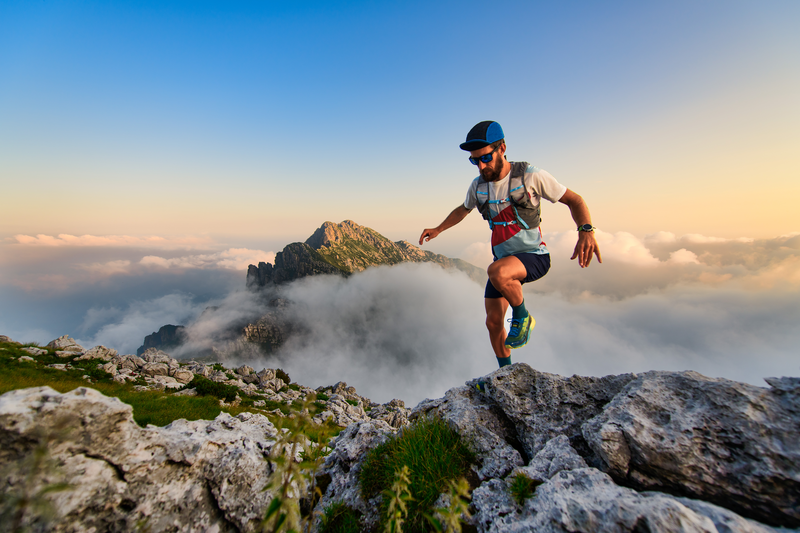
94% of researchers rate our articles as excellent or good
Learn more about the work of our research integrity team to safeguard the quality of each article we publish.
Find out more
REVIEW article
Front. Pharmacol. , 05 July 2021
Sec. Gastrointestinal and Hepatic Pharmacology
Volume 12 - 2021 | https://doi.org/10.3389/fphar.2021.694475
This article is part of the Research Topic Alcohol Consumption and Liver Diseases: from Pathology to Phytotherapy View all 9 articles
Excessive alcohol intake is a direct cause of alcoholic liver disease (ALD). ALD usually manifests as fatty liver in the initial stage and then develops into alcoholic hepatitis (ASH), fibrosis and cirrhosis. Severe alcoholism induces extensive hepatocyte death, liver failure, and even hepatocellular carcinoma (HCC). Currently, there are few effective clinical means to treat ALD, except for abstinence. Natural compounds are a class of compounds extracted from herbs with an explicit chemical structure. Several natural compounds, such as silymarin, quercetin, hesperidin, and berberine, have been shown to have curative effects on ALD without side effects. In this review, we pay particular attention to natural compounds and developing clinical drugs based on natural compounds for ALD, with the aim of providing a potential treatment for ALD.
Alcohol liver disease (ALD), a liver disease caused by long-term heavy alcohol intake, is the leading cause of chronic liver disease (CLD), especially in Europe and America (Hosseini et al., 2019). With economic development, the average daily alcohol intake of people in developing areas is gradually increasing, accompanied by an increasing ALD prevalence (Mukherjee et al., 2017; Wang et al., 2019; Mitra et al., 2020). Alcoholism causes nearly 2.5 million deaths annually, accounting for 4% of all deaths. Most mortality from alcoholism is caused by ALD (Jaurigue and Cappell, 2014). ALD is gradually becoming a worldwide public health problem.
Few specific medicines for ALD treatment exist. The development of effective and safer ALD treatments is an unmet clinical need (Singal et al., 2018). In China, herbs have been widely used to treat diseases for more than a thousand years. However, because of their complex composition, herbs cannot be used globally. Natural compounds in herbs are active ingredients with defined chemical compositions, and their curative effect has progressively been recognised (Zeng H. et al., 2019). Currently, various studies have verified the efficacy of numerous natural compounds on ALD. This review attempts to summarise and discuss the current research on ALD treatment with natural compounds, promoting the use of herbs in treating ALD.
ALD is always accompanied by hepatic steatosis induced by the imbalance of lipid metabolism originating from excess alcohol (Figure 1). CD36/FA transportase (FAT), a vital fatty acid (FA) transporter, plays a crucial role in FA uptake: upregulating the expression of CD36/FAT will promote the uptake of FAs by the liver (Febbraio et al., 2001; He et al., 2011). Animal experiments found that sustained alcohol intake upregulated the expression of CD36/FAT, causing excessive intake of FAs in mice and rats and resulting in hepatic steatosis (Ronis et al., 2011; Clugston et al., 2014). Very low-density lipoprotein (VLDL), the main form of endogenous triglyceride (TG) transport, contributes to the release of lipids in the liver. Excess alcohol intake will inhibit apolipoprotein B (ApoB) and VLDL synthesis, resulting in the inability to smoothly release hepatic lipids, resulting in steatosis (Tomita et al., 2004). Acetaldehyde, the intermediate product of alcohol, can also cause a decrease in VLDL synthesis by destroying the structure and function of Golgi apparatus, which in turn leads to lipid accumulation in the liver.
FIGURE 1. Mechanisms of disordered lipid metabolism on Alcoholic fatty liver. The abnormal accumulation of hepatic lipids caused by alcohol is mainly due to disorders of lipids transport and lipids synthesis and oxidation. Periodical alcohol intake upregulates CD36/FAT expression, leading to excessive intake of lipids, while inhibiting the synthesis of ApoB and VLDL, leading to the disorder of lipids excretion. Excessive alcohol intake increases the ratio of NADH/NAD+, which in turn inhibits β-oxidation of lipids. Alcohol can also promote lipids synthesis by up-regulating the expression of SREBP-1c.
Early studies also showed that excessive intake of alcohol increases the ratio of reduced nicotinamide adenine dinucleotide (NADH)/oxidised nicotinamide adenine dinucleotide (NAD+) in hepatocytes, which in turn inhibits the tricarboxylic acid (TCA) cycle, interferes with β-oxidation of FAs, and results in excessive accumulation of FAs (You and Arteel, 2019). Simultaneously, the increase in NADH directly promotes the synthesis of FAs so that excessive lipids accumulate in hepatocytes. Alcohol also inhibits β-oxidation of FAs in hepatocytes by inhibiting peroxisome proliferator-activated receptor α (PPARα) (Wagner et al., 2011).
Additionally, cytochrome P450 2E1 (CYP2E1), an enzyme found in both the endoplasmic reticulum (ER) and mitochondria of hepatocytes, could metabolise alcohol to acetaldehyde in the presence of oxygen (Lieber 2004). Acetaldehyde directly upregulates the expression of sterol regulatory element-binding protein-1c (SREBP-1c) to promote TG synthesis (You et al., 2002). Ji et al. found that destroying SREBP-1c in mice improved alcohol-induced fatty liver, thus confirming the critical role of SREBP-1c in the pathogenesis of ALD (Ji et al., 2006). Alcohol also directly upregulates SREBP-1c expression to cause liver steatosis by inhibiting genes regulating SREBP-1c, such as Sirtuin1 (Sirt1), adiponectin, and signal transducer and activator of transcription 3 (STAT3) (Horiguchi et al., 2008; You et al., 2008; You and Rogers, 2009).
The liver, a vital organ for alcohol metabolism, can be damaged by by-products of alcohol decomposition, such as reactive oxygen species (ROS) (D'Autréaux and Toledano, 2007). CYP2E1 metabolises alcohol and produces ROS, which is closely associated with ALD development. Butura et al. found that the expression of oxidative stress-related genes and alcohol-induced hepatic injuries in mice were increased in the presence of the CYP2E1 transgene (Butura et al., 2009). Upregulation of CYP2E1 expression by adenovirus-mediated gene transfer in mice also promoted oxidative stress and liver damage (Bai and Cederbaum, 2006). By constructing a HepG2 cell line overexpressing CYP2E1, Wu et al. proved that CYP2E1 could inhibit the expression of antioxidant genes, such as glutathione (GSH), catalase (CAT), microsomal glutathione transferase 1 (MGST1), and α-glutathione S-transferase (α-GST), and facilitate alcohol to induce oxidative stress (Wu and Cederbaum, 2005).
Acetaldehyde is linked to the pathway by which alcohol causes oxidative stress in the liver. Acetaldehyde is highly reactive and toxic, and causes structural and functional damages to organelles such as mitochondria (Seitz and Stickel, 2007). The functional impairment of mitochondria will decrease ATP produced by the respiratory chain and increase ROS generation (O'Shea et al., 2010a). Overproduction of ROS causes oxidative stress, therefore leading liver damage (Cichoż-Lach and Michalak, 2014).
Alcohol-induced sensitisation of Kupffer cells (KCs) to lipopolysaccharide (LPS) is considered a hallmark of inflammation and ALD. The level of circulating LPS in ALD patients is significantly elevated and increases with disease severity (Fujimoto et al., 2000). The secretion of pro-inflammatory cytokines, such as chemokines (IL-8 and CCL2), interleukins (IL-1β and IL-6), and tumour necrosis factor (TNF), will be activated after excess LPS is recognised by Toll-like receptor 4 (TLR4), the vital LPS receptor (McClain and Cohen, 1989; Massey and Arteel, 2012; Roy et al., 2015). The above pro-inflammatory cytokines subsequently cause inflammation and hepatic injury. TNF, for instance, is a crucial mediator of alcohol-induced hepatic damage (Ajakaiye et al., 2011). The upregulation of TNF causes liver inflammation and inhibits FA β-oxidation (Mandrekar and Szabo, 2009). Continuous intake of excessive alcohol may promote TLR4 in KCs to recognise LPS, leading to the secretion of pro-inflammatory cytokines and further liver inflammation and ALD development.
