- 1Department of Neurology, The Third People’s Hospital of Dalian, Non-Directly Affiliated Hospital of Dalian Medical University, Dalian, China
- 2Department of Ophthalmology, The Third People’s Hospital of Dalian, Non-Directly Affiliated Hospital of Dalian Medical University, Dalian, China
- 3Department of Neurosurgery, The Third People’s Hospital of Dalian, Non-Directly Affiliated Hospital of Dalian Medical University, Dalian, China
Most diabetic patients develop diabetic peripheral neuropathy (DPN). DPN is related to the increase of inflammatory cells in peripheral nerves, abnormal cytokine expression, oxidative stress, ischemia ,and pro-inflammatory changes in bone marrow. We summarized the progress of immune-inflammatory mechanism and treatment of DPN in recent years. Immune inflammatory mechanisms include TNF-α, HSPs, PARP, other inflammatory factors, and the effect of immune cells on DPN. Treatment includes tricyclic antidepressants and other drug therapy, immune and molecular therapy, and non-drug therapy such as exercise therapy, electrotherapy, acupuncture, and moxibustion. The pathogenesis of DPN is complex. In addition to strictly controlling blood glucose, its treatment should also start from other ways, explore more effective and specific treatment schemes for various causes of DPN, and find new targets for treatment will be the direction of developing DPN therapeutic drugs in the future.
Introduction
The prevalence of diabetes mellitus (DM) increased with age. DM is present in 19.9% of 65–79-year-olds (Saeedi et al., 2019). Diabetic peripheral neuropathy (DPN) is one of the common chronic complication of diabetes mellitus. Its Clinical Syndrome can be dysfunction of peripheral neuropathy, influencing the quality of life in the dabetic patients. DPN is a length-dependent sensory axonal lesion that usually the first manifestation is the sensory disturbance, pain, numbness or loss of balance (Edwards et al., 2008). DPN leads to the decline of patient’s quality of life. Studies have shown that although blood glucose control can reduce the incidence of peripheral neuropathy in type 1 diabetes, it will also increase the incidence of hypoglycemia, and aggressive blood glucose control can not reduce the incidence of peripheral neuropathy in type 2 diabetes (Group 1993; Boussageon et al., 2011; Callaghan et al., 2012a). Moreover, although neuropathy associated with T1DM and T2DM has been classified into the same category, we know that the pathogenesis of these two diseases is very different (Callaghan et al., 2012b; Eid Sas et al., 2019). It is essential to study the pathogenesis of DPN. In the past, most studies on DPN were limited to metabolism, genetic mechanism, and the effect of hypoglycemic drugs (Ferland-Mccollough et al., 2016; Sztanek et al., 2016; De et al., 2017; Stino and Smith, 2017; Jamwal et al., 2018). Previous drug trials mainly focused on antioxidants (Ziegler et al., 1999; Ametov et al., 2003; Ziegler et al., 2006), aldose reductase inhibitors (Hotta et al., 2006; Bril et al., 2009; Ramirez and Borja, 2012), neurotrophic factors (Apfel 2002; Decroli et al., 2019), GABA analogs (Ermis et al., 2010), cell metabolic agonists (Evans et al., 2008; Dan, Movsesyan et al., 2009) and vasodilators (Yuen et al., 2002). These drugs have limited effect in the clinical treatment of DPN associated with T1DM and T2DM (Coert, J. et al., 2016). However, with the further study of DM, the mechanism of immune inflammation is related to the pathogenesis of DPN. This review mainly focuses on the immune-inflammatory mechanism of DPN. (Figure 1).
Immunoinflammatory Factors and Diabetic Peripheral Neuropathy
Tumor Necrosis Factor-α
Figure 2 Hyperglycemia has been shown to activate tumor necrosis factor-α (TNF-α) secretion from immune cells. TNF-α causes nerve demyelination and stimulates monocytes and endothelial cells to secrete inflammatory substances, further increase nerve damage. DPN patient’s serum tumor necrosis factor-α (TNF-α) level was significantly higher than that of patients without DPN and normal controls. The risk of DPN in diabetic patients with elevated TNF-α was 2.594 times that of regular TNF-α patients (Mu et al., 2017). The serum tumor TNF-α of DPN patients was higher than patients without DPN and normal controls (Duksal et al., 2016). The most conclusive finding is that streptozocin (STZ) induced diabetes TNF- α-/-mice will not develop DPN like diabetic mice (Yamakawa et al., 2011). Hussain et al. found that TNF-α was negatively correlated with nerve conduction velocity (Hussain et al., 2013). Shi et al. showed that compared with untreated DPN, recombinant human TNF-α receptor antibody fusion protein inhibited TNF-α in STZ induced diabetic rats, partially improving motor nerve conduction velocity (MCV) and sensory nerve conduction velocity (SCV), increasing the expression of myelin basic protein (MBP), and preventing myelin and neural structural abnormalities (Shi et al., 2013).
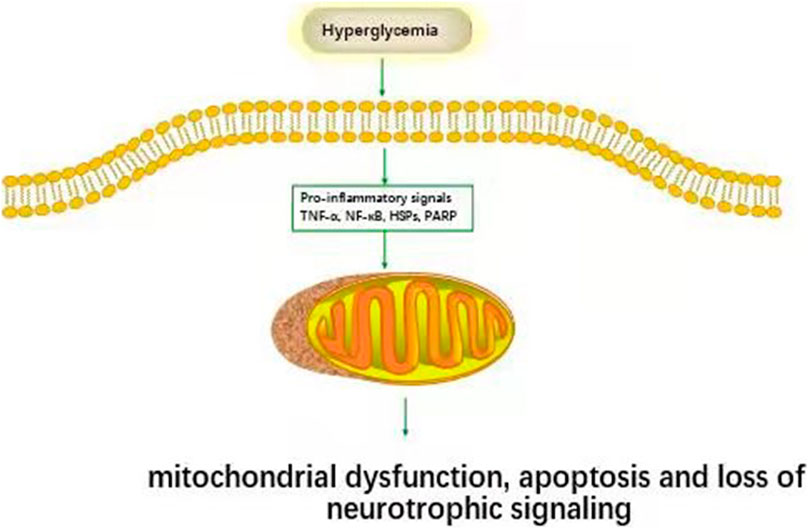
FIGURE2. Inflammatory pathways associated with DPN. Hyperglycemia activates inflammatory pathways (TNF-α, NF-κB, HSPs, PARP et al.) and causes cell damage.
Saleh et al. (2011) showed that the expression of TNF-α and interleukin-6 (IL-6) in the dorsal root ganglia (DRG) induced by STZ was downregulated in 2 and 5 months after induction, but it was found in the nerve. In addition, we also found that TNF-α induced neurite growth via the nuclear factor kappa-B (NF-κB) pathway in sensory neurons in vitro. However, the growth of this neurite was markedly impaired in sensory neurons of diabetic rats (A, A et al., 2011). It is concluded that the decrease of TNF-α in DRG may lead to the repair and regeneration of DPN -related nerve injury. Grosick et al. studied the response of macrophage phenotypes to high glucose and typical glucose environments. We found that in a high glucose environment, macrophages stimulated by LPS produced high levels of TNF-α and human macrophage chemoattractant protein-1 (MCP-1), but the effect of IL-6 was not so strong. However, lipopolysaccharide (LPS) stimulation did not significantly increase MCP-1 or TNF-α under normal glucose conditions compared with unstimulated macrophages. In addition, macrophages exposed to LPS and IL-10 maintained high TNF-α expression under high glucose conditions, while macrophages exposed to average glucose maintained high TNF-α expression; Only LPS stimulation could increase the secretion of TNF-α (Grosick et al., 2018).
