- School of Pharmacy, Shanghai University of Traditional Chinese Medicine, Shanghai, China
Pulmonary fibrosis, a common outcome of pulmonary interstitial disease of various different etiologies, is one of the most important causes of respiratory failure. Houttuynia cordata Thunb. (family: Saururaceae) (H. cordata), as has been reported, is a Chinese herbal medicine commonly used to treat upper respiratory tract infection and bronchitis. Our previous study has proven that sodium houttuyfonate (an additional compound from sodium bisulfite and houttuynin) had beneficial effects in the prevention of pulmonary fibrosis (PF) induced by bleomycin (BLM) in mice. In the present study, network pharmacology was used to investigate the efficiency and potential mechanisms of H. cordata in PF treatment. Upon manual collection from the literature and databases such as TCMSP and TCM-ID, 10 known representative ingredients of H. cordata species were screened. Then, the prediction of the potential active ingredients, action targets, and signaling pathways were conducted through the Gene Ontology (GO), protein–protein interaction (PPI),and Kyoto Encyclopedia of Genes and Genomes (KEGG) enrichment analyses. The results of network pharmacology prediction suggested that H. cordata may act through multiple signaling pathways to alleviate PF, including the phosphatidylinositol 3-kinase-protein kinase B (PI3K/AKT) pathways, mitogen-activated protein kinase (MAPK) pathways, the tumor necrosis factor (TNF) pathways, and interleukin-17 (IL-17) signaling pathways. Molecular docking experiments showed that the chemical constituents of H. cordata had good affinity with TNF, MAPK1, and AKT1, and using lipopolysaccharide (LPS)-induced A549 cells, a model was established to verify the anti-pulmonary fibrosis effects and related mechanisms of H. cordata–relevant constituents. Finally, these evidences collectively suggest H. cordata may alleviate PF progression via PI3K/Akt, MAPK, and TNF signaling pathways and provide novel insights to verify the mechanism of H. cordata in the treatment of PF.
Introduction
Pulmonary fibrosis (PF) is a chronic, progressive, and devastating interstitial lung disease mainly resulting from toxic insults, autoimmune injuries, drug-induced injuries, infectious injuries, or traumatic injuries. As can be affected by age, genetic predisposing factors, and environmental exposures, PF produces many different pathological types, which are mainly classified as idiopathic pulmonary fibrosis (IPF) and fibrosing alveolitis. PF is mainly characterized by diffuse pneumonia in the early period, secreting pro-inflammatory cytokines such as the tumor necrosis factor α (TNF-α), interleukin-1β (IL-1β), and IL-6. Alveolar epithelial cell (AEC) injury and excessive deposition of collagen in extracellular matrix (ECM) ultimately result in progressive scarring and loss of lung function (Wynn and Vannella, 2016). With approximately 3 million people being affected worldwide, as shown in the related literature, IPF has an increasing burden (George et al., 2020). At present, there is no effective means or drugs that can ameliorate PF except lung transplantation in patients with a median survival of 3–5 years from the time of diagnosis (Meyer, 2017).
PF, with clinical manifestations such as progressive dyspnea, cough, sputum, chest pain, vomiting, dry mouth, dry throat, shortness of breath, and fatigue, was defined as “lung impediment” or “lung wilting” in books of traditional Chinese medicine, and its syndrome types were divided into lung–kidney Qi deficiency (Fei shen qi xu), dual deficiency of the lung and spleen (Pi fei liang xu), Qi deficiency with blood stasis (Qi xu xue yu), and phlegm obstructing the vessels and collaterals (Tan zu mai luo). They were mainly treated by methods of invigorating the lungs and kidneys (Bu fei yi shen), fortifying the spleen and boosting the lung (Jian pi yi fei), promoting blood circulation and dredging collaterals (Huo xue tong luo), transforming dampness and dispelling phlegm (Hua shi qu tan), and harmonizing and percolating dampness (He jie shen shi). The treatment process used decoctions including Schisandra decoction, Bufei decoction, and Buyang Huanwu decoction , and heat-clearing and toxin-resolving Chinese medicinal materials such as Centella asiatica (L.) Urban, Ophiopogon japonicus (L.f.) Ker-Gawl., and Houttuynia cordata Thunb. (family: Saururaceae) (H. cordata). The traditional Chinese medicine (TCM) mainly produces synergistic effects via the synergistic mechanism of actions with the active ingredients, enhancing functions, and producing less toxicity, which makes enormous contributions to modern drug development and disease treatment. Some active ingredients in the traditional Chinese medicine play a good role in the progression of PF, just like paclitaxel (PTX) (Wang et al., 2013), tanshinone IIA (Tan IIA) (He et al., 2015), and andrographolide (Andro) (Guo et al., 2016).
H. cordata, famous in China, Japan, Korea, and Northeast India for its medicinal properties, is used in the treatment of a number of diseases such as pneumonia, diabetes, and even SARS (Lau et al., 2008). Studies have found that H. cordata also has a certain inhibitory effect on PF; for instance, the volatile oil of H. cordata contains 4-terpineol, α-terpineol, l-bornyl acetate, and methyl-n-nonyl ketone, and has good anti-inflammatory and anti-pulmonary fibrosis effects in vitro and in vivo (Du et al., 2012). The aqueous extract of H. cordata has effects of anti-pulmonary fibrosis by inhibiting the expression of superoxide dismutase, malondialdehyde, hydroxyproline, interferon-γ (IFN-γ), and TNF-α (Ng et al., 2007). Qing fei xie ding granules (Sun et al., 2018), mainly including Ephedra sinica, apricot kernels, and H. cordata, have a protective effect on PF as a novel Chinese traditional patent medicine. However, the anti-pulmonary fibrosis mode and the mechanism of action of H. cordata are not fully clarified. To address the protective effects of H. cordata on PF, our previous study has shown that sodium houttuyfonate is an attractive candidate alleviating IPF and pulmonary toxicity induced by BLM through downregulation of TGFβ1-Smad2/3 signal transduction (Shen et al., 2021).
Despite the obvious medicinal effects and clinical uses of TCM, little has been elucidated regarding the underlying molecular mechanisms. Network pharmacology integrates several disciplines, including systems biology, network biology, computational biology, multi-target pharmacology, and molecular pharmacology. And the core is the construction of a drug molecule–target–disease link network and the systematic analysis of the three relationships. It can be used to predict active molecules and actions of TCM, and then to search for new pharmacological mechanisms of action and broaden the indications of TCM, etc. Molecular docking is used to design drugs by in silico modeling of the interaction of target proteins with drug molecules. Molecular docking has been gradually applied to reveal the relevant mechanism of action of drugs in the development of new drugs. This study aims to utilize network pharmacology and molecular docking to seek the active compounds of H. cordata, construct the drug–compounds–genes–disease network, and to analyze the underlying mechanism of H. cordata in the treatment of PF.
