- 1Technology Research Center, Mogi das Cruzes University, Mogi das Cruzes, Brazil
- 2Department of Biochemistry, Federal University of São Paulo, São Paulo, Brazil
- 3Interdisciplinary Center of Biochemical Investigation, University of Mogi das Cruzes, Mogi das Cruzes, Brazil
- 4College of Dental Medicine-Illinois, Midwestern University, Downers Grove, IL, United States
The tissue engineering of dental oral tissue is tackling significant advances and the use of stem cells promises to boost the therapeutical approaches of regenerative dentistry. Despite advances in this field, the literature is still scarce regarding the modulatory effect of laser photobiomodulation (PBM) on genes related to inflammation and osteogenesis in Postnatal Human Dental Pulp Stem cells (DPSCs). This study pointedly investigated the effect of PBM treatment in proliferation, growth and differentiation factors, mineralization, and extracellular matrix remodeling genes in DPSCs. Freshly extracted human third molars were used as a source for DPSCs isolation. The isolated DPSCs were stimulated to an inflammatory state, using a lipopolysaccharide (LPS) model, and then subjected or not to laser PBM. Each experiment was statistically evaluated according to the sample distribution. A total of 85 genes related to inflammation and osteogenesis were evaluated regarding their expression by RT-PCR. Laser PBM therapy has shown to modulate several genes expression in DPSCs. PBM suppressed the expression of inflammatory gene TNF and RANKL and downregulated the gene expression for VDR and proteolytic enzymes cathepsin K, MMP-8 and MMP-9. Modulation of gene expression for proteinase-activated receptors (PARs) following PBM varied among different PARs. As expected, PBM blocked the odontoblastic differentiation of DPSCs when subjected to LPS model. Conversely, PBM has preserved the odontogenic potential of DPSCs by increasing the expression of TWIST-1/RUNEX-2/ALP signaling axis. PBM therapy notably played a role in the DPSCs genes expression that mediate inflammation process and tissue mineralization. The present data opens a new perspective for PBM therapy in mineralized dental tissue physiology.
Introduction
A primary objective of regenerative medicine and tissue engineering is to support the reinstatement of tissues and/or organ’s functions by means an in situ substitution/repair of their injured structures. However, intrinsic morphological complexities such as those found, for instance, in dental pulp can substantially compromise this tissue successful remodeling and repair. Dental pulp is a unique and specialized mesenchymal tissue that, by being confined inside a mineralized rigid chamber in the core of teeth, has limited or virtually no chance to expand as part of the inflammatory response to accommodate an uneventful tissue turnover. Stromal fibroblasts and odontoblasts are the main regenerative/formative cells in dental pulp tissue (Nor, 2006). Interestingly, the pulp tissue also harbors mesenchymal stem cells with self-renewal capacity and multidifferentiation potential (Botelho et al., 2017).
Postnatal stem cells have proven to be an excellent resource in regenerative medicine. Among mesenchymal postnatal cells found in dental pulp, the Dental pulp stem cells (DPSCs) (Kerkis et al., 2006) have shown to exhibit promising tissue regenerative cues, such as a more mature phenotype in comparison to stem cells derived from exfoliated deciduous teeth (SHEDs) (Goldberg and Smith, 2004), and higher plasticity (i.e. proliferative and differentiation capacity to turn into various cells) than Bone marrow stem cells (BMSCs) (Miura et al., 2003).
Overall, the inflammatory process is generally accompanied by imbalance in gene expression and signaling, as well as by the release of proinflammatory cytokines, such as tumor necrosis factor-alpha (TNF-α), reactive oxygen, nitrogen species and, interleukin-1β (IL-1β) and IL-6 (Hanisch, 2002). LPS can activate the NF-κB signalling pathway in DPSCs. NF-κB is a transcription factor that regulates a large variety of inflammatory cytokines, including TNF-α, IL-1, IL-6, and IL-8 (Baldwin, 2001). The LPS induces the production of pro-inflammatory cytokines in dental pulp fibroblasts (Nakane et al., 1995). Also, it has been shown that LPS can promote odontoblastic differentiation of human DPSCs via TLR-4, ERK, and P38 MAPK signalling pathways (He et al., 2015). The inflammatory interleukin(s)-1. -6. -11 (IL-1. IL-6. IL-11), and TNF-α can stimulate osteoclast development and thereby the process of bone resorption (Manolagas, 1995).
In addition to these canonical inflammatory pathways, cell signaling via Proteinase-activated receptors (PARs) are emerging as an important path in the study of inflammatory responses in various tissues (Russell and McDougall, 2009). PARs are part of the family of G-protein-coupled receptors that are activated by proteinases secreted into extracellular matrix (ECM) during inflammation. Whilst first described as thrombin receptors, various other proteinases are able to signal via PARs. While PAR-1, PAR-3, and PAR-4 are canonically activated by thrombin, PAR-2 is mainly activated by trypsin. Furthermore, it can be activated by tryptase, matrix metalloproteinases (MMPs), and tissue factor-VIIa-Xa complex (Vu et al., 1991; Bohm et al., 1996; Ruf and Mueller, 2006). PARs are also known to participate on extracellular matrix pathophysiological processes. Collagenase hydrolysis showed an antagonistic behavior on PAR2 activation, proposing an relevant negative feedback mechanism whereby canonical PAR2 activation induces MMP expression, and MMP activity can subsequently antagonize PAR2 (Falconer et al., 2019). PARs 1 and 2 have been recently demonstrated to play a role in inflamed odontoblasts (Alvarez et al., 2017).
Low-level laser therapy, also known as Photobiomodulation Therapy (PBM) (Hamblin, 2016), had its onset in the 1960’s and it relies on the use of light devices–lasers or light-emitting diodes (LEDs) - as resource to trigger tissue biological/medical responses (i.e. healing, immunity enhancement, anti-inflammatory and antibiotic properties) (Vu et al., 1991; Conlan et al., 1996; Bjordal et al., 2003; Yu et al., 2003; Ruf and Mueller, 2006; Ghanaat, 2010; Alvarez et al., 2017; Falconer et al., 2019). Studies have showed that PBM can significantly reduce inflammation, by inhibiting inflammatory cytokines expression and activity in different tissues (Sakurai et al., 2000; Arany et al., 2007; de Lima et al., 2011). Moreover, the use of lasers and LEDs demonstrated to be effective in modulating the cell viability and growth in different cell models, including mesenchymal stem cells (Kim et al., 2009; Li et al., 2010; Alghamdi et al., 2012; Ginani et al., 2015). Research that evaluated the effect of PBM on SHED cells has shown for instance that infrared LEDs (850 nm 40mW/cm2) could promote an in vitro increase in the levels of phosphates, synthesis of collagen and dentinal sialoprotein (Turrioni et al., 2014), and induce a significant increase in cells viability, proliferation, and production of mineralized tissues for SHEDs that remained in nutrient starvation after PBM (Turrioni et al., 2015).
