- 1Division of Clinical Pharmacology and Toxicology, Department of Anesthesiology, Pharmacology, Intensive Care, and Emergency Medicine, Geneva University Hospitals, Geneva, Switzerland
- 2Institute of Pharmaceutical Sciences of Western Switzerland (ISPSO), School of Pharmaceutical Sciences, University of Geneva, Geneva, Switzerland
- 3Faculty of Medicine, University of Geneva, Geneva, Switzerland
Background: Available in-vitro and animal studies indicate that inflammation impacts cytochromes P450 (CYP) activity via multiple and complex transcriptional and post-transcriptional mechanisms, depending on the specific CYP isoforms and the nature of inflammation mediators. It is essential to review the current published data on the impact of inflammation on CYP activities in adults to support drug individualization based on comorbidities and diseases in clinical practice.
Methods: This systematic review was conducted in PubMed through 7th January 2021 looking for articles that investigated the consequences of inflammation on CYP activities in adults. Information on the source of inflammation, victim drugs (and CYPs involved), effect of disease-drug interaction, number of subjects, and study design were extracted.
Results: The search strategy identified 218 studies and case reports that met our inclusion criteria. These articles were divided into fourteen different sources of inflammation (such as infection, autoimmune diseases, cancer, therapies with immunomodulator…). The impact of inflammation on CYP activities appeared to be isoform-specific and dependent on the nature and severity of the underlying disease causing the inflammation. Some of these drug-disease interactions had a significant influence on drug pharmacokinetic parameters and on clinical management. For example, clozapine levels doubled with signs of toxicity during infections and the concentration ratio between clopidogrel’s active metabolite and clopidogrel is 48-fold lower in critically ill patients. Infection and CYP3A were the most cited perpetrator of inflammation and the most studied CYP, respectively. Moreover, some data suggest that resolution of inflammation results in a return to baseline CYP activities.
Conclusion: Convincing evidence shows that inflammation is a major factor to be taken into account in drug development and in clinical practice to avoid any efficacy or safety issues because inflammation modulates CYP activities and thus drug pharmacokinetics. The impact is different depending on the CYP isoform and the inflammatory disease considered. Moreover, resolution of inflammation appears to result in a normalization of CYP activity. However, some results are still equivocal and further investigations are thus needed.
Introduction
Cytochromes P450 (CYP) are the major drug-metabolizing enzymes (DME) responsible for 75% of drug metabolism, making them decisive in the efficacy and safety of drugs (Wienkers and Heath, 2005). The interindividual variability in CYP activity is influenced by genetic factors, environmental factors and comorbidities (Lynch and Price, 2007). CYP genetic polymorphisms are well described, resulting in major functional differences (Zhou et al., 2017). CYP are also impacted by drug-drug interactions (DDIs) and several widely used drugs were removed from the market because of serious adverse drug reactions (ADRs) due to DDIs via the CYPs (Wilkinson, 2005). Therefore, the Food and Drug Administration (FDA) requires in-vitro evaluation of potential DDIs during the course of drug development (Kato, 2020; Food and Drug Administration).
A less well described but increasingly studied source of modulation of CYP activity and recently reviewed is that of endogenous inflammatory markers (de Jong et al., 2020; Stanke-Labesque et al., 2020). Inflammation is a response to endogenous or exogenous aggression that can be acute or chronic. It is prominent in many diseases, such as infection, trauma, surgery, arthritis, asthma, atherosclerosis, autoimmune disease, various immunologically mediated and crystal-induced inflammatory conditions, diabetes and cancer, to name a few (Gabay and Kushner, 1999; Germolec et al., 2018; Stavropoulou et al., 2018). This universal protective response involves innate and adaptative immunity and is present in virtually all tissues. Acute changes can be associated with variation in the concentrations of several plasma proteins, the acute-phase proteins (APP), and numerous behavioral, physiological, biochemical and nutritional changes (Gabay and Kushner, 1999). Cytokines are the main stimulators of APP production, and interleukin-6 (IL-6) is the key stimulator of APP while other cytokines (IL-1β, Tumor Necrosis Factor α, interferon-γ, transforming growth factor β and possible IL-8) influence APP subgroups (Gabay and Kushner, 1999). Thus, inflammation is a complex and well-orchestrated process involving many cell types and molecules that function as a cascade network, some of which initiate, amplify or sustain the process and others attenuate or resolve it (Gabay and Kushner, 1999; Stanke-Labesque et al., 2020).
Inflammation can impact drug PK through multiple mechanisms which typically occur in the liver, kidney, or intestinal epithelial cells (Stavropoulou et al., 2018; de Jong et al., 2020; Stanke-Labesque et al., 2020). The metabolic activities of CYPs are suppressed by inflammation in most cases, but some CYPs may be induced or remain unaffected (Morgan, 2001; de Jong et al., 2020; Stanke-Labesque et al., 2020). The positive and negative control of gene transcription is generally achieved by the interaction of regulatory proteins with specific DNA sequences on the regulated genes (Morgan, 1997). The impact of inflammation on the metabolic activity of CYPs has been studied in various in-vitro and animal models of inflammation, including trauma, infection and administration of endotoxin or cytokines (de Jong et al., 2020; Stanke-Labesque et al., 2020). Information available in the literature suggests that this impact on PK is triggered by cytokines and their intracellular signaling, directly or via interaction with the nuclear receptor pathway, on drug transporters and metabolizing enzymes (Liptrott and Owen, 2011; de Jong et al., 2020; Stanke-Labesque et al., 2020). Importantly, no single common pathway has been identified to explain the changes in the entire CYP family and involves different mediators but also different transcription factors (Renton, 2005; de Jong et al., 2020; Stanke-Labesque et al., 2020). Different effects of cytokines are observed in different cell types, which could be explained by a difference in the way intracellular signals from cytokine receptors are generated (Liptrott and Owen, 2011). Different cytokines exhibit a widely different spectrum of activity trough individual CYP isoforms and many different transcription factors (Morgan, 1997; Ruminy et al., 2001; Renton, 2005; Liptrott and Owen, 2011). Their activation by cytokines have been implicated in the downregulation and transcriptional regulation of different CYP isoforms (Morgan, 1997; Ruminy et al., 2001; Renton, 2005; Liptrott and Owen, 2011). Regulation of CYP during inflammation can occur trough pre- and post-transcriptional mechanisms that are cytokine and CYP specific (de Jong et al., 2020; Stanke-Labesque et al., 2020). Pre-transcriptional mechanisms currently described in the literature include transcriptional downregulation of transcription factors, interference with dimerization/translocation of (nuclear) transcription factors, altered liver-enriched C/EBP signaling, and direct regulation by NF-κB (de Jong et al., 2020). Overall, three main mechanisms have been described to explain the downregulation of inflammation in drug metabolizing enzyme and transporters expression and activity, namely inhibition of drug metabolizing enzyme transcription, epigenetic modifications in genes as a result of DNA methylation, modification of histone patterns, release of microRNA and NO-dependent proteasome degradation, which is a post-transcriptional mechanism (Stanke-Labesque et al., 2020).
Therefore, the aim of this systemic review is to evaluate the impact of inflammation on CYP activity in the adult population.
Methods
The method used to manage the literature search was based on the Preferred Reporting Items for Systematic Review and Meta-Analyses (PRISMA) statement (Moher et al., 2009). The detailed PICOS framework (i.e., participants, interventions, comparisons, outcomes, study design) was used as follows: Participants: adults with source of inflammation, -Intervention: victim drugs and CYPs concerned, -Comparison: healthy adults or before the onset of inflammation or receiving treatment for inflammation Outcomes: potential effect of interaction between inflammation and CYP activity, -Study design: clinical trials and case reports/series.
Database and Search Strategy
The literature search was performed in PubMed via MEDLINE, the database of biomedical publications, for studies and case reports/series until January 7, 2021. To expand it, we also performed a manual search of references for potentially relevant articles. The keywords used were “inflammation”, “cytochrome P450”, “cytochromes P450” and “CYP450.”
Study Selection
We applied the eligibility criteria described below in order to filter relevant publications from the total of results provided by the literature search.
The types of studies included in our literature search were randomized controlled trials, non-randomized studies, and observational studies, including case reports and series, published as full-text articles and congress abstracts in English. The year of publication selected was from database inception until January 7, 2021. Study participants had to be older than 18 years old, including healthy subjects and patients with an inflammatory condition, caused by disease, treatment or a medical or surgical procedure. The outcomes of interest were the effect of potential inflammation (suggested or provided) on metabolic ratios (MR) of CYP isoforms, the PK/PD and the safety profile of CYP substrates.
Successive steps in article selection included reading the title, abstract and full text according to the predefined eligibility criteria to screen for potentially relevant records. The selected articles were classified into literature reviews and in-vitro, animal, in-silico and human studies. Then, only studies involving adults (defined as over 18 years old) were kept, classified into studies or case reports/series. The same procedure was applied to assess the inclusion of additional articles identified by the manual search. The study selection process was summarized in a flowchart created according to the PRISMA statement requirements (Figure 1) (Moher et al., 2009).
Data Extraction and Management
Articles selected from the search results were collected and exported to the reference management software Zotero (version 5.0.85, © 2006–2018 Contributors) and merged to remove duplicates. Data from the included articles were extracted and synthetized. The authors extracted the following data according to the PICOS framework discussed above. These included study design, sample size, source of inflammation and comparators, victim drugs and CYP involved, and outcomes of interests (potential effect of interaction). When a CYP substrate was used in the article to determine whether or not inflammation or concomitant drugs altered its PK/PD profile, a verification of its metabolic pathway was performed. The verification process was performed using the Summary of Product Characteristics (SmPCs), the Lexi-Interact drug interaction checker and the Geneva table of CYP substrates, inhibitors, and inducers (Uptodate,; Samer et al., 2013).
Results
Identification and Selection of the Studies
The primary search, performed in PubMed, yielded a total of 2′283 articles that were screened according to their title and abstract. Of the remaining 523 articles, an additional 366 articles were identified by cross-referencing and handsearching of the reference list of the relevant articles (n = 889). Of these, 352 records were removed because the full text was not available (n = 128) or because they were considered irrelevant or not translated into English (n = 224). The remaining 537 articles were classified into review articles (n = 55), in-vitro (n = 77) or in-silico (n = 8) studies, and animal (n = 152) or human (n = 245) studies. The articles and case reports concerning the pediatric population (n = 27) are the subject of another systematic review and were excluded from this work (Lenoir et al., 2021). Finally, 218 articles conducted in adults were included and classified into studies (n = 180) and case reports/series (n = 38) for analysis (Figure 1).
Results of the Studies
The 218 eligible publications are summarized in Table 1 through 14. The drug-disease interactions found in the selected articles were divided into fourteen different sources of inflammation: unspecified source of inflammation (Table 1), infection (Table 2A), infection-example hepatitis (Table 2B), infection-example HIV (Table 3C), infection-example SARS-CoV-2 (Table 2D), vaccination (Table 3), kidney disease (Table 4), liver disease (Table 5), lung disease (Table 6), heart disease (Table 7), critically ill patients (Table 8), diabetes (Table 9), autoimmune diseases (Table 10), surgery (Table 11), cancer (Table 12), therapies with immunomodulator (Table 13) and therapies with anti-TNF-α and -mabs (Table 14). The most cited inflammation perpetrator was infection and the most studied CYP was CYP3A. CYP3A subfamilies refers to CYP3A4 and CYP3A5, because the probe drugs used to assess the activity of CYP3A4 are metabolized by these two isoenzymes and no distinction can be made between them. Distribution in percent of all the references in the different categories are illustrated in Figure 2.
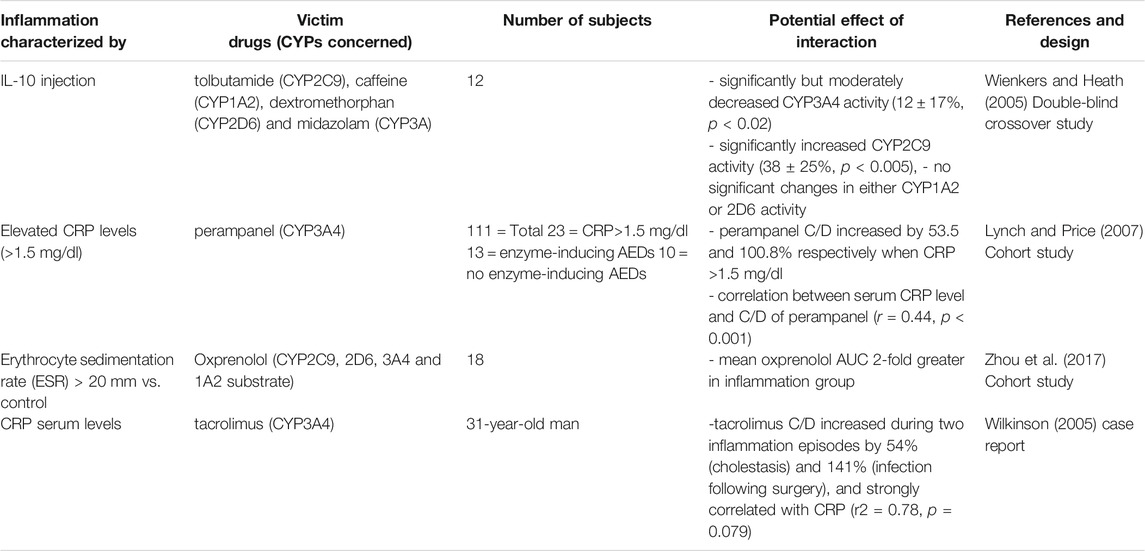
TABLE 1. Impact of unspecified source inflammation on CYP substrates, explained totally or partially by modulation of CYP activity.
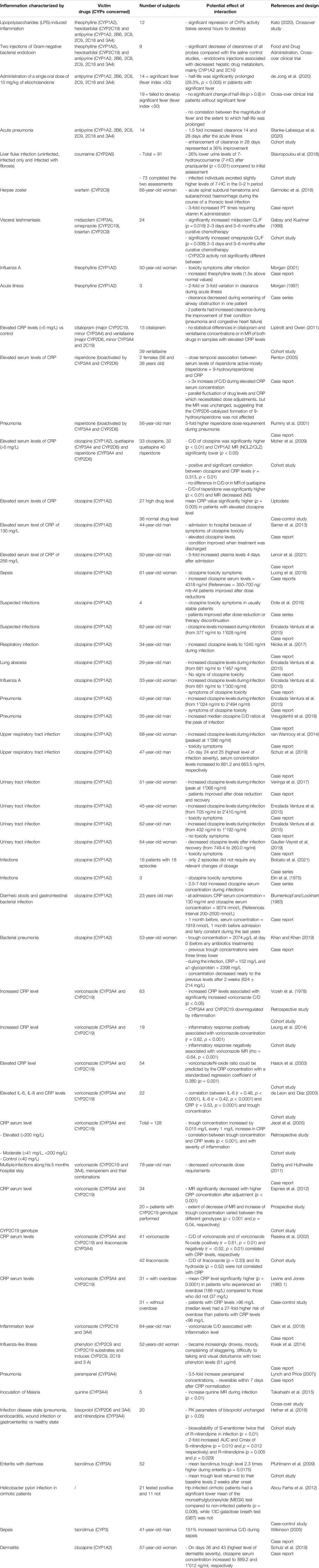
TABLE 2A. Impact of infection on CYP substrates, explained totally or partially by modulation of CYP activity.
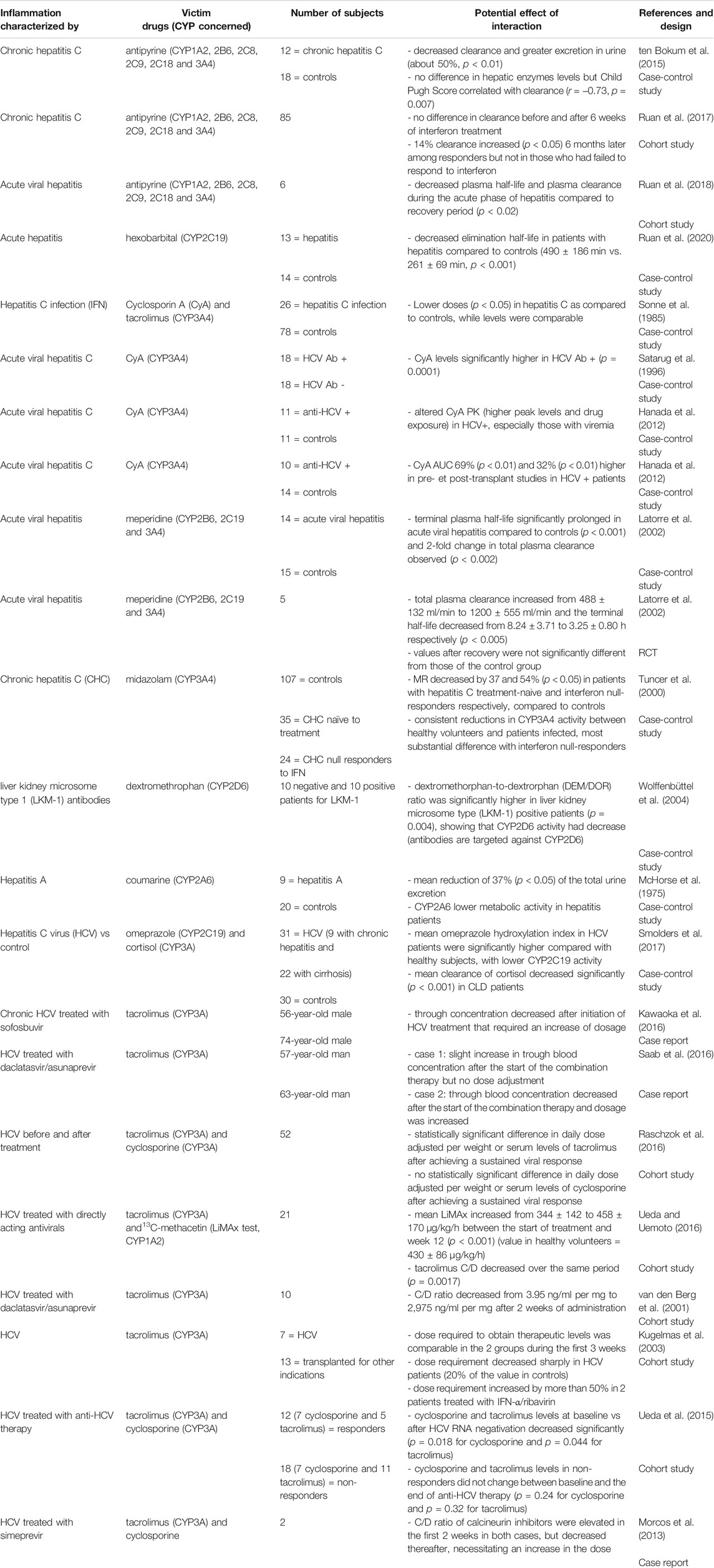
TABLE 2B. Impact of hepatitis on CYP substrates, explained totally or partially by modulation of CYP activity.
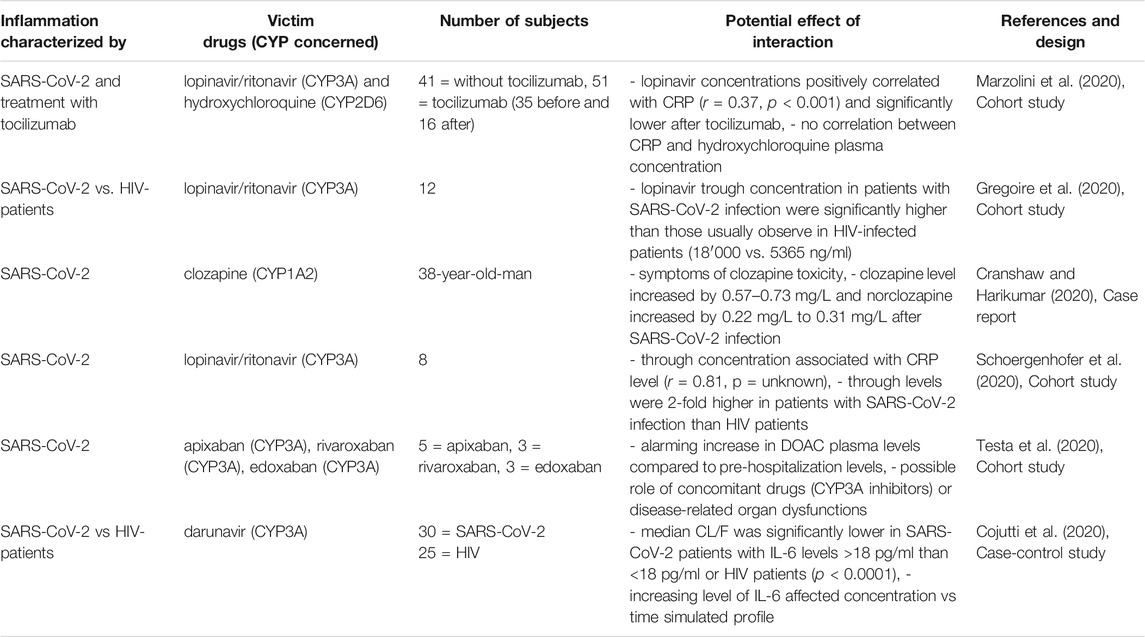
TABLE 2D. Impact of SARS-CoV-2 on CYP substrates, explained totally or partially by modulation of CYP activity.
