- 1Department of Orthopedics, Naval Hospital of Eastern Theater, Zhoushan, China
- 2Department of Orthopedics, Changhai Hospital, Naval Medical University, Shanghai, China
- 3Department of Emergency, Affiliated Hospital of Jiangsu University, Jiangsu, China
- 4Department of Orthopedics, Naval Medical University, Shanghai, China
Background: Defects of articular cartilage represent a common condition that usually progresses to osteoarthritis with pain and dysfunction of the joint. Current treatment strategies have yielded limited success in these patients. Stem cells are emerging as a promising option for cartilage regeneration. We aim to summarize the developmental history of stem cells for cartilage regeneration and to analyse the relevant trends and hotspots.
Methods: We screened all relevant literature on stem cells for cartilage regeneration from Web of Science during 2010–2020 and analysed the research trends in this field by VOSviewer and CiteSpace. We also summarized previous clinical trials.
Results: We screened 1,011 publications. China contributed the largest number of publications (317, 31.36%) and citations (81,376, 48.61%). The United States achieved the highest H-index (39). Shanghai Jiao Tong University had the largest number of publications (34) among all full-time institutions. The Journal of Biomaterials and Stem Cell Research and Therapy published the largest number of studies on stem cells for cartilage regeneration (35). SEKIYA I and YANG F published the majority of articles in this field (14), while TOH WS was cited most frequently (740). Regarding clinical research on stem cells for cartilage regeneration, the keyword “double-blind” emerged in recent years, with an average year of 2018.75. In tissue engineering, the keyword “3D printing” appeared latest, with an average year of 2019.625. In biological studies, the key word “extracellular vesicles” appeared latest, with an average year of 2018.9091. The current research trend indicates that basic research is gradually transforming to tissue engineering. Clinical trials have confirmed the safety and feasibility of stem cells for cartilage regeneration.
Conclusion: Multiple scientific methods were employed to reveal productivity, collaborations, and research hotspots related to the use of stem cells for cartilage regeneration. 3D printing, extracellular vesicles, and double-blind clinical trials are research hotspots and are likely to be promising in the near future. Further studies are needed for to improve our understanding of this field, and clinical trials with larger sample sizes and longer follow-up periods are needed for clinical transformation.
1 Introduction
Articular cartilage (AC) is a layer of hyaline cartilage with unique viscoelastic characteristics. Its principal function is to provide a smooth, lubricated surface for articulation and to facilitate the transmission of loads with a low frictional coefficient which disperses the stress in the joint to protect it from defects (Sophia Fox et al., 2009). However, traumatic and degenerative injuries of AC and untreated cartilage injuries eventually progress to osteoarthritis (OA), which is the most common type of musculoskeletal disorder (Carballo et al., 2017). Articular cartilage has very poor healing capability; because of its avascular nature, mature chondrocytes have a weak ability to proliferate and produce sufficient extracellular matrix (ECM) to fill a defect (Le et al., 2020). A large number of studies have been carried out to simulate cartilage regeneration. Surgical techniques to treat focal chondral defects include marrow stimulation (MST) surgery, such as the current first-line treatment microfracture (MF) surgery. Although the resulting ‘fibrocartilage’ provides some symptomatic relief, it has substantially weakened mechanical properties compared with those of normal articular cartilage (Toh et al., 2010; Murphy et al., 2020). In comparison, cell-based regenerative therapy can provide a long-term solution, alleviate symptoms and ultimately delay OA progression. Autologous chondrocyte implantation (ACI) has been approved by the FDA and is currently in clinical practice. ACI requires isolation of mature chondrocytes and culture them in vitro, which inevitably has limitations such as limited cells, in vitro chondrocyte dedifferentiation, and donor-site morbidity caused by cartilage harvest(Zellner et al., 2017). The potential of stem cells to overcome the limitations of the current methods for cartilage regeneration has attracted widespread interest.
Human embryonic stem cells (hESCs) are considered to be a promising cell source for cartilage regeneration because of their unlimited self-renewal capacity and multidirectional differentiation potential (Toh et al., 2011). As the field of stem cells continues to expand, more stem cell types are being discovered. Currently, the majority of cartilage regeneration research focuses on MSCs, which can be obtained from mesenchymal tissues and have the capacity to proliferate and differentiate into chondrocytes (Li et al., 2018; Wang et al., 2019). Clinical trials are systematic studies conducted in humans and are indispensable for determining efficacy and safety. The effectiveness of clinical trials is a prerequisite for further clinical applications. Clinical trials focusing on the potential of stem cells in cartilage regeneration have also been carried out (Wells et al., 2020). Some types of stem cells have already entered clinical trials, among which the hUCB-MSC-based therapeutic product CARTISTEM has been approved by the Korean FDA for marketing. Regenerative strategies for AC are the focus of study, and the scientific community (as well as researchers, research institutions, etc.) is constantly exploring this field; therefore, it is urgent to summarize and analyse the trends of this field.
The literature is the carrier of scientific progress. Bibliometric analysis uses mathematical and statistical methods to quantitatively and quantitatively analyse publications in medical databases, revealing the development history, research focus and future trends of a certain field. In addition, bibliometric analysis can implement comparisons between different countries or institutions by analysing the quality and quantity of previous research (Baldiotti et al., 2020; Yan et al., 2020). As much progress has been made concerning the potential of stem cells in cartilage regeneration, the number of related papers has also increased drastically, but related bibliometric studies have not been performed. In this study, we analysed publications about the use of stem cells for cartilage regeneration in the Web of Science (WoS) database during 2010–2020. We conducted statistical analysis on the number of publications, keyword frequency, and citation frequency. We conducted qualitative and quantitative analyses of research from different countries, institutions, journals and authors. We also summarized previous clinical trials. We aim to map thematic trends and hotspots related to the capability of stem cells for cartilage regeneration, providing a reference for future research.
2 Material and Methods
2.1 Data Acquisition and Search Strategy
Web of Science is an important academic database worldwide that has been widely used in bibliometric analysis. We selected WoS as a data source for a comprehensive search on the utilization of stem cells for cartilage regeneration from 2010 to 2020. The search strategies were as follows: TS = (stem cell* OR SC) and (*cartilage or chondro*) and regenera* AND language: (English). The screening process is shown in Figure 1. Relevant clinical trial data were obtained from ClinicalTrials.gov (https://clinicaltrials.gov/), the keywords were the same, and the limited condition was “completed study”. All data were obtained online, and no ethical certification was required. All searches were conducted on November 27, 2020, to avoid bias related to database updates.
2.2 Data Collection
Two authors (Demeng Xia and Jianghong Wu) independently screened and extracted all data from the final included articles, including titles, keywords, authors, institutions, journals, dates of publication, countries/regions, citations, H-index, etc. We then conducted analysis with Microsoft Excel 2016, CiteSpace version V and VOSviewer.
2.3 Bibliometric Analysis
Relative research interest (RRI) is an indicator of activity in a certain field, and RRI is defined by weighted publications per year (WPPY) in a certain field and all weighted publications per year in PubMed (AWPPY). We used Excel to calculate the RRI and to display the time curve. The impact factor (IF) was acquired in the newest edition of the journal citation report (JCR). The visualization software programs VOSviewer and CiteSpace are widely used in bibliometric analysis. We employed VOSviewer software to perform coupling analysis of nations and institutions, co-citation analysis of journals, and co-occurrence of key words. The average appearing year (AAY) was used to describe the relative novelty of keywords. We employed CiteSpace mainly to perform co-citation analysis of references.
3 Result
3.1 Field Activity and Global Contributions
3.1.1 Field Activity Analysis
Depending on the inclusion criteria, a total of 1,011 articles related to stem cells for cartilage regeneration were included in the final analysis. According to the annual distribution of publications, although there was a slight decline in 2010–2011 (35–26 publications per year), 2014–2015 (65–46 publications per year) and 2018–2019 (166–146 publications per year), the overall trend of the publication output increased from 2010 to 2020 (34–271 publications per year). The trend of the RRI was similar, suggesting that the field of stem cells for cartilage regeneration is receiving more attention in general (Figure 2A).
