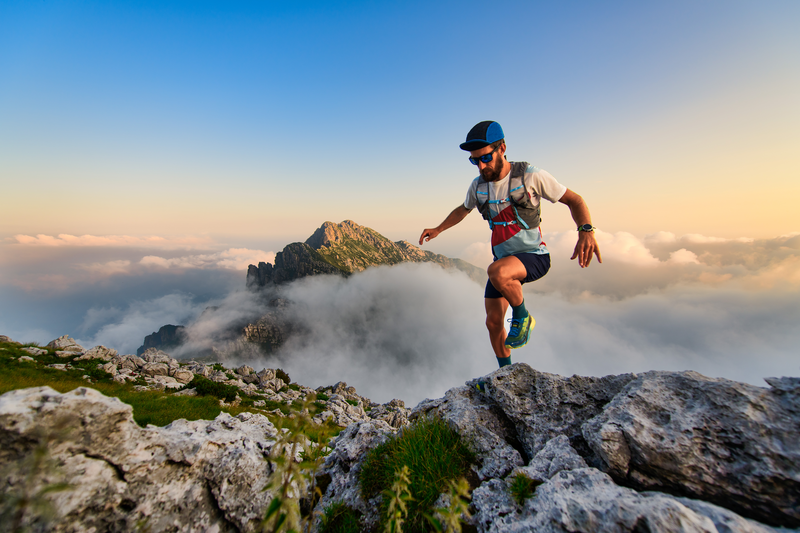
94% of researchers rate our articles as excellent or good
Learn more about the work of our research integrity team to safeguard the quality of each article we publish.
Find out more
REVIEW article
Front. Pharmacol. , 17 November 2021
Sec. Neuropharmacology
Volume 12 - 2021 | https://doi.org/10.3389/fphar.2021.765075
Ischemic stroke (IS) is a disease that is characterized by high mortality and disability. Recent studies have shown that LncRNA-mediated competing endogenous RNA (ceRNA) networks play roles in the occurrence and development of cerebral I/R injury by regulating different signaling pathways. However, no systematic analysis of ceRNA mechanisms in IS has been reported. In this review, we discuss molecular mechanisms of LncRNA-mediated ceRNA networks under I/R injury. The expression levels of LncRNAs, microRNAs (miRNAs), and messenger RNAs (mRNAs) and their effects in four major cell types of the neurovascular unit (NVU) are also involved. We further summarize studies of LncRNAs as biomarkers and therapeutic targets. Finally, we analyze the advantages and limitations of using LncRNAs as therapeutics for IS.
Ischemic stroke (IS) is a neurological disorder that is characterized by blockage of blood vessels and accounts for approximately 87% of strokes (Fann et al., 2013; Lin et al., 2016; Kuriakose and Xiao 2020). Currently, the accepted therapeutic strategy for IS is vascular recanalization therapy which including thrombolysis (with agents such as tissue plasminogen activators), mechanical thrombectomy, and the combination of them. However, reperfusion therapy must be applied within a very short period, which drastically limits the population that is eligible for treatment. Moreover, ischemia-reperfusion (I/R) injury occurs when blood is resupplied to cerebral ischemic tissues, as it is harmful to blood vessels and brain cells such as brain microvascular endothelial cells (BMECs), neurons, and microglial cells. The extent of the ensuing lesion is dependent on the active and complicated interaction between vascular cells, neurons, and glial cells (Trendelenburg and Dirnagl 2005). The precise mechanisms underlying I/R injury remain unknown. However, oxidative stress, inflammation, autophagy, apoptosis, and blood-brain barrier (BBB) disruption are potential mechanisms (Zhu et al., 2020).
Long non-coding RNAs (LncRNAs), a class of non-coding RNAs that are more than 200 nucleotides in length (Kapranov et al., 2007), have been widely studied. It has been reported that LncRNAs affect the occurrence and outcome of several diseases by regulating chromatin modification, post-transcription, and transcription (Ma et al., 2013; McDonel and Guttman 2019; Nair et al., 2020). LncRNAs are also involved in the pathological progression of tumors, nervous system disorders, cardiovascular diseases, and other diseases (Schmitz et al., 2016; Peng et al., 2017; Huang 2018; Wolska et al., 2020). Recent studies have shown that LncRNAs, such as lncRNA metastasis-associated lung adenocarcinoma transcript 1 (MALAT1), play important roles in IS (Zhang et al., 2017a). Another class of non-coding RNAs is microRNAs (miRNAs), which are single-stranded endogenous RNAs with a length of 19–25 nt (Lu and Rothenberg 2018). It has been shown that miRNAs function by targeting the 3ʹ-untranslated (3ʹ-UTR) region of messenger RNAs (mRNAs), thus inhibiting protein synthesis or promoting mRNA degradation (Kabekkodu et al., 2018). Since miRNAs are involved in disease development, they have been a potential target for therapeutic approaches, especially in cancer management (Rupaimoole and Slack 2017; Fan et al., 2019).
The competing endogenous RNA (ceRNA) hypothesis was first proposed by Salmena et al. in 2011 (Salmena et al., 2011). The concept suggests that there are ceRNAs such as LncRNAs, circular RNAs, pseudogenes, and mRNAs in cells. Additionally, ceRNAs can competitively bind to the same miRNA by interacting with miRNA response elements (MREs) to build communication networks between messenger RNAs and non-coding RNAs (Salmena et al., 2011; Sanchez-Mejias and Tay 2015). Thus, LncRNAs can compete with an mRNA, bind to the same miRNA, and regulate the expression of the mRNA if they have the same MRE (Figure 1).
In this review, we focus on LncRNAs that mediate ceRNA networks and regulate key processes involved in I/R injury to explore the potential roles of LncRNAs in regulating IS. We have also summarized the molecular mechanisms of the LncRNA-miRNA-mRNA axis in autophagy, apoptosis, angiogenesis, microglial activation, and polarization in different cell lines (Table 1). Furthermore, we have discussed the therapeutic implications and challenges of LncRNAs in IS.
Studies included in the review were obtained by searching the PubMed database. The following search syntaxes were used (long non-coding RNA) AND (ischemic stroke) and (competing endogenous RNA) AND (ischemic stroke). Review articles and meta-analyses were included for possible support (Figure 2).
The focal areas of IS are the ischemic core area and the penumbra. In the ischemic core area, failure of the ion pump of the neuronal cell membrane and energy metabolism leads to irreversible damage to the brain tissue. However, there are collateral circulations and surviving neurons in the ischemic penumbra. Thus, restoring blood supply to the ischemic penumbra as soon as possible and using effective neuroprotective drugs are important research aspects in IS. Furthermore, prevention and inhibition of neuronal cell injury are potential treatment strategies. In this section, we summarize the effects and ceRNA networks of LncRNAs in neurons and provide evidence for the development of RNA drugs (Figure 3).
In a previous study, LncRNA MEG3 expression was upregulated in middle cerebral artery occlusion/reperfusion (MCAO/R) models, which mainly protected ischemic neurons. Additionally, MEG3 served as a ceRNA for microRNA-21 (miR-21), which was downregulated in vivo and in vitro in contrast to MEG3. Furthermore, programmed cell death 4 (PDCD4), a gene that mediates neuronal cell death, binds to miR-21. Overexpression of miR-21 resulted in protection against oxygen-glucose deprivation and reoxygenation (OGD/R)-induced apoptosis, whereas knockdown of MEG3 resulted in protection against I/R injury and improved neurological function in IS patients (Yan et al., 2017). Similarly, Liang et al. observed that inhibition of MEG3 expression contributed to pyroptosis via activation of the miR-485/absent in melanoma 2 (AIM2)/caspase-1 pathway (Liang et al., 2020). Moreover, MEG3 targets miR-424-5p via neuronal apoptosis mediated via the mitogen-activated protein kinase (MAPK) pathway (Xiang et al., 2020).
In a previous study, LncRNA H19 expression was found to be upregulated in SD rats, C57 mice, and an OGD cell culture model. Additionally, H19 siRNA improved apoptosis and inflammation and influenced the expression of miR-29b, sirtuin1 (SIRT1), and peroxisome proliferator-activated receptor-gamma coactivator (PGC)-1α expression in OGD cell culture models (Xu et al., 2021). Another report indicated that H19 levels were elevated in patients with anterior circulation ischemia and that H19 knockdown resulted in reduced apoptosis in OGD neuronal cells. Furthermore, inhibition of H19 expression in a rat model of MCAO/R resulted in a marked decrease in brain infarct volume, neurological deficits, and neuronal apoptosis. It was also found that H19 plays a critical role in neuronal apoptosis as a ceRNA by interfering with the binding of inhibitor of DNA binding 2 (Id2) and PTEN to miR-19a and miR-19a-3p (Xiao et al., 2019; Gao N. et al., 2020).
LncRNA GAS5 expression is attributed to negative regulation of cell survival. Upregulation of GAS5 expression has been demonstrated both in vivo and in vitro. It has also been reported that GAS5 knockdown results in a significant increase in cell viability, suppression of caspase-3 activation, and the induction of neuronal apoptosis after OGD. Moreover, GAS5 serves as a molecular sponge for miR-137 to regulate Notch1 expression and reduce neuron survival (Chen et al., 2018). Wu et al. revealed the functions of the GAS5/miR-455-5p/phosphatase and tension homolog deleted on chromosome ten (PTEN) axis in cerebral IS. It was found that GAS5 and PTEN levels were upregulated whereas miR-445-5p level was downregulated in brain and PC12 cell models of MCAO/R injury that were subjected to OGD/R. Additionally, suppression of GAS5 expression or miR-455-5p overexpression improved neurobehavior and decreased apoptosis and oxidative injury (Wu et al., 2021).
