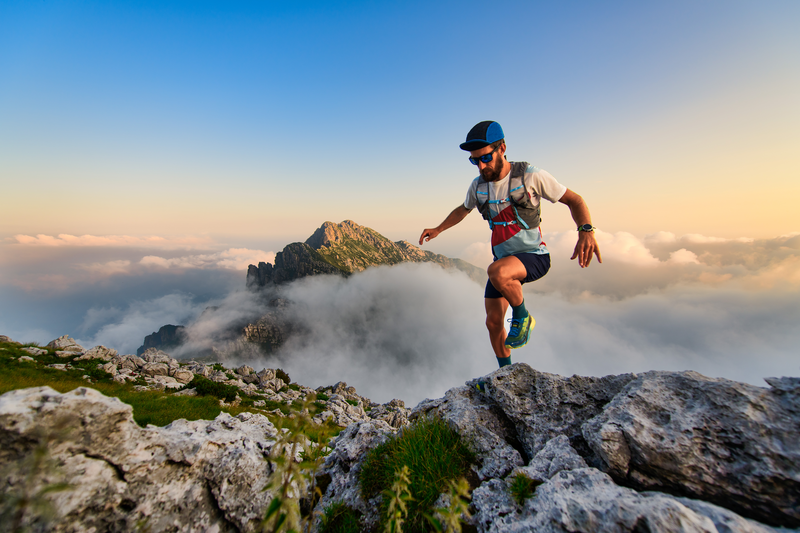
94% of researchers rate our articles as excellent or good
Learn more about the work of our research integrity team to safeguard the quality of each article we publish.
Find out more
ORIGINAL RESEARCH article
Front. Pharmacol. , 06 October 2021
Sec. Pharmacogenetics and Pharmacogenomics
Volume 12 - 2021 | https://doi.org/10.3389/fphar.2021.743494
This article is part of the Research Topic Insights in Pharmacogenetics and Pharmacogenomics: 2021 View all 11 articles
We investigated the association between genetic variations in pharmacodynamic genes and risperidone-induced increased prolactin levels in children and adolescents with autism spectrum disorder (ASD). In a retrospective study, variants of pharmacodynamic genes were analyzed in 124 ASD patients treated with a risperidone regimen for at least 3 months. To simplify genotype interpretation, we created an algorithm to calculate the dopamine D2 receptor (DRD2) gene genetic risk score. There was no relationship between prolactin levels and single SNPs. However, the H1/H3 diplotype (A2/A2-Cin/Cin-A/G) of DRD2/ankyrin repeat and kinase domain containing 1 (ANKK1) Taq1A, DRD2 -141C indel, and DRD2 -141A>G, which had a genetic risk score of 5.5, was associated with the highest median prolactin levels (23 ng/ml). As the dose-corrected plasma levels of risperidone, 9-OH-risperidone, and the active moiety increased, prolactin levels in patients carrying the H1/H3 diplotype were significantly higher than those of the other diplotypes. DRD2 diplotypes showed significantly high prolactin levels as plasma risperidone levels increased. Lower levels of prolactin were detected in patients who responded to risperidone. This is the first system for describing DRD2 haplotypes using genetic risk scores based on their protein expression. Clinicians should consider using pharmacogenetic-based decision-making in clinical practice to prevent prolactin increase.
Risperidone is an atypical antipsychotic used to treat autism spectrum disorder (ASD). Its effect is mediated via dopamine D2 receptor and 5-hydroxytryptamine type 2A receptor antagonism (Corena-McLeod, 2015). The US Food and Drug Administration has approved risperidone for the treatment of irritability in children and adolescents aged 5–16 years with ASD (Goel et al., 2018). Risperidone has a more favorable safety and efficacy profile than typical antipsychotic drugs (Peuskens et al., 2014). Two clinical trials (McCracken et al., 2002; Shea et al., 2004) established the efficacy and tolerability of risperidone in patients with ASD and showed that risperidone significantly attenuated disruptive behaviors compared with the placebo over 8 weeks, as measured by a reduction in the irritability subscale of the aberrant behavior checklist (ABC) scores. The improvement in the risperidone treatment group was higher (56.9–64.0%) compared with that in the placebo group (14.1–31.0%) (McCracken et al., 2002; Shea et al., 2004).
Several studies (Baumann et al., 2004; Riedel et al., 2005) have explored the utility of plasma drug monitoring as a biomarker of treatment response. Therapeutic drug monitoring can improve the efficacy and safety of risperidone. Plasma levels of 20–60 ng/L of the active moiety lead to better clinical outcomes in adults with schizophrenia (Baumann et al., 2004; Nazirizadeh et al., 2010). Plasma levels of the active moiety in non-responders were significantly higher than in responders in a 6 weeks clinical trial of risperidone treatment in schizophrenia patients (Riedel et al., 2005). However, no therapeutic drug monitoring data are available for pediatric patients with ASD treated with risperidone.
There is considerable variation in the response to risperidone that may be explained, at least partly, by genetic variation in drug targets. A polymorphism in the dopamine D2 receptor (DRD2)/ankyrin repeat and kinase domain containing 1 (ANKK1) gene (often referred to as the Taq1A polymorphism; rs1800497) was associated with a clinical response in risperidone-treated ASD patients (Nuntamool et al., 2017). The DRD2/ANKK1 Taq1A A2 or C allele (Sukasem et al., 2016) have been associated with high dopamine receptor densities (Jonsson et al., 1999), which may contribute to blocking dopaminergic activity. DRD2 -241A>G (rs1799978), located in the 5′-promoter region of DRD2, may contribute to increased expression levels. (Nyman et al., 2009). DRD2 -141C insertion/insertion has been associated with high prolactin levels in antipsychotic-treated male schizophrenia (Zhang et al., 2011). Carriers of the dopamine D3 receptor Gly/Gly allele (rs6280) showed significantly better response rates compared with the Ser/Ser genotype in children with ASD (Firouzabadi et al., 2017). Genetic variation in the 5-hydroxytryptamine type 2A receptor (HTR2A), 5-hydroxytryptamine type 2C receptor (HTR2C), and ATP binding cassette subfamily B member 1 genes also contribute to clinical outcomes and are possible markers for predicting a positive response to risperidone therapy in ASD (Correia et al., 2010). Moreover, the dopamine transporter [DAT; also known as solute carrier family 6 member 3 (SLC6A3)] and serotonin transporter [5-hydroxytryptamine transporter-linked promoter region (5-HTTLPR) also known as solute carrier family 6 member 4] genes, although not directly targeted by antipsychotic medications, may influence neurotransmitter availability, and thus contribute to the variability in treatment response (Kirchheiner et al., 2007; Porcelli et al., 2012; Outhred et al., 2016).
The anterior pituitary hormone prolactin has essential physiological functions in the brain. Prolactin acts as a neuropeptide, regulating neuroendocrine and emotional stress responses (Torner, 2016). Serum prolactin levels may also reflect the antipsychotic treatment response. Several clinical studies (Zhang et al., 2002; Ates et al., 2015; Stern et al., 2018) revealed that prolactin may mediate effects on the neuropsychiatric response to risperidone. Zhang et al. (2002) observed a significant positive relationship between the reduction rate of positive subscale scores of the Positive and Negative Syndrome Scale (PANSS) and the change in prolactin levels before and after treatment in chronic schizophrenia. Ates et al. (2015) found that in patients with hyperprolactinemia, the PANSS negative symptom scores were significantly higher than in patients without hyperprolactinemia (p = 0.041). Furthermore, several moderators and mediators affecting risperidone response have been described (Stern et al., 2018), and lower baseline levels of prolactin predict responder status in autistic children. Additionally, genetic polymorphisms in the prolactin (PRL) (Lee et al., 2007; Ivanova et al., 2017) and prolactin receptor (PRLR) (Lee et al., 2007) genes may also contribute to increased prolactin levels. The strongest association was between a single SNP of PRL (A>T, rs2244502) and prolactin levels, which showed higher prolactin levels in T carriers than in A carriers. Patients with hyperprolactinemia carried the G/G genotype of −1149 G>T (rs1341239) in the PRL gene more frequently than patients without hyperprolactinemia (p = 0.009) (Ivanova et al., 2017). Therefore, prolactin is a promising candidate biomarker for risperidone response.
