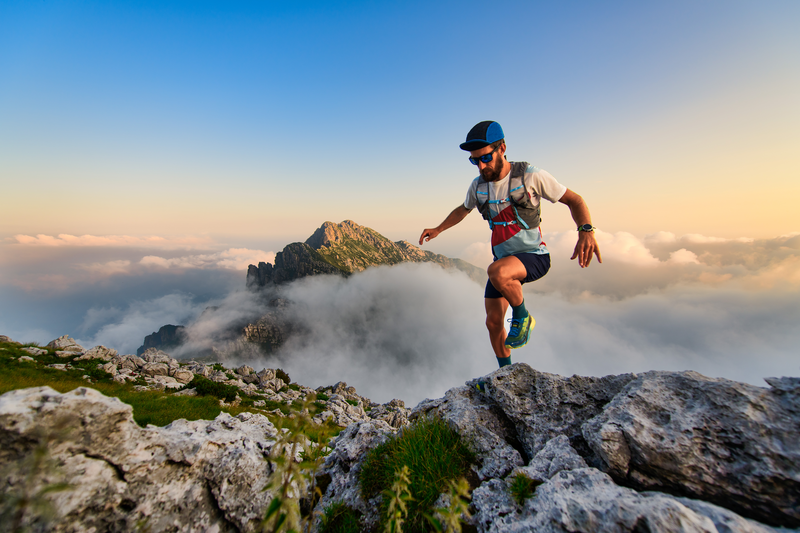
94% of researchers rate our articles as excellent or good
Learn more about the work of our research integrity team to safeguard the quality of each article we publish.
Find out more
ORIGINAL RESEARCH article
Front. Pharmacol. , 25 June 2021
Sec. Experimental Pharmacology and Drug Discovery
Volume 12 - 2021 | https://doi.org/10.3389/fphar.2021.686358
This article is part of the Research Topic Antimycobacterial Drug Discovery: Molecular Therapeutics and Target Identification View all 7 articles
Spontaneous mutants of Mycobacterium smegmatis strain mc2155 resistant to 1-PG (iridium-L-phenylglycine complex), an antimycobacterial antibiotic, were isolated. Based on the discovery that some 1-PG-resistant mutants (1-PGR) were also resistant to high concentrations of clarithromycin (≥250 μg/ml), but no other anti-mycobacterial antibiotics, the 23S rRNA region spanning the peptidyl transferase domain was sequenced and mutations shown to be localized in the peptidyl transferase domain of the 23S rRNA gene. Measurements showed that 1-PG bound to ribosomes isolated from the 1-PG-sensitive parental strain, but the ribosome binding values for the 1-PGR mutant reduced.
There is a well established recognition that new antibiotics for the treatment of mycobacterial infections are needed (Raviglione and Ditiu, 2013; Zumla et al., 2013). Further, the prevalence of mycobacterial pulmonary diseases caused by the nontuberculous mycobacteria (e.g., Mycobacterium avium complex, MAC) is estimated at 10–15 cases per 100,000 individuals and increasing at a rate of 5–8% per year in the United States and Canada (Marras et al., 2007; Billinger et al., 2009; Winthrop et al., 2010). In addition, as NTM-infected individuals are susceptible to re-activation or re-infection after anti-mycobacterial therapy (Wallace et al., 2002; Wallace et al., 2014; Min et al., 2015). In individuals over 60 years NTM-pulmonary disease prevalence approaches 100 cases per 100,000 (Prevots et al., 2010). It would follow that as the proportion of the United States population over 60 years increases to 25% by 2025, it is likely that the prevalence of nontuberculous mycobacteria (NTM) disease will continue to increase, as will the need for novel anti-mycobacterial drugs.