SNHGs are a group of LncRNAs that are overexpressed in various cancers. They include SNHG1, SNHG3, SNHG5, SNHG6, SNHG7, SNHG12, SNHG15, SNHG16, and SNHG20 (Zimta et al., 2020). It has been reported that SNHG6 functions as a ceRNA for miR-181c-5p to regulate Bcl2 interacting mediator of cell death (BIM) expression and promote apoptosis (Zhang X. et al., 2019). In a previous study, SNHG7 expression was downregulated in C57 mice and PC12 cells. Additionally, SNHG7 overexpression or suppression of miR-9 expression resulted in decreased reactive oxygen species and malondialdehyde levels and increased cell viability. It has been reported that SNHG7 reduces OGD/R-induced neuronal apoptosis and functions as a ceRNA for miR-9 and SIRT1 targeted by miR-9. In summary, LncRNA SNHG7 serves as a ceRNA for miR-9 to regulate SIRT1 activity, thus alleviating neuronal injury (Zhou et al., 2020).
In another study, SNHG12 was highly expressed in SH-SY5Y cells subjected to OGD/R. Furthermore, SNHG12 targeted miR-181a-5p and negatively regulated its expression. Moreover, miR-181a-5p is a target of nerve growth factor receptor 1 (NEGR1) and negatively regulates the expression of NEGR1 in OGD-induced neuronal apoptosis (Yan Y. et al., 2020). SNHG15 expression was also upregulated in C57 mice, neuro-2a (N2a) cells, and PC12 cells in previous studies (Guo et al., 2020; Fan et al., 2021). Guo et al. found that silencing SNHG15 resulted in upregulation of the expression of C-X-C motif chemokine ligand 13 (CXCL13) through suppression of the activation of miR-18a and extracellular signal-regulated kinase (ERK)/mitogen-activated protein kinase kinase (MEK). These resulted in reduced apoptosis and enhanced cell viability (Guo et al., 2020). Furthermore, Fan et al. revealed that downregulation of LncRNA SHNG15 expression plays a protective role in OGD/R-induced neuronal injury through downregulation of the expression of tumor protein p53 inducible nuclear protein 1 (TP53INP1) via miR-445-3p targeting (Fan et al., 2021).
The expression of LncRNA RMST was upregulated whereas that of miR-377 was downregulated in serum samples collected from patients with IS. Furthermore, suppression of RMST expression resulted in reduced oxidative stress and apoptosis in an N2a cell culture model of OGD. Semaphorin 3A (SEMA3A) is a target gene of miR-377, and RMST regulates SEMA3A expression as a sponge for miR-377 (Zhao et al., 2021). A previous study revealed that RMST interacts with heterogeneous nuclear ribonucleoprotein K (hnRNPK) and then regulates the p53/miR-107 axis, thus promoting apoptosis of HT-22 cells (Cheng et al., 2020).
It has been reported that LncRNA ANRIL is involved in neuronal apoptosis, and its expression was decreased in patients with acute IS, rat, and mouse models of MCAO/R, (Feng et al., 2019; Liu et al., 2019; Zhong et al., 2020). Interestingly, one study demonstrated that the level of LncRNA ANRIL in N2a cells significantly decreased within 12 h after OGD but increased at 18 h and peaked at 24 h after OGD (Zhong et al., 2020). Another study also revealed that LncRNA ANRIL expression is downregulated within 8 h after OGD in PC12 cells (Liu et al., 2019). Furthermore, silencing ANRIL aggravated OGD-induced PC12 cell injury via the reduction in cell viability and increase in apoptosis, while overexpression of ANRIL contributed to opposite effects. Overexpression of miR-127 and myeloid cell leukemia-1 (Mcl-1) results in significantly enhanced cell injury. Additionally, miR-127 negatively regulates Mcl-1 expression, whereas ANRIL upregulates Mcl-1 expression by downregulating miR-127 expression (Liu et al., 2019). It has been revealed that ANRIL competitively interacts with miR-199a-5p in N2a cells. Additionally, overexpression of ANRIL or suppression of miR-199a-5p expression results in the protection of cells against I/R injury and improved cell viability through the caveolin-1 (CAV-1)-mediated MEK/ERK pathway (Zhong et al., 2020).
LncRNA MALAT1 has been reported to be highly expressed in both in vivo and in vitro models of ischemia (Guo et al., 2017; Zhang G. et al., 2020; Jia et al., 2021). In addition, It has also been claimed that downregulation of MALAT1 expression alleviates neuronal apoptosis. Furthermore, suppression of MALAT1 expression or overexpression of miR-375 leads to a decrease in the levels of inflammatory factors and lactate dehydrogenase as well as a reduction in apoptosis. Furthermore, miR-375 targets both MALAT1 and phosphodiesterase 4D (PDE4D) (Zhang G. et al., 2020). It has also been reported that MALAT1 knockdown causes a reversal in OGD/R-induced apoptosis and endoplasmic reticulum stress through the targeting of miR-195a-5p and regulating the expression of high mobility group AT-hook1 (HMGA1) (Jia et al., 2021).
KCNQ1OT1 was highly expressed in patients with acute IS, MCAO/R models, and OGD cell culture models in a previous study. Further, downregulation of KCNQ1OT1 expression significantly ameliorated apoptosis in OGD/R treated PC12 cells. It has also been reported that KCNQ1OT1 serves as a ceRNA for miR-140-3p and regulates the expression of hypoxia-inducible factor (HIF)-1α, which is a target of miR-140-3p (Yi et al., 2020). KCNQ1OT1 and MMP8 expressions were significantly increased in neurons but miR-9 was downregulated. Inhibiting KCNQ1OT1 or overexpressing miR-9 protected OGD/R-induced neuronal injury (Wang HJ. et al., 2020; Ren et al., 2020). Another study revealed KCNQ1OT1 promoted neuronal apoptosis via miR-153-3p/forkhead box O3 (FOXO3) axis (Wang HJ. et al., 2020).
Other LncRNA-mediated ceRNA networks have been studied in neuronal cell cultures. For instance, LncRNA taurine-upregulated gene 1 (TUG1) has been shown to play an important role in apoptosis in IS. TUG1 expression was found to be upregulated in cultured neurons under OGD insult. Additionally, knockdown of TUG1 reportedly results in decreased apoptosis and increased cell survival in vitro. It has been found that TUG1 directly binds to miR-9 and that downregulating miR-9 expression reverses the suppressive effect of TUG1 on B-cell lymphoma-2 like-11 (Bcl2l11) expression (Chen et al., 2017). In a study conducted by Wang et al., LncRNA HOXA transcript at the distal tip (HOTTIP) was found to induce neuronal injury by modulating the miR-143/hexokinase 2 pathway (Wang Y. et al., 2018). In other studies, the LncRNA Gm11974/miR-766-3p/nuclear receptor subfamily 3 group C member 2 (NR3C2) and LncRNA cardiac hypertrophy-related factor (CHRF)/miR-126/sex-determining region Y box 6 (SOX6) axes protected against cerebral I/R injury and reduce neuronal apoptosis (Cai et al., 2019; Gai et al., 2019). Jing et al. also found that overexpression of LncRNA mu-1-opioid peptide receptor (Oprm1) results in the alleviation of apoptosis from cerebral I/R injury through the Oprm1/miR-155/GATA binding protein 3 (GATA3) axis (Jing et al., 2019). Furthermore, the LncRNA AK038897/miR-26a-5p/death-associated protein kinase 1 (DAPK1), FGD5 antisense RNA 1 (FGD5-AS1)/miRNA-223/insulin-like growth factor (IGF)-1 receptor (IGF1R), tumor-associated LncRNA expressed on chromosome 2 (TALNEC2)/miR-650/apoptotic peptidase activating factor 1 (APAF1), FOXD3-AS1/miR-765/BCL2L13, and RNA imprinted and accumulated in nucleus (Rian)/miR-144-3p/GATA3 axes have been found to attenuate apoptosis after cerebral I/R injury (Zhang XQ. et al., 2019; Wei et al., 2019; Lu et al., 2020; Yao et al., 2020; Chen M. et al., 2021; Cao et al., 2021).
Several ceRNA networks were related to the autophagy of neurons. Luo et al. suggested that the MEG3/miR-378/growth factor receptor-bound protein 2 (GRB2) axis plays a role in neuronal autophagy and impairment of neurological function in IS (Luo et al., 2020). Knockdown of KCNQ1OT1 resulted in reduced infarct volume in mice subjected to MCAO as well as neuronal autophagy via the miR-200a/forkhead box O3 (FOXO3)/autophagy-related protein 7 (ATG7) axis (Yu et al., 2019). Downregulation of MALAT1 expression was shown to result in ischemic injury and autophagy suppression. MALAT1 served as a molecular sponge for miR-30a and interfered with the inhibitory effect of miR-30a on ischemic injury and autophagy by suppressing the expression of Beclin-1, which is a direct target of miR-30a. Altogether, suppression of MALAT1 expression attenuates neuronal cell death via the miR-30a/Beclin-1 axis (Guo et al., 2017). LncRNA SNHG14 and BINP3, a pro-apoptotic mitochondrial protein, were upregulated in OGD/R-induced HT22 cells, but miR-182-5p was downregulated. What’s more, SNHG14 could regulate the expression of BNIP3 via targeting to miR-182-5p. Overall, SNHG14 promoted mitophagy via miR-182-5p/BINP3(Deng et al., 2020).