The polarisation of KCs is also essential for the progression of inflammation (Xu et al., 2013; Zeng et al., 2016). Polarised KCs can be classified into two phenotypes, M1 and M2. M1 macrophages are inflammatory. They are always polarised by LPS alone or in the presence of Th1 cytokines (such as IFN-γ and GM-CSF), and they secrete pro-inflammatory cytokines such as IL-1β, IL-6, IL-12, IL-23, and TNF-α. M2 macrophages polarised by Th2 cytokines such as IL-4 and IL-13 have anti-inflammatory effects and produce anti-inflammatory cytokines such as IL-10 and TGF-β (Shapouri-Moghaddam et al., 2018). Promoting the polarisation of anti-inflammatory M2 KCs will effectively prevent alcohol-induced steatosis and apoptosis of hepatocytes (Louvet et al., 2011). Wan et al. showed that the expression of M2-related genes (CD206 and CD163) in the liver of ALD patients with excessive alcohol intake was significantly reduced. After treatment, BALB/c mice with ALD showed superior M2 KC polarisation. Experimental results indicated that alcohol causes inflammation and ALD by inhibiting the preferential polarisation of KCs to M2. M2-induced apoptosis of M1 KCs may be a novel strategy to improve alcohol-induced inflammatory hepatic damage (Wan et al., 2014).
Autophagy, a cellular self-protection program, is responsible for maintaining cell homeostasis by routing cytoplasmic macromolecules or damaged organelles to lysosomes for degradation (Ueno and Komatsu, 2017; Yu et al., 2018). Selective autophagy mediates the degradation of specific cargo, such as mitochondria and lipid droplets (LDs) for self-protection (Schulze et al., 2017; Gatica et al., 2018). Interestingly, alcohol has a two-way regulatory effect on autophagy (both activating and inhibitory). Administration of alcohol activates autophagy in primary cultured mouse hepatocytes and hepatoma cells that express ADH and CYP2E1, as well as in the liver of acute alcohol binge-treated mice and mitigates alcohol-induced hepatotoxicity by spot clearing damaged mitochondria and accumulated LDs (Ding et al., 2010). A diet with a low alcohol content (accounting for 29% of the caloric intake) promotes autophagy in mice. Nevertheless, when the alcohol level is higher (accounting for 36% of the caloric intake), autophagic flux will be inhibited. Menk et al. found that long-term alcohol feeding in rats increased the protein level of p62 (Menk et al., 2018). Thomes et al. also proved that the expression of p62 protein in alcohol-fed mice increased along with decreased lysosomes and levels of autophagosome-lysosome fusion (Thomes et al., 2015). The above experiments have proven that autophagy is inhibited by excessive alcohol intake, which leads to lipid accumulation and severe oxidative stress and further accelerates alcoholic liver injury.
Apoptosis is the process by which cells actively end their lives to maintain organismal homeostasis. Apoptosis is an important pathological feature of ALD (Nagy et al., 2016). Alcohol can cause hepatocyte apoptosis by activating caspase-dependent/caspase-independent apoptotic pathways and ER stress. Petrasek et al. indicated that the STING-IRF3 pathway is a critical determinant of ALD, which links ER stress with hepatocyte apoptosis (Petrasek et al., 2013). Alcohol induces ER stress and triggers the binding of IRF3 to stimulator of interferon gene (STING) and subsequent activation of IRF3. Phosphorylated IRF3 activates the pro-apoptotic molecule B-cell lymphoma 2 (Bcl2)-associated X protein (Bax) and leads to hepatocyte apoptosis. The lack of STING will prevent alcohol-induced phosphorylation of IRF3 and subsequent hepatocyte apoptosis. Using loss- and gain-of-function mutations for transglutaminase 2 (TG2) and Sp1 transcription factor (Sp1) in vitro and in vivo, Tatsukawa et al. showed that TG2 is a vital cross-linking enzyme in alcohol-induced hepatocyte apoptosis (Tatsukawa et al., 2009). Compared with normal mice, TG2−/− mice showed significantly reduced hepatocyte apoptosis after alcohol administration. It has also been proven that in AS mice and patients with AS, TG2 translocates into the hepatocyte nucleus and inhibits the activity of Sp1, leading to the downregulation of hepatocyte growth factor receptor, cellular-mesenchymal epithelial transition factor (c-Met), and apoptosis (Tatsukawa and Kojima, 2010). The above-mentioned caspase-independent pro-apoptotic pathway is novel and deserves more attention.
The gut is the physiological habitat for various microbes, such as bacteria, archaea, fungi, yeast, and viruses, which carry more than three million unique genes (Guarner and Malagelada, 2003). Intestinal microbes are involved in the synthesis of amino acids, the catabolism of bile acids (BAs), the provision of energy, and intestinal barrier preservation and can better regulate alcohol-related hepatic damage, which is closely related to the development of ALD (Bajaj 2019). Researchers have regarded the intestinal microbiome as a viable target for ALD treatment (Sarin et al., 2019).
Alcohol abuse promotes the overgrowth of intestinal bacteria and causes changes in intestinal microbial composition (Bjørkhaug et al., 2019). Yan et al. constructed an ALD disease model by feeding C57/B6 mice intragastric ethanol for three weeks. The composition of intestinal microbes in ALD mice changed (Lactococcus, Pediococcus, Lactobacillus, Lactobacillales decreased, while Verrucomicrobia and Bacteroidetes significantly increased), and the expression of regenerating family member 3 gamma (Reg3γ) was inhibited. Prebiotic fructooligosaccharide (FOS) treatment effectively upregulated the expression of Reg3γ, reduced intestinal bacterial overgrowth, and improved hepatic inflammation in ALD mice (Yan et al., 2011). Antifungal treatment improves liver damage caused by alcohol. Mutlu et al. directly proved that transplantation of probiotic Lactobacillus strains could improve liver damage in ALD mice (Mutlu et al., 2009). Recent studies have also shown that changes in the composition and metabolites of intestinal microbes may affect brain function and behaviour (Hsiao et al., 2013). Changes in the intestinal microbes caused by alcohol may influence physical activity and cognitive ability, enhancing the tendency to drink, further causing a vicious cycle of alcoholic liver injury (Leclercq et al., 2019).
Intestine-liver axis is involved in the initiation of inflammation in ALD. Alcohol intake destroys the intestinal barrier and increases intestinal permeability, causing bacterial (such as LPS) translocation in ALD patients and ALD experimental models (Zhou and Zhong, 2017). Under normal circumstances, the level of LPS in blood is extremely low and does not cause significant responses. However, because alcohol change intestinal permeability, a large amount of LPS reaches the liver through the portal vein, activates the innate immune response, and causes inflammation, leading to ALD deterioration (Arab et al., 2018).
The possible mechanism linking intestinal microbes and intestine-liver axis to ALD is depicted in Figure 2.
FIGURE 2. Mechanisms of disordered intestinal microbes and intestine-liver axis on Alcoholic fatty liver. Excessive alcohol consumption alters the distribution of intestinal microbes and permeability. Changes in intestinal microbes directly cause hepatic inflammation, also indirectly aggravate inflammation by downregulating the expression of Reg3γ. Alterations in intestinal permeability leads to more LPS entering the liver via portal vein, activating innate immunity and aggravating hepatic inflammation.
Glucocorticoids are always used to treat severe alcoholic hepatitis (SAH) and acute alcoholic hepatitis (AAH). The potential therapeutic mechanism of glucocorticoids is to suppress inflammation by reducing immune and pro-inflammatory cytokine responses (Taïeb et al., 2000). However, glucocorticoids have many side effects. Long-term use will increase the risk of complications such as infections, gastrointestinal bleeding, and even kidney failure (Naveau et al., 2004). Moreover, the use of glucocorticoids has many contraindications, such as sepsis, hepatitis B, hepatorenal syndrome, etc. Therefore, its indication is relatively limited (Lucey et al., 2009).
Pentoxifylline (PTX) is an oral phosphodiesterase inhibitor that can suppress inflammation by reducing serum TNF-α levels (Doherty et al., 1991). PTX can be used for a short period in patients with AH when glucocorticoids are counter indicated (Akriviadis et al., 2000; O'Shea et al., 2010b). However, the study found that the efficacy of PTX alone or PTX combined with glucocorticoids in the treatment of AH was not better than that of glucocorticoids alone. The randomised clinical trial results of Whitfield, Kate et al. did not support that PTX has a positive effect on AH participants (Whitfield et al., 2009).
Metadoxine, a drug used to treat acute alcoholism, could accelerate ethanol excretion and lower the level of ethanol in the blood (Vonghia et al., 2008). However, Mao Yi-min et al. found that, among subjects who were treated with metadoxine, the total effective rate of liver function improvement in patients who quit drinking during the study period was better than that in patients who did not stop drinking (Mao et al., 2009). The results showed that metadoxine may improve liver function indirectly by improving alcohol excretion, but it cannot directly improve liver steatosis or inflammation.
PTX alone has poor efficacy and is always combined with glucocorticoids in treating acute alcoholic hepatitis or severe alcoholic hepatitis, which is the critical stage of ALD. However, glucocorticoids have significant side effects and are not suitable for long-term treatment. Metadoxine is commonly used clinically for acute alcoholism because it promotes alcohol excretion. However, all of them indirectly improved liver damage caused by long-term drinking by improving alcohol metabolism. Metadoxine is not a specific treatment for ALD per se.