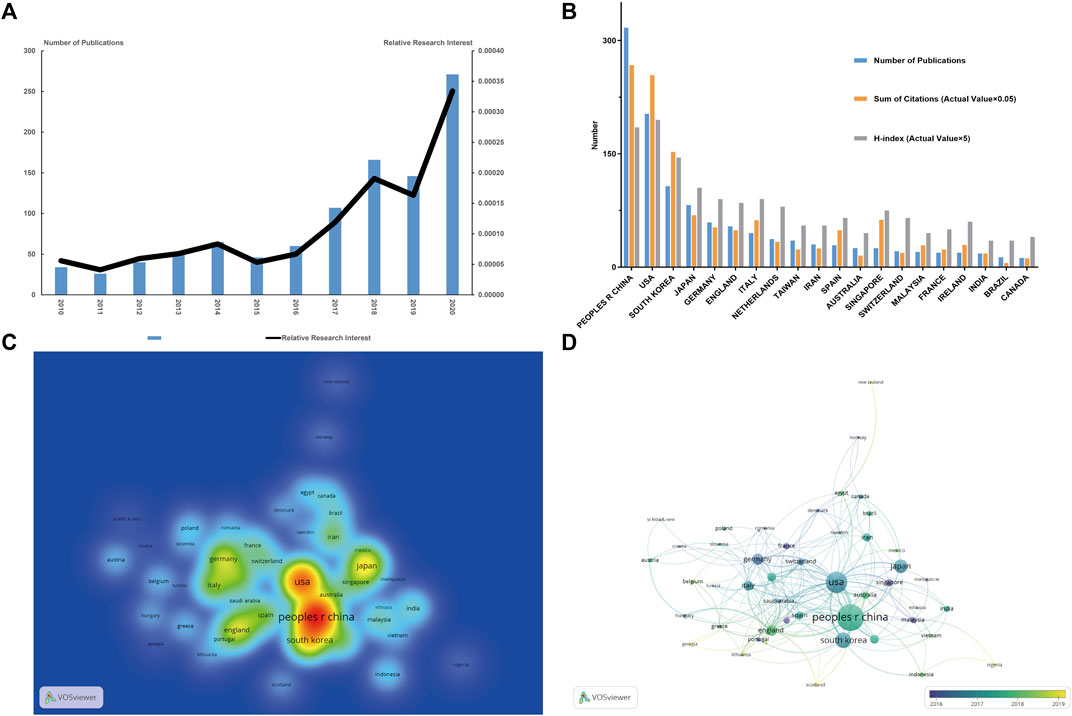
FIGURE 2. Articles related to stem cells for cartilage regeneration published worldwide. (A) Annual publications worldwide and the relative research interest (RRI) for stem cells for cartilage regeneration. (B) The number of publications, citation frequency (actual value × 0.05), and H-index (actual value × 5) in the top 20 countries or regions. (C) Density map of regional distribution of articles based on VOSviewer. (D) Collaboration between countries or regions based on VOSviewer.
3.1.2 Global Contribution to the Field
According to the national distribution of publications, China (317 publications) is the most productive country, followed by the United States (203 publications), South Korea (107 publications), Japan (82 publications) and Germany (59 publications) (Figure 2B). It is more intuitive to identify these publishing centres from the density map (Figure 2C). The top 20 countries with the most publications are listed in Table 1.
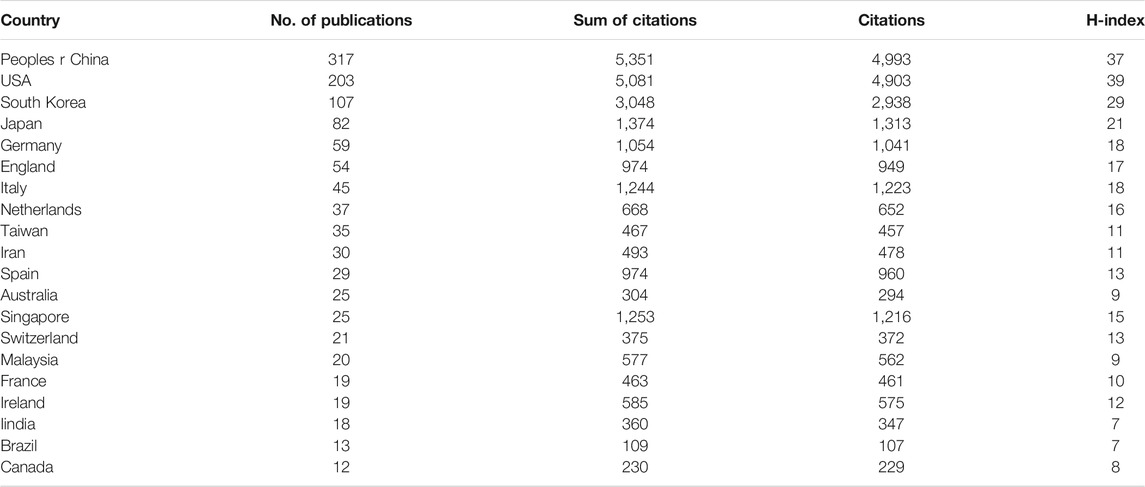
TABLE 1. The top 20 countries that contributed publications on stem cells for cartilage regeneration.
In terms of total citations, the top four countries are China (5,351 citations), the United States (5,081 citations), South Korea (3,048 citations) and Japan (1,374 citations). Singapore is not among in the top 10 productive countries (25 publications) but ranks fifth for total citations (1,253 citations). The countries with the highest H-index are as follows: first, the United States (39); second, China (37); third, South Korea (29); fourth, Japan (21); and fifth, Italy (18) (Figure 2B).
The collaborations between countries are shown in Figure 2D. The size of the circles indicates the number of publications, and the width of the connecting line between two circles indicates the degree of collaboration. The United States has the strongest total link strength, which means that the United States has the predominant influence in this field. Many countries have concentrated years of article output. France, Germany and Singapore had the most publications concentrated before 2016. Then, the U.S. and Japan intensively published articles during the next 2 years. Articles from England and mainland China were mainly published after 2018.
3.1.3 Analysis of Institution Distribution
The four institutions with the most publications are all located in China, and sequentially, they are Shanghai Jiao Tong University (34 publications), Peking University (27 publications), Sichuan University (26 publications), and Chinese PLA General Hospital (22 publications). The Chinese Academy of Science (21 publications) and National University of Singapore (21 publications) tied for fifth. The top 20 institutions with the most publications are listed in Table 2.
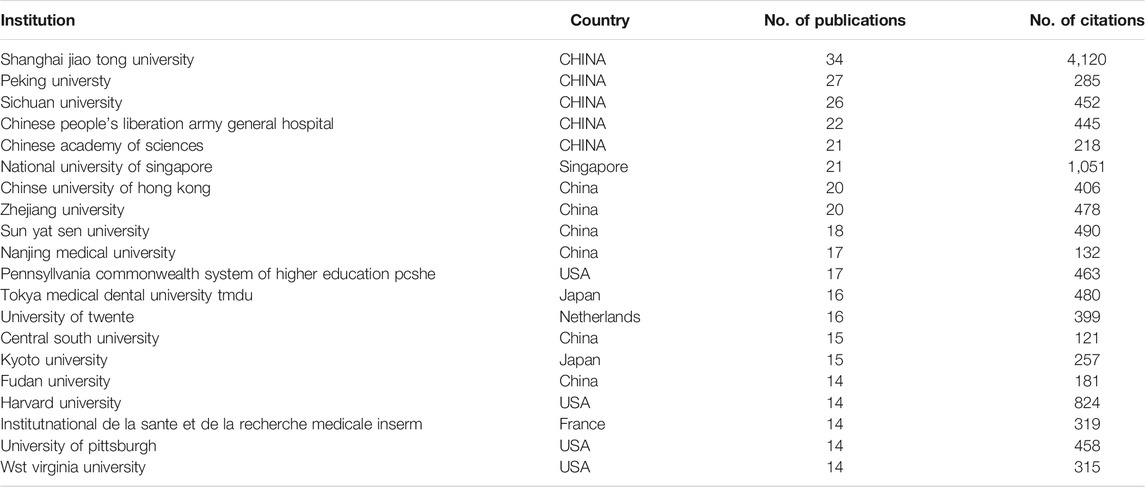
TABLE 2. Top 20 institutions with the most publications in the field of stem cells for cartilage regeneration.
Figure 3A highlights the close and complex collaborative relations between different institutions. CiteSpace was employed to analyse the centrality of institutions. The purple circle indicates centrality, and the area of the circle is proportional to the centrality. Shanghai Jiao Tong University and National University of Singapore are the most prominent institutions, which suggests that they are regarded as pivotal points (Figure 3B).