Methods
Main Candidate Active Ingredients and Targets of H. cordata
The keyword “Houttuyniae Herba” was searched in TCMSP (traditional Chinese medicine ystems pharmacology database and analysis platform, http://tcmspw.com/tcmsp.php) and TCM-ID (traditional Chinese medicine integrated database, http://www.megabionet.org/tcmid/). Active ingredients were retrieved from them. The chemical information of main active ingredients was collected from the PubChem database (https://pubchem.ncbi.nlm.nih.gov/) and the Canonical SMILES of all ingredients were traced back to PubChem (http://pubchem.ncbi.nih.gov). The major components in H. cordata were obtained by a literature review and screening of public databases. The potential therapeutic targets of the main active components were predicted by matching them in the TCMSP, SEA (https://sea.bkslab.org/), HitPick (http://mips.helmholtz-muenchen.de/hitpick/), SwissTargetPrediction (http://www.swisstargetprediction.ch/), and STITCH (http://stitch.embl.de/) tools. Among them, according to TC > 0.4 in SEA, according to precision >50% SwissTargetPrediction in HitPick, the top 15 with the highest scores were selected, and according to score >0.4 in stitch.
Mining of Pulmonary Fibrosis–Related Targets
With “pulmonary fibrosis” as the keyword, DisGeNET (https://www.disgenet.org/), GeneCards (https://www.genecards.org/), and OMIM (https://www.omim.org/) potential disease target analysis platforms were utilized to search for human genes related to PF. After data deduplication/integration, intersecting genes were obtained and considered as therapeutic targets relevant to PF.
Drug–Target–Disease Network Construction
Active ingredients of H. cordata–related targets and PF-related targets were imported into the Venn online tool (http://www.bioinformatics.com.cn/) to obtain the intersection and a Venn diagram, in which potential targets of H. cordata for the treatment of PF were revealed. The above potential target gene ID was identified and standardized through the UniProt database (https://www.uniprot.org). The target network of H. cordata major ingredients acting on PF was analyzed by Cytoscape 3.7.1 built-in tools.
Protein–Protein Interaction Network Analysis
To determine the key H. cordata targets, common targets were used to build a PPI network using the STRING database (https://string-db.org/) and Cytoscape software. And the core target proteins were identified in the PPI network.
Go Analysis and KEGG Pathway Enrichment Analysis
The overlapped targets were further analyzed by the GO function analysis and KEGG enrichment analysis using the clusterProfiler package based on R language. The filtering thresholds for the retrieved results were p < 0.05 and q < 0.05.
Molecular Docking Simulation
The binding ability of key components and key targets was verified to explore the accurate binding modes through molecular docking simulation. The PDB database (http://www.rcsb.org/) and the optimal models visualized using PyMOL (2.0) software (http://www.pymol.org/2/) and AutoDockVina software (http://vina.scripps.edu/) were used to perform the molecular docking of active components and core targets.
Experiment Validation
Materials and Methods
Chemicals and Regents
Sodium new houttuyfonate (SNH, MW: 330.41, purity ≥ 98%), quercetin (MW: 302.24, purity ≥ 97%), and 2-undecanone (MW: 170.29, purity ≥ 99%) were purchased from Shanghai Yuanye Biotechnology Co., Ltd. (Shanghai, China).
Cell Culture
A549 cells, obtained from the Cell Bank of the Chinese Academy of Sciences, were cultured in DMEM-H with 10% fetal bovine serum (Gibco, United States), 100 U/mL penicillin, and 100 ng/ml streptomycin with 5% CO2 at 37°C. The EMT model of lipopolysaccharide (LPS)-induced A549 was reproduced in our study (Cui et al., 2019). For the EMT model, when the A549 cells reached 70–80% confluence on 6-well tissue culture plates, they were placed in DMEM-H supplemented with 1% fetal bovine serum for 12 h. Subsequently, the culture was replaced to DMEM-H with 2% fetal bovine serum and then treated with 10 μg/ml LPS (from Escherichia coli 0111: B4, Sigma-Aldrich, St. Louis, MO, United States) and SNH, quercetin, and 2-undecanone alone or in combination for up to 24 h, respectively.
Western Blot Analyses
The cells were lysed in RIPA lysis buffer (Beyotime, China) with 1% phenylmethylsulfonylfluoride (PMSF, Beyotime, China), 2% phosphorylated proteinase inhibitor (Beyotime, China), and 2% protease inhibitor (Beyotime, China). And 30 μg of the protein was separated using sodium dodecyl sulfate polyacrylamide gel electrophoresis (SDS-PAGE) (10%), and then was transferred onto a PVDF membrane. After blocking, the membranes were incubated overnight at 4°C with the primary antibody against β-actin (1:2,000, Affinity, United States), Phospho-AKT (1:1,000, Proteintech, United States), AKT (1:1,000, CST, United States), p44/42 MAPK (ERK1/2) (1:1,000, CST, United States of America), Phospho-p44/42 MAPK (ERK1/2) (1:1,000, CST, United States), Phospho-p38 MAPK (1:1,000, CST, United States), and TNF-α (1:1,000, CST, United States). Next, membranes were washed in TBS-T several times, and then were incubated for 2 h in horseradish peroxidase (HRP)-linked anti-rabbit (1:2,000, CST, United States) or anti-mouse (1:2,000, CST, United States) at room temperature. After washing with TBS-T again, the protein bands were analyzed using ChemiScope 3500mini exposure instrument (Clinx Science Instruments Co., Ltd. Shanghai, China) with a chemiluminescence substrate.
Statistical Analysis
All values were expressed as mean ± standard deviation (SD), and data and analyses for graphing were processed with SPSS 25.0 statistical software and GraphPad Prism 8.0.2 software. Comparisons between groups were performed using one-way ANOVA. Values of p < 0.05 were considered statistically significant.
Results
This study reveals the mechanism of the anti-pulmonary fibrosis pharmacological action of H. cordata by a TCM network pharmacology–based strategy and provides ideas for H. cordata for further drug development. This workflow is shown in Figure 1.