Recently we have described the synthesis and antimycobacterial activity of transition metal-α-amino acid complexes (Karpin et al., 2013). The transition metals, iridium (Ir), ruthenium (Ru), rhodium (Ro) have been complexed with a variety of α-amino acids. Of the family of complexes synthesized, Ir-phenylglycine (1-PG) exhibited anti-mycobacterial activity with MICs of 5 μg/ml against Mycobacterium smegmatis, 31 μg/ml against Mycobacterium abscessus, and 15 μg/ml against Mycobacterium intracellulare, Mycobacterium chelonae, and Mycobacterium bovis BCG (Karpin et al., 2013). As 1-PG was shown to lack cytotoxic and hemolytic activities (Karpin et al., 2013), it was decided to proceed with the identification of the drug’s target.
Herein we report the isolation of 1-PG-resistant mutants of M. smegmatis and the identification of at least one target of the drug, the peptidyl transferase domain of the mycobacterial 23S rRNA.
The structure of the transition metal-α-amino acid complex 1-PG is illustrated in Figure 1. 1-PG is a cyclopentadienyl (Cp*) having an α-amino acid, here phenylglycine, complexed with iridium (Ir). 1-PG synthesis has been described (Karpin et al., 2013).
The antimycobacterial antibiotics, clarithromycin, ethambutol, ciprofloxacin, and were purchased from Sigma-Chemical Co. (St. Louis, MO)
M. smegmatis strain mc2155, was used in the study and its growth and preparation for susceptibility measurements are described in Falkinham et al. (Falkinham et al., 2012). Cultures were grown to mid-logarithmic phase to obtain uniformity of MIC measurements.
MICs and MBCs of compounds dissolved in M7H9 broth medium containing 0.5% (vol/vol) glycerol and 10% (vol/vol) oleic acid-albumin were measured by broth microdilution in 96-well microtitre plates (Falkinham et al., 2012).
Samples (0.1 ml) of a stationary phase culture of M. smegmatis strain mc2155 were spread on M7H10 agar medium containing 10% (vol/vol) oleic acid-albumin and concentrations of 1-PG ranging from 4–20 µg 1-PG/ml. Plates were incubated at 37°C and single colonies picked from the plate containing 20 µg 1_PG/mL and streaked for purification on both 1-PG-containing and 1-PG-free medium (to exclude 1-PG-dependant mutants). Following isolation, the MICs of the parent and mutants were measured against 1-PG and other antimycobacterial antibiotics as described above.
DNA was isolated from the parent and mutant A1 1-PGR mutant and the 23S rRNA gene was amplified by PCR as described (Meier et al., 1994; Jamal et al., 2000), resulting in production of 419 bp amplicon (domain V) of the 23S rRNA gene). The 419 bp amplicon was sequenced (Sanger) at the Virginia Tech Biocomplexity Institute employing an ABI 3730 (Applied Biosystems).
Ribosomes were isolated from M. smegmatis strain mc2155 and the 1-PGR mutants following the procedure of Doucet-Populaire et al. (Doucet-Populaire et al., 1998). Cells were harvested from 50 ml cultures by centrifugation (5,000 × g for 20 min), supernatant medium discarded, and cells washed twice in 50 ml of Buffer A (10 mM Tris-HCl, 4 mM MgCl2, 10 mM NH4Cl, 100 mM KCl, pH 7.2). Washed cells were suspended in 5 ml of Buffer A, cell suspensions were cooled on ice-water, and cells lyzed by sonication. DNase (RNase-free) was added to the cooled and broken cell suspensions at a final concentration of 5 units/ml and incubated on ice for 15 min. Whole cells were removed from the lysate by centrifugation (5,000 × g for 5 min) and the supernatant transferred to an ultracentrifuge tube and centrifuged at 30,000 × g for 30 min to pellet cell walls and membranes. The supernatant from that centrifugation was transferred to a fresh ultracentrifuge tube and ribosomes pelleted at 100,000 × g for 60 min. The pelleted ribosomes were suspended in 2 ml of Buffer A, aliquot in 0.5 ml samples, labeled and frozen at −70°C.