Increasing evidence indicates that natural compounds can effectively inhibit ALD progression. Natural compounds have been demonstrated to be effective towards ALD by engaging in a variety of pathways, including improvement of lipid metabolism, suppression of oxidative stress and inflammation, and reduction of programmed cell death (PCD). Natural compounds affect cellular functions broadly with few side effects, and can be administered at different stages of ALD. Table 1 summarizes the effects and the mechanisms of action of 23 natural compounds on ALD from recent studies. Figure 3 shows the chemical structure of these natural compounds. We will introduce these natural compounds in the following sections according to their mechanisms of action.
Curcumin, the main active ingredient in turmeric, has been shown to reduce lipid accumulation in hepatocytes and subsequent steatosis by regulating the NF-E2–related factor 2 (Nrf2)-farnesoid X receptor (FXR) pathway (Lu et al., 2015). Some scholars also found that treatment with curcumin could suppress oxidative stress in ALD mice by reducing the generation of ROS (Rong et al., 2012).
Dihydroquercetin, one of the most common dihydroflavonoids, is widely found in milk thistles and onions. Zhang et al. proposed that dihydroquercetin improves alcoholic liver steatosis by regulating the activation of the SIRT1-AMPK pathway (Zhang et al., 2018a). Dihydroquercetin increased the activity of AMPK, thereby reducing the expression of SREBP1 in ethanol-treated HepG2 cells. Dihydroquercetin shows the ability to inhibit lipid formation and protect the liver, suggesting that dihydroquercetin has the potential as a treatment for ALD.
Tanshinone IIA is superabundant in the root of Salvia miltiorrhiza BUNGE. Yin et al. found that tanshinone IIA can inhibit the synthesis of FAs and stimulate the oxidation of FAs, improving ALD (Yin et al., 2008). Tanshinone IIA has a lipid-modulating effect and attenuates lipid accumulation by regulating LXRα/SREBP1 pathway in HepG2 Cells (Gao et al., 2021).
Silymarin, a flavonoid extracted from Silybum marianum, has been introduced as a hepatoprotective agent. Silymarin mainly repairs hepatic injury by alleviating lipid peroxidation and oxidative stress (Song et al., 2006; Tighe et al., 2020). Zhang, Wei et al. found that silymarin significantly increases the activities of superoxide dismutase (SOD) and glutathione peroxidase (GSH-Px) and reduces the level of malondialdehyde (MDA) in ALD rats, which are all closely related to the oxidative stress pathway (Zhang et al., 2013).
Isoorientin was confirmed to be abundant in buckwheat. In an animal experiment, Chen et al. discovered that isoorientin effectively increases the activities of the antioxidant SOD and GSH-Px and the expression of alpha-SMA, thereby suppressing alcohol-induced oxidative stress and protecting from liver fibrosis in Wistar rats (Chen X. et al., 2013). In addition, the antioxidant activity of isoorientin was compared with some antioxidants, including α-Tocopherol, ascorbic acid and other plant derived compounds. In short, most studies support isoorientin is a strong antioxidant (Watanabe 2007; Ziqubu et al., 2020).
Oleanolic acid exists abundantly in fruits, vegetables, and herbs. Oleanolic acid has been proven to have promising pharmacological activities, such as hepatoprotective effects and antioxidant activities (Tian et al., 2017; Lim et al., 2019). The antioxidant function of oleanolic acid may be related to the regulation of Nrf2 expression (Wang et al., 2013). Liu et al. found that oleanolic acid could protect rats against alcohol-induced hepatic damage by controlling Nrf2-related antioxidants to maintain redox balance (Liu et al., 2014).
Anthocyanins are a group of plant pigments widely present in flowers, fruits, and cereals (Kong et al., 2003). The health promotion effect of anthocyanins has received increasing attention. Jiang et al. found that, after anthocyanin treatments, the expression of IFN-γ, TNF-a, TLR-4, VCAM-1, and CXCL-1 significantly decreased in ALD mice, suggesting that anthocyanin may suppress inflammation by downregulating proinflammatory cytokines (Jiang et al., 2016).
Baicalin, an active ingredient extracted from Scutellaria baicalensis, is widely used in herbal preparations to treat ALD and inflammation. According to recent studies, baicalin plays a beneficial role in various invasive toxicant models by inhibiting inflammatory responses (Lixuan et al., 2010; Guo et al., 2019). Additionally, Kim et al. directly confirmed that baicalin could reduce hepatocyte damage by inhibiting the TLR4-mediated inflammatory pathway in ALD rats (Kim and Lee, 2012).
Puerarin and sophoronol both increase the activity of ADH and ALDH, thereby promoting alcohol metabolism and reducing hepatotoxic damage caused by alcohol (Choi et al., 2009; Chen YX. et al., 2013). In addition, studies have shown that puerarin is able to treat ALD by inhibiting the expression of TNF-α, cyclooxygenase-2 (Cox-2), and 5-lipoxygenase (5-Lox) and other pro-inflammatory cytokines (Li et al., 2013).
Catechin, a polyphenol antioxidant, inhibits activation of NF-κB in rats upon excessive alcohol uptake and prevents necrotizing inflammatory changes (Bharrhan et al., 2011).
Corosolic acid, also known as 2α-hydroxyursolic acid, is the main active ingredient found in banaba leaves. Corosolic acid has attracted considerable attention due to its anti-diabetic effect and was called “Phyto-insulin” (Sivakumar et al., 2009). As a potential activator of AMPK, corosolic acid can restore ethanol-suppressed autophagy via AMPK activation in vivo and in vitro (Guo et al., 2016).
Gastrodin is one of the main bioactive components of Gastrodia elata, an ancient Chinese medicinal plant, geranium. Zhang et al. showed that gastrodin significantly inhibited caspase-3 (CASP3) activation and apoptosis from ethanol-induced toxicity in vivo and in vitro (Zhang et al., 2018b).
Ursolic acid is a pentacyclic terpenoid carboxylic acid with many health functions, such as anti-oxidative, anti-inflammatory, anti-cancer, and hepato-protective activities (Jinhua, 2019). Ma et al. directly confirmed that ursolic acid reduced hepatocellular apoptosis and alleviated alcohol-induced liver injury via inhibition of CASP3 in vivo and in vitro (Ma et al., 2020).
Berberine, a quaternary ammonia compound derived from many herbs, has been widely used to treat hepatic injury. Li et al. found that berberine increased the abundance of the bacteria Akkermansia muciniphila and changed the overall gut microbial flora in ALD mice, helping maintain metabolic balance and reduce inflammation (Zhang et al., 2019; Li et al., 2020). Interestingly, berberine also activates a population of granulocytic myeloid-derived suppressor cell (G-MDSC)-like cells with an immune suppressive function in the liver of mice, thus alleviating alcohol-induced hepatic injury.
Naringenin, the main flavanone in pomelo, could effectively improve oxidative stress and inflammation, decrease hepatic damage. Lin et al. have further confirmed that it can treat the ALD of zebrafish larvae by enhancing oxidative stress and lipid metabolism and attenuating the hepatocyte apoptosis (Lin et al., 2017).
Naringin is a flavanone rich in pomelo. The administration of naringin effectively suppressed the production of pro-inflammatory cytokines in ALD mice (Park et al., 2013). In addition, naringin also attenuated hepatic injury in zebrafish with ALD by reducing lipid accumulation and oxidative stress (Zhou et al., 2019).
Hesperidin, a flavonoid compound with pharmacological activity, is widely found in citrus peels (Hemanth Kumar et al., 2017). Various experiments have shown that hesperidin can reduce oxidative stress and inflammation and treat hyperglycaemia and hyperlipidaemia with few side effects (Roohbakhsh et al., 2015; Li and Schluesener, 2017). Recently, some researchers have confirmed its efficacy in the treatment of ALD. Zhou et al. demonstrated that hesperidin could inhibit the accumulation of hepatic lipids by regulating the expression of lipid metabolism-related genes, thus protecting zebrafish larvae from steatosis after alcoholic exposure (Zhou et al., 2017).
Quercetin, commonly present in fruits and vegetables, alleviates oxidative stress, glutathione depletion, and pro-inflammatory cytokine production in ALD (Lee et al., 2019). Zeng et al. proved that quercetin activated AMPK and increased the colocalisation of hepatic LC3II and Perilipin 2 (PLIN2) to promote lipophagy, thereby improving the steatosis of mice with ALD (Zeng H. et al., 2019). Zhao et al. also confirmed that quercetin directly mitigates alcohol-induced hepatic steatosis in zebrafish via the regulation of the PI3K/Keap1/Nrf2 pathway (Zhao et al., 2021).
Dihydromyricetin, a bioflavonoid isolated from Hovenia dulcis, has been proven to improve hepatic lipid metabolism by reducing the NADPH/NAD + ratio (Silva et al., 2020). Silva et al. suggest that treatment with dihydromyricetin could repair the mitochondrial functions damaged by acetaldehyde toxicity and decrease the generation of ROS by controlling the AMPK/Sirt-1/PGC-1α pathway, thereby suppressing oxidative stress and improving the corresponding ALD (Silva et al., 2021).