To better understand DPSC’s features for tissue regenerative applications, such as vital pulp therapy or regenerative endodontic procedures, we believe it is important to assess their biological responses when exposed to light sources under PBM parameters. As far as we know, this is the first study that assessed the potential for laser irradiation to modulate the gene expression for PARs and other genes related to the inflammatory process and osteogenesis using a DPSCs model. This study hypothesis was that PBM can interfere in inflammatory gene expression and in osteogenesis related genes.
Methods
Dental Pulp Tissues Obtainment
Approval for this protocol was obtained from the local Human Research Ethics Committee (# 98511618.8.0000.5497) to use five freshly extracted third molars from patients ages 19–39 years. The use of the third molars is the most convenient source of adult stem cells as they contain sufficient amount of dental pulp tissue to ensure proper isolation of DPSCs (Liu et al., 2006). After extraction teeth were copiously washed with deionized water and placed in a sterile solution containing saline, subsequently they were rinsed with 70% ethanol to reduce the biofilm contamination. Then, the dental elements were rinsed 5 times with sodium phosphate buffer (PBS) to remove ethanol. Subsequently, decontaminated teeth were cut with a sterile Zekrya (Dentsplay Sirona, United States) drill using a high-speed device to expose the pulp chamber and, consequently, provide access to the pulp tissue. The pulp tissue was gently removed from the pulp chamber with a sterile endodontic file and immediately transferred to a sterile screw-capped tube containing α-MEM cell culture medium without calf bovine serum (FCS).
Isolation of Postnatal Human Dental Pulp Stem Cells
Excised pulp tissue was digested in a solution containing 3 mg/ml collagenase type I (Merck KGaA, Darmstadt, Germany) and 4 mg/ml dispase (Merck KGaA, Darmstadt, Germany) for 1 h at 37°C. After digestion, 5 volumes of α-MEM medium containing 10% FCS were added. This solution was centrifuged at 120 × g for 10 min, at room temperature. The precipitated material was resuspended in α-MEM medium and filtered through filters with pores of 70 μM. This procedure resulted in a single-cell suspension for in vitro culture. The cells were seeded into culture flasks with α-MEM, supplemented with 10% FCS, 100 μM l-ascorbic acid, 2 mM l-glutamine and penicillin (100 U/mL)/streptomycin (100 mg/ml) and incubated at 37°C with 5% CO2. In the initial stage, after adhering to culture flasks, cells grew slowly. Cell colonies were identified after 10–14 days, with their fibroblastoid appearance, which showed to be dependent on the cell density obtained in initial plating. After reach 70% of confluence, cells were considered ready to proceed with experiments. Accordingly, isolated DPSCs were randomly assigned to the following experimental groups: Group 1–LPS stimulus and laser irradiation; Group 2–LPS stimulus and no laser irradiation; Group 3–Control group, no LPS or laser treatment.
Human Dental Pulp Stem Cells Inflammation Induction by Lipopolysaccharides Assay
The lipopolysaccharides induces the production of pro-inflammatory cytokines such as interleukins (IL-1β, IL-6 and IL-8), tumor necrosis factor (TNF-), platelet and prostaglandin activating factors by macrophages and neutrophils present in areas infected by bacteria (Lindemann et al., 1988; Wilson et al., 1996). To promote in vitro cellular inflammatory response, 2 × 106 of DPSCs were seeded in six wells plates with α-MEM, supplemented with 10% FCS, 100 μM l-ascorbic acid, 2 mM l-glutamine and penicillin (100U/mL)/streptomycin (100 mg/ml) and incubated from 24 h at 37°C with 5% CO2 for complete adhesion. Previous to the experiment cells were starved for 6hs in α-MEM without FCS. After starvation period, 10 μg/ml of LPS were added in α-MEM, supplemented with 10% FCS, 100 μM l-ascorbic acid, 2 mM l-glutamine and penicillin (100 U/mL)/streptomycin (100 mg/ml), for 24 hs.
Part of these cells was further irradiated (see PBM protocol following) with a laser device (Group 1); while the other part remained not irradiated for subsequent analysis (Group 2).
Photobiomodulation Irradiation Protocol
DPSCs assigned for Group 1 were irradiated using the Laser Duo device (MM Optics, São Carlos, BR), containing 1 light-emitting diode, in the infrared wavelength (808 nm) that delivered a total energy of 6 J (100 mW × 60 s). 0.4 × 105 cells per well were seeded in a 96 wells plate 24 hs before the experiment. All the three experimental groups were evaluated in triplicates. The plate cover and the culture medium were removed prior irradiation to avoid any interference related to light refraction. The laser probe was placed perpendicularly from bottom of the well with a 0.5 cm of distance for 60 s, in order to be sure that all cells received the radiation. After the treatment, the culture medium was replaced for 30 min before the genetic material be assessed. Device specifications: laser type, InGaAIP; wavelength, 808 nm; irradiation type, Infrared; laser beam output area, 0.3 cm2; Continuous; power output, 100 mW ± 20%; irradiance, 0.33 W/cm2; fluence, 6 J/cm2 (Table 1).
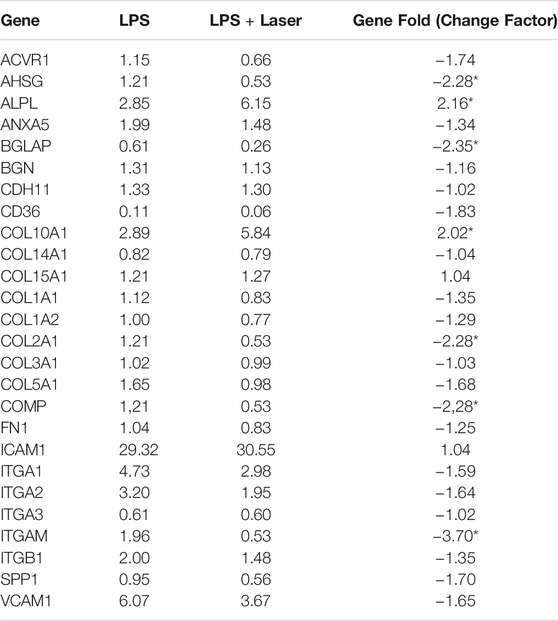
TABLE 1. mRNA expression of cell adhesion and extracellular matrix proteins in DPSCs after and before laser irradiation.