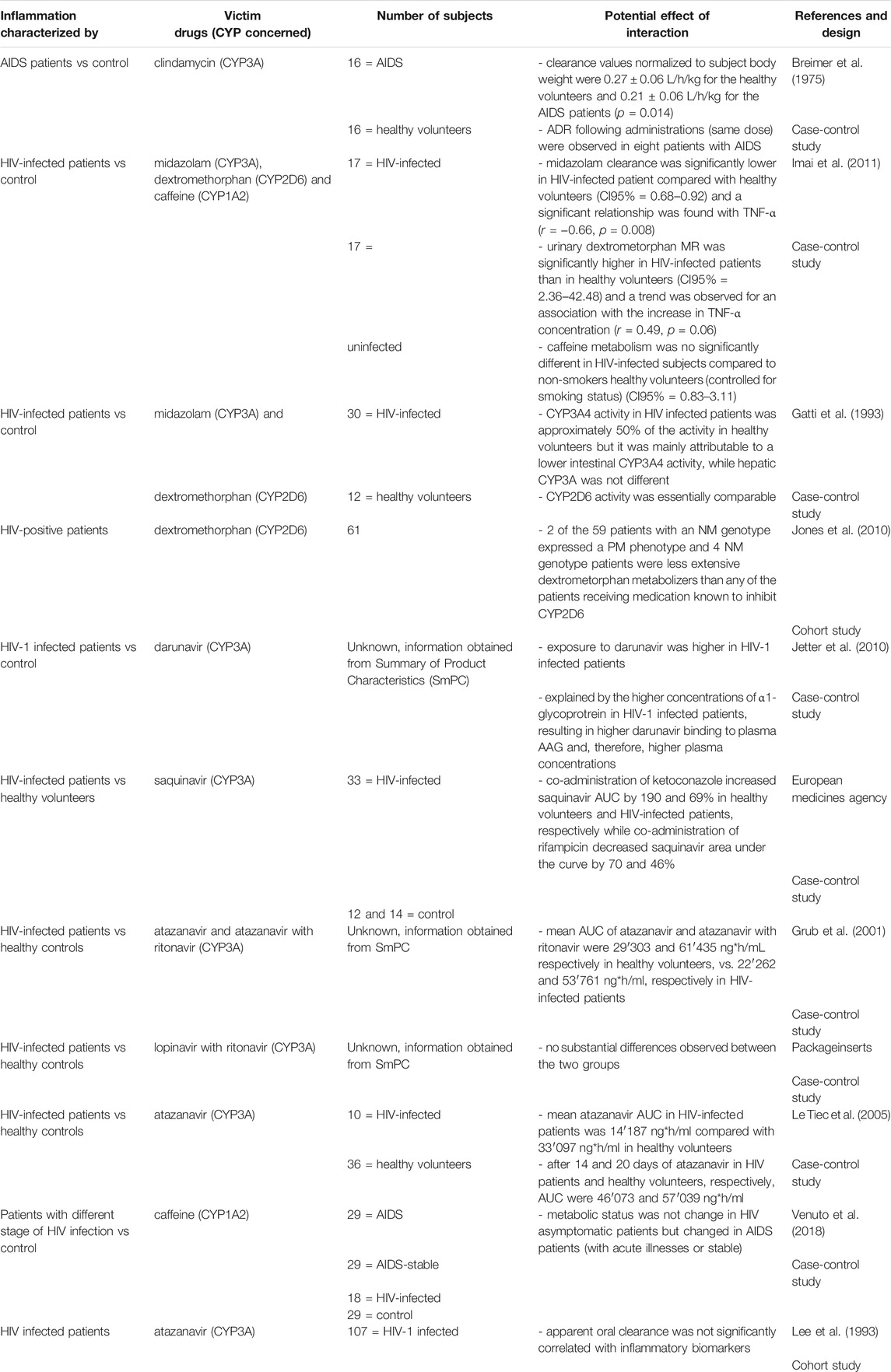
TABLE 3C. Impact of HIV on CYP substrates, explained totally or partially by modulation of CYP activity.
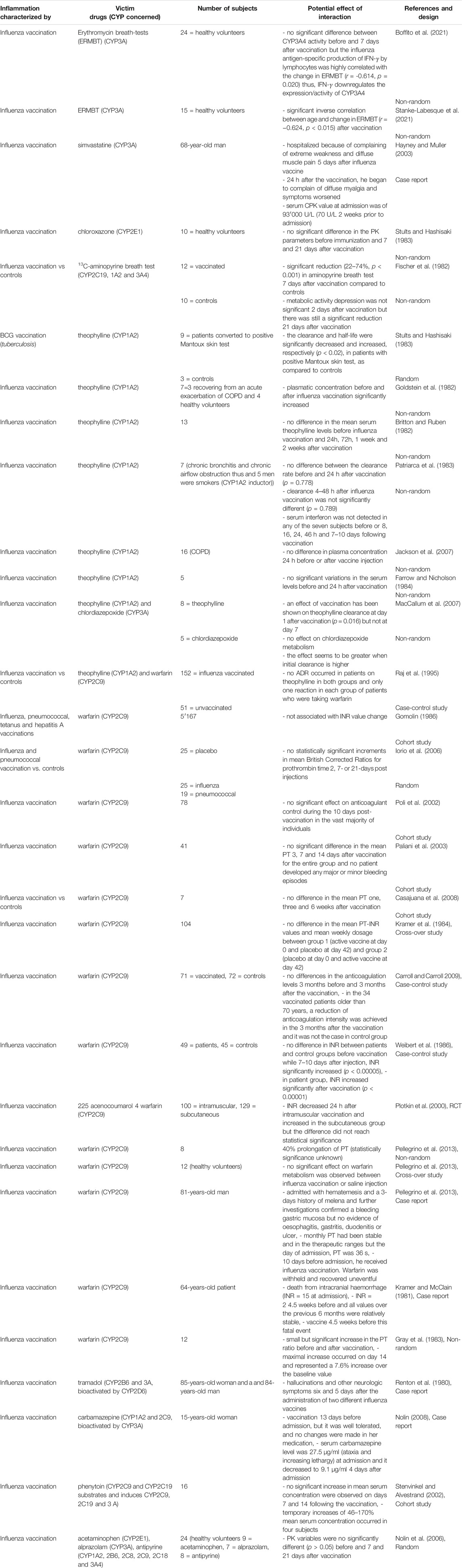
TABLE 3. Impact of vaccination on CYP substrates, explained totally or partially by modulation of CYP activity.
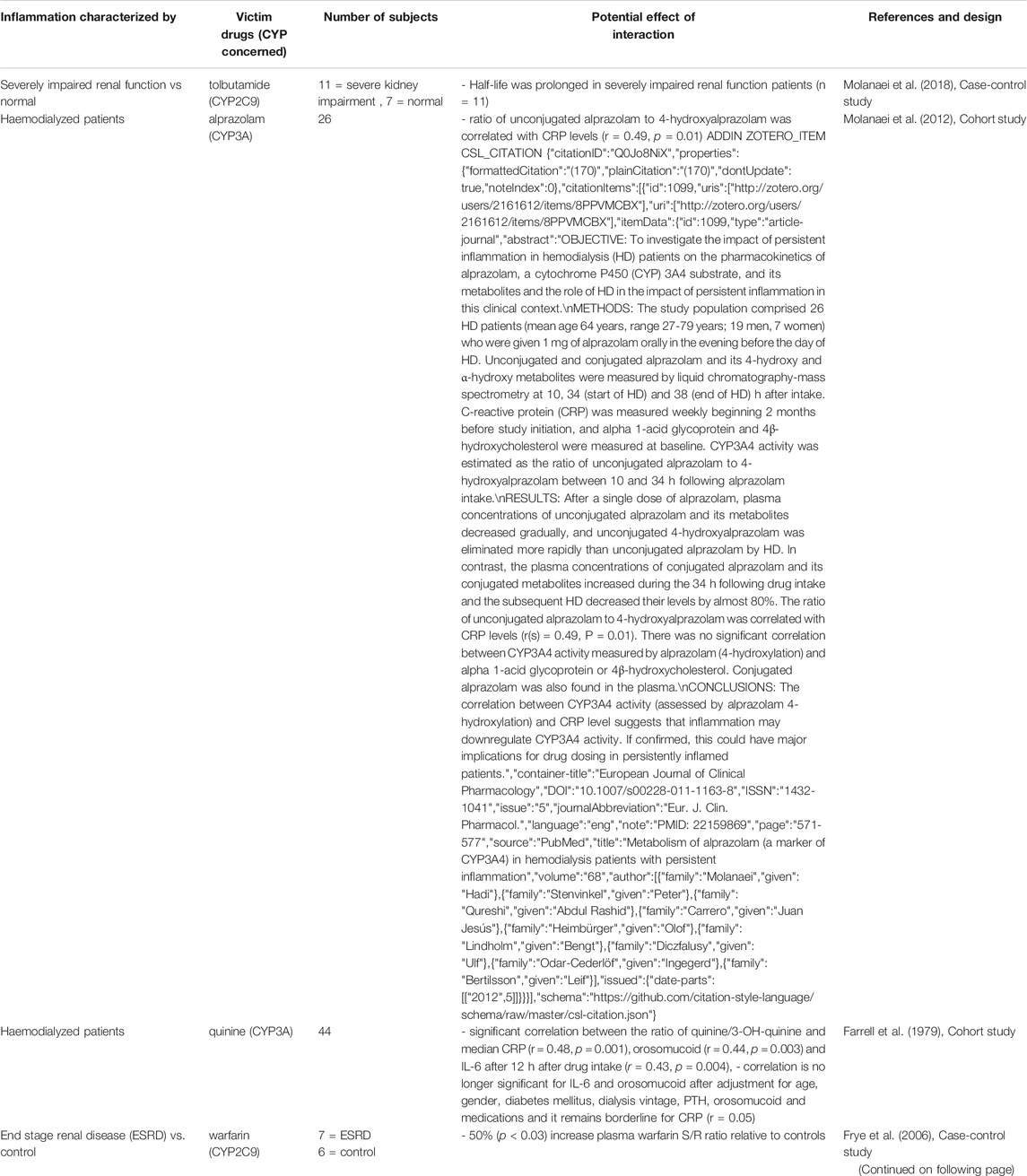
TABLE 4. Impact of renal diseases on CYP substrates, explained totally or partially by modulation of CYP activity.
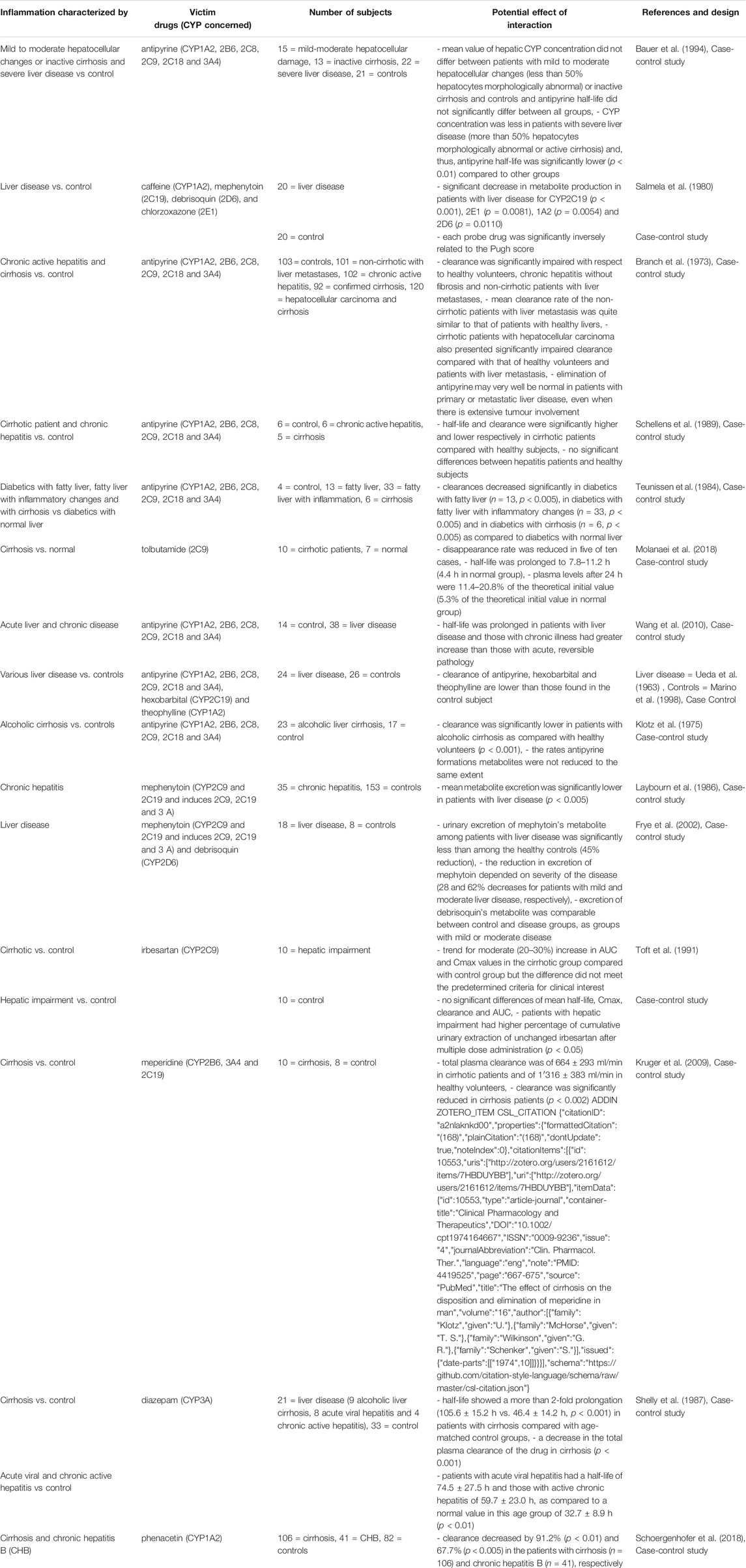
TABLE 5. Impact of liver diseases on CYP substrates, explained totally or partially by modulation of CYP activity.

TABLE 7. Impact of cardiac diseases on CYP substrates, explained totally or partially by modulation of CYP activity.
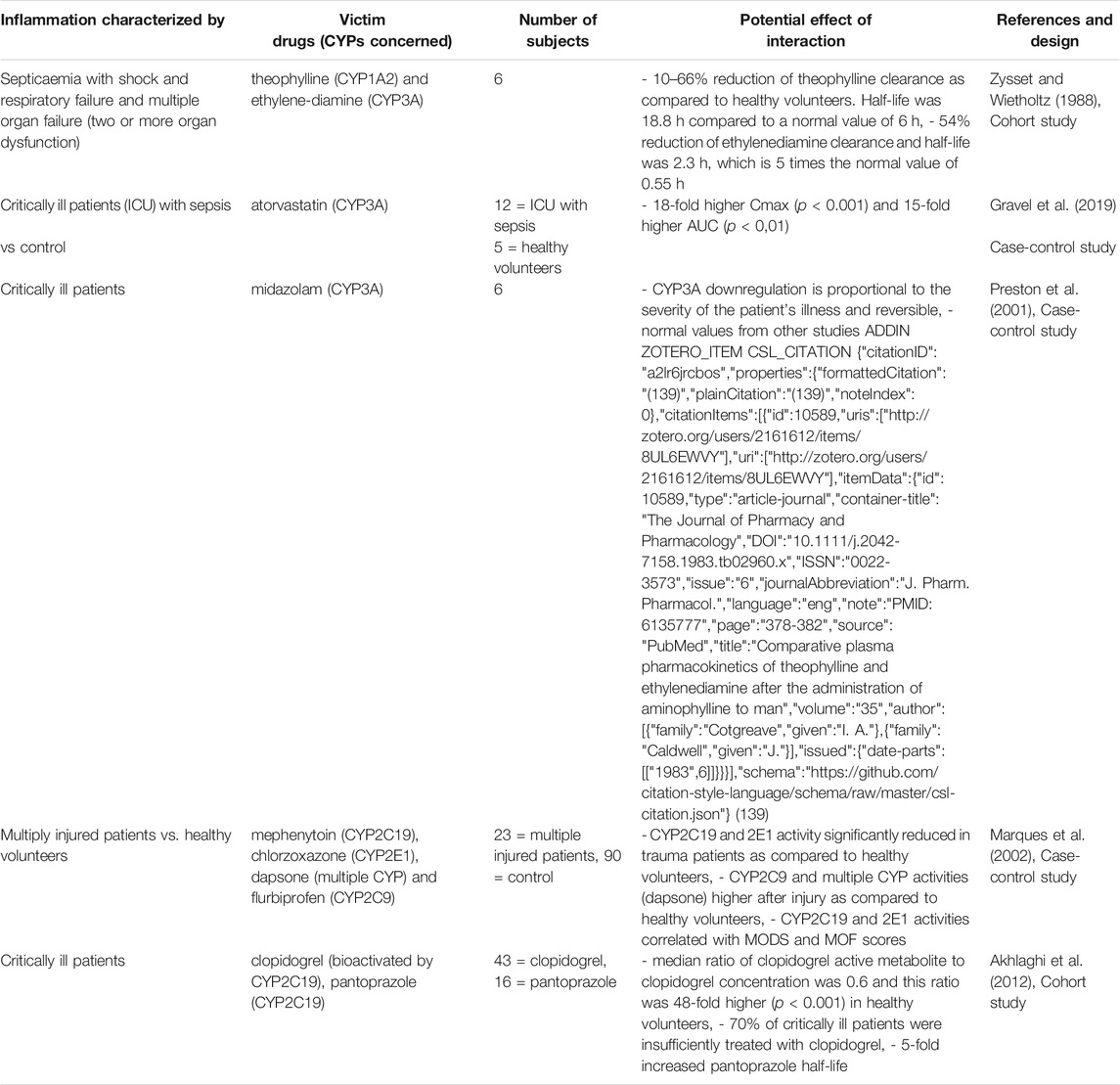
TABLE 8. Impact of critically ill patients on CYP substrates, explained totally or partially by modulation of CYP activity.
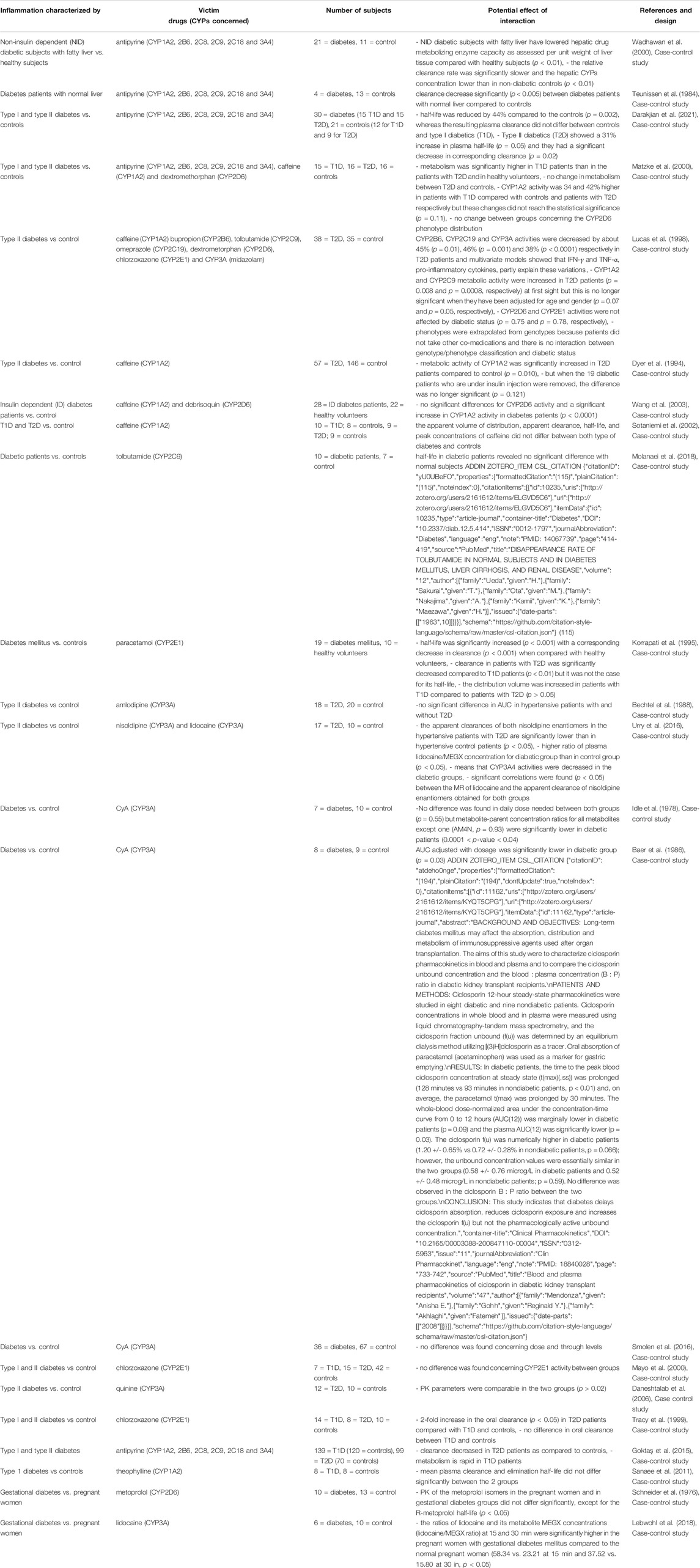
TABLE 9. Impact of diabetes on CYP substrates, explained totally or partially by modulation of CYP activity.