In a previous study, LncRNA SNHG14 was found upregulated in MCAO/R rats and OGD/R-induced PC-12 cells. SNHG14 acted as a sponge of miR-136-5p and positively regulated the expression of Rho-associated coiled-coil-containing protein kinase 1 (ROCK1), thus promoting neurological impairment and inflammation (Zhong et al., 2019). P65 is a subunit of nuclear factor NF-κB, which is related to an inflammatory response. Li et al. underlined that LncRNA H19 targeted p65 by sponging of miR-138-5p, thus promoting inflammatory response and improving neurological functions (Li et al., 2020).
BMECs are important components of the cerebral microvascular system and form a part of the blood-brain barrier (BBB). I/R injury leads to autophagy and apoptosis of BMECs, which accounts for BBB disruption and enhances vascular permeability, thus resulting in an unfavorable prognosis among patients suffering from IS (Li et al., 2014). Furthermore, vascular remodeling plays an important role in ischemic cardiovascular diseases. Angiogenesis in ischemic areas promotes blood supply to ischemic areas (Xu et al., 2018; Zhao et al., 2020). Thus, protecting BMECs from I/R injury or promoting angiogenesis can improve the prognosis of stroke (Zhu et al., 2021). In this section, we have discussed the roles of LncRNAs in BMECs after cerebral I/R injury (Figure 4).
FIGURE 4. LncRNA-mediated ceRNA networks in BMECs involved in angiogenesis, autophagy, apoptosis, and inflammation.
LncRNA MALAT1 is one of the most highly upregulated I/R- or OGD/R-responsive endothelial LncRNAs that plays roles in apoptosis and inflammation (Zhang et al., 2017b; Yang et al., 2018; Zhang et al., 2018). One study revealed that MALAT1 promoted BMEC autophagy and survival by binding to miR-26b and downregulating its expression. Furthermore, miR-26b inhibited autophagy and cell survival, whereas overexpression of MALAT1 resulted in a reversal of this effect by promoting the expression of Unc-51 like autophagy activating kinase 2 (ULK2), a target of miR-26b (Li Z. et al., 2017). However, it was found that MALAT1 expression was downregulated in an OGD BMEC model. It was also claimed that MALAT1 can suppress apoptosis and function as a molecular sponge of miR-205-3p to modulate PTEN expression (Gao and Wang 2020). Altogether, MALAT1 can be a protective factor against BMEC injury. Furthermore, it has been reported that overexpression of miR-15a-5p results in decreased cell proliferation and increased apoptosis via downregulation of Bcl2 expression. It has been indicated that SNHG16 expression gradually decreases following OGD/R and that its overexpression results in the downregulation of miR-15a-5p expression, which promotes cell proliferation and decreases apoptosis. Overall, SNHG16 protects HBMECs from OGD/R-induced apoptosis via the miR-15a-5p/Bcl2 axis (Teng et al., 2020). In another study, LncRMST expression was upregulated in HBMECs and bEnd.3 cells subjected to OGD. Silencing LncOGD-1006 also aggravated OGD-induced injury in bEnd.3 cells via increased apoptosis, whereas overexpression of LncOGD-1006 led to opposite effects. Furthermore, LncOGD-1006 functions as a ceRNA for miR-184-5p to facilitate the expression of conserved anti-apoptotic protein 1 (CAAP1) (Chen JY. et al., 2020).
Vascular endothelial growth factor (VEGF) is an angiogenesis inducer that promotes the growth of vascular endothelial cells. It has been reported that HIF-1 plays a key role in hypoxic responses and regulates VEGF expression (Ferrara 2004).
The LncRNA HIF1A-AS2 regulates the expression of HIF-1α by sponging miR-153-3p. In permanent MCAO and human umbilical vein endothelial cell (HUVEC) models, LncRNA HIF1A-AS2 expression is upregulated, whereas miR-153-3p expression is reduced as a result of higher protein levels of HIF-1α, VEGFA, and Notch1. Moreover, HUVEC viability, migration ability, and tube formation are promoted. Therefore, activating the LncRNA HIF1A-AS2/miR-153-3p/HIF-1α/VEGFA/Notch1 axis promotes angiogenesis in HUVECs (Li L. et al., 2017). Furthermore, SHNG12 upregulation or knockdown contributes to the regulation of VEGFA and fibroblast growth factor-beta mRNA and protein levels under OGD/R conditions. Additionally, capillary-like tube formation changes with SNHG12 expression, which indicates that SNHG12 promotes BMEC angiogenesis by targeting miR-199a (Long et al., 2018). It was shown in another study that SNHG12 improves angiogenesis following IS by regulating the miR-150/VEGF axis (Zhao et al., 2018). LncRNA Snhg1 promoted BMEC migration and tube formation after OGD insult in a previous study. Moreover, mechanistic studies have suggested that Snhg1 targets miR-338a and regulates HIF-1α and VEGF expression (Wang Z. et al., 2018). It has been reported that LncRNA MALAT1 protected against angiogenesis in HBMECs under OGD conditions via the miR-205-5p/VEGFA axis (Gao C. et al., 2020). Additionally, LncRNA metastasis-associated colon cancer 1 (MACC1)-AS1, which sponges miR-6867-5p/TWIST1, regulates the proliferation, survival, and migration of hypoxic HBMECs(Yan G. et al., 2020). Differentiation antagonizing non-protein coding RNA (DANCR) overexpression results in the promotion of spliced X-box binding protein l (XBP1s) expression in BMECs subjected to OGD. It has been shown that miR-33a-5p binds to DANCR and the 3ʹ-UTR of XBP1. Moreover, miR-33a-5p overexpression causes inhibition of cell proliferation, migration, and angiogenesis. In summary, DANCR promotes cell survival and angiogenesis by regulating the miR-33a-5p/XBP1s axis (Zhang M. et al., 2020).
Vascular cell adhesion molecule 1 (VCAM1), an endothelial-specific marker, is related to inflammation in cerebrovascular disease (Maglinger et al., 2021; Wang et al., 2021). It has been shown that miR-204-5p is a target of VCAM1 and that RMST serves as a ceRNA that regulates VCAM1 expression by sponging miR-204-5p. Consequently, knockdown of RMST results in reduced OGD-induced injury through regulation of the miR-204-5p/VCAM1 axis (Yin et al., 2021).
Resting microglia can be activated and polarized into two phenotypes in IS. M1 microglia produce pro-inflammatory mediators such as tumor necrosis factor α (TNF-α), interleukin (IL)-6, interferon-γ, IL-1β, inducible nitric oxide synthase, and proteolytic enzymes (matrix metalloproteinase [MMP] 9 and MMP3), which are harmful to brain tissues. Conversely, M2 microglia, which are also called tissue restorative microglia, produce pro-angiogenic and anti-inflammatory factors such as IL-10, transforming growth factor β, IGF, and VEGF, which promote ischemia (Qin et al., 2019). Here we have discussed the roles of several LncRNAs in microglial cells (Figure 5).
FIGURE 5. Mechanisms of action of LncRNA SNHG14, Snhg8, and OIP5-AS1 in microglial cells in the pathogenesis of IS. ↑ indicates upregulation, whereas ↓ indicates downregulation.
The expression of LncRNA Snhg8 was downregulated in ischemic regions in mice subjected to MCAO/R and in primary microglial cells subjected to OGD. Additionally, LncRNA Snhg8 serves as a ceRNA for miR-425-5p, which promotes microglial inflammation and BMEC injury by targeting the SIRT1/nuclear factor-κB (NF-κB) axis (Tian et al., 2021). The expression of the LncRNA SNHG14 was found to be strongly upregulated in mice with ischemic cerebral infarction and BV2 cells following OGD treatment. Gain and loss of function experiments revealed that SNHG14 regulates BV2 cell activation, TNF-α and nitric oxide production, and miR-145-5p and PLA2 group IVA (PLA2G4A) expression levels. Furthermore, overexpression of miR-145-5p caused a reversal of BV2 cell activation. Further studies have supported that SNHG14 directly binds to miR-145-5p and that the binding site of miR-145-5p exists on the 3ʹ-UTR of PLA2G4A. Overall, LncRNA SNHG14 promotes microglial activation by regulating the miR-145-5p/PLA2G4A axis (Qi et al., 2017). It has also been reported that the expression of LncRNA Opa-interacting protein 5 antisense RNA 1 (OIP5-AS1) and C1q/TNF-related protein 3 (CTRP3) is downregulated, while that of miR-186-5p is upregulated in BV2 cells subjected to OGD/R. Furthermore, it has been indicated that miR-186-5p promotes inflammation and oxidative stress in microglia and shares targets with CTRP3 and OIP5-AS1. Moreover, overexpression of LncRNA OIP5-AS1 promotes protection against I/R injury−induced inflammation and oxidative stress in microglia/macrophages via the miR-186-5p/CTRP3 axis (Chen Y. et al., 2021). Song. et al. found that the expression of LncRNA KCNQ1OT1 and NLRP3 was upregulated in HMC3 cells, while the expression of miR-30e-3p was downregulated. Further studies revealed that KCNQ1OT1 regulated cell inflammation and apoptosis by miR-30e-3p/NLRP3 pathway (Song et al., 2021). High-mobility group box 1(HMGB1) is a danger-associated molecular and takes part in inflammatory processes, which can be released under hypoxic and ischemic conditions. In previous studies, the expression of LncRNA Malat1 was augmented in MCAO/R mice. Further, Malat1 blocked the combination between miR-181c-5p and HMGB1, thus leading to an increase of the HMGB1 level. Totally, LncRNA Malat1/miR-181c-5p/HMGB1 axis may play a vital role in poststroke inflammation (Cao et al., 2020).
There are fewer studies on LncRNAs in astrocytes than in other cell lines. Aquaporin 4 (AQP4) is highly expressed in astrocytes and involved in the development of brain edema following intracerebral hemorrhage (Fu et al., 2007). It has been revealed that LncRNA MALAT1 exacerbates cerebral I/R injury, which regulates the expression of AQP4 by competitively binding to miR-375 (Wang H. et al., 2020). Moreover, LncRNA TUG1 aggravated apoptosis by upregulating AQP4 via miR-145 (Shan et al., 2020).
