- 1Pharm-Bio Technology and Traditional Medicine Center, Mbarara University of Science and Technology, Mbarara, Uganda
- 2Department of Pharmacy, Faculty of Pharmaceutical Sciences and Public Health, Official University of Bukavu, Bukavu, Democratic Republic of Congo
- 3Department of Pharmacy, Faculty of Medicine, Mbarara University of Science and Technology, Mbarara, Uganda
- 4Department of Pharmacology, School of Medicine and Pharmacy, University of Rwanda, Huye, Rwanda
- 5Department of Innovation, Technology Transfer and Commercialization, National Institute for Medical Research, Dar es Salaam, Tanzania
- 6Chemistry Section, Department of Applied Studies, Institute of Technology, Malawi University of Science and Technology, Limbe, Malawi
- 7Department of Pharmacognosy, Faculty of Pharmacy, Obafemi Awolowo University, Ile-Ife, Nigeria
- 8Department of Pharmacology and Therapeutics, Faculty of Medicine, Mbarara University of Science and Technology, Mbarara, Uganda
Several studies have been conducted and published on medicinal plants used to manage Diabetes Mellitus worldwide. It is of great interest to review available studies from a country or a region to resort to similarities/discrepancies and data quality. Here, we examined data related to ethnopharmacology and bioactivity of antidiabetic plants used in the Democratic Republic of Congo. Data were extracted from Google Scholar, Medline/PubMed, Scopus, ScienceDirect, the Wiley Online Library, Web of Science, and other documents focusing on ethnopharmacology, pharmacology, and phytochemistry antidiabetic plants used in the Democratic Republic of Congo from 2005 to September 2021. The Kew Botanic Royal Garden and Plants of the World Online web databases were consulted to verify the taxonomic information. CAMARADES checklist was used to assess the quality of animal studies and Jadad scores for clinical trials. In total, 213 plant species belonging to 72 botanical families were reported. Only one plant, Droogmansia munamensis, is typically native to the DRC flora; 117 species are growing in the DRC and neighboring countries; 31 species are either introduced from other regions, and 64 are not specified. Alongside the treatment of Diabetes, about 78.13% of plants have multiple therapeutic uses, depending on the study sites. Experimental studies explored the antidiabetic activity of 133 plants, mainly in mice, rats, guinea pigs, and rabbits. Several chemical classes of antidiabetic compounds isolated from 67 plant species have been documented. Rare phase II clinical trials have been conducted. Critical issues included poor quality methodological protocols, author name incorrectly written (16.16%) or absent (14.25%) or confused with a synonym (4.69%), family name revised (17.26%) or missing (1.10%), voucher number not available 336(92.05%), ecological information not reported (49.59%). Most plant species have been identified and authenticated (89.32%). Hundreds of plants are used to treat Diabetes by traditional healers in DRC. However, most plants are not exclusively native to the local flora and have multiple therapeutic uses. The analysis showed the scarcity or absence of high-quality, in-depth pharmacological studies. There is a need to conduct further studies of locally specific species to fill the gap before their introduction into the national pharmacopeia.
1 Introduction
Most African traditional healers who detain ancestral heritages are illiterate, and their knowledge transmitted verbally from generation to generation is at risk of disappearing. To minimize such risk, the World Health Organization (WHO) recommends scientists carry out ethnopharmacological and experimental studies to record folk knowledge, create databases, and validate scientifically traditional claims from the perspective of developing improved medications (WHO, 2013). WHO estimates that 80% of people rely on conventional medicine to meet primary health care needs, and most of them use remedies from plants (Surya et al., 2014). Ethnopharmacological surveys help gather holistic knowledge and practices of conventional healthcare systems. Experimental investigations evaluate efficacy and safety by developing suitable standardized pharmaceutical dosage forms that can complement, if not replace, current modern medicines. Medicinal plants used as complementary/alternative medicines (CAM) to manage various diseases provide a real opportunity in developed and developing societies. In this sense, herbal medications appear to offer readily available means of managing metabolic disorders by minimizing the risk of side effects and sometimes potentiating the treatment outcomes of modern drugs (Etxeberria et al., 2012). Medicinal plants are also used as food and contain several healthy dietary compounds. For example, some flavonoids interfere with metabolic events and play a crucial role in preventing and managing metabolic disorders through different pathways (Farzaei et al., 2019).
One of the most explored diseases is diabetes mellitus (DM). Over 800 plant species showing hypoglycemic activities can be essential sources for discovering and developing new types of antidiabetic molecules (Patel et al., 2012). The magnitude justifies this craze that Diabetes is gaining more and more globally, making it a severe public health problem. Not long ago, the disease was associated with industrialization. DM is no longer a disease of high-income countries but a global health pandemic. In 2013, according to the International Diabetes Federation, the global population of adults with both type-1(DMT1) and type-2(DMT2) was projected to increase from 382 million to 592 million by 2035, with DMT2 accounting for 90–95% of cases (Glezeva et al., 2017). In Africa, the number was expected to double from 14 million in 2015 to 34 million by 2040. With its continuous and rapid increase in its prevalence worldwide, it should be one of the leading causes of morbidity and mortality in the coming years (Glezeva et al., 2017).
Recent data show about 1.7 million people suffer from DM in the Democratic Republic of Congo (DRC), ranking fourth in the top ten countries by diabetes cases in Africa (Zhivov et al., 2015; Kasangana et al., 2018). Like other African countries, and not withdrawing modern medicines, 80% of people rely on traditional medicine to meet primary health care needs (Mahomoodally, 2013). Ethnopharmacological and pharmacological studies have been conducted globally; however, the related data are disparate and uncontrolled. A preliminary review reported vernacular names, parts used, and the formulation of 70 medicinal plants used to treat DM in DRC. A few phytoconstituents and antidiabetic mechanisms are also mentioned (Jacques et al., 2015).
This review aimed to describe what is known hitherto about ethnopharmacological, pharmacological, and clinical studies embracing medicinal plants used to manage DM in the traditional medicine of the DRC, to highlight which plants are native or introduced, how they are formulated and used, what valid experimental studies have been conducted in preclinical and clinical phases. A critical analysis is made to assess the quality of studies carried inside DRC and resort similarities/discrepancies with studies conducted outside.
2 Methods
2.1 Literature Search Process
The review was an Internet search on Google Scholar, Medline, PubMed, Scopus, ScienceDirect, the Wiley Online Library, Web of Science, and other documents focusing on ethnopharmacology, pharmacology phytochemistry of antidiabetic plants used in the Democratic Republic of Congo from 2005 to September 2021. The review was conducted following Preferred Regulatory Items for Systematic Reviews and Meta-Analysis (PRISMA) guidelines 2009. A total of 34 studies were included. Ethnopharmacological/Ethnobotanical/Ethnomedicinal (n = 24), preclinical bioactivity (n = 9); and one clinical trial (n = 1) studies. One paper includes both an in vivo study and an Ethnobotanical survey.
2.2 Quality Critical Assessment
Studies that reported ethnopharmacology, phytochemistry, experimental pharmacology, and related clinical data were assessed for eligibility. The Kew Botanic Royal Garden and Plants of the World Online web databases were consulted to verify the taxonomic information on the species mentioned. All species names were checked at the UOB University herbarium. The quality of animal experiments reported was evaluated by examining the peer-reviewed publication, statement of control of temperature, appropriate animal model, compliance with animal welfare regulations for preclinical experiments, random allocation to treatment or management, blinded assessment of outcome, allocation sample size calculation, statement of potential conflict of interests, concealment, use of co-interventions/co-morbid. We used a CAMARADES checklist to assess the quality. Each task was given a quality score out of a possible total of 10 points. Thus, studies were categorized into low quality for mean score 1–5 and high quality for mean score 6–10 (Hooijmans et al., 2014; Auboire et al., 2018). The quality assessment of clinical trials has been evaluated using the Jadad scale for reporting randomized controlled trials based on randomization, blinding, withdrawals, and dropouts methods (Halpern and Douglas, 2007).
2.3 Statistical Values of Plant Species
Some indexes often express the frequency of quoting for botanical families and plant species. In the present review, the following indexes have been used: Frequency of citation (FC = Number of times a particular species was mentioned/Total number of times that all species were mentioned x 100); Relative Frequency of Citation (RFC = FC/N; 0<RFC<1): index, where FC is the number of informants who mentioned the use of the species and N, is the total number of informants (Tardío and Pardo-De-Santayana, 2008); Use Value (UV = ƩU/n) where U is the number of usable reports for a given plant species cited by each informant and n is the total number of informants interviewed for a given plant (Bano et al., 2014). The Relative Importance Index (RII) of each plant species was calculated based on the normalized number of pharmacological properties attributed to it and the normalized number of body systems (BS) it affects (Bennett and Prance, 2000).
2.4 Study Sites
Figure 3 shows different locations where 24 Ethnopharmacological/Ethnobotanical/Ethnomedicinal studies were conducted on the DRC map. The studies were done in Kisangani (R1) by Katemo et al. (2012), Mpiana et al. (2015); in Beni and Lubero (R2) by (Kasika et al., 2015); in Bukavu (R3) by (Karhagomba et al., 2013; Kasali et al., 2013, 2021; Chiribagula et al., 2020; Manya et al., 2020); in Mbandaka, Bagdolite, and Kungu (R4) by (Mongeke et al., 2018) ; in Lubumbashi, Kafubu, Kasumbalesa, Kipushi, Likasi and Sambwa (R5) by (Muya et al., 2014; Mbayo et al., 2016; Amuri et al., 2017, 2018; Bashige-Chiribagula et al., 2017; Mbuyi et al., 2019; Valentin et al., 2020) (Amuri et al., 2017); in Kinshasa, Kwango and Kongo central (R6) by (Ngbolua et al., 2016a, 2016b, 2019; Latham and Mbuta, 2017; Masunda et al., 2019; Pathy et al., 2021); in non-specified sites (R7) by (Moswa et al., 2005; Manzo, 2012).
3 Results
3.1 Ethnopharmacological Data
Table 1 describes the names, parts, forms used, locations, and some statistical values of plants cited. From 24 reviewed papers, we identified 213 plant species belonging to 72 botanical families.
As shown in Figure 4, the most frequent botanical families were Fabaceae with 44(20.66%) species, Asteraceae 10(4.69%), Phyllanthaceae 9(4.23%), Malvaceae 8(3.76%), Solanaceae 8(3.76%), Euphorbiaceae 7(3.29%), Rubiaceae 7(3.29%), Apocynaceae 6(2.82%), and Lamiaceae 6(2.82%). Most plants were found at the site R5(33.66%) and R6(27.78%). The distribution varied from study to study. Catharanthus roseus was found in almost all locations (6/7 sites) and Allium cepa in 5 zones. The vernacular names were linked or not to ethnic dialects. Swahili is the most reported language 48(12.87%), followed by Kongo 46(12.33%), Luba 36(9.65%), and Bemba 32(8.58%). In most cases, the vernacular name is not specified 47(12.60%) or not reported 8(2.14%). The formulations prepared consisted more often of decoction 173(60.49%), maceration 31(10.84%), and infusion 29(10.14%). The leaf is the most used part 122(39.23%), followed by roots 73(23.47%), and stem bark 43(13.83%).
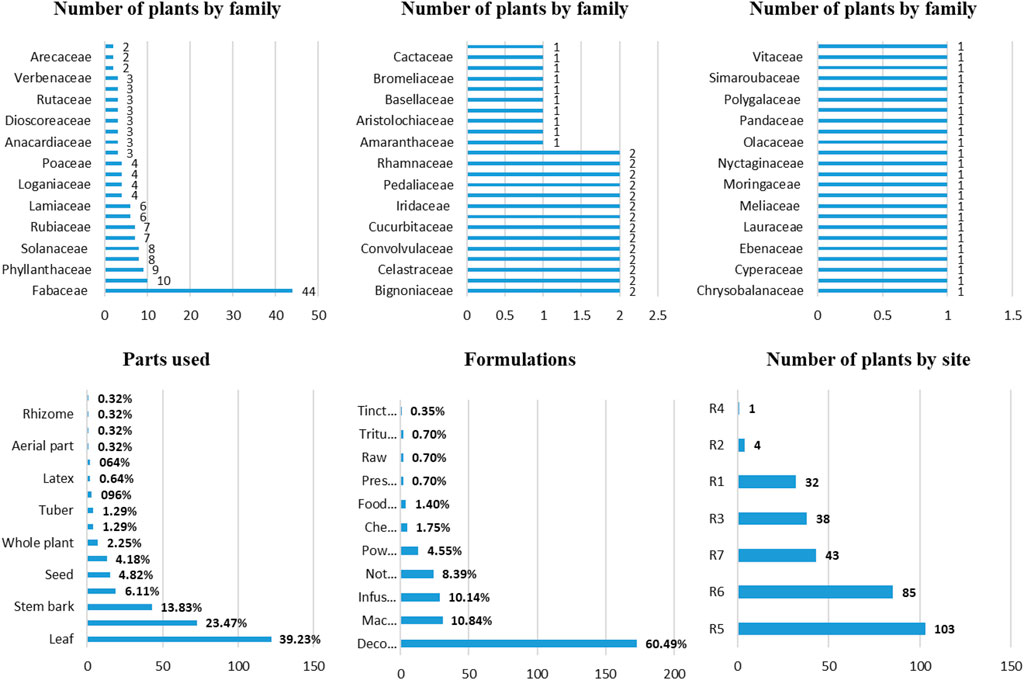
FIGURE 4. Frequencies of antidiabetic plants in DRC by botanical families, parts used, formulations, and sites.
3.2 Pharmacological Investigations Inside Democratic Republic of Congo
3.2.1 Preclinical Pharmacological and Toxicological Investigations Inside Democratic Republic of Congo
Only seven plants presented in Figure 5 were exclusively studied in experimental animals inside DRC; Albizia adianthifolia, Azanza garckeana, Gladiolus klattianus, Panda oleosa, Raphia gentiliana, Rauvolfia caffra, and Vitex madiensis; five studied in DRC. It also reported the only plant species native exclusively from DRC.
3.2.2 Preclinical Toxicological Investigations Inside Democratic Republic of Congo
Many toxicological studies have been carried out in animals (rodents) using plant extracts. Some studies have been undertaken in mice, guinea pigs, and rabbits to explore the acute toxicity of Panda oleosa. Endpoints consisted mainly of mortality, pathophysiological syndromes, and microscopic examination of the pancreas and other vital organs pathological changes. The sub-chronic evaluation focused on assessing biochemical, hematological, and histopathological markers after a relatively long period (14 days and sometimes 90 days). The level of exposure to different organs, including the fetus, liver, kidney, heart, etc., of different doses of plant extracts was also determined. Thus, most plant extracts produce a toxic effect in specific organs or systems at high doses.
3.2.3 Clinical Trials Inside Democratic Republic of Congo
Data from the present study showed the lack of local clinical trials of antidiabetic plants used to manage Diabetes in the DRC. Of seven native herbals, only Raphia gentiliana fruit extract was given to 25 males and 20 females, aged 18–50 years old, with normal blood sugar levels (Mpiana et al., 2013). Thirty persons were submitted to the fruits of R. gentiliana as food (0.14 g/kg), while fifteen were introduced to the glucose solution (0.07 g/kg) (standard). The glycemia was measured by spectrophotometry, and the triangle surface area ratio method was used to calculate the glycemic and load index. The observed values of glycemic index and load were −3.1% and −1.36%. The approach followed by the authors did not comply with any clinical trial requirements, and instead, their behavior went like traditional healers themselves.
3.3 Phytochemical Investigations
Some studies have been undertaken to explore the chemical composition of Panda oleosa, Physalis peruviana, and Vernonia amygdalina.
4 Discussion
4.1 Ethnopharmacological Data
4.1.1 Ethnobotanical Information Reported
The analysis presented in Table 2 showed that the ecological status was reported for 185(50.41%) plants and not for 182(49.59%). On the other hand, plant identification was reported in 326(89.32%) cases and not 39(10.68%).
The errors in plant authors included entirely different authors, spelling mistakes, inappropriate use of the period, improper use of bracket, and incomplete author name.
The origin of plants was specified in 69.95% and not in 30.05% of species. However, 54.93% of plants with known origin were native to Africa, 14.55% species were introduced, and Droogmansia munamensis was the only species exclusively native to DRC flora (“Haut-Katanga”). Concerning the data quality, the author names of plant species were correctly written in 69.59% of cases, not correctly registered in 16.16%, or absent in 14.25%. Furthermore, 17.26% of plants had family names changed, and 81.64% not changed. In few cases (n = 10), the main plant was confused with its synonym. For example, Antidesma metacarpus (A. membranaceum), Annona senegalensis (A. arenaria), Cassia alata (Senna alata), Chenopodium ambrosioides (Dysphania ambrosioides), Citrus x aurantium (C. sinensis), Hibiscus esculentus (Abelmoschus esculentus), Mitragyna stipulosa (Hallea stipulosa), Nauclea latifolia (Sarcocephalus latifolius), Solanum americanum (S. nigrum), and Solanum gilo (S. aethiopicum and S. subsessile). Most species (89.32%) from different sites were identified and authenticated in other herbariums or laboratories of ecology, but only a few (7.95%) had a voucher number published. This situation implicates the responsibilities of publishers and reviewers. Table 2 shows the quality analysis of findings compared to data from Plants of the World Online web (http://powo.science.kew.org) database and http://plantsoftheworldonline.org via the Royal Botanic Garden Kew database.