1-PG-binding to ribosomes was measured as described by Douthwait and Aagaard (Douthwaite and Aagaard, 1993). An aliquot of each strain’s (i.e., mc2155 and 1-PGR mutants) ribosome suspension was defrosted and 50 µl of ribosomes was mixed with 50 µl of 1 mg 1-PG/ml and incubated at 37°C. Immediately and at 10 min intervals up to 30 min, two 10 µl samples were withdrawn, filtered through 0.45 µm pore size filters, and washed with 5 ml of Buffer A. The filters were placed in a tube, 1 ml of 1 M HMO3 added, and the concentration of Ir measured by Inductively Coupled Plasma—Optical Emission Spectroscopy (ICP-OES).
The HNO3-digests were diluted with Nanopure® deionized water to a final volume of 5 ml. Each sample was allowed to sit for 15 min at room temperature. Iridium in samples was measured as all iridium isotopes using a Perkin Elmer 4300 DV ICP-OES. Calibration was performed following the manufacturer’s directions. Iridium in both the filters and the filtrates were measured independently to determine what may have been bound to the ribosome and/or washed through the filter paper containing the free complex. A standard curve was constructed using samples of iridium prepared for ICP-OES by Inorganic Ventures (Christiansburg, VA).
Seven (7) mutants of M. smegmatis strain mc2155 from a single culture resistant to 20 µg 1-PG/ml) were isolated (frequency = 3.5 × 10–7) and their susceptibility to anti-mycobacterial antibiotics measured (Table 1). MIC increases of the 1-PGR mutants was modest compared to their 1-PGS parent strain mc2155 and two, namely mutants D1 and F1, had MICs equal to that of the 1-PGS parent (Table 1). All seven were also resistant to clarithromycin (MIC = 2–16-fold higher than parent), but their susceptibilities to other anti-mycobacterial drugs were not different from that of the parent strain (Table 1). Based on the fact that mutants A1 and B1 retained the dry colony morphology of the parent and did not produce mucoid colonies as did mutants B2, C1, and E1, mutant strain A1 was investigated further.
TABLE 1. MICs of Standard Mycobacterial Drugs Against 1-PG-resistant Mutants of Mycobacterium smegmatis strain mc2 155. The bold values are short form name of the antimycobacterial compound in Figure 1, 1-PG.
As clarithromycin-resistant mutants have mutations in the peptidyl transferase domain (V) of the 23S rRNA gene (Meier et al., 1994; Jamal et al., 2000), DNA was isolated from the parent and mutant A1 and the 23S rRNA gene was amplified by PCR [12.13], resulting in production of 419 bp amplicon (domain V). Analysis of the sequence of the 419 bp amplicon in the 1-PGR/ClaR mutant revealed a substitution of T for G at position 79 (base 2057 of the 23S rRNA) and a substitution of C for a T at position 120 (base 2611of the 23S rRNA gene) (Table 2). Both are within the peptidyl transferase loop (domain V) of the 23S rRNA gene. As there were no other base changes in the 1-PGR-mutant, we surmise that those mutations rendered the M. smegmatis mutant strain resistant to 1-PG and clarithromycin because of alterations in the conformation of that loop (Doucet-Populaire et al., 1998; Douthwaite and Aagaard, 1993).
The concentrations of total iridium (ppm) in the filtrate and filter-bound ribosomes of the 1-PGR mutant strain A1 and its parent are shown in Table 3. Each value in the table is an average of five readings and the results of two independent Ir-binding studies are listed (Experiments 1 and 2). The ribosome fractions from the 1-PGR-strain bound less iridium than those of the parent 1-PGS-strain. (Table 2). Further, more iridium (as 1-PG) was recovered in the filtrate of the 1-PGR-strain than from the filtrate of the 1-PGS-parental strain which is in agreement with the hypothesis that a possible alteration in the structure of the peptidyl transferase site of the ribosomal fraction led to reduced 1-PG-binding and resistance.