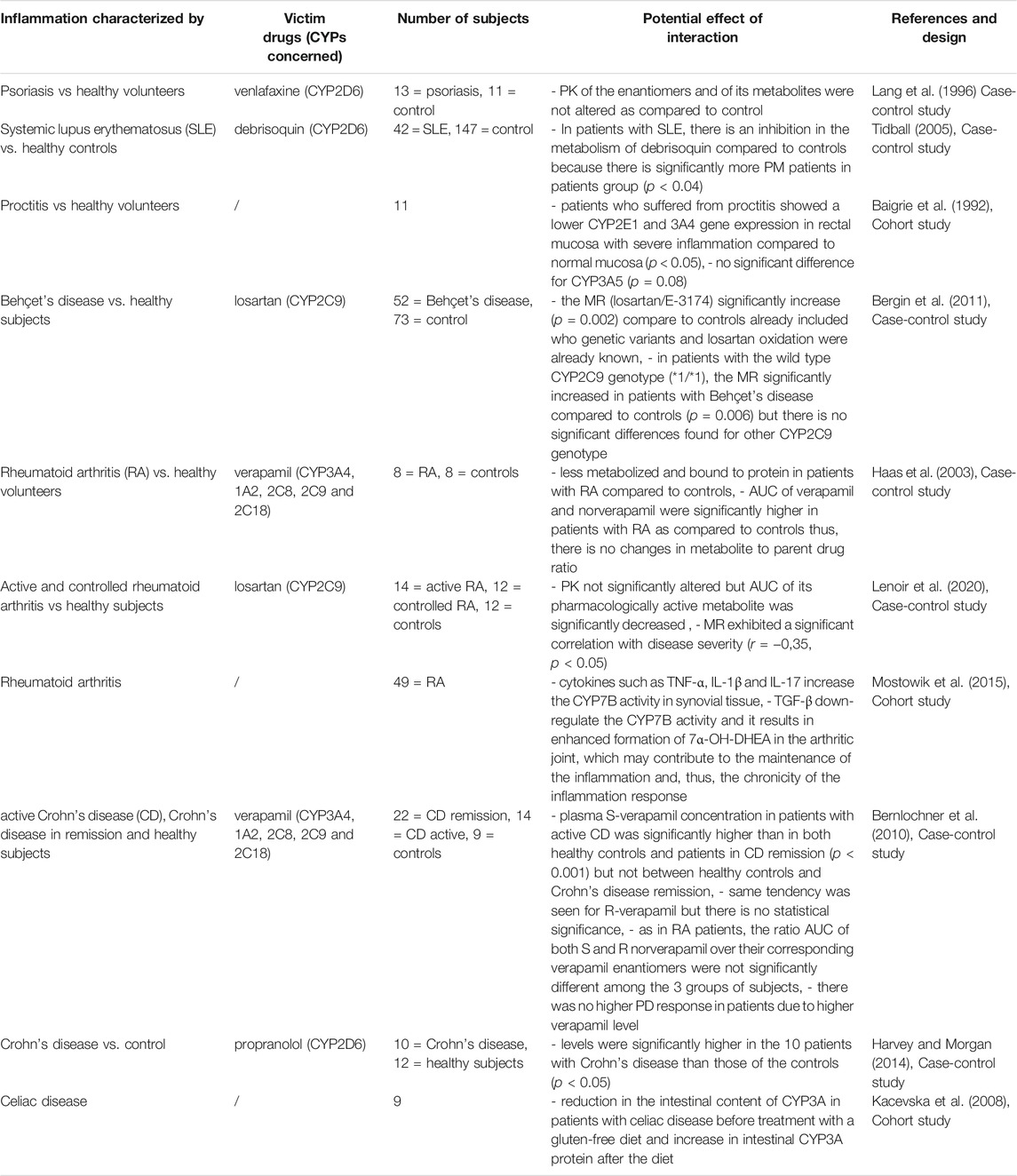
TABLE 10. Impact of autoimmune diseases on CYP substrates, explained totally or partially by modulation of CYP activity.
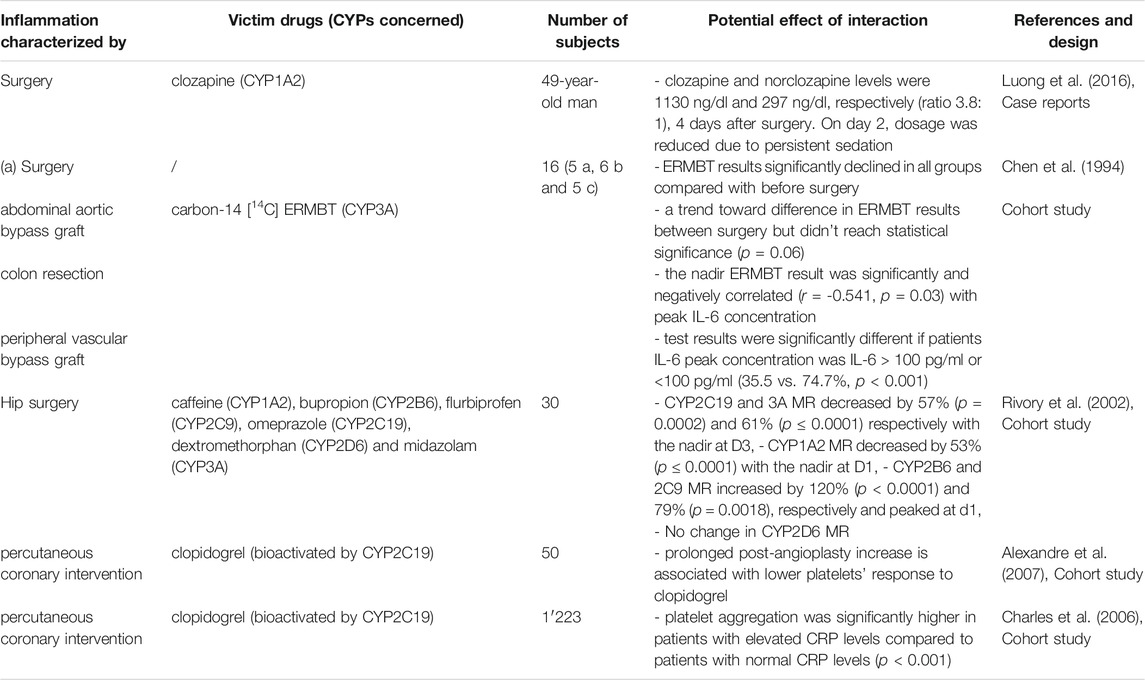
TABLE 11. Impact of surgery on CYP substrates, explained totally or partially by modulation of CYP activity.
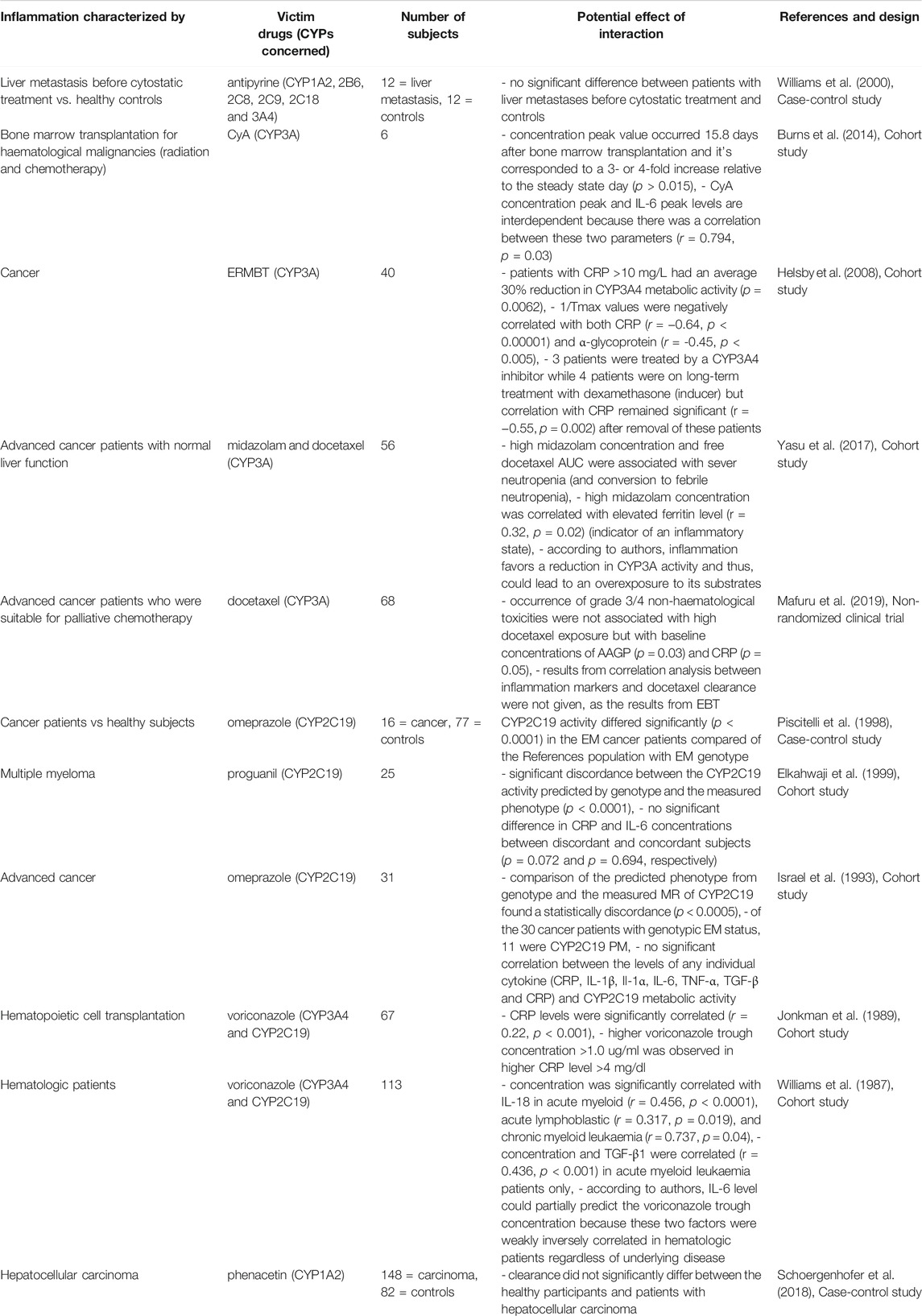
TABLE 12. Impact of cancer on CYP substrates, explained totally or partially by modulation of CYP activity.
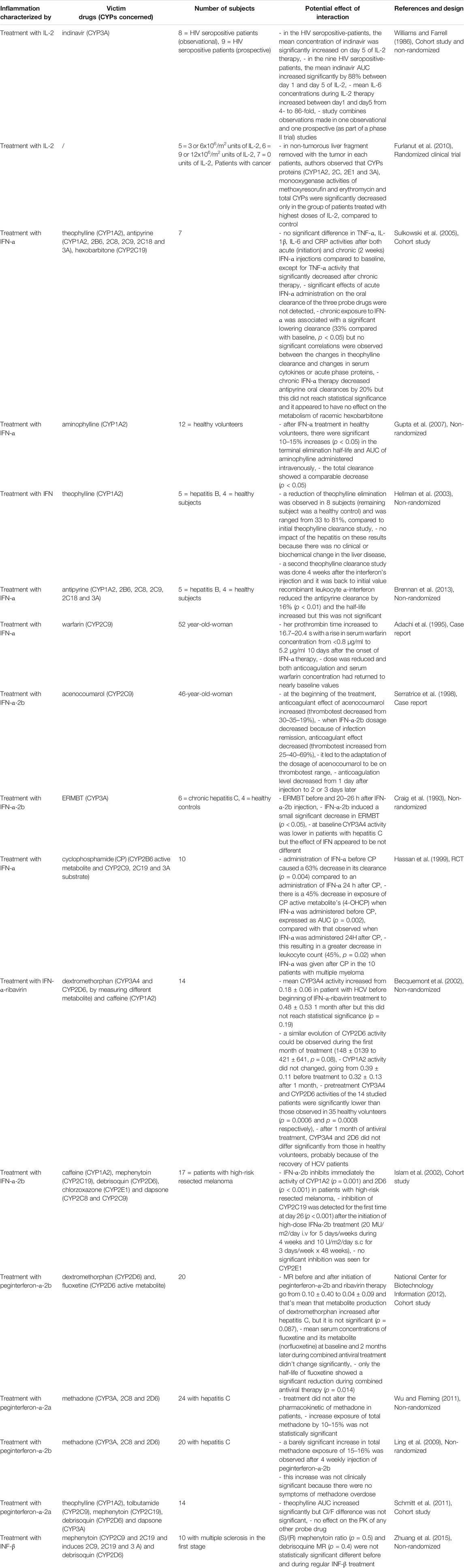
TABLE 13. Impact of therapies with immunomodulator on CYP substrates, explained totally or partially by modulation of CYP activity.
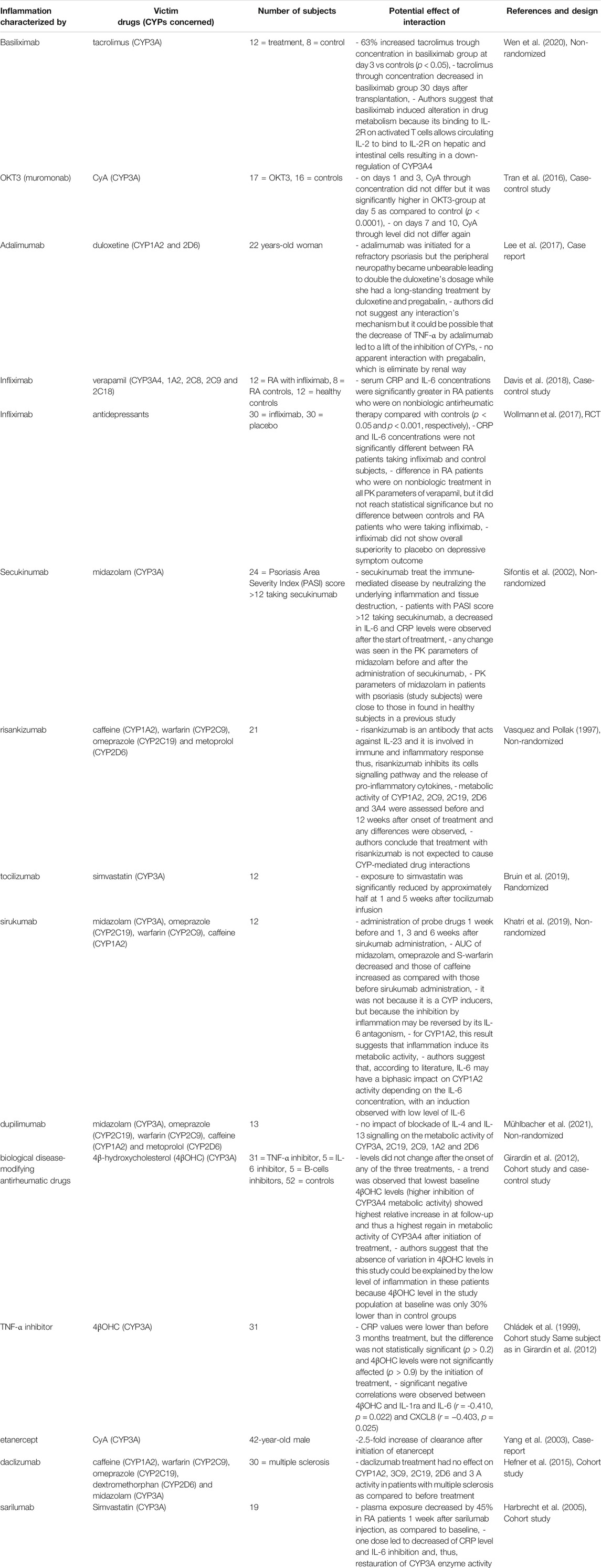
TABLE 14. Impact of therapies with anti-TNF-α and -mabs on CYP substrates, explained totally or partially by modulation of CYP activity.
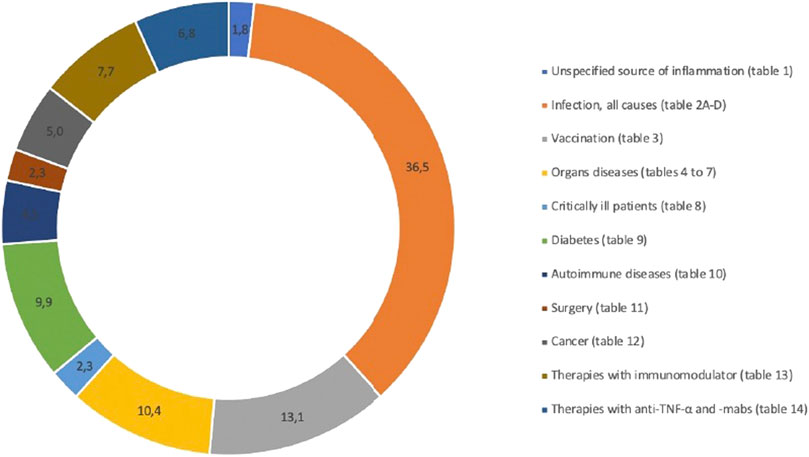
FIGURE 2. Distribution (%) of included references according to the different sources of inflammation.
Infection
Several studies have assessed the association between infection, represented by elevated levels of CRP, and PK variations of voriconazole. This is of particular interest and voriconazole therapeutic drug monitoring should thus be used to optimize clinical success and safety in these settings (Luong et al., 2016). Increased levels of CRP were correlated with increased voriconazole concentrations or decreased metabolic ratio of voriconazole/N-oxide and this could be explained by CYP2C19 and/or CYP3A downregulation, as voriconazole is mainly metabolized by these two CYPs (van Wanrooy et al., 2014; Encalada Ventura et al., 2015; Dote et al., 2016; Niioka et al., 2017; Vreugdenhil et al., 2018; Schulz et al., 2019). A positive correlation between inflammatory markers and voriconazole concentration was seen in adults, as well as with the severity of infection (van Wanrooy et al., 2014; Dote et al., 2016; Veringa et al., 2017; Gautier-Veyret et al., 2019). Drug metabolism appears to be influenced by the degree of inflammation and standardization of the classification of inflammatory markers elevation seems necessary (van Wanrooy et al., 2014; Niioka et al., 2017; Veringa et al., 2017; Gautier-Veyret et al., 2019). Indeed, voriconazole through concentration increased by 0.015 mg/L every 1 mg/L increase in CRP, and a recent meta-analysis showed that an increase in voriconazole through concentration of 6, 35 and 82% was associated with an increase in the CRP level of 10, 50 and 100 mg/L, respectively (van Wanrooy et al., 2014; Bolcato et al., 2021). As a final evidence to support of a correlation between inflammation and CYP downregulation, inflammation, and its resolution, decreased, and increased voriconazole clearance respectively, suggesting that the improvement of the inflammation allows a return to the baseline (Dote et al., 2016). However, no studies have investigated the duration of the resolution of inflammation-induced metabolic phenoconversion (Stanke-Labesque et al., 2020). This is an important limitation to allow individualization of treatment without therapeutic drug monitoring (TDM), as under-exposure to drug remains a risk (Stanke-Labesque et al., 2020).
CYP downregulation was also demonstrated as a consequence of sufficient inflammation and significant temperature elevation (Elin et al., 1975). Therefore, caution should be exercised in case of infection when administering CYP substrates, as this may result in toxicity and ADRs (Vozeh et al., 1978; Blumenkopf and Lockhart, 1983; Levine and Jones, 1983 1; Raaska et al., 2002; Haack et al., 2003; de Leon and Diaz, 2003; Jecel et al., 2005; Darling and Huthwaite, 2011; Espnes et al., 2012; Kwak et al., 2014; Leung et al., 2014; Takahashi et al., 2015; Clark et al., 2018; Khan and Khan, 2019).
Early works assessed the effect of an infection induced intentionally by lipopolysaccharides (LPS) injection on antipyrine pharmacokinetics, and several studies have assessed the impact of infection on psychotropic agents (clozapine, risperidone). The increase of clozapine levels, a CYP1A2 substrate, due to inflammation has been well studied and demonstrated (Raaska et al., 2002; Haack et al., 2003; de Leon and Diaz, 2003; Jecel et al., 2005; Pfuhlmann et al., 2009; Darling and Huthwaite, 2011; Espnes et al., 2012; Abou Farha et al., 2012; Leung et al., 2014; Kwak et al., 2014; Takahashi et al., 2015; ten Bokum et al., 2015; Hefner et al., 2016; Ruan et al., 2017; Clark et al., 2018; Ruan et al., 2018; Ruan et al., 2020). A positive and significant correlation between clozapine and CRP levels (r = 0.313, p < 0.01) was found, with a 2- to 6-fold increase in serum levels and the development of toxic symptoms, as well as improvement after dose reduction or infection recovery (Raaska et al., 2002; Haack et al., 2003; de Leon and Diaz, 2003; Jecel et al., 2005; Pfuhlmann et al., 2009; Darling and Huthwaite, 2011; Espnes et al., 2012; Kwak et al., 2014; Leung et al., 2014; Takahashi et al., 2015; ten Bokum et al., 2015; Hefner et al., 2016; Abou Farha et al., 2012; Ruan et al., 2017; Clark et al., 2018; Ruan et al., 2018; Ruan et al., 2020). Further investigations are needed concerning anticoagulant therapy, as only one case of severe bleeding in the context of infection was reported in the literature (Blumenkopf and Lockhart, 1983). First observation of a return to baseline metabolic activity after the end of the disruption that caused inflammation dates from 1985, with the gradual improvement of antipyrine clearance in days after the resolution of pneumonia (Sonne et al., 1985). Later, other authors demonstrated metabolic recovery after improvement of a liver fluke infection following praziquantel treatment (Satarug et al., 1996).
In hepatitis (Table 2B), a study suggested an overall downregulation of several hepatic CYPs and transporters with liver fibrosis progression, although the mechanisms of regulation differed and large inter-individual variation existed (Hanada et al., 2012). Indeed, this study assessed that the mRNA level was largely dependent on fibrosis stage and that the role of the different nuclear receptors tested is not the same in the hepatic expression of each CYP isoenzyme (Hanada et al., 2012). CYP3A4 downregulation during HCV infection has been well-described (McHorse et al., 1975; Tuncer et al., 2000; Latorre et al., 2002; Wolffenbüttel et al., 2004). Indeed, numerous studies have described a higher drug exposure of the two most commonly used immunosuppressants, tacrolimus and cyclosporine A, in patients with hepatitis and especially in those with viremia (Tuncer et al., 2000; Latorre et al., 2002; Wolffenbüttel et al., 2004). Moreover, when HCV is treated, CYP activities appear to return to baseline levels in several studies (McHorse et al., 1975; van den Berg et al., 2001; Kugelmas et al., 2003; Ueda et al., 2015; Kawaoka et al., 2016; Saab et al., 2016; Raschzok et al., 2016; Ueda and Uemoto, 2016; Smolders et al., 2017). Indeed, through concentration of tacrolimus decreased after initiation of HCV treatment, such as sofosbuvir, daclatasvir, asunaprevir, simeprivir, ribavirin and interferon, administered alone or in combination, and it required a dosage increase (Kawaoka et al., 2016; Raschzok et al., 2016; Saab et al., 2016; Smolders et al., 2017). Subgroups were identified, such as patients not responding to interferon with higher CYP3A downregulation related to higher levels of circulating cytokines, confirming that CYP modulation is proportional to intensity of inflammation (Morcos et al., 2013). However, conflicting results exist, and clinical recovery from acute liver disease was not accompanied by a corresponding recovery of drug-metabolizing capacity in a study (Breimer et al., 1975). This could be due to a lag between the return to baseline CYP levels and recovery, as clinical recovery from liver disease is not accompanied by a corresponding recovery of drug metabolizing capability (Breimer et al., 1975). Indeed, it is generally recognized that recovery half-lives are approximatively 20–50 h after mechanism-based inhibition and 40–60 h after enzyme induction (Imai et al., 2011).