4.1.2 Ethnopharmacological Data Reported
The country is home to different ethnic groups, making it one of the most diverse countries globally, with more than 200 other ethnic groups speaking an estimated 213 native languages. Sometimes referred to as the Baluba, the Luba people are the largest ethnic group. The community is native to the Kasai, Maniema, and Katanga regions. The Mongo people comprise several smaller constituent groups, including the Mbole, Ekonda, Boyela, Bolia, and Nkutu. The Kongo ethnic group is native to DRC and Angola, speaking Kongo alongside Lingala, Kyanzi, and Kintandu. The Mangbetu ethnicity is concentrated within the Orientale Province (Kisangani). The Zande people reside in the tropical rainforest and the savanna and speak nearly five dialects of the Azande language. The Pygmies are considered to have been some of the earliest peoples to inhabit the Congo River Basin. Their short stature characterizes them, they are mainly hunters and gatherers, and they occupy the rainforest. The plants are distributed within tropical and subtropical ecological regions, flooded grasslands, moist broad-leaf forests, savannas, and mangroves.
Swahili is the most reported language 48(12.87%), followed by Kongo 46(12.33%), Luba 36(9.65%), Bemba 32(8.58%), Tshiluba 29(7.77%), Mashi 26(6.97%), French 21(5.63%), and Lingala 14(3.75%). After le French, which is the official language, there are four regionally distributed national languages, including Ciluba (Tshiluba), Kongo, Lingala, and Swahili, among 213 native languages identified in DRC. Those four languages are used in out-group communication, in lower primary school years (mainly in rural and semi-urban areas), cultural and religious purposes, etc. (Kasanga, 2012).
Fabaceae was the most representative family, consistent with other studies that showed this family is commonly found in tropical rain and dry forests in the Americans and Africa (Burnham and Johnson, 2004). Around 60% of the Congo-basin lies in the DRC, the second-largest contiguous tract of tropical forests globally, and the greatest extent of tropical rainforests in Africa. It covers more than 100 million hectares (Abernethy et al., 2016).
The leaf was the most used part 122(39.23%), followed by roots 73(23.47%), and stem bark 43(13.83%). According to (Moshi et al., 2012), the frequent use of leaves is associated with ease of accessibility among the aboveground parts of plants in natural ecosystems. The formulations prepared consisted more often of decoction for 173(60.49%), followed by maceration 31(10.84%) and infusion 29(10.14%). However, in 24 cases (8.39%), the formulation has not been reported. Decoction has often been the effective formulation of herbal remedies as it is easy to prepare by mixing a drug with boiling water (Mahomoodally et al., 2016).
Out of 213 plants listed, 103(33.66%) were found at site R5 and 85(27.78%) at R6. The majority of plants had local vernacular names, except in few cases where the author did not mention the names. For instance, Catharanthus roseus was found at almost all locations (except site R4) and Allium cepa at five sites. However, A. sativum, Cassia alata, C. occidentalis, Mangifera indica, Persea americana, and Vernonia amygdalina were quoted at four locations.
4.1.3 Validation of Ethnopharmacological Data
Studies undertook outside DRC confirmed the use of the majority of plants cited as antidiabetic remedies. Albizia adianthifolia was the most reported antidiabetic with eight citations representing an RFC of 0.0063, followed by Catharanthus roseus 7(RFC = 0.0055). However, Allium cepa, Annona senegalensis, and Cassia occidentalis were reported six times (RFC = 0.0047), followed by Mangifera indica, Morinda morindoides, Phaseolus lunatus, and Vernonia amygdalina with five citations (RFC = 0.0039).
Comparatively, Elaeis guineensis was endorsed by 28 uses; the (UV score of 0.0221 was the highest compared to Ocimum gratissimum (0.0150), Antidesma membranaceum (0.0142), Jatropha curcas (0.0126), Bridelia ferruginea (0.0118), and Quassia africana (0.0118). Also, Balanites aegyptiaca, which is employed to treat nine body systems, showed the highest Relative Importance Index (32.5%), compared to Vitis vinifera (28.7%), Zingiber officinale (27.1%), Solanum seretii (24.9%), Thomandersia hensii (24.9%), Lippia multiflora (23.2%) and Stachytarpheta indica (23.2%). The use-value indicators (Table 1) are relative and susceptible to changing since, in the methodology, many authors generally limit themselves to the total number of participants in the studies. The lack of information on the number of informants interviewed was commonly observed in the reviewed studies. According to the Declaration of Helsinki, the direct consequence is that it is no longer possible to analyze the quantitative aspects of these studies (The World Medical Association, 2001).
Consequently, it is not possible to quantify certain vital indexes such as the Cultural Importance Index (CII), Fidelity Level of Citation (FL), Family Use Value, Importance Consensus Factor (ICF), etc. It should be noted that some rare studies make an effort to investigate these parameters, although the information on the number of respondents remains a challenge. One of the weaknesses of ethnopharmacological surveys is that the respondents are often the healers themselves and not or seldom the users. The questionnaires do not scrutinize evidence on the number of people treated and outcomes.
Table 3 illustrates the information gathered through the literature for some plants that can manage Diabetes and other comorbidities and complications. Among the plants listed as antidiabetic, 164(76.99%) species are being used locally in the treatment of several other diseases, mainly infections (bacterial, parasitic, viral, fungal), gastrointestinal and abdominal disorders, cardiac and neurological diseases, gynecological disorders, sexual problems, wounds, dermatological, hematological and metabolic diseases. Commonly, no one plant holds only one indication due to the complexity of the chemical content. The data combine both inside and outside studies.
4.2 Analysis of Pharmacological Data
4.2.1 Preclinical Studies
Different strategies and pathways are used to determine the mechanism of antidiabetic agents, as shown in Figure 6. No study explored in-depth pharmacological mechanisms of action, but all speculated over different modulating metabolic pathways, including 1) Reducing food intake; 2) Reducing carbohydrate digestion and absorption (alpha-amylase, alpha-glucosidase inhibition); 3) Increasing glycogenesis or reducing glycogenolysis and cholesterol synthesis; 4) Free radical scavenging action; 5) Insulin release and pancreas β-cells regeneration; 6) Enhancing glucose transport GLUT4 translocation; 7) dipeptidyl peptidase-4 (DPP-4) inhibition; 8) (PPARs); 9) Insulin-mimetic activity; 10) Modulation on Krebs cycle enzymes.
The analysis of the accurate data for all 213 plants listed showed that most studies used rats and mice, and in a few cases, guinea pigs and rabbits. Both streptozocin (35.55%) and alloxan (24.64%) represented 60.19% of all in vivo reported study models. Streptozotocin presents many advantages over alloxan, including its longer half-life, more productive, stable, and selective to islet beta cells, less toxic, and causing less mortality in animal models (Lenzen, 2008; Wang-fischer and Garyantes, 2018). The majority of the bioactivity investigations link the antioxidant or free radical-scavenging activity with the pathophysiology of Diabetes. However, currently, the in vitro antioxidant model using, for example, DPPH and the others, is not pharmacologically relevant. It can be used as a chemical screening tool. Only in vivo or cell-based models remain relevant (Heinrich et al., 2020). Enzymes are a frequent pharmacological target for establishing the mechanism of action of new drugs. Upon in vitro studies, alpha-glucosidase activity inhibition was the most common investigation (45.3%), followed by inhibition of PTP1B (13.8%), alpha-amylase (9.7%), DPP-4 (1.4%), and 11ß-HSD1 activity (1.0%). Additionally, in cell lines studies, glucose uptake (28.0%) was to be the most commonly used, followed by glucose uptake regulation markers such as GLUT4 translocation and expression levels (9.7%) and PPAR (9.6%) (Munhoz and Fröde, 2017). Some examples of studies are given below.
Azadirachta indica aqueous leaf extract (400 mg/kg bw) improved levels of BG, serum insulin, lipid profile, insulin signaling molecules, and GLUT4 proteins in the tissue of high-fat fructose-induced type-2 diabetic male rat after 30 days of treatment, compared to the control group. In Goto-Kakizaki rats, the acetone extract of Syzygium cumini seed was a potent inhibitor of alpha-glucosidase hydrolysis of maltose compared to untreated control animals (Shinde et al., 2008). Moreover, hepatic tissue demonstrated increased PPARɣ and PPARα protein expressions (Sharma et al., 2012). Oryza sativa extracts significantly elevated glucose uptake, GLUT1, and GLUT4, mRNA levels (Boue et al., 2016). Ethanol extract induced a significant gain in GLUT4 on plasma membranes of L6-GLUT4myc muscle cells at no cytotoxic concentrations (Kadan et al., 2013). Choosing an experimental model is not easy and usually depends on many factors. Ideally, the experiments should be carried out in several different models, considering that none of them ultimately reflects the complexity of human diabetes mellitus type DMT2 and that precautions should be taken to extrapolate the findings to the clinical practice (Arias-Díaz and Balibrea, 2007).
A. adianthifolia is also used to treat syphilis, hiccups, diarrhea, malaria, indigestion, blueness, and an aphrodisiac. Oral administration of 500 mg/kg of plant extract reduced hyperglycemia by 57% in guinea pigs subject to OGTT (Amuri et al., 2017). Albizia grandibracteata is used in filariasis and skin and urinary tract infections. A. garckeana is used in epilepsy and edema of the lower limbs. Oral administration of 500 mg/kg bw aqueous extract under OGTT conditions reduced fasting blood sugar to 36.9% compared to 49.6% of glibenclamide as the reference medicine (Amuri et al., 2017). Certain parts of the plant may be toxic or contain cytotoxic compounds, particularly with gossypol for non-ruminant animals (Randel et al., 1992). Gladiolus gregarius is used to treat hemorrhoids and back pain. Gladiolus klattianus is used for gonorrhea and fever. Under OGTT conditions, the aqueous extract of G. klattianus reduced 35% of blood sugar after 60 min (Amuri et al., 2017). Panda oleosa Pierre has been proposed for HIV/AIDS. The aqueous extract of P. oleosa (25–100 mg/kg) significantly reduced glucose levels in a dose-dependent manner in rabbits under OGTT conditions (Muhoya et al., 2017). Vitex madiensis is used in asthma, anemia, diarrhea, tuberculosis, cough, urinary tract infections, and intestinal amebiasis. The aqueous extract of V. madiensis (500 mg/kg bw) reduced hypoglycemia to 43% compared to 55% obtained with glibenclamide (Amuri et al., 2017). Raffia gentiliana is used for inflammation. Oral administration of aqueous fruit extracts in mice under OGTT conditions demonstrated 27 and 56% reduction after one and 2 hours (Mpiana et al., 2013).
Of the 213 plant species listed, 134(62.91%) underwent experimental studies in animals or in vitro, while only 8.92% reached the clinical trial phase. Inside DRC, only seven plants shown in Table 4 have been studied in animals. A critical analysis of the seven studies carried out inside DRC showed low quality (grade = 4–5). The majority (85.71%) used a single dose in antidiabetic evaluation. However, the Panda oleosa study used three-dose rages (25, 50, and 100 mg/kg body weight). Overall, it is not easy to define an exact upper cut-off dose. In most cases, an oral dose range of 100–200 mg/kg body weight for plant extracts in vivo investigations should be considered the upper limit (Heinrich et al., 2020). Experiments on Albizia adianthifolia, Azanza garckeana, Gladiolus klattianus, and Rauvolfia caffra extracts used the highest dose (500 mg/kg bw) calculated from the human patients of 60 kg treated with 750 ml of plant extract (corresponding to 250 g of dried herbal material per day). Par the way, differences in doses that normalize interspecies variation should be taken into account (Nair and Jacob, 2016).
Temperature and humidity were not reported. The effeteness of the treatment was based on the capacity of the extract to reduce baseline glycemia (hypoglycemia effect) or the capacity to reduce induced hyperglycemia; this varied between 25 and 75%, compared to reference drugs (glibenclamide and metformin). According to (Baker et al., 2014), over 85% of published animal studies do not describe randomization or blinding, and over 95% lack the estimation of sufficient sample size needed for detecting actual effects.
4.2.2 Toxicological Data
For acute toxicity, Figure 7 shows comparative values of LD50 reported for Cola nitida, Sida acuta, Ficus sycomorus, Moringa oleifera, Panda oleosa, Alchornea cordifolia, Morinda lucida, Physalis peruviana, Musanga cecropioides, Vitis vinifera, Erythrina abyssinica, Persea americana, Jatropha curcas, Momordica charantia, and Rauwolfia caffra. Almost all plants but Jatropha curcas are relatively non-toxic (LD50 > 500 mg/kg).
The highest value of LD50 was observed at 8,600 mg/kg bw in rodents with an oral administration of Alchornea cordifolia. The bark extract of Panda oleosa was practically non-toxic in guinea pigs with an LD50 of approximately 7,892 mg/kg bw; no signs of intoxication were observed with oral doses less than 2,000 mg/kg. However, at doses above 6,000 mg/kg, poor mobility, poor appetite, anuria, and death have been noted in animals (Katemo et al., 2018). The administration of the aqueous extract from the bark of Ficus benghalensis suggested an LD50 > 5,000 mg/kg. In some cases, the toxic effects depended on sex, like Alchornea cordifolia, which showed different values of LD50 in mice male compared to female animals (8,600 mg/kg in male and 3,800 mg/kg female) (Djimeli et al., 2017). Despite low acute toxicity, many plants exhibit some significant sub-chronic toxicity. Caesalpinia bonduc extract showed hematological changes after a subchronic study for 28 days at a dose up to 400 mg/kg bw in rats (Ogunlana et al., 2013). Except for the ripe fruit, solanine and other alkaloids present in all parts of Solanum americanum are toxic (Kuete, 2014). Aloe-emodin (anthraquinone) from Aloe species could be mutagenic or/and genotoxic in organs (Lynch et al., 2011). A daily and prolonged administration (28 days) of resveratrol in Vitis vinifera exhibited nephrotoxicity in the rat at the high dose (3000 mg/kg bw). Extracts of Aframomum melegueta (286–345 mg/kg bw) and Artemisia annua (300 mg/kg bw) produced a toxic effect on the development of fetus by discontinuation of first trimester pregnancies in rats (Inegbenebor et al., 2009; Abolaji et al., 2012). Leaf methanol extract of Jatropha curcas decreased the number of live fetuses and increased placental weight (Teixeira et al., 2017). Bulbs’ aqueous extract of Crinum ornatum had caused significant effects (Central Nervous System), including losing appetite, slow movement, depression, less aggression, and lying at the corners of the cage (Lawal and Dangoggo, 2014). Erythrina abyssinica showed similar nervous effects in mice, significantly decreasing motility, sedation, frequent urination, and tremors during the first 6 h after drug administration at different doses (Bunalema et al., 2011). Some compounds in Salvia officinalis (Camphor, thujone, and terpene ketones) are considered the most toxic. Their consumption is not recommended in pregnancy and lactation because they are harmful to the fetus and newborn (Ghorbani and Esmaeilizadeh, 2017). A methanol extract (500 and 1,000 mg/kg/day) of Catharanthus roseus in the subacute investigation for 14 days showed inevitable mortality and presented some of the signs of intoxication on the study of the liver and kidney rats (Kevin et al., 2012). Sometimes, there are some contradictions in findings from different authors on toxicological studies in animals. In Cassia occidentalis, Lagarto et al. (2011) and Mishra et al. (2018) are contradictory. The first group did not report any toxicological signs in biochemical, hematological, and morphological markers, while the second group noticed some changes.
4.2.3 Clinical Trials
Data from the present study showed the lack of local clinical trials of antidiabetic plants used to manage Diabetes in the DRC. Of seven native herbals, only Raphia gentiliana fruit extract was given to 25 males and 20 females, aged 18–50 years old, with normal blood sugar levels (Mpiana et al., 2013). The approach followed by the authors did not comply with any clinical trial requirements, and instead, their behavior went like traditional healers themselves.
Table 5 illustrates the assessment of the quality of clinical trials of antidiabetic plants using the Jadad scale for reporting randomized controlled trials based on randomization, blinding, withdrawals, and dropout methods.
In general, out of 213 plants censored, approximately 8.92% (n = 19) have been validated by clinical evidence. These are Allium cepa (Jafarpour-Sadegh et al., 2017), Allium sativum (Ashraf et al., 2011), Balanites aegyptiaca (Rashad et al., 2017), Citrus aurantium (Campbell-Tofte et al., 2011), Elaeis guineensis (Kalman et al., 2013), Laurus nobilis (Khan et al., 2009), Momordica charantia (Kim et al., 2020), Morinda cordifolia (Algenstaedt et al., 2018), Moringa oleifera (Leone et al., 2018), Rauvolfia-Citrus (Campbell-Tofte et al., 2011), Salvia officinalis (Kianbakht and Dabaghian, 2013), Terminalia chebula (Pingali et al., 2020), Trigonella foenum-graecum (Hadi et al., 2020); Urtica dioica (Kianbakht et al., 2013), Vernonia amygdalina (Okolie et al., 2008), Zea mays (Sands et al., 2009), and Zingiber officinale (Shidfar et al., 2015).
For example, a double-blind, placebo-controlled, randomized clinical trial conducted on 20–60 year-old DMT2 patients who did not receive insulin showed that 3 months supplementation of 3 g of ginger (Zingiber officinale) improved glycemic indices, total antioxidant capacity, malondialdehyde, C-reactive protein, serum paraoxonase, dietary intake, and physical activity, measured at the beginning and end of the study, and after 12 h fasting compared to control groups. A randomized, placebo-controlled, parallel-group study with 42 treated patients treated with leaf hydroethanolic extract (500 mg/8 h for 3 months) and 44 as placebo groups showed that the Salvia officinalis leaves lowered fasting glucose and HbA1c the baseline at the endpoint with no adverse effects reported. A clinical trial on a juice extract from the fruit of Morinda cordifolia (2 ml/kg bw once a day) in patients with DMT2, after 90 days of treatment, presented a significant reduction of morning BG in several cases, an improvement of hyperglycemia status. In a prospective, randomized, double-blind, placebo-controlled clinical investigation, the administration of Terminalia chebula (250 and 500 mg/kg bw, for 12 weeks) in 60 diabetic patients significantly improved the endothelial function (reflection index) compared to placebo (−2.55 ± 1.82%, and −5.21 ± 2.41%, respectively). In an 8-weeks randomized controlled clinical trial study of the effect of Trigonella foenum-graecum intake seed in 50 patients with T2DM, the plant significantly reduced fasting blood glucose. It improved some liver and kidney function compared with control interventions.