The impact of pharmacogenetics on increased prolactin levels has not been well investigated. Stern et al. (2018) reported the effect of hyperprolactinemia in pediatric ASD patients with disruptive behaviors undergoing risperidone therapy. Therefore, in this study we investigated the association between genetic variations in pharmacodynamic genes and risperidone-induced increases in prolactin levels in children and adolescents with ASD.
ASD children and adolescents aged 3–18 years were recruited at the Yuwaprasart Waithayopathum Child Psychiatric Hospital, Samut Prakan, Thailand, in 2017 and 2018. All participants were ethnic Thai. The clinical neuropsychiatric diagnosis was made according to the Diagnostic and Statistical Manual of Mental Disorders, Fifth Edition criteria. The Ethics Committee of the Faculty of Medicine Ramathibodi Hospital, Bangkok, Thailand (MURA2017/556) and Yuwaprasart Waithayopathum Child Psychiatric Hospital, Samut Prakan, Thailand approved the study. All participants or parents of the children signed an informed assent or consent after the study objectives and procedures were explained. Sociodemographic data (gender, age at assessment, daily risperidone dosage, duration of risperidone treatment, and concomitant medication) were collected with a questionnaire. Patients were excluded if they were receiving concomitant medication that could affect risperidone metabolism (e.g., haloperidol, fluoxetine, paroxetine, carbamazepine, and phenytoin) or prolactin levels (e.g., haloperidol, sertraline, and fluoxetine).
The retrospective study included 124 ASD patients treated with a risperidone-based regimen for at least 3 months. Serum prolactin levels, plasma levels of risperidone, 9-OH-risperidone, and the active moiety were measured, and the candidate genes were genotyped. We also included 19 risperidone-naïve patients who underwent a baseline assessment before starting risperidone therapy. They were available for a follow-up assessment 3–20 months after risperidone treatment was started. At the first and follow-up visits, assessments were performed using ABC subscales and serum prolactin and plasma drug levels.
The ABC subscale assessment consisted of 58 items, divided into the following five categories of behavior: irritability, agitation, and crying (15 items); lethargy and social withdrawal (16 items); stereotypic behavior (7 items); hyperactivity and non-compliance (16 items); and inappropriate speech (4 items). The probands were rated by a primary patient’s caregiver for the different severity of behavior problems from zero (no problems) to three (severe problems), with higher scores indicating problems that were more severe (Narkpongphun and Charnsil, 2018b). The ABC-irritability subscale score is an accepted gold standard for measuring irritability and aggression in medication trials for ASD (Fung et al., 2016). We used the ABC-C Thai version, which was created with a cross-cultural adaptation method, has been validated, and is highly reliable (Narkpongphun and Charnsil, 2018a). Patients with ASD were divided into responders and non-responders according to the reduction rate of the total ABC scores. Patients classified as responders had a reduction rate of total ABC scores higher or equal to 30%, whereas patients with ABC score reduction rates of less than 30% were classified as non-responders.
A fasting morning blood sample was analyzed with a chemiluminescent immunoassay system (IMMULITE1000, Siemens Healthcare Diagnostics Products Ltd., Erlangen, Germany) at the Yuwaprasart Waithayopathum Child and Adolescent Psychiatric Hospital, Thailand.
Steady-state plasma concentrations of risperidone and 9-OH-risperidone were quantified, between 8:00 and 10:00 AM, approximately 12 h after the bedtime dose, using a validated high-performance liquid chromatography method (Puangpetch et al., 2016; Vanwong et al., 2016). The active moiety levels were calculated by summing the levels of risperidone and 9-OH-risperidone. Plasma drug levels of risperidone, 9-OH-risperidone, and active moiety were corrected by the daily dose to give the dose-corrected risperidone (RIS C/D), dose-corrected 9-OH-risperidone (9-OH-RIS C/D), and dose-corrected active moiety (active moiety C/D).
Genomic DNA was obtained from EDTA blood using the MagNa Pure automated extraction system according to the manufacturer’s instructions (Roche Diagnostics, Basel, Switzerland). The DNA samples were subsequently genotyped for the following sequence variations: DRD2/ANKK1Taq1A A2>A1 (rs1800497), DRD2 -141C indel (rs1799732), and -241A>G (rs1799978); HTR2A -1438G>A (rs6311); HTR2C -759C>T (rs3813929); and PRL 13096T>A (rs2244502), and PRLR 163444A>C (rs37364). SNPs were selected based on functional significance (Kirchheiner et al., 2007; Correia et al., 2010; Porcelli et al., 2012; Outhred et al., 2016; Firouzabadi et al., 2017; Nuntamool et al., 2017) and minor allele frequencies of >0.05 across the Asian population. All SNPs were genotyped using the commercially available TaqMan Drug Metabolism Genotyping assay (Life Technologies, Carlsbad, CA, United States). Genotyping was carried out as recommended by the manufacturer using a real-time PCR system (ViiA7, Applied Biosystems, Life Technologies).
To determine the number of variable tandem repeats (VNTRs) of DAT, 60 ng genomic DNA was amplified in 25 μl PCR reactions, containing 2X Green PCR master mix (12.5 μl, BiotechRabbit, Hennigsdorf, Germany), and 1 μl of each 10 μM primer (forward, 5′-TCCTTGCGGTGTAGGGAACG-3′; reverse 5′-CCAGGCAGAGTGTGGTCTG-3′). Denaturation at 95°C for 2 min was followed by 35 cycles of 95°C for 30 s, 65°C for 40 s, and 72°C for 1 min, and a final extension step at 72°C for 10 min. The fragment sizes were 263 bp (5 repeats), 423 bp (9 repeats), 463 bp (10 repeats), and 503 bp (11 repeats), respectively. For 5-HTTLPR, 8 µl PCR reactions contained 4 µl KAPA 2GTM Fast ReadyMix (KAPA Biosystems, Woburn, MA, United States), 0.6 μl of each 5 μM primer (forward, 5′-CACAAACATGCTCATTTAAGAAGTG-3′; reverse, 5′-AAAGGAAATAGCAGTGACAAGTTTG-3′), and 20 ng genomic DNA. PCR was initiated by a 2 min incubation at 95°C, which was followed by 40 cycles of 95°C for 15 s, 62°C for 40 s, and 72°C for 30 s, and a final extension at 72°C for 1 min. Amplicons representing the short (733 bp) and long (777 bp) alleles were separated by 2% agarose gel electrophoreses.
We created an algorithm to describe haplotypes with genetic risk scores. For protein expression affecting the prolactin level, the alleles DRD2 Taq1A, -141C indel, and -241A>G had values of 1 assigned for a high-expression allele (A2, Cin, G) and 0.5 for a low-expression allele (A1, Cdel, A) (Arinami et al., 1997; Jonsson et al., 1999; Nyman et al., 2009). The score of each haplotype was the sum of the values assigned to each allele. A high risk score indicated a high prolactin level.