Glycycoumarin, a representative coumarin in liquorice, has good bioavailability in vivo. Liquorice is a popular edible and medicinal plant widely used to treat various diseases, including liver diseases and inflammatory diseases (Li et al., 2019). It has been shown that glycycoumarin has a strong preventive effect on chronic and acute alcoholic liver injury in mice. Further mechanistic studies showed that the hepato-protective effect of glycycoumarin could be attributed to the activation of Nrf2 and autophagy (Song et al., 2015).
Lycopene is rich in tomatoes, watermelon, pink grapefruit, papaya, apricot, and guava (Crowe-White et al., 2019). Lycopene is best-known for its antioxidant potential but has many other functions, including regulating the immune system and metabolism (Wang, 2012). Lycopene increased the richness and diversity of intestinal bacteria, indicating that lycopene has a protective effect on intestinal microorganisms (Li et al., 2018). Stice et al. confirmed that lycopene could reduce oxidative stress by regulating CYP2E1 (Stice et al., 2018).
Limonoids are natural tetracyclic triterpenoids with a variety of biological properties (Fan et al., 2019). Previous studies have indicated anti-cancer, anti-viral, and hepato-protective activities of limonoids. Valansa et al. showed that limonin had a protective effect on ALD mainly through its antioxidant and anti-inflammatory properties (Valansa et al., 2020).
Overall, the toxicity and side effects of these natural compounds are low, but more clinical trials are needed. In addition, although natural compounds are easy to obtain and functions through multiple pathways in the treatment of ALD, some natural compounds, such as curcumin and silymarin, are easily eliminated from the body due to reduced bioavailability and fast metabolism. Thus, more sophisticated research is required to improve their pharmacokinetics. It is crucial to optimise the chemical structure of natural compounds and develop drugs with high bioavailability, such as curcumin phospholipid complexes.
Despite much evidence supporting the effects of natural compounds on ALD, clinical trials are still limited and large-scale human studies may be needed to confirm these effects. In a clinical trial, Sasaki et al. found that Theracurmin, a highly absorptive curcumin dispersed with colloidal nanoparticles, could significantly decrease the concentration of acetaldehyde in the blood and reduce alcohol intoxication (Sasaki et al., 2011).
Silymarin consists of several ingredients such as silybin and silydianin, and silybin is the main active ingredient in silymarin, accounting for approximately 60% (Ha et al., 2019). Clinical studies have shown that silymarin can significantly reduce the levels of ALT, AST, and ALP in patients with ALD, and its effect is considerably superior to that of traditional hepato-protective agents (Saller et al., 2001; Saller et al., 2008). The results of combinatorial treatment with lamivudine in ALD patients with chronic hepatitis B showed that Silybum thistle could significantly reduce the levels of hyaluronic acid (HA), laminin (LN), type III collagen (PⅢP), and collagen IV (IVC), effectively improve liver functions and inhibit the development of hepatic fibrosis. Silymarin and its related nutrients or drugs have long been approved by the US Food and Drug Administration (FDA). Silymarin and its related herbal supplements are currently widely used in the clinic as liver protective agents characterised by their apparent hepato-protective effects and limited toxicity and side effects (Beyoğlu and Idle, 2020). Nearly a quarter of patients with liver diseases in the United States have been treated with silymarin and its related agents.
Among the 23 natural compounds mentioned above, silymarin is one of the few compounds commonly used in clinical practice. In contrast, other natural compounds have not been validated by clinical trials. Therefore, further in-depth studies are needed.
Natural compounds play a vital role in protecting human health and curing human diseases. Many drugs currently used in clinical practice are directly derived from or inspired by natural compounds. From the 1940s to 2010, 65% of anti-bacterial small molecules and 41% of anti-cancer small molecule drugs were derived from natural compounds and derivatives. Natural compounds are still a treasure trove of drug development and are the backbone of the modern drug development process.
ALD is a type of hepatic damage caused by excessive alcohol intake. The mechanism of liver injury caused by alcohol is complex and mainly involves the imbalance of lipid metabolism, liver inflammation, promotion of oxidative stress, and changes in the distribution of intestinal microbes. In the review, we summarised the current knowledge on 23 natural compounds whose efficacy against ALD has been confirmed through clinical studies or animal experiments. Compared with glucocorticoids and PTX, which are commonly used to treat AAH and SAH, natural compounds have the advantages of low toxicity and a broad range of applications. Among them, silymarin, hesperidin, curcumin, etc., have been proven to have excellent hepato-protective effects, are suitable treatment options by themselves or in combination with other drugs as a supplement.
However, natural compounds are not perfect, and they have obvious drawbacks. 1) The clinical efficacy of most natural compounds is limited due to their low bioavailability. 2) It is difficult to define the most appropriate drug form and dosage without standardised pharmaceutical technology. Thus, the optimal liver protection effect cannot be realised. 3) Most compounds have not been subjected to double-blind, placebo-controlled clinical trials to evaluate their efficacy and mechanisms of action of natural compounds are still unclear because of scarce studies.
Undoubtedly, natural compounds have enormous potentials to be developed as therapeutic drugs for ALD. However, in the field of ALD research, clinical trials are still needed for systematic reviews. Further research is needed on the potential role of natural compounds (Figure 4). We hope the review can provide theoretical support for the research on the mechanisms of ALD pathogenesis and progression, and subsequent therapeutic drug development for ALD.
JY, YN, ZC, and BH participated in drafting the manuscript. JY, YN, and ML provided technical assistance. ZC and BH revised the manuscript. All of the authors read and approved the final manuscript.
This study was supported by the Zhejiang Provincial Natural Science Foundation of China (LY17H290007), the Fund of State Administration of Traditional Chinese Medicine of Zhejiang Province (2020ZB081), the Fund of Health Commission of Zhejiang Province (2018KY550), Research fund project of Zhejiang Chinese Medical University (2019ZG03).
The authors declare that the research was conducted in the absence of any commercial or financial relationships that could be construed as a potential conflict of interest.
Ajakaiye, M. A., Jacob, A., Wu, R., Zhou, M., Ji, Y., Dong, W., et al. (2011). Upregulation of Kupffer Cell α2A-Adrenoceptors and Downregulation of MKP-1 Mediate Hepatic Injury in Chronic Alcohol Exposure. Biochem. Biophysical Res. Commun. 409, 406–411. doi:10.1016/j.bbrc.2011.05.007
Akriviadis, E., Botla, R., Briggs, W., Han, S., Reynolds, T., and Shakil, O. (2000). Pentoxifylline Improves Short-Term Survival in Severe Acute Alcoholic Hepatitis: A Double-Blind, Placebo-Controlled Trial. Gastroenterology 119, 1637–1648. doi:10.1053/gast.2000.20189
Arab, J. P., Martin-Mateos, R. M., and Shah, V. H. (2018). Gut-liver axis, Cirrhosis and portal Hypertension: The Chicken and the Egg. Hepatol. Int. 12, 24–33. doi:10.1007/s12072-017-9798-x
Bai, J., and Cederbaum, A. I. (2006). Adenovirus-mediated expression of cyp2e1 produces liver toxicity in mice. Toxicol. Sci. 91, 365–371. doi:10.1093/toxsci/kfj165
Bajaj, J. S. (2019). Alcohol, Liver Disease and the Gut Microbiota. Nat. Rev. Gastroenterol. Hepatol. 16, 235–246. doi:10.1038/s41575-018-0099-1
Beyoğlu, D., and Idle, J. R. (2020). Metabolomic Insights into the Mode of Action of Natural Products in the Treatment of Liver Disease. Biochem. Pharmacol. 180, 114171. doi:10.1016/j.bcp.2020.114171
Bharrhan, S., Koul, A., Chopra, K., and Rishi, P. (2011). Catechin Suppresses an Array of Signalling Molecules and Modulates Alcohol-Induced Endotoxin Mediated Liver Injury in a Rat Model. Plos. One. 6, e20635. doi:10.1371/journal.pone.0020635
Bjørkhaug, S. T., Aanes, H., Neupane, S. P., Bramness, J. G., Malvik, S., Henriksen, C., et al. (2019). Characterization of Gut Microbiota Composition and Functions in Patients with Chronic Alcohol Overconsumption. Gut. Microbes 10, 663–675. doi:10.1080/19490976.2019.1580097
Butura, A., Nilsson, K., Morgan, K., Morgan, T. R., French, S. W., Johansson, I., et al. (2009). The impact of cyp2e1 on the development of alcoholic liver disease as studied in a transgenic mouse model. J. Hepatol. 50, 572–583. doi:10.1016/j.jhep.2008.10.020
Chen, X., Li, R., Liang, T., Zhang, K., Gao, Y., and Xu, L. (2013a). Puerarin Improves Metabolic Function Leading to Hepatoprotective Effects in Chronic Alcohol-Induced Liver Injury in Rats. Phytomedicine 20, 849–852. doi:10.1016/j.phymed.2013.04.001
Chen, Y. X., Huang, Q. F., Lin, X., and Wei, J. B. (2013b). [Protective Effect of Isoorientin on Alcohol-Induced Hepatic Fibrosis in Rats]. Zhongguo Zhong Yao Za Zhi. 38, 3726–3730. doi:10.4268/cjcmm20132124
Choi, E. J., Kwon, H. C., Sohn, Y. C., Nam, C. W., Park, H. B., Kim, C. Y., et al. (2009). Four Flavonoids from Echinosophora Koreensis and Their Effects on Alcohol Metabolizing Enzymes. Arch. Pharm. Res. 32, 851–855. doi:10.1007/s12272-009-1606-2
Cichoż-Lach, H., and Michalak, A. (2014). Oxidative Stress as a Crucial Factor in Liver Diseases. World J. Gastroenterol. 20, 8082–8091. doi:10.3748/wjg.v20.i25.8082
Clugston, R. D., Yuen, J. J., Hu, Y., Abumrad, N. A., Berk, P. D., Goldberg, I. J., et al. (2014). Cd36-deficient Mice Are Resistant to Alcohol- and High-Carbohydrate-Induced Hepatic Steatosis. J. Lipid. Res. 55, 239–246. doi:10.1194/jlr.M041863
Crowe-White, K. M., Phillips, T. A., and Ellis, A. C. (2019). Lycopene and Cognitive Function. J. Nutr. Sci. 8, e20. doi:10.1017/jns.2019.16
D'Autréaux, B., and Toledano, M. B. (2007). Ros as Signalling Molecules: Mechanisms that Generate Specificity in Ros Homeostasis. Nat. Rev. Mol. Cel. Biol. 8, 813–824. doi:10.1038/nrm2256
Ding, W. X., Li, M., Chen, X., Ni, H. M., Lin, C. W., Gao, W., et al. (2010). Autophagy Reduces Acute Ethanol-Induced Hepatotoxicity and Steatosis in Mice. Gastroenterology 139, 1740–1752. doi:10.1053/j.gastro.2010.07.041
Doherty, G. M., Jensen, J. C., Alexander, H. R., Buresh, C. M., and Norton, J. A. (1991). Pentoxifylline Suppression of Tumor Necrosis Factor Gene Transcription. Surgery 110, 192–198.