A randomized, double-blind, placebo-controlled clinical trial of Urtica dioica leaf extract (500 mg/8 h, 3 months) combined with conventional oral antihyperglycemic drugs was conducted in 46 treated patients vs 46 placebo groups. At the endpoint, the extract significantly lowered the blood levels of fasting glucose, 2 h postprandial glucose, and HbA1c, without significant effects on other hepatic or cardiovascular parameters, vs the placebo. All considered these results demonstrated that nettle is safe and may have a beneficial effect on glycemic control in patients with advanced DMT2 needing insulin therapy. Vernonia amygdalina elicited a significant reduction in BG levels at the most postprandial time points and area-under-curve.
Unfortunately, many studies were carried out in poor quality conditions, with unclear randomization methods, threats to blinding, and lack of baseline demographics (Rios et al., 2015). The interpretation of Jadad score on clinical trials reviewed showed that studies conducted on A. cepa, A. sativum, B. aegyptiaca, E. guineensis, M. charantia, R. vomitoria, S. officinalis, U. dioica, and Z. officinale presented excellent quality (Jadad score ≥ 3)(Hartling et al., 2011). In addition to the effectiveness of the plant materials (extracts, isolated compounds), clinical trials must include other vital parameters to an antidiabetic evaluation in particular glycosylated hemoglobin A1c (HbA1c), personal medication, insulin, glycogen, lipid and protein profiles, and severity of adverse effects, patient’s risk factors, ease of use, patient’s financial situation, etc. (Chaudhury et al., 2017).
4.3 Analysis of Phytochemical Data
Various second metabolites have been identified and isolated, as shown in Table 6 and Figure 8. Qualitative and quantitative content may vary with the soil where the plants are growing.
To illustrate, Allium cepa contains ferulic acid, alliin (Tang et al., 2008), agavasaponin C (Tang et al., 2008), flavonoid alliuocide G (Mohamed, 2008), quercetin, sulfur compounds, S-methyl cystein sulfoxide, S-allyl cysteine and diallyl thiosulfanate (Bakhshaeshi et al., 2012; Noor et al., 2013). Allium sativum contains S-allylcysteine sulfoxide, alliin, diallyl trisulfide (Liu et al., 2007; Mikaili et al., 2013), isoeruboside B, agavasaponin C, proto-iso-erubisoide B, 2-Vinyl-4H-1,3-dithiin (Tang et al., 2008), allicin, diallyl disulfide, diallyl sulfide, ajoene, and allyl mercaptan (Bayan et al., 2014). In mangifera indica, one found mangiferin (Cruz-Vega et al., 2009), 1,2,3,4,6 Penta-O-galloyl-β-d-glucose (Mohan et al., 2013), curcumin, morin (Gothai et al., 2016), gallic acid, protocatechuic acid, kaempferol (Ediriweera et al., 2017). Catarantus has gallic acid, chlorogenic acid (Rianika and Robert, 2007), vindoline I, vindolidine II, vindolicine III and vindolinine (Tiong et al., 2013), catharanthine, vindoline, vindolinene, vinblastine, vincristine (Bharti et al., 2018). Brassica juncea (L.) Czern has cinnamic acid (Guzman, 2014), kaempferol (Gothai et al., 2016), aniline. Bidens pilosa has cytopiloyne, 2-β-D-Glucopyranosyloxy-1-hydroxytrideca-5,7,9,11-tetrayne (Chang et al., 2013a), polyynes (Bartolome et al., 2013), 3-β-D-glucopyranosyl-1-hydroxy-6(E)-tetradecene-8,10,12-triyne; 2-β-D-glucopyranosyloxy-1-hydroxy-5(E)-tridecene-7,9,11-triyne (Chang et al., 2013b). Caesalpinia decapetala has apigenin-7-rhamnoside, astragalin, 6-hydroxy kaempferol, quercitrin (Parveen et al., 2017). Erythrina abyssinica contains daidzein, genistein, glycitein, beta-Sitosterol, Soyasaponin A1-A6, soyasaponin V, stigmasterol (Tang et al., 2008), anthocyanins (Nizamutdinova et al., 2009), lyceollin I-II (Chang et al., 2013b), kaempferol glycoside, kaempferol (Zang et al., 2014), stigmasterol (Wang F. et al., 2017), genistein, diadzein) (Bharti et al., 2018). Phaseolus vulgaris contains stigmasterol (Tang et al., 2008), catechin (Gothai et al., 2016), flavonoids and their glucosides of delphinidin, petunidin, and malvidin, anthocyanins, catechin, myricetin 3-O-arabinoside, epicatechin, vanillic acid, syringic acid, and O-coumaric acid (Ganesan and Xu, 2017). Tephrosia vogelii has galactomannan (Anwar et al., 2011), 4-hydroxyisoleucine (Rangari et al., 2014; Naicker et al., 2016), diosgenin, galactomannan, flavonoids, trigonelline (Zameer et al., 2017). Syzygium guineense contains pinitol, β-sitosterol, quercetin, quercetin 3-O-α-L-rhamnopyranoside (Jawlal et al., 2013). Aframomum melegueta has 3 arylalkanes, 6-paradol, 6-shogaol, 6-gingerol, 6-gingeredione, a pentacyclic triterpene, oleanolic acid isolated from the fruit (Sugita et al., 2013; Mohammed et al., 2017).
4.3.1 Alkaloids
The sulfur compounds present in the onion can significantly control the blood glucose and lipids in serum and tissues and normalize liver hexokinase, glucose 6-phosphatase and HMG CoA reductase (Akash et al., 2014). It was shown that vindoline I, vindolidine II, vindolicine III, and vindolinine improve the hyperglycemia condition of type 2 diabetes by enhancing glucose uptake in pancreatic or muscle cells. In addition, they can inhibit in vitro PTP-1B, which lessens insulin resistance. Vindolicine III was the most potent (Tiong et al., 2013). Catharanthine, vindolinene, vinblastine, vincristine lower blood sugar levels through free radical scavenging action (Bharti et al., 2018). On the other hand, p-synephrine increased the glucose output concentration and ameliorated glycolysis and glycogenolysis (Suntar et al., 2018). N-trans-p-coumaroyloctopamine, N-trans-p-feruloyl-octopamine, N-trans-p-coumaroyltyramine, and N-trans-p-feruloyltyramine, amide alkaloids, showed alpha-glucosidase effect and free radicals inhibitions (Silva et al., 2017).
4.3.2 Amino Acids, Amines, and Carboxylic Acid Derivatives
Alliin offered protection against glucose or methylglyoxal-induced glycation of superoxide dismutase (Anwar and Younus, 2017). S-allyl cystein sulfoxide (SACS), allicin, and garlic oil precursor stimulated in vitro insulin secretion from beta cells isolated from normal rats (Kodera et al., 2017). It restored erectile function in diabetic rats (Yang et al., 2013). Unique and repeated intraperitoneal administrations of a protein (Mo-LPI) decreased blood glucose concentration at different times in rats. 2S, 3R,4S hydroxy isoleucine, an amino acid considered an insulinotropic agent, possesses antidiabetic potential by several mechanisms, including regulating glucose metabolism, lipid profile, and uric acid (Rangari et al., 2014).
4.3.3 Carbohydrates and Sucrose Esters
Peruvioses A,B,C,D,E, and F possess antidiabetic potential by alpha-amylase inhibition activity (Bernal et al., 2018). In the Streptozotocin-induced diabetic mice group, rhamnogalacturonan (a polysaccharide) decreased blood glucose level and glucose tolerance and slightly improved blood glucose within 30 min (Liu et al., 2017). Polysaccharides repaired the pancreatic β cells damages in a high-fat diet STZ-induced type 2 diabetic mice by improvement of SOD concentration and the reduction of MDA level and restoration of kidney and pancreas tissues (Wang et al., 2019). Furthermore, a water-soluble polysaccharide significantly lowered fasting blood glucose level and improved glucose tolerance and weight loss in alloxan-induced diabetic mice compared to the diabetic control group (Xu et al., 2015).
4.3.4 Glycosides
Cytopiloyne, a polyacetylene glucoside, reduced postprandial blood glucose levels, increased blood insulin, improved glucose tolerance, suppressed HbA1c level, and protected pancreatic islets in diabetic db/db mice (Chang et al., 2013b).
Supplementation of Naringin improved glucose intolerance and insulin resistance in a model of high-fat-diet–fed mice (Pu et al., 2012). Naringin (together with Neohesperidin, hesperidin, and nobiletin) significantly inhibited amylase-catalyzed starch digestion and played roles in hyperglycemia management by increasing hepatic glycolysis and glycogen concentration and lowering hepatic gluconeogenesis. Furthermore, hesperidin, naringin, and nobiletin reduced hepatic gluconeogenesis and improved insulin sensitivity in animal models (Lv et al., 2015). Neohesperidin significantly decreased fasting glucose, serum glucose, and glycosylated serum protein in mice. In addition, this compound significantly reduced serum triglycerides, total cholesterol, leptin level, and liver index; it inhibited lipid accumulation in the liver and decreased the size of epididymal adipocytes in the KK-Ay mice (Osfor et al., 2013; Jia et al., 2015).
Some phenolic glycosides, including niazirin A, S-Methyl-N-{4-[(α-l-rhamnosyloxy)benzyl]}thiocarbamate, reduced blood glucose levels in STZ-induced diabetic mice and promoted the glucose consumption of IR cells (Wang F. et al., 2017).
Isothiocyanates inhibited gluconeogenesis and hepatic glucose-6-phosphatase (G6P) expression in hepatoma cells and improved glucose tolerance and insulin signaling sensitivity (Waterman et al., 2016).
Galactomannan showed significant dose-related hypoglycaemic and antihyperglycaemic effects; the obtained results were better than glibenclamide used as reference (Anwar et al., 2011).
Aloe-emodin-8-O-glycoside enhanced glucose transport through proximal and distal marker modulation involved in glucose uptake and its transformation into glycogen (Salehi et al., 2018).
Syringin, a phenylpropanoid glucoside, indicated a significant reduction of blood glucose and HbA1c levels and improved transaminase enzymes, plasma protein, blood urea, serum creatinine, and uric acid levels. Inversely, it increased plasma insulin and hemoglobin levels in diabetic rats (Sundaram et al., 2014).
Rutin (a flavonol glycoside) significantly increased in vivo glucose-induced insulin secretion and acted as an insulin secretagogue in the management of glucose homeostasis (Kappel et al., 2013). Hirsutrin was suggested to prevent osmotic stress in hyperglycemia conditions by inhibiting RLAR activity and galactitol formation in rat lenses (Kim et al., 2013).
According to Fernando et al. (2019), three flavone C-glycosides, vicenin-1, isoschaftoside, and schaftoside, respectively, inhibited 60.3, 33.8, and 95.5% of pancreatic lipase enzyme, which plays a vital role in obesity (as a crucial factor in the occurrence of DMT2). Phenolic-C-glycosides enhanced and stimulated the glucose update process in mouse skeletal muscle cells (Mishra et al., 2013).
A stearoyl glucoside of ursolic acid (urs-12-en-3β-ol-28-oic acid 3β-D-glucopyranosyl-4′- octadecanoate) demonstrated an antidiabetic property by lowering sugar blood in rats from the 8th day to the 21st day of the experiment (Kazmi et al., 2012).
4.3.5 Phytosterols
Sanni et al. (2019) suggested that sitosterol, stigmasterol, campesterol, squalene, and nimbiol might have antidiabetic potential through their molecular docking with AMP-activated protein kinase (α-AMPK) and alpha-amylase and alpha-glucosidase inhibitions. Stigmasterol increased GLUT4 translocation and expression in vitro. In mice, it alleviated insulin resistance, glucose tolerance by reducing fasting blood glucose levels and blood lipid (triglyceride and cholesterol) (Wang F. et al., 2017).
4.3.6 Polyphenols
Quercetin and its glycosides protected β-cell mass and function under high-fructose induction (Li et al., 2013). 4'-methyl-quercetin-7-O-β-D-glucuronopyranoside enzymes, quercetin-3-O-glucoside, avicularin, castalagin, and 2,3-hexahydroxydiphenoyl-(α/β)-D-(4)C1-glucopyranose showed inhibition capacity of sucrase (Abdelhady et al., 2016). Moreover, they exhibited significant inhibition of alpha-glucosidase and alpha-amylase enzymes compared to acarbose (Wang et al., 2010; Olennikov and Kashchenko, 2014). A flavonoid named alliuocide G showed in vitro alpha-amylase inhibitory activity and radical scavenging potency (Mohamed, 2008).
Cinnamic acid and its derivatives (caffeic acid, ferulic acid, isoferulic acid, and p-hydroxycinnamic acid) are associated with a beneficial influence on Diabetes and its complications through many mechanisms. The most well-known are: stimulation of insulin secretion, improvement of pancreatic β-cell functionality, inhibition of hepatic gluconeogenesis, enhanced glucose uptake, increased insulin signaling pathway, delay of carbohydrate digestion and glucose absorption, and inhibition of protein glycation (Adisakwattana, 2017). Ferulic acid regenerated pancreatic beta-cells, reduced the risk of high-fat diet-induced hyperglycemia via insulin secretion and hepatic glucose-regulating enzyme activities, and regulated blood glucose levels by elevating glucokinase activity and production of glycogen (Silva and Batista, 2017). Caffeic acid produced a significant alpha-glucosidase inhibition comparing with acarbose (Olennikov and Kashchenko, 2014). In addition, together with chlorogenic acid and chicoric acid, it increased glucose uptake in muscle cells and stimulated insulin secretion from an insulin-sensitizing and insulin-secreting cell line and islets (Tousch et al., 2008; Ferrare et al., 2017). Caffeoylquinic acid derived from caffeic acid showed high inhibitory activity against digestive enzymes, exceptionally higher against alpha-amylase and alpha-glucosidase (Olennikov et al., 2018).
A study indicated that epigallocatechin and epigallocatechin gallate reduced fasting blood glucose levels, triglycerides, and total cholesterol in streptozotocin-induced diabetic mice (Bakoma et al., 2018). Also, apigenin-7-rhamnoside, astragalin, 6-hydroxy kaempferol, quercitrin exhibited significant activity against alpha-glucosidase enzyme (Parveen et al., 2017). Kaempferol (Fraction B) lowered blood glucose of alloxan-induced diabetic rats. It also inhibited alpha-amylase and alpha-glucosidase and reversed altered lipid profile and oxidative stress biomarkers in diabetic rats (Ibitoye et al., 2017). Kaempferol and myricetin showed high inhibitory activities against alpha-amylase and alpha-glucosidase (Wang et al., 2010).
Compared with the reference compound acarbose, Aesculetin and isorhamnetin demonstrated significantly higher inhibitory activity (Olennikov and Kashchenko, 2014). Polyphenols compounds such as proanthocyanidins and anthocyanins showed as potential natural alpha-glucosidase inhibitors (Dey and Mitra, 2013).
Anthocyanins efficiently protected pancreatic beta-cells from cell death in HIT-T15 cell culture and db/db mice (Hong et al., 2013). Johnson et al. (2015) demonstrated that prenylated anthranols possess an alpha-glucosidase inhibitory potential. According to their findings, the most antidiabetic activity was found with harunganol compared to acarbose.
Kolaviron, a bioflavonoid complex, demonstrated a significant reduction of glycemia in normoglycemic rats. Moreover, kolaviron showed a significant antidiabetic potential in streptozotocin-induced rats (Adaramoye and Adeyemi, 2006).
Ellagic acid and its derivatives act as a hypoglycaemic agent on carbohydrate digestion and absorption, insulin secretion (Bharti et al., 2018). Hydrolyzable tannins including 1,2,3,6-tetra-O-galloyl-4-O-cinnamoyl-b-D-glucose and 4-O-(200,400-di-O-gal-loyl-a-L-rhamnosyl) ellagic acid showed significant alpha-glucosidase inhibitory efficacy with IC50 values of 2.9 and 6.4 mM, respectively (Lee et al., 2017).
Chicoric acid lowered the glycaemic levels of diabetic mice (Casanova et al., 2014) significantly. Valoneic acid dilactone, a hydrolyzable tannin, showed a potential antidiabetic effect alpha-amylase enzyme activity compared to the value obtained by acarbose. In the same way, it significantly inhibited aldose reductase enzyme activity and PTP1B enzyme activity. However, in vivo evaluation, it reduced the BGL considerably in acute evaluation for 4 h. Furthermore, oral administration of the compound for 21 days significantly decreased BGL and improved the tolerance to glucose compared to control groups (Jain et al., 2012).
Gingerols demonstrated antidiabetic potential by enhancing glucose uptake. Primarily, (S)-[8]-Gingerol was found to be the most potent on glucose uptake and increase in the surface distribution of GLUT4 protein on the L6 myotube plasma membrane (Noipha and Ninla-aesong, 2018).
ρ-Coumaric acid exhibited higher inhibition activity against alpha-glucosidase (98.8%) than acarbose (62.5%). However, acarbose showed the most potent inhibition against alpha-amylase (98.6 vs 66.8%) (Aalim et al., 2019).