Proteinase-Activated Receptors Gene Expression Quantification
The tRNA from DPSCs was extracted using the TRIzol® (Thermo Fischer Scientific, Waltham, United States) reagent. The complementary DNA (cDNA) was obtained from the tRNA by reverse-transcription using the ImProm-II Reverse transcriptase System kit (Promega, Madison, United States) according to the manufacturer’s protocol. The gene expression evaluation was performed by Real-time quantitative polymerase chain reaction (qRT-PCR) assays, using the SYBR Green PCR Master Mix® (Thermo Fisher Scientific, Waltham, United States). The reaction cycling parameters were adjusted to 50°C for 2 min and 95°C for 10 min, followed by 40 cycles at 95°C for 15 s and 60°C for 1 min in an Real Time PCR System ABI PRISM 7500 (Applied Biosystems, Foster City, United States). Relative quantification was carried out using the ΔCt method. This method results in ratios between the target genes and the housekeeping reference gene, in this case, the enzyme β-2-microglobulin (positive control) for DSPCs. This assay is based on gene fold-Regulation approach, that represents in fold-change (fc) results of the evaluated genes. Fold-change values greater than one indicates a positive- or an up-regulation in gene expression, while fold-change values less than one indicate a negative or down-regulation, of the evaluated gene. The p-values were calculated based on a Student’s t-test of the replicate 2^ (ΔCt) values for each gene in the experimental or control groups. All primers sequences used in PARs genes evaluation were manufactured by Exxtend (São Paulo, SP, Brazil). The melting curve analysis was used to determine the specificity of the reaction. All experiments were run in triplicates.
Inflammation and Osteogeneses Genes Expression Array
Likewise, DPSCs from the 3 experimental groups were assessed for expression of eighty-five (85) genes related to inflammation and osteogenesis the RT2 Profiler ™ PCR array (Qiagen, Hilden, GER) was used. This device allows gene expression profiling technology by analyzing focused panels of genes using real-time PCR. Each experimental kit contains a set of the inflammatory/osteogenesis pathway-converged genes, and five housekeeping (positive controls) genes. The array also contains a panel of other reaction controls to monitor real-time PCR efficiency (PPC), genomic DNA contamination (GDC) as well as the first strand synthesis (RTC). All experiments were run in triplicates.
Statistical Analysis
Raw data for each experiment (PARs, inflammation and osteogenesis gene expression) was transformed in a fractional number as function of the data from control group, using a single rule of 3, wherein the gene expression values of control groups for each experiment were considered = 1. Lack of normal distribution demanded the application of non-parametric Kruskal–Wallis tests, complemented by Mann–Whitney tests for pairwise comparison, at a 5% level of significance.
Results
Proteinase-Activated Receptor Expression
Four PARs have been identified so far (PAR-1–4) and evidence shows that they can exhibit both anti- and pro-inflammatory properties. Figure 1 shows that PBM treatment can modulate the gene expression of all PARs members. While PAR-1 showed a downregulation in the gene expression, the other PARs show a significant increase in the number of gene copies.
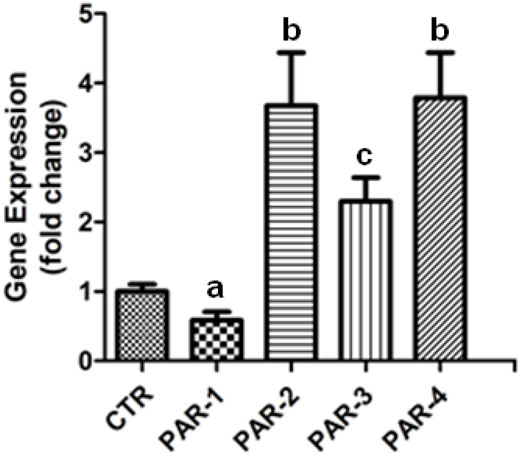
FIGURE 1. PBM modulate PARs genes expression in DPSCs. Real-time quantitative PCR (qRT-PCR) analysis of gene expression in LPS stimulated DPSCs irradiated with the infrared wavelength (808 nm) with total energy of 6 J (100mW × 60s). The bars represent the mean expression levels of the four PARs genes and standard error. The qRT-PCR analysis of gene expressions was normalized to the housekeeping gene enzyme β-2-microglobulin for DPSCs (control); the relative quantification of the expression levels (experimental/control) was determined based on the 2-ΔCt method. Experiments were performed in triplicate. Different letters indicate statistically significant differences (p < 0.05) in gene expression.
Photobiomodulation Effect on Gene Expression of Human Dental Pulp Stem Cells After Lipopolysaccharide Inflammatory Stimulus
PBM therapy showed a wide range of effects at the tissue, cellular, and molecular levels. LPS-inflamed DPSCs demonstrated to be sensitive to PBM irradiation with several genes related to inflammation and osteogenesis being modulated either up or down after treatment. Among the genes analyzed, Table 1 depicts the DPSCs genes related to proteins involved in the extracellular matrix (ECM) organization that were modulated by LPS inducement and/or then regulated or inhibited by PBM irradiation.
Photobiomodulation Effect on Expression of Proteolytic Enzymes Genes of Human Dental Pulp Stem Cells
Both in vivo and in vitro have used PBM because it is an important tool to positively stimulate bone. However, little is known about its association with anti-inflammatory and neoformative events taking place in human stem cells derived from a mineralized tissue. The data presented in Table 2 show the expression of proteolytic enzymes genes related to organic matrix remodeling in mineralized tissues that were upregulated in the inflammatory state and then, mainly downregulated after PBM therapy. From all enzymes evaluated only MMP-10 showed an increase in the expression (1.81-fold), while MMP-8 and MMP-9 gene expression were significantly reduced -3.03 and -2.68-fold, respectively.
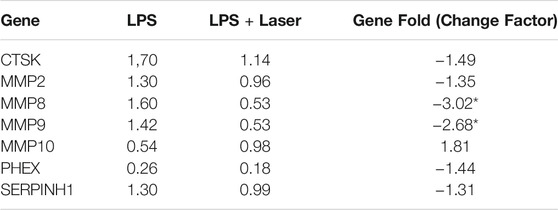
TABLE 2. mRNA expression of proteolytic enzymes related to organic matrix remodeling in the DPSCs before and after laser irradiation.