Statistical analyses were carried out using SPSS v24 for Windows (IBM, Armonk, NY, United States). Statistical significance is reported as p < 0.05 for a two-tailed distribution. Descriptive analyses were performed for the sociodemographic variables. Data are expressed as mean (standard deviation; SD) or median (interquartile range; IQR) in normal or non-normal distribution data, respectively. Parametric analysis of variance (comparisons between more than two groups) and Student’s t-test (comparisons between two groups) were used to assess at each time point the association between prolactin or drug levels and the genotypes. The nonparametric Kruskal-Wallis (comparisons among more than two groups) and Mann-Whitney (comparisons between two groups) tests were used to assess the association between prolactin or drug levels and the genotypes at each time point. The nonparametric Spearman rank correlation test was used to measure the correlation between serum prolactin level and plasma drug level. Because nine variants were tested, the p-value significance threshold was adjusted for multiple comparisons. Bonferroni’s correction was applied to adjust for multiple comparisons. According to Bonferroni’s procedure, the corrected p-values were calculated by multiplying the p-values by 9 for the numbers of variants. The significance threshold of the corrected p-values was set as 0.05 (Yi et al., 2014). The Hardy-Weinberg equilibrium, allele, and genotype frequencies of all candidate SNPs were analyzed using Haploview v4.2 (Broad Institute, Cambridge, MA, United States). PHASE v2.1.1 was used to reconstruct haplotype pairs on the same chromosome (Stephens and Scheet, 2005). Fisher’s exact test was used to compare the difference in patient characteristics between responders and non-responders in children and adolescents with ASD. Differences in serum prolactin levels between the DRD2 diplotypes were assessed by analysis of covariance (ANCOVA), controlling for plasma drug level as a covariate. Receiver operating characteristic (ROC) curves were analyzed and plotted. Performance parameters such as sensitivity, specificity, positive predictive value (PPV), negative predictive value (NPV), and accuracy of the association between serum cut-off value of serum prolactin levels and status of risperidone response were analyzed using MedCalc (https://www.medcalc.org/calc/diagnostic_test.php).
Our sample cohort consisted of 124 children and adolescents with a mean age of 8.81 years (SD = 4.04). All patients were diagnosed with ASD and treated with risperidone. Seventy-four patients (59.68%) received risperidone monotherapy. The remaining patients received concomitant medications that did not affect cytochrome P450 2D6 metabolite and prolactin levels. Demographic data are summarized in Table 1.
Genotype frequencies of the tested polymorphisms are shown in Supplementary Table S1. Considering codominant, dominant, and recessive genetic models, there were no differences in serum prolactin levels for DRD2/ANKK1 Taq1A A2>A1, DRD2 -141C indel, DRD2 -241A>G, HTR2A-1438G>A, HTR2C -759C>T, PRL g.13096T>A, PRLR g.163444A>C, and the number of variable tandem repeats of DAT and 5-HTTLPR.
Haplotypes were constructed using three SNPs of DRD2 on chromosome 11 (DRD2/ANKK1 Taq1A A2/A1, DRD2 -141C indel, and -241A>G) with PHASE v2.1.1. Six haplotypes with minor allelic frequencies of >1% were identified. The four main haplotypes were A2-Cin-A (H1), A1-Cin-A (H2), A2-Cin-G (H3), and A2-Cdel-A (H4), accounting for 91.4% of the observations (Table 2). Fifteen diplotypes accounted for 99.2% of the observations (Table 3). We compared the association of each pair of diplotype groups. Participants with the H1/H3 diplotype showed significantly higher prolactin levels than those of other diplotypes (23.00 ng/ml, p < 0.05).
We created an algorithm to describe a haplotype with a genetic risk score based on DRD2 expression of protein affecting the prolactin level. Forty-five (36.29%) patients had a common risk score of 4.5 and had a median prolactin level of 16.60 ng/ml. We compared the common risk score and found that prolactin levels in patients with a common genetic risk score of 4.5 (n = 45, 36.29%) were significantly lower than those with a genetic risk score of 5.5 (n = 12, 9.68%) (16.60 vs. 23.00 ng/ml, p = 0.033). Thus, higher DRD2 expression was related to higher prolactin levels (Table 4). However, there were high prolactin levels at the lowest and highest risk score (21.80 ng/ml at a risk score of 3.5 and 28.20 ng/ml at a risk score of 6, respectively), but there were no significant differences (p > 0.05).
TABLE 4. Associations between genetic risk scores for DRD2 gene haplotypes and serum prolactin levels.
There was no association between any of the interrogated sequence variations in the candidate pharmacodynamic genes and the risperidone response (Supplementary Table S2).
Plasma levels of risperidone, 9-OH-risperidone, and the active moiety were corrected with the daily dose. Serum prolactin levels were significantly correlated among RIS C/D (rs = 0.227, p = 0.012), 9-OH-RIS C/D (rs = 0.305, p = 0.001), and active moiety C/D (rs = 0.343, p < 0.001).
We conducted ANCOVA to compare the effect of DRD2 diplotypes on prolactin levels, using RIS C/D, 9-OH-RIS C/D, and active moiety C/D as covariates to adjust for possible confounding factors. Serum levels of prolactin were significantly higher in the H1/H3 diplotype group compared with the H1/H2 (Figure 1A; F = 5.420, p = 0.026), H1/H5 (Figure 1B; F = 4.552, p = 0.042), H1/H6 (Figure 1C; F = 4.848, p = 0.037), and H2/H2 (Figure 1D; F = 5.761, p = 0.027) groups after controlling for plasma drug levels of active moiety C/D.
FIGURE 1. Relationship between prolactin and active moiety C/D plasma levels in children and adolescents with DRD2 diplotypes of (A) H1/H3 and H1/H2 (p = 0.026), (B) H1/H3 and H1/H5 (p = 0.042), (C) H1/H3 and H1/H6 (p = 0.037), and (D) H1/H3 and H2/H2 (p = 0.027). The black line represents the linear relationship between prolactin and drug levels in patients carrying an H1/H3 or other diplotype.
A total of 124 participants were enrolled in this study; however, only 19 patients were available for naïve and follow-up after being treated for a minimum of 3 months. The mean age at baseline of the 19 patients was 5.21 years (SD 2.82), and most were male (n = 16, 84.21%). Table 5 summarizes the findings for the 19 patients by responder status according to the percentage decrease in the total ABC score. Of these, 53% (n = 10) responded to risperidone treatment, whereas 47% (n = 9) were non-responders. The mean age of responders was 5.50 ± 2.84 years and that of non-responders was 4.89 ± 2.93 years. Median prolactin levels after treatment in non-responders were higher compared with those in responders (20.10 vs. 10.25 ng/ml, p = 0.013). ABC subscale scores after 3 months were significantly lower than those before treatment (paired t-test; p < 0.05). Plasma levels of RIS C/D, 9-OH-RIS C/D, and active moiety C/D were not significantly different between responders and non-responders. Moreover, there was no significant difference in prolactin levels before and after treatment in responders (7.65 vs. 10.25 ng/ml, p = 0.878). In contrast, the prolactin level in non-responders after risperidone treatment was about twice that in responders (9.40 vs. 20.10 ng/ml, p = 0.028).
TABLE 5. ABC score and serum prolactin levels at baseline and after 3 months of treatment between responders and non-responders (n = 19).
The ROC curve showed that serum prolactin levels predicted the risperidone response (Figure 2 and Table 6). The area under the ROC curve for prolactin was 0.833 (p = 0.014), which was above the acceptable accuracy level of 0.8. The prolactin cut-off value with sensitivity and specificity of 0.5 or greater was 10.25–18.85 ng/ml, with an optimal prolactin cut-off value of 10.9 ng/ml (sensitivity, 100%; specificity, 60%; PPV, 69.23%; NPV, 100.00%; highest accuracy, 78.95%). The second accuracy for serum prolactin levels of 10.25, 12.20, 15.0, and 16.8 ng/ml was 73.68%.
FIGURE 2. ROC curve analysis showing that serum prolactin level has a high accuracy (68–78%) for identifying responders and non-responders.