Luteolin showed significant alpha-glucosidase and alpha-amylase inhibitory activities (Dekdouk et al., 2015). Chebulagic acid (a benzopyran tannin) reduced maltose-hydrolysis and sucrose-hydrolysis activities. Meanwhile, it induced a decrease at 11.1% of postprandial blood sugar value in maltose-loaded Sprague-Dawley rats (Huang et al., 2012).
Furofuran lignans with a free hydroxyl synthesized from herein demonstrated an inhibition potential against alpha-glucosidase and free radicals (Worawalai et al., 2016). Previously, Wikul et al. (2012) conducted bio-guided isolation and showed that (+)-pinoresinol, a lignan, had inhibitory activity against rat intestinal maltase. Also, N-trans-feruloyl tyramine, N-trans-p-coumaroyl tyramine, and N-cis-p-coumaroyl tyramine (Phenylmethyl cinnamates) showed inhibitory activity against alpha-glucosidase (Liu et al., 2011).
4.3.7 Saponins
Pseudoprototinosaponin AIII and prototinosaponins AIII produced a hypoglycaemic effect on glucose uptake and insulin release due to their actions on hepatic gluconeogenesis or glycogenolysis (Patel et al., 2012). Furostanol saponin showed significant antidiabetic potential in vitro by reducing the fasting plasma glucose level by 46.14% and increasing insulin and C-peptide levels (Ezzat et al., 2017). [3β,7β,25- trihydroxycucurbita-5,23(E)-dien-19-al, momordicine I, momordicine II, 3- hydroxycucurbita-5,24-dien-19-al-7,23-di-O-β-glucopyranoside, and kuguaglycoside G] were potent in the β-cell insulin secretion evaluation. Momordicine II and kuguaglycoside have stimulated insulin secretion 7.3 and 7.1 times and 8.1 and 7.8 times more, respectively, than the control group (Keller et al., 2011).
25-O-methylkaraviagein D, karaviloside II, and (19R,23E)-5b,19-epox y-19,25-dimethoxycucurbita-6,23-dien-3b-ol, cucurbitane exhibited significant inhibitory activity on alpha-glucosidase with IC50 values of 10.19, 28.55, and 20.20 µM, respectively (Yue et al., 2017). Oral administration of saponins improved body weight and insulin resistance. There was an increase in fasting blood glucose concentration and the proportion of hepatic phosphorylated adenosine monophosphate-activated protein kinase (p-AMPK)/total protein (Wang et al., 2019).
4.3.8 Terpenoids
Oleanolic acid, a plant-derived triterpenoid, boosted insulin secretion in vitro and stimulated insulin secretion at both basal and stimulatory glucose concentrations in INS-1 832/13 cells, and enhanced acute glucose-stimulated insulin secretion cultured β-cells (Teodoro et al., 2008). Furthermore, it decreased serum glucose and insulin concentrations in mice fed with a high-fat diet and enhanced glucose tolerance (Sato et al., 2007). Oleanolic and ursolic acids showed potent alpha-glucosidase and alpha-amylase inhibition. Ursolic acid showed uncompetitive inhibition of alpha-glucosidase compared to acarbose as a competitive inhibitor (Ali et al., 2006; Salah El Dine et al., 2014).
Thujone, a monoterpene existing as two stereoisomers (α- and β-Thujone), is an ingredient of essential oils of many great different herbs; it can increase free insulin-stimulated glucose transporter by activation of adenosine monophosphate-activated protein kinase (Daradka et al., 2014).
α-amyrin acetate (a pentacyclic triterpenoid) lowered the blood glucose profile in STZ-induced diabetic rats and db/db mice at 50 mg kg1 dose level (Singh et al., 2009). Pahlavani et al. (2019) showed that some compounds like charantin (a triterpenoid phytoconstituent), possess antidiabetic potential by several mechanisms, including insulin secretion increase, insulin resistance decrease, skeletal muscle cell glucose utilization increase, and inhibition of intestinal enzymes.
Cucurbitane-type compounds (3β,7β,25-trihydroxycucurbita-5,23(E)-dien-19-al, charantal, charantoside XI, and 25ξ-isopropenylchole-5, 6-ene-3-O-D-glucopyranoside), demonstrated an alpha-amylase and alpha-glucosidase inhibitory activities ranging from 56 to 79% (Shivanagoudra et al., 2019).
Two monoterpenes (1S,2R,3R,5S)-2-hydroxymethyl-6,6-dimethylbicyclo[3.1.1]heptane-2,3- diol, and sobrerol significantly increased glucose uptake in 3T3-L1 adipocytes (Li et al., 2013b). On the other hand, three germacrene sesquiterpenes increased glucose uptake substantially without significant toxic effects in 3T3-L1 adipocytes (Zhao et al., 2012).
5 Conclusion
Multiple investigations have been carried out on natural products, mainly plants used to treat Diabetes Mellitus worldwide. In DRC, a country with a high ecological, cultural and human diversity, traditional medicine through plants occupies an important place in the health system. Several ethnopharmacological and ethnobotanical studies have been conducted previously in this perspective, and various plant species have been identified. Contrary to the previous review, the present review assessed the quality of studies carried inside DRC and resorted similarities/discrepancies with studies conducted outside. The findings confirm the high diversity of the flora and the various ethnic groups in DRC. Most of the plants claimed as antidiabetic and used by traditional healers in the DRC are not specifically native to DRC. One hundred thirty-four native and introduced species have been experimentally validated by various pharmacological, toxicological, and phytochemical researches. Many plants are safe at doses < 500 mg/kg, but long-term use may trigger sub-chronic toxicity. Exclusively conducted in DRC, preclinical and clinical studies of some plant species demonstrated poor protocol quality. Locally specific species deserve in-depth investigations to meet scientific requirements for their introduction studies into the national pharmacopeia. Although a few plants reduced blood sugar levels, clinical data and antidiabetic studies of the isolated compounds remain limited to allow the availability and accessibility of standardized phytomedicines to Congolese. This review constitutes a primary database for further experimental studies, especially for unstudied species in the perspective of safe and efficient use of easily accessible natural resources.
Author Contributions
FK conceived the manuscript, wrote the first draft, and analyzed data. JK analyzed data and rewrote the manuscript draft. All authors conducted the literature search. All authors read, corrected, and approved the final manuscript.
Conflict of Interest
The authors declare that the research was conducted in the absence of any commercial or financial relationships that could be construed as a potential conflict of interest.
Publisher’s Note
All claims expressed in this article are solely those of the authors and do not necessarily represent those of their affiliated organizations, or those of the publisher, the editors and the reviewers. Any product that may be evaluated in this article, or claim that may be made by its manufacturer, is not guaranteed or endorsed by the publisher.
Acknowledgments
The authors are grateful to the Mbarara University of Science and Technology (MUST), Pharm-Bio Technology and Traditional Medicine Centre (PHARMBIOTRAC), and The World Academy of Science (TWAS), International Center of Chemical and Biological Sciences (ICCBS) for providing Ph.D. Scholarships to FK.
Supplementary Material
The Supplementary Material for this article can be found online at: https://www.frontiersin.org/articles/10.3389/fphar.2021.757090/full#supplementary-material
Abbreviations
11ß-HSD1, 11β-hydroxysteroid dehydrogenase type 1; BG, blood glucose; DM, diabetes mellitus; DMT2, Diabetes mellitus type 2; DPP-4, dipeptidyl peptidase-4; DRC, Democratic Republic of Congo; HIV/AIDS, human immunodeficiency virus/Acquired immunodeficiency syndrome; GLP-1, glucagon-like peptide- 1; GLUT, Glucose Transport; HbA1c, Glycosylated hemoglobin; LD50, lethal dose 50; mRNA, Messenger ribonucleic acid; OGTT, Oral Glucose Tolerance Test; PPAR, peroxisome proliferator-activated receptor; PTP1B, Protein tyrosine phosphatase 1B.
References
Aalim, H., Belwal, T., Jiang, L., Huang, H., Meng, X., and Luo, Z. (2019). Extraction Optimization, Antidiabetic and Antiglycation Potentials of Aqueous Glycerol Extract from rice (Oryza Sativa L.) Bran. Lwt 103, 147–154. doi:10.1016/j.lwt.2019.01.006
Abdelhady, M. I., Shaheen, U., Bader, A., and Youns, M. A. (2016). A New Sucrase Enzyme Inhibitor from Azadirachta indica. Pharmacogn. Mag. 12, S293–S296. doi:10.4103/0973-1296.185705
Abernethy, K., Maisels, F., and White, L. J. T. (2016). Environmental Issues in Central Africa. Annu. Rev. Environ. Resour. 41, 1–33. doi:10.1146/annurev-environ-110615-085415
Abolaji, A. O., Eteng, M. U., Ebong, P. E., Brisibe, E. A., Dar, A., Kabir, N., et al. (2012). A Safety Assessment of the Antimalarial HerbArtemisia annuaDuring Pregnancy in Wistar Rats. Phytother. Res. 27, 647–654. doi:10.1002/ptr.4760
Adaramoye, O. A., and Adeyemi, E. O. (2006). Hypoglycaemic and Hypolipidaemic Effects of Fractions from Kolaviron, a Biflavonoid Complex from Garcinia Kola in Streptozotocin-Induced Diabetes Mellitus Rats. J. Pharm. Pharmacol. 58, 121–128. doi:10.1211/jpp.58.1.0015
Adebayo, A. H., Zeng, G. Z., Zhang, Y. M., Ji, C. J., Akindahunsi, A., and Tan, N. H. (2010). Toxicological Evaluation of Precocene II Isolated from Ageratum Conyzoides L. (Asteraceae ) in Sprague Dawley Rats. Afr. J. Biotechnol. 9, 2938–2944.
Adisakwattana, S. (2017). Cinnamic Acid and its Derivatives: Mechanisms for Prevention and Management of Diabetes and its Complications. Nutrients 9, 163. doi:10.3390/nu9020163
Adrien Kasanga, L. (2012). English in the Democratic Republic of the Congo. World Englishes 31, 48–69. doi:10.1111/j.1467-971x.2011.01732.x
Agarwal, A., D'Souza, P., Johnson, T. S., Dethe, S. M., and Chandrasekaran, C. (2014). Use of In Vitro Bioassays for Assessing Botanicals. Curr. Opin. Biotechnol. 25, 39–44. doi:10.1016/j.copbio.2013.08.010
Akash, M. S. H., Rehman, K., and Chen, S. (2014). Spice Plant Allium cepa: Dietary Supplement for Treatment of Type 2 Diabetes Mellitus. Nutrition 30, 1128–1137. doi:10.1016/j.nut.2014.02.011
Algenstaedt, P., Stumpenhagen, A., and Westendorf, J. (2018). The Effect ofMorinda citrifoliaL. Fruit Juice on the Blood Sugar Level and Other Serum Parameters in Patients with Diabetes Type 2. Evidence-Based Complement. Altern. Med. 2018, 1–10. doi:10.1155/2018/3565427
Ali, H., Houghton, P. J., and Soumyanath, A. (2006). Alpha-Amylase Inhibitory Activity of Some Malaysian Plants Used to Treat Diabetes; with Particular Reference to Phyllanthus Amarus. J. Ethnopharmacol. 107, 449–455. doi:10.1016/j.jep.2006.04.004
Amuri, B., Maseho, M., Simbi, L., Duez, P., and Byanga, K. (2018). Ethnobotanical Survey of Herbs Used in the Management of Diabetes Mellitus in Southern Katanga Area/DR Congo. Pan Afr. Med. J. 30, 218. doi:10.11604/pamj.2018.30.218.11718
Amuri, B., Maseho, M., Simbi, L., Okusa, P., Duez, P., and Byanga, K. (2017). Hypoglycemic and Antihyperglycemic Activities of Nine Medicinal Herbs Used as Antidiabetic in the Region of Lubumbashi (DR Congo). Phytother Res. 31, 1029–1033. doi:10.1002/ptr.5814
Anh, H. L. T., Vinh, L. B., Lien, L. T., Cuong, P. V., Arai, M., Ha, T. P., et al. (2021). In Vitro study on α-amylase and α-glucosidase Inhibitory Activities of a New Stigmastane-type Steroid Saponin from the Leaves of Vernonia Amygdalina. Nat. Product. Res. 35, 873–879. doi:10.1080/14786419.2019.1607853
Anwar, S., and Younus, H. (2017). Inhibitory Effect of Alliin from Allium Sativum on the Glycation of Superoxide Dismutase. Int. J. Biol. Macromol. 103, 182–193. doi:10.1016/j.ijbiomac.2017.05.043
Anwar, S., Desai, S., Eidi, M., and Eidi, A. (2011). Antidiabetic Activities of Fenugreek (Trigonella Foenum-Graecum) Seeds. Nuts and Seeds in Health and Disease Prevention 469, 478. doi:10.1016/B978-0-12-375688-6.10056-8
Arias-Díaz, J., and Balibrea, J. (2007). Animal Models in Glucose Intolerance and Type-2 Diabetes. Nutr. Hosp. 22, 160–168. doi:10.2478/cipms-2019-0036
Ashraf, R., Khan, R., and Ashraf, I. (2011). Effects of Garlic on Blood Glucose Levels and HbA1c in Patients with Type 2 Diabetes Mellitus. J. Med. Plants Res. 5, 2922–2928.
Auboire, L., Sennoga, C. A., Hyvelin, J. M., Ossant, F., Escoffre, J. M., Tranquart, F., et al. (2018). Microbubbles Combined with Ultrasound Therapy in Ischemic Stroke: A Systematic Review of Iin-Vvivo Preclinical Studies. PLoS One 13, e0191788. doi:10.1371/journal.pone.0191788
Baker, D., Lidster, K., Sottomayor, A., and Amor, S. (2014). Two Years Later: Journals Are Not yet Enforcing the ARRIVE Guidelines on Reporting Standards for Pre-clinical Animal Studies. Plos Biol. 12, e1001756. doi:10.1371/journal.pbio.1001756
Bakhshaeshi, M., Khaki, A., Fathiazad, F., Khaki, A. A., and Ghadamkheir, E. (2012). Anti-oxidative Role of Quercetin Derived from Allium cepa on Aldehyde Oxidase (OX-LDL) and Hepatocytes Apoptosis in Streptozotocin-Induced Diabetic Rat. Asian Pac. J. Trop. Biomed. 2, 528–531. doi:10.1016/S2221-1691(12)60090-2
Bano, A., Ahmad, M., Ben Hadda, T., Saboor, A., Sultana, S., Zafar, M., et al. (2014). Quantitative Ethnomedicinal Study of Plants Used in the Skardu valley at High Altitude of Karakoram-Himalayan Range, Pakistan. J. Ethnobiol. Ethnomed. 10, 43. doi:10.1186/1746-4269-10-43
Bartolome, A. P., Villaseñor, I. M., and Yang, W.-C. (2013). Bidens pilosaL. (Asteraceae): Botanical Properties, Traditional Uses, Phytochemistry, and Pharmacology. Evidence-Based Complement. Altern. Med. 2013, 1–51. doi:10.1155/2013/340215
Bashige Chiribagula Valentin, B. C., Alombong Alonie Gracia, A. A., Kamwimba Mahungala Arlette, K. M., Bakari Amuri Salvius, B. A., and Okusa Ndjolo philippe, O. N. (2020). Ethnobotanical Study of Medicinal Plants Used in the Treatment of Sexual Dysfunctions in Traditional Medicine in Kampemba-Lubumbashi, DR Congo. World J. Adv. Res. Rev. 7, 016–032. doi:10.30574/wjarr.2020.7.3.0328
Bashige-Chiribagula, V., Bakari-Amuri, S., Mbuyi-Kalonji, S., Kahumba-Byanga, J., Duez, P., and Lumbu-Simbi, J. B. (2017). Étude ethnobotanique, phytochimique et évaluation de l'activité antiplasmodiale de 13 plantes réputées antipaludéennes dans la commune du Kenya (Lubumbashi, RDC). Phytothérapie. doi:10.1007/s10298-017-1152-x
Batomayena, B., Benedicte, B., Aboudoulatif, D., Kwashie, E.-G., Kodjo, A., Messanvi, G., et al. (2018). Catechins as Antidiabetic Compounds of Bridelia Ferruginea Benth Root Bark Extract. J. Pharmacognosy Phytother. 10, 182–186. doi:10.5897/JPP2018.0528
Bayan, L., Koulivand, P. H., and Gorji, A. (2014). Garlic: A Review of Potential Therapeutic Effects. Avicenna J. Phytomed 4, 1–14.
Belhadj, S., Hentati, O., Hammami, M., Ben Hadj, A., Boudawara, T., Dammak, M., et al. (2018). Metabolic Impairments and Tissue Disorders in Alloxan-Induced Diabetic Rats Are Alleviated by Salvia Officinalis L. Essential Oil. Biomed. Pharmacother. 108, 985–995. doi:10.1016/j.biopha.2018.09.108
Bennett, B. C., and Prance, G. T. (2000). Introduced Plants in the Indigenous Pharmacopoeia of Northern South America. Econ. Bot. 54, 90–102. doi:10.1007/bf02866603
Bernal, C. A., Castellanos, L., Aragón, D. M., Martínez-Matamoros, D., Jiménez, C., Baena, Y., et al. (2018). Peruvioses A to F, Sucrose Esters from the Exudate of Physalis Peruviana Fruit as α-amylase Inhibitors. Carbohydr. Res. 461, 4–10. doi:10.1016/j.carres.2018.03.003
Bharti, S. K., Krishnan, S., Kumar, A., and Kumar, A. (2018). Antidiabetic Phytoconstituents and Their Mode of Action on Metabolic Pathways. Ther. Adv. Endocrinol. Metab. 9, 81–100. doi:10.1177/2042018818755019
Boue, S. M., Daigle, K. W., Chen, M. H., Cao, H., and Heiman, M. L. (2016). Antidiabetic Potential of Purple and Red rice (Oryza Sativa L.) Bran Extracts. J. Agric. Food Chem. 64, 5345–5353. doi:10.1021/acs.jafc.6b01909
Bunalema, L., Kirimuhuzya, C., Tabuti, J. R., Waako, P., Magadula, J. J., Otieno, N., et al. (2011). The Efficacy of the Crude Root Bark Extracts of Erythrina Abyssinica on Rifampicin Resistant Mycobacterium tuberculosis. Afr. Health Sci. 11, 587–593.