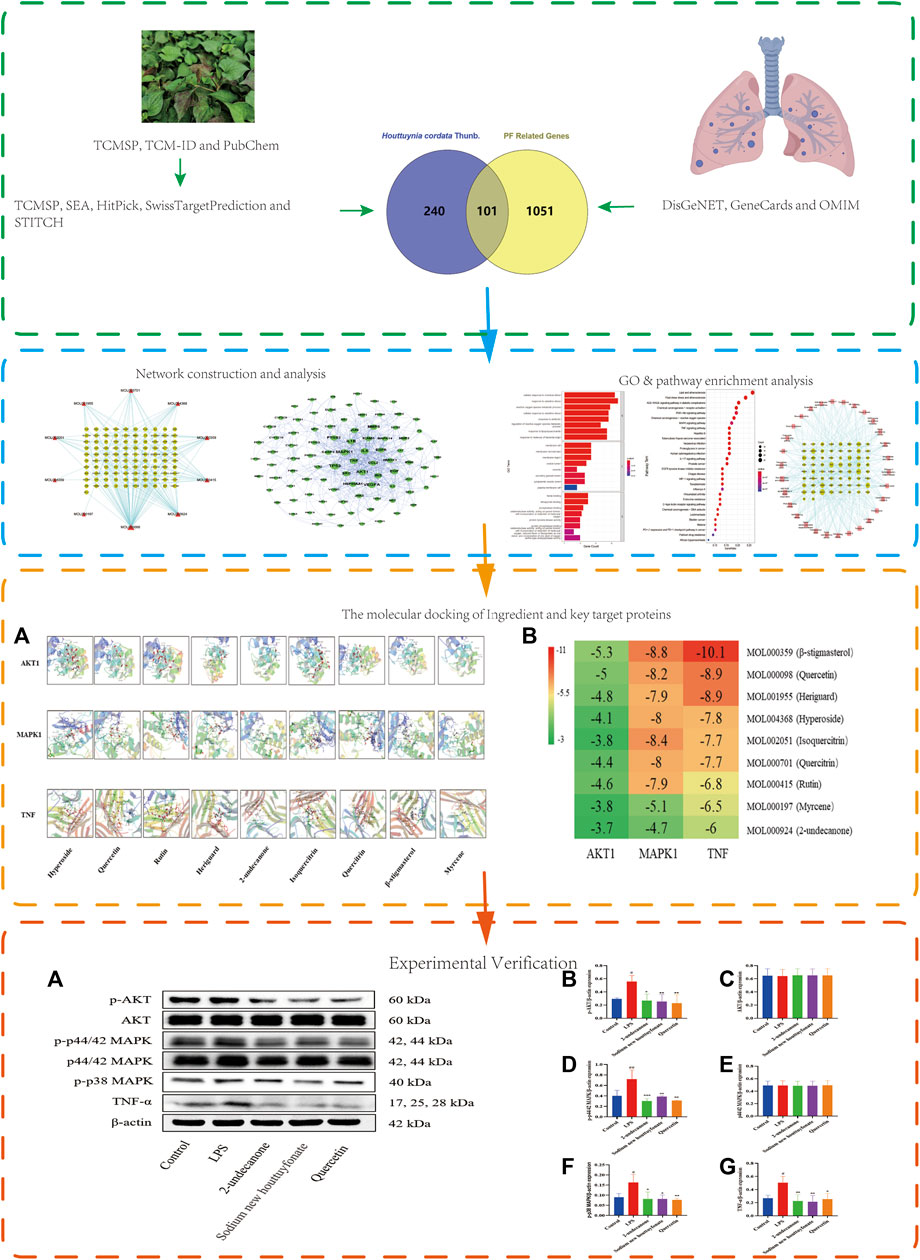
FIGURE 1. Flowchart of network pharmacology research on the mechanism of H. cordata in anti-pulmonary fibrosis.
H. cordata’s Active Ingredients and Potential Target Prediction Results
H. cordata’s chemical constituents were collected from TCMSP. A total of 10 main components were candidated after removing the redundant ones from the literature and public databases. The 10 components were as follows: hyperoside, quercetin, rutin, heriguard, houttuynin, 2-undecanone, isoquercitrin, quercitrin, β-stigmasterol, and myrcene (Table 1). A total of 341 targets for the 10 chemical compositions were obtained after gene standardization and the elimination of repetitive values.
Feature Analysis of Active Compound–Drug Target Network
PF-related targets were collected from DisGeNET, GeneCards, OMIM, and Drugbank databases. After merging and deleting duplicate values, a total of 1,152 targets were obtained. As shown in Figure 2, 101 predicted targets were obtained after the intersection of drug-related targets and PF-related targets. The details of common target genes are shown in Table 2. 101 therapeutic targets were confirmed by the UniProt database, and a predicted target network of H. cordata's major ingredient’s actions on PF was constructed using Cytoscape software (Figure 3).
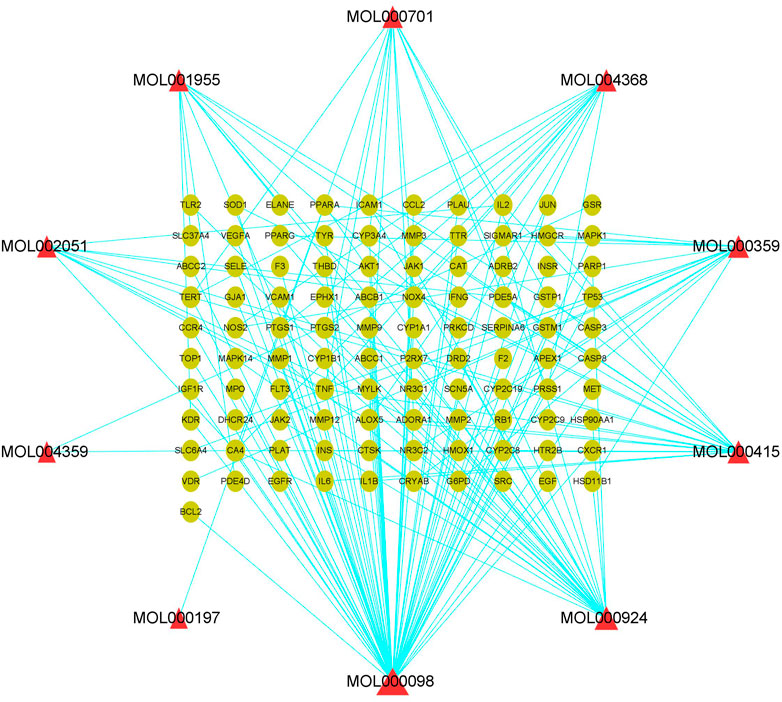
FIGURE 3. Component target network of 10 active ingredients of H. cordata against pulmonary fibrosis, in which triangles represent active ingredients and dots are action targets.