Fan, S., Zhang, C., Luo, T., Wang, J., Tang, Y., Chen, Z., et al. (2019). Limonin: A Review of its Pharmacology, Toxicity, and Pharmacokinetics. Molecules 24, 3679. doi:10.3390/molecules24203679
Febbraio, M., Hajjar, D. P., and Silverstein, R. L. (2001). Cd36: A Class B Scavenger Receptor Involved in Angiogenesis, Atherosclerosis, Inflammation, and Lipid Metabolism. J. Clin. Invest. 108, 785–791. doi:10.1172/jci14006
Fujimoto, M., Uemura, M., Nakatani, Y., Tsujita, S., Hoppo, K., Tamagawa, T., et al. (2000). Plasma Endotoxin and Serum Cytokine Levels in Patients with Alcoholic Hepatitis: Relation to Severity of Liver Disturbance. Alcohol. Clin. Exp. Res. 24, 48s–54s. doi:10.1111/j.1530-0277.2000.tb00012.x
Gao, W. Y., Chen, P. Y., Hsu, H. J., Lin, C. Y., Wu, M. J., and Yen, J. H. (2021). Tanshinone IIA Downregulates Lipogenic Gene Expression and Attenuates Lipid Accumulation through the Modulation of LXRα/SREBP1 Pathway in HepG2 Cells. Biomedicines 9. doi:10.3390/biomedicines9030326
Gatica, D., Lahiri, V., and Klionsky, D. J. (2018). Cargo Recognition and Degradation by Selective Autophagy. Nat. Cel. Biol. 20, 233–242. doi:10.1038/s41556-018-0037-z
Guarner, F., and Malagelada, J. R. (2003). Gut flora in Health and Disease. Lancet 361, 512–519. doi:10.1016/s0140-6736(03)12489-0
Guo, L. T., Wang, S. Q., Su, J., Xu, L. X., Ji, Z. Y., Zhang, R. Y., et al. (2019). Baicalin Ameliorates Neuroinflammation-Induced Depressive-like Behavior through Inhibition of Toll-like Receptor 4 Expression via the Pi3k/akt/foxo1 Pathway. J. Neuroinflammation. 16, 95. doi:10.1186/s12974-019-1474-8
Guo, X., Cui, R., Zhao, J., Mo, R., Peng, L., and Yan, M. (2016). Corosolic Acid Protects Hepatocytes against Ethanol-Induced Damage by Modulating Mitogen-Activated Protein Kinases and Activating Autophagy. Eur. J. Pharmacol. 791, 578–588. doi:10.1016/j.ejphar.2016.09.031
Ha, E.-S., Han, D.-G., Seo, S.-W., Kim, J.-M., Lee, S.-K., Sim, W.-Y., et al. (2019). A Simple Hplc Method for the Quantitative Determination of Silybin in Rat Plasma: Application to a Comparative Pharmacokinetic Study on Commercial Silymarin Products. Molecules 24 (11), 2180. doi:10.3390/molecules24112180
He, J., Lee, J. H., Febbraio, M., and Xie, W. (2011). The Emerging Roles of Fatty Acid Translocase/cd36 and the Aryl Hydrocarbon Receptor in Fatty Liver Disease. Exp. Biol. Med. (Maywood) 236, 1116–1121. doi:10.1258/ebm.2011.011128
Hemanth Kumar, B., Dinesh Kumar, B., and Diwan, P. V. (2017). Hesperidin, a Citrus Flavonoid, Protects against L-Methionine-Induced Hyperhomocysteinemia by Abrogation of Oxidative Stress, Endothelial Dysfunction and Neurotoxicity in Wistar Rats. Pharm. Biol. 55, 146–155. doi:10.1080/13880209.2016.1231695
Horiguchi, N., Wang, L., Mukhopadhyay, P., Park, O., Jeong, W. I., Lafdil, F., et al. (2008). Cell Type-dependent Pro- and Anti-inflammatory Role of Signal Transducer and Activator of Transcription 3 in Alcoholic Liver Injury. Gastroenterology 134, 1148–1158. doi:10.1053/j.gastro.2008.01.016
Hosseini, N., Shor, J., and Szabo, G. (2019). Alcoholic Hepatitis: A Review. Alcohol. Alcoholism. (Oxford, Oxfordshire) 54, 408–416. doi:10.1093/alcalc/agz036
Hsiao, E. Y., McBride, S. W., Hsien, S., Sharon, G., Hyde, E. R., McCue, T., et al. (2013). Microbiota Modulate Behavioral and Physiological Abnormalities Associated with Neurodevelopmental Disorders. Cell 155, 1451–1463. doi:10.1016/j.cell.2013.11.024
Jaurigue, M. M., and Cappell, M. S. (2014). Therapy for Alcoholic Liver Disease. World J. Gastroenterol. 20, 2143–2158. doi:10.3748/wjg.v20.i9.2143
Ji, C., Chan, C., and Kaplowitz, N. (2006). Predominant Role of Sterol Response Element Binding Proteins (Srebp) Lipogenic Pathways in Hepatic Steatosis in the Murine Intragastric Ethanol Feeding Model. J. Hepatol. 45, 717–724. doi:10.1016/j.jhep.2006.05.009
Jiang, Z., Chen, C., Xie, W., Wang, M., Wang, J., and Zhang, X. (2016). Anthocyanins Attenuate Alcohol-Induced Hepatic Injury by Inhibiting Pro-inflammation Signalling. Nat. Prod. Res. 30, 469–473. doi:10.1080/14786419.2015.1020492
Jinhua, W. (2019). Ursolic Acid: Pharmacokinetics Process In Vitro and In Vivo, a Mini Review. Arch. Pharm. (Weinheim) 352, e1800222. doi:10.1002/ardp.201800222
Kim, S. J., and Lee, S. M. (2012). Effect of Baicalin on Toll-like Receptor 4-mediated Ischemia/reperfusion Inflammatory Responses in Alcoholic Fatty Liver Condition. Toxicol. Appl. Pharmacol. 258, 43–50. doi:10.1016/j.taap.2011.10.005
Kong, J. M., Chia, L. S., Goh, N. K., Chia, T. F., and Brouillard, R. (2003). Analysis and Biological Activities of Anthocyanins. Phytochemistry 64, 923–933. doi:10.1016/s0031-9422(03)00438-2
Leclercq, S., Stärkel, P., Delzenne, N. M., and de Timary, P. (2019). The Gut Microbiota: A New Target in the Management of Alcohol Dependence? Alcohol 74, 105–111. doi:10.1016/j.alcohol.2018.03.005
Lee, S., Lee, J., Lee, H., and Sung, J. (2019). Relative Protective Activities of Quercetin, Quercetin-3-Glucoside, and Rutin in Alcohol-Induced Liver Injury. J. Food Biochem. 43, e13002. doi:10.1111/jfbc.13002
Li, C., and Schluesener, H. (2017). Health-promoting Effects of the Citrus Flavanone Hesperidin. Crit. Rev. Food Sci. Nutr. 57, 613–631. doi:10.1080/10408398.2014.906382
Li, C. C., Liu, C., Fu, M., Hu, K. Q., Aizawa, K., Takahashi, S., et al. (2018). Tomato Powder Inhibits Hepatic Steatosis and Inflammation Potentially through Restoring Sirt1 Activity and Adiponectin Function Independent of Carotenoid Cleavage Enzymes in Mice. Mol. Nutr. Food Res. 62, e1700738. doi:10.1002/mnfr.201700738
Li, R., Liang, T., He, Q., Guo, C., Xu, L., Zhang, K., et al. (2013). Puerarin, Isolated from Kudzu Root (Willd.), Attenuates Hepatocellular Cytotoxicity and Regulates the GSK-3β/nf-Κb Pathway for Exerting the Hepatoprotection against Chronic Alcohol-Induced Liver Injury in Rats. Int. Immunopharmacol. 17, 71–78. doi:10.1016/j.intimp.2013.05.023
Li, S., Wang, N., Tan, H. Y., Chueng, F., Zhang, Z. J., Yuen, M. F., et al. (2020). Modulation of Gut Microbiota Mediates Berberine-Induced Expansion of Immuno-Suppressive Cells to against Alcoholic Liver Disease. Clin. Transl. Med. 10, e112. doi:10.1002/ctm2.112
Li, X., Sun, R., and Liu, R. (2019). Natural Products in Licorice for the Therapy of Liver Diseases: Progress and Future Opportunities. Pharmacol. Res. 144, 210–226. doi:10.1016/j.phrs.2019.04.025
Lieber, C. S. (2004). Alcoholic Fatty Liver: Its Pathogenesis and Mechanism of Progression to Inflammation and Fibrosis. Alcohol 34, 9–19. doi:10.1016/j.alcohol.2004.07.008
Lim, H. J., Jang, H.-J., Kim, M. H., Lee, S., Lee, S. W., Lee, S.-J., et al. (2019). Oleanolic Acid Acetate Exerts Anti-inflammatory Activity via IKKα/β Suppression in TLR3-Mediated NF-Κb Activation. Molecules 24 (21), 4002. doi:10.3390/molecules24214002