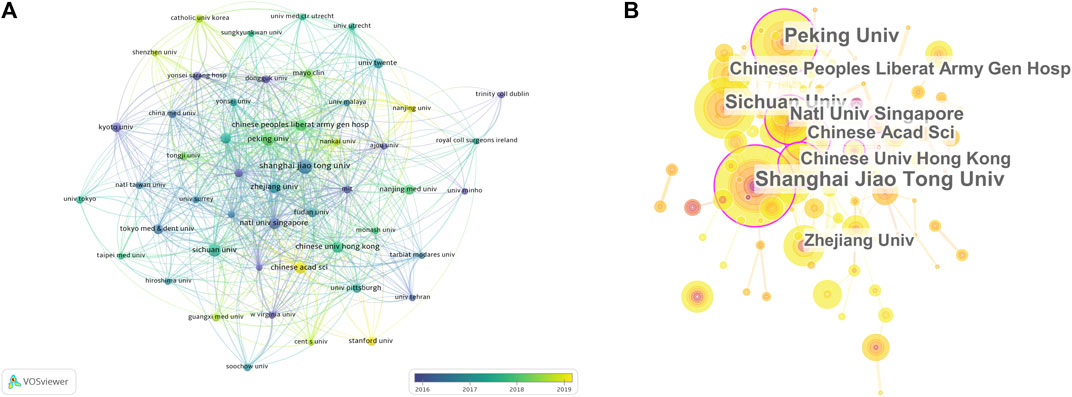
FIGURE 3. Articles on stem cells for cartilage regeneration published at different institutions. (A) Collaboration between institutions based on VOSviewer (B) cooperation network analysis of institutions based on CiteSpace.
3.1.3 Analysis of Journal Distribution
Different journals include different fields of publication. Therefore, we performed a journal distribution analysis of publications on the use of stem cells for cartilage regeneration. The journals Biomaterials (impact factor = 10.317, 2019) and Stem Cell Research and Therapy (impact factor = 5.116, 2019) published the most studies, with 35 publications each. There were 34 articles on stem cell research for cartilage regeneration in Stem Cells International (IF = 3.869, 2019), 33 articles in Acta Biomaterialia (IF = 7.242, 2019) and 32 articles in Journal of Tissue Engineering Part A (IF = 3.776, 2019). The top 20 journals with the most publications are listed in Figure 4A.
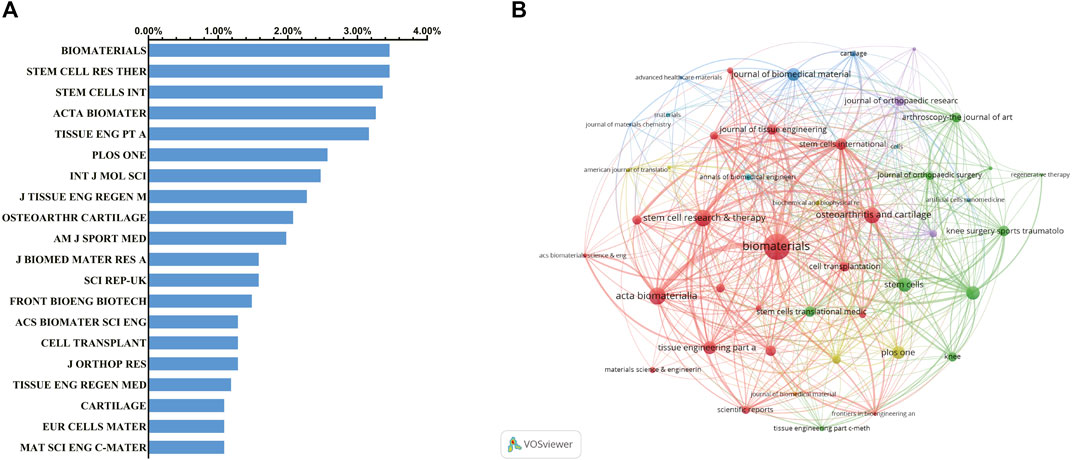
FIGURE 4. Articles published in different journals on stem cells for cartilage regeneration. (A) Top 20 journals that produced the largest number of articles. (B) Cocitation analysis of journals based on VOSviewer.
The journals are not kept in isolation. Biomaterials and Acta Biomaterialia have the closest relationship. As far as this field is concerned, journals with a high volume of publications also have high centrality (Figure 4B).
3.2 Overview of Landmark Articles and Authors
The authors with the highest number of publications from 2010 to 2020 are shown in Table 3, and the total citations of the publications are also listed. SEKIYA I and YANG F tied for first place. However, TOH WS of the National University of Singapore is particularly noteworthy. Although TOH WS published only 10 articles, the total number of citations reached 740, contributing greatly to Singapore’s total citations. This shows that his viewpoint has drawn widespread attention in the field.
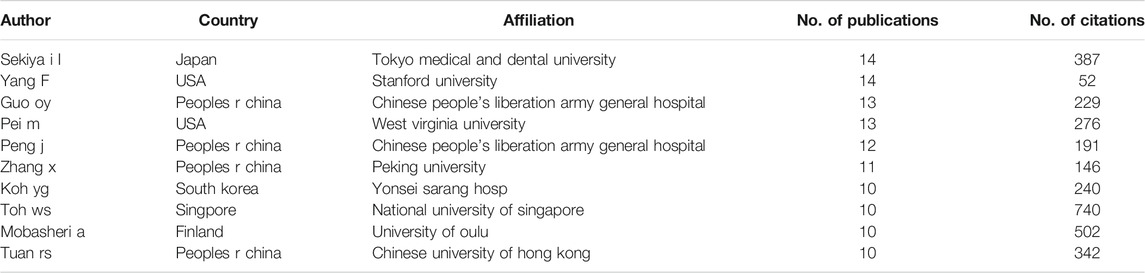
TABLE 3. Top 10 authors in the field of stem cells for cartilage regeneration ranked by publication number.
We analysed the references of the shortlisted publications and constructed the cocitation network. Jo Ch’s article (doi:10.1002/stem.1634) published in Stem Cells in 2014 is at a key node in the co-citation network (Figure 5A). We further performed an in-depth analysis of the article with the highest citation rate (Figure 5B). Then, we implemented a cluster analysis. The references in the co-citation network are divided into 12 diverse clusters (Figure 5C). The clusters are labelled by extricating terms from the titles of the cited publications. From a timeline perspective, the theoretical basis of earlier publications is mainly osteogenesis and growth factors. Articles in recent years have been based on hydrogels and mesenchymal stem cells. The top 10 articles with the most total citations are detailed in Table 4.