Burlando, B., and Cornara, L. (2014). Therapeutic Properties of rice Constituents and Derivatives (Oryza Sativa L.): A Review Update. Trends Food Sci. Technology 40, 82–98. doi:10.1016/j.tifs.2014.08.002
Burnham, R. J., and Johnson, K. R. (2004). South American Palaeobotany and the Origins of Neotropical Rainforests. Philos. Trans. R. Soc. Lond. B Biol. Sci. 359, 1595–1610. doi:10.1098/rstb.2004.1531
Campbell-Tofte, J. I., Mølgaard, P., Josefsen, K., Abdallah, Z., Hansen, S. H., Cornett, C., et al. (2011). Randomized and Double-Blinded Pilot Clinical Study of the Safety and Anti-diabetic Efficacy of the Rauvolfia-Citrus tea, as Used in Nigerian Traditional Medicine. J. Ethnopharmacol. 133, 402–411. doi:10.1016/j.jep.2010.10.013
Casanova, L. M., Da Silva, D., Sola-Penna, M., Camargo, L. M., Celestrini, Dde. M., Tinoco, L. W., et al. (2014). Identification of Chicoric Acid as a Hypoglycemic Agent from Ocimum Gratissimum Leaf Extract in a Biomonitoring In Vivo Study. Fitoterapia 93, 132–141. doi:10.1016/j.fitote.2013.12.024
Chang, C. L.-T., Liu, H.-Y., Kuo, T.-F., Hsu, Y.-J., Shen, M.-Y., Pan, C.-Y., et al. (2013a). Antidiabetic Effect and Mode of Action of Cytopiloyne. Evidence-Based Complement. Altern. Med. 2013, 1–13. doi:10.1155/2013/685642
Chang, C. L. T., Lin, Y., Bartolome, A. P., Chen, Y.-C., Chiu, S.-C., and Yang, W.-C. (2013b). Herbal Therapies for Type 2 Diabetes Mellitus: Chemistry, Biology, and Potential Application of Selected Plants and Compounds. Evidence-Based Complement. Altern. Med. 2013, 1–33. doi:10.1155/2013/378657
Chaudhury, A., Duvoor, C., Reddy Dendi, V. S., Kraleti, S., Chada, A., Ravilla, R., et al. (2017). Clinical Review of Antidiabetic Drugs: Implications for Type 2 Diabetes Mellitus Management. Front. Endocrinol. (Lausanne) 8, 6. doi:10.3389/fendo.2017.00006
Chauhan, A., Sharma, P., Srivastava, P., Kumar, N., and Dudhe, R. (2010). Plants Having Potential Antidiabetic Activity: a Review. Der Pharm. Lett. 2, 369–387. doi:10.5958/j.0975-4261.2.1.005
Cruz-Vega, D., Verde-Star, M. J., Salinas-Gonzalez, N. R., Rosales-Hernandez, B., Estrada-Garcia, I., Mendez-Aragon, P., et al. (2009). Review of Pharmacological Effects of Glycyrrhiza Radix and its Bioactive Compounds. Zhongguo Zhong Yao Za Zhi 22, 557–559. doi:10.1002/ptr
Daradka, H. M., Abas, M. M., Mohammad, M. A. M., and Jaffar, M. M. (2014). Antidiabetic Effect of Artemisia Absinthium Extracts on Alloxan-Induced Diabetic Rats. Comp. Clin. Pathol. 23, 1733–1742. doi:10.1007/s00580-014-1963-1
de Oliveira Silva, E., and Batista, R. (2017). Ferulic Acid and Naturally Occurring Compounds Bearing a Feruloyl Moiety: A Review on Their Structures, Occurrence, and Potential Health Benefits. Compr. Rev. Food Sci. Food Saf. 16, 580–616. doi:10.1111/1541-4337.12266
Dekdouk, N., Malafronte, N., Russo, D., Faraone, I., De Tommasi, N., Ameddah, S., et al. (2015). Phenolic Compounds fromOlea europaeaL. Possess Antioxidant Activity and Inhibit Carbohydrate Metabolizing EnzymesIn Vitro. Evidence-Based Complement. Altern. Med. 2015, 1–9. doi:10.1155/2015/684925
Dey, B., and Mitra, A. (2013). Chemo-profiling of eucalyptus and Study of its Hypoglycemic Potential. World J. Diabetes 4, 170–176. doi:10.4239/wjd.v4.i5.170
Djimeli, M. N., Fodouop, S. P. C., Njateng, G. S. S., Fokunang, C., Tala, D. S., Kengni, F., et al. (2017). Antibacterial Activities and Toxicological Study of the Aqueous Extract from Leaves of Alchornea Cordifolia (Euphorbiaceae). BMC Complement. Altern. Med. 17, 349. doi:10.1186/s12906-017-1854-5
Ediriweera, M. K., Tennekoon, K. H., and Samarakoon, S. R. (2017). A Review on Ethnopharmacological Applications, Pharmacological Activities, and Bioactive Compounds ofMangifera indica(Mango). Evidence-Based Complement. Altern. Med. 2017, 1–24. doi:10.1155/2017/6949835
El Deeb, K. S., Eid, H. H., Ali, Z. Y., Shams, M. M., and Elfiky, A. M. (2021). Bioassay-guided Fractionation and Identification of Antidiabetic Compounds from the Rind of Punica granatum Var. Nana. Nat. Prod. Res. 35, 2103–2106. doi:10.1080/14786419.2019.1655411
El-baz, F. K., Aly, H. F., Abd-alla, H. I., and Saad, S. A. (2014). Bioactive Flavonoid Glycosides and Antidiabetic Activity of Jatropha Curcas on Streptozotocin-Induced Diabetic Rats. Int. J. Pharm. Sci. Rev. Res. 29, 143–156.
El-mostafa, K., El Kharrassi, Y., Badreddine, A., Andreoletti, P., Vamecq, J., El Kebbaj, M. S., et al. (2014). Nopal Cactus (Opuntia Ficus-indica) as a Source of Bioactive Compounds for Nutrition, Health and Disease. Molecules 19, 14879–14901. doi:10.3390/molecules190914879
Erukainure, O. L., Oyebode, O. A., Sokhela, M. K., Koorbanally, N. A., and Islam, M. S. (2017). Caffeine - Rich Infusion from Cola Nitida (Kola Nut) Inhibits Major Carbohydrate Catabolic Enzymes; Abates Redox Imbalance; and Modulates Oxidative Dysregulated Metabolic Pathways and Metabolites in Fe2+-Induced Hepatic Toxicity. Biomed. Pharmacother. 96, 1065–1074. doi:10.1016/j.biopha.2017.11.120
Erukainure, O. L., Sanni, O., Ijomone, O. M., Ibeji, C. U., Chukwuma, C. I., and Islam, M. S. (2019). The Antidiabetic Properties of the Hot Water Extract of Kola Nut (Cola Nitida (Vent.) Schott & Endl.) in Type 2 Diabetic Rats. J. Ethnopharmacol. 242, 112033. doi:10.1016/j.jep.2019.112033
Etxeberria, U., de la Garza, A. L., Campión, J., Martínez, J. A., and Milagro, F. I. (2012). Antidiabetic Effects of Natural Plant Extracts via Inhibition of Carbohydrate Hydrolysis Enzymes with Emphasis on Pancreatic Alpha Amylase. Expert Opin. Ther. Targets 16, 269–297. doi:10.1517/14728222.2012.664134
Ezzat, S. M., Abdel Motaal, A., El Awdan, S. A. W., and El, W. (2017). In Vitro and In Vivo Antidiabetic Potential of Extracts and a Furostanol Saponin from Balanites Aegyptiaca. Pharm. Biol. 55, 1931–1936. doi:10.1080/13880209.2017.1343358
Fajrin, F. A., Nugroho, A. E., Nurrochmad, A., and Susilowati, R. (2020). Ginger Extract and its Compound, 6-shogaol, Attenuates Painful Diabetic Neuropathy in Mice via Reducing TRPV1 and NMDAR2B Expressions in the Spinal Cord. J. Ethnopharmacol. 249, 112396. doi:10.1016/j.jep.2019.112396
Farzaei, M. H., Singh, A. K., Kumar, R., Croley, C. R., Pandey, A. K., Coy-Barrera, E., et al. (2019). Targeting Inflammation by Flavonoids: Novel Therapeutic Strategy for Metabolic Disorders. Ijms 20, 4957. doi:10.3390/ijms20194957
Fernando, W. I. T., Attanayake, A. M. K. C., Perera, H. K. I., Sivakanesan, R., Jayasinghe, L., Araya, H., et al. (2019). Isolation, Identification and Characterization of Pancreatic Lipase Inhibitors from Trigonella Foenum-graecum Seeds. South Afr. J. Bot. 121, 418–421. doi:10.1016/j.sajb.2018.10.023
Ferrare, K., Bidel, L. P. R., Awwad, A., Poucheret, P., Cazals, G., Lazennec, F., et al. (2018). Increase in Insulin Sensitivity by the Association of Chicoric Acid and Chlorogenic Acid Contained in a Natural Chicoric Acid Extract (NCRAE) of Chicory (Cichorium Intybus L.) for an Antidiabetic Effect. J. Ethnopharmacology 215, 241–248. doi:10.1016/j.jep.2017.12.035
Fred-Jaiyesimi, A., Kio, A., and Richard, W. (2009). α-Amylase Inhibitory Effect of 3β-olean-12-en-3-yl (9Z)-Hexadec-9-Enoate Isolated from Spondias Mombin Leaf. Food Chem. 116, 285–288. doi:10.1016/j.foodchem.2009.02.047
Gandhi, G. R., Vanlalhruaia, P., Stalin, A., Irudayaraj, S. S., Ignacimuthu, S., and Paulraj, M. G. (2014). Polyphenols-rich Cyamopsis Tetragonoloba (L.) Taub. Beans Show Hypoglycemic and β-cells Protective Effects in Type 2 Diabetic Rats. Food Chem. Toxicol. 66, 358–365. doi:10.1016/j.fct.2014.02.001
Ganesan, K., and Xu, B. (2017). Polyphenol-rich Dry Common Beans (Phaseolus vulgaris L.) and Their Health Benefits. Int. J. Mol. Sci. 18. doi:10.3390/ijms18112331
Ghorbani, A., and Esmaeilizadeh, M. (2017). Pharmacological Properties of Salvia Officinalis and its Components. J. Traditional Complement. Med. 7, 433–440. doi:10.1016/j.jtcme.2016.12.014
Glezeva, N., Chisale, M., Mcdonald, K., Ledwidge, M., Gallagher, J., and Watson, C. J. (2018). Diabetes and Complications of the Heart in Sub-saharan Africa: An Urgent Need for Improved Awareness, Diagnostics and Management. Diabetes Res. Clin. Pract. 137, 10–19. doi:10.1016/j.diabres.2017.12.019
Gothai, S., Ganesan, P., Park, S. Y., Fakurazi, S., Choi, D. K., and Arulselvan, P. (2016). Natural Phyto-Bioactive Compounds for the Treatment of Type 2 Diabetes: Inflammation as a Target. Nutrients 8. doi:10.3390/nu8080461
Guo, Y., Hou, E., Ma, N., Liu, Z., Fan, J., and Yang, R. (2020). Discovery, Biological Evaluation and Docking Studies of Novel N-Acyl-2-Aminothiazoles Fused (+)-nootkatone from Citrus Paradisi Macf. As Potential α-glucosidase Inhibitors. Bioorg. Chem. 104, 104294. doi:10.1016/j.bioorg.2020.104294
Gupta, R. C., Chang, D., Nammi, S., Bensoussan, A., Bilinski, K., and Roufogalis, B. D. (2017). Interactions between Antidiabetic Drugs and Herbs: an Overview of Mechanisms of Action and Clinical Implications. Diabetol. Metab. Syndr. 9, 59. doi:10.1186/s13098-017-0254-9
Guzman, J. D. (2014). Natural Cinnamic Acids, Synthetic Derivatives and Hybrids with Antimicrobial Activity. Molecules 19, 19292–19349. doi:10.3390/molecules191219292
Hadi, A., Arab, A., Hajianfar, H., Talaei, B., Miraghajani, M., Babajafari, S., et al. (2020). The Effect of Fenugreek Seed Supplementation on Serum Irisin Levels, Blood Pressure, and Liver and Kidney Function in Patients with Type 2 Diabetes Mellitus: A Parallel Randomized Clinical Trial. Complement. Ther. Med. 49, 102315. doi:10.1016/j.ctim.2020.102315
Hafizur, R. M., Maryam, K., Hameed, A., zaheer, L., Bano, S., Sumbul, S., et al. (2018). Insulin Releasing Effect of Some Pure Compounds from Moringa Oleifera on Mice Islets. Med. Chem. Res. 27, 1408–1418. doi:10.1007/s00044-018-2157-1
Halpern, S. H., and Douglas, M. J. (2007). Appendix: Jadad Scale for Reporting Randomized Controlled Trials. Evid Based. Obstet. Anesth., 237–238. doi:10.1002/9780470988343.app1
Han, J.-H., Tuan, N. Q., Park, M.-H., Quan, K. T., Oh, J., Heo, K.-S., et al. (2018). Cucurbitane Triterpenoids from the Fruits of Momordica Charantia Improve Insulin Sensitivity and Glucose Homeostasis in Streptozotocin-Induced Diabetic Mice. Mol. Nutr. Food Res. 62, 1700769. doi:10.1002/mnfr.201700769
Harinantenaina, L., Tanaka, M., Takaoka, S., Oda, M., Mogami, O., Uchida, M., et al. (2006). Momordica Charantia Constituents and Antidiabetic Screening of the Isolated Major Compounds. Chem. Pharm. Bull. (Tokyo) 54, 1017–1021. doi:10.1248/cpb.54.1017
Hartling, L., Bond, K., Vandermeer, B., Seida, J., Dryden, D. M., and Rowe, B. H. (2011). Applying the Risk of Bias Tool in a Systematic Review of Combination Long-Acting Beta-Agonists and Inhaled Corticosteroids for Persistent Asthma. PLoS One 6, e17242. doi:10.1371/journal.pone.0017242
Hassanin, K. M. A., Mahmoud, M. O., Hassan, H. M., Abdel-Razik, A. H., Aziz, L. N., and Rateb, M. E. (2018). Balanites Aegyptiaca Ameliorates Insulin Secretion and Decreases Pancreatic Apoptosis in Diabetic Rats: Role of SAPK/JNK Pathway. Biomed. Pharmacother. 102, 1084–1091. doi:10.1016/j.biopha.2018.03.167
Hong, S. H., Heo, J. I., Kim, J. H., Kwon, S. O., Yeo, K. M., Bakowska-Barczak, A. M., et al. (2013). Antidiabetic and Beta Cell-protection Activities of Purple Corn Anthocyanins. Biomol. Ther. (Seoul) 21, 284–289. doi:10.4062/biomolther.2013.016
Hooijmans, C. R., Rovers, M. M., de Vries, R. B., Leenaars, M., Ritskes-hoitinga, M., and Langendam, M. W. (2014). SYRCLE's Risk of Bias Tool for Animal Studies. BMC Med. Res. Methodol. 14, 43. doi:10.1186/1471-2288-14-43
Huang, T. H., Peng, G., Kota, B. P., Li, G. Q., Yamahara, J., Roufogalis, B. D., et al. (2005). Anti-diabetic Action of Punica granatum Flower Extract: Activation of PPAR-Gamma and Identification of an Active Component. Toxicol. Appl. Pharmacol. 207, 160–169. doi:10.1016/j.taap.2004.12.009
Huang, Y. N., Zhao, D. D., Gao, B., Zhong, K., Zhu, R. X., Zhang, Y., et al. (2012). Anti-hyperglycemic Effect of Chebulagic Acid from the Fruits of Terminalia Chebula Retz. Int. J. Mol. Sci. 13, 6320–6333. doi:10.3390/ijms13056320
Ibitoye, O. B., Uwazie, J. N., and Ajiboye, T. O. (2017). Bioactivity-guided Isolation of Kaempferol as the Antidiabetic Principle from Cucumis Sativus L. Fruits. J. Food Biochem. 42, e12479. doi:10.1111/jfbc.12479
Inegbenebor, U., Ebomoyi, M. I., Onyia, K. A., Amadi, K., and Aigbiremolen, A. E. (2009). Effect of alligator Pepper (Zingiberaceae Aframomum Melegueta) on First Trimester Pregnancy in Sprague Dawley Rats. Niger. J. Physiol. Sci. 24, 161–164. doi:10.4314/njps.v24i2.52901
Jafarpour-Sadegh, F., Montazeri, V., Adili, A., Esfehani, A., Rashidi, M. R., and Pirouzpanah, S. (2017). Consumption of Fresh Yellow Onion Ameliorates Hyperglycemia and Insulin Resistance in Breast Cancer Patients during Doxorubicin-Based Chemotherapy: A Randomized Controlled Clinical Trial. Integr. Cancer Ther. 16, 276–289. doi:10.1177/1534735416656915
Jain, V., Viswanatha, G. L., Manohar, D., and Shivaprasad, H. N. (2012). Isolation of Antidiabetic Principle from Fruit Rinds of Punica granatum. Evidence-Based Complement. Altern. Med. 2012, 1–11. doi:10.1155/2012/147202
Jalil, A., Ashfaq, U. A., Shahzadi, S., Rasul, I., Rehman, S. U., Shah, M., et al. (2013). Screening and Design of Anti-diabetic Compounds Sourced from the Leaves of Neem (Azadirachta indica). Bioinformation 9, 1031–1035. doi:10.6026/97320630091031
Jawla, S., Kumar, Y., and Khan, M. (2013). Isolation of Antidiabetic Principle from Bougainvillea Spectabilis Willd (Nyctaginaceae) Stem Bark. Trop. J. Pharm. Res. 12, 761–765. doi:10.4314/tjpr.v12i5.15
Jia, S., Hu, Y., Zhang, W., Zhao, X., Chen, Y., Sun, C., et al. (2015). Hypoglycemic and Hypolipidemic Effects of Neohesperidin Derived from Citrus Aurantium L. In Diabetic KK-A(y) Mice. Food Funct. 6, 878–886. doi:10.1039/C4FO00993B
Johnson, O. O., Zhao, M., Gunn, J., Santarsiero, B. D., Yin, Z.-Q., Ayoola, G. A., et al. (2016). α-Glucosidase Inhibitory Prenylated Anthranols from Harungana Madagascariensis. J. Nat. Prod. 79, 224–229. doi:10.1021/acs.jnatprod.5b00924
Jong-anurakkun, N., Bhandari, M. R., Hong, G., and Kawabata, J. (2008). Alpha-glucosidase Inhibitor from Chinese Aloes. Fitoterapia 79, 456–457. doi:10.1016/j.fitote.2008.02.010
Kadan, S., Saad, B., Sasson, Y., and Zaid, H. (2013). In VitroEvaluations of Cytotoxicity of Eight Antidiabetic Medicinal Plants and Their Effect on GLUT4 Translocation. Evidence-Based Complement. Altern. Med. 2013, 1–9. doi:10.1155/2013/549345
Kalman, D. S., Schwartz, H. I., Feldman, S., and Krieger, D. R. (2013). Efficacy and Safety of Elaeis Guineensis and Ficus Deltoidea Leaf Extracts in Adults with Pre-diabetes. Nutr. J. 12, 36. doi:10.1186/1475-2891-12-36
Kappel, V. D., Frederico, M. J., Postal, B. G., Mendes, C. P., Cazarolli, L. H., and Silva, F. R. (2013). The Role of Calcium in Intracellular Pathways of Rutin in Rat Pancreatic Islets: Potential Insulin Secretagogue Effect. Eur. J. Pharmacol. 702, 264–268. doi:10.1016/j.ejphar.2013.01.055
Karhagomba, I. B., Mirindi T, A., Mushagalusa, T. B., Nabino, V. B., Koh, K., and Kim, H. S. (2013). The Cultivation of Wild Food and Medicinal Plants for Improving Community Livelihood: The Case of the Buhozi Site, DR Congo. Nutr. Res. Pract. 7, 510–518. doi:10.4162/nrp.2013.7.6.510
Kasali, M. F., Mahano, A. O., Bwironde, F. M., Amani, A. C., Mangambu, J. D., Nyakabwa, D. S., et al. (2013). Ethnopharmacological Survey of Plants Used against Diabetes in Bukavu City (D.R. Congo). J. Ethnobiol. Tradit. Med. 119, 538–546.