Lin, H., Zhou, Z., Zhong, W., Huang, P., Ma, N., Zhang, Y., et al. (2017). Naringenin Inhibits Alcoholic Injury by Improving Lipid Metabolism and Reducing Apoptosis in Zebrafish Larvae. Oncol. Rep. 38, 2877–2884. doi:10.3892/or.2017.5965
Liu, J., Wang, X., Liu, R., Liu, Y., Zhang, T., Fu, H., et al. (2014). Oleanolic Acid Co-administration Alleviates Ethanol-Induced Hepatic Injury via Nrf-2 and Ethanol-Metabolizing Modulating in Rats. Chem. Biol. Interact. 221, 88–98. doi:10.1016/j.cbi.2014.07.017
Lixuan, Z., Jingcheng, D., Wenqin, Y., Jianhua, H., Baojun, L., and Xiaotao, F. (2010). Baicalin Attenuates Inflammation by Inhibiting Nf-Kappab Activation in Cigarette Smoke Induced Inflammatory Models. Pulm. Pharmacol. Ther. 23, 411–419. doi:10.1016/j.pupt.2010.05.004
Louvet, A., Teixeira-Clerc, F., Chobert, M. N., Deveaux, V., Pavoine, C., Zimmer, A., et al. (2011). Cannabinoid Cb2 Receptors Protect against Alcoholic Liver Disease by Regulating Kupffer Cell Polarization in Mice. Hepatology 54, 1217–1226. doi:10.1002/hep.24524
Lu, C., Zhang, F., Xu, W., Wu, X., Lian, N., Jin, H., et al. (2015). Curcumin Attenuates Ethanol-Induced Hepatic Steatosis through Modulating Nrf2/fxr Signaling in Hepatocytes. IUBMB. Life 67, 645–658. doi:10.1002/iub.1409
Lucey, M. R., Mathurin, P., and Morgan, T. R. (2009). Alcoholic Hepatitis. N. Engl. J. Med. 360, 2758–2769. doi:10.1056/NEJMra0805786
Ma, X. Y., Zhang, M., Fang, G., Cheng, C. J., Wang, M. K., Han, Y. M., et al. (2021). Ursolic Acid Reduces Hepatocellular Apoptosis and Alleviates Alcohol-Induced Liver Injury via Irreversible Inhibition of Casp3 In Vivo. Acta Pharmacol. Sin. 42 (7), 1101–1110. doi:10.1038/s41401-020-00534-y
Mandrekar, P., and Szabo, G. (2009). Signalling Pathways in Alcohol-Induced Liver Inflammation. J. Hepatol. 50, 1258–1266. doi:10.1016/j.jhep.2009.03.007
Mao, Y. M., Zeng, M. D., Li, Y. M., Wang, B. Y., Shang, J., Shi, R. H., et al. (2009). [Capsule Metadoxine in the Treatment of Alcoholic Liver Disease: a Randomized, Double-Blind, Placebo-Controlled, Multicenter Study]. Zhonghua Gan Zang Bing Za Zhi 17, 213–216.
Massey, V. L., and Arteel, G. E. (2012). Acute Alcohol-Induced Liver Injury. Front. Physiol. 3, 193. doi:10.3389/fphys.2012.00193
McClain, C. J., and Cohen, D. A. (1989). Increased Tumor Necrosis Factor Production by Monocytes in Alcoholic Hepatitis. Hepatology 9, 349–351. doi:10.1002/hep.1840090302
Menk, M., Graw, J. A., Poyraz, D., Möbius, N., Spies, C. D., and von Haefen, C. (2018). Chronic Alcohol Consumption Inhibits Autophagy and Promotes Apoptosis in the Liver. Int. J. Med. Sci. 15, 682–688. doi:10.7150/ijms.25393
Mitra, S., De, A., and Chowdhury, A. (2020). Epidemiology of Non-alcoholic and Alcoholic Fatty Liver Diseases. Transl. Gastroenterol. Hepatol. 5, 16. doi:10.21037/tgh.2019.09.08
Mukherjee, P. S., Vishnubhatla, S., Amarapurkar, D. N., Das, K., Sood, A., Chawla, Y. K., et al. (2017). Etiology and Mode of Presentation of Chronic Liver Diseases in india: A Multi Centric Study. Plos. One 12, e0187033. doi:10.1371/journal.pone.0187033
Mutlu, E., Keshavarzian, A., Engen, P., Forsyth, C. B., Sikaroodi, M., and Gillevet, P. (2009). Intestinal Dysbiosis: A Possible Mechanism of Alcohol-Induced Endotoxemia and Alcoholic Steatohepatitis in Rats. Alcohol. Clin. Exp. Res. 33, 1836–1846. doi:10.1111/j.1530-0277.2009.01022.x
Nagy, L. E., Ding, W. X., Cresci, G., Saikia, P., and Shah, V. H. (2016). Linking Pathogenic Mechanisms of Alcoholic Liver Disease with Clinical Phenotypes. Gastroenterology 150, 1756–1768. doi:10.1053/j.gastro.2016.02.035
Naveau, S., Chollet-Martin, S., Dharancy, S., Mathurin, P., Jouet, P., Piquet, M. A., et al. (2004). A Double-Blind Randomized Controlled Trial of Infliximab Associated with Prednisolone in Acute Alcoholic Hepatitis. Hepatology 39, 1390–1397. doi:10.1002/hep.20206
O'Shea, R. S., Dasarathy, S., and McCullough, A. J. (2010a). Alcoholic Liver Disease. Am. J. Gastroenterol. 105, 14–33. quiz 33. doi:10.1038/ajg.2009.593
O'Shea, R. S., Dasarathy, S., and McCullough, A. J. (2010b). Alcoholic Liver Disease. Hepatology 51, 307–328. doi:10.1002/hep.23258
Park, H. Y., Ha, S. K., Eom, H., and Choi, I. (2013). Narirutin Fraction from Citrus Peels Attenuates Alcoholic Liver Disease in Mice. Food Chem. Toxicol. 55, 637–644. doi:10.1016/j.fct.2013.01.060
Petrasek, J., Iracheta-Vellve, A., Csak, T., Satishchandran, A., Kodys, K., Kurt-Jones, E. A., et al. (2013). Sting-irf3 Pathway Links Endoplasmic Reticulum Stress with Hepatocyte Apoptosis in Early Alcoholic Liver Disease. Proc. Natl. Acad. Sci. U S A. 110, 16544–16549. doi:10.1073/pnas.1308331110
Rong, S., Zhao, Y., Bao, W., Xiao, X., Wang, D., Nussler, A. K., et al. (2012). Curcumin Prevents Chronic Alcohol-Induced Liver Disease Involving Decreasing Ros Generation and Enhancing Antioxidative Capacity. Phytomedicine 19, 545–550. doi:10.1016/j.phymed.2011.12.006
Ronis, M. J., Hennings, L., Stewart, B., Basnakian, A. G., Apostolov, E. O., Albano, E., et al. (2011). Effects of Long-Term Ethanol Administration in a Rat Total Enteral Nutrition Model of Alcoholic Liver Disease. Am. J. Physiol. Gastrointest. Liver Physiol. 300, G109–G119. doi:10.1152/ajpgi.00145.2010
Roohbakhsh, A., Parhiz, H., Soltani, F., Rezaee, R., and Iranshahi, M. (2015). Molecular Mechanisms behind the Biological Effects of Hesperidin and Hesperetin for the Prevention of Cancer and Cardiovascular Diseases. Life Sci. 124, 64–74. doi:10.1016/j.lfs.2014.12.030
Roy, S., Benz, F., Luedde, T., and Roderburg, C. (2015). The Role of Mirnas in the Regulation of Inflammatory Processes during Hepatofibrogenesis. Hepatobiliary. Surg. Nutr. 4, 24–33. doi:10.3978/j.issn.2304-3881.2015.01.05
Saller, R., Brignoli, R., Melzer, J., and Meier, R. (2008). An Updated Systematic Review with Meta-Analysis for the Clinical Evidence of Silymarin. Forsch. Komplementmed. 15, 9–20. doi:10.1159/000113648
Saller, R., Meier, R., and Brignoli, R. (2001). The Use of Silymarin in the Treatment of Liver Diseases. Drugs 61, 2035–2063. doi:10.2165/00003495-200161140-00003
Sarin, S. K., Pande, A., and Schnabl, B. (2019). Microbiome as a Therapeutic Target in Alcohol-Related Liver Disease. J. Hepatol. 70, 260–272. doi:10.1016/j.jhep.2018.10.019
Sasaki, H., Sunagawa, Y., Takahashi, K., Imaizumi, A., Fukuda, H., Hashimoto, T., et al. (2011). Innovative Preparation of Curcumin for Improved Oral Bioavailability. Biol. Pharm. Bull. 34, 660–665. doi:10.1248/bpb.34.660
Schulze, R. J., Sathyanarayan, A., and Mashek, D. G. (2017). Breaking Fat: The Regulation and Mechanisms of Lipophagy. Biochim. Biophys. Acta Mol. Cel. Biol. Lipids 1862, 1178–1187. doi:10.1016/j.bbalip.2017.06.008
Seitz, H. K., and Stickel, F. (2007). Molecular Mechanisms of Alcohol-Mediated Carcinogenesis. Nat. Rev. Cancer 7, 599–612. doi:10.1038/nrc2191