Heat Shock ProteinS
Heat shock protein (HSP) is a chaperone protein synthesized by the body under stress (Sun and Li, 2003). HSP regulates the biological activities of various proteins under non-stress conditions, including regulating DNA replication, gene transcription, protein transfer of subcellular structure, cell signal transduction, immune response, growth, development, and apoptosis (Takahashi et al., 2003). Korngut et al. demonstrated that STZ induced diabetic mice overexpressed HSP27 (Korngut et al., 2012). Diabetic animals with high expression of HSP27 had axonal terminal density and mechanical sensitivity similar to those in the non-diabetic control group, which was in contrast with those in the diabetic non-transgenic control group. In addition, SCV in diabetic mice with high expression of HSP27 did not slow down compared with diabetic non-transgenic mice, but MCV was similar to that in diabetic mice. This study also showed that over expression of HSP27 in diabetic mice decreased receptor for advanced glycation endproducts (RAGE) positive sensory neurons compared with diabetic non transgenic-mice. It is worth further noting that compared with non-transgenic mice, the expression of activated caspase-3 as a cytotoxic marker of diabetes decreased in HSP27 overexpression mice. Elevated levels of HSP70 may be related to insulin resistance in type 2 diabetic patients. The number of endothelial progenitor cells decreased, and their function was impaired in T2DM patients, positively correlated with atherosclerotic cardiovascular events. These factors are closely related to complications such as diabetic peripheral neuropathy (Nakhjavani et al., 2010).
Poly ADP-Ribose Polymerase
Poly ADP-ribose polymerase-1(PARP-1) is a ribozyme with many regulatory functions and rich content. It can repair the DNA, maintain genome integrity and regulate the expression of many proteins, apoptosis, and death at the transcriptional level. Transplantation of bone marrow from poly ADP-ribose polymerase (PARP)-/- mice into wild type (WT) mice could inhibit the occurrence of DPN. On the contrary, when reversed (bone marrow from WT mice was transplanted into PARP mice), PARP−/− mice were vulnerable to DPN. In vitro experiments supported these results: when WT mice BM-derived cells and DRG were co-cultured in a high glucose environment, this led to the fusion of the two cells. This did not occur in a low glucose environment nor fuse with bone marrow-derived cells from PARP-/- mice (Terashima et al., 2012). PARP activation plays a vital role in the pathogenesis of diabetes and its complications. Activation of PARP in diabetic nerves can cause energy failure through vascular and non-vascular mechanisms. The activation of PARP can lead to the shortage of nutrient blood flow and sensory and motor conduction velocity in the neuro intima and lead to the degeneration of large and small nerve fibers. The motor nerve conduction velocity and the formation of sensory nerve conduction need PARP activation. Motor nerve conduction velocity and sensory nerve conduction function were less damaged in PARP deficient diabetic mice (Tesfaye and Selvarajah, 2012). Additional evidence found that the use of PARP inhibitor treatment in experimental diabetic patients could reduce the slowing of MCV and SCV and prevent the decrease of axon diameter and myelin sheath thickness. It is worth noting that inhibition of PARP prevents the increase of TNF-α and nitrotyrosine in the sciatic nerve and spinal cord (Drel et al., 2010).
Long term exposure to glucose leads to nonenzymatic glycosylation of peripheral myelin, which leads to further changes in the interaction between macrophages and myelin. It is worth noting that macrophages recognize the newly formed age products in the myelin sheath and internalize and attack it (Vlassara et al., 1984). Bekircan-Kurt et al. found that Rage was observed in distal skin biopsies of DPN patients, and the number of T cells adhering to blood vessels increased (Bekir Ca N-Kurt et al., 2015). In addition, when RAGE was deleted from diabetic male mice, it had a partial protective effect on the early electrophysiological parameters of chronic DPN. In contrast, insulin treatment had little impact on diabetic mice (De et al., 2017). IL-6 has a therapeutic effect on DPN. Giving IL-6 1 or 3 times a week had a significant preventive effect on decreasing MCV and SCV in STZ induced diabetic rats. It also improves heat sensitivity, reduced abnormal nerve fiber’s proportion, and prevents myelin thinning (Andriambeloson et al., 2010). Studies showed that AGE/RAGE signal led to the increase of NF-κB and IL-6 in DPN (Bierhaus et al., 2004). Advanced glycation end products (AGE) may accumulate naturally during aging. Interestingly, soluble RAGE therapy and RAGE knockout resulted in a decrease in NF-κB (Ravichandran et al., 2005; Figure 2).
NLRP3
NLRP3 belongs to the pattern recognition receptor of nucleotide-binding oligomerization domain-like receptors (NLR). NLRs have nucleotide-binding oligomerization domain (Nacht), which is supplemented by C-terminal leucine-rich repeats (LRRs structure) and N-terminal caspase (card) or pyrin domain. The function of LRRs is ligand sensing and self-regulation, while card and PYD structures regulate the interaction of downstream signal hemoproteins. Nacht structure is the only typical structure of all NLR families and is activated by ATP-dependent oligomerization. NLRPs subgroup includes PYD and LRR, and NLRP3 is a part of the NLRs subgroup. NLRP3 inflammasome is the most characteristic inflammasome in the NLRs. It comprises NLRP3, autophagy-related spotted protein (ACS), and cysteine aspartate specific protease caspase-1. Some scholars believe that the NLRP3 inflammatory body is a receptor of metabolic risk. NLRP3 inflammatory bodies play an important role in many non-infectious inflammatory diseases, such as gout, atherosclerosis, and diabetes. LRP3 inflammasome can activate caspase-1, then cut the precursor forms of inflammatory factors such as in IL-1β and IL-18, mature them and release them outside the cell, causing inflammatory response. IL-1β, reactive oxygen species (ROS), and thioredoxin interacting proteins are associated with the pathogenesis of type 2 diabetes. Sustained hyperglycemia induces ROS. ROS directly activates NLRP3 inflammasome or indirectly activates NLRP3 inflammasome by stimulating thioredoxin to release thioredoxin interacting protein. This process further aggravates chronic hyperglycemia and worsens diabetes. NLRP3 activation is the central link in the inflammatory mechanism of diabetes.
Immune Cells and Diabetic Peripheral Neuropathy
Lymphocytes
In the pathogenesis of DPN, the immune mechanism should not be ignored. The cellular immune mechanism with regulatory T lymphocyte subsets is the most important, which participates in the negative regulation mechanism of immune-mediated inflammation.
The cytotoxicity of CD8+ T lymphocytes to Schwann cells is also involved in the development of DPN. A quantitative immunohistochemical study was carried out on 20 cases of DPN sural nerve biopsy specimens to determine whether there was the infiltration of neural and epicardial lymphocytes in the diabetic nerve. There were 129 CD3+ cells in each tissue section of DPN patients and 0–5 cells in normal control. Diabetic nerve T cells infiltrated mainly CD8+ cells. The activated lymphocytes expressed immunoreactive cytokines and primary histocompatibility class II antigens. Infiltrating T cells may participate in the pathogenesis of diabetic neuropathy through various mechanisms (Younger et al., 2015).
Myelin protein correlated with the insulin receptors. After insulin treatment, the expression of myelin protein is up-regulated, which alleviates the nerve damage. It indirectly reflects the down-regulation of the insulin receptor, up-regulation of myelin associated glycoprotein, and down-regulation of myelin basic protein, which directly inhibited the myelin sheath formation, thus causing damage to DPN (Younger et al., 2015).
In addition, the number of pericytes is also involved in immune regulation, which is inversely proportional to CD4+, CD8+ T lymphocytes. In mice models lacking pericyte, the immune-related cells reduced, indirectly confirming that the peripheral cells participate in the mediated immunity (Hong et al., 2015).