Neuroprotective treatment strategies for IS face significant challenges in clinical settings. Numerous preclinical studies have demonstrated the potential benefits of neuroprotective therapy in animal models of IS. However, the clinical application of neuroprotective therapies tested in preclinical studies has mostly failed. Thus, it is critical to identify multi-target drugs to treat IS. The NVU is a multicellular complex composed of neurons, endothelial cells, astrocytes, myocytes, pericytes, microglia, and extracellular matrix. It is an intricate network that maintains a balanced neuronal microenvironment in the body (Muoio et al., 2014). LncRNAs that are widely expressed in cells of the NVU exert specific biological functions, which include multi-target and multi-link comprehensive regulation and precise regulation of the downstream network. However, to date, no study has investigated the relationship between LncRNAs and the NVU. In this section, we have summarized the roles of several LncRNAs expressed in different cells and identified their functions in IS.
The functions of LncRNA MALAT1 have been examined in different brain cells in previous studies. MALAT1 expression is upregulated after OGD/R treatment in neurons, BV-2 cells, and astrocytes, which results in MALAT1 acting as a harmful factor in I/R injury by promoting cell death via apoptosis and inflammation (Guo et al., 2017; Wang H. et al., 2020; Zhang G. et al., 2020; Cao et al., 2020; Jia et al., 2021). However, MALAT1 plays a protective role in BMECs subjected to OGD/R because it reduces apoptosis and promotes angiogenesis (Li Z. et al., 2017; Gao C. et al., 2020; Gao and Wang 2020). In summary, the roles of LncRNA MALAT1 in IS include a protective effect on endothelial cells; however, it may induce cell death in neurons and astrocytes.
It has been reported that the expression of LncRNA RMST is upregulated in HT22, bEnd.3, N2a, and BV2 cells, as well as in HBMECs and IS patients (Hou and Cheng 2018; Sun et al., 2019; Cheng et al., 2020; Yin et al., 2021; Zhao et al., 2021). Additionally, suppressing RMST expression results in reduced apoptosis of bEnd.3, HBMECs, and N2a cells, whereas overexpression of RMST promotes apoptosis of HT22 and BV2 cells. Collectively, these data indicate that LncRNA RMST may serve as an apoptosis promoter to regulate cerebral I/R injury.
Some studies related to lncRNAs have been conducted in patients, in order to explore the clinical values (Figure 6). One study showed that lnc-ITSN1-2 expression was positively correlated with the National Institutes of Health Stroke Scale (NIHSS) score and reflected the severity of stroke. (Zhang and Niu 2020). Another case study was conducted to investigate the relationship between LncRNA MEG3 and prognostic value. MEG3 was upregulated in IS patients, positively associated with the NIHSS score, and negatively related to the prognosis of IS patients (Wang M. et al., 2020). Thus, MEG3 is a potential marker for diagnosis and prognosis. For prognosis, high lnc-ITSN1-2 expression was correlated with worse Relapse-Free Survival (RFS) in AIS patients (Zhang and Niu 2020). Higher Myocardial infarction associated transcript (MIAT) expression had a relatively poor prognosis. Meanwhile, the multivariate analysis revealed that MIAT was an independent prognostic marker of functional outcome and death in patients with IS. Data suggested that MIAT might be a potential diagnostic and prognostic indicator in IS (Zhu et al., 2018). LncRNA HULC is correlated with higher AIS risk, increased disease severity, and worse prognosis in AIS patients (Ren et al., 2021). Meanwhile, it associates with higher IL-6, elevated intercellular adhesion molecule 1 (ICAM1), and lower miR-9 AIS patients (Chen X. et al., 2020). The H19 gene plays a functional role in increasing the prevalence of IS risk factors. The upregulation of H19 may be considered as a diagnostic biomarker in IS among the Iranian population. But ROC curve analysis revealed that the peripheral blood expression level of H19 could not be considered as a promising marker for the functional outcome and mortality prediction of IS patients, thus it cannot serve as a useful prognostic marker (Rezaei et al., 2021).
Nucleic acid-based RNA targeting approaches have been concerned by researchers. CeRNA networks provide a variety of therapies, such as ncRNA replacement therapy and ncRNA inhibition therapy (Dragomir et al., 2020). NcRNA replacement therapy is devoted to improving conditions of IS patients by supplementing ncRNAs. NcRNA inhibition therapy focuses on inhibiting the expression of harmful ncRNAs to slow the progress of IS. Furthermore, LncRNAs can be detected in the serum, which is more efficient in supporting the diagnosis and classification of IS patients.
However, the clinical application of LncRNAs has several limitations. Firstly, besides ceRNA network mechanisms, lncRNAs regulate gene expressions at multiple levels, including regulating chromatin modification, post-transcription, and transcription. Secondly, the levels of LncRNA, miRNA, and mRNA can be influenced by other molecular counterpart conditions, which makes it difficult to fully explore the intrinsic regulation mechanism of ceRNA and therefore require further investigations. Thirdly, since lncRNAs are not highly conserved among species, studies are mostly conducted in animal and cell models. Thus, there is a lack of reports on clinical studies on LncRNAs. Forthly, The development of ncRNA drugs is facing great difficulties, such as the degradation and delivery of ncRNA drugs. Finally, there is no sufficient information about exosomal lncRNAs in stroke pathogenesis, thus the functions of LncRNAs in NUV remain unclear and considerable research is needed in this field.
The pathological process of cerebral I/R injury is complex. The lack of oxygen and energy causes ion pump failure, apoptosis, inflammation, glutamate excitatory toxicity, and oxidative stress, which are harmful to the cell components of NVU, such as neurons, glial cells, BMECs, and astrocytes. Previous studies have identified LncRNAs that are related to the occurrence and progression of IS. In this review, we first summarized how the LncRNA-mediated ceRNA networks take part in the process of ischemic stroke in different brain cell lines. Available data indicate that LncRNA MALAT1, MEG3, and RMST serving as ceRNAs, play an important role in neuroprotection, which mainly involves regulation of neuronal cell death by targeting miRNAs and mRNAs, such as PDC4D, caspases, SIRT1, and Beclin-1. Furthermore, some studies have identified the anti-apoptotic, anti-inflammatory, and angiogenic roles of LncRNAs in BMECs. Several LncRNAs function as ceRNAs to bind to miRNAs and regulate the expression of VEGF, which is an important angiogenic factor. Some ceRNA networks are also involved in the activation and polarization of microglia, whereas others can regulate the expression of inflammatory factors. Secondly, we analyzed the functions of LncRNAs that are expressed in different types of brain cells to better understand the relationship between LncRNAs and the NVU. Thirdly, some researchers have shown that the expression level of LncRNAs is related to neurological deficits and prognosis among IS patients. These results indicate that LncRNAs may be potential therapeutic targets and biomarkers. What’s more, lncRNA mediated ceRNA networks are involved in different pathological mechanisms in aggravating I/R damage; however, the underlying mechanisms are not fully understood. Although studies related to lncRNAs have been conducted based on clinical samples, the differences between different races and nationalities blocked lncRNAs from being effective IS markers in diagnosing and prognosing. More studies should be conducted to explore the clinical and medical value of lncRNAs or ceRNAs in IS.
SZ initiated this review, collected the references, mapped figures, and drafted the manuscript. TZ revised our first draft and provided valuable comments. QL revised our manuscript. GS and XS supported the funding and revised the manuscript. All authors approved the submitted version.
The work was supported by the National Natural Science Foundation of China (No.U20A20405), the major projects from the National Natural Science Foundation of China (No.81891012), the National Natural Science Foundation of China (No.81773938), and Guangdong Province Key Field R&D Program Project (No. 2020B111111002).
The authors declare that the research was conducted in the absence of any commercial or financial relationships that could be construed as a potential conflict of interest.
The Reviewer M.P declared a shared affiliation with one of the Authors T.Z to the Handling Editor at the time of review.
All claims expressed in this article are solely those of the authors and do not necessarily represent those of their affiliated organizations, or those of the publisher, the editors and the reviewers. Any product that may be evaluated in this article, or claim that may be made by its manufacturer, is not guaranteed or endorsed by the publisher.