Kasangana, P. B., Nachar, A., Eid, H. M., Stevanovic, T., and Haddad, P. S. (2018). Root Bark Extracts of Myrianthus Arboreus P. Beauv. (Cecropiaceae) Exhibit Anti-diabetic Potential by Modulating Hepatocyte Glucose Homeostasis. J. Ethnopharmacol. 211, 117–125. doi:10.1016/j.jep.2017.09.017
Kasika, E. L., Vasombolwa, V. K., and Lejoly, J. (2015). Contribution to the Knowledge of Plants Used by Bantu and Pygmy Healers in Beni and Lubero Territories (Democratic Republic of Congo). Jps 4, 157–176. doi:10.5539/jps.v4n2p157
Katemo, F. M., Kadima, J. N., and Marini, R. D. (2018). Determination of In Vitro Antioxidant Activity, Acute Toxicity in guinea-pig and TLC-Fingerprint of Panda Oleosa (Pierre) Bark Extract Used to Treat Diabetes in Folk Medicine. Int. J. Pharm. Pharm. Res. 13, 38–50.
Katemo, M., Mpiana, P. T., Mbala, B. M., Mihigo, S. O., Ngbolua, K. N., Tshibangu, D. S., et al. (2012). Ethnopharmacological Survey of Plants Used against Diabetes in Kisangani City (DR Congo). J. Ethnopharmacol. 144, 39–43. doi:10.1016/j.jep.2012.08.022
Kazmi, I., Rahman, M., Afzal, M., Gupta, G., Saleem, S., Afzal, O., et al. (2012). Anti-diabetic Potential of Ursolic Acid Stearoyl Glucoside: A New Triterpenic Gycosidic Ester from Lantana Camara. Fitoterapia 83, 142–146. doi:10.1016/j.fitote.2011.10.004
Keller, A. C., Ma, J., Kavalier, A., He, K., Brillantes, A. M., and Kennelly, E. J. (2011). Saponins from the Traditional Medicinal Plant Momordica Charantia Stimulate Insulin Secretion In Vitro. Phytomedicine 19, 32–37. doi:10.1016/j.phymed.2011.06.019
Kevin, L., Hussin, A., Zhari, I., and Chin, J. (2012). Sub-acute Oral Toxicity Study of Methanol Leaves Extract of Catharanthus Roseus in Rats. J. Acute Dis. 1, 38–41. doi:10.1016/s2221-6189(13)60009-8
Khan, A., Zaman, G., and Anderson, R. A. (2009). Bay Leaves Improve Glucose and Lipid Profile of People with Type 2 Diabetes. J. Clin. Biochem. Nutr. 44, 52–56. doi:10.3164/jcbn.08-188
Kianbakht, S., and Dabaghian, F. H. (2013). Improved Glycemic Control and Lipid Profile in Hyperlipidemic Type 2 Diabetic Patients Consuming Salvia Officinalis L. Leaf Extract: A Randomized Placebo. Controlled Clinical Trial. Complement. Ther. Med. 21, 441–446. doi:10.1016/j.ctim.2013.07.004
Kianbakht, S., Khalighi-Sigaroodi, F., and Dabaghian, F. H. (2013). Improved Glycemic Control in Patients with Advanced Type 2 Diabetes Mellitus Taking Urtica Dioica Leaf Extract: A Randomized Double-Blind Placebo-Controlled Clinical Trial. Clin. Lab. 59, 1071–1076. doi:10.7754/Clin.Lab.2012.121019
Kim, M.-H., Jo, S.-H., Jang, H.-D., Lee, M. S., and Kwon, Y.-I. (2010). Antioxidant Activity and α-glucosidase Inhibitory Potential of Onion (Allium cepa L.) Extracts. Food Sci. Biotechnol. 19, 159–164. doi:10.1007/s10068-010-0022-1
Kim, S. K., Jung, J., Jung, J. H., Yoon, N., Kang, S. S., Roh, G. S., et al. (2020). Hypoglycemic Efficacy and Safety of Momordica Charantia (Bitter Melon) in Patients with Type 2 Diabetes Mellitus. Complement. Ther. Med. 52, 102524. doi:10.1016/j.ctim.2020.102524
Kim, T. H., Kim, J. K., Kang, Y.-H., Lee, J.-Y., Kang, I. J., and Lim, S. S. (2013). Aldose Reductase Inhibitory Activity of Compounds from Zea maysL. Biomed. Res. Int. 2013, 1–8. doi:10.1155/2013/727143
Kodera, Y., Ushijima, M., Amano, H., Suzuki, J. I., and Matsutomo, T. (2017). Chemical and Biological Properties of S-1-Propenyl-L-Cysteine in Aged Garlic Extract. Molecules 22. doi:10.3390/molecules22040570
Kuete, V. (2014). “Health Effects of Alkaloids from African Medicinal Plants,” in Toxicological Survey of African Medicinal Plants. Editor V. Kuete (Elsevier), 611–633. doi:10.1016/B978-0-12-800018-2.00021-2
Kulkarni, P., Lohidasan, S., and Mahadik, K. (2021). Isolation, Characterisation and Investigation of In Vitro Antidiabetic and Antioxidant Activity of Phytoconstituents from Fruit of Momordica Charantia Linn. Nat. Product. Res. 35, 1035–1037. doi:10.1080/14786419.2019.1613400
Latham, P., and Mbuta, K. ku. A. (2017). Useful Plants of Kongo central Province,. Third edition. Democratic Republic of Congo.
Lawal, A., and Dangoggo, S. (2014). Phytochemical, Proximate and Toxicity Studies of Aqueous Extract of Crinum Ornatum (Toad’ S Onion). Chemsearch J. 5, 45–50.
Le, L. (2014). Computational Study of Antidiabetic Activities of Bioactive Compounds in Zingiber Officinale. World J. Pharm. Pharm. Sci. 3, 1995–2011.
Lee, D. Y., Kim, H. W., Yang, H., and Sung, S. H. (2017). Hydrolyzable Tannins from the Fruits of Terminalia Chebula Retz and Their α-glucosidase Inhibitory Activities. Phytochemistry 137, 109–116. doi:10.1016/j.phytochem.2017.02.006
Lee, Y. H., Yoon, S. Y., Baek, J., Kim, S. J., Yu, J. S., Kang, H., et al. (2021). Metabolite Profile of Cucurbitane-type Triterpenoids of Bitter Melon (Fruit of Momordica Charantia) and Their Inhibitory Activity against Protein Tyrosine Phosphatases Relevant to Insulin Resistance. J. Agric. Food Chem. 69, 1816–1830. doi:10.1021/acs.jafc.0c06085
Lenzen, S. (2008). The Mechanisms of Alloxan- and Streptozotocin-Induced Diabetes. Diabetologia 51, 216–226. doi:10.1007/s00125-007-0886-7
Leone, A., Bertoli, S., Di Lello, S., Bassoli, A., Ravasenghi, S., Borgonovo, G., et al. (2018). Effect of Moringa Oleifera Leaf Powder on Postprandial Blood Glucose Response: In Vivo Study on Saharawi People Living in Refugee Camps. Nutrients 10, 1494. doi:10.3390/nu10101494
Li, J.-M., Wang, W., Fan, C.-Y., Wang, M.-X., Zhang, X., Hu, Q.-H., et al. (2013). Quercetin Preservesβ-Cell Mass and Function in Fructose-Induced Hyperinsulinemia through Modulating Pancreatic Akt/FoxO1 Activation. Evidence-Based Complement. Altern. Med. 2013, 1–12. doi:10.1155/2013/303902
Li, X., Huang, G., Zhao, G., Chen, W., Li, J., and Sun, L. (2013). Two New Monoterpenes from Tithonia Diversifolia and Their Anti-hyperglycemic Activity. Fitoterapia 83. doi:10.1016/j.fitote.2012.09.007
Liu, C. T., Sheen, L. Y., and Lii, C. K. (2007). Does Garlic Have a Role as an Antidiabetic Agent? Mol. Nutr. Food Res. 51, 1353–1364. doi:10.1002/mnfr.200700082
Liu, J., Zhao, Y., Wu, Q., John, A., Jiang, Y., Yang, J., et al. (2018). Structure Characterisation of Polysaccharides in Vegetable “Okra” and Evaluation of Hypoglycemic Activity. Food Chem. 242, 211–216. doi:10.1016/j.foodchem.2017.09.051
Liu, X., Luo, J., and Kong, L. (2011). Phenylethyl Cinnamides as Potential Alpha-Glucosidase Inhibitors from the Roots of Solanum Melongena. Nat. Prod. Commun. 6, 851–853. doi:10.1177/1934578X1100600623
Lv, X., Zhao, S., Ning, Z., Zeng, H., Shu, Y., Tao, O., et al. (2015). Citrus Fruits as a Treasure Trove of Active Natural Metabolites that Potentially Provide Benefits for Human Health. Chem. Cent. J. 9, 68. doi:10.1186/s13065-015-0145-9
Lynch, B., Simon, R., and Roberts, A. (2011). In Vitro and In Vivo Assessment of the Genotoxic Activity of Aloesin. Regul. Toxicol. Pharmacol. 61, 215–221. doi:10.1016/j.yrtph.2011.07.011
MacIel, M. I., de Mendonça Cavalcanti, Mdo. S., Napoleão, T. H., Paiva, P. M., De Almeida Catanho, M. T., and Coelho, L. C. (2012). Anacardium Occidentale Bark Lectin: Purification, Immobilization as an Affinity Model and Influence in the Uptake of technetium-99M by Rat Adipocytes. Appl. Biochem. Biotechnol. 168, 580–591. doi:10.1007/s12010-012-9798-1
Mahomoodally, M. F., Mootoosamy, A., and Wambugu, S. (2016). Traditional Therapies Used to Manage Diabetes and Related Complications in Mauritius: A Comparative Ethnoreligious Study. Evidence-Based Complement. Altern. Med. 2016, 1–25. doi:10.1155/2016/4523828
Mahomoodally, M. F. (2013). Traditional Medicines in Africa: An Appraisal of Ten Potent African Medicinal Plants. Evidence-Based Complement. Altern. Med. 2013, 1–14. doi:10.1155/2013/617459
Manya, M. H., Keymeulen, F., Ngezahayo, J., Bakari, A. S., Kalonda, M. E., Kahumba, B. J., et al. (2020). Antimalarial Herbal Remedies of Bukavu and Uvira Areas in DR Congo: An Ethnobotanical Survey. J. Ethnopharmacol. 249, 112422. doi:10.1016/j.jep.2019.112422
Manzo, M. L. (2012). Etude phytochimique et pharmacologique de plantes antipaludiques utilisées en médecine traditionnelle congolaise. Liège: Université de Liège.
Mbayo, K. M., Kalonda, M. E., Tshisand, T. P., Kisimba, K. E., Mulamba, M., Richard, M. K., et al. (2016). Contribution to Ethnobotanical Knowledge of Some Euphorbiaceae Used in Traditional Medicine in Lubumbashi and its Surroundings (DRC). J. Adv. Bot. Zool. 4. doi:10.15297/JALS.V4I2.01
Mbuyi, K. S., Kalunga, M. R., Kalonda, M. E., Cimanga, C. C., Numbi, W. I. E., Kahumba, B. J., et al. (2019). Aperçu ethnobotanique de plantes réputées antipaludéennes utilisées dans la ville de Lubumbashi et ses environs, dans le Haut-Katanga en RD Congo. Ethnopharmacologia 61, 75–84.
Mikaili, P., Maadirad, S., Moloudizargari, M., Aghajanshakeri, S., and Sarahroodi, S. (2013). Therapeutic Uses and Pharmacological Properties of Garlic, Shallot, and Their Biologically Active Compounds. Iran. J. Basic Med. Sci. 16, 1031–1048.
Misawa, E., Tanaka, M., Nomaguchi, K., Nabeshima, K., Yamada, M., Toida, T., et al. (2012). Oral Ingestion of Aloe Vera Phytosterols Alters Hepatic Gene Expression Profiles and Ameliorates Obesity-Associated Metabolic Disorders in Zucker Diabetic Fatty Rats. J. Agric. Food Chem. 60, 2799–2806. doi:10.1021/jf204465j
Mishra, A., Srivastava, R., Srivastava, S. P., Gautam, S., Tamrakar, A. K., Maurya, R., et al. (2013). Antidiabetic Activity of Heart wood of Pterocarpus Marsupium Roxb. And Analysis of Phytoconstituents. Indian J. Exp. Biol. 51, 363–374.
Mohamed, G. A. (2008). Alliuocide G, a New Flavonoid with Potent α-amylase Inhibitory Activity from Allium cepa L. Arkivoc 2008, 202–209. doi:10.3998/ark.5550190.0009.b20
Mohammed, A., Gbonjubola, V. A., Koorbanally, N. A., and Islam, M. S. (2017). Inhibition of Key Enzymes Linked to Type 2 Diabetes by Compounds Isolated from Aframomum Melegueta Fruit. Pharm. Biol. 55, 1010–1016. doi:10.1080/13880209.2017.1286358
Mohammed, A., Victoria Awolola, G., Ibrahim, M. A., Anthony Koorbanally, N., and Islam, M. S. (2021). Oleanolic Acid as a Potential Antidiabetic Component of Xylopia Aethiopica (Dunal) A. Rich. (Annonaceae) Fruit: Bioassay Guided Isolation and Molecular Docking Studies. Nat. Prod. Res. 35, 788–791. doi:10.1080/14786419.2019.1596094
Mohan, C. G., Viswanatha, G. L., Savinay, G., Rajendra, C. E., and Halemani, P. D. (2013). 1,2,3,4,6 Penta-O-Galloyl-β-D-Glucose, a Bioactivity Guided Isolated Compound from Mangifera Indica Inhibits 11β-HSD-1 and Ameliorates High Fat Diet-Induced Diabetes in C57BL/6 Mice. Phytomedicine 20, 417–426. doi:10.1016/j.phymed.2012.12.020
Mongeke, M. M., Ngbolua, K., Bakola, R. D., Inkoto, C. L., Elikanpani, P. N., and Mouli, C. (2018). Enquête sur les plantes utilisées en médecine traditionnelle par les Bambenga: pygmées du secteur de Dongo en République Démocratique du Congo. Rev. Mar. Sci. Agron. Vét 6, 469–475.