Microglia
As innate immune cells, microglia can clear cell debris and foreign bodies and play the critical role of immune monitoring. Hyperglycemia and reactive oxygen species can also affect the local microenvironment of spinal cord and activate microglia. In turn, activated microglia synthesize and released inflammatory cytokines and neuroactive molecules, which induce the spinal cord injury-sensitive neurons (Couture et al., 2014). Activated microglia release various neuromodulators and neuroactive substances, such as reactive oxygen species, nitric oxide, peroxynitrite, prostaglandins, and pro-inflammatory cytokines, involved in hyperalgesia neuropathic pain of DPN. The expression of IL-1β and TNF-α in the spinal cord of STZ diabetic rats increased. The increase of IL-1β and TNF-α, as well as the thermal and mechanical hypersensitivity of rats, were inhibited by systemic or spinal administration of flucytosine, a non-selective metabolic inhibitor of glial cells, or minocycline, a selective microglia inhibitor. Systemic or spinal administration of flucytosine or minocycline inhibited IL-1 β And TNF- α As well as thermal and mechanical allergies in rats. A recent study found that atommoxetine (a new effective 5-hydroxytryptamine and norepinephrine reuptake inhibitor) had a sustained analgesic effect on STZ induced diabetic rats and could improve their depressive behavior. It could inhibit the activation of microglia and the phosphorylation of p38 and c-Jun N-terminal kinase (JNK) and reduce the inflammatory cytokines (Zhang et al., 2018).
Macrophages
Due to the reports of many macrophages in DPN, some researchers used these immune cells as a means of treatment. Macrophages have two polarization states: M1 and M2 macrophages, which express many inflammatory factors such as inducible nitric oxide synthase (iNOS), IL-1β, TNF-α, and anti-inflammatory cytokines such as Arg-1 (Huo et al., 2018), respectively. Under high glucose, M1 macrophages and other immune cells were activated to express a large number of inflammatory factors, which led to Schwann cell apoptosis and the occurrence of PDPN. Studies showed that the inhibition of TNF- α, and the release of M1 and macrophages into M2 macrophages could induce the gradual recovery of nerve conduction velocity, nerve blood flow, and axonal morphology in streptomycin -induced diabetic rats (Omi et al., 2017).
Macrophages can alleviate some symptoms of DPN in STZ induced diabetic rats. The researchers used liposomes encapsulated chlorondronate and found that compared with untreated diabetic rats, treatment resulted in lower blood glucose levels, higher serum insulin levels, and reduced mechanical hyperalgesia. The treatment did not affect heat sensitivity and body weight (Mert et al., 2009). Strangely, when liposome-encapsulated chlorophosphonate was used in combination with the pulsed magnetic field, the treatment effect was insufficient, while each treatment used alone affected DPN symptoms (Tufan et al., 2013).
Schwann Cells
Mitochondrial dysfunction in Schwann cells leads to lipid oxidation, early consumption of myelin, and accumulation of acylcarnitine lipid intermediates, leading to axonal degeneration and neuropathy (Viader et al., 2013). In addition, human Schwann cells exposed to high glucose can reduce the synthesis of phospholipids, which can be improved by aldose reductase inhibitors, suggesting that high glucose promotes the dyslipidemia of Schwann cells (Gonçalves et al., 2017). One study showed that glucose-stimulated Schwann cells to produce chemokines CXCchemokineligand-9(CXCL-9), CXCL-10, and CXCL-11 could induce the aggregation of T cells into diabetic neuropathy patients, thus promoting the development of neuropathy. These results also support that Schwann cells may be involved in the development of painful DPN (Tang et al., 2013).
Therapy of Diabetic Peripheral Neuropathy
First-Line Therapy
Tricyclic antidepressants include imipramine and amitriptyline, desipramine and nortriptyline. These blocked the reuptake of norepinephrine and serotonin by presynaptic neurons. Another mechanism that may contribute to its analgesic effect is to secure the uptake of 5HT and norepinephrine. A case control study showed that tricyclic antidepressive agents (TCAS) was influential in the treatment of DPN (Finnerup et al., 2005; Brouwers et al., 2010). Tricyclic antidepressants are well absorbed by oral administration, and their lipophilicity makes them widely distributed and easy to penetrate the central nervous system (CNS). However, due to the first pass metabolic changes in the liver, the bioavailability of tricyclic antidepressants is inconsistent. It needs to be increased to an effective dose, which must be administered continuously for 6–8 weeks (Max et al., 1992; Finnerup et al., 2010; Rudroju et al., 2013; Singh, 2014). Side effects include dry mouth, orthostatic hypotension, constipation, and urinary retention. In addition, the contraindications of TCAS are glaucoma and prostatic hypertrophy (Gandarias et al., 1998; Benbouzid et al., 2008).
Serotonin and noradrenaline reuptake inhibitors: Simultaneous inhibition of norepinephrine and serotonin reuptake can reduce DPN-related pain (Iqbal et al., 2018). We searched 13 studies showing the effect of serotonin and norepinephrine reuptake inhibitor (SNRI) on DPN. Duloxetine is the most studied SNRI. Eight randomized controlled trials showed that duloxetine was effective in the treatment of DPN (Goldstein et al., 2005; Wernicke et al., 2006; Raskin et al., 2006; Beard, Mccrink et al., 2008, Ajay D.; Wasan 2009; Joel et al., 2010; Tanenberg et al., 2011; Boyle et al., 2012; Lunn et al., 2014).
Calcium channel a2-δ ligands: Pain is one of the main symptoms of diabetic peripheral neuropathy, which seriously reduces patient’s quality of life. Studies showed that the first-line drug for alleviating diabetic peripheral neuropathic pain was calcium channel a2-δl, a modulator. Commonly used drugs are pregabalin and Gaba Martin. These drugs reduce the influx of neurons Na+ and Ca2+ and indirectly enhance the inhibition of γ-aminobutyric acid (GABA). It can reduce the activity of the N-methyl-D-aspartate receptor (NMDA receptor) by consuming the storage of excitatory neurotransmitter glutamate or blocking the active site of glutamate (Decroli et al., 2019).
Second-Line Therapy
Opioid analgesics can be used as the first-line clinical treatment, but due to their safety and potential abuse, many guidelines consider opioids as the second-line treatment (Finnerup et al., 2010). Some studies have shown that strong opioids play a positive role in the treatment of peripheral neuropathy (Huse et al., 2001; Raja et al., 2002; Morley et al., 2003).
Tramadol is a weak opioid, which can inhibit the reuptake of norepinephrine and serotonin. Most international guidelines consider tramadol as second-line therapy (Boureau et al., 2003; Duhmke et al., 2006).
Immunoinflammatory Therapy
In animal experiments, many methods were used to treat DPN. The combination of insulin and curcumin or resveratrol in STZ induced diabetic mice reduced the TNF-α and hypersensitivity in vivo, which was more evident than insulin or the use of two drugs alone. Thakur et al. (Thakur et al., 2016) also used virus-mediated IL-10 to reduce the mechanical and thermal hypersensitivity of STZ induced diabetic rats. This treatment prevented the increase of Toll-like receptors4 (TLR4), IL-1, phosphorylated p38, and phosphorylated protein kinase C levels and decreased NeuN and HSP70 protein levels in the diabetic control group. It is also noteworthy that IL-10 treatment prevented the activation of macrophages in DRG. Under hyperglycemic conditions, cultured DRGs exposed to TLR4 antagonists could avoid increasing protein TNF-α and showed a significant increase of HSP70. In vivo tests, diabetic animals exposed to TLR4 antagonists reduced TLR4 and TNF-α levels and increased mechanical sensitivity but did not increase heat sensitivity.
Ni et al., 2017) studied the effect of salidroside. Salidroside had anti-inflammatory properties. When salidroside treated DPN rats, they found that it decreased mechanical sensitivity only after 8 weeks of treatment and decreased thermal sensitivity until 8 weeks after 5 weeks. They also found that sensory nerve conduction velocity improved after 5 weeks of treatment. In terms of mechanism, the authors found that treatment could reduce the levels of pro-inflammatory cytokines TNF-α and IL-1 in the spinal cord and sciatic nerve. The study also found that treatment could reduce the protein expression of the P2X7 receptor in the spinal cord. P2X7 receptor played a role in mediating neuropathic pain by secreting pro-inflammatory factors.