PPI Network Analysis Results
A predicted PPI relationship network was obtained after the input of 101 predicted targets into the STRING platform and Cytoscape for topological analysis. MAPK1 (ERK2), TNF, AKT1, VEGFA, TP53, IL-1β, and IL-6 were advised to be the core (hub) node owing to a high network degree value, betweenness centrality, and closeness centrality in the PPI regulatory network (Figure 4).
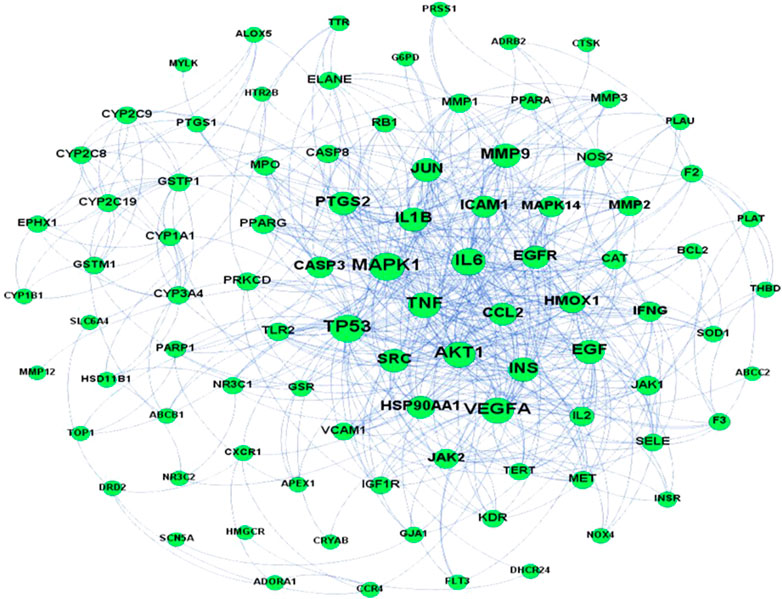
FIGURE 4. PPI network of potential pulmonary fibrosis targets acted upon by major ingredients of H. cordata.
GO Analysis and KEGG Pathway Enrichment Analysis of Shared Targets
With the clusterProfiler package based on R language and a filtering condition of p < 0.05 and q < 0.05, GO and KEGG pathway enrichment analyses of the 101 intersection targets were used to determine the biological functions of PF that were affected by H. cordata. GO enrichment items were composed of biological process (BP) terms, cell composition (CC) terms, and molecular function (MF) terms (Figure 5). The BP was related to the response to oxidative stress (GO: 0006979), cellular response to chemical stress (GO: 0062197), reactive oxygen species metabolic process (GO: 0072593), cellular response to oxidative stress (GO: 0034599), response to antibiotic (GO: 0046677), regulation of the reactive oxygen species metabolic process (GO: 2000377), response to lipopolysaccharide (GO: 0032496), and response to molecules of bacterial origin (GO: 0002237); in the part of CC, we obtained membrane raft (GO: 0045121), membrane microdomain (GO: 0098857), membrane region (GO: 0098589), vesicle lumen (GO: 0031983), caveola (GO: 0005901), secretory granule lumen (GO: 0034774), cytoplasmic vesicle lumen (GO: 0060205), and plasma membrane raft (GO: 0044853); in the aspect of MF, there were heme binding (GO: 0020037), tetrapyrrole binding (GO: 0046906), phosphatase binding (GO: 0019902), oxidoreductase activity, acting on paired donors, with incorporation or reduction of molecular oxygen (GO: 0016705), protein tyrosine kinase activity (GO: 0004713), protein phosphatase binding (GO: 0019903), oxidoreductase activity, acting on paired donors, with incorporation or reduction of molecular oxygen, reduced flavin or flavoprotein as one donor, and incorporation of one atom of oxygen (GO: 0016712), and serine-type endopeptidase activity (GO: 0004252). The mechanism of H. cordata treatment of PF may be the result of the synergistic effects of multiple pathways. 101 targets were subjected to KEGG pathway enrichment analysis, with a total of 143 pathways, and the top 30 significantly enriched KEGG pathways were identified and selected for visualization (Figure 6). The action target main enriched pathway network is shown in Figure 7.
The Molecular Docking of Ingredient and Key Target Proteins
Three core target proteins (AKT1, MAPK1, and TNF-α) with high degrees were identified with nine active compounds (quercetin, hyperoside, rutin, heriguard, 2-undecanone, isoquercitrin, quercitrin, β-stigmasterol, and myrcene) by AutoDock Vina. According to the results of the molecular docking activity, the compounds selected generally had a moderate binding potential with a good medicinal reference value (Figure 8A). The docking scores were visualized using a heatmap (Figure 8B). Ultimately, three target proteins and three small molecule compounds with good docking affinity and higher content in H. cordata were used for subsequent experimental studies.
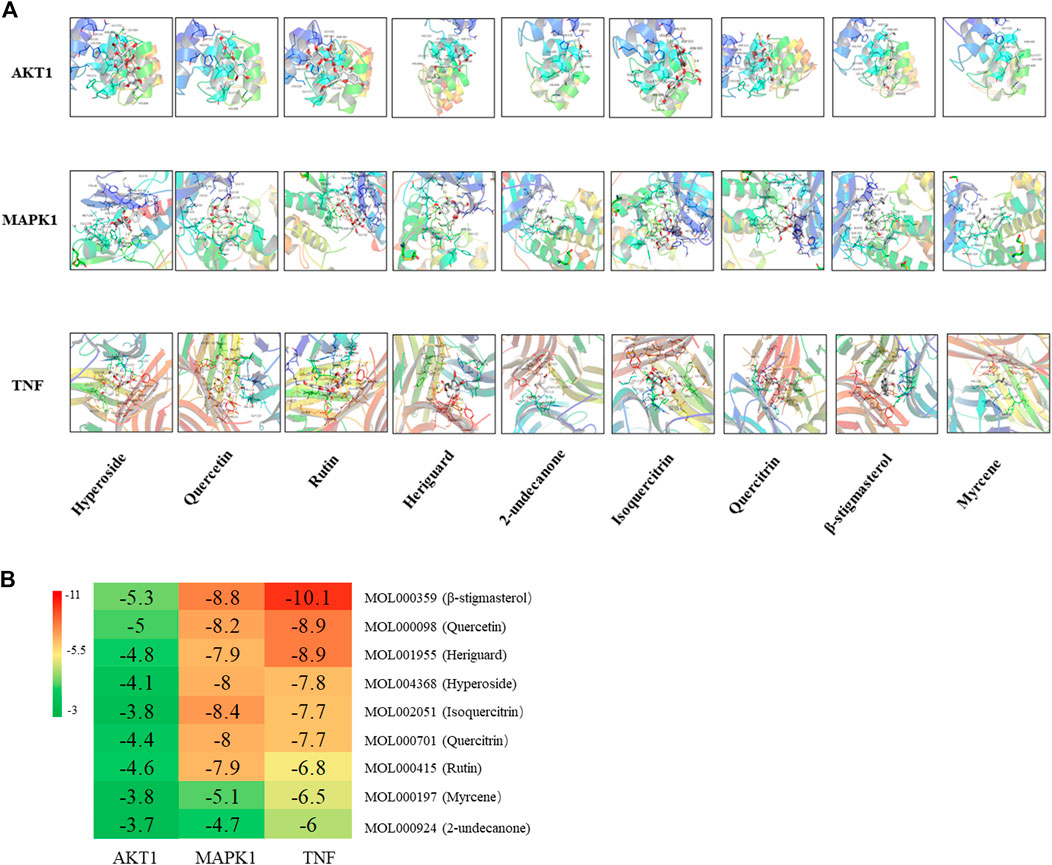
FIGURE 8. (A) Molecular docking results of the “bioactive compound-target gene.” (B) Heatmap of the docking scores of the main chemical constituents of H. cordata and the target proteins.