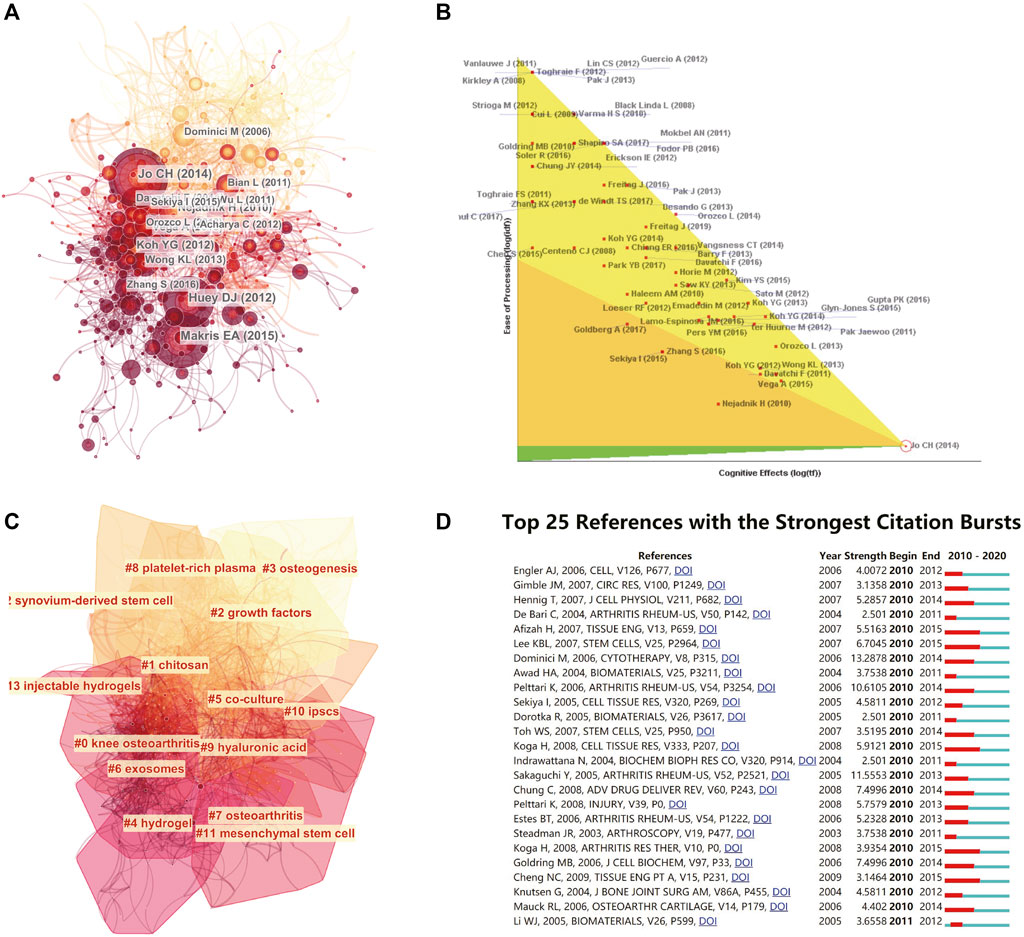
FIGURE 5. Mapping of references in studies on stem cells for cartilage regeneration. (A) A simplified co-citation network of references about stem cells for cartilage regeneration based on CiteSpace (burst references are represented by red nodes). (B) The article with the highest citation rate was also processed for in-depth analysis. (C) Clustering analysis of the co-citation network based on CiteSpace. (D) Top 25 references with strongest citation bursts based on CiteSpace.
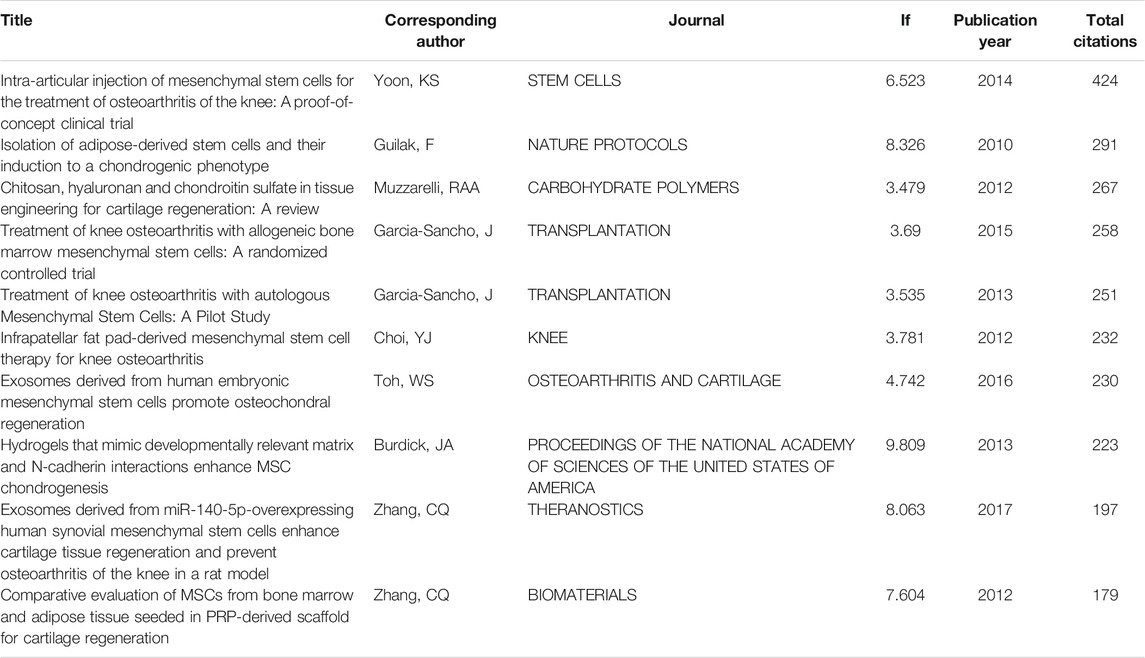
TABLE 4. Top 10 references with the most citations in the field of stem cells for cartilage regeneration.
The burst detection function in CiteSpace is helpful to understanding the research frontiers. The top 25 strongest citation bursts of the references are presented, and there was no article with a sudden change in the number of citations in the past 3 years (Figure 5D).
3.3 Co-Occurrence Analysis of Key Words
Keywords represent the main topic of publications. Co-occurrence analysis of keywords is conducive to systematically understanding the relationship between keywords and consequently grasping the relationship between various topics in this field. Further cluster analysis helps to systematically understand the current progress in this field. VOSviewer was employed to analyse keywords (defined as words that were used more than five times in titles and abstracts in all publications) in all included publications. A total of 203 identified keywords were mainly divided into three clusters: ‘clinical study,’ ‘tissue engineering’ and ‘biological study’. In clinical studies, the keywords with the highest frequency were repair (273 times), articular cartilage (238 times), osteoarthritis (264 times), regeneration (222 times) and bone marrow (193 times). In tissue engineering, these words were chondrogenic differentiation (203 times), scaffold* (160 times), mesenchymal stem cells (152 times), tissue engineering (108 times) and bone (97 times). In biological studies, the words were differentiation (282 times), chondrogenesis (208 times), cartilage (196 times), tissue (191 times) and chondrocytes (186 times) (Figures 6A,B). The annual distribution of keywords is indicated. Chronological order is presented from dark blue to bright yellow. In “Clinical studies,” the earliest keyword was “autologous chondrocyte transplantation” (AAY 2014.7143), with 14 instances, and the latest word was “double-blind” (AAY 2018.75), with 8 times. In tissue engineering, the earliest word was “growth factors” (AAY 2014.125), with 8 times, and the latest word was “3D printing” (AAY 2019.625), with nine instances. In the biological study, the earliest word was “cartilage formation” (AAY 2014.875), with 8 times, and the latest word was “extracellular vesicles” (AAY 2018.9091), with 12 times (Figure 6C). According to the colour intensity, we found that the research hotspot on stem cells for cartilage regeneration gradually shifted to tissue engineering in recent years (Table 5, Figure 5). The 20 keywords with the strongest citation burst are listed in Figure 6D, and no keywords exhibited any sudden changes in the number of citations over the past 3 years.
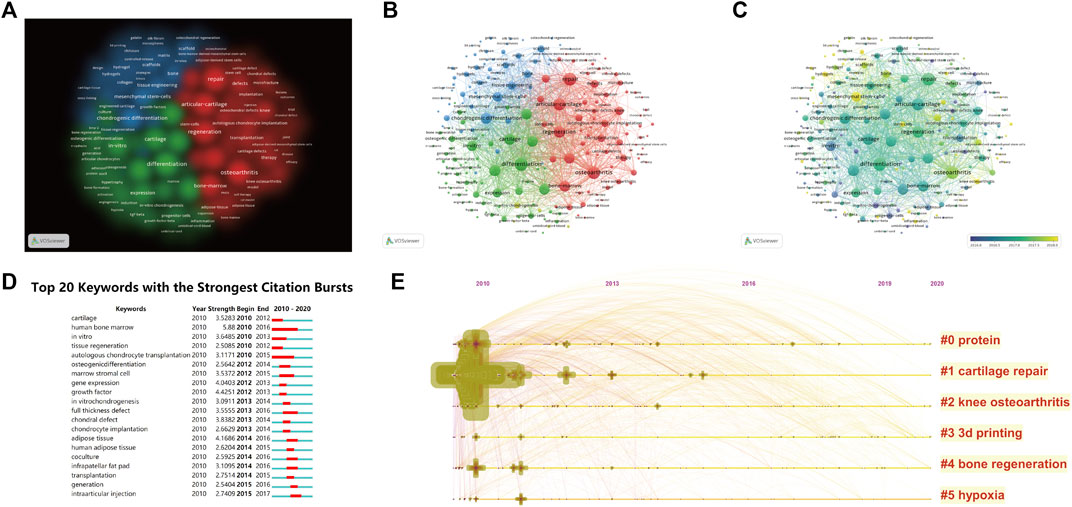
FIGURE 6. Mapping of keywords in studies on stem cells for cartilage regeneration. (A) Clustering analysis of key words based on VOSviewer. (B) Network visualization of keywords based on VOSviewer. (C) Chronological order of keywords based on VOSviewer. (D) Top 20 keywords with the strongest citation bursts based on CiteSpace. (E) Keyword timeline visualization from 2010 to 2020.