Molecular Therapy
Virus vector technology: Viral vector is the most efficient vector for gene transmission. Except for adenovirus, other viral vectors can integrate foreign genes into chromosomes by infecting host cells, which can be used in cells that are difficult to transfect. After the viral DNA was integrated into the host cell genome, it could express stable gene therapy potential (Liu et al., 2020). Lentivirus (LV) belongs to Retroviridae and is a diploid RNA virus. Lentiviral vectors are vectors based on the genome of lentivirus, in which multiple sequence structures related to virus activity are removed to ensure biological safety. Then exogenous genes are introduced into the genome skeleton. Tasyurek et al. injected LV carrying human glucagon-like peptide-1 (GLP-1) gene, which could reduce the blood glucose level of the T2DM rat model induced by a high-fat diet combined with streptozotocin, and its triglyceride level returns to normal. These results showed that LV could effectively transfer potentially therapeutic genes into islet cells for DPN treatment (Tasyurek et al., 2018). Adenovirus vectors (AdV) can infect a variety of human tissue cells. AdV has the advantages of high titer, strong gene transfection ability, non-integration into the host genome, and will not affect islet transplantation. At present, it has been widely used in gene transduction in vitro, vaccination in vivo, and gene therapy (Jane and Bradbury, 2003). In the treatment of diabetes, AdV is still in animal experiments. Suzuki et al. (Suzuki et al., 2004)used AdV to introduce insulin receptor substrate-2 (IRS-2) gene into IRS-2 deficient mice and found that the blood glucose level of mice returned to normal. The transcription factor pancreaticoduodenal homeobox-1 gene can be transfected into mouse islet cells by AdV to participate in islet maturation. In addition, the pancreatic differentiation transcription factor neurogenin 3 (Ngn3) could be transfected into mouse hepatocytes by adv to secrete insulin (Reach, 2001).
Stem cell therapy: Stem cells can differentiate into tissues such as fat, bone, and cartilage. They have strong self-renewal ability, multidirectional differentiation potential, and secrete a variety of cytokines. They have a good application prospect in the treatment of DPN. Mesenchymal stem cells (MSCs) therapy can improve the blood glucose level of diabetic patients from the vital point of diabetes. Bone marrow MSCs and pancreatic MSCs can be differentiated into Islet β Cells under the regulation of multiple growth factors and hormones. Then the blood glucose concentration of diabetic mice could be reduced by increasing secretion of insulin and C peptides (Lin et al., 2010; Mu and Li 2015). Transplantation of MSCs can promote the proliferation of islet cells, increase the number of insulin-secreting cells, improve the structure of islets, increase the synthesis and secretion of insulin, and reduce blood glucose concentration.
Non-drug Therapy
Exercise: Exercise plays an irreplaceable role in the treatment of diseases. It is the basis of therapy and should run through the whole process of DPN treatment. Exercise therapy mainly includes aerobic exercise and anti-resistance exercise. Anti-resistance exercise has a better therapeutic effect on DPN. Kluding et al. intervened 17 DPN patients with moderate intensity, aerobic and anti-resistance exercise under the guidance and supervision of professional coaches. After 10 weeks of intervention, they carried out self-control. The research results showed that exercise could alleviate DPN patient’s pain symptoms, increase the density of nerve fibers in the epidermis, and improve their neurological symptoms. Exercise can also protect the nerve injury of DPN patients by enhancing the ability of nerve regeneration (Kluding et al., 2012; Singleton et al., 2014). In terms of optimizing the exercise therapy program, we can work out a safe and effective individualized exercise program through multidisciplinary cooperation.
Electrotherapy: In recent years, more and more scholars have pay attention to electrotherapy, including transcutaneous electrical nerve stimulation (TENS); Peripheral nerve, nerve root, spinal cord, deep brain, and epidural motor cortex stimulation; Pulsed magnetic field and static magnetic field; High-frequency external muscle stimulation (Pieber et al., 2010). However, among all electrotherapy measures, only tens are recommended by the American Academy of Neurology (AAN) for pain treatment of DPN (Dubinsky and Miyasaki, 2010). Upton GA et al. discussed the effects of two different intensities of TENS on patients with DPN. The results showed that the acupuncture-like stimulation mode of 2 Hz and 200 ms could better alleviate the pain caused by DPN without adverse reactions (Zotz and De, 2015). Current studies believe that the theoretical basis of electrical stimulation improves the microcirculation of DPN patients and increases the nerve blood flow to alleviate the pain caused by ischemia. However, Gossrau g et al. conducted a randomized placebo-controlled experiment. The results showed that the therapeutic effect of TENS was not better than that of the placebo group, and the difference was not statistically significant (Gudrun et al., 2011). TENS has the advantages of safety, noninvasive, simple operation, and good compliance. However, its exact role is still controversial, which may be related to the small sample size of existing studies, short intervention cycle, unclear long-term efficacy, and lack of evidence-based basis, limiting its clinical application.
Acupuncture: Acupuncture and moxibustion can improve nerve conduction and alleviate patient’s clinical symptoms with DPN. The mechanism may be related to acupuncture, and moxibustion can improve microcirculation and reduce ischemia and hypoxia of nerve tissue. Jeon et al. treated 9 outpatients with painful DPN with acupuncture and moxibustion for 4 weeks. The results showed that acupuncture and moxibustion could significantly improve the pain symptoms of patients. They believed that acupuncture and moxibustion were effective but did not study their mechanism (Jeon et al., 2014). The relevant systematic evaluation analyzed the effect of acupuncture and moxibustion on DPN. Although the results showed that the outcome was better than conventional treatment, it also pointed out that the development of acupuncture and moxibustion on DPN was uncertain due to the low quality of relevant RCT experimental literature included in the study (Dimitrova et al., 2017).
Complications and Comorbidities Therapy
Most patients with diabetic peripheral neuropathy are accompanied by depression, anxiety, insomnia, poor appetite, lower body weight, suicidal ideation, drug abuse. These complications affect their mental health and have adverse effects on the treatment and rehabilitation of their patients, which is not conducive to their physical health. Compared with patients with uncomplicated diabetes mellitus, diabetic peripheral neuropathy patients have more severe pain, and the severity of negative emotions and comorbidities is more serious. They can be treated with first-line drugs for DPN, symptomatic pain relief, and relief of negative emotions.
In addition, for patients with diabetic peripheral neuropathy, clinical targeted psychological intervention should be carried out to alleviate patient’s negative emotions. Psychological intervention should be based on different causes of anxiety and depression, including cognitive intervention, environmental intervention, and emotional intervention. Particular attention should be paid to patient’s family and social intervention because family warmth, social understanding, and support are good drugs for treating psychosomatic diseases.
Conclusion
In summary, diabetic peripheral neuropathy is related to many factors, such as metabolism, immunity, heredity, etc., the etiology is currently uncertain. In this review, we highlighted the latest immunological mechanism of DPN and explored their corresponding potential therapeutic targets. Its complex mechanism makes it difficult to treat. There is no specific drug therapy. With the increase of diabetic patients, more and more patients suffer from the complications of diabetes. This makes the requirement to control diabetes, and its complications become more urgent. The pathogenesis of diabetic peripheral neuropathy needs to be further in-depth and comprehensive research to achieve more standardized management of diabetic peripheral neuropathy.
Author Contributions
MY and LZ initiated the work, designed the idea. XZ, YX, and SL prepared and collected material and data. TX and XW wrote the paper. All authors reviewed the manuscript. All authors read and approved the final manuscript.
Funding
The work was supported by Liaoning Provincial Nature Fund guidance plan (No. 2019-ZD-1006).
Conflict of Interest
The authors declare that the research was conducted in the absence of any commercial or financial relationships that could be construed as a potential conflict of interest.