Photobiomodulation Effects on Genes Expression of Growth and Differentiation Factors in Human Dental Pulp Stem Cells
Initially known as an active secreted molecule that can affect the growth of the cells, the growth factors family has been expanded his activities to include secreted molecules that can affect cellular differentiation and promote or inhibit mitosis. Table 3 show the expression profile of genes that can act on specific cell surface receptors that subsequently transmit their growth signals to other intracellular components, after inflammatory stimulus, and after PBM treatment. Our results clearly show that the growth factors were predominantly upregulated by LPS, while PBM treatment lowered the gene expression of proteins related to tissue mineralization, such as BMP-1 (-3.67-fold), BMP-4 (-3.73-fold), and BMP-7 (-2.28-fold). In the same sense, VEGF-B was significantly downregulated by laser treatment (-9.43-fold).
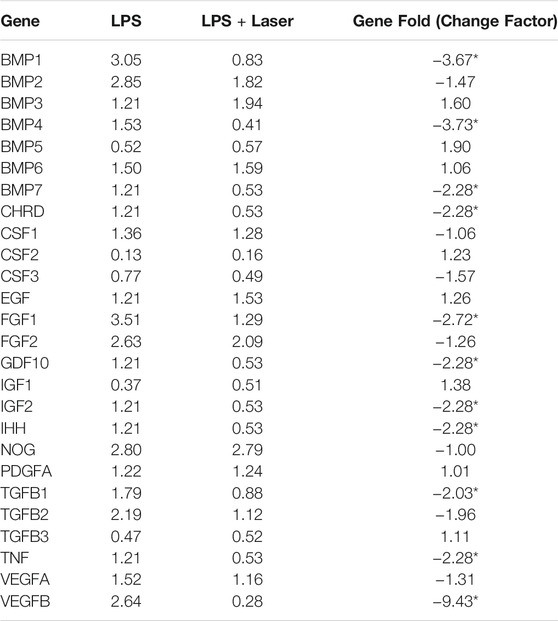
TABLE 3. mRNA expression of growth and differentiation factors in the DPSCs before and after laser irradiation.
Photobiomodulation-Modulating Effect on Gene Expression of Cell Surface Receptors
Once the expression of growth factors was evaluated, the gene expression for cell receptors related to these specific ligands was also evaluated. As expected, the expression of the cellular receptors followed the trend profiling found as far expression of their respective ligands. For most of evaluated cellular receptors, it was observed an increase in their gene expression after induction with LPS, while PBM treatment downregulated all evaluated genes. Interestingly, the most significantly inhibited receptors were those related to tissue mineralization processes (Table 4), including: Calcium Receptor (-2.28-fold), and Vitamin D Receptor (-37.66-fold).
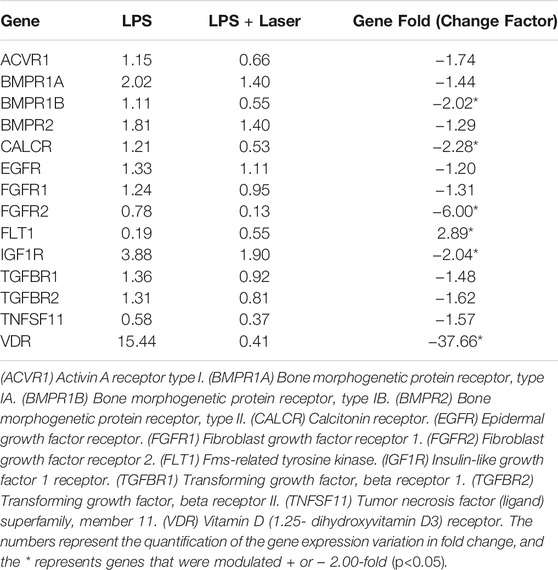
TABLE 4. mRNA Expression of Cellular Receptors Related to Osteogenesis in the DPSCs After and Before Laser Irradiation.
Photobiomodulation-Modulating Effect on Gene Expression of Transcription Factors
Transcription factors are proteins that regulates the transcription of genes. They have DNA-binding sequences that give them the ability to bind to specific of DNA domains called enhancer sequences. The evaluated members of this family were basically not modulated by LPS (Table 5). Except for the SOX-9 gene that showed a 3.68-fold increase in expression levels. Conversely, the PBM treatment downregulated the expression of all analyzed genes with the SP-7 (−3.28-fold), GLI-1 (−2.85-fold), and TWIST-1 (−2.35-fold) showing to be the most sensitive genes to laser irradiation.
Discussion
In the regenerative dentistry field researchers face two major challenges, both related to the pulp tissue pathophysiology. The first is the tissue ability to turn back to a healthy metabolic state after a transitory inflammation. The second concerns to its intrinsic potential to produce mineralized tissue through its specialized cells, the odontoblasts. The appreciation of human dental pulp stem cells (DPSCs) as suitable for dental tissue engineering applications (Kaigler and Mooney, 2001; Young et al., 2005; Duailibi et al., 2008; Zhang et al., 2009) renders the overall ambition of tissue regeneration in dentistry more accomplishable. The PBM therapy or PBM treatment has been used in medicine and dentistry for its well demonstrated analgesic, anti-inflammatory, and biostimulation effects (Reddy, 2004; Silveira et al., 2007; Moosavi et al., 2016). In addition, the present study has shown, for the first time, that such modulatory physio-biological effects of PBM is accompanied by a modulation on the gene expression of inflammation and osteogenic biomarkers in DPSCs.
Proteinase-activated receptors (PARs) have a distinct mechanism of activation that involves limited proteolysis and unmasking of a receptor activating motif called tethered ligand (Huesa et al., 2016). PAR-1 and PAR-4 are canonically described as thrombin-activated receptors, while PAR-2 is activated by trypsin. Interestingly, PAR-3 was related to play role during embryonic development (Gieseler et al., 2013). Our results clearly showed that PBM therapy can increase the expression of PAR-2 (3.7-fold), PAR-3 (2.3-fold), and PAR-4 (3.8-fold) while decreasing the expression of PAR-1 (-0.6-fold). Interestingly, matrix metalloproteinases (MMPs), which genes have shown to be upregulated by PBM in the present study, have been described to activate PARs in a noncanonical way (Alvarez et al., 2017). Thus, taken in conjunction, these results strongly suggest that PARs can play a role in the anti-inflammatory process promoted by PBM therapy by not only increasing their expression, but also by increasing the MMPs expression levels.
Even under basal conditions DPSCs produce these molecular markers, including but not limited to CD-36, BMPs, NOG, Type II collagen, RUNX2, SOX-9 that are responsible for maintenance of pluripotency in early embryos and embryonic stem cells (Govindasamy et al., 2010; Karaoz et al., 2010; Martinez-Sarra et al., 2017), which indicate a promising primitiveness and multipotency of DPSCs for regenerative dentistry.