TABLE 3. Iridium-binding of filter-bound ribosomes of 1-PGSM. smegmatis parent and 1-PGR mutant A1. The bold values are short form name of the antimycobacterial compound in Figure 1, 1-PG.
Independent ClaR-mutants of mc2155 were also isolated, but only 4/7 were 1-PGR, suggesting the two antibiotics do not share exactly the same range of activity. This separation of targets is consistent with observations that clarithromycin inhibits peptidyl transferase activity, ribosome assembly, and outer membrane assembly in mycobacteria (Doucet-Populaire et al., 1998). Support for that contention could be obtained by demonstration of co-transduction of resistance to both antibiotics (Lee et al., 2004). The discovery that a substantial fraction (43%) of clarithromycin-resistant M. smegmatis mutants (ClaR) were still susceptible to Ir-phenylglycine (1-PGS) encourages us that 1-PG will prove to be a useful anti-mycobacterial drug, even in infections due to a ClaRMycobacterium spp. strain.
The datasets presented in this study can be found in online repositories. The names of the repository/repositories and accession number(s) can be found in the article/Supplementary Material.
All authors listed have made a substantial, direct, and intellectual contribution to the work and approved it for publication.
The authors declare that the research was conducted in the absence of any commercial or financial relationships that could be construed as a potential conflict of interest.
The authors acknowledge the guidance and technical assistance of Ms. Myra D. Williams with the preparation of cultures, and measurements of MICs, MBCs, and hemolysis.
Billinger, M. E., Olivier, K. N., Viboud, C., Montes de Oca, R., Steiner, C., Holland, S. M., et al. (2009). Nontuberculous Mycobacteria-Associated Lung Disease in Hospitalized Persons, United States, 1998-2005. Emerg. Infect. Dis. 15, 1562–1569. doi:10.3201/eid1510.090196
Doucet-Populaire, F., Jo, Capobianco., Zakula, D., Jarlier, V., and Goldman, R. C. (1998). Molecular Basis of Clarithromycin Activity against Mycobacterium avium and Mycobacterium Smegmatis. J. Antimicrob. Chemother. 41, 179–187. doi:10.1093/jac/41.2.179
Douthwaite, S., and Aagaard, C. (1993). Erythromycin Binding Is Reduced in Ribosomes with Conformational Alterations in the 23 S rRNA Peptidyl Transferase Loop. J. Mol. Biol. 232, 725–731. doi:10.1006/jmbi.1993.1426
Falkinham, J. O., Macri, R. V., Maisuria, B. B., Actis, M. L., Sugandhi, E. W., Williams, A. A., et al. (2012). Antibacterial Activities of Dendritic Amphiphiles against Nontuberculous Mycobacteria. Tuberculosis 92, 173–181. doi:10.1016/j.tube.2011.12.002
Jamal, M. A., Maeda, S., Nakata, N., Kai, M., Fukuchi, K., and Kashiwabara, Y. (2000). Molecular Basis of Clarithromycin-Resistance in Mycobacterium avium Intracellulare Complex. Tuber Lung Dis. 80, 1–4. doi:10.1054/tuld.1999.0227
Karpin, G. W., Merola, J. S., and Falkinham, J. O. (2013). Transition Metal-α-Amino Acid Complexes with Antibiotic Activity against Mycobacterium Spp. Antimicrob. Agents Chemother. 57, 3434–3436. doi:10.1128/aac.00452-13
Lee, S., Kriakov, J., Vilcheze, C., Dai, Z., Hatfull, G. F., and Jacobs, W. R. (2004). BXZ1, a New Generalized Transducing Phage for Mycobacteria. FEMS Microbiol. Lttrs 241, 271–276. doi:10.1016/j.femsle.2004.10.032
Marras, T. K., Chedore, P., Ying, A. M., and Jamieson, F. (2007). Isolation Prevalence of Pulmonary Non-tuberculous Mycobacteria in Ontario, 1997 2003. Thorax 62, 661–666. doi:10.1136/thx.2006.070797