Publisher’s Note
All claims expressed in this article are solely those of the authors and do not necessarily represent those of their affiliated organizations, or those of the publisher, the editors and the reviewers. Any product that may be evaluated in this article, or claim that may be made by its manufacturer, is not guaranteed or endorsed by the publisher.
References
Ametov, A. S., Barinov, A., Dyck, P. J., Hermann, R., Kozlova, N., Litchy, W. J., et al. (2003). The Sensory Symptoms of Diabetic Polyneuropathy Are Improved with Alpha-Lipoic Acid: the SYDNEY Trial. Diabetes Care 26 (3), 770–776. doi:10.2337/diacare.26.3.770
Andriambeloson, E., Baillet, C., Vitte, P. A., Garotta, G., Dreano, M., and Callizot, N. (2010). Interleukin-6 Attenuates the Development of Experimental Diabetes-Related Neuropathy. Neuropathology 26 (1), 32–42. doi:10.1111/j.1440-1789.2006.00651.x
Apfel, S. C. (2002). Nerve Growth Factor for the Treatment of Diabetic Neuropathy: what Went Wrong, what Went Right, and what Does the Future Hold. Int. Rev. Neurobiol. 50, 393–413. doi:10.1016/s0074-7742(02)50083-0
Beard, S. M., McCrink, L., Le, T. K., Garcia-Cebrian, A., Monz, B., and Malik, R. A. (2008). Cost Effectiveness of Duloxetine in the Treatment of Diabetic Peripheral Neuropathic Pain in the UK. Curr. Med. Res. Opin. 24 (2), 385–399. doi:10.1185/030079908x253852
Bekircan-Kurt, C. E., Uçeyler, N., and Sommer, C. (2015). Cutaneous Activation of Rage in Nonsystemic Vasculitic and Diabetic Neuropathy. Muscle Nerve 50 (3), 377–383. doi:10.1002/mus.24164
Benbouzid, M., Gavériaux-Ruff, C., Yalcin, I., Waltisperger, E., Tessier, L. H., Muller, A., et al. (2008). Delta-opioid Receptors Are Critical for Tricyclic Antidepressant Treatment of Neuropathic Allodynia. Biol. Psychiatry 63 (6), 633–636. doi:10.1016/j.biopsych.2007.06.016
Bierhaus, A., Haslbeck, K. M., Humpert, P. M., Liliensiek, B., Dehmer, T., Morcos, M., et al. (2004). Loss of Pain Perception in Diabetes Is Dependent on a Receptor of the Immunoglobulin Superfamily. J. Clin. Invest. 114 (12), 1741–1751. doi:10.1172/JCI18058
Boureau, F., Legallicier, P., and Kabir-Ahmadi, M. (2003). Tramadol in post-herpetic Neuralgia: a Randomized, Double-Blind, Placebo-Controlled Trial. Pain 104 (1-2), 323–331. doi:10.1016/s0304-3959(03)00020-4
Boussageon, R., Bejan-Angoulvant, T., Saadatian-Elahi, M., Bergeonneau, C., Kassai, B., et al. (2011). Effect of Intensive Glucose Lowering Treatment on All Cause Mortality, Cardiovascular Death, and Microvascular Events in Type 2 Diabetes: Meta-Analysis of Randomised Controlled Trials. BMJ 343, d5301. doi:10.1136/bmj.d5301
Boyle, J., Eriksson, M. E., Gribble, L., Gouni, R., Johnsen, S., Coppini, D. V., et al. (2012). Randomized, Placebo-Controlled Comparison of Amitriptyline, Duloxetine, and Pregabalin in Patients with Chronic Diabetic Peripheral Neuropathic Pain: Impact on Pain, Polysomnographic Sleep, Daytime Functioning, and Quality of Life. Diabetes Care 35 (12), 2451–2458. doi:10.2337/dc12-0656
Bril, V., Hirose, T., Tomioka, S., and Buchanan, R. (2009). Ranirestat for the Management of Diabetic Sensorimotor Polyneuropathy. Diabetes Care 32 (7), 1256–1260. doi:10.2337/dc08-2110
Brouwers, M. C., Kho, M. E., Browman, G. P., Burgers, J. S., Cluzeau, F., Feder, G., et al. (2010). AGREE II: Advancing Guideline Development, Reporting and Evaluation in Health Care. CMAJ 182 (18), E839–E842. doi:10.1503/cmaj.090449
Callaghan, B. C., Hur, J., and Feldman, E. L. (2012). Diabetic Neuropathy: One Disease or Two. Curr. Opin. Neurol. 25 (5), 536–541. doi:10.1097/WCO.0b013e328357a797
Callaghan, B. C., Little, A. A., Feldman, E. L., and Hughes, R. A. (2012b). Enhanced Glucose Control for Preventing and Treating Diabetic Neuropathy. Cochrane Database Syst. Rev. 6 (6), CD007543. doi:10.1002/14651858.CD007543.pub2
Coert, J. H., Fakkel, T. M., van Neck, J. W., Verhagen, A. P., and Çakici, N. (2016). Systematic Review of Treatments for Diabetic Peripheral Neuropathy. Diabet Med. 33, 1466–1476. doi:10.1111/dme.13083
Dan, Z., Movsesyan, L., Mankovsky, B., Gurieva, I., Abylaiuly, Z., Strokov, I., et al. (2009). Treatment of Symptomatic Polyneuropathy with Actovegin in Type 2 Diabetic Patients. Diabetes Care 32 (8), 1479–1484. doi:10.2337/dc09-0545
de Gandarias, J. M., Echevarria, E., Acebes, I., Silio, M., and Casis, L. (1998). Effects of Imipramine Administration on Mu-Opioid Receptor Immunostaining in the Rat Forebrain. Arzneimittelforschung 48 (7), 717–719.