TABLE 6. Sensitivity, specificity, and accuracy of prolactin levels predicting risperidone response.
Several studies have reported that genetic variation (Young et al., 2004; Calarge et al., 2009; Yasui-Furukori et al., 2011) or plasma drug levels of risperidone and its metabolite (Knegtering et al., 2005; Melkersson, 2006; Ngamsamut et al., 2016) are related to hyperprolactinemia. However, there are no studies on the relationship between prolactin elevation and genetic variation, or whether controlling plasma drug levels of RIS C/D, 9-OH-RIS C/D, and active moiety C/D can prevent prolactin elevation. The findings of the present study suggest that the patients with the H1/H3 haplotype of DRD2 were at higher risk than patients with other diplotypes of presenting with significantly higher prolactin levels with increasing plasma RIS C/D, 9-OH-RIS C/D, and active moiety C/D. Furthermore, low prolactin levels were associated with response to risperidone treatment in Thai ASD children and adolescents.
Hyperprolactinemia is commonly observed in patients treated with antipsychotics. In our study, 44.35% (55 of 124) of patients had high prolactin levels, which is a concern for adverse drug reactions in children and adolescents treated with risperidone. The reported prevalence of hyperprolactinemia in patients on risperidone ranges between 44 and 61% (Hongkaew et al., 2015; An et al., 2016; Bonete Llacer et al., 2019), and abnormal prolactin levels occur in about 27% of patients taking risperidone (Lally et al., 2017). Hypothalamic dopamine inhibits prolactin secretion by acting on dopamine D2 receptors, which inhibits lactotroph cells (Ben-Jonathan and Hnasko, 2001). Hypothalamic dopamine inhibits pituitary prolactin secretion and proliferation of prolactin-producing lactotroph cells by activating lactotroph dopamine D2 receptors (Ben-Jonathan and Hnasko, 2001; Fitzgerald and Dinan, 2008). There are several hypotheses for why prolactin elevation occurs during treatment with certain antipsychotics. Risperidone may cause high prolactin levels because of its slow dissociation from dopamine D2 receptors, which increases central dopamine D2 receptor occupancy (Peuskens et al., 2014), resulting in a prolonged blockade and higher rates of prolactin release. Risperidone is also associated with weaker blood-brain barrier penetration (Peuskens et al., 2014) compared with olanzapine and quetiapine (Arakawa et al., 2010), resulting in greater dopamine D2 receptor occupancy in the pituitary area, which also increases prolactin levels.
We also investigated whether DRD2 haplotypes are more informative than each SNP on its own, and the H1/H3 diplotype had the highest serum prolactin levels. These findings are consistent with increased prolactin levels in patients carrying the DRD2/ANKK1 Taq1A A2 or C allele (Sukasem et al., 2016). These alleles are associated with high dopamine receptor densities (Jonsson et al., 1999), which may contribute to blocking dopaminergic activity. DRD2 -241A>G (rs1799978), which is located in the 5′-promoter region of DRD2, may contribute to increased expression levels. Data obtained with lymphoblastoid cell lines suggested that -241G carriers have higher DRD2 expression levels than non-carriers (Nyman et al., 2009), which is consistent with our conclusion that DRD2 -241A>G is involved in hyperprolactinemia. Our results are also consistent with previous findings. Calarge et al. (2009) found that the -241G allele had the largest effect on serum prolactin levels when combined with the Taq1A A1 allele, whereas Arinami et al. (1997) reported an association between the DRD2 -141C indel and high DRD2 expression levels in vitro. Stronger binding of risperidone to dopamine D2 receptors in the pituitary area is more likely to inhibit dopaminergic activity in prolactin secretion (Peuskens et al., 2014). Therefore, the findings of these studies support our observation that increased DRD2 expression levels, mediated by the DRD2 -141C indel, contribute to the increase in prolactin levels. Our finding is also consistent with a study describing antipsychotic-induced sexual dysfunction in male schizophrenia, which was attributed to high prolactin levels in the presence of the DRD2 -141C indel (Zhang et al., 2011). Therefore, the synergistic effects of the DRD2/ANKK1 Taq1A A2>A1, DRD2 -141C indel, and -241A>G SNPs may be better captured by the association of haplotypes, rather than SNPs, with serum prolactin levels. Based on our results, we suggest that clinicians should perform DNA analysis of the three SNPs of the DRD2 haplotype as pre-emptive genetic testing, and prolactin levels should be monitored before and after 3 months to prevent hyperprolactinemia, especially in patients with the H1/H3 diplotype.
We developed an algorithm to categorize DRD2 haplotypes simply according to their DRD2 expression (Arinami et al., 1997; Jonsson et al., 1999; Nyman et al., 2009). High-expression alleles (A2, Cin, G) were assigned a score of 1 and low-expression alleles (A1, Cdel, A) were assigned a score of 0.5. The score of each haplotype was the sum of the values of each allele. A high risk score was expected to indicate a high prolactin level. In this study, a higher DRD2 expression score was significantly related to a higher prolactin level. The increase in prolactin levels with risperidone can be explained by risperidone inhibiting dopamine binding to dopamine D2 receptors (Rosenbloom, 2010). The higher the number of dopamine receptors is, the greater the inhibition by risperidone, and the higher the increase in prolactin. Thus, high prolactin levels occurred at the highest risk score of 6. However, this study also observed the high prolactin level at the lowest risk score of 3.5. This could be explained by the upregulation of dopamine receptors in patients with low DRD2 expression and who received long-term risperidone treatment. Lidow and Goldman-Rakic (1997) found that 6 months treatment with antipsychotics upregulated prefrontal and temporal cortical dopamine D2 receptor mRNA expression in primates. However, there was no significant difference in expression due to the small sample size. The genetic risk score system is an easy-to-use tool for translating genotype data into DRD2 expression prediction in a clinical setting. However, further study is needed to validate this tool.
Because variations in the HTR2A gene (encoding the 5-hydroxytryptamine type 2A receptor) and HTR2C gene (encoding the 5-hydroxytryptamine type 2C receptor) influence the binding affinities of antipsychotic medication, in previous studies, two genetic polymorphisms related to hyperprolactinemia in schizophrenic patients treated with classical and/or atypical antipsychotic treatment have been analyzed. Several studies found contradictory associations between hyperprolactinemia and HTR2A (Correia et al., 2010; Ivanova et al., 2017) and HTR2C polymorphisms (Alladi et al., 2017; Koller et al., 2020). Our study was consistent with a study of risperidone in 289 Indian schizophrenia patients (Alladi et al., 2017). They reported no association between HTR2C (−759 C>T) genetic variants and prolactin levels during risperidone treatment. Genetic polymorphisms in the promoter region of the HTR2C gene (−759 C>T) have been investigated in antipsychotic drug-induced weight gain (Vanwong et al., 2021). Moreover, transporter and prolactin-related genes involving the neurotransmitter mechanism of prolactin secretion were studied. Osmanova et al. (2019) found an association between two variants in the DAT (SLC6A3) gene and hyperprolactinemia in the subgroup of patients taking risperidone/paliperidone in 446 Caucasian schizophrenia patients. Smith et al. (2004) reported that the short allele of the 5-HTTLPR indel polymorphism was associated with less of an increase in prolactin and cortisol than the long/long genotype in control participants. Lee et al. (2007) found a nominally significant association between PRL and PRLR tagSNPs and plasma prolactin levels in 95 advanced breast cancer cases. However, the results were inconsistent because of the different underlying disease groups and treatments. Thus, until our results are validated in a prospective study, they should not be applied to patients with other diseases or undergoing other treatments.