Experimental Demonstration In Vitro
Based on the results of network pharmacology and molecular docking, the anti-pulmonary fibrosis effect of 2-undecanone, sodium new houttuyfonate, and quercetin was necessary to be assessed with three protein-related pathways. According to the results of pre-experiment and literature data, 0.2 mM 2-undecanone, 0.3 mM sodium new houttuyfonate, and 0.05 mM quercetin were used in subsequent research. As the results were obtained, the expression of p-AKT, AKT, p44/42 MAPK (ERK1/2), p-p44/42 MAPK (ERK1/2), p-p38 MAPK, and TNF-α protein in the drug group was significantly decreased compared with that in the model group (p < 0.05 or p < 0.01) (Figures 9A–G). Our results showed that 2-undecanone, sodium new houttuyfonate, and quercetin inhibited the production of fibrosis in LPS-induced A549 cells.
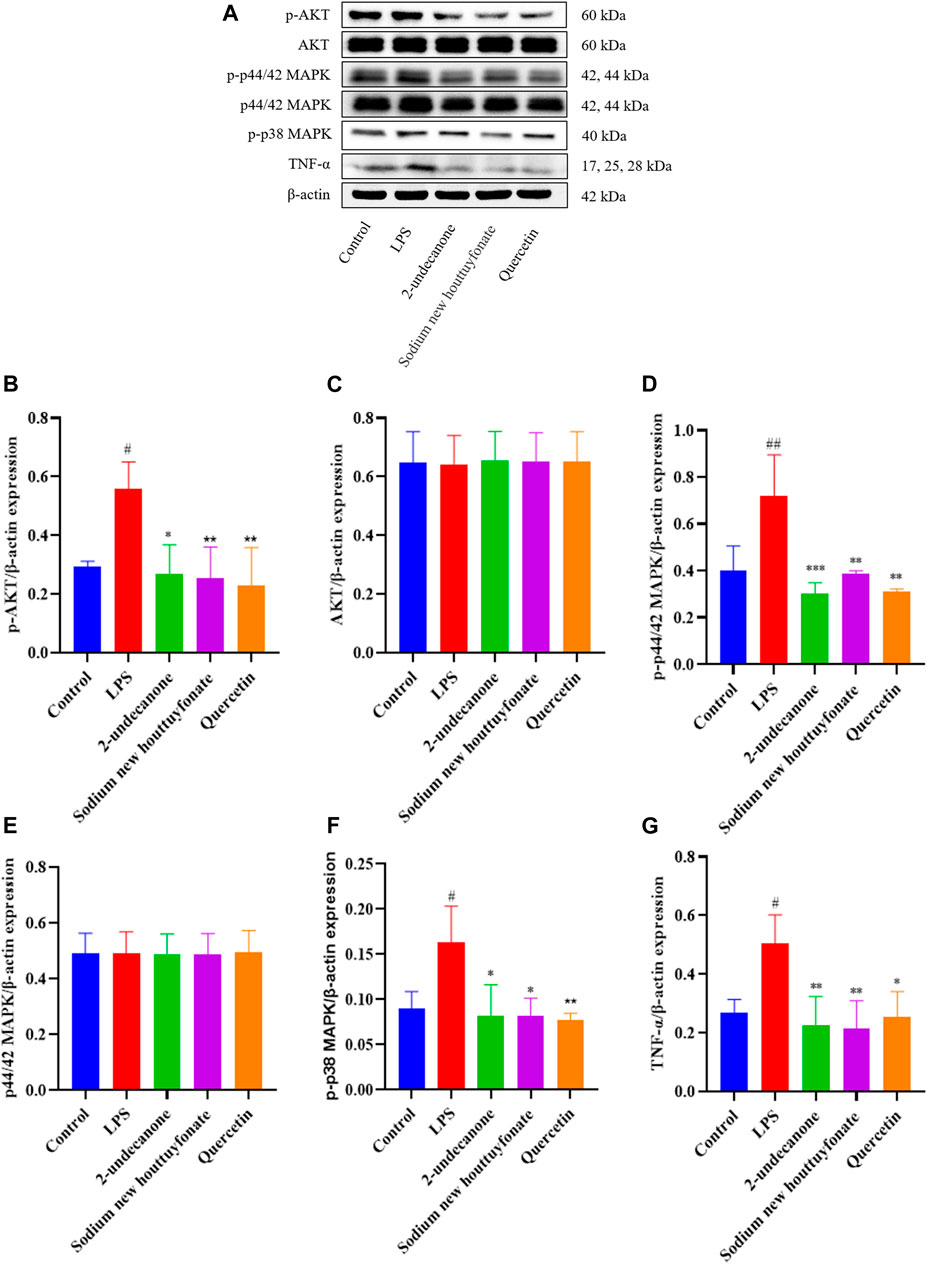
FIGURE 9. Effects of 2-undecanone, sodium new houttuyfonate, and quercetin on the protein expression of AKT, p-AKT, p44/42 MAPK (ERK1/2), p-p44/42 MAPK (ERK1/2), p-p38 MAPK, and TNF-α of LPS-induced A549 cells (A–G). #p < 0.05, ##p < 0.01, compared with the control group. *p < 0.05, **p < 0.01, compared with the LPS group.