Several studies have examined the impact of HIV on CYP metabolism (Table 2C) and have shown that several concomitant treatments and antiretroviral drugs metabolized by CYP3A have reduced metabolism in HIV-infected individuals, with an increased risk of ADRs. For instance, clindamycin clearance decreased from 0.27 in healthy volunteers to 0.21 L/h/kg in AIDS patients (p = 0.014) and a negative correlation between TNF-α and midazolam clearance was found (Gatti et al., 1993; Jones et al., 2010). Moreover CYP3A inhibitor (ketoconazole or ritonavir) and inducer (rifampicin) effects were less pronounced on antiviral PK in HIV-patients (Gatti et al., 1993; Grub et al., 2001; Jetter et al., 2010; European medicines agency; Packageinserts). It is important to characterize CYP3A modulation in HIV, as many antiviral treatments are metabolized by this pathway, and this could lead to efficacy or safety concerns. However, the AUC of atazanavir was lower in HIV-infected patients than in healthy volunteers and this could be explained by the absence of correlation between its oral clearance and inflammatory markers in a cohort study, the lack of identical study conditions (doses, sample schedule, meals … etc.) between the two groups and the fact that HIV infection was well-controlled (Packageinserts; Le Tiec et al., 2005; Venuto et al., 2018). Indeed, caffeine metabolism was not altered in HIV-infected patient compared with healthy volunteers, but was decreased in AIDS patients (Lee et al., 1993; Jones et al., 2010). Moreover, atazanavir was administered with the booster ritonavir to decrease its clearance, and the effect of inflammation could have been minimized.
More recently, some studies have shown increased plasma concentration of CYPs substrates (mostly CYP3A) during SARS-CoV-2 infection, which may have led to believe that there was a CYPs downregulation due to inflammation (Table 2D) (Cojutti et al., 2020; Cranshaw and Harikumar, 2020; Gregoire et al., 2020; Marzolini et al., 2020; Schoergenhofer et al., 2020; Testa et al., 2020). Indeed, the plasma concentrations of some CYP3A substrates (lopinavir, darunavir and direct oral anticoagulants) were significantly increased in patients with SARS-CoV-2 infection (Cojutti et al., 2020; Gregoire et al., 2020; Schoergenhofer et al., 2020; Testa et al., 2020). CRP and IL-6 were also associated with lopinavir concentrations and a trend toward a return to baseline was observed after treatment with tocilizumab (Marzolini et al., 2020; Schoergenhofer et al., 2020). Indeed, lopinavir through level in patients with SARS-CoV-2 infection was twice as high as in HIV patients but concentrations decreased when tocilizumab was administered (Marzolini et al., 2020; Schoergenhofer et al., 2020). However, the impact of inflammation induced by SARS-CoV-2 infection on lopinavir through concentration may be also due to increased orosomucoid levels (Boffito et al., 2021; Stanke-Labesque et al., 2021). Lopinavir is a highly protein-bound drug and the misinterpretation of its overexposure during inflammation could be explained by the fact that total and not unbound concentration was considered (Boffito et al., 2021; Stanke-Labesque et al., 2021). Furthermore, a case report described clozapine toxicity and increased clozapine level from 0.57 to 0.73 mg/L during SARS-CoV-2 infection (Cranshaw and Harikumar, 2020). However, no correlation was found between CRP and hydroxychloroquine plasma concentrations (Marzolini et al., 2020).
Vaccination
Regarding vaccination (Table 3), several reports and studies assessed variations of PK/PD parameters of drugs after vaccination, but data remain contradictory. Of the 31 articles included, 28 were exclusively about influenza vaccination while two were about concomitant vaccinations including influenza (pneumococcus, tetanus and hepatitis A). Only one article did not evaluate the influenza vaccination but reported on the impact of tuberculosis vaccination (BCG). No significant difference of CYP activity between before or after vaccination was shown in several studies (Britton and Ruben, 1982; Fischer et al., 1982; Goldstein et al., 1982; Patriarca et al., 1983; Stults and Hashisaki, 1983; Stults and Hashisaki, 1983; Hayney and Muller, 2003). In particular, the impact of vaccination on anticoagulants effects has been well-studied but the majority of studies showed no variation of PT time or INR (Farrow and Nicholson, 1984; Kramer et al., 1984; Gomolin, 1986; Raj et al., 1995; Poli et al., 2002; Paliani et al., 2003; Iorio et al., 2006; Jackson et al., 2007; MacCallum et al., 2007; Casajuana et al., 2008). However, the occurrence of bleeding events a few days after vaccination, when the PT time was previously stable, has been described (Kramer et al., 1984; Weibert et al., 1986; Carroll and Carroll, 2009). Moreover, the case of a patient hospitalized because of serum CPK level of 93,000 U/L during treatment with cerivastatin and bezafibrate or the occurrence of tramadol toxicity has been reported (Plotkin et al., 2000; Pellegrino et al., 2013). The patient had been vaccinated 5 days earlier (Plotkin et al., 2000). Other studies, few in number, have found an effect of vaccination on the PK of CYP substrates (Renton et al., 1980; Kramer and McClain, 1981; Gray et al., 1983). However, no study has correlated the data with pro-inflammatory markers.
Organs Diseases
The influence of liver and kidney function on disposition of drugs excreted by the liver and kidney is widely recognized and used to derive dosing adaptations. However, there is now an increasing appreciation that kidney impairment can also reduce non-renal clearance and alter the bioavailability of drugs predominantly metabolized by the liver (Nolin, 2008). Indeed, uremic toxin has been implicated in transcriptional, translational and acute posttranslational modifications of CYP, and it has been recognized that inflammation is a common feature in end-stage renal disease (ESRD) patients (Nolin, 2008; Stenvinkel and Alvestrand, 2002). For example, CYP3A activity increased post-dialysis, meaning that it is the presence of uremic toxin that is responsible for CYP downregulation and not the underlying disease (Nolin et al., 2006). An inverse relationship between hepatic CYP3A activity was found in this study, but it did not prove causality (Nolin et al., 2006). It indicates that uremia can be used as a surrogate for dialyzable toxins that contribute to alterations in CYP3A function (Nolin et al., 2006). Indeed, hemodialysis improved CYP3A activity with a 27% increase 2 h post-dialysis in uremic patients, suggesting that potential toxins responsible for this alteration were removed (Nolin et al., 2006). Authors suggested that this improvement occurred independently of transcriptional or translational modifications, contrary to what has been suggested previously (Nolin et al., 2006). However, as shown in Table 4, two studies found an association between the modification of CYP activity and inflammation in ESRD patients (Molanaei et al., 2012; Molanaei et al., 2018).
All studies in patients with liver disease described a decrease in CYP activity, compared to controls, as shown in Table 5. Indeed, several studies studied antipyrine, an old drug that is metabolized by multiple CYP (Branch et al., 1973; Farrell et al., 1979; Salmela et al., 1980; Teunissen et al., 1984; Schellens et al., 1989; Bauer et al., 1994; Grieco et al., 1998; Frye et al., 2006). They showed that CYP activity and antipyrine metabolism decreased only in severe disease compared to inactive cirrhosis, mild-moderate liver disease or healthy volunteers (Farrell et al., 1979; Bauer et al., 1994; Grieco et al., 1998). Moreover, chronic liver disease appeared to have a higher impact than an acute/reversible pathology (Branch et al., 1973). However, few studies have focused on a specific CYP substrate, and no studies found an association with inflammatory markers. One study demonstrated that CYP2C19, 2E1, 1A2 and 2D6 probe drugs concentrations were inversely correlated to the Child-Pugh score and another one demonstrated that phenacetin clearance decreased by 90% in patients with cirrhosis (Frye et al., 2006; Wang et al., 2010). Concerning CYP2C9, tolbutamide plasma levels increased by 10–20% and irbesartan AUC increased by 20–30% in cirrhotic patients (Ueda et al., 1963; Marino et al., 1998). The same results were found with CYP3A as diazepam clearance decreased in cirrhosis (Klotz et al., 1975). These variations may therefore be attributed to the loss of liver function due to tissue destruction. CYP metabolism appeared to be influenced by other organ’s disease, such as clozapine serum levels that increased by 2-fold during chronic obstructive pulmonary disease (COPD) exacerbation and antipyrine clearance that was significantly lower in patient with COPD and antitrypsin deficiency than in healthy volunteers (Laybourn et al., 1986; Leung et al., 2014). In addition, one study showed that inflammatory markers were inversely correlated with CYP1A2 and CYP2C19 activity but not with CYP2D6 and CYP2E1 activity in patients with congestive heart failure (Frye et al., 2002).
Some studies conducted in critically ill patients (Table 8), showed that CYP1A2 and 3A metabolic activity were downregulated, and that it may be proportional to the severity and reversibility of the illness (Shelly et al., 1987; Toft et al., 1991; Kruger et al., 2009). For instance, theophylline clearance decreased by 10–66%, atorvastatin AUC increased by 15-fold, and clopidogrel active metabolite decreased by 48-fold, raising concerns about treatment efficacy (Toft et al., 1991; Kruger et al., 2009; Schoergenhofer et al., 2018). However, a systematic review reported that 20–65% of critically patients had an increased renal clearance, defined as a creatinine clearance greater than 130 ml/min/1.73 m2 (Bilbao-Meseguer et al., 2018). This underscores the fact that inflammation has a different effect on drug clearance through the different mechanisms of drug elimination.
Diabetes
In diabetes (Table 9), CYP metabolism has been shown to be downregulated (Salmela et al., 1980; Pirttiaho et al., 1984). Indeed, antipyrine metabolism was decreased compared with controls in several studies (Salmela et al., 1980; Pirttiaho et al., 1984; Zysset and Wietholtz, 1988). One study using a cocktail approach showed that CYP2B6, CYP2C19 and CYP3A activity decreased, CYP1A2 and CYP2C9 activity increased, and CYP2D6 and CYP2E1 activity was unaffected in type II diabetes (T2D) (Gravel et al., 2019). However, conflicting results exist with tolbutamide and paracetamol half-lifes which were unchanged and increased respectively (Ueda et al., 1963; Adithan et al., 1988). Regarding CYP3A, one study found no impact on amlodipine or immunosuppressant metabolism while nisoldipine clearance was decreased (Wadhawan et al., 2000; Preston et al., 2001; Marques et al., 2002; Akhlaghi et al., 2012). The underlying mechanisms are associated with systemic inflammation and inflammatory cytokines. Indeed, it is well-established that chronic inflammation is involved in the pathophysiology of diabetes and the more complex condition of metabolic syndrome (Gravel et al., 2019). TNF-α can lead to the development of diabetes by affecting insulin action, and levels of inflammatory cytokines and markers are reported to be increased in diabetes patients (Darakjian et al., 2021). In a multivariate analysis, IFN-γ, IL-1β, IL-6 and TNF-α were associated with CYP activities, depending on the CYP isoenzyme (Gravel et al., 2019). However, type I (T1D) and type II diabetes did not appear to have the same impact on CYP metabolism (Dyer et al., 1994; Korrapati et al., 1995; Lucas et al., 1998; Zysset and Wietholtz, 1988; Matzke et al., 2000; Sotaniemi et al., 2002; Wang et al., 2003). The impact of inflammation may be different partly because of obesity, which is more common in T2D (Wang et al., 2003). Indeed, obese patients had a 40% increase in CYP2E1 activity (Lucas et al., 1998; Wang et al., 2003). CYP2E1 increased activity could also be attributed to hypo-insulinemia, as administration of insulin reverses this induction at the mRNA level (Lucas et al., 1998). Moreover, moderate controlled T1D had comparable CYP2E1 activity to healthy volunteers (Wang et al., 2003). This was confirmed in other studies that showed an unaffected metabolic clearance rate of antipyrine in well-controlled (by insulin) T1D (Zysset and Wietholtz, 1988; Sotaniemi et al., 2002). This could also be explained by insulin supplementation and the subsequent correction of ketones that leads to a return to baseline level for CYP2E1 expression (Wang et al., 2003). Indeed, ketones have been shown to be an important modulator of CYP2E1 by enhancing its protein expression and mRNA level (Wang et al., 2003). This has been confirmed with CYP1A2, where fluctuations in growth hormone levels, hyperketonemia and variation in glucose metabolic steady state and HbA1C levels may contribute to these changes (Bechtel et al., 1988; Korrapati et al., 1995; Matzke et al., 2000). The difference in classification criteria for T1D and type 2 diabetes may explain the inconsistent findings (Matzke et al., 2000). Further studies to discriminate between these two entities are needed (Zysset and Wietholtz, 1988).
Overall, CYP3A, 2C19 and 2B6 activity appear to be downregulated while CYP1A2 activity was increased and CYP2D6 activity was unchanged in diabetic patients (Bechtel et al., 1988; Urry et al., 2016; Gravel et al., 2019). Conflicting results remain regarding CYP2C9 and CYP2E1 (Ueda et al., 1963; Adithan et al., 1988; Lucas et al., 1998; Gravel et al., 2019).
Auto-Immune Diseases
Few studies observed the impact of auto-immune disease on CYP activities, such as psoriasis, systemic lupus erythematosus (SLE), Behçet’s disease, rheumatoid arthritis (RA), Crohn’s disease and celiac disease (Table 10). In contrast to what has been observed for CYP2D6 in other inflammatory states, two studies observed CYP2D6 downregulation in patient with SLE (Idle et al., 1978; Baer et al., 1986). However, these studies have some limitations, such as the presence of concomitant medications inhibiting the metabolism of CYP2D6 and the absence of adequate randomization (Baer et al., 1986). Even though RA is one of the most prevalent chronic inflammatory disease, only two case-control studies were found in the literature studying the impact of RA on the PK and PD of verapamil and losartan, respectively (Mayo et al., 2000; Daneshtalab et al., 2006; Smolen et al., 2016). Verapamil is metabolized by CYP3A and 1A2 into norverapamil (Tracy et al., 1999). Verapamil and norverapamil metabolism has been shown to be reduced in patients with RA compared to healthy volunteers (Mayo et al., 2000). Verapamil was not more dromotropic or hypotensive in RA patients (Mayo et al., 2000). Inhibition of CYP2C9 was proportional to RA disease severity in another study, but this was not accompanied by reduced clinical response after losartan administration (Daneshtalab et al., 2006). Same results were found in patients with Behcet’s disease. Indeed, one study observed downregulation of CYP2C9 in Behcet’s patients (Goktaş et al., 2015). However, losartan’s MR in nine patients with Behçet’s disease taking colchicine were similar to those not taking colchicine (Goktaş et al., 2015). This may be because the drug had been taken for only 2 weeks (Goktaş et al., 2015).
In Crohn’s disease, S-verapamil concentration was higher than R-verapamil while the opposite was found in normal conditions and higher plasma levels of propranolol were found in Crohn’s with reduced metabolic activities of CYP1A2, 2D6 and 2C19 (Schneider et al., 1976; Sanaee et al., 2011). Furthermore, there were no difference between healthy controls and Crohn’s disease patients in remission, implying that CYP downregulation is proportional to disease severity and that recovery resulted in a return to baseline metabolic activity (Sanaee et al., 2011). Norverapamil goes through the same process and it is expected that the enantiomers ratio of norverapamil to verapamil remains unchanged (Sanaee et al., 2011).
Celiac disease is an autoimmune disease that is triggered by an immune response to gluten and may result in increased morbidity or mortality (Lebwohl et al., 2018). The reduction in intestinal CYP3A content during celiac disease and its increase after a gluten-free diet indicate that local inflammation reduced CYP3A activity but that it returns to baseline with disease improvement (Lang et al., 1996).
Surgery
The impact of surgery on concomitant treatment and analgesia management has been assessed in several studies (Table 11). Surgery is associated with an inflammatory response due to muscle or tissue injury to induce repair, regeneration and growth and so inflammatory markers increase after surgery, but not equally (Tidball, 2005; Stavropoulou et al., 2018). IL-1β was only detected during the early perioperative period and for a very short time (Baigrie et al., 1992). IL-6 plasma level peaked 4–48 h after surgery and declined drastically by 48–72 h in all patients without any postoperative complication (Baigrie et al., 1992). CRP level rose more slowly postoperatively compared with the cytokine levels (IL-6, TNF-α and IL-1β) (Bergin et al., 2011). Acute inflammation after elective surgery was associated with a significant decrease in CYP3A metabolic activity (Haas et al., 2003). A recent study with a cocktail approach has concluded that there is an isoform specific impact of inflammation on CYP activities (Lenoir et al., 2020). Indeed, this study showed that CYP1A2, CYP2C19 and CYP3A activities decreased significantly by 53, 57 and 61%, whereas CYP2B6 and CYP2C9 activities increased significantly by 120 and 79% (Lenoir et al., 2020). However, surgery did not significantly impact CYP2D6 activity (Lenoir et al., 2020). These findings were confirmed by a case report that showed a toxic increase in clozapine levels 4 days after surgery and by authors who further showed that clopidogrel efficacy was reduced in patients undergoing percutaneous coronary intervention, because clopidogrel must be bioactivated by CYP2C19 to be effective (Bernlochner et al., 2010; Leung et al., 2014; Mostowik et al., 2015).
Cancer
Inflammation is linked to all stages of cancer (risk of development, initiation, invasion, metastasis and mortality) as highlighted in Table 12 (Harvey and Morgan, 2014). Certain immune-mediated diseases have been associated with cancer such as inflammatory bowel disease (IBD), chronic infection by Helicobacter pylori and chronic psoriasis associated with an increased risk of colorectal, gastric and skin cancer, respectively (Harvey and Morgan, 2014). The first pro-cancer immune signals are via tumor cells that successively produce cytokines and act to increase transcription factors, induce epigenetic changes and initiate angiogenesis (Harvey and Morgan, 2014). Cytokines are involved from neoplastic transformation of cells to tumor progression and metastasis, and are thus involved in several cellular events leading to cancer (Kacevska et al., 2008). These signals and others induced to respond to cancer are opposed by antigen-presentating cell-mediated anticancer immune responses (Harvey and Morgan, 2014). Moreover, the greater the antitumoral response is, the more the cancer outcome is improved whereas some T-cells subsets are associated with tumor promotion (Harvey and Morgan, 2014). Some cytokines have tumor-promoting, antitumor effects or both (Kacevska et al., 2008). Some cytokines could be produced by the tumor itself (Kacevska et al., 2008). Inflammation has therefore a pivotal role in cancer and the proliferation of malignant cells by a dynamic equilibrium in the tumor environment (Harvey and Morgan, 2014). Cytokines present in the tumor environment are also launched in the systemic circulation and have general effects on the function of distant organs such as the liver (Kacevska et al., 2008). Inflammatory markers levels are dependent on tumor types, but high level of CRP, IL-6, IL-1β have been associated with poor prognosis (Kacevska et al., 2008). Some results suggest that high IL-6 is associated with decreased CYP3A metabolic activity but can also nonspecifically downregulate CYP-dependent drug metabolism (Chen et al., 1994). CRP and α-glycoprotein were also negatively correlated with CYP3A activity and cancer patients with significant acute-phase response may have reduced CYP3A drug metabolism, which may have implications for the safety and efficacy of chemotherapy (Rivory et al., 2002; Charles et al., 2006; Alexandre et al., 2007). Inflammatory status and lymphocyte count should thus be included in the evaluation of the benefit/risk ratio before the initiation of a cytotoxic chemotherapy (Alexandre et al., 2007). Concerning CYP2C19, studies showed that CYP2C19 activity was not solely predicted by the genotype in cancer patients (Williams et al., 2000; Helsby et al., 2008; Burns et al., 2014). Indeed, CYP2C19 activity was reduced in cancer patients, with a discordance between the measured phenotype and the predicted phenotype from the genotype. However, no significant correlation was found between CYP2C19 activity and the levels of cytokine, whereas this was the case for voriconazole through concentration (Helsby et al., 2008; Burns et al., 2014; Yasu et al., 2017; Mafuru et al., 2019). The mechanism behind the decrease of CYP2C19 activity observed in cancer patients may be related to the inflammatory response even though it remains debated (Helsby et al., 2008; Burns et al., 2014; Yasu et al., 2017; Mafuru et al., 2019). Other authors showed that cancer has no impact on CYP1A2 metabolic activity as compared to liver disease or infection (Wang et al., 2010).
Therapies With Immunomodulator, anti-TNF-α and -Mabs
As biological therapies aim to decrease the underlying inflammation of the disease, interleukins (IL) injections are expected to have an impact on CYP activity, as underlined in Table 13. As an example, IL-2 doses of 9–12 × 106 units daily may downregulate CYP activities in patients with HIV infection and cancer in whom this treatment is administered to boost the immune system (Piscitelli et al., 1998; Elkahwaji et al., 1999). Conflicting results exist regarding IFN administration, with a discrepancy between acute and chronic treatment (Williams and Farrell, 1986; Williams et al., 1987; Jonkman et al., 1989; Israel et al., 1993; Hellman et al., 2003; Sulkowski et al., 2005; Gupta et al., 2007; Furlanut et al., 2010; Brennan et al., 2013). However, case reports and more specific studies assessing CYP metabolic activity lean toward CYP downregulation and care must be taken to avoid interactions and ADRs (Craig et al., 1993; Adachi et al., 1995; Serratrice et al., 1998; Hassan et al., 1999; Becquemont et al., 2002). The level of anticoagulation should be closely monitored when interferon is given together with warfarin, as it appears that CYP are downregulated (Adachi et al., 1995; Serratrice et al., 1998). Additionally, the timing of IFN-α administration relative to concomitant chemotherapy should be considered to avoid a decrease in CYP3A4 and 2B6 activity and thus to achieve better efficacy (Hassan et al., 1999). For example, interferon-α-2b inhibits CYP1A2, 2D6 and 2C19 and these findings pose new challenges for patients on these therapies with respect to PK interaction with concomitant drugs commonly used (Islam et al., 2002). Further studies are needed to measure the impact of IFN and new cytokine therapies coming on the market on CYP activities. Cytokines act on CYP in an isoform-specific manner, and it is likely that IFN or IL modulate different CYP while they have no impact on others. Moreover, it is crucial to understand whether the modulation of CYP activity is due to this kind of therapy, to the underlying disease which may be inflammatory, or to its resolution by these same therapies (reduction of inflammation caused by the disease).