Moshi, M. J., Otieno, D. F., Weisheit, A., Almeida, C., Amorim, E., Albuquerque, U., et al. (2012). Ethnomedicine of the Kagera Region, North Western Tanzania. Part 3: Plants Used in Traditional Medicine in Kikuku Village, Muleba District. J. Ethnobiol. Ethnomed. 8, 14. doi:10.1186/1746-4269-8-14
Moswa, J. L., Ciamala, C., Bongombola, B., Nzingula, N., Kapanda, N., Bokatshinde, N., et al. (2005). Plants Used for the Treatment of Diabetes Mellitus in the Democratic Republic of Congo. Ann. Pharm. 3, 87–93.
Mpiana, P., Masunda, T. A., Longoma, B. F., Tshibangu, D. S. T., and Ngbolua, K. N. (2013). Anti-hyperglycemic Activity of Raphia Gentiliana De Wild. (Arecaceae). Ejmp 3, 233–240. doi:10.9734/ejmp/2013/2459
Mpiana, P. T., Mbula, J. P., Kwembe, J. T. K., Tshilanda, D. D., Asimonio, J., Toegaho, A., et al. (2015). Ethnobotanical Survey of Aromatic Plants of Masako forest reserve (Kisangani, DR Congo). J. Adv. Bot. Zool. 2. doi:10.15297/JABZ.V2I3.04
Muhoya, F. K., Kadima, J. N., Ranarivelo, N., Frédérich, M., Hubert, P., and Djang’eing’a, R. M. (2017). Preliminary Phytochemical Content and Antidiabetic Potential Investigations of Panda Oleosa (Pierre) Used in Kisangani Areas. Ajac 08, 564–581. doi:10.4236/ajac.2017.89041
Munhoz, A. C. M., and Fröde, T. S. (2017). Isolated Compounds from Natural Products with Potential Antidiabetic Activity - A Systematic Review. Cdr 14, 36–106. doi:10.2174/1573399813666170505120621
Mushagalusa Kasali, F., Ahadi Irenge, C., Murhula Hamuli, P., Birindwa Mulashe, P., Murhula Katabana, D., Mangambu Mokoso, J. D. D., et al. (2021). Ethnopharmacological Survey on Treatment of Hypertension by Traditional Healers in Bukavu City, DR Congo. Evidence-Based Complement. Altern. Med. 2021, 1–10. doi:10.1155/2021/6684855
Mustafa, N. R., and Verpoorte, R. (2007). Phenolic Compounds in Catharanthus Roseus. Phytochem. Rev. 6, 243–258. doi:10.1007/s11101-006-9039-8
Muya, K., Tshoto, K., Cioci, C. C., Aseho, M. M., Kalonji, M., Byanga, K., et al. (2014). Survol ethnobotanique de quelques plantes utilisées contre la schistosomiase urogénitale à Lubumbashi et environs. Phytothérapie 12, 213–228. doi:10.1007/s10298-014-0877-z
Naicker, N., Nagiah, S., Phulukdaree, A., and Chuturgoon, A. (2016). Trigonella Foenum-graecum Seed Extract, 4-hydroxyisoleucine, and Metformin Stimulate Proximal Insulin Signaling and Increase Expression of Glycogenic Enzymes and GLUT2 in HepG2 Cells. Metab. Syndr. Relat. Disord. 14, 114–120. doi:10.1089/met.2015.0081
Nasser Singab, A., Youssef, F. S., and Mohamed, L. A. (2014). Medicinal Plants with Potential Antidiabetic Activity and Their Assessment. Med. Aromat. Plants 03, 1–12. doi:10.4172/2167-0412.1000151
Ndinteh, D. T. (2018). Antidiabetic Potential of Erythrina Abyssinica via Protein Tyrosine Phosphate 1B Inhibitory Activity. Emerg. Trends Chem. Sci., 377–389. doi:10.1007/978-3-319-60408-4_22
Ngbolua, K., Inkoto, C., Mongo, N., Ashande, M. C., Masens, Y., and Mpiana, P. T. (2019). Étude ethnobotanique et floristique de quelques plantes médicinales commercialisées à Kinshasa, République Démocratique du Congo. Rev. Marocaine Des Sci. Agron. Vétérinaires 7, 118–128.
Ngbolua, K., Mandjo, B. L., Munsebi, J. M., Ashande, M. C., Moke, L. E., Asamboa, L. S., et al. (2016a). Etudes ethnobotanique et écologique des plantes utilisées en médecine traditionnelle dans le District de la Lukunga à Kinshasa (RD du Congo) [ Ethno-botanical and ecological studies of medicinal plant species traditionally used in Lukunga district , Kinsh. Int. J. Innov. Sci. Res. 26, 612–633.
Ngbolua, K., Mihigo, S. O., Liyongo, C. I., Masengo, C., Tshibangu, D. S. T., Zoawe, B. G., et al. (2016b). Ethno-botanical Survey of Plant Species Used in Traditional Medicine in Kinshasa City (Democratic Republic of the Congo). Trop. Plant Res. 3, 413–427.
Nguyen, P. H., Nguyen, T. N., Dao, T. T., Kang, H. W., Ndinteh, D. T., Mbafor, J. T., et al. (2010). AMP-activated Protein Kinase (AMPK) Activation by Benzofurans and Coumestans Isolated from Erythrina Abyssinica. J. Nat. Prod. 73, 598–602. doi:10.1021/np900745g
Nile, S. H., and Park, S. W. (2014). Antioxidant, α-glucosidase and Xanthine Oxidase Inhibitory Activity of Bioactive Compounds from maize (Zea mays L.). Chem. Biol. Drug Des. 83, 119–125. doi:10.1111/cbdd.12205
Nizamutdinova, I. T., Jin, Y. C., Chung, J. I., Shin, S. C., Lee, S. J., Seo, H. G., et al. (2009). The Anti-diabetic Effect of Anthocyanins in Streptozotocin-Induced Diabetic Rats through Glucose Transporter 4 Regulation and Prevention of Insulin Resistance and Pancreatic Apoptosis. Mol. Nutr. Food Res. 53, 1419–1429. doi:10.1002/mnfr.200800526
Nnamonu, L., Tor-Anyiin, T., Ugbenyo, N., and Anyam, J. (2016). Isolation and Characterization of α -Amyrin from Stem Bark of Ficus Exasperata (Vahl). Bji 16, 1–7. doi:10.9734/BJI/2016/29737
Noipha, K., and Ninla-aesong, P. (2018). Antidiabetic Activity of Zingiber Officinale Roscoe Rhizome Extract: An In Vitro Study. Hayati J. Biosci. 25, 160–168. doi:10.4308/hjb.25.4.160
Noor, A., Bansal, V. S., and Vijayalakshmi, M. A. (2013). Current Update on Anti-diabetic Biomolecules from Key Traditional Indian Medicinal Plants. Curr. Sci. 104, 721–727.
Noruddin, N. A. A., Hamzah, M. F., Rosman, Z., Salin, N. H., Shu-Chien, A. C., and Muhammad, T. S. T. (2021). Natural Compound 3β,7β,25-trihydroxycucurbita-5,23(E)-dien-19-al from Momordica Charantia Acts as PPARγ Ligand. Molecules 26. doi:10.3390/molecules26092682
Ochieng, C. O., Nyongesa, D. W., Yamo, K. O., Onyango, J. O., Langat, M. K., and Manguro, L. A. O. (2020). α-Amylase and α-glucosidase Inhibitors from Zanthoxylum Chalybeum Engl. Root Bark. Fitoterapia 146, 104719. doi:10.1016/j.fitote.2020.104719
Ogunlana, O. O., Ogunlana, O. E., Adeneye, A. A., Udo-Chijioke, O., Dare-Olipede, T., Olagunju, J. A., et al. (2013). Evaluation of the Toxicological Profile of the Leaves and Young Twigs of Caesalpinia Bonduc (Linn) Roxb. Afr. J. Tradit Complement. Altern. Med. 10, 504–512. doi:10.4314/ajtcam.v10i6.20
Okolie, U. V., Okeke, C. E., Oli, J. M., and Ehiemere, J. (2008). Hypoglycemic Indices of Vernonia Amygdalina on Postprandial Blood Glucose Concentration of Healthy Humans. Afr. J. Biotechnol. 7, 4581–4585. doi:10.5897/AJB08.903
Olennikov, D. N., Chirikova, N. K., Kashchenko, N. I., Nikolaev, V. M., Kim, S. W., and Vennos, C. (2018). Bioactive Phenolics of the Genus Artemisia (Asteraceae): HPLC-DAD-ESI-TQ-MS/MS Profile of the Siberian Species and Their Inhibitory Potential against α-Amylase and α-Glucosidase. Front. Pharmacol. 9, 756. doi:10.3389/fphar.2018.00756
Olennikov, D. N., and Kashchenko, N. I. (2014). Componential Profile and Amylase Inhibiting Activity of Phenolic Compounds fromCalendula officinalisL. Leaves. Scientific World J. 2014, 1–9. doi:10.1155/2014/654193
Osfor, M. M. H., Hegazy, A., Abd El-moaty, M., Elmadbouly, M., Afify, A., and Elbahnasawy, A. S. (2013). Hypo-cholesterolemic and Hypoglycemic Effects of orange Albedo Powder (Citrus Aurantium L.) on Male Albino Rats. Ijnfs 2, 70–76. doi:10.11648/j.ijnfs.20130202.17
Pahlavani, N., Roudi, F., Zakerian, M., Ferns, G. A., Navashenaq, J. G., Mashkouri, A., et al. (2019). Possible Molecular Mechanisms of Glucose-Lowering Activities of Momordica Charantia (Karela) in Diabetes. J. Cel. Biochem., 1–9. doi:10.1002/jcb.28483
Pan, J., Yi, X., Zhang, S., Cheng, J., Wang, Y., Liu, C., et al. (2018). Bioactive Phenolics from Mango Leaves (Mangifera Indica L.). Ind. Crops Prod. 111, 400–406. doi:10.1016/j.indcrop.2017.10.057
Park, S. H., Do, M. H., Lee, J. H., Jeong, M., Lim, O. K., and Kim, S. Y. (2017). Inhibitory Effect of Arachis hypogaea (Peanut) and its Phenolics against Methylglyoxal-Derived Advanced Glycation End Product Toxicity. Nutrients 9. doi:10.3390/nu9111214
Parveen, A., Z, Z., Farooqi, M. Q., Kyunn, W. W., Arshad, M., and Farooqi, Q. (2017). Phytochemical Screening and Content Determination of Different Species of Genus Caesalpinia Belonging to Different Origin with Antidiabetic Activity. Pj 9, 743–749. doi:10.5530/pj.2017.6.117
Patel, D. K., Prasad, S. K., Kumar, R., and Hemalatha, S. (2012). An Overview on Antidiabetic Medicinal Plants Having Insulin Mimetic Property. Asian Pac. J. Trop. Biomed. 2, 320–330. doi:10.1016/S2221-1691(12)60032-X
Pathy, K. K., Flavien, N. B., Honoré, B. K., Vanhove, W., and Van Damme, P. (2021). Correction to: Ethnobotanical Characterization of Medicinal Plants Used in Kisantu and Mbanza-Ngungu Territories, Kongo-Central Province in DR Congo. J. Ethnobiol. Ethnomed. 17, 8. doi:10.1186/s13002-021-00436-1
Paula, P. C., Sousa, D. O., Oliveira, J. T., Carvalho, A. F., Alves, B. G., Pereira, M. L., et al. (2017). A Protein Isolate from Moringa Oleifera Leaves Has Hypoglycemic and Antioxidant Effects in Alloxan-Induced Diabetic Mice. Molecules 22. doi:10.3390/molecules22020271
Perera, P. R. D., Ekanayake, S., and Ranaweera, K. K. D. S. (2017). Antidiabetic Compounds in Syzygium Cumini Decoction and Ready to Serve Herbal Drink. Evidence-Based Complement. Altern. Med. 2017, 1–5. doi:10.1155/2017/1083589
Perera, W. H., Shivanagoudra, S. R., Pérez, J. L., Kim, D. M., Sun, Y., K. Jayaprakasha, G., et al. (2021). Anti-inflammatory, Antidiabetic Properties and In Silico Modeling of Cucurbitane-type Triterpene Glycosides from Fruits of an Indian Cultivar of Momordica Charantia L. Molecules 26, 1038. doi:10.3390/molecules26041038
Pingali, U., Sukumaran, D., and Nutalapati, C. (2020). Effect of an Aqueous Extract of Terminalia Chebula on Endothelial Dysfunction, Systemic Inflammation, and Lipid Profile in Type 2 Diabetes Mellitus: A Randomized Double-Blind, Placebo-Controlled Clinical Study. Phytother Res. 34, 3226–3235. doi:10.1002/ptr.6771
Pu, P., Gao, D. M., Mohamed, S., Chen, J., Zhang, J., Zhou, X. Y., et al. (2012). Naringin Ameliorates Metabolic Syndrome by Activating AMP-Activated Protein Kinase in Mice Fed a High-Fat Diet. Arch. Biochem. Biophys. 518, 61–70. doi:10.1016/j.abb.2011.11.026
Puri, D., Prabhu, K. M., Dev, G., Agarwal, S., and Murthy, P. S. (2011). Mechanism of Antidiabetic Action of Compound GII Purified from Fenugreek (Trigonella Foenum Graecum) Seeds. Indian J. Clin. Biochem. 26, 335–346. doi:10.1007/s12291-011-0150-2
Raffaelli, F., Nanetti, L., Montecchiani, G., Borroni, F., Salvolini, E., Faloia, E., et al. (2015). In Vitro effects of Fermented Papaya (Carica Papaya, L.) on Platelets Obtained from Patients with Type 2 Diabetes. Nutr. Metab. Cardiovasc. Dis. 25, 224–229. doi:10.1016/j.numecd.2014.10.013
Raju, P., Mamidala, E., and Mamidala, E. (2015). Anti Diabetic Activity of Compound Isolated from Physalis Angulata Fruit Extracts in Alloxan Induced Diabetic Rats. Ajsmr 1, 40–43. doi:10.17812/ajsmr2015111
Randel, R. D., Chase, C. C., and Wyse, S. J. (1992). Effects of Gossypol and Cottonseed Products on Reproduction of Mammals. J. Anim. Sci. 70, 1628–1638. doi:10.2527/1992.7051628x
Rangari, V. D., Shukla, P., and Badole, S. L. (2015). “4-Hydroxyisoleucine: A Potential Antidiabetic Agent from Trigonella Foenum-Graecum,” in Glucose Intake and Utilization in Pre-diabetes and Diabetes (Elsevier), 191–198. doi:10.1016/b978-0-12-800093-9.00015-6
Rashad, H., Metwally, F. M., Ezzat, S. M., Salama, M. M., Hasheesh, A., and Abdel Motaal, A. (2017). Randomized Double-Blinded Pilot Clinical Study of the Antidiabetic Activity of Balanites Aegyptiaca and UPLC-ESI-MS/MS Identification of its Metabolites. Pharm. Biol. 55, 1954–1961. doi:10.1080/13880209.2017.1354388
Ríos, J. L., Francini, F., and Schinella, G. R. (2015). Natural Products for the Treatment of Type 2 Diabetes Mellitus. Planta Med. 81, 975–994. doi:10.1055/s-0035-1546131
Salah El Dine, R., Ma, Q., Kandil, Z. A., and El-Halawany, A. M. (2014). Triterpenes as Uncompetitive Inhibitors of α-glucosidase from Flowers of Punica granatum L. Nat. Product. Res. 28, 2191–2194. doi:10.1080/14786419.2014.928292
Salehi, B., Albayrak, S., Antolak, H., Kręgiel, D., Pawlikowska, E., Sharifi-Rad, M., et al. (2018). Aloe Genus Plants: From Farm to Food Applications and Phytopharmacotherapy. Int. J. Mol. Sci. 19. doi:10.3390/ijms19092843
Sands, A. L., Leidy, H. J., Hamaker, B. R., Maguire, P., and Campbell, W. W. (2009). Consumption of the Slow-Digesting Waxy maize Starch Leads to Blunted Plasma Glucose and Insulin Response but Does Not Influence Energy Expenditure or Appetite in Humans. Nutr. Res. 29, 383–390. doi:10.1016/j.nutres.2009.05.009
Sanni, O., Erukainure, O. L., Chukwuma, C. I., Koorbanally, N. A., Ibeji, C. U., and Islam, M. S. (2019). Azadirachta indica Inhibits Key Enzyme Linked to Type 2 Diabetes In Vitro, Abates Oxidative Hepatic Injury and Enhances Muscle Glucose Uptake Ex Vivo. Biomed. Pharmacother. 109, 734–743. doi:10.1016/j.biopha.2018.10.171
Sato, H., Genet, C., Strehle, A., Thomas, C., Lobstein, A., Wagner, A., et al. (2007). Anti-hyperglycemic Activity of a TGR5 Agonist Isolated from Olea Europaea. Biochem. Biophys. Res. Commun. 362, 793–798. doi:10.1016/j.bbrc.2007.06.130
Sekiya, K., Ohtani, A., and Kusano, S. (2004). Enhancement of Insulin Sensitivity in Adipocytes by Ginger. BioFactors 22, 153–156. doi:10.1002/biof.5520220130
Sharma, A. K., Bharti, S., Kumar, R., Krishnamurthy, B., Bhatia, J., Kumari, S., et al. (2012). Syzygium Cumini Ameliorates Insulin Resistance and β-cell Dysfunction via Modulation of PPAR, Dyslipidemia, Oxidative Stress, and TNF-α in Type 2 Diabetic Rats. J. Pharmacol. Sci. 119, 205–213. doi:10.1254/jphs.11184FP
Shidfar, F., Rajab, A., Rahideh, T., Khandouzi, N., Hosseini, S., and Shidfar, S. (2015). The Effect of Ginger (Zingiber Officinale) on Glycemic Markers in Patients with Type 2 Diabetes. J. Complement. Integr. Med. 12, 165–170. doi:10.1515/jcim-2014-0021
Shinde, J., Taldone, T., Barletta, M., Kunaparaju, N., Hu, B., Kumar, S., et al. (2008). Alpha-glucosidase Inhibitory Activity of Syzygium Cumini (Linn.) Skeels Seed Kernel In Vitro and in Goto-Kakizaki (GK) Rats. Carbohydr. Res. 343, 1278–1281. doi:10.1016/j.carres.2008.03.003
Shivanagoudra, S. R., Perera, W. H., Perez, J. L., Athrey, G., Sun, Y., Jayaprakasha, G. K., et al. (2019). Cucurbitane-type Compounds from Momordica Charantia: Isolation, In Vitro Antidiabetic, Anti-inflammatory Activities and In Silico Modeling Approaches. Bioorg. Chem. 87, 31–42. doi:10.1016/j.bioorg.2019.02.040
Shyni, G. L., Kavitha, S., Indu, S., Arya, A. D., Anusree, S. S., Vineetha, V. P., et al. (2014). Chebulagic Acid from Terminalia Chebula Enhances Insulin Mediated Glucose Uptake in 3T3-L1 Adipocytes via PPARγ Signaling Pathway. BioFactors 40, 646–657. doi:10.1002/biof.1193
Silva, E. L., Almeida-Lafetá, R. C., Borges, R. M., and Staerk, D. (2017). Dual High-Resolution Inhibition Profiling and HPLC-HRMS-SPE-NMR Analysis for Identification of α-glucosidase and Radical Scavenging Inhibitors in Solanum Americanum Mill. Fitoterapia 118, 42–48. doi:10.1016/j.fitote.2017.02.002
Singh, A. B., Yadav, D. K., Maurya, R., and Srivastava, A. K. (2009). Antihyperglycaemic Activity of Alpha-Amyrin Acetate in Rats and Db/db Mice. Nat. Prod. Res. 23, 876–882. doi:10.1080/14786410802420416
Sugita, J., Yoneshiro, T., Hatano, T., Aita, S., Ikemoto, T., Uchiwa, H., et al. (2013). Grains of paradise (Aframomum Melegueta) Extract Activates Brown Adipose Tissue and Increases Whole-Body Energy Expenditure in Men. Br. J. Nutr. 110, 733–738. doi:10.1017/S0007114512005715
Sundaram Chinna Krishnan, S., Pillai Subramanian, I., and Pillai Subramanian, S. (2014). Isolation, Characterization of Syringin, Phenylpropanoid Glycoside from Musa Paradisiaca Tepal Extract and Evaluation of its Antidiabetic Effect in Streptozotocin-Induced Diabetic Rats. Biomed. Prev. Nutr. 4, 105–111. doi:10.1016/j.bionut.2013.12.009
Sundowo, A., Anita, Y., Maryani, F., Banjarnahor, S. D. S., and Widiyarti, G. (2018). Synthesis of Aniline Catalyzed by Brassica Juncea Peroxidase and its Antidiabetic Activities. AIP Conf. Proc. 2024. doi:10.1063/1.5064324
Suntar, I., Khan, H., Patel, S., Celano, R., and Rastrelli, L. (2018). An Overview onCitrus aurantiumL.: Its Functions as Food Ingredient and Therapeutic Agent. Oxidative Med. Cell Longevity 2018, 1–12. doi:10.1155/2018/7864269
Surya, S., Salam, A. D., Tomy, D. V., Carla, B., Kumar, R. A., and Sunil, C. (2014). Diabetes Mellitus and Medicinal Plants-A Review. Asian Pac. J. Trop. Dis. 4, 337–347. doi:10.1016/S2222-1808(14)60585-5
Sutedja, A. M., Yanase, E., Batubara, I., Fardiaz, D., and Lioe, H. N. (2020). Antidiabetic Components from the Hexane Extract of Red Kidney Beans (Phaseolus vulgaris L.): Isolation and Structure Determination. Biosci. Biotechnol. Biochem. 84, 598–605. doi:10.1080/09168451.2019.1691911
Tahora, S. B., Akhi, A. A., Karim, S. S., Hasan, R., Nabeela, S. A., and Ali, R. (2018). In Silico study of Some Isolated Compound Present in Ageratum Conyzoides against Diabetes. J. Nutr. Diet. Prat. 2, 1–4.