Discussion
PF is a progressive and severe condition characterized by mesenchymal cell proliferation and differentiation, ECM deposition, and remodeling of the lung parenchyma and airways. Although progress has been made in the research on PF, our understanding of its pathological mechanism remains rudimentary. There is still no complete cure for PF, and current therapies merely act to delay but not completely stop the progression of the disease.
H. cordata, a Chinese herb with special odor, is mainly used to treat bronchitis and upper respiratory tract infection. And studies have reported that it can inhibit rapid PF by upregulating IFN-γ and inhibiting the TGF-β1/Smad pathway (Du et al., 2012). 2-Undecanone, houttuynin, and quercetin are the main constituents of H. cordata. Houttuynin is the easily polymerized major constituent of the volatile oil, and the content is usually about 15%, which was first isolated from H. cordata in 1952 (Pan et al., 2010). Because houttuynin was unstable in chemical characteristics, sodium new houttuyfonate (sodium lauroyl-α-hydroxyethyl sulfonate, C14H27NaO5S, MW = 330.4), an adduct compound of houttuynin and sodium bisulfite, was developed and put in use. Sodium new houttuyfonate has better water solubility and stability and can be transformed into houttuynin under physiological conditions. Previous studies have shown that the lung is the target organ of houttuynin, and it has good therapeutic effects on pneumonia (Deng et al., 2012). Also, sodium new houttuyfonate is an effective clinical therapeutic agent applied to respiratory infection and inflammation such as acute or chronic bronchitis and pneumonia. 2-Undecanone is used as a standard marker for the quality control of H. cordata in the Chinese pharmacopoeia, and its content is usually about 17.0–27.5% (Yu-hong et al., 2015). Studies have shown that 2-undecanone performed various types of pharmacological functions, including anti-inflammatory and antitumor effects (Chen et al., 2014; Lou et al., 2019). Quercetin is one of the major flavonoid-active components in H. cordata, and the content is usually about 0.50 mg/g (Xiao-lei and Xiao-yin, 2013), which could overexpress the FasL receptor and caveolin-1 expression, reducing AKT activation and eliminating apoptosis resistance in IPF (Hohmann et al., 2019). Quercetin increases the Nrf2 activity to defend against oxidation, restore the disturbed redox balance, and reduce inflammation (Veith et al., 2017). As has been reported, quercetin can also reduce the expression of IL-6, TNF-α, and IL-1β in the bronchoalveolar lavage fluid of bleomycin-induced PF in vivo (Baowen et al., 2010).
Our results analyzed the ingredients in H. cordata, as well as the functions and potential targets against PF. According to the topological property analysis of the “drug–disease” network and the results of the PPI network analysis, the targets of the action of H. cordata were members of the mitogen-activated protein kinase (MAPK) family, TNF, AKT, or the cytokine VEGF. The results of the GO functional enrichment analysis revealed that H. cordata is mainly involved in signal transduction, inflammatory immunity, regulation of chemokine production, etc. The KEGG enrichment analysis showed that the possible pathways involved in the anti-pulmonary fibrosis of H. cordata mainly included the phosphatidylinositol-3-kinase (PI3K)/Akt signaling pathway, MAPK signaling pathway, TNF signaling pathway, IL-17 signaling pathway, HIF-1 signaling pathway, and NOD-like receptor signaling pathway. All the above signaling pathways are related to inflammation and immune responses, indicating that H. cordata exerts immunomodulatory and anti-inflammatory effects through multiple pathways, exactly in accordance with our previous study which shows that sodium houttuyfonate reduces BLM-induced elevation of inflammatory cytokines such as IL-1, IL-6, and TNF-α in mice (Shen et al., 2021). The PI3K/Akt signaling pathway mainly regulates cell proliferation, inflammation, and survival, and maintains the biological properties of malignant cells. PI3K, consisting of a regulatory subunit, p85, and a catalytic subunit, p110, primarily exist in the cytoplasm. Akt, also known as the protein kinase B (PKB), regulates and controls cell proliferation and apoptosis. After PI3K activation by tyrosine kinases, PIP3 was generated on the plasma membrane to interact with the PH domain of Akt, and Thr308 and Ser473 of Akt will be phosphorylated with the help of 3-phosphoinositide–dependent protein kinase 1 (PDK1) and PDK2, Akt-activated (Assinder et al., 2009). PI3K/Akt contributes to the development and progression of PF by upregulating cell growth and collagen expression (Lu et al., 2010). TNF-α, a key regulator of inflammation, could initiate and drive many pulmonary pathological diseases by inducing the accumulation of inflammatory cells, causing oxidative and nitrosative stress, airway hyperactivity, and tissue remodeling (Blaser et al., 2016). TNF-α stimulates epithelial cell proliferation and contributes to epithelial thickening and PF (Sasaki et al., 2000). MAPKs, serine–threonine protein kinases, have been proven to control cellular processes associated with fibrosis, such as myofibroblast transformation and others (Xu et al., 2004). The extracellular signal–regulated kinases 1 and 2 (ERK1/2) cascade can regulate cell proliferation, differentiation, EMT, and tumorigenesis. The activation of ERK signaling causes morphological changes and the downregulation of E-cadherin expression in epithelial cells (Grände et al., 2002). Next, molecular docking studies for MAPK1, AKT1, and TNF-α were performed using the active constituents 2-undecanone, quercitrin, β-stigmasterol, myrcene, isoquercitrin, heriguard, rutin, quercetin, and hyperoside to evaluate the thermodynamic binding effects of proteins and ligands. The results showed that the target proteins and components had good binding effects. And quercetin showed great binding strength with MAPK1, AKT1, and TNF-α, which may play an essential role in PF.
LPS, an endotoxin component of Gram-negative bacilli as an inflammatory inducer, is a vital promoter of acute lung injury (ALI) as well as promoting EMT formation in various cell types including alveolar epithelial cells (AECs) (Dong et al., 2017; Xiao et al., 2020). A549 cells were added to LPS coculture to induce EMT validating the predicted outcome of network pharmacology. Evidence showed that quercetin was well validated by its docking to IL-6, TNF, and IL-1β proteins (Yu et al., 2021). These findings strikingly supported the molecular docking and experimental results of our study that quercetin may exert anti-PF effects by targeted binding to TNF. And sodium houttuyfonate inhibited the expression of inflammatory cytokine TNF-α, which was consistent with our previous findings in bleomycin-induced pulmonary fibrosis in mice. Results from our study revealed the potential mechanism of H. cordata and suggested that H. cordata has a clinical application value in attenuating PF because of its effect in inhibiting the expression of AKT, p-AKT, p44/42 MAPK (ERK1/2), p-p44/42 MAPK (ERK1/2), p-p38 MAPK, and TNF-α via the PI3K/AKT, MAPK, and TNF signaling pathways. However, the mechanism needs more investigation in vitro and in vivo models.