de la Hoz, C. L., Cheng, C., Fernyhough, P., and Zochodne, D. W. (2017). A Model of Chronic Diabetic Polyneuropathy: Benefits from Intranasal Insulin Are Modified by Sex and RAGE Deletion. Am. J. Physiol. Endocrinol. Metab. 312 (5), E407–E419. doi:10.1152/ajpendo.00444.2016
Decroli, E., Manaf, A., Syahbuddin, S., Syafrita, Y., and Dillasamola, D. (2019). The Correlation between Malondialdehyde and Nerve Growth Factor Serum Level with Diabetic Peripheral Neuropathy Score. Open Access Maced J. Med. Sci. 7 (1), 103–106. doi:10.3889/oamjms.2019.029
Dimitrova, A., Murchison, C., and Oken, B. (2017). Acupuncture for the Treatment of Peripheral Neuropathy: A Systematic Review and Meta-Analysis. J. Altern. Complement. Med. 23 (3), 164–179. doi:10.1089/acm.2016.0155
Drel, V. R., Lupachyk, S., Shevalye, H., Vareniuk, I., Xu, W., Zhang, J., et al. (2010). New Therapeutic and Biomarker Discovery for Peripheral Diabetic Neuropathy: PARP Inhibitor, Nitrotyrosine, and Tumor Necrosis Factor-{alpha}. Endocrinology 151 (6), 2547–2555. doi:10.1210/en.2009-1342
Dubinsky, R. M., and Miyasaki, J. (2010). Assessment: Efficacy of Transcutaneous Electric Nerve Stimulation in the Treatment of Pain in Neurologic Disorders (An Evidence-Based Review): Report of the Therapeutics and Technology Assessment Subcommittee of the American Academy of Neurology. Neurology 74 (2), 173–176. doi:10.1212/WNL.0b013e3181c918fc
Duhmke, R. M., Cornblath, D. D., and Hollingshead, J. R. (2006). Tramadol for Neuropathic Pain. Cochrane Database Syst. Rev. 3 (2), CD003726. doi:10.1002/14651858.CD003726.pub2
Duksal, T., Tiftikcioglu, B. I., Bilgin, S., Kose, S., and Zorlu, Y. (2016). Role of Inflammation in Sensory Neuropathy in Prediabetes or Diabetes. Acta Neurol. Scand. 133 (5), 384–390. doi:10.1111/ane.12474
Edwards, J. L., Vincent, A. M., Cheng, H. T., and Feldman, E. L. (2008). Diabetic Neuropathy: Mechanisms to Management. Pharmacol. Ther. 120 (1), 1–34. doi:10.1016/j.pharmthera.2008.05.005
Eid, S., Sas, K. M., Abcouwer, S. F., Feldman, E. L., Gardner, T. W., Pennathur, S., et al. (2019). New Insights into the Mechanisms of Diabetic Complications: Role of Lipids and Lipid Metabolism. Diabetologia 62 (12), 1539–1549. doi:10.1007/s00125-019-4959-1
Ermis, N., Gullu, H., Caliskan, M., Unsal, A., Kulaksizoglu, M., and Muderrisoglu, H. (2010). Gabapentin Therapy Improves Heart Rate Variability in Diabetic Patients with Peripheral Neuropathy. J. Diabetes Complications 24 (4), 229–233. doi:10.1016/j.jdiacomp.2008.12.001
Evans, J. D., Jacobs, T. F., and Evans, E. W. (2008). Role of Acetyl-L-Carnitine in the Treatment of Diabetic Peripheral Neuropathy. Ann. Pharmacother. 42 (11), 1686–1691. doi:10.1345/aph.1L201
Ferland-Mccollough, D., Slater, S., Richard, J., Reni, C., and Mangialardi, G. (2016). Pericytes, an Overlooked Player in Vascular Pathobiology. Pharmacol. Ther. 171, 30–42. doi:10.1016/j.pharmthera.2016.11.008
Finnerup, N. B., Otto, M., McQuay, H. J., Jensen, T. S., and Sindrup, S. H. (2005). Algorithm for Neuropathic Pain Treatment: an Evidence Based Proposal. Pain 118 (3), 289–305. doi:10.1016/j.pain.2005.08.013
Finnerup, N. B., Sindrup, S. H., and Jensen, T. S. (2010). The Evidence for Pharmacological Treatment of Neuropathic Pain. Pain 150 (3), 573–581. doi:10.1016/j.pain.2010.06.019
Goldstein, D. J., Lu, Y., Detke, M. J., Lee, T. C., and Iyengar, S. (2005). Duloxetine vs. Placebo in Patients with Painful Diabetic Neuropathy. Pain 116 (1-2), 109–118. doi:10.1016/j.pain.2005.03.029
Gonçalves, N. P., Vægter, C. B., Andersen, H., Østergaard, L., Calcutt, N. A., and Jensen, T. S. (2017). Schwann Cell Interactions with Axons and Microvessels in Diabetic Neuropathy. Nat. Rev. Neurol. 13 (3), 135–147. doi:10.1038/nrneurol.2016.201
Gossrau, G., Wähner, M., Kuschke, M., Konrad, B., Reichmann, H., Wiedemann, B., et al. (2011). Microcurrent Transcutaneous Electric Nerve Stimulation in Painful Diabetic Neuropathy: A Randomized Placebo-Controlled Study. Pain Med. 12 (6), 953–960. doi:10.1111/j.1526-4637.2011.01140.x
Grosick, R., Alvarado-Vazquez, P. A., Messersmith, A. R., and Romero-Sandoval, E. A. (2018). High Glucose Induces a Priming Effect in Macrophages and Exacerbates the Production of Pro-inflammatory Cytokines after a challenge. J. Pain Res. 11, 1769–1778. doi:10.2147/JPR.S164493
Group, D. R., Nathan, D. M., Genuth, S., Lachin, J., Cleary, P., Crofford, O., et al. (1993). The Effect of Intensive Treatment of Diabetes on the Development and Progression of Long-Term Complications in Insulin-dependent Diabetes Mellitus. N. Engl. J. Med. 329, 977–986. doi:10.1056/NEJM199309303291401
Hong, J., Tobin, N. P., Rundqvist, H., Li, T., Lavergne, M., García-Ibáñez, Y., et al. (2015). Role of Tumor Pericytes in the Recruitment of Myeloid-Derived Suppressor Cells. JNCI.J 107, djv209. doi:10.1093/jnci/djv209
Hotta, N., Akanuma, Y., Kawamori, R., Matsuoka, K., Oka, Y., Shichiri, M., et al. (2006). Long-term Clinical Effects of Epalrestat, an Aldose Reductase Inhibitor, on Diabetic Peripheral Neuropathy: the 3-year, Multicenter, Comparative Aldose Reductase Inhibitor-Diabetes Complications Trial. Diabetes Care 29 (7), 1538–1544. doi:10.2337/dc05-2370
Huo, W., Zhang, Y., Liu, Y., Lei, Y., Sun, R., Zhang, W., et al. (2018). Dehydrocorydaline Attenuates Bone Cancer Pain by Shifting Microglial M1/M2 Polarization toward the M2 Phenotype. Mol. Pain 14, 1744806918781733. doi:10.1177/1744806918781733
Huse, E., Larbig, W., Flor, H., and Birbaumer, N. (2001). The Effect of Opioids on Phantom Limb Pain and Cortical Reorganization. Pain 90 (1-2), 47–55. doi:10.1016/s0304-3959(00)00385-7
Hussain, G., Rizvi, S. A., Singhal, S., Zubair, M., and Ahmad, J. (2013). Serum Levels of TNF-α in Peripheral Neuropathy Patients and its Correlation with Nerve Conduction Velocity in Type 2 Diabetes Mellitus. Diabetes Metab. Syndr. 7 (4), 238–242. doi:10.1016/j.dsx.2013.02.005
Iqbal, Z., Azmi, S., Yadav, R., Ferdousi, M., Kumar, M., Cuthbertson, D. J., et al. (2018). Diabetic Peripheral Neuropathy: Epidemiology, Diagnosis, and Pharmacotherapy. Clin. Ther. 40 (6), 828–849. doi:10.1016/j.clinthera.2018.04.001
Jamwal, S., and Sharma, S. (2018). Vascular Endothelium Dysfunction: a Conservative Target in Metabolic Disorders. Inflamm. Res. 67, 391–405. doi:10.1007/s00011-018-1129-8
Jane, B. (2003). Potential Gene Therapy Cure for Diabetes Reported. Lancet 361 (9367), 1443. doi:10.1016/s0140-6736(03)13154-6()
Jeon, E., Kwon, H., Shin, I., Kang, S., and Shon, H. (2014). Effect of Acupuncture on Diabetic Peripheral Neuropathy: an Uncontrolled Preliminary Study from Korea. Acupunct Med. 32, 350–352. doi:10.1136/acupmed-2013-010479
Kluding, P. M., Pasnoor, M., Singh, R., Jernigan, S., Farmer, K., Rucker, J., et al. (2012). The Effect of Exercise on Neuropathic Symptoms, Nerve Function, and Cutaneous Innervation in People with Diabetic Peripheral Neuropathy. J. Diabetes Complications 26 (5), 424–429. doi:10.1016/j.jdiacomp.2012.05.007
Korngut, L., Ma, C. H., Martinez, J. A., Toth, C. C., Guo, G. F., Singh, V., et al. (2012). Overexpression of Human HSP27 Protects Sensory Neurons from Diabetes. Neurobiol. Dis. 47 (3), 436–443. doi:10.1016/j.nbd.2012.04.017
Lin, H. Y., Tsai, C. C., Chen, L. L., Chiou, S. H., Wang, Y. J., and Hung, S. C. (2010). Fibronectin and Laminin Promote Differentiation of Human Mesenchymal Stem Cells into Insulin Producing Cells through Activating Akt and ERK. J. Biomed. Sci. 17 (1), 56. doi:10.1186/1423-0127-17-56
Liu, X., Huang, H., Gao, Y., Zhou, L., Yang, J., Li, X., et al. (2020). Visualization of Gene Therapy with a Liver Cancer-Targeted Adeno-Associated Virus 3 Vector. J. Cancer 11 (8), 2192–2200. doi:10.7150/jca.39579
Lunn, M. P., Hughes, R. A., and Wiffen, P. J. (2014). Duloxetine for Treating Painful Neuropathy, Chronic Pain or Fibromyalgia. Cochrane Database Syst. Rev. 3, CD007115. doi:10.1002/14651858.cd007115.pub3
Max, M. B., Lynch, S. A., Muir, J., Shoaf, S. E., Smoller, B., and Dubner, R. (1992). Effects of Desipramine, Amitriptyline, and Fluoxetine on Pain in Diabetic Neuropathy. N. Engl. J. Med. 326 (19), 1250–1256. doi:10.1056/NEJM199205073261904
Mert, T., Gunay, I., Ocal, I., Guzel, A. I., Inal, T. C., Sencar, L., et al. (2009). Macrophage Depletion Delays Progression of Neuropathic Pain in Diabetic Animals. Naunyn Schmiedebergs Arch. Pharmacol. 379 (5), 445–452. doi:10.1007/s00210-008-0387-3
Mert, T., Ocal, I., Guzel, A. I., and Gunay, I. (2013). Clodronate Changes Neurobiological Effects of Pulsed Magnetic Field on Diabetic Rats with Peripheral Neuropathy. Electromagn. Biol. Med. 32 (3), 342–354. doi:10.3109/15368378.2012.721846
Morley, J. S., Bridson, J., Nash, T. P., Miles, J. B., White, S., and Makin, M. K. (2003). Low-dose Methadone Has an Analgesic Effect in Neuropathic Pain: a Double-Blind Randomized Controlled Crossover Trial. Palliat. Med. 17 (7), 576–587. doi:10.1191/0269216303pm815oa
Mu, Y. M., and Li, Z. (2015). Stem Cells: a New Choice for Diabetes Therapy. Med. J. Chin. Peoples Liberation Army 40 (7), 515–518.