Meier, A., Kirschner, P., Springer, B., Steingrube, V. A., Brown, B. A., Wallace, R. J., et al. (1994). Identification of Mutations in 23S rRNA Gene of Clarithromycin-Resistant Mycobacterium Intracellulare. Antimicrob. Agents Chemother. 38, 381–384. doi:10.1128/aac.38.2.3811994
Min, J., Park, J., Lee, Y. J., Kim, S. J., Park, J. S., Cho, Y.-J., et al. (2015). Determinants of Recurrence after Successful Treatment of Mycobacterium avium Complex Lung Disease. Int. J. Tuberc. Lung Dis. 19, 1239–1245. doi:10.5588/ijtld.14.0139
Prevots, D. R., Shaw, P. A., Strickland, D., Jackson, L. A., Raebel, M. A., Blosky, M. A., et al. (2010). Nontuberculous Mycobacterial Lung Disease Prevalence at Four Integrated Health Care Delivery Systems. Am. J. Respir. Crit. Care Med. 182, 970–976. doi:10.1164/rccm.201002-0310oc
Raviglione, M. C., and Ditiu, L. (2013). Setting New Targets in the Fight against Tuberculosis. Nat. Med. 19, 263. doi:10.1038/nm.3129
Wallace, R. J., Zhang, Y., Brown-Elliott, B. A., Yakrus, M. A., Wilson, R. W., Mann, L., et al. (2002). Repeat Positive Cultures in Mycobacterium Intracellulare Lung Disease after Macrolide Therapy Represent New Infections in Patients with Nodular Bronchiectasis Lung Disease after Macrolide Therapy Represent New Infections in Patients with Nodular Bronchiectasis. J. Infect. Dis. 186, 266–273. doi:10.1086/341207
Wallace, R. J., Brown-Elliott, B. A., McNulty, S., Philley, J. V., Killingley, J., Wilson, R. W., et al. (2014). Macrolide/azalide Therapy for Nodular/bronchiectatic Mycobacterium avium Complex Lung Disease. Chest 146, 276–282. doi:10.1378/chest.13-2538
Winthrop, K. L., McNelley, E., Kendall, B., Marshall-Olson, A., Morris, C., Cassidy, M., et al. (2010). Pulmonary Nontuberculous Mycobacterial Disease Prevalence and Clinical Features. Am. J. Respir. Crit. Care Med. 182, 977–982. doi:10.1164/rccm.201003-0503oc
Keywords: transition metal-α-amino acid complexes, mycobacteria, clarithromycin, 23S rRNA, peptidyl transferase
Citation: Karpin GW, Merola JS and Falkinham JO (2021) Identification of the Target for a Transition Metal-α-Amino Acid Complex Antibiotic Against Mycobacterium smegmatis. Front. Pharmacol. 12:686358. doi: 10.3389/fphar.2021.686358
Received: 26 March 2021; Accepted: 07 June 2021;
Published: 25 June 2021.
Edited by:
Gert Kruger, University of KwaZulu-Natal, South AfricaReviewed by:
Suparna Sanyal, Uppsala University, SwedenCopyright © 2021 Karpin, Merola and Falkinham. This is an open-access article distributed under the terms of the Creative Commons Attribution License (CC BY). The use, distribution or reproduction in other forums is permitted, provided the original author(s) and the copyright owner(s) are credited and that the original publication in this journal is cited, in accordance with accepted academic practice. No use, distribution or reproduction is permitted which does not comply with these terms.
*Correspondence: Joseph O. Falkinham III, am9maWlpQHZ0LmVkdQ==
Disclaimer: All claims expressed in this article are solely those of the authors and do not necessarily represent those of their affiliated organizations, or those of the publisher, the editors and the reviewers. Any product that may be evaluated in this article or claim that may be made by its manufacturer is not guaranteed or endorsed by the publisher.
Research integrity at Frontiers
Learn more about the work of our research integrity team to safeguard the quality of each article we publish.