The impact of–mabs therapies are summarized in Table 14. Monoclonal antibodies have a high degree of specificity against an antigen or an epitope (National Center for Biotechnology Information, 2012). In 2018, more than sixty therapeutic monoclonal antibodies were approved and used in the United States for their action against specific immune cells such as lymphocytes and cytokines or against specific enzymes, cell surface transporters or signaling molecules (National Center for Biotechnology Information, 2012). Consequently, a number of studies have examined the impact of monoclonal antibodies on CYP metabolic activity, assuming that these drugs, by reducing inflammation, return CYP metabolic activity to baseline (Ling et al., 2009; Schmitt et al., 2011; Wu and Fleming, 2011; Zhuang et al., 2015; Tran et al., 2016; Lee et al., 2017; Wen et al., 2020) (Table 14).
A return to baseline level after treatment of inflammation was not always observed (Wollmann et al., 2017; Davis et al., 2018). A lag was observed in some cases, such as basiliximab coadministration, which increased tacrolimus through concentration on day 3 but decreased on day 30 (Sifontis et al., 2002). Moreover, OKT3 (also known as muromonab, a CD3 receptor antibody) treatment transiently increased CyA through concentration, and authors suggested that OKT3 inhibits CYP3A4 metabolic activity by inducing transient cytokine release (Vasquez and Pollak, 1997). No changes were observed in drugs PK parameters before and after monoclonal antibodies administration, possibly because CYP metabolic activity was similar in psoriasis disease and in healthy volunteers (Bruin et al., 2019; Khatri et al., 2019). However, these therapies are used for a variety of diseases, with different levels of proinflammatory markers. In addition, a recently published study assessed the impact of clazakizumab, an anti-IL-6 antibody, in kidney transplant recipients with antibody-mediated rejection (ABMR) on CYP3A and CYP2C19 activity by pantoprazole and on tacrolimus and CyA concentrations (Mühlbacher et al., 2021). In contrast to earlier observations, prolonged blockade of IL-6 did not enhance CYP metabolism (Mühlbacher et al., 2021). This could be because the included patients did not have systemic inflammation before initiation of clazakizumab, with IL-6 and CRP levels in the normal range (Mühlbacher et al., 2021). Thus, clazakizumab did not increase CYP metabolism because the included patients had unaltered CYP expression, as ABMR may be different from other disease states, such as infection or autoimmune disease, where systemic inflammation is present (Mühlbacher et al., 2021).
Discussion and Perspectives
Our systematic review identified 218 publications that evaluated the impact of inflammation on CYP activities which we divided into 17 sources of inflammation. Indeed, current literature suggests that cytokine signalling pathways differ according to the trigger of inflammation, leading to heterogeneous effects on CYP activity, with different magnitude, potency and time-course (de Jong et al., 2020; Stanke-Labesque et al., 2020). This analysis allowed us to identify areas where the literature is abundant, such as infections like pulmonary infection, hepatitis or HIV and for some therapeutic agents like immunosuppressants or clozapine, and others where further research is needed, such as for auto-immune diseases, and other specific diseases such as diabetes or the anti-inflammation treatments.
Our analysis also identified that studies should be more specifically conducted to assess whether resolution of inflammatory episodes allows a return to baseline of CYP activities. Indeed, inflammatory diseases are chronic, but with a possibility of remission, and acute inflammatory events can punctuate life (infection, surgery, cancer…). A better understanding of the mechanisms of modulation and return to the initial state would make it possible to anticipate changes in the PK of concomitant treatments at different phases of the disease or of the patient’s life. This could be done through the impact of anti-inflammatory treatments as well as monoclonal antibody therapies. These therapies are relatively new and much remains to be discovered, but they are highly targeted, and the impact of these different molecules could be isoform specific.
Our literature review highlighted the different effect of inflammation according to the CYP considered. Several studies have investigated the impact of infection on drugs of the nervous systems, mainly CYP2D6 substrates without always showing a significant impact. It now appears that CYP2D6 activity is not modulated by inflammation and this is confirmed in chronic hepatitis C patients where downregulation is linked to the presence of liver kidney microsomal type 1 (LKM-1) antibodies (Girardin et al., 2012). LKM-1 antibodies are often produced during chronic HCV infection and appear to be proportional to liver disease severity (Girardin et al., 2012). Moreover, it is well-known that CYP2D6 has an important inter- and intra-individual variability, in accordance with the available literature (Chládek et al., 1999). All sources of inflammation combined, the most studied CYP was CYP3A, which is in fact the CYP that metabolizes nearly 50% of the drugs on the market. Patients with inflammation/infection are, however, prone to receiving multiple drugs, and the impact on other CYPs should be carefully evaluated, in particular in critically ill patients or patients at different stages of HIV, where data is scarce. Studies should also be careful to exclude the impact of co-medications (CYP inhibitor and inducer) as a confounding factor.
In organ diseases, current studies in liver diseases have not been able to determine whether CYP downregulation is caused by a decrease of CYP content or not, and in renal diseases it was not possible to identify whether the modulation of CYP activity was rather due to elimination issues (Farrell et al., 1979; Yang et al., 2003). Therefore, it is challenging to study inflammation as an independent factor in PK variability and not as a consequences of organ damage.
Our literature review also found that inflammation is a complex process, which is expressed differently depending on the disease and conditions and therefore, extrapolation between different types of inflammation should be avoided. Indeed, the hepatic expression of CYP2C19 could for example be regulated by other tumor-associated inflammatory factors than those regulating CYP3A (Burns et al., 2014). Moreover, different levels of inflammation led to different magnitudes of voriconazole through concentration increases for instance in association with CRP levels (van Wanrooy et al., 2014; Bolcato et al., 2021). In most studies, significant changes in CYP activities occurred in the presence of severe inflammation, characterized by elevated levels of inflammatory markers or a severe disease state, such as AIDS, advanced cancer or polytrauma patients (Gatti et al., 1993; Lee et al., 1993; Farrell et al., 1979; Grieco et al., 1998; Bauer et al., 1994; Harbrecht et al., 2005; Charles et al., 2006; Alexandre et al., 2007; Helsby et al., 2008; Abou Farha et al., 2012; ten Bokum et al., 2015; Hefner et al., 2015; Yasu et al., 2017; Gautier-Veyret et al., 2019). A minority of studies have evaluated the impact of inflammation on drugs PK and metabolism as an independent factor of variability, as only a few have included inflammation factors as covariates, such as biomarkers of renal or liver function (Stanke-Labesque et al., 2020).
Additionally, inflammation may have a different impact on CYPs activities depending on their baseline activity and on genotypic and environmental factors, such has concomitant treatments. Indeed, inflammation further increased the perampanel concentration/dose (C/D) ratio in patients not treated with drug inducers (Yamamoto et al., 2018). Voriconazole is also metabolized by highly polymorphic CYPs and inflammatory marker levels have a differential impact on voriconazole trough concentration whether patients are extensive, intermediate or ultra-rapid metabolized for CYP2C19 (Veringa et al., 2017). Moreover, a recent meta-analysis showed that voriconazole trough concentrations were independently influenced by both CYP2C19 and CYP3A4 genotype, considered individually or by a combined genetic score, in addition to CRP levels (Bolcato et al., 2021). In contrast, another cohort study showed that voriconazole overdoses were significantly associated with elevated CRP levels (>96 mg/L) but that CYP2C19 and CYP3A4 genotype, considered alone or combined in a genetic score, were not significantly different between overdose and non-overdose patients (Gautier-Veyret et al., 2019). Therefore, inflammation and pharmacogenomics may mutually minimize their reciprocal influence on CYP phenotype. Indeed, genotype did not predict correctly the phenotype in patients with inflammatory disease and the effect of inflammation was not as important as expected in CYP variants carriers (Helsby et al., 2008; Goktaş et al., 2015; Burns et al., 2014; O’Neil et al., 2000; Williams et al., 2000; ). Consequently, inflammation could induce dynamic phenoconversion, characterized by dynamic phenotype-genotype mismatch, and studies examining the impact of inflammation on CYPs should assess CYP genotypes and phenotypes as covariates. It should however be pointed out that most of the included studies did not take into account routine treatment given to treat the diseases themselves.
Predictive models based on known interactions between molecular, environmental and lifestyle data by computational algorithm are increasingly developed to support the decision to individualize treatment (Iriart, 2019). Simulation of the concentration-time profiles of a drug and its metabolite(s) and concomitant estimation of PK parameters using dynamic physiologically based pharmacokinetic (PBPK) models allow prediction of plasma concentration curves (Sager et al., 2015). There are increasing developments in regulatory guidances (Sager et al., 2015). Inflammatory disease is an example of a special population and numerous PBPK models have been developed and validated to predict IL-6 mediated drug-disease (Machavaram et al., 2013; Xu et al., 2015; Jiang et al., 2016; Radke et al., 2017; Xu et al., 2018; Machavaram et al., 2019). While IL-6 appears to be the key element in modulating CYP activities during inflammation, a recent study developed a model that predicted the impact of systemic CRP levels on CYP3A4 and CYP2C19 activities (Simon et al., 2021). Optimal drug use leads to takes into account the contribution of covariates to predict the dose needed to achieve a target concentration and thus reduce the inter- and intra-individual variability in drug response (Darwich et al., 2021).
This review focuses on CYP regulation, but other mechanisms, such as enzymes and transporters, involved in drug absorption, distribution, metabolism and elimination may be involved in changes in drugs PK during inflammatory states, although they are less studied. Studies described changes in plasma protein binding and renal excretion during inflammation that could affect CYP substrates metabolism (Gorski et al., 2000; Hefner et al., 2015; Helland et al., 2018). Plasma protein binding may influence total clearance for low-extraction drugs but not unbound clearance and may or may not influence half-life, depending on clearance and volume of distribution (Boffito et al., 2021). The unbound concentration and not the total concentration must be considered when assessing drug exposure to a highly protein-bound drug, otherwise there is a risk of misinterpretation of lopinavir overexposure (Boffito et al., 2021; Stanke-Labesque et al., 2021). For example, by taking into account plasma protein concentration, the authors concluded that CyA biotransformation by CYP3A may be downregulated by diabetes (Akhlaghi et al., 2012). Decreased albumin concentration may increase the unbound concentration in diabetics, which should theoretically increase CyA metabolic clearance (Akhlaghi et al., 2012). But the lower production of almost all metabolites has shown that the correct hypothesis is rather a reduced CYP activity (Akhlaghi et al., 2012). In fact, CyA metabolites that involved amino acid 1 showed significantly lower dose-normalized AUC values in diabetic patients compared with nondiabetics suggesting that CYP3A4 metabolic activity was not decreased (Mendonza et al., 2008). Its dose-adjusted metabolite-parent concentration ratio was decreased in the diabetic groups, but no difference was found concerning doses and trough levels of CyA in a retrospective study (Wadhawan et al., 2000; Akhlaghi et al., 2012).
Phase 2 drug metabolic enzymes appear to be affected in a cytokine-specific manner, as infection resulted in a significant downregulation of several genes encoding hepatic uridine 5′-diphospho-glucuronosyltransferases (UGT) (Stanke-Labesque et al., 2020). Pregnane X receptor (PXR) and constitutive androstane receptor (CAR), two nuclear receptors, are also cytokine dependent and mediate the expression of glutathione S-transferases (GST), UGTs and sulfo-transferases (SULT) in humans (Wu and Lin, 2019). However, unlike voriconazole, posaconazole’s PK did not appear to be influenced by inflammation. This could be explained by a metabolism by phase 2 enzymes mainly (Märtson et al., 2019). Literature reviews on physiological changes related to drug PK and PD during inflammation may be useful to determine what investigations are needed to complement the data in the literature, such as the impact of inflammation on P-gp and other drug transporters, as one study showed that an increase in bioavailability due to downregulation of P-gp could not be ruled out (Sanaee et al., 2011).
Moreover, hepatic transporters that belong to ATP-binding cassette (ABC) and solute carrier (SLC) transporters have been shown to be significantly reduced during inflammatory states in animal and in-vitro studies (Stanke-Labesque et al., 2020). For instance, animals studies have shown that mRNA levels of MRP, OATP or BSEP were decreased in mice during inflammation (Wu and Lin, 2019). NF-κB, a transcription factors involved in the mechanism of action of cytokines on metabolizing enzyme gene expression, is also known to regulate the expression of numerous ABC and SLC transporters, including ABCB1 in humans and MDR1, MRP, BCRP, OATP, NTCP in rats and mice (Wu and Lin, 2019).
Given all of the above, it should be acknowledged that our literature search has some limitations. First, the completeness of the search cannot be guaranteed as we only searched one database and only published articles. Second, there is inevitably heterogeneity between the studies selected due to the different methodologies employed and low comparability between the studies identified. In addition, the diversity of the sources of inflammation studied and assessment of the clinical impact severity limits the robustness and generalizability of the results. Interpretations should therefore be addressed with particular caution.
Conclusion
This systematic literature review shows that inflammation is a major contributing factor to CYP metabolic activity variations. The proportion of the drug cleared by CYP metabolism, the patient’s genotype and concomitant medications should also be taken into account.
Compelling evidence suggests that inflammation has a differential impact on the various CYP isoforms with a different magnitude. CYP3A and CYP2C19 are downregulated and inflammation has no impact on CYP2D6 activity. Regarding other main CYPs, the impact remains unclear and requires further investigation. Moreover, the effect of inflammation depends on its severity and the inflammatory markers released, even if this remains debated. Indeed, the origin of the inflammation may differ as well as the inflammatory mediators involved, possibly leading to different impact on CYP activities. The reason why some CYP metabolic activities were modulated in some diseases and not in others may be partly explained by this heterogeneity in inflammatory markers.
Nonetheless, some results are still debated such as the impact of vaccination and infection, and further investigations are required to well characterize the impact of inflammation on CYP activity.
CYP is a major source of interindividual variability, and it appears crucial to be able to predict their activity to individualize drug dosing and take into account the patient’s underlying pathophysiological conditions and the PK characteristics of the drug concerned. Measurement of inflammation induced CYP phenoconversion and the development of endogenous markers of CYP metabolism should enable the measurement of CYP activity variation due to disease progression and could have implications for personalized medicine and provide new opportunities.
To conclude, inflammatory conditions in patients are a major factor to be considered to predict variability in drug response and avoid efficacy or safety issue in clinical practice.
Data Availability Statement
The original contributions presented in the study are included in the article/Supplementary Material, further inquiries can be directed to the corresponding author.
Author Contributions
CL participated in the manuscript conceptualization, experimental design, writing and data analysis. CFS, JAD and VR participated in the manuscript conceptualization, supervision, overall manuscript review and English review.
Conflict of Interest
The authors declare that the research was conducted in the absence of any commercial or financial relationships that could be construed as a potential conflict of interest.
Publisher’s Note
All claims expressed in this article are solely those of the authors and do not necessarily represent those of their affiliated organizations, or those of the publisher, the editors and the reviewers. Any product that may be evaluated in this article, or claim that may be made by its manufacturer, is not guaranteed or endorsed by the publisher.
References
Abou Farha, K., van Vliet, A., Knegtering, H., and Bruggeman, R. (2012). The Value of Desmethylclozapine and Serum CRP in Clozapine Toxicity: A Case Report. Case Rep. Psychiatry 2012, 592784. doi:10.1155/2012/592784
Adachi, Y., Yokoyama, Y., Nanno, T., and Yamamoto, T. (1995). Potentiation of Warfarin by Interferon. BMJ 311 (7000), 292. doi:10.1136/bmj.311.7000.292a
Adithan, C., Danda, D., Swaminathan, R. P., Indhiresan, J., Shashindran, C. H., Bapna, J. S., et al. (1988). Effect of Diabetes Mellitus on Salivary Paracetamol Elimination. Clin. Exp. Pharmacol. Physiol. 15 (6), 465–471. doi:10.1111/j.1440-1681.1988.tb01102.x
Akhlaghi, F., Dostalek, M., Falck, P., Mendonza, A. E., Amundsen, R., Gohh, R. Y., et al. (2012). The Concentration of Cyclosporine Metabolites Is Significantly Lower in Kidney Transplant Recipients with Diabetes Mellitus. Ther. Drug Monit. 34 (1), 38–45. doi:10.1097/FTD.0b013e318241ac71
Alexandre, J., Rey, E., Girre, V., Grabar, S., Tran, A., Montheil, V., et al. (2007). Relationship between Cytochrome 3A Activity, Inflammatory Status and the Risk of Docetaxel-Induced Febrile Neutropenia: a Prospective Study. Ann. Oncol. 18 (1), 168–172. doi:10.1093/annonc/mdl321
Baer, A. N., McAllister, C. B., Wilkinson, G. R., Woosley, R. L., and Pincus, T. (1986). Altered Distribution of Debrisoquine Oxidation Phenotypes in Patients with Systemic Lupus Erythematosus. Arthritis Rheum. 29 (7), 843–850. doi:10.1002/art.1780290705
Baigrie, R. J., Lamont, P. M., Kwiatkowski, D., Dallman, M. J., and Morris, P. J. (1992). Systemic Cytokine Response after Major Surgery. Br. J. Surg. 79 (8), 757–760. doi:10.1002/bjs.1800790813
Bauer, L. A., O'Sullivan, T., Reiss, W. G., Horn, J. R., Opheim, K., Strandness, D. E., et al. (1994). Liver Blood Flow, Antipyrine Clearance, and Antipyrine Metabolite Formation Clearance in Patients with Chronic Active Hepatitis and Alcoholic Cirrhosis. Br. J. Clin. Pharmacol. 37 (4), 375–381. doi:10.1111/j.1365-2125.1994.tb04292.x
Bechtel, Y. C., Joanne, C., Grandmottet, M., and Bechtel, P. R. (1988). The Influence of Insulin-dependent Diabetes on the Metabolism of Caffeine and the Expression of the Debrisoquin Oxidation Phenotype. Clin. Pharmacol. Ther. 44 (4), 408–417. doi:10.1038/clpt.1988.173
Becquemont, L., Chazouilleres, O., Serfaty, L., Poirier, J. M., Broly, F., Jaillon, P., et al. (2002). Effect of Interferon Alpha-Ribavirin Bitherapy on Cytochrome P450 1A2 and 2D6 and N-Acetyltransferase-2 Activities in Patients with Chronic Active Hepatitis C. Clin. Pharmacol. Ther. 71 (6), 488–495. doi:10.1067/mcp.2002.124468
Bergin, P. F., Doppelt, J. D., Kephart, C. J., Benke, M. T., Graeter, J. H., Holmes, A. S., et al. (2011). Comparison of Minimally Invasive Direct Anterior versus Posterior Total Hip Arthroplasty Based on Inflammation and Muscle Damage Markers. J. Bone Jt. Surg Am 93 (15), 1392–1398. doi:10.2106/JBJS.J.00557
Bernlochner, I., Steinhubl, S., Braun, S., Morath, T., Jaitner, J., Stegherr, J., et al. (2010). Association between Inflammatory Biomarkers and Platelet Aggregation in Patients under Chronic Clopidogrel Treatment. Thromb. Haemost. 104 (6), 1193–1200. doi:10.1160/TH10-05-0266
Bilbao-Meseguer, I., Rodríguez-Gascón, A., Barrasa, H., Isla, A., and Solinís, M. Á. (2018). Augmented Renal Clearance in Critically Ill Patients: A Systematic Review. Clin. Pharmacokinet. 57 (9), 1107–1121. doi:10.1007/s40262-018-0636-7
Blumenkopf, B., and Lockhart, W. S. (1983). Herpes Zoster Infection and Use of Oral Anticoagulants. A Potentially Dangerous Association. JAMA 250 (7), 936–937. doi:10.1001/jama.1983.03340070042025
Boffito, M., Back, D. J., Flexner, C., Sjö, P., Blaschke, T. F., Horby, P. W., et al. (2021). Toward Consensus on Correct Interpretation of Protein Binding in Plasma and Other Biological Matrices for COVID-19 Therapeutic Development. Clin. Pharmacol. Ther. 110 (1), 64–68. doi:10.1002/cpt.2099
Bolcato, L., Khouri, C., Veringa, A., Alffenaar, J. W. C., Yamada, T., Naito, T., et al. (2021). Combined Impact of Inflammation and Pharmacogenomic Variants on Voriconazole Trough Concentrations: A Meta-Analysis of Individual Data. J. Clin. Med. 10 (10), 2089. doi:10.3390/jcm10102089
Branch, R. A., Herbert, C. M., and Read, A. E. (1973). Determinants of Serum Antipyrine Half-Lives in Patients with Liver Disease. Gut 14 (7), 569–573. doi:10.1136/gut.14.7.569
Breimer, D. D., Zilly, W., and Richter, E. (1975). Pharmacokinetics of Hexobarbital in Acute Hepatitis and after Apparent Recovery. Clin. Pharmacol. Ther. 18 (4), 433–440. doi:10.1002/cpt1975184433
Brennan, B. J., Xu, Z. X., and Grippo, J. F. (2013). Effect of Peginterferon Alfa-2a (40KD) on Cytochrome P450 Isoenzyme Activity. Br. J. Clin. Pharmacol. 75 (2), 497–506. doi:10.1111/j.1365-2125.2012.04373.x
Britton, L., and Ruben, F. L. (1982). Serum Theophylline Levels after Influenza Vaccination. Can. Med. Assoc. J. 126 (12), 1375.