Shapouri-Moghaddam, A., Mohammadian, S., Vazini, H., Taghadosi, M., Esmaeili, S. A., Mardani, F., et al. (2018). Macrophage Plasticity, Polarization, and Function in Health and Disease. J. Cel. Physiol. 233, 6425–6440. doi:10.1002/jcp.26429
Silva, J., Spatz, M. H., Folk, C., Chang, A., Cadenas, E., Liang, J., et al. (2021). Dihydromyricetin Improves Mitochondrial Outcomes in the Liver of Alcohol-Fed Mice via the AMPK/Sirt-1/PGC-1α Signaling axis. Alcohol 91, 1–9. doi:10.1016/j.alcohol.2020.10.002
Silva, J., Yu, X., Moradian, R., Folk, C., Spatz, M. H., Kim, P., et al. (2020). Dihydromyricetin Protects the Liver via Changes in Lipid Metabolism and Enhanced Ethanol Metabolism. Alcohol. Clin. Exp. Res. 44, 1046–1060. doi:10.1111/acer.14326
Singal, A. K., Bataller, R., Ahn, J., Kamath, P. S., and Shah, V. H. (2018). Acg Clinical Guideline: Alcoholic Liver Disease. Am. J. Gastroenterol. 113, 175–194. doi:10.1038/ajg.2017.469
Sivakumar, G., Vail, D. R., Nair, V., Medina-Bolivar, F., and Lay, J. O. (2009). Plant-based Corosolic Acid: Future Anti-diabetic Drug? Biotechnol. J. 4, 1704–1711. doi:10.1002/biot.200900207
Song, X., Yin, S., Huo, Y., Liang, M., Fan, L., Ye, M., et al. (2015). Glycycoumarin Ameliorates Alcohol-Induced Hepatotoxicity via Activation of Nrf2 and Autophagy. Free Radic. Biol. Med. 89, 135–146. doi:10.1016/j.freeradbiomed.2015.07.006
Song, Z., Deaciuc, I., Song, M., Lee, D. Y., Liu, Y., Ji, X., et al. (2006). Silymarin Protects against Acute Ethanol-Induced Hepatotoxicity in Mice. Alcohol. Clin. Exp. Res. 30, 407–413. doi:10.1111/j.1530-0277.2006.00063.x
Stice, C. P., Xia, H., and Wang, X. D. (2018). Tomato Lycopene Prevention of Alcoholic Fatty Liver Disease and Hepatocellular Carcinoma Development. Chronic. Dis. Transl. Med. 4, 211–224. doi:10.1016/j.cdtm.2018.11.001
Tabeshpour, J., Hosseinzadeh, H., Hashemzaei, M., and Karimi, G. (2020). A Review of the Hepatoprotective Effects of Hesperidin, a Flavanon Glycoside in Citrus Fruits, against Natural and Chemical Toxicities. Daru 28, 305–317. doi:10.1007/s40199-020-00344-x
Taïeb, J., Mathurin, P., Elbim, C., Cluzel, P., Arce-Vicioso, M., Bernard, B., et al. (2000). Blood Neutrophil Functions and Cytokine Release in Severe Alcoholic Hepatitis: Effect of Corticosteroids. J. Hepatol. 32, 579–586. doi:10.1016/s0168-8278(00)80219-6
Tatsukawa, H., Fukaya, Y., Frampton, G., Martinez-Fuentes, A., Suzuki, K., Kuo, T. F., et al. (2009). Role of Transglutaminase 2 in Liver Injury via Cross-Linking and Silencing of Transcription Factor Sp1. Gastroenterology 136, 1783–e10. doi:10.1053/j.gastro.2009.01.007
Tatsukawa, H., and Kojima, S. (2010). Recent Advances in Understanding the Roles of Transglutaminase 2 in Alcoholic Steatohepatitis. Cell. Biol. Int. 34, 325–334. doi:10.1042/cbi20090130
Thomes, P. G., Trambly, C. S., Fox, H. S., Tuma, D. J., and Donohue, T. M. (2015). Acute and Chronic Ethanol Administration Differentially Modulate Hepatic Autophagy and Transcription Factor Eb. Alcohol. Clin. Exp. Res. 39, 2354–2363. doi:10.1111/acer.12904
Tian, T., Liu, X., Lee, E. S., Sun, J., Feng, Z., Zhao, L., et al. (2017). Synthesis of Novel Oleanolic Acid and Ursolic Acid in C-28 Position Derivatives as Potential Anticancer Agents. Arch. Pharm. Res. 40, 458–468. doi:10.1007/s12272-016-0868-8
Tighe, S. P., Akhtar, D., Iqbal, U., and Ahmed, A. (2020). Chronic Liver Disease and Silymarin: A Biochemical and Clinical Review. J. Clin. Transl. Hepatol. 8, 454–458. doi:10.14218/jcth.2020.00012
Tomita, K., Azuma, T., Kitamura, N., Nishida, J., Tamiya, G., Oka, A., et al. (2004). Pioglitazone Prevents Alcohol-Induced Fatty Liver in Rats through Up-Regulation of C-Met. Gastroenterology 126, 873–885. doi:10.1053/j.gastro.2003.12.008
Ueno, T., and Komatsu, M. (2017). Autophagy in the Liver: Functions in Health and Disease. Nat. Rev. Gastroenterol. Hepatol. 14, 170–184. doi:10.1038/nrgastro.2016.185
Valansa, A., Tietcheu Galani, B. R., Djamen Chuisseu, P. D., Tontsa Tsamo, A., Ayissi Owona, V. B., and Yanou Njintang, N. (2020). Natural Limonoids Protect Mice from Alcohol-Induced Liver Injury. J. Basic Clin. Physiol. Pharmacol. 31. doi:10.1515/jbcpp-2019-0271
Vonghia, L., Leggio, L., Ferrulli, A., Bertini, M., Gasbarrini, G., and Addolorato, G. (2008). Acute Alcohol Intoxication. Eur. J. Intern. Med. 19, 561–567. doi:10.1016/j.ejim.2007.06.033
Wagner, M., Zollner, G., and Trauner, M. (2011). Nuclear Receptors in Liver Disease. Hepatology 53, 1023–1034. doi:10.1002/hep.24148
Wan, J., Benkdane, M., Alons, E., Lotersztajn, S., and Pavoine, C. (2014). M2 Kupffer Cells Promote Hepatocyte Senescence: An Il-6-dependent Protective Mechanism against Alcoholic Liver Disease. Am. J. Pathol. 184, 1763–1772. doi:10.1016/j.ajpath.2014.02.014
Wang, W. J., Xiao, P., Xu, H. Q., Niu, J. Q., and Gao, Y. H. (2019). Growing burden of Alcoholic Liver Disease in china: A Review. World J. Gastroenterol. 25, 1445–1456. doi:10.3748/wjg.v25.i12.1445
Wang, X., Liu, R., Zhang, W., Zhang, X., Liao, N., Wang, Z., et al. (2013). Oleanolic Acid Improves Hepatic Insulin Resistance via Antioxidant, Hypolipidemic and Anti-inflammatory Effects. Mol. Cel. Endocrinol. 376, 70–80. doi:10.1016/j.mce.2013.06.014
Wang, X. D. (2012). Lycopene Metabolism and its Biological Significance. Am. J. Clin. Nutr. 96, 1214s–22s. doi:10.3945/ajcn.111.032359
Watanabe, M. (2007). An Anthocyanin Compound in Buckwheat Sprouts and its Contribution to Antioxidant Capacity. Biosci. Biotechnol. Biochem. 71, 579–582. doi:10.1271/bbb.60471
Whitfield, K., Rambaldi, A., Wetterslev, J., and Gluud, C. (2009). Pentoxifylline for Alcoholic Hepatitis. Cochrane. Database. Syst. Rev. 2009, Cd007339. doi:10.1002/14651858.CD007339.pub2
Wu, D., and Cederbaum, A. I. (2005). Oxidative stress mediated toxicity exerted by ethanol-inducible cyp2e1. Toxicol. Appl. Pharmacol. 207, 70–76. doi:10.1016/j.taap.2005.01.057
Xu, W., Zhao, X., Daha, M. R., and van Kooten, C. (2013). Reversible Differentiation of Pro- and Anti-inflammatory Macrophages. Mol. Immunol. 53, 179–186. doi:10.1016/j.molimm.2012.07.005
Yan, A. W., Fouts, D. E., Brandl, J., Stärkel, P., Torralba, M., Schott, E., et al. (2011). Enteric Dysbiosis Associated with a Mouse Model of Alcoholic Liver Disease. Hepatology 53, 96–105. doi:10.1002/hep.24018
Yin, H. Q., Kim, Y. S., Choi, Y. J., Kim, Y. C., Sohn, D. H., Ryu, S. Y., et al. (2008). Effects of Tanshinone Iia on the Hepatotoxicity and Gene Expression Involved in Alcoholic Liver Disease. Arch. Pharm. Res. 31, 659–665. doi:10.1007/s12272-001-1209-2
You, M., and Arteel, G. E. (2019). Effect of Ethanol on Lipid Metabolism. J. Hepatol. 70, 237–248. doi:10.1016/j.jhep.2018.10.037