Tang, D., Liu, L., Ajiakber, D., Ye, J., Xu, J., Xin, X., et al. (2018). Anti-diabetic Effect of Punica granatum Flower Polyphenols Extract in Type 2 Diabetic Rats: Activation of Akt/GSK-3β and Inhibition of IRE1α-XBP1 Pathways. Front. Endocrinol. (Lausanne) 9, 586–611. doi:10.3389/fendo.2018.00586
Tang, G. Y., Li, X. J., and Zhang, H. Y. (2008). Antidiabetic Components Contained in Vegetables and Legumes. Molecules 13, 1189–1194. doi:10.3390/molecules13051189
Tardío, J., and Pardo-De-Santayana, M. (2008). Cultural Importance Indices: A Comparative Analysis Based on the Useful Wild Plants of Southern Cantabria (Northern Spain)1. Econ. Bot. 62, 24–39. doi:10.1007/s12231-007-9004-5
Tedong, L., Madiraju, P., Martineau, L. C., Vallerand, D., Arnason, J. T., Desire, D. D., et al. (2010). Hydro-ethanolic Extract of Cashew Tree (Anacardium Occidentale) Nut and its Principal Compound, Anacardic Acid, Stimulate Glucose Uptake in C2C12 Muscle Cells. Mol. Nutr. Food Res. 54, 1753–1762. doi:10.1002/mnfr.201000045
Teixeira Sousa Moura, L., Palomaris Mariano Souza, D., Mendonça, S., de Aquino Ribeiro, J. A., Fernandes Sousa, L., Tony Ramos, A., et al. (2017). Histopathological and Reproductive Evaluation in Male Rats Fed Jatropha Curcas Seed Cake with or without Alkaline Hydrolysis and Subjected to Heat Treatment. Biomed. Res. Int. 2017, 1–11. doi:10.1155/2017/6123408
Teodoro, T., Zhang, L., Alexander, T., Yue, J., Vranic, M., and Volchuk, A. (2008). Oleanolic Acid Enhances Insulin Secretion in Pancreatic Beta-Cells. FEBS Lett. 582, 1375–1380. doi:10.1016/j.febslet.2008.03.026
The World Medical Association, (2001). Declaration of Helsinki. Bull. World Health Organ. 79, 373–374.
Tiong, S. H., Looi, C. Y., Hazni, H., Arya, A., Paydar, M., Wong, W. F., et al. (2013). Antidiabetic and Antioxidant Properties of Alkaloids from Catharanthus Roseus (L.) G. Don. Molecules 18, 9770–9784. doi:10.3390/molecules18089770
Tousch, D., Lajoix, A. D., Hosy, E., Azay-milhau, J., Ferrare, K., Jahannault, C., et al. (2008). Chicoric Acid, a New Compound Able to Enhance Insulin Release and Glucose Uptake. Biochem. Biophys. Res. Commun. 377, 131–135. doi:10.1016/j.bbrc.2008.09.088
Tuwisana Masunda, A., Inkoto, C. L., Bongo, G. N., Wa Oloko, J. D., Ngbolua, K.-T.-N., Tshibangu, D. S., et al. (2019). Ethnobotanical and Ecological Studies of Plants Used in the Treatment of Diabetes in Kwango, Kongo central and Kinshasa in the Democratic Republic of the Congo. Ijde 4, 18. doi:10.11648/j.ijde.20190401.14
Uwazie, J. N., Yakubu, M. T., Ashafa, A. O. T., and Ajiboye, T. O. (2020). Identification and Characterization of Anti-diabetic Principle in Senna alata (Linn.) Flower Using Alloxan-Induced Diabetic Male Wistar Rats. J. Ethnopharmacol. 261, 112997. doi:10.1016/j.jep.2020.112997
V, B. C., S, B. A., Philippe, O. N., J, K. B., Pierre, D., and Jb, L. S. (2020). Ethnobotanical Study of Plants Used as Antimalarial in Traditional Medicine in Bagira in Eastern RD Congo. J. Pharmacogn. Phytochem. 9, 01–14. doi:10.22271/phyto.2020.v9.i4a.11661
Vadivel, V., Nandety, A., and Biesalski, H. K. (2011). Antioxidant, Free Radical Scavenging and Type II Diabetes-Related Enzyme Inhibition Properties of Traditionally Processed Jequirity Bean (Abrus precatorius L.). Int. J. Food Sci. Technol. 46, 2505–2512. doi:10.1111/j.1365-2621.2011.02774.x
Vu, N. K., Kim, C. S., Ha, M. T., Ngo, Q. T., Park, S. E., Kwon, H., et al. (2020). Antioxidant and Antidiabetic Activities of Flavonoid Derivatives from the Outer Skins of Allium cepa L. J. Agric. Food Chem. 68, 8797–8811. doi:10.1021/acs.jafc.0c02122
Wang, F., Zhong, H. H., Chen, W. K., Liu, Q. P., Li, C. Y., Zheng, Y. F., et al. (2017a). Potential Hypoglycaemic Activity Phenolic Glycosides from Moringa Oleifera Seeds. Nat. Prod. Res. 31, 1869–1874. doi:10.1080/14786419.2016.1263846
Wang, H., Du, Y.-J., and Song, H.-C. (2010). α-Glucosidase and α-amylase Inhibitory Activities of Guava Leaves. Food Chem. 123, 6–13. doi:10.1016/j.foodchem.2010.03.088
Wang, J., Huang, M., Yang, J., Ma, X., Zheng, S., Deng, S., et al. (2017b). Anti-diabetic Activity of Stigmasterol from Soybean Oil by Targeting the GLUT4 Glucose Transporter. Food Nutr. Res. 61, 1364117. doi:10.1080/16546628.2017.1364117
Wang, Q., Wu, X., Shi, F., and Liu, Y. (2019). Comparison of Antidiabetic Effects of Saponins and Polysaccharides from Momordica Charantia L. In STZ-Induced Type 2 Diabetic Mice. Biomed. Pharmacother. 109, 744–750. doi:10.1016/j.biopha.2018.09.098
Wang-fischer, Y., and Garyantes, T. (2018). Improving the Reliability and Utility of Streptozotocin-Induced Rat Diabetic Model. J. Diabetes Res. 2018, 1–14. doi:10.1155/2018/8054073
Waterman, C., Rojas-silva, P., Tumer, T. B., Kuhn, P., Richard, A. J., Wicks, S., et al. (2016). Isothiocyanate-rich Moringa Oleifera Extract Reduces Weight Gain, Insulin Resistance, and Hepatic Gluconeogenesis in Mice. Mol. Nutr. Food Res. 59, 1013–1024. doi:10.1002/mnfr.201400679.Isothiocyanate-rich
Wikul, A., Damsud, T., Kataoka, K., and Phuwapraisirisan, P. (2012). (+)-Pinoresinol Is a Putative Hypoglycemic Agent in Defatted Sesame (Sesamum indicum) Seeds Though Inhibiting α-glucosidase. Bioorg. Med. Chem. Lett. 22, 5215–5217. doi:10.1016/j.bmcl.2012.06.068
Worawalai, W., Khongchai, P., Surachaitanawat, N., and Phuwapraisirisan, P. (2016). Synthesis of Furofuran Lignans as Antidiabetic Agents Simultaneously Achieved by Inhibiting α-glucosidase and Free Radical. Arch. Pharm. Res. 39, 1370–1381. doi:10.1007/s12272-016-0778-9
Xu, X., Shan, B., Liao, C. H., Xie, J. H., Wen, P. W., and Shi, J. Y. (2015). Anti-diabetic Properties of Momordica Charantia L. Polysaccharide in Alloxan-Induced Diabetic Mice. Int. J. Biol. Macromol. 81, 538–543. doi:10.1016/j.ijbiomac.2015.08.049
Yagi, A., Hegazy, S., Kabbash, A., and Wahab, E. A. (2009). Possible Hypoglycemic Effect of Aloe Vera L. High Molecular Weight Fractions on Type 2 Diabetic Patients. Saudi Pharm. J. 17, 209–215. doi:10.1016/j.jsps.2009.08.007
Yang, D., Chen, X., Liu, X., Han, N., Liu, Z., Li, S., et al. (2020). Antioxidant and α-Glucosidase Inhibitory Activities Guided Isolation and Identification of Components from Mango Seed Kernel. Oxidative Med. Cell Longevity 2020, 1–15. doi:10.1155/2020/8858578
Yang, J., Wang, T., Yang, J., Rao, K., Zhan, Y., Chen, R. B., et al. (2013). S-allyl Cysteine Restores Erectile Function through Inhibition of Reactive Oxygen Species Generation in Diabetic Rats. Andrology 1, 487–494. doi:10.1111/j.2047-2927.2012.00060.x
Yonemoto, R., Shimada, M., Gunawan-Puteri, M. D., Kato, E., and Kawabata, J. (2014). α-Amylase Inhibitory Triterpene from Abrus precatorius Leaves. J. Agric. Food Chem. 62, 8411–8414. doi:10.1021/jf502667z
Yoon, K. D., Lee, J. Y., Kim, T. Y., Kang, H., Ha, K. S., Ham, T. H., et al. (2020). In Vitro and In Vivo Anti-hyperglycemic Activities of Taxifolin and its Derivatives Isolated from Pigmented Rice (Oryzae Sativa L. Cv. Superhongmi). J. Agric. Food Chem. 68, 742–750. doi:10.1021/acs.jafc.9b04962
Yue, J., Xu, J., Cao, J., Zhang, X., and Zhao, Y. (2017). Cucurbitane Triterpenoids from Momordica Charantia L. And Their Inhibitory Activity against α-glucosidase, α-amylase and Protein Tyrosine Phosphatase 1B (PTP1B). J. Funct. Foods 37, 624–631. doi:10.1016/j.jff.2017.07.041
Zameer, S., Najmi, A. K., Vohora, D., and Akhtar, M. (2017). A Review on Therapeutic Potentials of Trigonella Foenum Graecum (Fenugreek) and its Chemical Constituents in Neurological Disorders: Complementary Roles to its Hypolipidemic, Hypoglycemic, and Antioxidant Potential. Nutr. Neurosci. 21, 539–545. doi:10.1080/1028415X.2017.1327200
Zang, Y., Sato, H., and Igarashi, K. (2014). Anti-diabetic Effects of a Kaempferol Glycoside-Rich Fraction from Unripe Soybean (Edamame, Glycine max L. Merrill. 'Jindai') Leaves on KK-A(y) Mice. Biosci. Biotechnol. Biochem. 75, 1677–1684. doi:10.1271/bbb.110168
Zhang, C., Huang, M., Hong, R., and Chen, H. (2019). Preparation of a Momordica Charantia L. Polysaccharide-chromium (III) C-omplex and its A-nti-hyperglycemic A-ctivity in M-ice with S-treptozotocin-I-nduced D-iabetes. Int. J. Biol. Macromol. 122, 619–627. doi:10.1016/j.ijbiomac.2018.10.200
Zhang, H., Xu, J., Wang, M., Xia, X., Dai, R., and Zhao, Y. (2020). Steroidal Saponins and Sapogenins from Fenugreek and Their Inhibitory Activity against α-glucosidase. Steroids 161, 108690. doi:10.1016/j.steroids.2020.108690
Zhang, Z., Kong, F., Ni, H., Mo, Z., Wan, J. B., Hua, D., et al. (2016). Structural Characterization, α-glucosidase Inhibitory and DPPH Scavenging Activities of Polysaccharides from Guava. Carbohydr. Polym. 144, 106–114. doi:10.1016/j.carbpol.2016.02.030
Zhao, G., Li, X., Chen, W., Xi, Z., and Sun, L. (2012). Three New Sesquiterpenes from Tithonia Diversifolia and Their Anti-hyperglycemic Activity. Fitoterapia 83, 1590–1597. doi:10.1016/j.fitote.2012.09.007
Keywords: antidiabetic plants, ethnopharmacology, phytochemicals, bioactivity, Democratic Republic of Congo
Citation: Kasali FM, Kadima JN, Peter EL, Mtewa AG, Ajayi CO, Tusiimire J, Tolo CU, Ogwang PE, Weisheit A and Agaba AG (2021) Antidiabetic Medicinal Plants Used in Democratic Republic of Congo: A Critical Review of Ethnopharmacology and Bioactivity Data. Front. Pharmacol. 12:757090. doi: 10.3389/fphar.2021.757090
Received: 11 August 2021; Accepted: 08 October 2021;
Published: 27 October 2021.
Edited by:
Alessandra Durazzo, Council for Agricultural Research and Economics, ItalyReviewed by:
Annah Moteetee, University of Johannesburg, South AfricaRoodabeh Bahramsoltani, Tehran University of Medical Sciences, Iran
Da-Cheng Hao, Dalian Jiaotong University, China
Fikri-Benbrahim Kawtar, Sidi Mohamed Ben Abdellah University, Morocco
Copyright © 2021 Kasali, Kadima, Peter, Mtewa, Ajayi, Tusiimire, Tolo, Ogwang, Weisheit and Agaba. This is an open-access article distributed under the terms of the Creative Commons Attribution License (CC BY). The use, distribution or reproduction in other forums is permitted, provided the original author(s) and the copyright owner(s) are credited and that the original publication in this journal is cited, in accordance with accepted academic practice. No use, distribution or reproduction is permitted which does not comply with these terms.
*Correspondence: Félicien Mushagalusa Kasali, ZmVsaWNpZW5rYXNhbGlAZ21haWwuY29t