Bruin, G., Hasselberg, A., Koroleva, I., Milojevic, J., Calonder, C., Soon, R., et al. (2019). Secukinumab Treatment Does Not Alter the Pharmacokinetics of the Cytochrome P450 3A4 Substrate Midazolam in Patients with Moderate to Severe Psoriasis. Clin. Pharmacol. Ther. 106 (6), 1380–1388. doi:10.1002/cpt.1558
Burns, K. E., Goldthorpe, M. A., Porteus, F., Browett, P., and Helsby, N. A. (2014). CYP2C19 Genotype-Phenotype Discordance in Patients with Multiple Myeloma Leads to an Acquired Loss of Drug-Metabolising Activity. Cancer Chemother. Pharmacol. 73 (3), 651–655. doi:10.1007/s00280-014-2409-9
Carroll, D. N., and Carroll, D. G. (2009). Fatal Intracranial Bleed Potentially Due to a Warfarin and Influenza Vaccine Interaction. Ann. Pharmacother. 43 (4), 754–760. doi:10.1345/aph.1L413
Casajuana, J., Iglesias, B., Fàbregas, M., Fina, F., Vallès, J. A., Aragonès, R., et al. (2008). Safety of Intramuscular Influenza Vaccine in Patients Receiving Oral Anticoagulation Therapy: a Single Blinded Multi-centre Randomized Controlled Clinical Trial. BMC Blood Disord. 8, 1. doi:10.1186/1471-2326-8-1
Charles, K. A., Rivory, L. P., Stockler, M. R., Beale, P., Beith, J., Boyer, M., et al. (2006). Predicting the Toxicity of Weekly Docetaxel in Advanced Cancer. Clin. Pharmacokinet. 45 (6), 611–622. doi:10.2165/00003088-200645060-00004
Chen, Y. L., Le Vraux, V., Leneveu, A., Dreyfus, F., Stheneur, A., Florentin, I., et al. (1994). Acute-phase Response, Interleukin-6, and Alteration of Cyclosporine Pharmacokinetics. Clin. Pharmacol. Ther. 55 (6), 649–660. doi:10.1038/clpt.1994.82
Chládek, J., Zimová, G., Martínková, J., and Tůma, I. (1999). Intra-individual Variability and Influence of Urine Collection Period on Dextromethorphan Metabolic Ratios in Healthy Subjects. Fundam. Clin. Pharmacol. 13 (4), 508–515. doi:10.1111/j.1472-8206.1999.tb00011.x
Clark, S. R., Warren, N. S., Kim, G., Jankowiak, D., Schubert, K. O., Kisely, S., et al. (2018). Elevated Clozapine Levels Associated with Infection: A Systematic Review. Schizophr Res. 192, 50–56. doi:10.1016/j.schres.2017.03.045
Cojutti, P. G., Londero, A., Della Siega, P., Givone, F., Fabris, M., Biasizzo, J., et al. (2020). Comparative Population Pharmacokinetics of Darunavir in SARS-CoV-2 Patients vs. HIV Patients: The Role of Interleukin-6. Clin. Pharmacokinet. 59 (10), 1251–1260. doi:10.1007/s40262-020-00933-8
Craig, P. I., Tapner, M., and Farrell, G. C. (1993). Interferon Suppresses Erythromycin Metabolism in Rats and Human Subjects. Hepatology 17 (2), 230–235. doi:10.1002/hep.1840170212
Cranshaw, T., and Harikumar, T. (2020). COVID-19 Infection May Cause Clozapine Intoxication: Case Report and Discussion. Schizophr Bull. 46 (4), 751. doi:10.1093/schbul/sbaa070
Daneshtalab, N., Lewanczuk, R. Z., Russell, A. S., and Jamali, F. (2006). Drug-disease Interactions: Losartan Effect Is Not Downregulated by Rheumatoid Arthritis. J. Clin. Pharmacol. 46 (11), 1344–1355. doi:10.1177/0091270006292163
Darakjian, L., Deodhar, M., Turgeon, J., and Michaud, V. (2021). Chronic Inflammatory Status Observed in Patients with Type 2 Diabetes Induces Modulation of Cytochrome P450 Expression and Activity. Int. J. Mol. Sci. 22 (9), 4967. doi:10.3390/ijms22094967
Darling, P., and Huthwaite, M. A. (2011). Infection-associated Clozapine Toxicity. Clin. Schizophr Relat. Psychoses 5 (3), 159–160. doi:10.3371/CSRP.5.3.7
Darwich, A. S., Polasek, T. M., Aronson, J. K., Ogungbenro, K., Wright, D. F. B., Achour, B., et al. (2021). Model-Informed Precision Dosing: Background, Requirements, Validation, Implementation, and Forward Trajectory of Individualizing Drug Therapy. Annu. Rev. Pharmacol. Toxicol. 61, 225–245. doi:10.1146/annurev-pharmtox-033020-113257
Davis, J. D., Bansal, A., Hassman, D., Akinlade, B., Li, M., Li, Z., et al. (2018). Evaluation of Potential Disease-Mediated Drug-Drug Interaction in Patients with Moderate-To-Severe Atopic Dermatitis Receiving Dupilumab. Clin. Pharmacol. Ther. 104 (6), 1146–1154. doi:10.1002/cpt.1058
de Jong, L. M., Jiskoot, W., Swen, J. J., and Manson, M. L. (2020). Distinct Effects of Inflammation on Cytochrome P450 Regulation and Drug Metabolism: Lessons from Experimental Models and a Potential Role for Pharmacogenetics. Genes (Basel) 11 (12). doi:10.3390/genes11121509
de Leon, J., and Diaz, F. J. (2003). Serious Respiratory Infections Can Increase Clozapine Levels and Contribute to Side Effects: a Case Report. Prog. Neuropsychopharmacol. Biol. Psychiatry 27 (6), 1059–1063. doi:10.1016/S0278-5846(03)00148-9
Dote, S., Sawai, M., Nozaki, A., Naruhashi, K., Kobayashi, Y., and Nakanishi, H. (2016). A Retrospective Analysis of Patient-specific Factors on Voriconazole Clearance. J. Pharm. Health Care Sci. 2, 10. doi:10.1186/s40780-016-0044-9
Dyer, J. R., Davis, T. M., Giele, C., Annus, T., Garcia-Webb, P., and Robson, J. (1994). The Pharmacokinetics and Pharmacodynamics of Quinine in the Diabetic and Non-diabetic Elderly. Br. J. Clin. Pharmacol. 38 (3), 205–212. doi:10.1111/j.1365-2125.1994.tb04343.x
Elin, R. J., Vesell, E. S., and Wolff, S. M. (1975). Effects of Etiocholanolone-Induced Fever on Plasma Antipyrine Half-Lives and Metabolic Clearance. Clin. Pharmacol. Ther. 17 (4), 447–457. doi:10.1002/cpt1975174447
Elkahwaji, J., Robin, M. A., Berson, A., Tinel, M., Lettéron, P., Labbe, G., et al. (1999). Decrease in Hepatic Cytochrome P450 after Interleukin-2 Immunotherapy. Biochem. Pharmacol. 57 (8), 951–954. doi:10.1016/s0006-2952(98)00372-4
Encalada Ventura, M. A., Span, L. F., van den Heuvel, E. R., Groothuis, G. M., and Alffenaar, J. W. (2015). Influence of Inflammation on Voriconazole Metabolism. Antimicrob. Agents Chemother. 59 (5), 2942–2943. doi:10.1128/AAC.04789-14
Espnes, K. A., Heimdal, K. O., and Spigset, O. (2012). A Puzzling Case of Increased Serum Clozapine Levels in a Patient with Inflammation and Infection. Ther. Drug Monit. 34 (5), 489–492. doi:10.1097/FTD.0b013e3182666c62
European medicines agency Prezista, INN-darunavir - prezista-epar-product-information_en.pdf. [Internet]. [cited 2019 Oct 1]. Available from: https://www.ema.europa.eu/en/documents/product-information/prezista-epar-product-information_en.pdf.
Farrell, G. C., Cooksley, W. G., and Powell, L. W. (1979). Drug Metabolism in Liver Disease: Activity of Hepatic Microsomal Metabolizing Enzymes. Clin. Pharmacol. Ther. 26 (4), 483–492. doi:10.1002/cpt1979264483
Farrow, P. R., and Nicholson, K. G. (1984). Lack of Effect of Influenza and Pneumococcal Vaccines on Anticoagulation by Warfarin. J. Infect. 9 (2), 157–160. doi:10.1016/s0163-4453(84)91156-3
Fischer, R. G., Booth, B. H., Mitchell, D. Q., and Kibbe, A. H. (1982). Influence of Trivalent Influenza Vaccine on Serum Theophylline Levels. Can. Med. Assoc. J. 126 (11), 1312–1313.
Food and Drug Administration In Vitro Metabolism- and Transporter- Mediated Drug-Drug Interaction Studies Guidance for Industry. download [Internet]. [cited 2020 Apr 23]. Available from: https://www.fda.gov/media/108130/download.
Frye, R. F., Schneider, V. M., Frye, C. S., and Feldman, A. M. (2002). Plasma Levels of TNF-Alpha and IL-6 Are Inversely Related to Cytochrome P450-dependent Drug Metabolism in Patients with Congestive Heart Failure. J. Card. Fail. 8 (5), 315–319. doi:10.1054/jcaf.2002.127773
Frye, R. F., Zgheib, N. K., Matzke, G. R., Chaves-Gnecco, D., Rabinovitz, M., Shaikh, O. S., et al. (2006). Liver Disease Selectively Modulates Cytochrome P450-Mmediated Metabolism. Clin. Pharmacol. Ther. 80 (3), 235–245. doi:10.1016/j.clpt.2006.05.006
Furlanut, M., Soardo, G., Donnini, D., Sechi, L., and Franceschi, L. (2010). Fluoxetine Disposition in Patients with Chronic Hepatitis C Treated with Interferon-α. Clin. Pharmacokinet. 49 (11), 767–772. doi:10.2165/11534720-000000000-00000
Gabay, C., and Kushner, I. (1999). Acute-phase Proteins and Other Systemic Responses to Inflammation. N. Engl. J. Med. 340 (6), 448–454. doi:10.1056/NEJM199902113400607
Gatti, G., Flaherty, J., Bubp, J., White, J., Borin, M., and Gambertoglio, J. (1993). Comparative Study of Bioavailabilities and Pharmacokinetics of Clindamycin in Healthy Volunteers and Patients with AIDS. Antimicrob. Agents Chemother. 37 (5), 1137–1143. doi:10.1128/aac.37.5.1137
Gautier-Veyret, E., Truffot, A., Bailly, S., Fonrose, X., Thiebaut-Bertrand, A., Tonini, J., et al. (2019). Inflammation Is a Potential Risk Factor of Voriconazole Overdose in Hematological Patients. Fundam. Clin. Pharmacol. 33 (2), 232–238. doi:10.1111/fcp.12422
Germolec, D. R., Shipkowski, K. A., Frawley, R. P., and Evans, E. (2018). Markers of Inflammation. Methods Mol. Biol. 1803, 57–79. doi:10.1007/978-1-4939-8549-4_5
Girardin, F., Daali, Y., Gex-Fabry, M., Rebsamen, M., Roux-Lombard, P., Cerny, A., et al. (2012). Liver Kidney Microsomal Type 1 Antibodies Reduce the CYP2D6 Activity in Patients with Chronic Hepatitis C Virus Infection. J. Viral Hepat. 19 (8), 568–573. doi:10.1111/j.1365-2893.2011.01578.x
Goktaş, M. T., Hatta, F., Karaca, O., Kalkisim, S., Kilic, L., Akdogan, A., et al. (2015). Lower CYP2C9 Activity in Turkish Patients with Behçet's Disease Compared to Healthy Subjects: a Down-Regulation Due to Inflammation. Eur. J. Clin. Pharmacol. 71 (10), 1223–1228. doi:10.1007/s00228-015-1899-7
Goldstein, R. S., Cheung, O. T., Seguin, R., Lobley, G., and Johnson, A. C. (1982). Decreased Elimination of Theophylline after Influenza Vaccination. Can. Med. Assoc. J. 126 (5), 470.
Gomolin, I. H. (1986). Lack of Effect of Influenza Vaccine on Warfarin Anticoagulation in the Elderly. CMAJ 135 (1), 39–41.
Gorski, J. C., Hall, S. D., Becker, P., Affrime, M. B., Cutler, D. L., and Haehner-Daniels, B. (2000). In Vivo effects of Interleukin-10 on Human Cytochrome P450 Activity. Clin. Pharmacol. Ther. 67 (1), 32–43. doi:10.1067/mcp.2000.103860
Gravel, S., Chiasson, J-L., Turgeon, J., Grangeon, A., and Michaud, V. (2019). Modulation of CYP450 Activities in Patients with Type 2 Diabetes. Clin. Pharmacol. Ther. 106 (6), 1280–1289. doi:10.1002/cpt.1496
Gray, J. D., Renton, K. W., and Hung, O. R. (1983). Depression of Theophylline Elimination Following BCG Vaccination. Br. J. Clin. Pharmacol. 16 (6), 735–737. doi:10.1111/j.1365-2125.1983.tb02253.x
Gregoire, M., Le Turnier, P., Gaborit, B. J., Veyrac, G., Lecomte, R., Boutoille, D., et al. (2020). Lopinavir Pharmacokinetics in COVID-19 Patients. J. Antimicrob. Chemother. 75 (9), 2702–2704. doi:10.1093/jac/dkaa195
Grieco, A., Castellano, R., Matera, A., Marcoccia, S., Di Rocco, P., Ragazzoni, E., et al. (1998). Antipyrine Clearance in Chronic and Neoplastic Liver Diseases: a Study of 518 Patients. J. Gastroenterol. Hepatol. 13 (5), 460–466. doi:10.1111/j.1440-1746.1998.tb00668.x
Grub, S., Bryson, H., Goggin, T., Lüdin, E., and Jorga, K. (2001). The Interaction of Saquinavir (Soft Gelatin Capsule) with Ketoconazole, Erythromycin and Rifampicin: Comparison of the Effect in Healthy Volunteers and in HIV-Infected Patients. Eur. J. Clin. Pharmacol. 57 (2), 115–121. doi:10.1007/s002280100277
Gupta, S. K., Sellers, E., Somoza, E., Angles, L., Kolz, K., and Cutler, D. L. (2007). The Effect of Multiple Doses of Peginterferon Alfa-2b on the Steady-State Pharmacokinetics of Methadone in Patients with Chronic Hepatitis C Undergoing Methadone Maintenance Therapy. J. Clin. Pharmacol. 47 (5), 604–612. doi:10.1177/0091270007299760
Haack, M. J., Bak, M. L., Beurskens, R., Maes, M., Stolk, L. M., and Delespaul, P. A. (2003). Toxic Rise of Clozapine Plasma Concentrations in Relation to Inflammation. Eur. Neuropsychopharmacol. 13 (5), 381–385. doi:10.1016/s0924-977x(03)00042-7
Haas, C. E., Kaufman, D. C., Jones, C. E., Burstein, A. H., and Reiss, W. (2003). Cytochrome P450 3A4 Activity after Surgical Stress. Crit. Care Med. 31 (5), 1338–1346. doi:10.1097/01.CCM.0000063040.24541.49
Hanada, K., Nakai, K., Tanaka, H., Suzuki, F., Kumada, H., Ohno, Y., et al. (2012). Effect of Nuclear Receptor Downregulation on Hepatic Expression of Cytochrome P450 and Transporters in Chronic Hepatitis C in Association with Fibrosis Development. Drug Metab. Pharmacokinet. 27 (3), 301–306. doi:10.2133/dmpk.dmpk-11-rg-077
Harbrecht, B. G., Frye, R. F., Zenati, M. S., Branch, R. A., and Peitzman, A. B. (2005). Cytochrome P-450 Activity Is Differentially Altered in Severely Injured Patients. Crit. Care Med. 33 (3), 541–546. doi:10.1097/01.ccm.0000155989.54344.e0
Harvey, R. D., and Morgan, E. T. (2014). Cancer, Inflammation, and Therapy: Effects on Cytochrome P450-Mediated Drug Metabolism and Implications for Novel Immunotherapeutic Agents. Clin. Pharmacol. Ther. 96 (4), 449–457. doi:10.1038/clpt.2014.143
Hassan, M., Nilsson, C., Olsson, H., Lundin, J., and Osterborg, A. (1999). The Influence of Interferon-Alpha on the Pharmacokinetics of Cyclophosphamide and its 4-hydroxy Metabolite in Patients with Multiple Myeloma. Eur. J. Haematol. 63 (3), 163–170. doi:10.1111/j.1600-0609.1999.tb01764.x
Hayney, M. S., and Muller, D. (2003). Effect of Influenza Immunization on CYP3A4 Activity In Vivo. J. Clin. Pharmacol. 43 (12), 1377–1381. doi:10.1177/0091270003260330
Hefner, G., Falter, T., Bruns, K., and Hiemke, C. (2015). Elevated Risperidone Serum Concentrations during Acute Inflammation, Two Cases. Int. J. Psychiatry Med. 50 (3), 335–344. doi:10.1177/0091217415610313
Hefner, G., Shams, M. E., Unterecker, S., Falter, T., and Hiemke, C. (2016). Inflammation and Psychotropic Drugs: the Relationship between C-Reactive Protein and Antipsychotic Drug Levels. Psychopharmacology (Berl) 233 (9), 1695–1705. doi:10.1007/s00213-015-3976-0
Helland, A., Habib, S., Ulvestad, L., and Spigset, O. (2018). Systemic Inflammation Complicates the Interpretation of Therapeutic Drug Monitoring of Risperidone. J. Clin. Psychopharmacol. 38 (3), 263–265. doi:10.1097/JCP.0000000000000873
Hellman, K., Roos, E., Osterlund, A., Wahlberg, A., Gustafsson, L. L., Bertilsson, L., et al. (2003). Interferon-beta Treatment in Patients with Multiple Sclerosis Does Not Alter CYP2C19 or CYP2D6 Activity. Br. J. Clin. Pharmacol. 56 (3), 337–340. doi:10.1046/j.0306-5251.2003.01859.x
Helsby, N. A., Lo, W. Y., Sharples, K., Riley, G., Murray, M., Spells, K., et al. (2008). CYP2C19 Pharmacogenetics in Advanced Cancer: Compromised Function Independent of Genotype. Br. J. Cancer 99 (8), 1251–1255. doi:10.1038/sj.bjc.6604699
Idle, J. R., Mahgoub, A., Lancaster, R., and Smith, R. L. (1978). Hypotensive Response to Debrisoquine and Hydroxylation Phenotype. Life Sci. 22 (11), 979–983. doi:10.1016/0024-3205(78)90363-6
Imai, H., Kotegawa, T., and Ohashi, K. (2011). Duration of Drug Interactions: Putative Time Courses after Mechanism-Based Inhibition or Induction of CYPs. Expert Rev. Clin. Pharmacol. 4 (4), 409–411. doi:10.1586/ecp.11.30
Iorio, A. M., Camilloni, B., Basileo, M., Guercini, F., Conti, S., Ferrante, F., et al. (2006). Influenza Vaccination in Patients on Long-Term Anticoagulant Therapy. Vaccine 24 (44–46), 6624–6628. doi:10.1016/j.vaccine.2006.05.036
Iriart, J. A. B. (2019). Precision Medicine/personalized Medicine: a Critical Analysis of Movements in the Transformation of Biomedicine in the Early 21st century. Cad Saude Publica 35 (3), e00153118. doi:10.1590/0102-311X00153118
Islam, M., Frye, R. F., Richards, T. J., Sbeitan, I., Donnelly, S. S., Glue, P., et al. (2002). Differential Effect of IFNalpha-2b on the Cytochrome P450 Enzyme System: a Potential Basis of IFN Toxicity and its Modulation by Other Drugs. Clin. Cancer Res. 8 (8), 2480–2487.