You, M., Fischer, M., Deeg, M. A., and Crabb, D. W. (2002). Ethanol Induces Fatty Acid Synthesis Pathways by Activation of Sterol Regulatory Element-Binding Protein (Srebp). J. Biol. Chem. 277, 29342–29347. doi:10.1074/jbc.M202411200
You, M., Liang, X., Ajmo, J. M., and Ness, G. C. (2008). Involvement of Mammalian Sirtuin 1 in the Action of Ethanol in the Liver. Am. J. Physiol. Gastrointest. Liver Physiol. 294, G892–G898. doi:10.1152/ajpgi.00575.2007
You, M., and Rogers, C. Q. (2009). Adiponectin: A Key Adipokine in Alcoholic Fatty Liver. Exp. Biol. Med. (Maywood) 234, 850–859. doi:10.3181/0902-mr-61
Yu, L., Chen, Y., and Tooze, S. A. (2018). Autophagy Pathway: Cellular and Molecular Mechanisms. Autophagy 14, 207–215. doi:10.1080/15548627.2017.1378838
Zeng, H., Guo, X., Zhou, F., Xiao, L., Liu, J., Jiang, C., et al. (2019a). Quercetin Alleviates Ethanol-Induced Liver Steatosis Associated with Improvement of Lipophagy. Food Chem. Toxicol. 125, 21–28. doi:10.1016/j.fct.2018.12.028
Zeng, P., Li, J., Chen, Y., and Zhang, L. (2019b). The Structures and Biological Functions of Polysaccharides from Traditional Chinese Herbs. Prog. Mol. Biol. Transl. Sci. 163, 423–444. doi:10.1016/bs.pmbts.2019.03.003
Zeng, T., Zhang, C. L., Xiao, M., Yang, R., and Xie, K. Q. (2016). Critical Roles of Kupffer Cells in the Pathogenesis of Alcoholic Liver Disease: From Basic Science to Clinical Trials. Front. Immunol. 7, 538. doi:10.3389/fimmu.2016.00538
Zhang, T., Li, Q., Cheng, L., Buch, H., and Zhang, F. (2019). Akkermansia Muciniphila Is a Promising Probiotic. Microb. Biotechnol. 12, 1109–1125. doi:10.1111/1751-7915.13410
Zhang, W., Hong, R., and Tian, T. (2013). Silymarin's Protective Effects and Possible Mechanisms on Alcoholic Fatty Liver for Rats. Biomol. Ther. (Seoul) 21, 264–269. doi:10.4062/biomolther.2013.020
Zhang, Y., Jin, Q., Li, X., Jiang, M., Cui, B. W., Xia, K. L., et al. (2018b). Amelioration of Alcoholic Liver Steatosis by Dihydroquercetin through the Modulation of Ampk-dependent Lipogenesis Mediated by P2x7r-Nlrp3-Inflammasome Activation. J. Agric. Food Chem. 66, 4862–4871. doi:10.1021/acs.jafc.8b00944
Zhang, Y., Wang, C., Yu, B., Jiang, J. D., and Kong, W. J. (2018a). Gastrodin Protects against Ethanol-Induced Liver Injury and Apoptosis in Hepg2 Cells and Animal Models of Alcoholic Liver Disease. Biol. Pharm. Bull. 41, 670–679. doi:10.1248/bpb.b17-00825
Zhao, X., Gong, L., Wang, C., Liu, M., Hu, N., Dai, X., et al. (2021). Quercetin Mitigates Ethanol-Induced Hepatic Steatosis in Zebrafish via P2x7r-Mediated Pi3k/Keap1/nrf2 Signaling Pathway. J. Ethnopharmacol. 268, 113569. doi:10.1016/j.jep.2020.113569
Zhou, C., Lai, Y., Huang, P., Xie, L., Lin, H., Zhou, Z., et al. (2019). Naringin Attenuates Alcoholic Liver Injury by Reducing Lipid Accumulation and Oxidative Stress. Life Sci. 216, 305–312. doi:10.1016/j.lfs.2018.07.031
Zhou, Z., Zhong, W., Lin, H., Huang, P., Ma, N., Zhang, Y., et al. (2017). Hesperidin Protects against Acute Alcoholic Injury through Improving Lipid Metabolism and Cell Damage in Zebrafish Larvae. Evid. Based Complement. Alternat. Med. 2017, 7282653. doi:10.1155/2017/7282653
Zhou, Z., and Zhong, W. (2017). Targeting the Gut Barrier for the Treatment of Alcoholic Liver Disease. Liver Res. 1, 197–207. doi:10.1016/j.livres.2017.12.004
Ziqubu, K., Dludla, P. V., Joubert, E., Muller, C. J. F., Louw, J., Tiano, L., et al. (2020). Isoorientin: A Dietary Flavone with the Potential to Ameliorate Diverse Metabolic Complications. Pharmacol. Res. 158, 104867. doi:10.1016/j.phrs.2020.104867
5-Lox 5-lipoxygenase
α-GST α-glutathione S-transferase
ADH alcohol dehydrogenase
AFL alcoholic fatty liver
ALD alcoholic liver disease
ALDH2 aldehyde dehydrogenase 2
ApoB apolipoprotein B
ASH alcoholic hepatitis
BAs bile acids
Bax B-cell lymphoma 2-associated X protein
Bcl2 B-cell lymphoma 2
CASP3 caspase-3
CAT catalase
CLD chronic liver disease
c-Met cellular-mesenchymal epithelial transition factor
Cox-2 cyclooxygenase-2
CYP2E1 cytochrome P450 2E1
ER endoplasmic reticulum
FAs fatty acids
FAT CD36/FA transportase
FOS fructooligosaccharides
FXR farnesoid X receptor
GPx glutathione peroxidase
GSH-Px glutathione peroxidase
HCC hepatocellular carcinoma
KCs kupffer cells
LDs lipid droplets
LIR LC3-interacting region
LPS lipopolysaccharide
MDA malondialdehyde
MF molecular formula
MGST1 microsomal glutathione transferase 1
MW molecular weight
NAD+ oxidized nicotinamide adenine dinucleotide
NADH nicotinamide adenine dinucleotide
NCLD noncirrhotic liver damage
Nrf2 NF-E2-related factor 2
OPTN optineurin
PCD programmed cell death
PINK phosphatase and tensin homolog-induced kinase
PLIN2 perilipin 2
PPARα peroxisome proliferator-activated receptor α
PTEN phosphatase and tensin homolog
Reg3g regenerating family member 3 gamma
ROS reactive oxygen species
Sirt1 sirtuin1
SOD superoxide dismutase superoxide dismutase
SOD superoxide dismutase superoxide dismutase
SREBP-1c sterol regulatory element-binding protein-1c
STAT3 signal transducer and activator of transcription 3
STING stimulator of interferon gene
TAC tricarboxylic acid cycle
TCM traditional chinese medicine
TG2 transglutaminase 2
TGs triglycerides
TLRs toll-like receptors
TNF tumour necrosis factor
VLDL very low-density lipoprotein
Keywords: alcoholic liver disease, natural compounds, oxidative stress, inflammatory pathway, autophagy, apoptosis
Citation: Yan J, Nie Y, Luo M, Chen Z and He B (2021) Natural Compounds: A Potential Treatment for Alcoholic Liver Disease?. Front. Pharmacol. 12:694475. doi: 10.3389/fphar.2021.694475
Received: 13 April 2021; Accepted: 23 June 2021;
Published: 05 July 2021.
Edited by:
Zhenyuan Song, University of Illinois at Chicago, United StatesReviewed by:
Sheikh Raisuddin, Jamia Hamdard University, IndiaCopyright © 2021 Yan, Nie, Luo, Chen and He. This is an open-access article distributed under the terms of the Creative Commons Attribution License (CC BY). The use, distribution or reproduction in other forums is permitted, provided the original author(s) and the copyright owner(s) are credited and that the original publication in this journal is cited, in accordance with accepted academic practice. No use, distribution or reproduction is permitted which does not comply with these terms.
*Correspondence: Beihui He, Z3JhZjMwM0BzaW5hLmNvbQ==
†These authors have contributed equally to this work
Disclaimer: All claims expressed in this article are solely those of the authors and do not necessarily represent those of their affiliated organizations, or those of the publisher, the editors and the reviewers. Any product that may be evaluated in this article or claim that may be made by its manufacturer is not guaranteed or endorsed by the publisher.
Research integrity at Frontiers
Learn more about the work of our research integrity team to safeguard the quality of each article we publish.