3.4 Clinical Research Summary
ClinicalTrials.gov (https://clinicaltrials.gov/) is one of the most important databases of clinical studies conducted around the world, and it was developed by the National Library of Medicine (NML) and the U.S. Food and Drug Administration (FDA). ClinicalTrials could provide clinical trial information inquiry services of clinical trial information to the general public and could also provide clinical trial registration services to medical researchers and institutions. We used ClinicalTrials.gov to list the currently completed clinical studies on stem cells for cartilage regeneration (Table 5). A total of 16 clinical trials have been completed, and among them, CARTISTEM has been approved by the Korean FDA. The 16 clinical trials came from 12 countries, with the top three countries being Iran (3), Republic of Korea 2) and Spain (2). Less than one-third of clinical trials have a comparison group, and more than two-thirds have not yet entered Phase 3. The overall size of the included samples is relatively small. Only NCT01041001 and NCT01626677 exceed 100, both of which are from the Republic of Korea and relevant to CARTISTEM. NCT01041001 assesses and compares the safety and efficacy of the allogeneic-unrelated umbilical cord blood-derived mesenchymal stem cell product (CARTISTEM) to that of a microfracture treatment, and NCT01626677 is a long-term follow-up study to investigate its safety and efficacy. In addition, the most important condition of these clinical trials is knee-related cartilage regeneration, which may be related to its high incidence and feasibility for performing clinical research. From the perspective of article publication, only 6 projects have obtained results.
4 Discussion
This study offered a bibliometric analysis of stem cells for cartilage regeneration from 2010 to 2020. With the development of bibliometric software, bibliometric analysis is now widely pursued. It can help beginners to understand the development process and trends of a specific field intuitively and systematically. It is also beneficial to find milestone achievements and new research hotspots.
Regenerative therapy uses multidisciplinary knowledge and technology to achieve regeneration of damaged tissues or organs. Regenerative therapy integrates knowledge and technology from multiple disciplines, aiming to regenerate damaged tissues or organs (Zhang et al., 2016; El-Jawhari et al., 2017). The number of publications and RRI in stem cells for cartilage regeneration have been on the rise, especially in the past 5 years, suggesting that the proportion of this field among all academic fields is increasing (Figure 2A). In addition, more than half of the top five journals in this field have an impact factor of five or more (Figure 4), which means that the topic of stem cells for cartilage regeneration has drawn widespread attention according to the method used to calculate the impact factor. Therefore, more in-depth studies will be published in the future and prove the feasibility and application prospects of stem cells for cartilage.
In terms of national and regional distribution, the total number of publications and citations of China ranks first in the world. However, the United States still ranked first in terms of the H-index, indicating its predominant influence (Figures 2B,D). When we further analyse the average citation rate (only comparing countries with more than 100 publications), the average citation rate of China is 16.88, while those of the United States and South Korea are 25.03 and 28.49, respectively (Table 1). The influence and contribution of scientific institutions represent a fundamentally important aspect of a country or region. In terms of total publications, 9 of the top 10 institutions are located in China, accounting for 70% of the total publication number. However, among the top 5 institutions in China in terms of publication volume, none of the top 5 in the world in terms of total citations (Table 2; Figure 3). Although the citation rate of the articles are not completely equal to the importance of academic achievements, practical problems may be indicated when low citation rates becomes a common phenomena. From the perspective of time distribution, the concentrated years of article output of China were approximately 2018 (Figure 2D). However, there were no keywords or references with any sudden change in the number of citations in the past 3 years (Figures 5D, 6D). This discrepancy suggests that scientific achievements in China have not formed a hot topic in this field. These results indicate that most academic achievements are the completion and supplementation of previous breakthrough results instead of original creative discoveries and techniques. The National Natural Science Foundation of China (NSFC) is one of the main channels for supporting basic research in China, and a series of changes in the NSFC application rules have taken place in recent years, aiming to improve the quality and innovativeness of publications. We hypothesize that the centrality of China will gradually increase in the future.
As far as authors are concerned, SEKIYA I (Tokyo Medical and Dental University, JAPAN) and YANG F (Stanford University, United States) are the most productive. However, in terms of the number of citations, the data of Toh WS (National University of Singapore, Singapore) are particularly attractive (Table 3). He has published 10 papers on stem cells for cartilage regeneration, but the total number of citations has reached 740. The total citations of Toh WS accounted for approximately 60% of Singapore and 70% of National University of Singapore in the study (Table 1, Table 2). In 2007, Toh WS successfully constructed an experimental system in which human embryoid body (EB)-derived cells could directly differentiate into chondrocytes under specific culture conditions (Toh et al., 2007). In 2009, Toh WS and his team improved this high-density micromass model system. Through using a more effective combination of growth factors and extracellular matrix substrate, they isolated a highly expandable and homogenous chondrogenic cell population named TC1 (Toh et al., 2009). In 2010, Toh WS and his team constructed hESC-derived chondrogenic cell-engineered cartilage (HCCEC) from the abovementioned cell population in the culture of hyaluronic acid (HA)-based hydrogel and verified its long-term viability and safety in a rat model, providing a practical strategy of applying hESCs for cartilage regeneration (Toh et al., 2010). Afterwards, Toh WS and his team shifted the focus of research to mesenchymal stem cell-derived exosomes and illustrated the underlying mechanisms of MSC exosomes in the biological behaviour of chondrocytes, extracellular matrix homeostasis, and immune reactivity in cartilage regeneration (Toh et al., 2017; Zhang et al., 2018). The achievements in MSC-derived exosomes study grapes attention of scientists around the world, and the references in this place had more than 100 citations. In addition, the author and his team published three reviews and summarized the potential and perspective of human embryonic stem cells (ESCs) in cartilage tissue engineering and regenerative medicine, the interactions between stem cells and extracellular matrix for cartilage regeneration, and the potential and perspective of MSC exosomes in cartilage regeneration.
As a key node in the co-citation network, Jo Ch’s article (doi:10.1002/stem.1634) not only had the largest number of citations (Table 4) but also had the highest impact (Figures 5A,B). Jo Ch is the first author, and the corresponding author of this article is Kang Sup Yoon. Kang Sup Yoon and his team substantiated the safety and efficacy of intra-articular injection of autologous AD-MSCs through clinical index, radiological evaluation, arthroscopic evaluation and histological evaluations. More importantly, Kang Sup Yoon and his team evaluated the effects of different doses of AD-MSCs injected into articular tissue. It is currently known that a sufficient number of MSCs can inhibit articular cartilage degradation and promote its regeneration (Ichiseki et al., 2018). However, when evaluating efficacy and safety comprehensively, the optimal cell dose needs to be clarified. Preliminarily exploring the therapeutic dose, Kang Sup Yoon and his team suggested that at least 1.0 × 108 MSCs per injection would be an initial prerequisite for consistently good results (Jo et al., 2014). In conclusion, this proof-of-concept clinical trial confirmed the effectiveness of intra-articular injection of MSCs and presented a fair opinion on the injection dose, promising to encourage continued clinical research and application.
In the “Clinical studies,” the latest word was “double-blind” (AAY 2018.75), with 8 times (Figure 6C), which heralds future trends in the biological study of stem cells for cartilage regeneration. A double-blind trial is a test where neither the tester nor the testees know the exact group of testees, which helps to avoid cognitive bias (Cho et al., 2019). Due to its higher cost, there are very few double-blind trials in this field (Table 5). A double-blind trial from South Korea explored the effectiveness and safety of genetically engineered autologous chondrocytes, which were named TissueGene-C (TG-C), for knee osteoarthritis. TG-C was administered by a single intra-articular injection, and then subjective and objective assessments were performed according to a clinical rating scale and radiographic evaluation (Kim et al., 2018). Although subjective evaluation attests to the effectiveness of this treatment, changes in joint space widths, bone area and cartilage thicknesses only show a trend that are not statistically significant. This research has laid the foundation for the development of double-blind trials in this field. On the basis of reality and possibility, double-blind experiments in stem cells for cartilage regeneration should also be attempted.
