cited, in accordance with accepted academic practice. No use, distribution or reproduction is permitted which does not comply with these terms.
*Correspondence: Yan Guo, Z3VveWFuQGZ1ZGFuLmVkdS5jbg==; Jing Zhang, emhhbmdqX2Z1ZGFuQGFsaXl1bi5jb20=
†These authors have contributed equally to this work and share first authorship