The osteogenic gene profile analysis was performed to provide better understanding on the underlying mechanisms for DPSCs differentiation and modulation of mineralization process upon the effects of an inflammatory model (LPS) and PBM (Tables 2–5). The treatment of DPSCs with LPS was capable to remarkably induce the gene expression of odontoblastic differentiation biomarkers, BMP-1, BMP-2, BMPR-1A, FGF-1, FGF-2, TGF-B2, IGF-1R, SMAD-4, SMAD-5, COL-10A1, ITG-A1, ITG-A2, ITG-B, ITG-A, and Alkaline phosphatase (ALP) in DPSCs. ALP is a widely accepted as an earlier marker for the differentiation of cells forming mineralized tissues. It has been shown that BMP-2 is required to induce the differentiation of DPSCs into odontoblast (Casagrande et al., 2010), and in mesenchymal stem cells, BMP-2 efficiently induced the expression of transcriptional factor Sox9 responsible for chondrogenic differentiation via BMP-2/Smad (Pan et al., 2008). Transforming growth factor-beta (TGF-β), also via SMAD pathways, plays a major role in tooth development and the reparative process by regulating cell proliferation, differentiation, and reparative dentinogenesis (Niwa et al., 2018). FGF-1 and TGF-β1 have a synergic effect to promote morphological and functional features of differentiated odontoblasts, whereas FGF-2 seems to modulate TGF-β1 action (Unda et al., 2000). TGF-β1 and TGF-β3 are predominantly expressed in odontoblasts, whereas TGF-β2 is high expressed in dental pulp (Niwa et al., 2018). IGF-I stimulate osteoblast differentiation in human mesenchymal stem cells (HMSCs), it stimulates the biosynthesis of 1α.25(OH)2D in synergy with 25OHD3. Osteoblast differentiation and skeletal homeostasis may be regulated by autocrine/paracrine actions of 25(OH)D (3) in HMSCs (Geng et al., 2011). Here, we demonstrate that LPS can increases both IGF-1R and VDR expression in DPSCs favoring odontoblast differentiation. Taken together, our data suggest that LPS treatment induced odontoblastic differentiation of human DPSCs.
RANKL is a tumor necrosis factor (TNF)-like factor produced by mesenchymal cells, osteoblast derivatives, and T cells that is essential for osteoclastogenesis. In osteoblasts, RANKL expression is regulated by two major calcemic hormones, 1.25-dihydroxyvitamin D (3) [1.25(OH) (2)D (3)] and parathyroid hormone (PTH), as well as by several inflammatory/osteoclastogenic cytokines (Kim et al., 2006).
It is important to mention that 1.25(OH)2 vitamin D stimulates osteoblast maturation, increasing expression of the mature osteoblast marker osteocalcin (BGLAP) in osteoblasts, and 1.25(OH)2 vitamin D also stimulates the expression of the osteoclast differentiation factor RANKL (Pereira et al., 2019). The biological actions of 1.25-(OH)2D3 are mediated by the vitamin D receptor (VDR), a protein that binds to target genes and alters their expression. 1.25-(OH)2D3 is also able of inducing transcription of the VDR gene itself (Zella et al., 2006). VDR signaling in osteoprogenitors cells increases RANKL expression and stimulates osteoclastogenesis (Kim et al., 2006).
In this sense, PBM treatment significantly suppressed the mRNA expression of TNF and RANKL (TNFSF11) triggered by LPS in DSPC. Interesting, PBM treatment greatly decreased (37.66-fold) the expression Vitamin D Receptor (VDR) triggered by LPS in the cell model. PBM treatment can inhibit the transcriptional activity of NF-κB in human periodontal ligament cells (Lee et al., 2018), which is a crucial transcription factor involved in the regulation of the inflammatory process triggered by LPS in dental pulp stem cells (Baldwin, 2001). PBM decreased cell death and attenuated the NLRP3 inflammasome in the ischemic brain. In mice experimental model with ischemic stroke, PBM therapy showed suppressed TLR-2 levels, MAPK signaling and NF-kB activation. The suppression of NF-kB activation induced by PBM is related to the major anti-inflammatory activity of Laser (Lee et al., 2017).
Our data suggest that the decrease in the VDR expression promoted by PBM treatment can lead to inhibition of 1.25(OH)2D3/VTR signaling, and downregulating RANKL expression in DPSCs (Kim et al., 2006). PBM therapy also decreased the expression of proteolytic enzymes cathepsin K, MMP-8 and MMP-9 after LPS assay. Overall, PBM blocked the odontoblastic differentiation of human dental pulp stem cells subjected to LPS assay that were dependent on BMP, FGF, IGF and TGFB signaling, decreasing the expression of the transcriptional factor DLX-5 and SP-7 as expected. SP-7 and Dlx5, in turn, was shown to drive the differentiation of mesenchymal precursor cells into osteoblasts (Nakashima et al., 2002). Conversely, it is important to mention that PBM did not block the mRNA expression of ALP, Twist homolog 1 (TWIST-1) and Runt-related transcription factor 2 (RUNX-2) in DPSCs, even in the presence of LPS. TWIST-1 protein regulates several genes that are known to be key players in bone formation, including the FGF-R2 and RUNX-2 genes. In conjunction, our data strongly suggest that PBM decreased inflammatory mineral matrix resorption in DPSCs by decreasing the activation of RANKL expression via inhibition of 1.25(OH)2D3/VTR signaling. Moreover, PBM treatment preserved the odontogenic potential of DPSCs by increasing the expression of TWIST-1/RUNEX-2/ALP signaling axis.
In spite of the study limitations, were possible to conclude that biomodulation of DPSCs by irradiation with laser device at the infrared wavelength (808 nm) showed not only to mediate the gene expression related to habitual anti-inflammatory molecular canons, but also participate in the regulation of genes that can express signaling molecules and factors associated with other non-classic inflammation molecules (i.e. PARs, cell receptors and transcription factors) as well as with molecules of bone tissue protection from resorption, even if it has not clearly shown yet to influence the expression of genes that stimulate bone neoformation. Further studies still need to be performed to better elucidate the role of PBM therapy on the DPSCs and bone. However, this study certainly brings new data to tissue engineering related to the regenerative pulp therapy field.
Data Availability Statement
The datasets presented in this study can be found in online repositories. The data can be accessed from the Gene Expression Omnibus (GEO) database, using accession number GSE193448.