In tissue engineering, the latest word was “3D printing” (AAY 2019.625), with 9 times (Figure 6C). 3D printing was proposed by Chuck Hull in 1983 and includes two main processes: computer-aided design (CAD) and 3D printing to form products. In the 2000s, 3D printing was used in the production of surgical models and later developed to be used in the production of live cell structures, including articular cartilage. There are also different strategies regarding the product formation process, inkjet printing, laser-assisted printing, and bioextrusion (Wu et al., 2018; Messaoudi et al., 2020). The materials used for 3D printing (bioink) are a hot and difficult point. Bioink mainly consists of two parts: biomaterials as scaffolds (second highest frequency keywords in tissue engineering clusters) and embedded cells (Kim M. K. et al., 2020). Biomaterials used for tissue engineering need to have biocompatibility, biodegradability, and porosity, and they are mainly divided into natural polymers (alginate, gelatine, chitosan, and hyaluronan) or synthetic polymers (PCL, PGA, PEG) at present (Shen et al., 2019). After being modified, natural polymers can be made into a more stable form, hydrogels, which have been widely used in 3D printing (Diloksumpan et al., 2020). Synthetic polymers could be combined or coated with hydrogels to enhance their biocompatibility. Biomaterials can play a variety of roles depending on the embedded cell types, including chondrogenic differentiation (highest frequency keywords in tissue engineering clusters).
As mentioned earlier, chondrocytes have limited expansion efficiency and potential for dedifferentiation in vivo. In contrast, the application of stem cells in tissue engineering is more promising. According to current research, the mechanism underlying cartilage regeneration in stem cells is mainly attributed to direct differentiation of chondrocytes and paracrine secretion (Iturriaga et al., 2018; Kim Y. et al., 2020). BM-MSCs are among the most commonly used cell types in tissue engineering (Table 5), and they can be induced into multiple cell types, including chondrocytes. AD-MSCs are easily accessible via minimally invasive procedures, and they have the characteristics of synthesizing more collagen than other MSC types. SF-MSCs and Dental pulp MSCs are also have unique advantages. (Tan and Hung, 2017; Kabir et al., 2020). In addition to biological materials and stem cell types, environmental conditions and differentiation-promoting factors are also key points for 3D printing technology. For example, the surrounding medium’s oxygen content affects the chondrogenic differentiation of MSCs(Portron et al., 2015; Anderson et al., 2016), and TGF-β1 can promote and stabilize the chondrogenic phenotype (Futrega et al., 2021). Overall, 3D printing is a microcosm of tissue engineering: whether it can be promoted clinically depends on many factors, but 3D printing clearly constitutes an important direction of future research.
In the biological study, the latest term was “extracellular vesicles” (AAY 2018.9091), with 12 times (Figure 6C). Extracellular vesicles are released from the cell surface to body fluid, and they can transfer multiple types of cargo, such as lipids, nucleic acids and proteins, thus contributing to intercellular communication (Allan et al., 2020). Exosomes, microvesicles (MVs) and apoptotic bodies are currently the most attractive categories of extracellular vesicles, and they are different in terms of generation mechanism and size (Lawson et al., 2016). According to publication numbers, exosomes have attracted more attention among researchers. Currently, exosomes have been identified from multiple MSCs, including those derived from AD-MSCs, BM-MSCs, US-MSCs, hESCs, and iPSCs (Zhang et al., 2018; Xu et al., 2020). When MSCs sense changes in the surrounding microenvironment, they can secrete exosomes to interact with other cells. Exosomes have been proven to regulate the biological behaviour of chondrocytes, to modulate immune responses and to restore homeostasis to the ECM, thereby promoting cartilage regeneration (Zhang et al., 2018; Tan et al., 2020). Although the therapeutic efficacy, biosafety, kinetics and biodistribution of MSC exosomes need to be studied in depth, the regenerative potency of MSC exosomes provides new perspectives for the development of a cell-free MSC therapy strategy for cartilage regeneration.
The purpose of clinical trials is to repair damaged articular cartilage. The application of stem cells is expected to replace traditional medical treatment and achieve the goal of radical cures without undergoing such a major operation as joint replacement. According to current clinical trials, except for CARTISTEM, which uses commercial hUCB-MSCs(Park et al., 2017), all other clinical trials use autologous stem cells. The entire process includes collection, in vitro expansion, and intra-articular injection (Al-Najar et al., 2017). Subjective clinical scores, imaging evidence, and histological evaluation are currently the most important evaluation methods. The effectiveness, feasibility and safety of the concept of using mesenchymal stem cells in cartilage repair have been verified (Table 5). However, there are still some issues that need to be resolved. First, the most suitable cell source necessitates further exploration. Except for CARTISTEM, all other clinical trials use autologous stem cells, and the most commonly used type is BM-MSCs. The horizontal comparison of different types of cells is still missing. Second, it is unclear whether MSCs mainly differentiate into chondrocytes directly or more through paracrine forms to promote cartilage formation. Third, the optimal therapeutic course and dose need to be further explored. NCT01207661 indicated that the effect of a single injection was improved in the first 12 months and decreased from the 12th month onward (Emadedin et al., 2015). NCT01873625 also explored the number of cells per injection and provided a reference value of 40 million (Shadmanfar et al., 2018). However, several concerns about the use of stem cells remain unsolved, particularly regarding side effects (Rivera-Izquierdo et al., 2019). For example, challenges of stem cell transplantation include poor survivability, migration of the transplanted cells, and adverse immune reactions (Dunn et al., 2019). What’s worse, the tumorigenic properties of stem cells have been reported, they not only possess intrinsic tumorigenic properties, but can be induced to possess tumorigenic properties (Patel et al., 2008). The last but not the least, ethical considerations for stem cell therapy cannot be ignored. In thus, the best course of treatment and therapeutic dose need more exploration. In addition, current clinical trials are mainly short-term follow-ups, typically only 24 months or less. Long-term efficacy and safety evaluations need further verification.
This study investigated publications on stem cells for cartilage regeneration extracted from the Web of Science database and discussed hot issues in this comprehensive and objective field. However, limitations are inevitable. First, we only enrolled publications in the language of English, which may cause some important non-English studies in this field to be overlooked. In addition, although keywords can reflect the subject of the article to a certain extent, the preferences of different authors when using keywords and the shortness of keywords will lead to bias in trend analysis.
Conclusion
Overall, this study summarized and analysed the global research trends concerning the use of stem cells for cartilage regeneration. The annual output of related publications has grown remarkably, and stem cells have positive development prospects for cartilage regeneration. China and the United States have made the largest contributions in this field. 3D printing, extracellular vesicles, and double-blind clinical trials are research hotspots and which are likely to be promising in the near future. Clinical trials with larger sample sizes and longer follow-up periods are needed for clinical transformation.
Data Availability Statement
The original contributions presented in the study are included in the article/Supplementary Material, further inquiries can be directed to the corresponding authors.
Author Contributions
SX and PZ were responsible for the study design and administrative support. JW and FZ were responsible for data collection. SW and ZZ were responsible for analyzing the data. DX and JW were responsible for manuscript drafting. All authors have critically reviewed and approved the final manuscript.
Funding
We appreciate support from the National Science Foundation for Young Scientists of China (No. 81601910), the Shanghai Rising-Star Program (No. 18QA1405400), the Military Medical Research Foundation from the Naval Military Medical University (No. 2017JS15), and the China Postdoctoral Science Foundation Grant (No. 2016M592936), Incubation Project of Military Medical Science and Technology Youth Cultivation Plan (No. 20QNPY036).
Conflict of Interest
The authors declare that the research was conducted in the absence of any commercial or financial relationships that could be construed as a potential conflict of interest.
Publisher’s Note
All claims expressed in this article are solely those of the authors and do not necessarily represent those of their affiliated organizations, or those of the publisher, the editors and the reviewers. Any product that may be evaluated in this article, or claim that may be made by its manufacturer, is not guaranteed or endorsed by the publisher.