Cai, J., Shangguan, S., Li, G., Cai, Y., Chen, Y., Ma, G., et al. (2019). Knockdown of lncRNA Gm11974 Protect against Cerebral Ischemic Reperfusion through miR-766-3p/NR3C2 axis. Artif. Cell Nanomed Biotechnol 47 (1), 3847–3853. doi:10.1080/21691401.2019.1666859
Cao, D. W., Liu, M. M., Duan, R., Tao, Y. F., Zhou, J. S., Fang, W. R., et al. (2020). The lncRNA Malat1 Functions as a ceRNA to Contribute to Berberine-Mediated Inhibition of HMGB1 by Sponging miR-181c-5p in Poststroke Inflammation. Acta Pharmacol. Sin 41 (1), 22–33. doi:10.1038/s41401-019-0284-y
Cao, Y., Gao, W., Tang, H., Wang, T., and You, C. (2021). Long Non-coding RNA TALNEC2 Aggravates Cerebral Ischemia/Reperfusion Injury via Acting as a Competing Endogenous RNAs for miR-650 to Target Apoptotic Peptidase Activating Factor 1. Neuroscience 458, 64–76. doi:10.1016/j.neuroscience.2020.10.010
Chen, F., Zhang, L., Wang, E., Zhang, C., and Li, X. (2018). LncRNA GAS5 Regulates Ischemic Stroke as a Competing Endogenous RNA for miR-137 to Regulate the Notch1 Signaling Pathway. Biochem. Biophys. Res. Commun. 496 (1), 184–190. doi:10.1016/j.bbrc.2018.01.022
Chen, J. Y., Chen, H., Li, T., Yang, L., Ye, X. M., Gao, W. Y., et al. (2020a). LncRNA LncOGD-1006 Alleviates OGD-Induced Ischemic Brain Injury Regulating Apoptosis through miR-184-5p/CAAP1 axis. Eur. Rev. Med. Pharmacol. Sci. 24 (23), 12324–12333. doi:10.26355/eurrev_202012_24025
Chen, M., Wang, F., and Wang, H. (2021a). Silencing of lncRNA XLOC_035088 Protects Middle Cerebral Artery Occlusion-Induced Ischemic Stroke by Notch1 Signaling. J. Neuropathol. Exp. Neurol. 80 (1), 60–70. doi:10.1093/jnen/nlaa129
Chen, S., Wang, M., Yang, H., Mao, L., He, Q., Jin, H., et al. (2017). LncRNA TUG1 Sponges microRNA-9 to Promote Neurons Apoptosis by Up-Regulated Bcl2l11 under Ischemia. Biochem. Biophys. Res. Commun. 485 (1), 167–173. doi:10.1016/j.bbrc.2017.02.043
Chen, X., Zhang, X., Su, C., and Huang, S. (2020b). Long Noncoding RNA HULC in Acute Ischemic Stroke: Association with Disease Risk, Severity, and Recurrence-free Survival and Relation with IL-6, ICAM1, miR-9, and miR-195. J. Clin. Lab. Anal. 34 (11), e23500. doi:10.1002/jcla.23500
Chen, Y., Liu, W., Chen, M., Sun, Q., Chen, H., and Li, Y. (2021b). Up-regulating lncRNA OIP5-AS1 Protects Neuron Injury against Cerebral Hypoxia-Ischemia Induced Inflammation and Oxidative Stress in Microglia/macrophage through Activating CTRP3 via Sponging miR-186-5p. Int. Immunopharmacol 92, 107339. doi:10.1016/j.intimp.2020.107339
Cheng, H., Sun, M., Wang, Z. L., Wu, Q., Yao, J., Ren, G., et al. (2020). LncRNA RMST-Mediated miR-107 Transcription Promotes OGD-Induced Neuronal Apoptosis via Interacting with hnRNPK. Neurochem. Int. 133, 104644. doi:10.1016/j.neuint.2019.104644
Deng, Z., Ou, H., Ren, F., Guan, Y., Huan, Y., Cai, H., et al. (2020). LncRNA SNHG14 Promotes OGD/R-induced Neuron Injury by Inducing Excessive Mitophagy via miR-182-5p/BINP3 axis in HT22 Mouse Hippocampal Neuronal Cells. Biol. Res. 53 (1), 38. doi:10.1186/s40659-020-00304-4
Dragomir, M. P., Kopetz, S., Ajani, J. A., and Calin, G. A. (2020). Non-coding RNAs in GI Cancers: from Cancer Hallmarks to Clinical Utility. Gut 69 (4), 748–763. doi:10.1136/gutjnl-2019-318279
Fan, H., Yuan, F., Yun, Y., Wu, T., Lu, L., Liu, J., et al. (2019). MicroRNA-34a Mediates Ethanol-Induced Impairment of Neural Differentiation of Neural Crest Cells by Targeting Autophagy-Related Gene 9a. Exp. Neurol. 320, 112981. doi:10.1016/j.expneurol.2019.112981
Fan, Y., Wei, L., Zhang, S., Song, X., Yang, J., He, X., et al. (2021). LncRNA SNHG15 Knockdown Protects against OGD/R-Induced Neuron Injury by Downregulating TP53INP1 Expression via Binding to miR-455-3p. Neurochem. Res. 46 (4), 1019–1030. doi:10.1007/s11064-020-03222-9
Fann, D. Y., Lee, S. Y., Manzanero, S., Chunduri, P., Sobey, C. G., and Arumugam, T. V. (2013). Pathogenesis of Acute Stroke and the Role of Inflammasomes. Ageing Res. Rev. 12 (4), 941–966. doi:10.1016/j.arr.2013.09.004
Feng, L., Guo, J., and Ai, F. (2019). Circulating Long Noncoding RNA ANRIL Downregulation Correlates with Increased Risk, Higher Disease Severity and Elevated Pro-inflammatory Cytokines in Patients with Acute Ischemic Stroke. J. Clin. Lab. Anal. 33 (1), e22629. doi:10.1002/jcla.22629
Ferrara, N. (2004). Vascular Endothelial Growth Factor: Basic Science and Clinical Progress. Endocr. Rev. 25 (4), 581–611. doi:10.1210/er.2003-0027
Fu, X., Li, Q., Feng, Z., and Mu, D. (2007). The Roles of Aquaporin-4 in Brain Edema Following Neonatal Hypoxia Ischemia and Reoxygenation in a Cultured Rat Astrocyte Model. Glia 55 (9), 935–941. doi:10.1002/glia.20515
Gai, H. Y., Wu, C., Zhang, Y., and Wang, D. (2019). Long Non-coding RNA CHRF Modulates the Progression of Cerebral Ischemia/reperfusion Injury via miR-126/SOX6 Signaling Pathway. Biochem. Biophys. Res. Commun. 514 (2), 550–557. doi:10.1016/j.bbrc.2019.04.161
Gao, C., Zhang, C. C., Yang, H. X., and Hao, Y. N. (2020a). MALAT1 Protected the Angiogenesis Function of Human Brain Microvascular Endothelial Cells (HBMECs) under Oxygen Glucose Deprivation/re-Oxygenation (OGD/R) Challenge by Interacting with miR-205-5p/VEGFA Pathway. Neuroscience 435, 135–145. doi:10.1016/j.neuroscience.2020.03.027
Gao, N., Tang, H., Gao, L., Tu, G. L., Luo, H., and Xia, Y. (2020b). LncRNA H19 Aggravates Cerebral Ischemia/Reperfusion Injury by Functioning as a ceRNA for miR-19a-3p to Target PTEN. Neuroscience 437, 117–129. doi:10.1016/j.neuroscience.2020.04.020
Gao, Q., and Wang, Y. (2020). Long Noncoding RNA MALAT1 Regulates Apoptosis in Ischemic Stroke by Sponging miR-205-3p and Modulating PTEN Expression. Am. J. Transl Res. 12 (6), 2738–2748.
Guo, D., Ma, J., Yan, L., Li, T., Li, Z., Han, X., et al. (2017). Down-Regulation of Lncrna MALAT1 Attenuates Neuronal Cell Death through Suppressing Beclin1-dependent Autophagy by Regulating Mir-30a in Cerebral Ischemic Stroke. Cell Physiol Biochem 43 (1), 182–194. doi:10.1159/000480337
Guo, T., Liu, Y., Ren, X., Wang, W., and Liu, H. (2020). Promoting Role of Long Non-coding RNA Small Nucleolar RNA Host Gene 15 (SNHG15) in Neuronal Injury Following Ischemic Stroke via the MicroRNA-18a/CXC Chemokine Ligand 13 (CXCL13)/ERK/MEK Axis. Med. Sci. Monit. 26, e923610. doi:10.12659/MSM.923610
Hou, X. X., and Cheng, H. (2018). Long Non-coding RNA RMST Silencing Protects against Middle Cerebral Artery Occlusion (MCAO)-induced Ischemic Stroke. Biochem. Biophys. Res. Commun. 495 (4), 2602–2608. doi:10.1016/j.bbrc.2017.12.087
Huang, Y. (2018). The Novel Regulatory Role of lncRNA-miRNA-mRNA axis in Cardiovascular Diseases. J. Cel Mol Med 22 (12), 5768–5775. doi:10.1111/jcmm.13866
Jia, Y., Yi, L., Li, Q., Liu, T., and Yang, S. (2021). LncRNA MALAT1 Aggravates Oxygen-Glucose Deprivation/reoxygenation-Induced Neuronal Endoplasmic Reticulum Stress and Apoptosis via the miR-195a-5p/HMGA1 axis. Biol. Res. 54 (1), 8. doi:10.1186/s40659-021-00331-9
Jing, H., Liu, L., Jia, Y., Yao, H., and Ma, F. (2019). Overexpression of the Long Non-coding RNA Oprm1 Alleviates Apoptosis from Cerebral Ischemia-Reperfusion Injury through the Oprm1/miR-155/GATA3 axis. Artif. Cell Nanomed Biotechnol 47 (1), 2431–2439. doi:10.1080/21691401.2019.1626408
Kabekkodu, S. P., Shukla, V., Varghese, V. K., D' Souza, J., and Satyamoorthy, K. (2018). Clustered miRNAs and Their Role in Biological Functions and Diseases. Biol. Rev. Camb Philos. Soc. 93 (4), 1955–1986. doi:10.1111/brv.12428