Mu, Z. P., Wang, Y. G., Li, C. Q., Lv, W. S., Wang, B., Jing, Z. H., et al. (2017). Association between Tumor Necrosis Factor-α and Diabetic Peripheral Neuropathy in Patients with Type 2 Diabetes: a Meta-Analysis. Mol. Neurobiol. 54 (2), 983–996. doi:10.1007/s12035-016-9702-z
Nakhjavani, M., Morteza, A., Khajeali, L., Esteghamati, A., Khalilzadeh, O., Asgarani, F., et al. (2010). Increased Serum HSP70 Levels Are Associated with the Duration of Diabetes. Cell Stress Chaperones 15 (6), 959–964. doi:10.1007/s12192-010-0204-z
Ni, G. L., Cui, R., Shao, A. M., and Wu, Z. M. (2017). Salidroside Ameliorates Diabetic Neuropathic Pain in Rats by Inhibiting Neuroinflammation. J. Mol. Neurosci. 63 (1), 9–16. doi:10.1007/s12031-017-0951-8
Omi, M., Hata, M., Nakamura, N., Miyabe, M., Ozawa, S., Nukada, H., et al. (2017). Transplantation of Dental Pulp Stem Cells Improves Long-Term Diabetic Polyneuropathy Together with Improvement of Nerve Morphometrical Evaluation. Stem Cel Res Ther 8 (1), 279. doi:10.1186/s13287-017-0729-5
Pieber, K., Herceg, M., and Paternostro-Sluga, T. (2010). Electrotherapy for the Treatment of Painful Diabetic Peripheral Neuropathy: a Review. J. Rehabil. Med. 42 (4), 289–295. doi:10.2340/16501977-0554
Raja, S. N., Haythornthwaite, J. A., Pappagallo, M., Clark, M. R., Travison, T. G., Sabeen, S., et al. (2002). Opioids versus Antidepressants in Postherpetic Neuralgia: A Randomized, Placebo-Controlled Trial. Neurology 59 (7), 1015–1021. doi:10.1212/wnl.59.7.1015
Ramirez, M. A., and Borja, N. L. (2012). Epalrestat: an Aldose Reductase Inhibitor for the Treatment of Diabetic Neuropathy. Pharmacotherapy 28 (5), 646–655. doi:10.1592/phco.28.5.646
Raskin, J., Pritchett, Y. L., Wang, F., D'Souza, D. N., Waninger, A. L., Iyengar, S., et al. (2005). A Double-Blind, Randomized Multicenter Trial Comparing Duloxetine with Placebo in the Management of Diabetic Peripheral Neuropathic Pain. Pain Med. 6 (5), 346–356. doi:10.1111/j.1526-4637.2005.00061.x
Raskin, J., Smith, T. R., Wong, K., Pritchett, Y. L., D'Souza, D. N., Iyengar, S., et al. (2006). Duloxetine versus Routine Care in the Long-Term Management of Diabetic Peripheral Neuropathic Pain. J. Palliat. Med. 9 (1), 29–40. doi:10.1089/jpm.2006.9.29
Ravichandran, R., Vannucci, S. J., Yan, S. S., Herold, K., Yan, S. F., Schmidt, A. M., et al. (2005). Advanced Glycation End Products and RAGE: a Common Thread in Aging, Diabetes, Neurodegeneration, and Inflammation. Glycobiology 15, 16R. doi:10.1093/glycob/cwi053
Reach, G. (2001). Towards a Cell Therapy for Diabetes? an Epistemiological Perspective. J. Soc. Biol. 195 (1), 83–90. doi:10.1051/jbio/2001195010083
Rudroju, N., Bansal, D., Talakokkula, S. T., Gudala, K., Hota, D., Bhansali, A., et al. (2013). Comparative Efficacy and Safety of Six Antidepressants and Anticonvulsants in Painful Diabetic Neuropathy: a Network Meta-Analysis. Pain Physician 16 (6), E705–E714.
Saeedi, P., Petersohn, I., Salpea, P., Malanda, B., Karuranga, S., Unwin, N., et al. (2019). Global and Regional Diabetes Prevalence Estimates for 2019 and Projections for 2030 and 2045: Results from the International Diabetes Federation Diabetes Atlas, 9th Edition. Diabetes Res. Clin. Pract. 157, 107843. doi:10.1016/j.diabres.2019.107843
Saleh, A., Smith, D. R., Balakrishnan, S., Dunn, L., Martens, C., Tweed, C. W., et al. (2011). Tumor Necrosis Factor-α Elevates Neurite Outgrowth through an NF-κb-dependent Pathway in Cultured Adult Sensory Neurons: Diminished Expression in Diabetes May Contribute to Sensory Neuropathy. Brain Res. 1423 (1), 87–95. doi:10.1016/j.brainres.2011.09.029
Shi, X., Chen, Y., Nadeem, L., and Xu, G. (2013). Beneficial Effect of TNF-α Inhibition on Diabetic Peripheral Neuropathy. J. Neuroinflammation 10, 69. doi:10.1186/1742-2094-10-69
Singh, S. K. (2014). Treatment of Painful Diabetic Neuropathy. J. Assoc. Physicians India 62 (5), 381–382.