Supplementary Material
The Supplementary Material for this article can be found online at: https://www.frontiersin.org/articles/10.3389/fphar.2021.737939/full#supplementary-material
References
Al-Najar, M., Khalil, H., Al-Ajlouni, J., Al-Antary, E., Hamdan, M., Rahmeh, R., et al. (2017). Intra-articular Injection of Expanded Autologous Bone Marrow Mesenchymal Cells in Moderate and Severe Knee Osteoarthritis Is Safe: a Phase I/II Study. J. Orthop. Surg. Res. 12, 190. doi:10.1186/s13018-017-0689-6
Allan, D., Tieu, A., Lalu, M., and Burger, D. (2020). Mesenchymal Stromal Cell-Derived Extracellular Vesicles for Regenerative Therapy and Immune Modulation: Progress and Challenges toward Clinical Application. Stem Cell Transl Med 9, 39–46. doi:10.1002/sctm.19-0114
Anderson, D. E., Markway, B. D., Bond, D., McCarthy, H. E., and Johnstone, B. (2016). Responses to Altered Oxygen Tension Are Distinct between Human Stem Cells of High and Low Chondrogenic Capacity. Stem Cel Res Ther 7, 154. doi:10.1186/s13287-016-0419-8
Baldiotti, A., Amaral-Freitas, G., Barcelos, J., Freire-Maia, J., Perazzo, M., Freire-Maia, F., et al. (2020). The Top 100 Most-Cited Papers in Cariology: A Bibliometric Analysis. Caries Res. 55, 32–40. doi:10.1159/000509862
Carballo, C. B., Nakagawa, Y., Sekiya, I., and Rodeo, S. A. (2017). Basic Science of Articular Cartilage. Clin. Sports Med. 36, 413–425. doi:10.1016/j.csm.2017.02.001
Cho, S. H., Han, S., Ghim, J. L., Nam, M. S., Yu, S., Park, T., et al. (2019). A Randomized, Double-Blind Trial Comparing the Pharmacokinetics of CT-P16, a Candidate Bevacizumab Biosimilar, with its Reference Product in Healthy Adult Males. BioDrugs 33, 173–181. doi:10.1007/s40259-019-00340-x
Diloksumpan, P., de Ruijter, M., Castilho, M., Gbureck, U., Vermonden, T., van Weeren, P. R., et al. (2020). Combining Multi-Scale 3D Printing Technologies to Engineer Reinforced Hydrogel-Ceramic Interfaces. Biofabrication 12, 025014. doi:10.1088/1758-5090/ab69d9
Dunn, A., Talovic, M., Patel, K., Patel, A., Marcinczyk, M., and Garg, K. (2019). Biomaterial and Stem Cell-Based Strategies for Skeletal Muscle Regeneration. J. Orthop. Res. 37, 1246–1262. official publication of the Orthopaedic Research Society. doi:10.1002/jor.24212
El-Jawhari, J. J., Cuthbert, R., McGonagle, D., Jones, E., and Giannoudis, P. V., (2017). The CD45lowCD271high Cell Prevalence in Bone Marrow Samples May Provide a Useful Measurement of the Bone Marrow Quality for Cartilage and Bone Regenerative Therapy. J. Bone Jt. Surg. 99, 1305–1313. doi:10.2106/jbjs.16.01138
Emadedin, M., Ghorbani Liastani, M., Fazeli, R., Mohseni, F., Moghadasali, R., Mardpour, S., et al. (2015). Long-Term Follow-Up of Intra-articular Injection of Autologous Mesenchymal Stem Cells in Patients with Knee, Ankle, or Hip Osteoarthritis. Arch. Iran Med. 18, 336–344. 015186/AIM.003.
Futrega, K., Robey, P. G., Klein, T. J., Crawford, R. W., and Doran, M. R. (2021). A Single Day of TGF-Β1 Exposure Activates Chondrogenic and Hypertrophic Differentiation Pathways in Bone Marrow-Derived Stromal Cells. Commun. Biol. 4, 29. doi:10.1038/s42003-020-01520-0
Ichiseki, T., Shimazaki, M., Ueda, Y., Ueda, S., Tsuchiya, M., Souma, D., et al. (2018). Intraarticularly-Injected Mesenchymal Stem Cells Stimulate Anti-inflammatory Molecules and Inhibit Pain Related Protein and Chondrolytic Enzymes in a Monoiodoacetate-Induced Rat Arthritis Model. Int. J. Mol. Sci. 19, 203. doi:10.3390/ijms19010203
Iturriaga, L., Hernáez-Moya, R., Erezuma, I., Dolatshahi-Pirouz, A., and Orive, G. (2018). Advances in Stem Cell Therapy for Cartilage Regeneration in Osteoarthritis. Expert Opin. Biol. Ther. 18, 883–896. doi:10.1080/14712598.2018.1502266
Jo, C. H., Lee, Y. G., Shin, W. H., Kim, H., Chai, J. W., Jeong, E. C., et al. (2014). Intra-articular Injection of Mesenchymal Stem Cells for the Treatment of Osteoarthritis of the Knee: a Proof-Of-Concept Clinical Trial. Stem Cells 32, 1254–1266. doi:10.1002/stem.1634
Kabir, W., Di Bella, C., Jo, I., Gould, D., and Choong, P. F. M. (2020). Human Stem Cell Based Tissue Engineering for In Vivo Cartilage Repair: A Systematic Review. Tissue Eng. Part. B Rev. 27 (1), 74–93. doi:10.1089/ten.TEB.2020.0155
Kim, M. K., Ha, C. W., In, Y., Cho, S. D., Choi, E. S., Ha, J. K., et al. (2018). A Multicenter, Double-Blind, Phase III Clinical Trial to Evaluate the Efficacy and Safety of a Cell and Gene Therapy in Knee Osteoarthritis Patients. Hum. Gene Ther. Clin. Dev. 29, 48–59. doi:10.1089/humc.2017.249
Kim, M. K., Jeong, W., Lee, S. M., Kim, J. B., Jin, S., and Kang, H.-W. (2020a). Decellularized Extracellular Matrix-Based Bio-Ink with Enhanced 3D Printability and Mechanical Properties. Biofabrication 12, 025003. doi:10.1088/1758-5090/ab5d80
Kim, Y., Choi, J., and Kim, K. (2020b). Mesenchymal Stem Cell-Derived Exosomes for Effective Cartilage Tissue Repair and Treatment of Osteoarthritis. Biotechnol. J. 15, e2000082. doi:10.1002/biot.202000082
Lawson, C., Vicencio, J. M., Yellon, D. M., and Davidson, S. M. (2016). Microvesicles and Exosomes: New Players in Metabolic and Cardiovascular Disease. J. Endocrinol. 228, R57–R71. doi:10.1530/JOE-15-0201
Le, H., Xu, W., Zhuang, X., Chang, F., Wang, Y., and Ding, J. (2020). Mesenchymal Stem Cells for Cartilage Regeneration. J. Tissue Eng. 11, 2041731420943839. doi:10.1177/2041731420943839
Li, J., Huang, Y., Song, J., Li, X., Zhang, X., Zhou, Z., et al. (2018). Cartilage Regeneration Using Arthroscopic flushing Fluid-Derived Mesenchymal Stem Cells Encapsulated in a One-step Rapid Cross-Linked Hydrogel. Acta Biomater. 79, 202–215. doi:10.1016/j.actbio.2018.08.029
Messaoudi, O., Henrionnet, C., Bourge, K., Loeuille, D., Gillet, P., and Pinzano, A. J. C., (20202021). Stem Cells and Extrusion 3D Printing for Hyaline Cartilage Engineering. Cells 10, 2. doi:10.3390/cells10010002
Murphy, M. P., Koepke, L. S., Lopez, M. T., Tong, X., Ambrosi, T. H., Gulati, G. S., et al. (2020). Articular Cartilage Regeneration by Activated Skeletal Stem Cells. Nat. Med. 26, 1583–1592. doi:10.1038/s41591-020-1013-2
Park, Y. B., Ha, C. W., Lee, C. H., Yoon, Y. C., and Park, Y. G. (2017). Cartilage Regeneration in Osteoarthritic Patients by a Composite of Allogeneic Umbilical Cord Blood-Derived Mesenchymal Stem Cells and Hyaluronate Hydrogel: Results from a Clinical Trial for Safety and Proof-Of-Concept with 7 Years of Extended Follow-Up. Stem Cell Transl Med 6, 613–621. doi:10.5966/sctm.2016-0157
Patel, S. A., Sherman, L., Munoz, J., and Rameshwar, P. (2008). Immunological Properties of Mesenchymal Stem Cells and Clinical Implications. Arch. Immunol. Ther. Exp. (Warsz) 56, 1–8. doi:10.1007/s00005-008-0001-x