There were no significant differences between any genetic variants selected and responses to risperidone in this study. Thus, although the DRD2 haplotypes relate to prolactin increase, they are likely to be predictive for poor markers for treatment response. Our results agree with Sakumoto et al. (2007), who found that non-responders without the deletion allele of the DRD2 -141C indel showed a higher score for psychiatric, extrapyramidal, and total side-effects than those with the deletion allele after 3 weeks of treatment with dopamine antagonists. The small number of 19 patients in our study cohort may explain why there were no significant differences among the associations between genetic variants and treatment response.
Several studies have examined optimizing risperidone dosing in children and adolescents based on their clinical response (Research Units on Pediatric Psychopharmacology Autism, 2005; Haas et al., 2009; Pozzi et al., 2016). We found no significant association between plasma drug levels and risperidone response due to the small sample size, although non-responders had numerically higher drug plasma levels than responders, which is consistent with other published work (Wang et al., 2007; Lostia et al., 2009). However, our results contradict other studies that show a relationship between therapeutic drug levels and clinical efficacy (Baumann et al., 2004; Riedel et al., 2005). This discrepancy may be a result of the different underlying disease in patients with schizophrenia. In addition, there may be differences in the timing of the clinical outcome assessment and the sociodemographic profile of the patient population among studies. We performed our assessment at least 3 months into risperidone treatment rather than at 1–6 weeks because prolactin levels in children receiving long-term risperidone tend to peak within the first 2 months, and then steadily decline to values within or close to normal after 3–5 months (Findling et al., 2003). Although we did not discover an association between plasma drug concentration and efficacy, the association between plasma drug and serum prolactin levels remained significant. Therefore, monitoring risperidone plasma levels may help to prevent adverse drug reactions, such as hyperprolactinemia.
Although other studies have found that prolactin levels are inconsistently associated with efficacy (Wang et al., 2007; Lostia et al., 2009), our study revealed that prolactin levels may induce changes in neurogenesis, potentially affecting aberrant behaviors in ASD, consistent with several other studies (Zhang et al., 2002; Lee and Kim, 2006; Ates et al., 2015). Prolactin is an anterior pituitary peptide hormone, under inhibitory control by dopamine released from the tuberoinfundibular dopaminergic neurons (Grattan, 2015). Because of dopamine regulation of serum prolactin levels through dopamine D2 receptors in the hypothalamic-pituitary pathway, the serum prolactin levels may be a marker of central dopamine function. Risperidone could induce hyperprolactinemia in excessive dopamine receptor blockade (Oda et al., 2015) due to its high dopamine D2 receptor occupancy (68–70%) (Tsuboi et al., 2013), resulting in hyperprolactinemia in early treatment. Long-term treatment with antipsychotics could lead to increased dopamine D2 receptor density according to the dopamine supersensitivity psychosis hypothesis (Oda et al., 2015), and thus could cause adequate blockade, leading to behavioral improvement. This hypothesis may explain why some ASD patients had increased prolactin levels early in risperidone treatment and subsequently presented with improved symptoms.
The association between high prolactin and worse response could be explained by the brain/plasma concentration ratio of risperidone. Arakawa et al. (2010) reported that risperidone showed a slightly higher brain/plasma concentration ratio. The brain/plasma concentration ratio was calculated from dopamine D2 receptor occupancy in the temporal cortex and pituitary, which represented the permeability of antipsychotics into the brain. The brain/plasma concentration ratio of risperidone was 1.61 (Arakawa et al., 2010), indicating that the risperidone concentration in the temporal cortex was 1.5-fold greater than that in the pituitary. The permeability of risperidone into the brain resulted in a higher concentration in the brain than in plasma. Dopamine D2 receptor occupancy of risperidone in the temporal cortex was higher than that in the pituitary, causing an improvement in behaviors and reducing the risk of prolactin elevation. Therefore, our results suggest that low prolactin levels are an indicator of response to risperidone, and thus serum prolactin levels could be used as an indirect biomarker for risperidone response.
The present study is the first to report a cut-off value for prolactin-related poor behavioral responses of children treated with risperidone of less than 1 mg/day. The prediction of risperidone response in ASD had an area under the ROC curve of 0.833. The cut-off point of the prolactin level associated with sensitivity and specificity of 0.5 or higher was 10.25–18.85 ng/ml, and the optimal cut-off value for prolactin of 10.9 ng/ml had the highest accuracy of 78.95%. We applied the prolactin measurement as a biomarker for unusual behaviors in patients who did not respond to risperidone treatment. It might be used as a screening test to exclude false negatives. When NPV was 100%, meaning that when the test was negative (low prolactin value), the patient was responding to treatment, when the PPV was 60%, meaning that when the test was positive (high prolactin value) of 100 patients, only 60 patients were not responding to treatment. This information would be beneficial to health practitioners in clinical practice. However, further studies are needed to determine the optimal daily dose of risperidone for prolactin monitoring.
There are several limitations to this study. The small sample size in the cohort study prevented us from detecting significant genotype-phenotype associations, and thus the results should be viewed with caution. No relationship was observed for risperidone dose, weight-adjusted dose, and treatment duration between responders and non-responders. However, the study used cohorts under clinical conditions and did not control for risperidone dose and treatment duration.
We created a system for representing the expression of DRD2 haplotypes using genetic risk scores. Our findings suggest that patients with the H1/H3 diplotype, which had a DRD2 genetic risk score of 5.5, showed the highest serum prolactin levels, correlated with increasing plasma RIS C/D, 9-OH-risperidone C/D, and active moiety C/D. Lower levels of prolactin were detected in patients who responded to risperidone. These findings further our understanding of hyperprolactinemia, which is a commonly observed adverse effect of risperidone treatment. Preemptive pharmacogenetic testing may allow clinicians to identify patients at risk of developing high prolactin levels at the outset of therapy and use this information to guide therapeutic decision-making.
The original contributions presented in the study are included in the article/Supplementary Material, further inquiries can be directed to the corresponding author.
The studies involving human participants were reviewed and approved by the Faculty of Medicine Ramathibodi Hospital, Bangkok, Thailand (MURA 2017/556) and Yuwaprasart Waithayopathum Child Psychiatric Hospital, Samut Prakan, Thailand. Written informed consent to participate in this study was provided by the participants’ legal guardian/next of kin.
YH, AG, BW, and CS conceptualized the study; YH, RG, AP, and RS analyzed the data; WK, NN, and PL collected the samples YH wrote the original draft; YH, AG, BW, and CS reviewed and revised the paper. All authors have read and agreed to the published version of the article.
This study was supported by grants of the 1) the Thailand Research Fund through the Royal Golden Jubilee Ph.D. Program (Grant No. PHD/0107/2557), 2) Office of National Research Council of Thailand, 3) Pharmacogenomics for Autistic Child Project, Khoon Poom Foundation, The Project of Her Royal Highness Princess Ubonratana Rajakanya Siriwatana Bhanawadee, 4) Faculty of Medicine Ramathibodi Hospital and 5) Mahidol University.
The authors declare that the research was conducted in the absence of any commercial or financial relationships that could be construed as a potential conflict of interest.
All claims expressed in this article are solely those of the authors and do not necessarily represent those of their affiliated organizations, or those of the publisher, the editors and the reviewers. Any product that may be evaluated in this article, or claim that may be made by its manufacturer, is not guaranteed or endorsed by the publisher.
Financial support from the Thailand Research Fund through the Royal Golden Jubilee Ph.D. Program (Grant No. PHD/0107/2557) to YH and CS is acknowledged. The authors thank all the staff in Yuwaprasart Waithayopathum Child and Adolescent Psychiatric Hospital and all the children and adolescents with ASD who participated in the study.