Kapranov, P., Cheng, J., Dike, S., Nix, D. A., Duttagupta, R., Willingham, A. T., et al. (2007). RNA Maps Reveal New RNA Classes and a Possible Function for Pervasive Transcription. Science 316 (5830), 1484–1488. doi:10.1126/science.1138341
Kuriakose, D., and Xiao, Z. (2020). Pathophysiology and Treatment of Stroke: Present Status and Future Perspectives. Int. J. Mol. Sci. 21 (20), 7609. doi:10.3390/ijms21207609
Lin, L., Wang, X., and Yu, Z. (2016). Ischemia-reperfusion Injury in the Brain: Mechanisms and Potential Therapeutic Strategies. Biochem. Pharmacol. (Los Angel) 5 (4), 213. doi:10.4172/2167-0501.1000213
Li, H., Gao, A., Feng, D., Wang, Y., Zhang, L., Cui, Y., et al. (2014). Evaluation of the Protective Potential of Brain Microvascular Endothelial Cell Autophagy on Blood-Brain Barrier Integrity during Experimental Cerebral Ischemia-Reperfusion Injury. Transl Stroke Res. 5 (5), 618–626. doi:10.1007/s12975-014-0354-x
Li, H., Tang, C., and Wang, D. (2020). LncRNA H19 Promotes Inflammatory Response Induced by Cerebral Ischemia-Reperfusion Injury through Regulating the miR-138-5p-P65 axis. Biochem. Cel Biol 98 (4), 525–536. doi:10.1139/bcb-2019-0281
Li, L., Wang, M., Mei, Z., Cao, W., Yang, Y., Wang, Y., et al. (2017a). lncRNAs HIF1A-AS2 Facilitates the Up-Regulation of HIF-1α by Sponging to miR-153-3p, Whereby Promoting Angiogenesis in HUVECs in Hypoxia. Biomed. Pharmacother. 96, 165–172. doi:10.1016/j.biopha.2017.09.113
Li, Z., Li, J., and Tang, N. (2017b). Long Noncoding RNA Malat1 Is a Potent Autophagy Inducer Protecting Brain Microvascular Endothelial Cells against Oxygen-Glucose Deprivation/reoxygenation-Induced Injury by Sponging miR-26b and Upregulating ULK2 Expression. Neuroscience 354, 1–10. doi:10.1016/j.neuroscience.2017.04.017
Liang, J., Wang, Q., Li, J. Q., Guo, T., and Yu, D. (2020). Long Non-coding RNA MEG3 Promotes Cerebral Ischemia-Reperfusion Injury through Increasing Pyroptosis by Targeting miR-485/AIM2 axis. Exp. Neurol. 325, 113139. doi:10.1016/j.expneurol.2019.113139
Liu, B., Cao, W., and Xue, J. (2019). LncRNA ANRIL Protects against Oxygen and Glucose Deprivation (OGD)-induced Injury in PC-12 Cells: Potential Role in Ischaemic Stroke. Artif. Cell Nanomed Biotechnol 47 (1), 1384–1395. doi:10.1080/21691401.2019.1596944
Long, F. Q., Su, Q. J., Zhou, J. X., Wang, D. S., Li, P. X., Zeng, C. S., et al. (2018). LncRNA SNHG12 Ameliorates Brain Microvascular Endothelial Cell Injury by Targeting miR-199a. Neural Regen. Res. 13 (11), 1919–1926. doi:10.4103/1673-5374.238717
Lu, T. X., and Rothenberg, M. E. (2018). MicroRNA. J. Allergy Clin. Immunol. 141 (4), 1202–1207. doi:10.1016/j.jaci.2017.08.034
Lu, Y., Han, Y., He, J., Zhou, B., Fang, P., and Li, X. (2020). LncRNA FOXD3-AS1 Knockdown Protects against Cerebral Ischemia/reperfusion Injury via miR-765/BCL2L13 axis. Biomed. Pharmacother. 132, 110778. doi:10.1016/j.biopha.2020.110778
Luo, H. C., Yi, T. Z., Huang, F. G., Wei, Y., Luo, X. P., and Luo, Q. S. (2020). Role of Long Noncoding RNA MEG3/miR-378/GRB2 axis in Neuronal Autophagy and Neurological Functional Impairment in Ischemic Stroke. J. Biol. Chem. 295 (41), 14125–14139. doi:10.1074/jbc.RA119.010946
Ma, L., Bajic, V. B., and Zhang, Z. (2013). On the Classification of Long Non-coding RNAs. RNA Biol. 10 (6), 925–933. doi:10.4161/rna.24604
Maglinger, B., Sands, M., Frank, J. A., McLouth, C. J., Trout, A. L., Roberts, J. M., et al. (2021). Intracranial VCAM1 at Time of Mechanical Thrombectomy Predicts Ischemic Stroke Severity. J. Neuroinflammation 18 (1), 109. doi:10.1186/s12974-021-02157-4
McDonel, P., and Guttman, M. (2019). Approaches for Understanding the Mechanisms of Long Noncoding RNA Regulation of Gene Expression. Cold Spring Harb Perspect. Biol. 11 (12), a032151. doi:10.1101/cshperspect.a032151
Muoio, V., Persson, P. B., and Sendeski, M. M. (2014). The Neurovascular Unit - Concept Review. Acta Physiol. (Oxf) 210 (4), 790–798. doi:10.1111/apha.12250
Nair, L., Chung, H., and Basu, U. (2020). Regulation of Long Non-coding RNAs and Genome Dynamics by the RNA Surveillance Machinery. Nat. Rev. Mol. Cel Biol 21 (3), 123–136. doi:10.1038/s41580-019-0209-0
Peng, W. X., Koirala, P., and Mo, Y. Y. (2017). LncRNA-mediated Regulation of Cell Signaling in Cancer. Oncogene 36 (41), 5661–5667. doi:10.1038/onc.2017.184
Qi, X., Shao, M., Sun, H., Shen, Y., Meng, D., and Huo, W. (2017). Long Non-coding RNA SNHG14 Promotes Microglia Activation by Regulating miR-145-5p/PLA2G4A in Cerebral Infarction. Neuroscience 348, 98–106. doi:10.1016/j.neuroscience.2017.02.002
Qin, C., Zhou, L. Q., Ma, X. T., Hu, Z. W., Yang, S., Chen, M., et al. (2019). Dual Functions of Microglia in Ischemic Stroke. Neurosci. Bull. 35 (5), 921–933. doi:10.1007/s12264-019-00388-3
Ren, B., Song, Z., Chen, L., Niu, X., and Feng, Q. (2021). Long Non-coding RNA UCA1 Correlates with Elevated Disease Severity, Th17 Cell Proportion, Inflammatory Cytokines, and Worse Prognosis in Acute Ischemic Stroke Patients. J. Clin. Lab. Anal. 35, e23697. doi:10.1002/jcla.23697
Ren, Y., Gao, X. P., Liang, H., Zhang, H., and Hu, C. Y. (2020). LncRNA KCNQ1OT1 Contributes to Oxygen-Glucose-Deprivation/reoxygenation-Induced Injury via Sponging miR-9 in Cultured Neurons to Regulate MMP8. Exp. Mol. Pathol. 112, 104356. doi:10.1016/j.yexmp.2019.104356
Rezaei, M., Mokhtari, M. J., Bayat, M., Safari, A., Dianatpuor, M., Tabrizi, R., et al. (2021). Long Non-coding RNA H19 Expression and Functional Polymorphism Rs217727 Are Linked to Increased Ischemic Stroke Risk. BMC Neurol. 21 (1), 54. doi:10.1186/s12883-021-02081-3
Rupaimoole, R., and Slack, F. J. (2017). MicroRNA Therapeutics: towards a new era for the Management of Cancer and Other Diseases. Nat. Rev. Drug Discov. 16 (3), 203–222. doi:10.1038/nrd.2016.246
Salmena, L., Poliseno, L., Tay, Y., Kats, L., and Pandolfi, P. P. (2011). A ceRNA Hypothesis: the Rosetta Stone of a Hidden RNA Language. Cell 146 (3), 353–358. doi:10.1016/j.cell.2011.07.014
Sanchez-Mejias, A., and Tay, Y. (2015). Competing Endogenous RNA Networks: Tying the Essential Knots for Cancer Biology and Therapeutics. J. Hematol. Oncol. 8, 30. doi:10.1186/s13045-015-0129-1
Schmitz, S. U., Grote, P., and Herrmann, B. G. (2016). Mechanisms of Long Noncoding RNA Function in Development and Disease. Cell Mol Life Sci 73 (13), 2491–2509. doi:10.1007/s00018-016-2174-5
Shan, W., Chen, W., Zhao, X., Pei, A., Chen, M., Yu, Y., et al. (2020). Long Noncoding RNA TUG1 Contributes to Cerebral Ischaemia/reperfusion Injury by Sponging Mir-145 to Up-Regulate AQP4 Expression. J. Cel Mol Med 24 (1), 250–259. doi:10.1111/jcmm.14712
Song, A., Yang, Y., He, H., Sun, J., Chang, Q., and Xue, Q. (2021). Inhibition of Long Non-coding RNA KCNQ1OT1 Attenuates Neuroinflammation and Neuronal Apoptosis through Regulating NLRP3 Expression via Sponging miR-30e-3p. J. Inflamm. Res. 14, 1731–1742. doi:10.2147/JIR.S291274
Sun, X. L., Wang, Z. L., Wu, Q., Jin, S. Q., Yao, J., and Cheng, H. (2019). LncRNA RMST Activates TAK1-Mediated NF-Κb Signaling and Promotes Activation of Microglial Cells via Competitively Binding with hnRNPK. IUBMB Life 71 (11), 1785–1793. doi:10.1002/iub.2125
Teng, H., Li, M., Qian, L., Yang, H., and Pang, M. (2020). Long Non-coding RNA SNHG16 Inhibits the Oxygen-Glucose Deprivation and Reoxygenation-Induced Apoptosis in Human Brain Microvascular Endothelial Cells by Regulating miR-15a-5p/bcl-2. Mol. Med. Rep. 22 (4), 2685–2694. doi:10.3892/mmr.2020.11385