Conclusion
Our present study has important implications for the current understanding of the molecular mechanism of H. cordata in anti-pulmonary fibrosis, which involves multicomponents, multi-targets, and multi-pathways. An in-depth study should be considered by taking H. cordata as a novel anti-pulmonary fibrotic drug.
Data Availability Statement
The original contributions presented in the study are included in the article/Supplementary Material; further inquiries can be directed to the corresponding author.
Author Contributions
Y-HS: conception and design, methodology, data curation, visualization, investigation, supervision, validation, writing—reviewing and editing, project administration, and funding acquisition. D-WZ: investigation, methodology, data curation, data and image processing, visualization, validation, writing—original draft preparation, and writing—reviewing and editing, QY: software, data curation, data and image processing, visualization, and validation. J-JS: conceptualization, methodology, software, data curation, data and image processing, and visualization. All authors contributed to and have approved the final manuscript.
Funding
This work was supported by the Foundation of Shanghai Municipal Education Commission (No. 09JW08), the “Out-oriented Xinglin Talent Program.”
Conflict of Interest
The authors declare that the research was conducted in the absence of any commercial or financial relationships that could be construed as a potential conflict of interest.
Publisher’s Note
All claims expressed in this article are solely those of the authors and do not necessarily represent those of their affiliated organizations, or those of the publisher, the editors, and the reviewers. Any product that may be evaluated in this article, or claim that may be made by its manufacturer, is not guaranteed or endorsed by the publisher.
Supplementary Material
The Supplementary Material for this article can be found online at: https://www.frontiersin.org/articles/10.3389/fphar.2021.733618/full#supplementary-material
References
Assinder, S. J., Dong, Q., Kovacevic, Z., and Richardson, D. R. (2009). The TGF-Beta, PI3K/Akt and PTEN Pathways: Established and Proposed Biochemical Integration in Prostate Cancer. Biochem. J. 417 (2), 411–421. doi:10.1042/bj20081610
Baowen, Q., Yulin, Z., Xin, W., Wenjing, X., Hao, Z., Zhizhi, C., et al. (2010). A Further Investigation Concerning Correlation between Anti-fibrotic Effect of Liposomal Quercetin and Inflammatory Cytokines in Pulmonary Fibrosis. Eur. J. Pharmacol. 642 (1-3), 134–139. doi:10.1016/j.ejphar.2010.05.019
Blaser, H., Dostert, C., Mak, T. W., and Brenner, D. (2016). TNF and ROS Crosstalk in Inflammation. Trends Cel Biol. 26 (4), 249–261. doi:10.1016/j.tcb.2015.12.002
Chen, J., Wang, W., Shi, C., and Fang, J. (2014). A Comparative Study of Sodium Houttuyfonate and 2-undecanone for Their In Vitro and In Vivo Anti-inflammatory Activities and Stabilities. Int. J. Mol. Sci. 15 (12), 22978–22994. doi:10.3390/ijms151222978
Cui, Y., Jiang, L., Yu, R., Shao, Y., Mei, L., and Tao, Y. (2019). β-Carboline Alkaloids Attenuate Bleomycin Induced Pulmonary Fibrosis in Mice through Inhibiting NF-Kb/p65 Phosphorylation and Epithelial-Mesenchymal Transition. J. Ethnopharmacol 243, 112096. doi:10.1016/j.jep.2019.112096
Deng, Z. P., Zhong, D. F., Meng, J., and Chen, X. Y. (2012). Covalent Protein Binding and Tissue Distribution of Houttuynin in Rats after Intravenous Administration of Sodium Houttuyfonate. Acta Pharmacol. Sin. 33 (4), 568–576. doi:10.1038/aps.2011.174
Dong, W. W., Zhang, Y. Q., Zhu, X. Y., Mao, Y. F., Sun, X. J., Liu, Y. J., et al. (2017). Protective Effects of Hydrogen-Rich Saline against Lipopolysaccharide-Induced Alveolar Epithelial-To-Mesenchymal Transition and Pulmonary Fibrosis. Med. Sci. Monit. 23, 2357–2364. doi:10.12659/msm.900452
Du, S., Li, H., Cui, Y., Yang, L., Wu, J., Huang, H., et al. (2012). Houttuynia Cordata Inhibits Lipopolysaccharide-Induced Rapid Pulmonary Fibrosis by Up-Regulating IFN-γ and Inhibiting the TGF-β1/Smad Pathway. Int. Immunopharmacol 13 (3), 331–340. doi:10.1016/j.intimp.2012.03.011
George, P. M., Wells, A. U., and Jenkins, R. G. (2020). Pulmonary Fibrosis and COVID-19: the Potential Role for Antifibrotic Therapy. Lancet Respir. Med. 8 (8), 807–815. doi:10.1016/s2213-2600(20)30225-3
Grände, M., Franzen, A., Karlsson, J. O., Ericson, L. E., Heldin, N. E., and Nilsson, M. (2002). Transforming Growth Factor-Beta and Epidermal Growth Factor Synergistically Stimulate Epithelial to Mesenchymal Transition (EMT) through a MEK-dependent Mechanism in Primary Cultured Pig Thyrocytes. J. Cel Sci. 115 (Pt 22), 4227–4236. doi:10.1242/jcs.00091
Guo, H., Zhang, Z., Su, Z., Sun, C., Zhang, X., Zhao, X., et al. (2016). Enhanced Anti-tumor Activity and Reduced Toxicity by Combination Andrographolide and Bleomycin in Ascitic Tumor-Bearing Mice. Eur. J. Pharmacol. 776, 52–63. doi:10.1016/j.ejphar.2016.02.032
He, H., Tang, H., Gao, L., Wu, Y., Feng, Z., Lin, H., et al. (2015). Tanshinone IIA Attenuates Bleomycin-Induced Pulmonary Fibrosis in Rats. Mol. Med. Rep. 11 (6), 4190–4196. doi:10.3892/mmr.2015.3333