The Supplementary Material for this article can be found online at: https://www.frontiersin.org/articles/10.3389/fphar.2021.743494/full#supplementary-material
A, N., and C, C. (2018a). Reliability and Validity of Aberrant Behavior Checklist-Community, Thai Version. J. Child. Adolesc. Behav. 06, 1–4. doi:10.4172/2375-4494.1000373
Alladi, C. G., Mohan, A., Shewade, D. G., Rajkumar, R. P., Adithan, S., and Subramanian, K. (2017). Risperidone-Induced Adverse Drug Reactions and Role of DRD2 (-141 C Ins/Del) and 5HTR2C (-759 C>T) Genetic Polymorphisms in Patients with Schizophrenia. J. Pharmacol. Pharmacother. 8, 28–32. doi:10.4103/jpp.JPP_197_16
An, F. R., Yang, R., Wang, Z. M., Ungvari, G. S., Ng, C. H., Chiu, H. F., et al. (2016). Hyperprolactinemia, Prolactin-Related Side Effects and Quality of Life in Chinese Psychiatric Patients. Compr. Psychiatry 71, 71–76. doi:10.1016/j.comppsych.2016.08.009
Arakawa, R., Okumura, M., Ito, H., Takano, A., Takahashi, H., Takano, H., et al. (2010). Positron Emission Tomography Measurement of Dopamine D₂ Receptor Occupancy in the Pituitary and Cerebral Cortex: Relation to Antipsychotic-Induced Hyperprolactinemia. J. Clin. Psychiatry 71, 1131–1137. doi:10.4088/JCP.08m04307yel
Arinami, T., Gao, M., Hamaguchi, H., and Toru, M. (1997). A Functional Polymorphism in the Promoter Region of the Dopamine D2 Receptor Gene Is Associated with Schizophrenia. Hum. Mol. Genet. 6, 577–582. doi:10.1093/hmg/6.4.577
Ates, A., Oner, I., Tutuncu, R., Basoglu, C., Cetin, M., Balibey, H., et al. (2015). Relationship between Plasma Levels of Prolactin and the Severity of Negative Symptoms in Patients with Schizophrenia. Klinik Psikofarmakoloji Bülteni-Bulletin Clin. Psychopharmacol. 25, 27–37. doi:10.5455/bcp.20141212113905
Baumann, P., Hiemke, C., Ulrich, S., Eckermann, G., Gaertner, I., Gerlach, M., et al. (2004). The AGNP-TDM Expert Group Consensus Guidelines: Therapeutic Drug Monitoring in Psychiatry. Pharmacopsychiatry 37, 243–265. doi:10.1055/s-2004-832687
Ben-Jonathan, N., and Hnasko, R. (2001). Dopamine as a Prolactin (PRL) Inhibitor. Endocr. Rev. 22, 724–763. doi:10.1210/edrv.22.6.0451
Bonete Llácer, J. M., Martínez Hortelano, A., and Richart Albelda, B. (2019). Hyperprolactinemia in Psychotic Patients Treated in Monotherapy with Long-Acting Injectable Antipsychotics. Int. J. Psychiatry Clin. Pract. 23, 189–193. doi:10.1080/13651501.2019.1576905
Calarge, C. A., Ellingrod, V. L., Acion, L., Miller, D. D., Moline, J., Tansey, M. J., et al. (2009). Variants of the Dopamine D2 Receptor Gene and Risperidone-Induced Hyperprolactinemia in Children and Adolescents. Pharmacogenet Genomics 19, 373–382. doi:10.1097/FPC.0b013e328329a60f
Corena-McLeod, M. (2015). Comparative Pharmacology of Risperidone and Paliperidone. Drugs R. D 15, 163–174. doi:10.1007/s40268-015-0092-x
Correia, C. T., Almeida, J. P., Santos, P. E., Sequeira, A. F., Marques, C. E., Miguel, T. S., et al. (2010). Pharmacogenetics of Risperidone Therapy in Autism: Association Analysis of Eight Candidate Genes with Drug Efficacy and Adverse Drug Reactions. Pharmacogenomics J. 10, 418–430. doi:10.1038/tpj.2009.63
Findling, R. L., Kusumakar, V., Daneman, D., Moshang, T., De Smedt, G., and Binder, C. (2003). Prolactin Levels during Long-Term Risperidone Treatment in Children and Adolescents. J. Clin. Psychiatry 64, 1362–1369. doi:10.4088/jcp.v64n1113
Firouzabadi, N., Nazariat, A., and Zomorrodian, K. (2017). DRD3 Ser9Gly Polymorphism and its Influence on Risperidone Response in Autistic Children. J. Pharm. Pharm. Sci. 20, 445–452. doi:10.18433/J3H63T
Fitzgerald, P., and Dinan, T. G. (2008). Prolactin and Dopamine: what Is the Connection? A Review Article. J. Psychopharmacol. 22, 12–19. doi:10.1177/0269216307087148
Fung, L. K., Mahajan, R., Nozzolillo, A., Bernal, P., Krasner, A., Jo, B., et al. (2016). Pharmacologic Treatment of Severe Irritability and Problem Behaviors in Autism: A Systematic Review and Meta-Analysis. Pediatrics 137 Suppl 2 (Suppl. 2), S124–S135. doi:10.1542/peds.2015-2851K
Goel, R., Hong, J. S., Findling, R. L., and Ji, N. Y. (2018). An Update on Pharmacotherapy of Autism Spectrum Disorder in Children and Adolescents. Int. Rev. Psychiatry 30, 78–95. doi:10.1080/09540261.2018.1458706
Grattan, D. R. (2015). 60 YEARS of NEUROENDOCRINOLOGY: The Hypothalamo-Prolactin axis. J. Endocrinol. 226, T101–T122. doi:10.1530/JOE-15-0213
Haas, M., Unis, A. S., Armenteros, J., Copenhaver, M. D., Quiroz, J. A., and Kushner, S. F. (2009). A 6-week, Randomized, Double-Blind, Placebo-Controlled Study of the Efficacy and Safety of Risperidone in Adolescents with Schizophrenia. J. Child. Adolesc. Psychopharmacol. 19, 611–621. doi:10.1089/cap.2008.0144
Hongkaew, Y., Ngamsamut, N., Puangpetch, A., Vanwong, N., Srisawasdi, P., Chamnanphon, M., et al. (2015). Hyperprolactinemia in Thai Children and Adolescents with Autism Spectrum Disorder Treated with Risperidone. Neuropsychiatr. Dis. Treat. 11, 191–196. doi:10.2147/ndt.s76276
Ivanova, S. A., Osmanova, D. Z., Boiko, A. S., Pozhidaev, I. V., Freidin, M. B., Fedorenko, O. Y., et al. (2017). Prolactin Gene Polymorphism (-1149 G/T) Is Associated with Hyperprolactinemia in Patients with Schizophrenia Treated with Antipsychotics. Schizophr Res. 182, 110–114. doi:10.1016/j.schres.2016.10.029
Ivanova, S. A., Osmanova, D. Z., Freidin, M. B., Fedorenko, O. Y., Boiko, A. S., Pozhidaev, I. V., et al. (2017). Identification of 5-hydroxytryptamine Receptor Gene Polymorphisms Modulating Hyperprolactinaemia in Antipsychotic Drug-Treated Patients with Schizophrenia. World J. Biol. Psychiatry 18, 239–246. doi:10.1080/15622975.2016.1224926
Jönsson, E. G., Nöthen, M. M., Grünhage, F., Farde, L., Nakashima, Y., Propping, P., et al. (1999). Polymorphisms in the Dopamine D2 Receptor Gene and Their Relationships to Striatal Dopamine Receptor Density of Healthy Volunteers. Mol. Psychiatry 4, 290–296. doi:10.1038/sj.mp.4000532
Kirchheiner, J., Nickchen, K., Sasse, J., Bauer, M., Roots, I., and Brockmöller, J. (2007). A 40-basepair VNTR Polymorphism in the Dopamine Transporter (DAT1) Gene and the Rapid Response to Antidepressant Treatment. Pharmacogenomics J. 7, 48–55. doi:10.1038/sj.tpj.6500398