Ethics Statement
The studies involving human participants were reviewed and approved by Approval for this protocol was obtained from the University of Mogi das Cruzes Human Research Ethics Committee (protocol # 98511618.8.0000.5497). The patients/participants provided their written informed consent to participate in this study.
Author Contributions
ER contributed to conception, design, data acquisition and interpretation, drafted and critically revised the manuscript and project administration; MA contributed to data acquisition and interpretation, drafted and critically revised the manuscript; AP contributed to data acquisition, interpretation and analyses, drafted and critically revised the manuscript; MM contributed to conception, data curation and analyses, drafted and critically revised the manuscript; SO contributed to data acquisition, interpretation and analyses and critically revised the manuscript; MRC contributed to data interpretation, drafted and critically revised the manuscript. IT contributed to data interpretation, drafted and critically revised the manuscript; and FN contributed to conception, design and data acquisition, drafted and critically revised the manuscript and funding acquisition. All authors gave their final approval and agreed to be accountable for all aspects of the work.
Funding
This study was funded with grant FAPESP (13/05822-9 and 2017/07687-2 P.I. Nascimento).
Conflict of Interest
The authors declare that the research was conducted in the absence of any commercial or financial relationships that could be construed as a potential conflict of interest.
Publisher’s Note
All claims expressed in this article are solely those of the authors and do not necessarily represent those of their affiliated organizations, or those of the publisher, the editors and the reviewers. Any product that may be evaluated in this article, or claim that may be made by its manufacturer, is not guaranteed or endorsed by the publisher.
References
Alghamdi, K. M., Kumar, A., and Moussa, N. A. (2012). Low-level Laser Therapy: a Useful Technique for Enhancing the Proliferation of Various Cultured Cells. Lasers Med. Sci. 27, 237–249. doi:10.1007/s10103-011-0885-2
Alvarez, M. M. P., Moura, G. E., Machado, M. F. M., Viana, G. M., De Souza Costa, C. A., Tjäderhane, L., et al. (2017). PAR-1 and PAR-2 Expression Is Enhanced in Inflamed Odontoblast Cells. J. Dent. Res. 96, 1518–1525. doi:10.1177/0022034517719415
Arany, P. R., Nayak, R. S., Hallikerimath, S., Limaye, A. M., Kale, A. D., and Kondaiah, P. (2007). Activation of Latent TGF-Beta1 by Low-Power Laser In Vitro Correlates with Increased TGF-Beta1 Levels in Laser-Enhanced Oral Wound Healing. Wound Repair Regen. 15, 866–874. doi:10.1111/j.1524-475X.2007.00306.x
Baldwin, A. S. (2001). Series Introduction: the Transcription Factor NF-kappaB and Human Disease. J. Clin. Invest. 107, 3–6. doi:10.1172/JCI11891
Bjordal, J. M., Couppé, C., Chow, R. T., Tunér, J., and Ljunggren, E. A. (2003). A Systematic Review of Low Level Laser Therapy with Location-specific Doses for Pain from Chronic Joint Disorders. Aust. J. Physiother. 49, 107–116. doi:10.1016/s0004-9514(14)60127-6
Bohm, S. K., Kong, W., Bromme, D., Smeekens, S. P., Anderson, D. C., Connolly, A., et al. (1996). Molecular Cloning, Expression and Potential Functions of the Human Proteinase-Activated Receptor-2. Biochem. J. 314 (Pt 3), 1009–1016. doi:10.1042/bj3141009
Botelho, J., Cavacas, M. A., Machado, V., and Mendes, J. J. (2017). Dental Stem Cells: Recent Progresses in Tissue Engineering and Regenerative Medicine. Ann. Med. 49, 644–651. doi:10.1080/07853890.2017.1347705
Casagrande, L., Demarco, F. F., Zhang, Z., Araujo, F. B., Shi, S., and Nör, J. E. (2010). Dentin-derived BMP-2 and Odontoblast Differentiation. J. Dent. Res. 89, 603–608. doi:10.1177/0022034510364487
Conlan, M. J., Rapley, J. W., and Cobb, C. M. (1996). Biostimulation of Wound Healing by Low-Energy Laser Irradiation. A Review. J. Clin. Periodontol. 23, 492–496. doi:10.1111/j.1600-051x.1996.tb00580.x
De Lima, F. M., Villaverde, A. B., Albertini, R., Corrêa, J. C., Carvalho, R. L., Munin, E., et al. (2011). Dual Effect of Low-Level Laser Therapy (LLLT) on the Acute Lung Inflammation Induced by Intestinal Ischemia and Reperfusion: Action on Anti- and Pro-inflammatory Cytokines. Lasers Surg. Med. 43, 410–420. doi:10.1002/lsm.21053
Duailibi, S. E., Duailibi, M. T., Zhang, W., Asrican, R., Vacanti, J. P., and Yelick, P. C. (2008). Bioengineered Dental Tissues Grown in the Rat Jaw. J. Dent. Res. 87, 745–750. doi:10.1177/154405910808700811
Falconer, A. M. D., Chan, C. M., Gray, J., Nagashima, I., Holland, R. A., Shimizu, H., et al. (2019). Collagenolytic Matrix Metalloproteinases Antagonize Proteinase-Activated Receptor-2 Activation, Providing Insights into Extracellular Matrix Turnover. J. Biol. Chem. 294, 10266–10277. doi:10.1074/jbc.RA119.006974
Geng, S., Zhou, S., and Glowacki, J. (2011). Effects of 25-hydroxyvitamin D(3) on Proliferation and Osteoblast Differentiation of Human Marrow Stromal Cells Require CYP27B1/1α-Hydroxylase. J. Bone Miner. Res. 26, 1145–1153. doi:10.1002/jbmr.298
Ghanaat, M. (2010). Types of Hair Loss and Treatment Options, Including the Novel Low-Level Light Therapy and its Proposed Mechanism. South. Med. J. 103, 917–921. doi:10.1097/SMJ.0b013e3181ebcf71