Hohmann, M. S., Habiel, D. M., Coelho, A. L., Verri, W. A., and Hogaboam, C. M. (2019). Quercetin Enhances Ligand-Induced Apoptosis in Senescent Idiopathic Pulmonary Fibrosis Fibroblasts and Reduces Lung Fibrosis In Vivo. Am. J. Respir. Cel Mol Biol. 60 (1), 28–40. doi:10.1165/rcmb.2017-0289OC
Lau, K. M., Lee, K. M., Koon, C. M., Cheung, C. S., Lau, C. P., Ho, H. M., et al. (2008). Immunomodulatory and Anti-SARS Activities of Houttuynia Cordata. J. Ethnopharmacol. 118 (1), 79–85. doi:10.1016/j.jep.2008.03.018
Lou, Y., Guo, Z., Zhu, Y., Kong, M., Zhang, R., Lu, L., et al. (2019). Houttuynia Cordata Thunb. And its Bioactive Compound 2-undecanone Significantly Suppress Benzo(a)pyrene-Induced Lung Tumorigenesis by Activating the Nrf2-HO-1/nqo-1 Signaling Pathway. J. Exp. Clin. Cancer Res. 38 (1), 242. doi:10.1186/s13046-019-1255-3
Lu, Y., Azad, N., Wang, L., Iyer, A. K., Castranova, V., Jiang, B. H., et al. (2010). Phosphatidylinositol-3-kinase/akt Regulates Bleomycin-Induced Fibroblast Proliferation and Collagen Production. Am. J. Respir. Cel Mol Biol. 42 (4), 432–441. doi:10.1165/rcmb.2009-0002OC
Meyer, K. C. (2017). Pulmonary Fibrosis, Part I: Epidemiology, Pathogenesis, and Diagnosis. Expert Rev. Respir. Med. 11 (5), 343–359. doi:10.1080/17476348.2017.1312346
Ng, L. T., Yen, F. L., Liao, C. W., and Lin, C. C. (2007). Protective Effect of Houttuynia Cordata Extract on Bleomycin-Induced Pulmonary Fibrosis in Rats. Am. J. Chin. Med. 35 (3), 465–475. doi:10.1142/s0192415x07004989
Pan, P., Wang, Y. J., Han, L., Liu, X., Zhao, M., and Yuan, Y. F. (2010). Effects of Sodium Houttuyfonate on Expression of NF-Κb and MCP-1 in Membranous Glomerulonephritis. J. Ethnopharmacol 131 (1), 203–209. doi:10.1016/j.jep.2010.06.020
Sasaki, M., Kashima, M., Ito, T., Watanabe, A., Izumiyama, N., Sano, M., et al. (2000). Differential Regulation of Metalloproteinase Production, Proliferation and Chemotaxis of Human Lung Fibroblasts by PDGF, Interleukin-1beta and TNF-Alpha. Mediators Inflamm. 9 (3-4), 155–160. doi:10.1080/09629350020002895
Shen, Y. H., Cheng, M. H., Liu, X. Y., Zhu, D. W., and Gao, J. (2021). Sodium Houttuyfonate Inhibits Bleomycin Induced Pulmonary Fibrosis in Mice. Front. Pharmacol. 12, 596492. doi:10.3389/fphar.2021.596492
Sun, L., Mao, M., Yan, Z., Zuo, C., and Zhang, X. (2018). A Chinese Traditional Therapy for Bleomycin-Induced Pulmonary Fibrosis in Mice. Can. Respir. J. 2018, 8491487. doi:10.1155/2018/8491487
Veith, C., Drent, M., Bast, A., van Schooten, F. J., and Boots, A. W. (2017). The Disturbed Redox-Balance in Pulmonary Fibrosis Is Modulated by the Plant Flavonoid Quercetin. Toxicol. Appl. Pharmacol. 336, 40–48. doi:10.1016/j.taap.2017.10.001
Wang, C., Song, X., Li, Y., Han, F., Gao, S., Wang, X., et al. (2013). Low-dose Paclitaxel Ameliorates Pulmonary Fibrosis by Suppressing TGF-β1/Smad3 Pathway via miR-140 Upregulation. PLoS One 8 (8), e70725. doi:10.1371/journal.pone.0070725
Wynn, T. A., and Vannella, K. M. (2016). Macrophages in Tissue Repair, Regeneration, and Fibrosis. Immunity 44 (3), 450–462. doi:10.1016/j.immuni.2016.02.015
Xiao, K., He, W., Guan, W., Hou, F., Yan, P., Xu, J., et al. (2020). Mesenchymal Stem Cells Reverse EMT Process through Blocking the Activation of NF-Κb and Hedgehog Pathways in LPS-Induced Acute Lung Injury. Cell Death Dis. 11 (10), 863. doi:10.1038/s41419-020-03034-3
Xiao-lei, H., and Xiao-yin, C. (2013). Efficient Extraction of Flavonoids from Houttuynia Cordata. Chin. Med. Mod. Distance Edu. China 11 (15), 161–162. doi:10.3969/j.issn.1672-2779.2013.15.108
Xu, S. W., Howat, S. L., Renzoni, E. A., Holmes, A., Pearson, J. D., Dashwood, M. R., et al. (2004). Endothelin-1 Induces Expression of Matrix-Associated Genes in Lung Fibroblasts through MEK/ERK. J. Biol. Chem. 279 (22), 23098–23103. doi:10.1074/jbc.M311430200
Yu, M. X., Song, X., Ma, X. Q., Hao, C. X., Huang, J. J., and Yang, W. H. (2021). Investigation into Molecular Mechanisms and High-Frequency Core TCM for Pulmonary Fibrosis Secondary to COVID-19 Based on Network Pharmacology and Data Mining. Ann. Palliat. Med. 10 (4), 3960–3975. doi:10.21037/apm-20-1384
Keywords: pulmonary fibrosis, Houttuynia cordata Thunb., molecular mechanism, network pharmacology, signaling pathway analysis
Citation: Zhu D-W, Yu Q, Sun J-J and Shen Y-H (2021) Evaluating the Therapeutic Mechanisms of Selected Active Compounds in Houttuynia cordata Thunb. in Pulmonary Fibrosis via Network Pharmacology Analysis. Front. Pharmacol. 12:733618. doi: 10.3389/fphar.2021.733618
Received: 30 June 2021; Accepted: 17 August 2021;
Published: 30 September 2021.
Edited by:
Tao Xu, Anhui Medical University, ChinaReviewed by:
Bin Li, Shanghai Jiao Tong University School of Medicine, ChinaLi Xiu Fang, Yunnan University of Traditional Chinese Medicine, China
Hong Nie, Jinan University, China
Copyright © 2021 Zhu, Yu, Sun and Shen. This is an open-access article distributed under the terms of the Creative Commons Attribution License (CC BY). The use, distribution or reproduction in other forums is permitted, provided the original author(s) and the copyright owner(s) are credited and that the original publication in this journal is cited, in accordance with accepted academic practice. No use, distribution or reproduction is permitted which does not comply with these terms.
*Correspondence: Yun-Hui Shen, bravesyh@163.com
†These authors have contributed equally to this work