Knegtering, R., Baselmans, P., Castelein, S., Bosker, F., Bruggeman, R., and van den Bosch, R. J. (2005). Predominant Role of the 9-hydroxy Metabolite of Risperidone in Elevating Blood Prolactin Levels. Am. J. Psychiatry 162, 1010–1012. doi:10.1176/appi.ajp.162.5.1010
Koller, D., Belmonte, C., Saiz-Rodríguez, M., Zubiaur, P., Román, M., Ochoa, D., et al. (2020). Effects of Aripiprazole on Circadian Prolactin Secretion Related to Pharmacogenetics in Healthy Volunteers. Basic Clin. Pharmacol. Toxicol. 126, 236–246. doi:10.1111/bcpt.13323
Lally, J., Ajnakina, O., Stubbs, B., Williams, H. R., Colizzi, M., Carra, E., et al. (2017). Hyperprolactinaemia in First Episode Psychosis - A Longitudinal Assessment. Schizophr Res. 189, 117–125. doi:10.1016/j.schres.2017.07.037
Lee, B. H., and Kim, Y. K. (2006). The Relationship between Prolactin Response and Clinical Efficacy of Risperidone in Acute Psychotic Inpatients. Prog. Neuropsychopharmacol. Biol. Psychiatry 30, 658–662. doi:10.1016/j.pnpbp.2005.11.037
Lee, S. A., Haiman, C. A., Burtt, N. P., Pooler, L. C., Cheng, I., Kolonel, L. N., et al. (2007). A Comprehensive Analysis of Common Genetic Variation in Prolactin (PRL) and PRL Receptor (PRLR) Genes in Relation to Plasma Prolactin Levels and Breast Cancer Risk: the Multiethnic Cohort. BMC Med. Genet. 8, 72. doi:10.1186/1471-2350-8-72
Lidow, M. S., and Goldman-Rakic, P. S. (1997). Differential Regulation of D2 and D4 Dopamine Receptor mRNAs in the Primate Cerebral Cortex vs. Neostriatum: Effects of Chronic Treatment with Typical and Atypical Antipsychotic Drugs. J. Pharmacol. Exp. Ther. 283, 939–946.
Lostia, A. M., Mazzarini, L., Pacchiarotti, I., Lionetto, L., De Rossi, P., Sanna, L., et al. (2009). Serum Levels of Risperidone and its Metabolite, 9-hydroxyrisperidone: Correlation between Drug Concentration and Clinical Response. Ther. Drug Monit. 31, 475–481. doi:10.1097/FTD.0b013e3181aa4780
McCracken, J. T., McGough, J., Shah, B., Cronin, P., Hong, D., Aman, M. G., et al. (2002). Risperidone in Children with Autism and Serious Behavioral Problems. N. Engl. J. Med. 347, 314–321. doi:10.1056/NEJMoa013171
Melkersson, K. I. (2006). Prolactin Elevation of the Antipsychotic Risperidone Is Predominantly Related to its 9-hydroxy Metabolite. Hum. Psychopharmacol. 21, 529–532. doi:10.1002/hup.811
Narkpongphun, A., and Charnsil, C. (2018b). Reliability and Validity of Aberrant Behavior Checklist-Community, Thai Version. J. Child. Adolesc. Behav. 6, 373. doi:10.4172/2375-4494.1000373
Nazirizadeh, Y., Vogel, F., Bader, W., Haen, E., Pfuhlmann, B., Gründer, G., et al. (2010). Serum Concentrations of Paliperidone versus Risperidone and Clinical Effects. Eur. J. Clin. Pharmacol. 66, 797–803. doi:10.1007/s00228-010-0812-7
Ngamsamut, N., Hongkaew, Y., Vanwong, N., Srisawasdi, P., Puangpetch, A., Chamkrachangpada, B., et al. (2016). 9-Hydroxyrisperidone-Induced Hyperprolactinaemia in Thai Children and Adolescents with Autism Spectrum Disorder. Basic Clin. Pharmacol. Toxicol. 119, 267–272. doi:10.1111/bcpt.12570
Nuntamool, N., Ngamsamut, N., Vanwong, N., Puangpetch, A., Chamnanphon, M., Hongkaew, Y., et al. (2017). Pharmacogenomics and Efficacy of Risperidone Long-Term Treatment in Thai Autistic Children and Adolescents. Basic Clin. Pharmacol. Toxicol. 121, 316–324. doi:10.1111/bcpt.12803
Nyman, E. S., Loukola, A., Varilo, T., Ekelund, J., Veijola, J., Joukamaa, M., et al. (2009). Impact of the Dopamine Receptor Gene Family on Temperament Traits in a Population-Based Birth Cohort. Am. J. Med. Genet. B Neuropsychiatr. Genet. 150B, 854–865. doi:10.1002/ajmg.b.30908
Oda, Y., Kanahara, N., and Iyo, M. (2015). Alterations of Dopamine D2 Receptors and Related Receptor-Interacting Proteins in Schizophrenia: The Pivotal Position of Dopamine Supersensitivity Psychosis in Treatment-Resistant Schizophrenia. Int. J. Mol. Sci. 16, 30144–30163. doi:10.3390/ijms161226228
Osmanova, D. Z., Freidin, M. B., Fedorenko, O. Y., Pozhidaev, I. V., Boiko, A. S., Vyalova, N. M., et al. (2019). A Pharmacogenetic Study of Patients with Schizophrenia from West Siberia Gets Insight into Dopaminergic Mechanisms of Antipsychotic-Induced Hyperprolactinemia. BMC Med. Genet. 20, 47. doi:10.1186/s12881-019-0773-3
Outhred, T., Das, P., Dobson-Stone, C., Felmingham, K. L., Bryant, R. A., Nathan, P. J., et al. (2016). Impact of 5-HTTLPR on SSRI Serotonin Transporter Blockade during Emotion Regulation: A Preliminary fMRI Study. J. Affect Disord. 196, 11–19. doi:10.1016/j.jad.2016.02.019
Peuskens, J., Pani, L., Detraux, J., and De Hert, M. (2014). The Effects of Novel and Newly Approved Antipsychotics on Serum Prolactin Levels: a Comprehensive Review. CNS Drugs 28, 421–453. doi:10.1007/s40263-014-0157-3
Porcelli, S., Fabbri, C., and Serretti, A. (2012). Meta-analysis of Serotonin Transporter Gene Promoter Polymorphism (5-HTTLPR) Association with Antidepressant Efficacy. Eur. Neuropsychopharmacol. 22, 239–258. doi:10.1016/j.euroneuro.2011.10.003
Pozzi, M., Cattaneo, D., Baldelli, S., Fucile, S., Capuano, A., Bravaccio, C., et al. (2016). Therapeutic Drug Monitoring of Second-Generation Antipsychotics in Pediatric Patients: an Observational Study in Real-Life Settings. Eur. J. Clin. Pharmacol. 72, 285–293. doi:10.1007/s00228-015-1982-0
Puangpetch, A., Vanwong, N., Nuntamool, N., Hongkaew, Y., Chamnanphon, M., and Sukasem, C. (2016). CYP2D6 Polymorphisms and Their Influence on Risperidone Treatment. Pharmgenomics Pers Med. 9, 131–147. doi:10.2147/PGPM.S107772
Research Units on Pediatric Psychopharmacology Autism, Network (2005). Risperidone Treatment of Autistic Disorder: Longer-Term Benefits and Blinded Discontinuation after 6 Months. Am. J. Psychiatry 162, 1361–1369. doi:10.1176/appi.ajp.162.7.1361
Riedel, M., Schwarz, M. J., Strassnig, M., Spellmann, I., Müller-Arends, A., Weber, K., et al. (2005). Risperidone Plasma Levels, Clinical Response and Side-Effects. Eur. Arch. Psychiatry Clin. Neurosci. 255, 261–268. doi:10.1007/s00406-004-0556-4