Tian, J., Liu, Y., Wang, Z., Zhang, S., Yang, Y., Zhu, Y., et al. (2021). LncRNA Snhg8 Attenuates Microglial Inflammation Response and Blood-Brain Barrier Damage in Ischemic Stroke through Regulating miR-425-5p Mediated SIRT1/NF-Κb Signaling. J. Biochem. Mol. Toxicol. 35, e22724. doi:10.1002/jbt.22724
Trendelenburg, G., and Dirnagl, U. (2005). Neuroprotective Role of Astrocytes in Cerebral Ischemia: Focus on Ischemic Preconditioning. Glia 50 (4), 307–320. doi:10.1002/glia.20204
Wang, H., Zheng, X., Jin, J., Zheng, L., Guan, T., Huo, Y., et al. (2020a). LncRNA MALAT1 Silencing Protects against Cerebral Ischemia-Reperfusion Injury through miR-145 to Regulate AQP4. J. Biomed. Sci. 27 (1), 40. doi:10.1186/s12929-020-00635-0
Wang, H. J., Tang, X. L., Huang, G., Li, Y. B., Pan, R. H., Zhan, J., et al. (2020b). Long Non-coding KCNQ1OT1 Promotes Oxygen-Glucose-Deprivation/Reoxygenation-Induced Neurons Injury through Regulating MIR-153-3p/FOXO3 Axis. J. Stroke Cerebrovasc. Dis. 29 (10), 105126. doi:10.1016/j.jstrokecerebrovasdis.2020.105126
Wang, M., Chen, W., Geng, Y., Xu, C., Tao, X., and Zhang, Y. (2020c). Long Non-coding RNA MEG3 Promotes Apoptosis of Vascular Cells and Is Associated with Poor Prognosis in Ischemic Stroke. J. Atheroscler. Thromb. 27 (7), 718–726. doi:10.5551/jat.50674
Wang, T., Tian, J., and Jin, Y. (2021). VCAM1 Expression in the Myocardium Is Associated with the Risk of Heart Failure and Immune Cell Infiltration in Myocardium. Sci. Rep. 11 (1), 19488. doi:10.1038/s41598-021-98998-3
Wang, Y., Li, G., Zhao, L., and Lv, J. (2018a). Long Noncoding RNA HOTTIP Alleviates Oxygen-Glucose Deprivation-Induced Neuronal Injury via Modulating miR-143/hexokinase 2 Pathway. J. Cel Biochem 119 (12), 10107–10117. doi:10.1002/jcb.27348
Wang, Z., Wang, R., Wang, K., and Liu, X. (2018b). Upregulated Long Noncoding RNA Snhg1 Promotes the Angiogenesis of Brain Microvascular Endothelial Cells after Oxygen-Glucose Deprivation Treatment by Targeting miR-199a. Can. J. Physiol. Pharmacol. 96 (9), 909–915. doi:10.1139/cjpp-2018-0107
Wei, R., Zhang, L., Hu, W., Wu, J., and Zhang, W. (2019). Long Non-coding RNA AK038897 Aggravates Cerebral Ischemia/reperfusion Injury via Acting as a ceRNA for miR-26a-5p to Target DAPK1. Exp. Neurol. 314, 100–110. doi:10.1016/j.expneurol.2019.01.009
Wolska, M., Jarosz-Popek, J., Junger, E., Wicik, Z., Porshoor, T., Sharif, L., et al. (2020). Long Non-coding RNAs as Promising Therapeutic Approach in Ischemic Stroke: a Comprehensive Review. Mol. Neurobiol. 58 (4), 1664–1682. doi:10.1007/s12035-020-02206-8
Wu, R., Yun, Q., Zhang, J., and Bao, J. (2021). Long Non-coding RNA GAS5 Retards Neural Functional Recovery in Cerebral Ischemic Stroke through Modulation of the microRNA-455-5p/PTEN axis. Brain Res. Bull. 167, 80–88. doi:10.1016/j.brainresbull.2020.12.002
Xiang, Y., Zhang, Y., Xia, Y., Zhao, H., Liu, A., and Chen, Y. (2020). LncRNA MEG3 Targeting miR-424-5p via MAPK Signaling Pathway Mediates Neuronal Apoptosis in Ischemic Stroke. Aging (Albany NY) 12 (4), 3156–3174. doi:10.18632/aging.102790
Xiao, Z., Qiu, Y., Lin, Y., Medina, R., Zhuang, S., Rosenblum, J. S., et al. (2019). Blocking lncRNA H19-miR-19a-Id2 axis Attenuates Hypoxia/ischemia Induced Neuronal Injury. Aging (Albany NY) 11 (11), 3585–3600. doi:10.18632/aging.101999
Xu, J., Wang, C., Meng, F., and Xu, P. (2021). Long Non-coding RNA H19 Inhibition Ameliorates Oxygen-Glucose Deprivation-Induced Cell Apoptosis and Inflammatory Cytokine Expression by Regulating the microRNA-29b/SIRT1/PGC-1α axis. Mol. Med. Rep. 23 (2), 1. doi:10.3892/mmr.2020.11770
Xu, Z. M., Huang, F., and Huang, W. Q. (2018). Angiogenic lncRNAs: A Potential Therapeutic Target for Ischaemic Heart Disease. Life Sci. 211, 157–171. doi:10.1016/j.lfs.2018.09.022
Yan, G., Zhao, H., and Hong, X. (2020a). LncRNA MACC1-AS1 Attenuates Microvascular Endothelial Cell Injury and Promotes Angiogenesis under Hypoxic Conditions via Modulating miR-6867-5p/TWIST1 in Human Brain Microvascular Endothelial Cells. Ann. Transl Med. 8 (14), 876. doi:10.21037/atm-20-4915
Yan, H., Rao, J., Yuan, J., Gao, L., Huang, W., Zhao, L., et al. (2017). Long Non-coding RNA MEG3 Functions as a Competing Endogenous RNA to Regulate Ischemic Neuronal Death by Targeting miR-21/PDCD4 Signaling Pathway. Cell Death Dis 8 (12), 3211. doi:10.1038/s41419-017-0047-y
Yan, Y., Chen, L., Zhou, J., and Xie, L. (2020b). SNHG12 Inhibits Oxygen-glucose D-eprivation-induced N-euronal A-poptosis via the miR-181a-5p/NEGR1 axis. Mol. Med. Rep. 22 (5), 3886–3894. doi:10.3892/mmr.2020.11459
Yang, H., Xi, X., Zhao, B., Su, Z., and Wang, Z. (2018). KLF4 Protects Brain Microvascular Endothelial Cells from Ischemic Stroke Induced Apoptosis by Transcriptionally Activating MALAT1. Biochem. Biophys. Res. Commun. 495 (3), 2376–2382. doi:10.1016/j.bbrc.2017.11.205
Yao, P., Li, Y. L., Chen, Y., Shen, W., Wu, K. Y., and Xu, W. H. (2020). Overexpression of Long Non-coding RNA Rian Attenuates Cell Apoptosis from Cerebral Ischemia-Reperfusion Injury via Rian/miR-144-3p/GATA3 Signaling. Gene 737, 144411. doi:10.1016/j.gene.2020.144411
Yi, M., Li, Y., Wang, D., Zhang, Q., Yang, L., and Yang, C. (2020). KCNQ1OT1 Exacerbates Ischemia-Reperfusion Injury through Targeted Inhibition of miR-140-3P. Inflammation 43 (5), 1832–1845. doi:10.1007/s10753-020-01257-2
Yin, D., Xu, F., Lu, M., and Li, X. (2021). Long Non-coding RNA RMST Promotes Oxygen-Glucose Deprivation-Induced Injury in Brain Microvascular Endothelial Cells by Regulating miR-204-5p/VCAM1 axis. Life Sci. 284, 119244. doi:10.1016/j.lfs.2021.119244
Yu, S., Yu, M., He, X., Wen, L., Bu, Z., and Feng, J. (2019). KCNQ1OT1 Promotes Autophagy by Regulating miR-200a/FOXO3/ATG7 Pathway in Cerebral Ischemic Stroke. Aging Cell 18 (3), e12940. doi:10.1111/acel.12940
Zhang, G., Wang, Q., Su, D., and Xie, Y. (2020a). Long Non-coding RNAMALAT1 Knockdown Alleviates Cerebral Ischemia/Reperfusion Injury of Rats through Regulating the miR-375/PDE4D Axis. Front. Neurol. 11, 578765. doi:10.3389/fneur.2020.578765
Zhang, M., Tang, M., Wu, Q., Wang, Z., Chen, Z., Ding, H., et al. (2020b). LncRNA DANCR Attenuates Brain Microvascular Endothelial Cell Damage Induced by Oxygen-Glucose Deprivation through Regulating of miR-33a-5p/XBP1s. Aging (Albany NY) 12 (2), 1778–1791. doi:10.18632/aging.102712
Zhang, T., Wang, H., Li, Q., Fu, J., Huang, J., and Zhao, Y. (2018). MALAT1 Activates the P53 Signaling Pathway by Regulating MDM2 to Promote Ischemic Stroke. Cel Physiol Biochem 50 (6), 2216–2228. doi:10.1159/000495083
Zhang, X., Hamblin, M. H., and Yin, K. J. (2017a). The Long Noncoding RNA Malat1: Its Physiological and Pathophysiological Functions. RNA Biol. 14 (12), 1705–1714. doi:10.1080/15476286.2017.1358347
Zhang, X., Liu, Z., Shu, Q., Yuan, S., Xing, Z., and Song, J. (2019a). LncRNA SNHG6 Functions as a ceRNA to Regulate Neuronal Cell Apoptosis by Modulating miR-181c-5p/BIM Signalling in Ischaemic Stroke. J. Cel Mol Med 23 (9), 6120–6130. doi:10.1111/jcmm.14480
Zhang, X., Tang, X., Liu, K., Hamblin, M. H., and Yin, K. J. (2017b). Long Noncoding RNA Malat1 Regulates Cerebrovascular Pathologies in Ischemic Stroke. J. Neurosci. 37 (7), 1797–1806. doi:10.1523/JNEUROSCI.3389-16.2017
Zhang, X. Q., Song, L. H., Feng, S. J., and Dai, X. M. (2019b). LncRNA FGD5-AS1 Acts as a Competing Endogenous RNA for miRNA-223 to Lessen Oxygen-Glucose Deprivation and Simulated Reperfusion (OGD/R)-induced Neurons Injury. Folia Neuropathol. 57 (4), 357–365. doi:10.5114/fn.2019.91194