Israel, B. C., Blouin, R. A., McIntyre, W., and Shedlofsky, S. I. (1993). Effects of Interferon-Alpha Monotherapy on Hepatic Drug Metabolism in Cancer Patients. Br. J. Clin. Pharmacol. 36 (3), 229–235. doi:10.1111/j.1365-2125.1993.tb04222.x
Jackson, M. L., Nelson, J. C., Chen, R. T., Davis, R. L., and Jackson, L. A. (2007). Vaccines and Changes in Coagulation Parameters in Adults on Chronic Warfarin Therapy: a Cohort Study. Pharmacoepidemiol. Drug Saf. 16 (7), 790–796. doi:10.1002/pds.1386
Jecel, J., Michel, T. M., Gutknecht, L., Schmidt, D., Pfuhlmann, B., and Jabs, B. E. (2005). Toxic Clozapine Serum Levels during Acute Urinary Tract Infection: a Case Report. Eur. J. Clin. Pharmacol. 60 (12), 909–910. doi:10.1007/s00228-004-0867-4
Jetter, A., Fätkenheuer, G., Frank, D., Klaassen, T., Seeringer, A., Doroshyenko, O., et al. (2010). Do activities of Cytochrome P450 (CYP)3A, CYP2D6 and P-Glycoprotein Differ between Healthy Volunteers and HIV-Infected Patients. Antivir. Ther. 15 (7), 975–983. doi:10.3851/IMP1648
Jiang, X., Zhuang, Y., Xu, Z., Wang, W., and Zhou, H. (2016). Development of a Physiologically Based Pharmacokinetic Model to Predict Disease-Mediated Therapeutic Protein-Drug Interactions: Modulation of Multiple Cytochrome P450 Enzymes by Interleukin-6. AAPS J. 18 (3), 767–776. doi:10.1208/s12248-016-9890-5
Jones, A. E., Brown, K. C., Werner, R. E., Gotzkowsky, K., Gaedigk, A., Blake, M., et al. (2010). Variability in Drug Metabolizing Enzyme Activity in HIV-Infected Patients. Eur. J. Clin. Pharmacol. 66 (5), 475–485. doi:10.1007/s00228-009-0777-6
Jonkman, J. H., Nicholson, K. G., Farrow, P. R., Eckert, M., Grasmeijer, G., Oosterhuis, B., et al. (1989). Effects of Alpha-Interferon on Theophylline Pharmacokinetics and Metabolism. Br. J. Clin. Pharmacol. 27 (6), 795–802. doi:10.1111/j.1365-2125.1989.tb03442.x
Kacevska, M., Robertson, G. R., Clarke, S. J., and Liddle, C. (2008). Inflammation and CYP3A4-Mediated Drug Metabolism in Advanced Cancer: Impact and Implications for Chemotherapeutic Drug Dosing. Expert Opin. Drug Metab. Toxicol. 4 (2), 137–149. doi:10.1517/17425255.4.2.137
Kato, H. (2020). Computational Prediction of Cytochrome P450 Inhibition and Induction. Drug Metab. Pharmacokinet. 35 (1), 30–44. doi:10.1016/j.dmpk.2019.11.006
Kawaoka, T., Imamura, M., Morio, K., Nakamura, Y., Tsuge, M., Nelson Hayes, C., et al. (2016). Three Patients Treated with Daclatasvir and Asunaprevir for Recurrent Hepatitis C after Liver Transplantation: Case Report. Hepatol. Res. 46 (7), 707–712. doi:10.1111/hepr.12602
Khan, Z., and Khan, M. S. (2019). Rare Case of Theophylline Toxicity Due to Influenza A Infection in an Adult with Asthma. Am. J. Ther. 26 (4), e553–5. doi:10.1097/MJT.00000000000008177
Khatri, A., Cheng, L., Camez, A., Ignatenko, S., Pang, Y., and Othman, A. A. (2019). Lack of Effect of 12-Week Treatment with Risankizumab on the Pharmacokinetics of Cytochrome P450 Probe Substrates in Patients with Moderate to Severe Chronic Plaque Psoriasis. Clin. Pharmacokinet. 58 (6), 805–814. doi:10.1007/s40262-018-0730-x
Kim, R. B., and Wilkinson, G. R. (1996). CYP2E1 Activity Is Not Altered by Influenza Vaccination. Br. J. Clin. Pharmacol. 42 (4), 529–530. doi:10.1111/j.1365-2125.1996.tb00022.x
Klotz, U., Avant, G. R., Hoyumpa, A., Schenker, S., and Wilkinson, G. R. (1975). The Effects of Age and Liver Disease on the Disposition and Elimination of Diazepam in Adult Man. J. Clin. Invest. 55 (2), 347–359. doi:10.1172/JCI107938
Korrapati, M. R., Vestal, R. E., and Loi, C. M. (1995). Theophylline Metabolism in Healthy Nonsmokers and in Patients with Insulin-dependent Diabetes Mellitus. Clin. Pharmacol. Ther. 57 (4), 413–418. doi:10.1016/0009-9236(95)90210-4
Kramer, P., and McClain, C. J. (1981). Depression of Aminopyrine Metabolism by Influenza Vaccination. N. Engl. J. Med. 305 (21), 1262–1264. doi:10.1056/NEJM198111193052106
Kramer, P., Tsuru, M., Cook, C. E., McClain, C. J., and Holtzman, J. L. (1984). Effect of Influenza Vaccine on Warfarin Anticoagulation. Clin. Pharmacol. Ther. 35 (3), 416–418. doi:10.1038/clpt.1984.52
Kruger, P. S., Freir, N. M., Venkatesh, B., Robertson, T. A., Roberts, M. S., and Jones, M. (2009). A Preliminary Study of Atorvastatin Plasma Concentrations in Critically Ill Patients with Sepsis. Intensive Care Med. 35 (4), 717–721. doi:10.1007/s00134-008-1358-3
Kugelmas, M., Osgood, M. J., Trotter, J. F., Bak, T., Wachs, M., Forman, L., et al. (2003). Hepatitis C Virus Therapy, Hepatocyte Drug Metabolism, and Risk for Acute Cellular Rejection. Liver Transpl. 9 (11), 1159–1165. doi:10.1053/jlts.2003.50233
Kwak, Y. T., Yang, Y., and Koo, M. S. (2014). Clozapine-associated Asterixis: Case Report. J. Clin. Psychopharmacol. 34 (1), 165–167. doi:10.1097/JCP.0b013e3182a5959b
Lang, C. C., Brown, R. M., Kinirons, M. T., Deathridge, M. A., Guengerich, F. P., Kelleher, D., et al. (1996). Decreased Intestinal CYP3A in Celiac Disease: Reversal after Successful Gluten-free Diet: a Potential Source of Interindividual Variability in First-Pass Drug Metabolism. Clin. Pharmacol. Ther. 59 (1), 41–46. doi:10.1016/S0009-9236(96)90022-3
Latorre, A., Morales, E., Gonzalez, E., Herrero, J. C., Ortiz, M., Sierra, P., et al. (2002). Clinical Management of Renal Transplant Patients with Hepatitis C Virus Infection Treated with Cyclosporine or Tacrolimus. Transpl. Proc 34 (1), 63–64. doi:10.1016/s0041-1345(01)02678-1
Laybourn, C., Tønnesen, P., Loft, S., Sonne, J., and Døssing, M. (1986). Pulmonary Disease and Antipyrine Clearance. Clin. Pharmacol. Ther. 40 (4), 415–419. doi:10.1038/clpt.1986.199
Le Tiec, C., Barrail, A., Goujard, C., and Taburet, A. M. (2005). Clinical Pharmacokinetics and Summary of Efficacy and Tolerability of Atazanavir. Clin. Pharmacokinet. 44 (10), 1035–1050. doi:10.2165/00003088-200544100-00003
Lebwohl, B., Sanders, D. S., and Green, P. H. R. (2018). Coeliac Disease. Lancet 391 (10115), 70–81. doi:10.1016/S0140-6736(17)31796-8
Lee, B. L., Wong, D., Benowitz, N. L., and Sullam, P. M. (1993). Altered Patterns of Drug Metabolism in Patients with Acquired Immunodeficiency Syndrome. Clin. Pharmacol. Ther. 53 (5), 529–535. doi:10.1038/clpt.1993.66
Lee, E. B., Daskalakis, N., Xu, C., Paccaly, A., Miller, B., Fleischmann, R., et al. (2017). Disease-Drug Interaction of Sarilumab and Simvastatin in Patients with Rheumatoid Arthritis. Clin. Pharmacokinet. 56 (6), 607–615. doi:10.1007/s40262-016-0462-8
Lenoir, C., Daali, Y., Rollason, V., Curtin, F., Gloor, Y., Bosilkovska, M., et al. (2020). Impact of Acute Inflammation on Cytochromes P450 Activity Assessed by the Geneva Cocktail. Clin. Pharmacol. Ther. 109, 1668–1676. doi:10.1002/cpt.2146
Lenoir, C., Rodieux, F., Desmeules, J. A., Rollason, V., and Samer, C. F. (2021). Impact of Inflammation on Cytochromes P450 Activity in Pediatrics: A Systematic Review. Clin. Pharmacokinet. doi:10.1007/s40262-021-01064-4
Leung, J. G., Nelson, S., Takala, C. R., and Gören, J. L. (2014). Infection and Inflammation Leading to Clozapine Toxicity and Intensive Care: a Case Series. Ann. Pharmacother. 48 (6), 801–805. doi:10.1177/1060028014526701
Levine, M., and Jones, M. W. (1983). Toxic Reaction to Phenytoin Following a Viral Infection. Can. Med. Assoc. J. 128 (11), 1270–1271.
Ling, S., Lewanczuk, R. Z., Russell, A. S., Ihejirika, B., and Jamali, F. (2009). Influence of Controlled Rheumatoid Arthritis on the Action and Disposition of Verapamil: Focus on Infliximab. J. Clin. Pharmacol. 49 (3), 301–311. doi:10.1177/0091270008328099
Liptrott, N. J., and Owen, A. (2011). The Role of Cytokines in the Regulation of Drug Disposition: Extended Functional Pleiotropism. Expert Opin. Drug Metab. Toxicol. 7 (3), 341–352. doi:10.1517/17425255.2011.553600
Lucas, D., Farez, C., Bardou, L. G., Vaisse, J., Attali, J. R., and Valensi, P. (1998). Cytochrome P450 2E1 Activity in Diabetic and Obese Patients as Assessed by Chlorzoxazone Hydroxylation. Fundam. Clin. Pharmacol. 12 (5), 553–558. doi:10.1111/j.1472-8206.1998.tb00985.x
Luong, M. L., Al-Dabbagh, M., Groll, A. H., Racil, Z., Nannya, Y., Mitsani, D., et al. (2016). Utility of Voriconazole Therapeutic Drug Monitoring: a Meta-Analysis. J. Antimicrob. Chemother. 71 (7), 1786–1799. doi:10.1093/jac/dkw099
Lynch, T., and Price, A. (2007). The Effect of Cytochrome P450 Metabolism on Drug Response, Interactions, and Adverse Effects. Am. Fam. Physician 76 (3), 391–396.
MacCallum, P., Madhani, M., Mt-Isa, S., and Ashby, D. (2007). Lack of Effect of Influenza Immunisation on Anticoagulant Control in Patients on Long-Term Warfarin. Pharmacoepidemiol. Drug Saf. 16 (7), 786–789. doi:10.1002/pds.1347
Machavaram, K. K., Almond, L. M., Rostami-Hodjegan, A., Gardner, I., Jamei, M., Tay, S., et al. (2013). A Physiologically Based Pharmacokinetic Modeling Approach to Predict Disease-Drug Interactions: Suppression of CYP3A by IL-6. Clin. Pharmacol. Ther. 94 (2), 260–268. doi:10.1038/clpt.2013.79
Machavaram, K. K., Endo-Tsukude, C., Terao, K., Gill, K. L., Hatley, O. J., Gardner, I., et al. (2019). Simulating the Impact of Elevated Levels of Interleukin-6 on the Pharmacokinetics of Various CYP450 Substrates in Patients with Neuromyelitis Optica or Neuromyelitis Optica Spectrum Disorders in Different Ethnic Populations. AAPS J. 21 (3), 42. doi:10.1208/s12248-019-0309-y
Mafuru, M., Wu, S., He, S., Lu, X., Huang, J., and Jiang, H. (2019). The Influence of Proinflammatory Cytokines on Voriconazole Trough Concentration in Patients with Different Forms of Hematologic Disorders. J. Clin. Pharmacol. 59 (10), 1340–1350. doi:10.1002/jcph.1422
Marino, M. R., Langenbacher, K. M., Raymond, R. H., Ford, N. F., and Lasseter, K. C. (1998). Pharmacokinetics and Pharmacodynamics of Irbesartan in Patients with Hepatic Cirrhosis. J. Clin. Pharmacol. 38 (4), 347–356. doi:10.1002/j.1552-4604.1998.tb04434.x
Marques, M. P., Coelho, E. B., Dos Santos, N. A., Geleilete, T. J., and Lanchote, V. L. (2002). Dynamic and Kinetic Disposition of Nisoldipine Enantiomers in Hypertensive Patients Presenting with Type-2 Diabetes Mellitus. Eur. J. Clin. Pharmacol. 58 (9), 607–614. doi:10.1007/s00228-002-0528-4
Märtson, A. G., Veringa, A., Bakker, M., van den Heuvel, E. R., Touw, D. J., van der Werf, T. S., et al. (2019). Posaconazole Trough Concentrations Are Not Influenced by Inflammation: A Prospective Study. Int. J. Antimicrob. Agents 53 (3), 325–329. doi:10.1016/j.ijantimicag.2019.01.006
Marzolini, C., Stader, F., Stoeckle, M., Franzeck, F., Egli, A., Bassetti, S., et al. (2020). Effect of Systemic Inflammatory Response to SARS-CoV-2 on Lopinavir and Hydroxychloroquine Plasma Concentrations. Antimicrob. Agents Chemother. 64 (9), 64. doi:10.1128/AAC.01177-20
Matzke, G. R., Frye, R. F., Early, J. J., Straka, R. J., and Carson, S. W. (2000). Evaluation of the Influence of Diabetes Mellitus on Antipyrine Metabolism and CYP1A2 and CYP2D6 Activity. Pharmacotherapy 20 (2), 182–190. doi:10.1592/phco.20.3.182.34775
Mayo, P. R., Skeith, K., Russell, A. S., and Jamali, F. (2000). Decreased Dromotropic Response to Verapamil Despite Pronounced Increased Drug Concentration in Rheumatoid Arthritis. Br. J. Clin. Pharmacol. 50 (6), 605–613. doi:10.1046/j.1365-2125.2000.00314.x
McHorse, T. S., Wilkinson, G. R., Johnson, R. F., and Schenker, S. (1975). Effect of Acute Viral Hepatitis in Man on the Disposition and Elimination of Meperidine. Gastroenterology 68 (4 Pt 1), 775–780. doi:10.1016/s0016-5085(75)80289-7
Mendonza, A. E., Gohh, R. Y., and Akhlaghi, F. (2008). Blood and Plasma Pharmacokinetics of Ciclosporin in Diabetic Kidney Transplant Recipients. Clin. Pharmacokinet. 47 (11), 733–742. doi:10.2165/00003088-200847110-00004
Moher, D., Liberati, A., Tetzlaff, J., and Altman, D. G.PRISMA Group (2009). Preferred Reporting Items for Systematic Reviews and Meta-Analyses: the PRISMA Statement. BMJ 339, b2535. doi:10.1136/bmj.b2535
Molanaei, H., Qureshi, A. R., Heimbürger, O., Lindholm, B., Diczfalusy, U., Anderstam, B., et al. (2018). Inflammation Down-Regulates CYP3A4-Catalysed Drug Metabolism in Hemodialysis Patients. BMC Pharmacol. Toxicol. 19 (1), 33. doi:10.1186/s40360-018-0221-6
Molanaei, H., Stenvinkel, P., Qureshi, A. R., Carrero, J. J., Heimbürger, O., Lindholm, B., et al. (2012). Metabolism of Alprazolam (A Marker of CYP3A4) in Hemodialysis Patients with Persistent Inflammation. Eur. J. Clin. Pharmacol. 68 (5), 571–577. doi:10.1007/s00228-011-1163-8
Morcos, P. N., Moreira, S. A., Brennan, B. J., Blotner, S., Shulman, N. S., and Smith, P. F. (2013). Influence of Chronic Hepatitis C Infection on Cytochrome P450 3A4 Activity Using Midazolam as an In Vivo Probe Substrate. Eur. J. Clin. Pharmacol. 69 (10), 1777–1784. doi:10.1007/s00228-013-1525-5
Morgan, E. T. (2001). Regulation of Cytochrome P450 by Inflammatory Mediators: Why and How. Drug Metab. Dispos 29 (3), 207–212.
Morgan, E. T. (1997). Regulation of Cytochromes P450 during Inflammation and Infection. Drug Metab. Rev. 29 (4), 1129–1188. doi:10.3109/03602539709002246
Mostowik, M., Siniarski, A., Gołębiowska-Wiatrak, R., Nessler, J., and Gajos, G. (2015). Prolonged CRP Increase after Percutaneous Coronary Intervention Is Associated with High Thrombin Concentrations and Low Platelet' Response to Clopidogrel in Patients with Stable Angina. Adv. Clin. Exp. Med. 24 (6), 979–985. doi:10.17219/acem/46935
Mühlbacher, J., Schörgenhofer, C., Doberer, K., Dürr, M., Budde, K., Eskandary, F., et al. (2021). Anti‐interleukin‐6 Antibody Clazakizumab in Late Antibody‐mediated Kidney Transplant Rejection: Effect on Cytochrome P450 Drug Metabolism. Transpl. Int. 34 (8), 1542–1552. doi:10.1111/tri.13954
National Center for Biotechnology Information (2012). “Monoclonal Antibodies,” in LiverTox: Clinical and Research Information on Drug-Induced Liver Injury (Bethesda (MD): National Institute of Diabetes and Digestive and Kidney Diseases). [cited 2020 Apr 20]. Available from: http://www.ncbi.nlm.nih.gov/books/NBK548844/.
Niioka, T., Fujishima, N., Abumiya, M., Yamashita, T., Ubukawa, K., Nara, M., et al. (2017). Relationship between the CYP2C19 Phenotype Using the Voriconazole-To-Voriconazole N-Oxide Plasma Concentration Ratio and Demographic and Clinical Characteristics of Japanese Patients with Different CYP2C19 Genotypes. Ther. Drug Monit. 39 (5), 514–521. doi:10.1097/FTD.0000000000000441
Nolin, T. D. (2008). Altered Nonrenal Drug Clearance in ESRD. Curr. Opin. Nephrol. Hypertens. 17 (6), 555–559. doi:10.1097/MNH.0b013e3283136732
Nolin, T. D., Appiah, K., Kendrick, S. A., Le, P., McMonagle, E., and Himmelfarb, J. (2006). Hemodialysis Acutely Improves Hepatic CYP3A4 Metabolic Activity. J. Am. Soc. Nephrol. 17 (9), 2363–2367. doi:10.1681/ASN.2006060610
O'Neil, W. M., Gilfix, B. M., Markoglou, N., Di Girolamo, A., Tsoukas, C. M., and Wainer, I. W. (2000). Genotype and Phenotype of Cytochrome P450 2D6 in Human Immunodeficiency Virus-Positive Patients and Patients with Acquired Immunodeficiency Syndrome. Eur. J. Clin. Pharmacol. 56 (3), 231–240. doi:10.1007/s002280000116
Packageinserts pi_reyataz.pdf, [Internet]. [cited 2019 Oct 1]. Available from: https://packageinserts.bms.com/pi/pi_reyataz.pdf
Paliani, U., Filippucci, E., and Gresele, P. (2003). Significant Potentiation of Anticoagulation by Flu-Vaccine during the Season 2001-2002. Haematologica 88 (5), 599–600.
Patriarca, P. A., Kendal, A. P., Stricof, R. L., Weber, J. A., Meissner, M. K., and Dateno, B. (1983). Influenza Vaccination and Warfarin or Theophylline Toxicity in Nursing-home Residents. N. Engl. J. Med. 308 (26), 1601–1602. doi:10.1056/NEJM198306303082615
Pellegrino, P., Carnovale, C., Borsadoli, C., Danini, T., Speziali, A., Perrone, V., et al. (2013). Two Cases of Hallucination in Elderly Patients Due to a Probable Interaction between Flu Immunization and Tramadol. Eur. J. Clin. Pharmacol. 69 (8), 1615–1616. doi:10.1007/s00228-013-1517-5
Pfuhlmann, B., Hiemke, C., Unterecker, S., Burger, R., Schmidtke, A., Riederer, P., et al. (2009). Toxic Clozapine Serum Levels during Inflammatory Reactions. J. Clin. Psychopharmacol. 29 (4), 392–394. doi:10.1097/JCP.0b013e3181acd20b
Pirttiaho, H. I., Salmela, P. I., Sotaniemi, E. A., Pelkonen, R. O., Pitkänen, U., and Luoma, P. V. (1984). Drug Metabolism in Diabetic Subjects with Fatty Livers. Br. J. Clin. Pharmacol. 18 (6), 895–899. doi:10.1111/j.1365-2125.1984.tb02561.x
Piscitelli, S. C., Vogel, S., Figg, W. D., Raje, S., Forrest, A., Metcalf, J. A., et al. (1998). Alteration in Indinavir Clearance during Interleukin-2 Infusions in Patients Infected with the Human Immunodeficiency Virus. Pharmacotherapy 18 (6), 1212–1216.
Plotkin, E., Bernheim, J., Ben-Chetrit, S., Mor, A., and Korzets, Z. (2000). Influenza Vaccine-Aa Possible Trigger of Rhabdomyolysis Induced Acute Renal Failure Due to the Combined Use of Cerivastatin and Bezafibrate. Nephrol. Dial. Transpl. 15 (5), 740–741. doi:10.1093/ndt/15.5.740
Poli, D., Chiarugi, L., Capanni, M., Antonucci, E., Abbate, R., Gensini, G. F., et al. (2002). Need of More Frequent International Normalized Ratio Monitoring in Elderly Patients on Long-Term Anticoagulant Therapy after Influenza Vaccination. Blood Coagul. Fibrinolysis 13 (4), 297–300. doi:10.1097/00001721-200206000-00004
Preston, R. A., Chung, M., Gaffney, M., Alonso, A., Baltodano, N. M., and Epstein, M. (2001). Comparative Pharmacokinetics and Pharmacodynamics of Amlodipine in Hypertensive Patients with and without Type II Diabetes Mellitus. J. Clin. Pharmacol. 41 (11), 1215–1224. doi:10.1177/00912700122012760
Raaska, K., Raitasuo, V., Arstila, M., and Neuvonen, P. J. (2002). Bacterial Pneumonia Can Increase Serum Concentration of Clozapine. Eur. J. Clin. Pharmacol. 58 (5), 321–322. doi:10.1007/s00228-002-0486-x
Radke, C., Horn, D., Lanckohr, C., Ellger, B., Meyer, M., Eissing, T., et al. (2017). Development of a Physiologically Based Pharmacokinetic Modelling Approach to Predict the Pharmacokinetics of Vancomycin in Critically Ill Septic Patients. Clin. Pharmacokinet. 56 (7), 759–779. doi:10.1007/s40262-016-0475-3
Raj, G., Kumar, R., and McKinney, W. P. (1995). Safety of Intramuscular Influenza Immunization Among Patients Receiving Long-Term Warfarin Anticoagulation Therapy. Arch. Intern. Med. 155 (14), 1529–1531. doi:10.1001/archinte.1995.00430140104011
Raschzok, N., Schott, E., Reutzel-Selke, A., Damrah, I., Gül-Klein, S., Strücker, B., et al. (2016). The Impact of Directly Acting Antivirals on the Enzymatic Liver Function of Liver Transplant Recipients with Recurrent Hepatitis C. Transpl. Infect. Dis. 18 (6), 896–903. doi:10.1111/tid.12606
Renton, K. W., Gray, J. D., and Hall, R. I. (1980). Decreased Elimination of Theophylline after Influenza Vaccination. Can. Med. Assoc. J. 123 (4), 288–290.
Renton, K. W. (2005). Regulation of Drug Metabolism and Disposition during Inflammation and Infection. Expert Opin. Drug Metab. Toxicol. 1 (4), 629–640. doi:10.1517/17425255.1.4.629
Rivory, L. P., Slaviero, K. A., and Clarke, S. J. (2002). Hepatic Cytochrome P450 3A Drug Metabolism Is Reduced in Cancer Patients Who Have an Acute-phase Response. Br. J. Cancer 87 (3), 277–280. doi:10.1038/sj.bjc.6600448
Ruan, C. J., Zang, Y. N., Cheng, Y. H., Wang, C. Y., and de Leon, J. (2020). Around 3% of 1,300 Levels Were Elevated during Infections in a Retrospective Review of 131 Beijing Hospital In-Patients with More Than 24,000 Days of Clozapine Treatment. Psychother Psychosom 89 (4), 255–257. doi:10.1159/000506355
Ruan, C. J., Zhang, X. L., Guo, W., Li, W. B., Zhuang, H. Y., Li, Y. Q., et al. (2018). Two Cases of High Serum Clozapine Concentrations Occurring during Inflammation in Chinese Patients. Int. J. Psychiatry Med. 53 (4), 292–305. doi:10.1177/0091217417749799
Ruan, C. J., Zhen, X. Y., Ge, X. L., Wang, C. Y., Guo, W., Tang, Y. L., et al. (2017). Pneumonia Can Cause Clozapine Intoxication: A Case Report. Psychosomatics 58 (6), 652–656. doi:10.1016/j.psym.2017.05.003
Ruminy, P., Gangneux, C., Claeyssens, S., Scotte, M., Daveau, M., and Salier, J. P. (2001). Gene Transcription in Hepatocytes during the Acute Phase of a Systemic Inflammation: from Transcription Factors to Target Genes. Inflamm. Res. 50 (8), 383–390. doi:10.1007/PL00000260
Saab, S., Rheem, J., Jimenez, M., Bau, S., Choi, G., Durazo, F., et al. (2016). Curing Hepatitis C in Liver Transplant Recipients Is Associated with Changes in Immunosuppressant Use. J. Clin. Transl Hepatol. 4 (1), 32–38. doi:10.14218/JCTH.2016.00001
Sager, J. E., Yu, J., Ragueneau-Majlessi, I., and Isoherranen, N. (2015). Physiologically Based Pharmacokinetic (PBPK) Modeling and Simulation Approaches: A Systematic Review of Published Models, Applications, and Model Verification. Drug Metab. Dispos 43 (11), 1823–1837. doi:10.1124/dmd.115.065920
Salmela, P. I., Sotaniemi, E. A., and Pelkonen, R. O. (1980). The Evaluation of the Drug-Metabolizing Capacity in Patients with Diabetes Mellitus. Diabetes 29 (10), 788–794. doi:10.2337/diacare.20.10.788
Samer, C. F., Lorenzini, K. I., Rollason, V., Daali, Y., and Desmeules, J. A. (2013). Applications of CYP450 Testing in the Clinical Setting. Mol. Diagn. Ther. 17 (3), 165–184. doi:10.1007/s40291-013-0028-5
Sanaee, F., Clements, J. D., Waugh, A. W., Fedorak, R. N., Lewanczuk, R., and Jamali, F. (2011). Drug-disease Interaction: Crohn's Disease Elevates Verapamil Plasma Concentrations but Reduces Response to the Drug Proportional to Disease Activity. Br. J. Clin. Pharmacol. 72 (5), 787–797. doi:10.1111/j.1365-2125.2011.04019.x
Satarug, S., Lang, M. A., Yongvanit, P., Sithithaworn, P., Mairiang, E., Mairiang, P., et al. (1996). Induction of Cytochrome P450 2A6 Expression in Humans by the Carcinogenic Parasite Infection, Opisthorchiasis Viverrini. Cancer Epidemiol. Biomarkers Prev. 5 (10), 795–800.