Gieseler, F., Ungefroren, H., Settmacher, U., Hollenberg, M. D., and Kaufmann, R. (2013). Proteinase-activated Receptors (PARs) - Focus on Receptor-Receptor-Interactions and Their Physiological and Pathophysiological Impact. Cell Commun. Signal. 11, 86. doi:10.1186/1478-811X-11-86
Ginani, F., Soares, D. M., Barreto, M. P., and Barboza, C. A. (2015). Effect of Low-Level Laser Therapy on Mesenchymal Stem Cell Proliferation: a Systematic Review. Lasers Med. Sci. 30, 2189–2194. doi:10.1007/s10103-015-1730-9
Goldberg, M., and Smith, A. J. (2004). Cells and Extracellular Matrices of Dentin and Pulp: A Biological Basis for Repair and Tissue Engineering. Crit. Rev. Oral Biol. Med. 15, 13–27. doi:10.1177/154411130401500103
Govindasamy, V., Abdullah, A. N., Ronald, V. S., Musa, S., Ab Aziz, Z. A., Zain, R. B., et al. (2010). Inherent Differential Propensity of Dental Pulp Stem Cells Derived from Human Deciduous and Permanent Teeth. J. Endod. 36, 1504–1515. doi:10.1016/j.joen.2010.05.006
Hamblin, M. R. (2016). Photobiomodulation or Low-Level Laser Therapy. J. Biophotonics 9, 1122–1124. doi:10.1002/jbio.201670113
Hanisch, U. K. (2002). Microglia as a Source and Target of Cytokines. Glia 40, 140–155. doi:10.1002/glia.10161
He, W., Wang, Z., Luo, Z., Yu, Q., Jiang, Y., Zhang, Y., et al. (2015). LPS Promote the Odontoblastic Differentiation of Human Dental Pulp Stem Cells via MAPK Signaling Pathway. J. Cel. Physiol. 230, 554–561. doi:10.1002/jcp.24732
Huesa, C., Ortiz, A. C., Dunning, L., Mcgavin, L., Bennett, L., Mcintosh, K., et al. (2016). Proteinase-activated Receptor 2 Modulates OA-Related Pain, Cartilage and Bone Pathology. Ann. Rheum. Dis. 75, 1989–1997. doi:10.1136/annrheumdis-2015-208268
Kaigler, D., and Mooney, D. (2001). Tissue Engineering's Impact on Dentistry. J. Dent. Educ. 65, 456–462. doi:10.1002/j.0022-0337.2001.65.5.tb03415.x
Karaöz, E., Doğan, B. N., Aksoy, A., Gacar, G., Akyüz, S., Ayhan, S., et al. (2010). Isolation and In Vitro Characterisation of Dental Pulp Stem Cells from Natal Teeth. Histochem. Cel. Biol. 133, 95–112. doi:10.1007/s00418-009-0646-5
Kerkis, I., Kerkis, A., Dozortsev, D., Stukart-Parsons, G. C., Gomes Massironi, S. M., Pereira, L. V., et al. (2006). Isolation and Characterization of a Population of Immature Dental Pulp Stem Cells Expressing OCT-4 and Other Embryonic Stem Cell Markers. Cells Tissues Organs 184, 105–116. doi:10.1159/000099617
Kim, H. K., Kim, J. H., Abbas, A. A., Kim, D. O., Park, S. J., Chung, J. Y., et al. (2009). Red Light of 647 Nm Enhances Osteogenic Differentiation in Mesenchymal Stem Cells. Lasers Med. Sci. 24, 214–222. doi:10.1007/s10103-008-0550-6
Kim, S., Yamazaki, M., Zella, L. A., Shevde, N. K., and Pike, J. W. (2006). Activation of Receptor Activator of NF-kappaB Ligand Gene Expression by 1,25-dihydroxyvitamin D3 Is Mediated through Multiple Long-Range Enhancers. Mol. Cel. Biol. 26, 6469–6486. doi:10.1128/MCB.00353-06
Lee, H. I., Lee, S. W., Kim, N. G., Park, K. J., Choi, B. T., Shin, Y. I., et al. (2017). Low-level Light Emitting Diode (LED) Therapy Suppresses Inflammasome-Mediated Brain Damage in Experimental Ischemic Stroke. J. Biophotonics 10, 1502–1513. doi:10.1002/jbio.201600244
Lee, J. H., Chiang, M. H., Chen, P. H., Ho, M. L., Lee, H. E., and Wang, Y. H. (2018). Anti-inflammatory Effects of Low-Level Laser Therapy on Human Periodontal Ligament Cells: In Vitro Study. Lasers Med. Sci. 33, 469–477. doi:10.1007/s10103-017-2376-6
Li, W. T., Leu, Y. C., and Wu, J. L. (2010). Red-light Light-Emitting Diode Irradiation Increases the Proliferation and Osteogenic Differentiation of Rat Bone Marrow Mesenchymal Stem Cells. Photomed. Laser Surg. 28 (Suppl. 1), S157–S165. doi:10.1089/pho.2009.2540
Lindemann, R. A., Economou, J. S., and Rothermel, H. (1988). Production of Interleukin-1 and Tumor Necrosis Factor by Human Peripheral Monocytes Activated by Periodontal Bacteria and Extracted Lipopolysaccharides. J. Dent. Res. 67, 1131–1135. doi:10.1177/00220345880670081401
Liu, H., Gronthos, S., and Shi, S. (2006). Dental Pulp Stem Cells. Methods Enzymol. 419, 99–113. doi:10.1016/S0076-6879(06)19005-9
Manolagas, S. C. (1995). Role of Cytokines in Bone Resorption. Bone 17, 63S–67S. doi:10.1016/8756-3282(95)00180-l
Martínez-Sarrà, E., Montori, S., Gil-Recio, C., Núñez-Toldrà, R., Costamagna, D., Rotini, A., et al. (2017). Human Dental Pulp Pluripotent-like Stem Cells Promote Wound Healing and Muscle Regeneration. Stem Cel. Res. Ther. 8, 175. doi:10.1186/s13287-017-0621-3
Miura, M., Gronthos, S., Zhao, M., Lu, B., Fisher, L. W., Robey, P. G., et al. (2003). SHED: Stem Cells from Human Exfoliated Deciduous Teeth. Proc. Natl. Acad. Sci. U S A. 100, 5807–5812. doi:10.1073/pnas.0937635100
Moosavi, H., Arjmand, N., Ahrari, F., Zakeri, M., and Maleknejad, F. (2016). Effect of Low-Level Laser Therapy on Tooth Sensitivity Induced by In-Office Bleaching. Lasers Med. Sci. 31, 713–719. doi:10.1007/s10103-016-1913-z
Nakane, A., Yoshida, T., Nakata, K., Horiba, N., and Nakamura, H. (1995). Effects of Lipopolysaccharides on Human Dental Pulp Cells. J. Endod. 21, 128–130. doi:10.1016/s0099-2399(06)80437-1