Rosenbloom, A. L. (2010). Hyperprolactinemia with Antipsychotic Drugs in Children and Adolescents. Int. J. Pediatr. Endocrinol. 2010, 1–6. doi:10.1155/2010/159402
Sakumoto, N., Kondo, T., Mihara, K., Suzuki, A., and Yasui-Furukori, N. (2007). Dopamine D2 Receptor Gene Polymorphisms Predict Well the Response to Dopamine Antagonists at Therapeutic Dosages in Patients with Schizophrenia. Psychiatry Clin. Neurosci. 61, 174–180. doi:10.1111/j.1440-1819.2007.01633.x
Shea, S., Turgay, A., Carroll, A., Schulz, M., Orlik, H., Smith, I., et al. (2004). Risperidone in the Treatment of Disruptive Behavioral Symptoms in Children with Autistic and Other Pervasive Developmental Disorders. Pediatrics 114, e634–41. doi:10.1542/peds.2003-0264-F
Smith, G. S., Lotrich, F. E., Malhotra, A. K., Lee, A. T., Ma, Y., Kramer, E., et al. (2004). Effects of Serotonin Transporter Promoter Polymorphisms on Serotonin Function. Neuropsychopharmacology 29, 2226–2234. doi:10.1038/sj.npp.1300552
Stephens, M., and Scheet, P. (2005). Accounting for Decay of Linkage Disequilibrium in Haplotype Inference and Missing-Data Imputation. Am. J. Hum. Genet. 76, 449–462. doi:10.1086/428594
Stern, S., Linker, S., Vadodaria, K. C., Marchetto, M. C., and Gage, F. H. (2018). Prediction of Response to Drug Therapy in Psychiatric Disorders. Open Biol. 8. doi:10.1098/rsob.180031
Sukasem, C., Hongkaew, Y., Ngamsamut, N., Puangpetch, A., Vanwong, N., Chamnanphon, M., et al. (2016). Impact of Pharmacogenetic Markers of CYP2D6 and DRD2 on Prolactin Response in Risperidone-Treated Thai Children and Adolescents with Autism Spectrum Disorders. J. Clin. Psychopharmacol. 36, 141–146. doi:10.1097/JCP.0000000000000474
Torner, L. (2016). Actions of Prolactin in the Brain: From Physiological Adaptations to Stress and Neurogenesis to Psychopathology. Front. Endocrinol. (Lausanne) 7, 25. doi:10.3389/fendo.2016.00025
Tsuboi, T., Bies, R. R., Suzuki, T., Mamo, D. C., Pollock, B. G., Graff-Guerrero, A., et al. (2013). Hyperprolactinemia and Estimated Dopamine D2 Receptor Occupancy in Patients with Schizophrenia: Analysis of the CATIE Data. Prog. Neuropsychopharmacol. Biol. Psychiatry 45, 178–182. doi:10.1016/j.pnpbp.2013.05.010
Vanwong, N., Prommas, S., Hongkaew, Y., Nuntamool, N., Nakorn, C. N, et al. (2016). Development and Validation of Liquid Chromatography/Tandem Mass Spectrometry Analysis for Therapeutic Drug Monitoring of Risperidone and 9-Hydroxyrisperidone in Pediatric Patients With Autism Spectrum Disorders. J. Clin. Lab. Anal. 30, 1236–1246. doi:10.1002/jcla.22009
Vanwong, N., Puangpetch, A., Unaharassamee, W., Jiratjintana, N., Na Nakorn, C., Hongkaew, Y., et al. (2021). Effect of 5-HT2C Receptor Gene Polymorphism (HTR2C-759C/T) on Metabolic Adverse Effects in Thai Psychiatric Patients Treated with Risperidone. Pharmacoepidemiol. Drug Saf. 30, 806–813. doi:10.1002/pds.5224
Wang, L., Yu, L., Zhang, A. P., Fang, C., Du, J., Gu, N. F., et al. (2007). Serum Prolactin Levels, Plasma Risperidone Levels, Polymorphism of Cytochrome P450 2D6 and Clinical Response in Patients with Schizophrenia. J. Psychopharmacol. 21, 837–842. doi:10.1177/0269881107077357
Yasui-Furukori, N., Tsuchimine, S., Saito, M., Nakagami, T., Sugawara, N., Fujii, A., et al. (2011). Comparing the Influence of Dopamine D₂ Polymorphisms and Plasma Drug Concentrations on the Clinical Response to Risperidone. J. Clin. Psychopharmacol. 31, 633–637. doi:10.1097/JCP.0b013e31822c09a7
Yi, N., Xu, S., Lou, X. Y., and Mallick, H. (2014). Multiple Comparisons in Genetic Association Studies: a Hierarchical Modeling Approach. Stat. Appl. Genet. Mol. Biol. 13, 35–48. doi:10.1515/sagmb-2012-0040
Young, R. M., Lawford, B. R., Barnes, M., Burton, S. C., Ritchie, T., Ward, W. K., et al. (2004). Prolactin Levels in Antipsychotic Treatment of Patients with Schizophrenia Carrying the DRD2*A1 Allele. Br. J. Psychiatry 185, 147–151. doi:10.1192/bjp.185.2.147
Zhang, X. R., Zhang, Z. J., Zhu, R. X., Yuan, Y. G., Jenkins, T. A., and Reynolds, G. P. (2011). Sexual Dysfunction in Male Schizophrenia: Influence of Antipsychotic Drugs, Prolactin and Polymorphisms of the Dopamine D2 Receptor Genes. Pharmacogenomics 12, 1127–1136. doi:10.2217/pgs.11.46
Keywords: risperidone, prolactin, autism spectrum disorder, genetic risk score, dopamine D2 receptor (DRD2)
Citation: Hongkaew Y, Gaedigk A, Wilffert B, Gaedigk R, Kittitharaphan W, Ngamsamut N, Limsila P, Puangpetch A, Sukprasong R and Sukasem C (2021) Pharmacogenomics Factors Influencing the Effect of Risperidone on Prolactin Levels in Thai Pediatric Patients With Autism Spectrum Disorder. Front. Pharmacol. 12:743494. doi: 10.3389/fphar.2021.743494
Received: 18 July 2021; Accepted: 14 September 2021;
Published: 06 October 2021.
Edited by:
Elena García-Martín, University of Extremadura, SpainReviewed by:
Balram Chowbay, National Cancer Centre Singapore, SingaporeCopyright © 2021 Hongkaew, Gaedigk, Wilffert, Gaedigk, Kittitharaphan, Ngamsamut, Limsila, Puangpetch, Sukprasong and Sukasem. This is an open-access article distributed under the terms of the Creative Commons Attribution License (CC BY). The use, distribution or reproduction in other forums is permitted, provided the original author(s) and the copyright owner(s) are credited and that the original publication in this journal is cited, in accordance with accepted academic practice. No use, distribution or reproduction is permitted which does not comply with these terms.
*Correspondence: Chonlaphat Sukasem, Y2hvbmxhcGhhdC5zdWtAbWFoaWRvbC5hYy50aA==
Disclaimer: All claims expressed in this article are solely those of the authors and do not necessarily represent those of their affiliated organizations, or those of the publisher, the editors and the reviewers. Any product that may be evaluated in this article or claim that may be made by its manufacturer is not guaranteed or endorsed by the publisher.
Research integrity at Frontiers
Learn more about the work of our research integrity team to safeguard the quality of each article we publish.