Schellens, J. H., Janssens, A. R., van der Wart, J. H., van der Velde, E. A., and Breimer, D. D. (1989). Relationship between the Metabolism of Antipyrine, Hexobarbital and Theophylline in Patients with Liver Disease as Assessed by a 'cocktail' Approach. Eur. J. Clin. Invest. 19 (5), 472–479. doi:10.1111/j.1365-2362.1989.tb00262.x
Schmitt, C., Kuhn, B., Zhang, X., Kivitz, A. J., and Grange, S. (2011). Disease-drug-drug Interaction Involving Tocilizumab and Simvastatin in Patients with Rheumatoid Arthritis. Clin. Pharmacol. Ther. 89 (5), 735–740. doi:10.1038/clpt.2011.35
Schneider, R. E., Babb, J., Bishop, H., Mitchard, M., and Hoare, A. M. (1976). Plasma Levels of Propranolol in Treated Patients with Coeliac Disease and Patients with Crohn's Disease. Br. Med. J. 2 (6039), 794–795. doi:10.1136/bmj.2.6039.794
Schoergenhofer, C., Hobl, E. L., Schellongowski, P., Heinz, G., Speidl, W. S., Siller-Matula, J. M., et al. (2018). Clopidogrel in Critically Ill Patients. Clin. Pharmacol. Ther. 103 (2), 217–223. doi:10.1002/cpt.878
Schoergenhofer, C., Jilma, B., Stimpfl, T., Karolyi, M., and Zoufaly, A. (2020). Pharmacokinetics of Lopinavir and Ritonavir in Patients Hospitalized with Coronavirus Disease 2019 (COVID-19). Ann. Intern. Med. 173 (8), 670–672. doi:10.7326/M20-1550
Schulz, J., Kluwe, F., Mikus, G., Michelet, R., and Kloft, C. (2019). Novel Insights into the Complex Pharmacokinetics of Voriconazole: a Review of its Metabolism. Drug Metab. Rev. 51 (3), 247–265. doi:10.1080/03602532.2019.1632888
Serratrice, J., Durand, J. M., and Morange, S. (1998). Interferon-alpha 2b Interaction with Acenocoumarol. Am. J. Hematol. 57 (1), 89. doi:10.1002/(sici)1096-8652(199801)57:1<89:aid-ajh18>3.0.co;2-4
Shelly, M. P., Mendel, L., and Park, G. R. (1987). Failure of Critically Ill Patients to Metabolise Midazolam. Anaesthesia 42 (6), 619–626. doi:10.1111/j.1365-2044.1987.tb03086.x
Sifontis, N. M., Benedetti, E., and Vasquez, E. M. (2002). Clinically Significant Drug Interaction between Basiliximab and Tacrolimus in Renal Transplant Recipients. Transpl. Proc 34 (5), 1730–1732. doi:10.1016/s0041-1345(02)03000-2
Simon, F., Gautier-Veyret, E., Truffot, A., Chenel, M., Payen, L., Stanke-Labesque, F., et al. (2021). Modeling Approach to Predict the Impact of Inflammation on the Pharmacokinetics of CYP2C19 and CYP3A4 Substrates. Pharm. Res. 38 (3), 415–428. doi:10.1007/s11095-021-03019-7
Smolders, E. J., Pape, S., de Kanter, C. T., van den Berg, A. P., Drenth, J. P., and Burger, D. M. (2017). Decreased Tacrolimus Plasma Concentrations during HCV Therapy: a Drug-Drug Interaction or Is There an Alternative Explanation. Int. J. Antimicrob. Agents 49 (3), 379–382. doi:10.1016/j.ijantimicag.2016.12.004
Smolen, J. S., Aletaha, D., and McInnes, I. B. (2016). Rheumatoid Arthritis. Lancet 388 (10055), 2023–2038. doi:10.1016/S0140-6736(16)30173-8
Sonne, J., Døssing, M., Loft, S., and Andreasen, P. B. (1985). Antipyrine Clearance in Pneumonia. Clin. Pharmacol. Ther. 37 (6), 701–704. doi:10.1038/clpt.1985.117
Sotaniemi, E. A., Pelkonen, O., Arranto, A. J., Tapanainen, P., Rautio, A., and Pasanen, M. (2002). Diabetes and Elimination of Antipyrine in Man: an Analysis of 298 Patients Classified by Type of Diabetes, Age, Sex, Duration of Disease and Liver Involvement. Pharmacol. Toxicol. 90 (3), 155–160. doi:10.1034/j.1600-0773.2002.900308.x
Stanke-Labesque, F., Concordet, D., Djerada, Z., Bouchet, S., Solas, C., Mériglier, E., et al. (2021). Neglecting Plasma Protein Binding in COVID-19 Patients Leads to a Wrong Interpretation of Lopinavir Overexposure. Clin. Pharmacol. Ther. 109 (4), 1030–1033. doi:10.1002/cpt.2196
Stanke-Labesque, F., Gautier-Veyret, E., Chhun, S., and Guilhaumou, R.French Society of Pharmacology and Therapeutics (2020). Inflammation Is a Major Regulator of Drug Metabolizing Enzymes and Transporters: Consequences for the Personalization of Drug Treatment. Pharmacol. Ther. 215, 107627. doi:10.1016/j.pharmthera.2020.107627
Stavropoulou, E., Pircalabioru, G. G., and Bezirtzoglou, E. (2018). The Role of Cytochromes P450 in Infection. Front. Immunol. 9, 89. doi:10.3389/fimmu.2018.00089
Stenvinkel, P., and Alvestrand, A. (2002). Inflammation in End-Stage Renal Disease: Sources, Consequences, and Therapy. Semin. Dial. 15 (5), 329–337. doi:10.1046/j.1525-139x.2002.00083.x
Stults, B. M., and Hashisaki, P. A. (1983). Influenza Vaccination and Theophylline Pharmacokinetics in Patients with Chronic Obstructive Lung Disease. West. J. Med. 139 (5), 651–654.
Sulkowski, M., Wright, T., Rossi, S., Arora, S., Lamb, M., Wang, K., et al. (2005). Peginterferon Alfa-2a Does Not Alter the Pharmacokinetics of Methadone in Patients with Chronic Hepatitis C Undergoing Methadone Maintenance Therapy. Clin. Pharmacol. Ther. 77 (3), 214–224. doi:10.1016/j.clpt.2004.09.008
Takahashi, T., Masuya, Y., Ueno, K., Watanabe, K., Takahashi, M., Morita, S., et al. (2015). Clozapine-related Negative Myoclonus Associated with Urinary Tract Infection: a Case Report. J. Clin. Psychopharmacol. 35 (2), 205–206. doi:10.1097/JCP.0000000000000290
ten Bokum, E. M., van de Oever, H. L., Radstake, D. W., and Arbouw, M. E. (2015). Clozapine Intoxication Due to Cessation of Smoking and Infection. Neth. J. Med. 73 (7), 345–347.
Testa, S., Prandoni, P., Paoletti, O., Morandini, R., Tala, M., Dellanoce, C., et al. (2020). Direct Oral Anticoagulant Plasma Levels' Striking Increase in Severe COVID-19 Respiratory Syndrome Patients Treated with Antiviral Agents: The Cremona Experience. J. Thromb. Haemost. 18 (6), 1320–1323. doi:10.1111/jth.14871
Teunissen, M. W., Spoelstra, P., Koch, C. W., Weeda, B., van Duyn, W., Janssens, A. R., et al. (1984). Antipyrine Clearance and Metabolite Formation in Patients with Alcoholic Cirrhosis. Br. J. Clin. Pharmacol. 18 (5), 707–715. doi:10.1111/j.1365-2125.1984.tb02533.x
Tidball, J. G. (2005). Inflammatory Processes in Muscle Injury and Repair. Am. J. Physiol. Regul. Integr. Comp. Physiol. 288 (2), R345–R353. doi:10.1152/ajpregu.00454.2004
Toft, P., Heslet, L., Hansen, M., and Klitgaard, N. A. (1991). Theophylline and Ethylenediamine Pharmacokinetics Following Administration of Aminophylline to Septic Patients with Multiorgan Failure. Intensive Care Med. 17 (8), 465–468. doi:10.1007/BF01690768
Tracy, T. S., Korzekwa, K. R., Gonzalez, F. J., and Wainer, I. W. (1999). Cytochrome P450 Isoforms Involved in Metabolism of the Enantiomers of Verapamil and Norverapamil. Br. J. Clin. Pharmacol. 47 (5), 545–552. doi:10.1046/j.1365-2125.1999.00923.x
Tran, J. Q., Othman, A. A., Wolstencroft, P., and Elkins, J. (2016). Therapeutic Protein-Drug Interaction Assessment for Daclizumab High-Yield Process in Patients with Multiple Sclerosis Using a Cocktail Approach. Br. J. Clin. Pharmacol. 82 (1), 160–167. doi:10.1111/bcp.12936
Tuncer, M., Süleymanlar, G., Ersoy, F. F., and Yakupoğlu, G. (2000). Effects of Hepatitis C Virus Infection on Cyclosporine Trough Levels in Renal Transplant Patients. Transpl. Proc 32 (3), 569–571. doi:10.1016/s0041-1345(00)00893-9
Ueda, H., Sakurai, T., Ota, M., Nakajima, A., Kamii, K., and Maezawa, H. (1963). Disappearance Rate of Tolbutamide in Normal Subjects and in Diabetes Mellitus, Liver Cirrhosis, and Renal Disease. Diabetes 12, 414–419. doi:10.2337/diab.12.5.414
Ueda, Y., Kaido, T., and Uemoto, S. (2015). Fluctuations in the Concentration/dose Ratio of Calcineurin Inhibitors after Simeprevir Administration in Patients with Recurrent Hepatitis C after Liver Transplantation. Transpl. Int. 28 (2), 251–252. doi:10.1111/tri.12438
Ueda, Y., and Uemoto, S. (2016). Decreased Tacrolimus Concentration Following a Temporal Increase during Interferon-free Therapy with Asunaprevir and Daclatasvir in Patients with Recurrent Hepatitis C after Liver Transplantation. Transpl. Int. 29 (1), 119–121. doi:10.1111/tri.12653
Uptodate Smarter Decisions. Better Care. [Internet]. [cited 2020 Apr 21]. Available from: https://www.uptodate.com/home.
Urry, E., Jetter, A., and Landolt, H. P. (2016). Assessment of CYP1A2 Enzyme Activity in Relation to Type-2 Diabetes and Habitual Caffeine Intake. Nutr. Metab. (Lond) 13, 66. doi:10.1186/s12986-016-0126-6
van den Berg, A. P., Haagsma, E. B., Gouw, A. S., Slooff, M. J., and Jansen, P. L. (2001). Recurrent HCV Infection Reduces the Requirement for Tacrolimus after Liver Transplantation. Transpl. Proc 33 (1–2), 1467. doi:10.1016/s0041-1345(00)02553-7
van Wanrooy, M. J., Span, L. F., Rodgers, M. G., van den Heuvel, E. R., Uges, D. R., van der Werf, T. S., et al. (2014). Inflammation Is Associated with Voriconazole Trough Concentrations. Antimicrob. Agents Chemother. 58 (12), 7098–7101. doi:10.1128/AAC.03820-14
Vasquez, E. M., and Pollak, R. (1997). OKT3 Therapy Increases Cyclosporine Blood Levels. Clin. Transpl. 11 (1), 38–41.
Venuto, C. S., Lim, J., Messing, S., Hunt, P. W., McComsey, G. A., and Morse, G. D. (2018). Inflammation Investigated as a Source of Pharmacokinetic Variability of Atazanavir in AIDS Clinical Trials Group Protocol A5224s. Antivir. Ther. 23 (4), 345–351. doi:10.3851/IMP3209
Veringa, A., Ter Avest, M., Span, L. F., van den Heuvel, E. R., Touw, D. J., Zijlstra, J. G., et al. (2017). Voriconazole Metabolism Is Influenced by Severe Inflammation: a Prospective Study. J. Antimicrob. Chemother. 72 (1), 261–267. doi:10.1093/jac/dkw349
Vozeh, S., Powell, J. R., Riegelman, S., Costello, J. F., Sheiner, L. B., and Hopewell, P. C. (1978). Changes in Theophylline Clearance during Acute Illness. JAMA 240 (17), 1882–1884. doi:10.1001/jama.1978.03290170064030
Vreugdenhil, B., van der Velden, W. J. F. M., Feuth, T., Kox, M., Pickkers, P., van de Veerdonk, F. L., et al. (2018). Moderate Correlation between Systemic IL-6 Responses and CRP with Trough Concentrations of Voriconazole. Br. J. Clin. Pharmacol. 84 (9), 1980–1988. doi:10.1111/bcp.13627
Wadhawan, S., Jauhari, H., and Singh, S. (2000). Cyclosporine Trough Levels in Diabetic and Nondiabetic Renal Transplant Patients. Transpl. Proc 32 (7), 1683–1684. doi:10.1016/s0041-1345(00)01416-0
Wang, X. R., Qu, Z. Q., Li, X. D., Liu, H. L., He, P., Fang, B. X., et al. (2010). Activity of Sulfotransferase 1A1 Is Dramatically Upregulated in Patients with Hepatocellular Carcinoma Secondary to Chronic Hepatitis B Virus Infection. Cancer Sci. 101 (2), 412–415. doi:10.1111/j.1349-7006.2009.01404.x
Wang, Z., Hall, S. D., Maya, J. F., Li, L., Asghar, A., and Gorski, J. C. (2003). Diabetes Mellitus Increases the In Vivo Activity of Cytochrome P450 2E1 in Humans. Br. J. Clin. Pharmacol. 55 (1), 77–85. doi:10.1046/j.1365-2125.2003.01731.x
Weibert, R. T., Lorentz, S. M., Norcross, W. A., Klauber, M. R., and Jagger, P. I. (1986). Effect of Influenza Vaccine in Patients Receiving Long-Term Warfarin Therapy. Clin. Pharm. 5 (6), 499–503.
Wen, H., Chen, D., Lu, J., Jiao, Z., Chen, B., Zhang, B., et al. (2020). Probable Drug Interaction between Etanercept and Cyclosporine Resulting in Clinically Unexpected Low Trough Concentrations: First Case Report. Front. Pharmacol. 11, 939. doi:10.3389/fphar.2020.00939
Wienkers, L. C., and Heath, T. G. (2005). Predicting In Vivo Drug Interactions from In Vitro Drug Discovery Data. Nat. Rev. Drug Discov. 4 (10), 825–833. doi:10.1038/nrd1851
Wilkinson, G. R. (2005). Drug Metabolism and Variability Among Patients in Drug Response. N. Engl. J. Med. 352 (21), 2211–2221. doi:10.1056/NEJMra032424
Williams, M. L., Bhargava, P., Cherrouk, I., Marshall, J. L., Flockhart, D. A., and Wainer, I. W. (2000). A Discordance of the Cytochrome P450 2C19 Genotype and Phenotype in Patients with Advanced Cancer. Br. J. Clin. Pharmacol. 49 (5), 485–488. doi:10.1046/j.1365-2125.2000.00189.x
Williams, S. J., Baird-Lambert, J. A., and Farrell, G. C. (1987). Inhibition of Theophylline Metabolism by Interferon. Lancet 2 (8565), 939–941. doi:10.1016/s0140-6736(87)91422-x
Williams, S. J., and Farrell, G. C. (1986). Inhibition of Antipyrine Metabolism by Interferon. Br. J. Clin. Pharmacol. 22 (5), 610–612. doi:10.1111/j.1365-2125.1986.tb02943.x
Wolffenbüttel, L., Poli, D. D., Manfro, R. C., and Gonçalves, L. F. (2004). Cyclosporine Pharmacokinetics in Anti-hcv+ Patients. Clin. Transpl. 18 (6), 654–660. doi:10.1111/j.1399-0012.2004.00256.x
Wollmann, B. M., Syversen, S. W., Lie, E., Gjestad, C., Mehus, L. L., Olsen, I. C., et al. (2017). 4β-Hydroxycholesterol Level in Patients with Rheumatoid Arthritis before vs. After Initiation of bDMARDs and Correlation with Inflammatory State. Clin. Transl Sci. 10 (1), 42–49. doi:10.1111/cts.12431
Wu, J. J., and Fleming, K. F. (2011). Interaction between Adalimumab with Concurrent Pregabalin and Duloxetine Administration in a Psoriasis Patient with Diabetic Peripheral Neuropathy. Cutis 87 (5), 249–250.
Wu, K. C., and Lin, C. J. (2019). The Regulation of Drug-Metabolizing Enzymes and Membrane Transporters by Inflammation: Evidences in Inflammatory Diseases and Age-Related Disorders. J. Food Drug Anal. 27 (1), 48–59. doi:10.1016/j.jfda.2018.11.005
Xu, R., Ge, W., and Jiang, Q. (2018). Application of Physiologically Based Pharmacokinetic Modeling to the Prediction of Drug-Drug and Drug-Disease Interactions for Rivaroxaban. Eur. J. Clin. Pharmacol. 74 (6), 755–765. doi:10.1007/s00228-018-2430-8
Xu, Y., Hijazi, Y., Wolf, A., Wu, B., Sun, Y. N., and Zhu, M. (2015). Physiologically Based Pharmacokinetic Model to Assess the Influence of Blinatumomab-Mediated Cytokine Elevations on Cytochrome P450 Enzyme Activity. CPT Pharmacometrics Syst. Pharmacol. 4 (9), 507–515. doi:10.1002/psp4.12003
Yamamoto, Y., Takahashi, Y., Horino, A., Usui, N., Nishida, T., Imai, K., et al. (2018). Influence of Inflammation on the Pharmacokinetics of Perampanel. Ther. Drug Monit. 40 (6), 725–729. doi:10.1097/FTD.0000000000000556
Yang, L. Q., Li, S. J., Cao, Y. F., Man, X. B., Yu, W. F., Wang, H. Y., et al. (2003). Different Alterations of Cytochrome P450 3A4 Isoform and its Gene Expression in Livers of Patients with Chronic Liver Diseases. World J. Gastroenterol. 9 (2), 359–363. doi:10.3748/wjg.v9.i2.359
Yasu, T., Konuma, T., Kato, S., Kurokawa, Y., Takahashi, S., and Tojo, A. (2017). Serum C-Reactive Protein Levels Affect the Plasma Voriconazole Trough Levels in Allogeneic Hematopoietic Cell Transplant Recipients. Leuk. Lymphoma 58 (11), 2731–2733. doi:10.1080/10428194.2017.1300897
Zhou, Y., Ingelman-Sundberg, M., and Lauschke, V. M. (2017). Worldwide Distribution of Cytochrome P450 Alleles: A Meta-Analysis of Population-Scale Sequencing Projects. Clin. Pharmacol. Ther. 102 (4), 688–700. doi:10.1002/cpt.690
Zhuang, Y., de Vries, D. E., Xu, Z., Marciniak, S. J., Chen, D., Leon, F., et al. (2015). Evaluation of Disease-Mediated Therapeutic Protein-Drug Interactions between an Anti-interleukin-6 Monoclonal Antibody (Sirukumab) and Cytochrome P450 Activities in a Phase 1 Study in Patients with Rheumatoid Arthritis Using a Cocktail Approach. J. Clin. Pharmacol. 55 (12), 1386–1394. doi:10.1002/jcph.561
Keywords: inflammation, cytochrome P450, pharmacokinetic, disease-drug interaction, cytokines
Citation: Lenoir C, Rollason V, Desmeules JA and Samer CF (2021) Influence of Inflammation on Cytochromes P450 Activity in Adults: A Systematic Review of the Literature. Front. Pharmacol. 12:733935. doi: 10.3389/fphar.2021.733935
Received: 30 June 2021; Accepted: 13 October 2021;
Published: 16 November 2021.
Edited by:
Celine Verstuyft, Université Paris-Saclay, FranceReviewed by:
Francoise Stanke-Labesque, Université Grenoble Alpes, FranceTomoyuki Mizuno, Cincinnati Children’s Hospital Medical Center, United States
Copyright © 2021 Lenoir, Rollason, Desmeules and Samer. This is an open-access article distributed under the terms of the Creative Commons Attribution License (CC BY). The use, distribution or reproduction in other forums is permitted, provided the original author(s) and the copyright owner(s) are credited and that the original publication in this journal is cited, in accordance with accepted academic practice. No use, distribution or reproduction is permitted which does not comply with these terms.
*Correspondence: Camille Lenoir, Q2FtaWxsZS5MZW5vaXJAaGN1Z2UuY2g=