Zhang, Y., and Niu, C. (2020). The Correlation of Long Non-coding RNA Intersectin 1-2 with Disease Risk, Disease Severity, Inflammation, and Prognosis of Acute Ischemic Stroke. J. Clin. Lab. Anal. 34 (2), e23053. doi:10.1002/jcla.23053
Zhao, L., Zhang, M., Yan, F., and Cong, Y. (2021). Knockdown of RMST Impedes Neuronal Apoptosis and Oxidative Stress in OGD/R-Induced Ischemic Stroke via Depending on the miR-377/SEMA3A Signal Network. Neurochem. Res. 46 (3), 584–594. doi:10.1007/s11064-020-03194-w
Zhao, M., Wang, J., Xi, X., Tan, N., and Zhang, L. (2018). SNHG12 Promotes Angiogenesis Following Ischemic Stroke via Regulating miR-150/VEGF Pathway. Neuroscience 390, 231–240. doi:10.1016/j.neuroscience.2018.08.029
Zhao, Z., Sun, W., Guo, Z., Zhang, J., Yu, H., and Liu, B. (2020). Mechanisms of lncRNA/microRNA Interactions in Angiogenesis. Life Sci. 254, 116900. doi:10.1016/j.lfs.2019.116900
Zhong, W., Li, Y. C., Huang, Q. Y., and Tang, X. Q. (2020). lncRNA ANRIL Ameliorates Oxygen and Glucose Deprivation (OGD) Induced Injury in Neuron Cells via miR-199a-5p/CAV-1 Axis. Neurochem. Res. 45 (4), 772–782. doi:10.1007/s11064-019-02951-w
Zhong, Y., Yu, C., and Qin, W. (2019). LncRNA SNHG14 Promotes Inflammatory Response Induced by Cerebral Ischemia/reperfusion Injury through Regulating miR-136-5p/ROCK1. Cancer Gene Ther. 26 (7-8), 234–247. doi:10.1038/s41417-018-0067-5
Zhou, T., Wang, S., Lu, K., and Yin, C. (2020). Long Non-coding RNA SNHG7 Alleviates Oxygen and Glucose Deprivation/Reoxygenation-Induced Neuronal Injury by Modulating miR-9/SIRT1 Axis in PC12 Cells: Potential Role in Ischemic Stroke. Neuropsychiatr. Dis. Treat. 16, 2837–2848. doi:10.2147/NDT.S273421
Zhu, M., Li, N., Luo, P., Jing, W., Wen, X., Liang, C., et al. (2018). Peripheral Blood Leukocyte Expression of lncRNA MIAT and its Diagnostic and Prognostic Value in Ischemic Stroke. J. Stroke Cerebrovasc. Dis. 27 (2), 326–337. doi:10.1016/j.jstrokecerebrovasdis.2017.09.009
Zhu, T., Wang, L., Tian, F., Zhao, X., Pu, X. P., Sun, G. B., et al. (2020). Anti-ischemia/reperfusion Injury Effects of Notoginsenoside R1 on Small Molecule Metabolism in Rat Brain after Ischemic Stroke as Visualized by MALDI-MS Imaging. Biomed. Pharmacother. 129, 110470. doi:10.1016/j.biopha.2020.110470
Zhu, T., Xie, W. J., Wang, L., Jin, X. B., Meng, X. B., Sun, G. B., et al. (2021). Notoginsenoside R1 Activates the NAMPT-NAD+-SIRT1 cascade to Promote Postischemic Angiogenesis by Modulating Notch Signaling. Biomed. Pharmacother. 140, 111693. doi:10.1016/j.biopha.2021.111693
Zimta, A. A., Tigu, A. B., Braicu, C., Stefan, C., Ionescu, C., and Berindan-Neagoe, I. (2020). An Emerging Class of Long Non-coding RNA with Oncogenic Role Arises from the snoRNA Host Genes. Front. Oncol. 10, 389. doi:10.3389/fonc.2020.00389
3ʹ-UTR 3ʹ-untranslated region
AIM2 absent in melanoma 2
ANRIL antisense non-coding RNA in the INK4 locus
APAF1 apoptotic peptidase activating factor 1
AQP4 aquaporin 4
ATG7 autophagy related protein 7
BBB blood-brain-barrier
Bcl2 B-cell lymphoma-2
BMEC brain microvascular endothelial cell
BIM Bcl2 interacting mediator of cell death
CAAP1 conserved anti-apoptotic protein 1
CAV-1 caveolin-1
ceRNA competing endogenous RNA
CHRF cardiac hypertrophy-related factor
CTRP3 C1q/TNF-related protein 3
CXCL13 C-X-C motif chemokine ligand 13
DANCR differentiation antagonizing non-protein coding RNA
DAPK1 death-associated protein kinase 1
ERK extracellular signal-regulated kinase
FGD5-AS1 FGD5 antisense RNA 1
FOXD3-AS1 FOXD3 antisense RNA 1
FOXO3 forkhead box O3
GAS5 growth arrest-specific 5
GATA3 GATA binding protein 3
GRB2, growth factor receptor-bound protein 2
HIF-1 hypoxia-inducible factor 1
HMGA1 high mobility group AT-hook1
hnRNPK heterogeneous nuclear ribonucleoprotein K
HOTTIP HOXA transcript at the distal tip
HUVEC human umbilical vein endothelial cell
I/R ischemia-reperfusion
Id2 inhibitor of DNA binding 2
IGF insulin-like growth factor
IGF1R IGF-1 receptor
IL interleukin
IS ischemic stroke
KCNQ1OT1 potassium voltage-gated channel subfamily Q member 1 opposite strand 1
LncRNA long non-coding RNA
MACC1 metastasis-associated colon cancer 1
MALAT1 metastasis associated lung adenocarcinoma transcript 1
MAPK mitogen-activated protein kinase
MCAO/R middle cerebral artery occlusion/reperfusion
Mcl-1 myeloid cell leukemia-1
MEG3 maternally expressed gene 3
MEK mitogen-activated protein kinase
miRNA microRNA
MMP matrix metalloproteinase
MRE microRNA response element
mTOR mechanistic target of rapamycin
N2a neuro-2a
NEGR1 neuronal growth regulator 1
NO nitric oxide
NF-κB nuclear factor-κB
NR3C2 nuclear receptor subfamily 3 group C member 2
NVU neurovascular unit
OGD/R oxygen-glucose deprivation and reoxygenation
OIP5-AS1 Opa-interacting protein 5 antisense transcript 1
Oprm1 opioid receptor mu 1
PDCD4 programmed cell death 4
PDE4D phosphodiesterase 4D
PGC-1α peroxisome proliferator-activated receptor-gamma coactivator 1 alpha
PLA2G4A phospholipase A2 group IVA
PTEN phosphatase and tensin homolog deleted on chromosome ten
PUMA p53 upregulated modulator of apoptosis
Rian RNA imprinted and accumulated in nucleus
RMST rhabdomyosarcoma 2-associated transcript
SD Sprague Dawley
SEMA3A semaphorin 3A
SIRT1 sirtuin1
SNHGs small nucleolar RNA host genes
SOX6 sex-determining region Y box six
TALNEC2 tumor-associated lncRNA expressed on chromosome two
TNF-α tumor necrosis factor α
TP53INP1 tumor protein p53 inducible nuclear protein one
TUG1 taurine upregulated gene one
ULK2 Unc-51 like autophagy activating kinase two
VCAM1 vascular cell adhesion molecule one
VEGF vascular endothelial growth factor
XBP1s X-box binding protein 1
Keywords: long non-coding RNA, competing endogenous RNA network, ischemic stroke, ischemia-reperfusion injury, therapeutic target
Citation: Zhang S, Zhu T, Li Q, Sun G and Sun X (2021) Long Non-Coding RNA-Mediated Competing Endogenous RNA Networks in Ischemic Stroke: Molecular Mechanisms, Therapeutic Implications, and Challenges. Front. Pharmacol. 12:765075. doi: 10.3389/fphar.2021.765075
Received: 26 August 2021; Accepted: 20 October 2021;
Published: 17 November 2021.
Edited by:
Francisco Lopez-Munoz, Camilo José Cela University, SpainReviewed by:
Ping Sun, University of Pittsburgh, United StatesCopyright © 2021 Zhang, Zhu, Li, Sun and Sun. This is an open-access article distributed under the terms of the Creative Commons Attribution License (CC BY). The use, distribution or reproduction in other forums is permitted, provided the original author(s) and the copyright owner(s) are credited and that the original publication in this journal is cited, in accordance with accepted academic practice. No use, distribution or reproduction is permitted which does not comply with these terms.
*Correspondence: Guibo Sun, c3VuZ3VpYm9AMTI2LmNvbQ==; Xiaobo Sun, c3VuX3hpYW9ibzE2M0AxNjMuY29t
Disclaimer: All claims expressed in this article are solely those of the authors and do not necessarily represent those of their affiliated organizations, or those of the publisher, the editors and the reviewers. Any product that may be evaluated in this article or claim that may be made by its manufacturer is not guaranteed or endorsed by the publisher.
Research integrity at Frontiers
Learn more about the work of our research integrity team to safeguard the quality of each article we publish.