Nakashima, K., Zhou, X., Kunkel, G., Zhang, Z., Deng, J. M., Behringer, R. R., et al. (2002). The Novel Zinc finger-containing Transcription Factor Osterix Is Required for Osteoblast Differentiation and Bone Formation. Cell 108, 17–29. doi:10.1016/s0092-8674(01)00622-5
Niwa, T., Yamakoshi, Y., Yamazaki, H., Karakida, T., Chiba, R., Hu, J. C., et al. (2018). The Dynamics of TGF-β in Dental Pulp, Odontoblasts and Dentin. Sci. Rep. 8, 4450. doi:10.1038/s41598-018-22823-7
Nör, J. E. (2006). Tooth Regeneration in Operative Dentistry. Oper. Dent. 31, 633–642. doi:10.2341/06-000
Pan, Q., Yu, Y., Chen, Q., Li, C., Wu, H., Wan, Y., et al. (2008). Sox9, a Key Transcription Factor of Bone Morphogenetic Protein-2-Induced Chondrogenesis, Is Activated through BMP Pathway and a CCAAT Box in the Proximal Promoter. J. Cel Physiol 217, 228–241. doi:10.1002/jcp.21496
Pereira, R. C., Salusky, I. B., Bowen, R. E., Freymiller, E. G., and Wesseling-Perry, K. (2019). Vitamin D Sterols Increase FGF23 Expression by Stimulating Osteoblast and Osteocyte Maturation in CKD Bone. Bone 127, 626–634. doi:10.1016/j.bone.2019.07.026
Reddy, G. K. (2004). Photobiological Basis and Clinical Role of Low-Intensity Lasers in Biology and Medicine. J. Clin. Laser Med. Surg. 22, 141–150. doi:10.1089/104454704774076208
Ruf, W., and Mueller, B. M. (2006). Thrombin Generation and the Pathogenesis of Cancer. Semin. Thromb. Hemost. 32 (Suppl. 1), 61–68. doi:10.1055/s-2006-939555
Russell, F. A., and Mcdougall, J. J. (2009). Proteinase Activated Receptor (PAR) Involvement in Mediating Arthritis Pain and Inflammation. Inflamm. Res. 58, 119–126. doi:10.1007/s00011-009-8087-0
Sakurai, Y., Yamaguchi, M., and Abiko, Y. (2000). Inhibitory Effect of Low-Level Laser Irradiation on LPS-Stimulated Prostaglandin E2 Production and Cyclooxygenase-2 in Human Gingival Fibroblasts. Eur. J. Oral Sci. 108, 29–34. doi:10.1034/j.1600-0722.2000.00783.x
Silveira, P. C., Streck, E. L., and Pinho, R. A. (2007). Evaluation of Mitochondrial Respiratory Chain Activity in Wound Healing by Low-Level Laser Therapy. J. Photochem. Photobiol. B 86, 279–282. doi:10.1016/j.jphotobiol.2006.10.002
Turrioni, A. P., Basso, F. G., Montoro, L. A., Almeida, Lde. F., Costa, C. A., and Hebling, J. (2014). Phototherapy Up-Regulates Dentin Matrix Proteins Expression and Synthesis by Stem Cells from Human-Exfoliated Deciduous Teeth. J. Dent. 42, 1292–1299. doi:10.1016/j.jdent.2014.07.014
Turrioni, A. P., Montoro, L. A., Basso, F. G., de Almeida, Lde. F., Costa, C. A., and Hebling, J. (2015). Dose-responses of Stem Cells from Human Exfoliated Teeth to Infrared LED Irradiation. Braz. Dent. J. 26, 409–415. doi:10.1590/0103-6440201300148
Unda, F. J., Martín, A., Hilario, E., Bègue-Kirn, C., Ruch, J. V., and Aréchaga, J. (2000). Dissection of the Odontoblast Differentiation Process In Vitro by a Combination of FGF1, FGF2, and TGFbeta1. Dev. Dyn. 218, 480–489. doi:10.1002/1097-0177(200007)218:3<480:AID-DVDY1011>3.0.CO;2-O
Vu, T. K., Hung, D. T., Wheaton, V. I., and Coughlin, S. R. (1991). Molecular Cloning of a Functional Thrombin Receptor Reveals a Novel Proteolytic Mechanism of Receptor Activation. Cell 64, 1057–1068. doi:10.1016/0092-8674(91)90261-v
Wilson, M., Reddi, K., and Henderson, B. (1996). Cytokine-inducing Components of Periodontopathogenic Bacteria. J. Periodontal Res. 31, 393–407. doi:10.1111/j.1600-0765.1996.tb00508.x
Young, C. S., Abukawa, H., Asrican, R., Ravens, M., Troulis, M. J., Kaban, L. B., et al. (2005). Tissue-engineered Hybrid Tooth and Bone. Tissue Eng. 11, 1599–1610. doi:10.1089/ten.2005.11.1599
Yu, H. S., Wu, C. S., Yu, C. L., Kao, Y. H., and Chiou, M. H. (2003). Helium-neon Laser Irradiation Stimulates Migration and Proliferation in Melanocytes and Induces Repigmentation in Segmental-type Vitiligo. J. Invest. Dermatol. 120, 56–64. doi:10.1046/j.1523-1747.2003.12011.x
Zella, L. A., Kim, S., Shevde, N. K., and Pike, J. W. (2006). Enhancers Located within Two Introns of the Vitamin D Receptor Gene Mediate Transcriptional Autoregulation by 1,25-dihydroxyvitamin D3. Mol. Endocrinol. 20, 1231–1247. doi:10.1210/me.2006-0015
Keywords: human dental pulp stem cells, photobiomodualtion therapy, gene expreesion, dental pulp stem cells, inflammation, bone osteogenesis
Citation: Rocha EAd, Alvarez MMP, Pelosine AM, Carrilho MRO, Tersariol ILS and Nascimento FD (2022) Laser Photobiomodulation 808 nm: Effects on Gene Expression in Inflammatory and Osteogenic Biomarkers in Human Dental Pulp Stem Cells. Front. Pharmacol. 12:782095. doi: 10.3389/fphar.2021.782095
Received: 23 September 2021; Accepted: 19 October 2021;
Published: 17 January 2022.
Edited by:
Emer S. Ferro, University of São Paulo, BrazilReviewed by:
Paulo Jorge Palma, University of Coimbra, PortugalCamila Squarzoni Dale, University of São Paulo, Brazil
Copyright © 2022 Rocha, Alvarez, Pelosine, Carrilho, Tersariol and Nascimento. This is an open-access article distributed under the terms of the Creative Commons Attribution License (CC BY). The use, distribution or reproduction in other forums is permitted, provided the original author(s) and the copyright owner(s) are credited and that the original publication in this journal is cited, in accordance with accepted academic practice. No use, distribution or reproduction is permitted which does not comply with these terms.
*Correspondence: Fábio D. Nascimento, fdnascimento@gmail.com