Singleton, J. R., Marcus, R. L., Jackson, J. E., K Lessard, M., Graham, T. E., and Smith, A. G. (2014). Exercise Increases Cutaneous Nerve Density in Diabetic Patients without Neuropathy. Ann. Clin. Transl Neurol. 1 (10), 844–849. doi:10.1002/acn3.125
Stino, A. M., and Smith, A. G. (2017). Peripheral Neuropathy in Prediabetes and the Metabolic Syndrome. J. Diabetes Investig. 8 (5), 646–655. doi:10.1111/jdi.12650
Suzuki, R., Tobe, K., Aoyama, M., Inoue, A., Sakamoto, K., Yamauchi, T., et al. (2004). Both insulin Signaling Defects in the Liver and Obesity Contribute to Insulin Resistance and Cause Diabetes in Irs2(-/-) Mice. J. Biol. Chem. 279 (24), 25039–25049. doi:10.1074/jbc.M311956200
Sztanek, F., Molnárné Molnár, Á., and Balogh, Z. (2016). The Role of Oxidative Stress in the Development of Diabetic Neuropathy. Orv Hetil 157 (49), 1939–1946. doi:10.1556/650.2016.30609
Tanenberg, R. J., Irving, G. A., Risser, R. C., Ahl, J., Robinson, M. J., Skljarevski, V., et al. (2011). Duloxetine, Pregabalin, and Duloxetine Plus Gabapentin for Diabetic Peripheral Neuropathic Pain Management in Patients with Inadequate Pain Response to Gabapentin: An Open-Label, Randomized, Noninferiority Comparison. Mayo Clin. Proc. 86 (7), 615–626. doi:10.4065/mcp.2010.0681
Tang, W., Lv, Q., Chen, X. F., Zou, J. J., Liu, Z. M., and Shi, Y. Q. (2013). CD8(+) T Cell-Mediated Cytotoxicity toward Schwann Cells Promotes Diabetic Peripheral Neuropathy. Cell Physiol Biochem 32 (4), 827–837. doi:10.1159/000354485
Tasyurek, H. M., Altunbas, H. A., Balci, M. K., Griffith, T. S., and Sanlioglu, S. (2018). Therapeutic Potential of Lentivirus-Mediated Glucagon-like Peptide-1 Gene Therapy for Diabetes. Hum. Gene Ther. 29, 802–815. doi:10.1089/hum.2017.180
Terashima, T., Kojima, H., and Chan, L. (2012). Bone Marrow Expression of poly(ADP-Ribose) Polymerase Underlies Diabetic Neuropathy via Hematopoietic-Neuronal Cell Fusion. FASEB J. 26 (1), 295–308. doi:10.1096/fj.11-186262
Tesfaye, S., and Selvarajah, D. (2012). Advances in the Epidemiology, Pathogenesis and Management of Diabetic Peripheral Neuropathy. Diabetes Metab. Res. Rev. 28 Suppl 1 (Suppl. S1), 8–14. doi:10.1002/dmrr.2239
Thakur, V., Gonzalez, M., Pennington, K., and Chattopadhyay, M. (2016). Viral Vector Mediated Continuous Expression of Interleukin-10 in DRG Alleviates Pain in Type 1 Diabetic Animals. Mol. Cel Neurosci 72, 46–53. doi:10.1016/j.mcn.2016.01.006
Viader, A., Sasaki, Y., Kim, S., Strickland, A., Workman, C. S., Yang, K., et al. (2013). Aberrant Schwann Cell Lipid Metabolism Linked to Mitochondrial Deficits Leads to Axon Degeneration and Neuropathy. Neuron 77 (5), 886–898. doi:10.1016/j.neuron.2013.01.012
Vlassara, H., Brownlee, M., and Cerami, A. (1984). Accumulation of Diabetic Rat Peripheral Nerve Myelin by Macrophages Increases with the Presence of Advanced Glycosylation Endproducts. J. Exp. Med. 160 (1), 197–207. doi:10.1084/jem.160.1.197
Wang, D., Couture, R., and Hong, Y. (2014). Activated Microglia in the Spinal Cord Underlies Diabetic Neuropathic Pain. Eur. J. Pharmacol. 728, 59–66. doi:10.1016/j.ejphar.2014.01.057
Wasan, A. D., Raskin, M. J., Wernicke, J. F., Robinson, M. J., Hall, J. A., et al. (2009). Safety and Efficacy of Duloxetine in the Treatment of Diabetic Peripheral Neuropathic Pain in Older Patients. Curr. Drug Saf. 4 (1), 22–29. doi:10.2174/157488609787354404
Wernicke, J. F., Pritchett, Y. L., D'Souza, D. N., Waninger, A., Tran, P., Iyengar, S., et al. (2006). A Randomized Controlled Trial of Duloxetine in Diabetic Peripheral Neuropathic Pain. Neurology 67 (8), 1411–1420. doi:10.1212/01.wnl.0000240225.04000.1a
Yamakawa, I., Kojima, H., Terashima, T., Katagi, M., Oi, J., Urabe, H., et al. (2011). Inactivation of TNF-α Ameliorates Diabetic Neuropathy in Mice. Am. J. Physiol. Endocrinol. Metab. 301 (5), E844–E852. doi:10.1152/ajpendo.00029.2011
Younger, D. S., Rosoklija, G., Hays, A. P., Trojaborg, W., and Latov, N. (2015). Diabetic Peripheral Neuropathy: A Clinicopathologic and Immunohistochemical Analysis of Sural Nerve Biopsies. Muscle Nerve 19 (6), 722–727. doi:10.1002/(SICI)1097-4598(199606)19:6<722:AID-MUS6>3.0.CO;2-C
Yuen, K. C., Baker, N. R., and Rayman, G. (2002). Treatment of Chronic Painful Diabetic Neuropathy with Isosorbide Dinitrate spray: a Double-Blind Placebo-Controlled Cross-Over Study. Diabetes Care 25 (10), 1699–1703. doi:10.2337/diacare.25.10.1699
Zhang, T. T., Xue, R., Fan, S. Y., Fan, Q. Y., An, L., Li, J., et al. (2018). Ammoxetine Attenuates Diabetic Neuropathic Pain through Inhibiting Microglial Activation and Neuroinflammation in the Spinal Cord. J. Neuroinflammation 15, 176. doi:10.1186/s12974-018-1216-3
Ziegler, D., Ametov, A., Barinov, A., Dyck, P. J., Gurieva, I., Low, P. A., et al. (2006). Oral Treatment with Alpha-Lipoic Acid Improves Symptomatic Diabetic Polyneuropathy: the SYDNEY 2 Trial. Diabetes Care 29 (11), 2365–2370. doi:10.2337/dc06-1216
Ziegler, D., Hanefeld, M., Ruhnau, K. J., Hasche, H., Lobisch, M., Schütte, K., et al. (1999). Treatment of Symptomatic Diabetic Polyneuropathy with the Antioxidant Alpha-Lipoic Acid: a 7-month Multicenter Randomized Controlled Trial (ALADIN III Study). Aladin III Study Group. Alpha-Lipoic Acid in Diabetic NeuropathyAlpha-Lipoic Acid in Diabetic Neuropathy. Diabetes Care 22 (8), 1296–1301. doi:10.2337/diacare.22.8.1296
Keywords: diabetic peripheral neuropathy, immune inflammation, therapy, immune cells, pathogenesis
Citation: Xue T, Zhang X, Xing Y, Liu S, Zhang L, Wang X and Yu M (2021) Advances About Immunoinflammatory Pathogenesis and Treatment in Diabetic Peripheral Neuropathy. Front. Pharmacol. 12:748193. doi: 10.3389/fphar.2021.748193
Received: 27 July 2021; Accepted: 20 September 2021;
Published: 04 October 2021.
Edited by:
Jian Gao, Shanghai Children’s Medical Center, ChinaReviewed by:
Zhongling Zhang, First Affiliated Hospital of Harbin Medical University, ChinaChunxue Wang, Beijing Tiantan Hospital, China
Copyright © 2021 Xue, Zhang, Xing, Liu, Zhang, Wang and Yu. This is an open-access article distributed under the terms of the Creative Commons Attribution License (CC BY). The use, distribution or reproduction in other forums is permitted, provided the original author(s) and the copyright owner(s) are credited and that the original publication in this journal is cited, in accordance with accepted academic practice. No use, distribution or reproduction is permitted which does not comply with these terms.
*Correspondence: Min Yu, eXVtaW4xOTk3QHNpbmEuY29t; Xun Wang, d2FuZ3h1bjE5ODBAMTI2LmNvbQ==; Lijun Zhang, bGlqdW56aGFuZ3dAZ21haWwuY29t
†These authors have contributed equally to this work