Portron, S., Hivernaud, V., Merceron, C., Lesoeur, J., Masson, M., Gauthier, O., et al. (2015). Inverse Regulation of Early and Late Chondrogenic Differentiation by Oxygen Tension Provides Cues for Stem Cell-Based Cartilage Tissue Engineering. Cell Physiol BiochemCell Physiol Biochem 35, 841–857. doi:10.1159/000369742
Rivera-Izquierdo, M., Cabeza, L., Láinez-Ramos-Bossini, A., Quesada, R., Perazzoli, G., Alvarez, P., et al. (2019). An Updated Review of Adipose Derived-Mesenchymal Stem Cells and Their Applications in Musculoskeletal Disorders. Expert Opin. Biol. Ther. 19, 233–248. doi:10.1080/14712598.2019.1563069
Shadmanfar, S., Labibzadeh, N., Emadedin, M., Jaroughi, N., Azimian, V., Mardpour, S., et al. (2018). Intra-articular Knee Implantation of Autologous Bone Marrow-Derived Mesenchymal Stromal Cells in Rheumatoid Arthritis Patients with Knee Involvement: Results of a Randomized, Triple-Blind, Placebo-Controlled Phase 1/2 Clinical Trial. Cytotherapy 20, 499–506. doi:10.1016/j.jcyt.2017.12.009
Shen, S., Chen, M., Guo, W., Li, H., Li, X., Huang, S., et al. (2019). Three Dimensional Printing-Based Strategies for Functional Cartilage Regeneration. Tissue Eng. Part. B Rev. 25, 187–201. doi:10.1089/ten.TEB.2018.0248
Sophia Fox, A. J., Bedi, A., and Rodeo, S. A. (2009). The Basic Science of Articular Cartilage: Structure, Composition, and Function. Sports health 1, 461–468. doi:10.1177/1941738109350438
Tan, A. R., and Hung, C. T. (2017). Concise Review: Mesenchymal Stem Cells for Functional Cartilage Tissue Engineering: Taking Cues from Chondrocyte-Based Constructs. Stem Cell Transl Med 6, 1295–1303. doi:10.1002/sctm.16-0271
Tan, S., Tjio, C., Wong, J., Wong, K., Chew, J., Hui, J., et al. (2020). Mesenchymal Stem Cell Exosomes for Cartilage Regeneration: A Systematic Review of Preclinical In Vivo Studies. Tissue Eng. Part. B Rev. 27 (1), 1–13. doi:10.1089/ten.TEB.2019.0326
Toh, W. S., Guo, X. M., Choo, A. B., Lu, K., Lee, E. H., and Cao, T. (2009). Differentiation and Enrichment of Expandable Chondrogenic Cells from Human Embryonic Stem Cells In Vitro. J. Cel Mol Med 13, 3570–3590. doi:10.1111/j.1582-4934.2009.00762.x
Toh, W. S., Lai, R. C., Hui, J. H. P., and Lim, S. K. (2017). MSC Exosome as a Cell-free MSC Therapy for Cartilage Regeneration: Implications for Osteoarthritis Treatment. Semin. Cel Dev Biol 67, 56–64. doi:10.1016/j.semcdb.2016.11.008
Toh, W. S., Lee, E. H., and Cao, T. (2011). Potential of Human Embryonic Stem Cells in Cartilage Tissue Engineering and Regenerative Medicine. Stem Cel Rev Rep 7, 544–559. doi:10.1007/s12015-010-9222-6
Toh, W. S., Lee, E. H., Guo, X. M., Chan, J. K., Yeow, C. H., Choo, A. B., et al. (2010). Cartilage Repair Using Hyaluronan Hydrogel-Encapsulated Human Embryonic Stem Cell-Derived Chondrogenic Cells. Biomaterials 31, 6968–6980. doi:10.1016/j.biomaterials.2010.05.064
Toh, W. S., Yang, Z., Heng, B. C., and Cao, T. (2007). Differentiation of Human Embryonic Stem Cells toward the Chondrogenic Lineage. Methods Mol. Biol. 407, 333–349. doi:10.1007/978-1-59745-536-7_23
Toh, W. S., and Cao, T., (2014). Derivation of Chondrogenic Cells from Human Embryonic Stem Cells for Cartilage Tissue Engineering. Methods Mol. Biol. 1. 1. (Clifton, NJ). doi:10.1007/7651_2014_89
Wang, K., Li, J., Li, Z., Wang, B., Qin, Y., Zhang, N., et al. (2019). Chondrogenic Progenitor Cells Exhibit Superiority over Mesenchymal Stem Cells and Chondrocytes in Platelet-Rich Plasma Scaffold-Based Cartilage Regeneration. Am. J. Sports Med. 47, 2200–2215. doi:10.1177/0363546519854219
Wells, K., Klein, M., Hurwitz, N., Santiago, K., Cheng, J., Abutalib, Z., et al. (2020). Cellular and Clinical Analyses of Autologous Bone Marrow Aspirate Injectate for Knee Osteoarthritis: A Pilot Study. PM R. 13 (4), 387–396. doi:10.1002/pmrj.12429
Wu, L., Magaz, A., Wang, T., Liu, C., Darbyshire, A., Loizidou, M., et al. (2018). Stiffness Memory of Indirectly 3D-Printed Elastomer Nanohybrid Regulates Chondrogenesis and Osteogenesis of Human Mesenchymal Stem Cells. Biomaterials 186, 64–79. doi:10.1016/j.biomaterials.2018.09.013
Xu, X., Liang, Y., Li, X., Ouyang, K., Wang, M., Cao, T., et al. (2020). Exosome-mediated Delivery of Kartogenin for Chondrogenesis of Synovial Fluid-Derived Mesenchymal Stem Cells and Cartilage Regeneration. Biomaterials 269, 120539. doi:10.1016/j.biomaterials.2020.120539
Yan, W., Zheng, K., Weng, L., Chen, C., Kiartivich, S., Jiang, X., et al. (2020). Bibliometric Evaluation of 2000-2019 Publications on Functional Near-Infrared Spectroscopy. NeuroImage 220, 117121. doi:10.1016/j.neuroimage.2020.117121
Zellner, J., Grechenig, S., Pfeifer, C. G., Krutsch, W., Koch, M., Welsch, G., et al. (2017). Clinical and Radiological Regeneration of Large and Deep Osteochondral Defects of the Knee by Bone Augmentation Combined with Matrix-Guided Autologous Chondrocyte Transplantation. Am. J. Sports Med. 45, 3069–3080. doi:10.1177/0363546517717679
Zhang, S., Chuah, S. J., Lai, R. C., Hui, J. H. P., Lim, S. K., and Toh, W. S. (2018). MSC Exosomes Mediate Cartilage Repair by Enhancing Proliferation, Attenuating Apoptosis and Modulating Immune Reactivity. Biomaterials 156, 16–27. doi:10.1016/j.biomaterials.2017.11.028
Keywords: stem cells, cartilage regeneration, bibliometric analysis, hotspot, web of science
Citation: Xia D, Wu J, Zhou F, Wang S, Zhang Z, Zhou P and Xu S (2022) Mapping Thematic Trends and Analysing Hotspots Concerning the Use of Stem Cells for Cartilage Regeneration: A Bibliometric Analysis From 2010 to 2020. Front. Pharmacol. 12:737939. doi: 10.3389/fphar.2021.737939
Received: 07 July 2021; Accepted: 19 November 2021;
Published: 03 January 2022.
Edited by:
Elham Ahmadian, Tabriz University of Medical Sciences, IranReviewed by:
Zohreh Bahareh Bagher, Iran University of Medical Sciences, IranAlexander V. Zholos, Taras Shevchenko National University of Kyiv, Ukraine
Copyright © 2022 Xia, Wu, Zhou, Wang, Zhang, Zhou and Xu. This is an open-access article distributed under the terms of the Creative Commons Attribution License (CC BY). The use, distribution or reproduction in other forums is permitted, provided the original author(s) and the copyright owner(s) are credited and that the original publication in this journal is cited, in accordance with accepted academic practice. No use, distribution or reproduction is permitted which does not comply with these terms.
*Correspondence: Panyu Zhou, cGFueXVfemhvdUAxNjMuY29t; Shuogui Xu, MTg1MTYxMTY2NzJAMTYzLmNvbQ==
†These authors have contributed equally to this work