- 1Department of Cardiology, The Second Affiliated Hospital of Soochow University, Suzhou, China
- 2Department of Pathophysiology, School of Biology and Basic Medical Sciences, Soochow University, Suzhou, China
- 3Institutes of Biology and Medical Sciences, Soochow University, Suzhou, China
Vascular adhesion protein-1 (VAP-1) is a semicarbazide-sensitive amine oxidase (SSAO), whose enzymatic activity regulates the adhesion/exudation of leukocytes in/from blood vessels. Due to its abundant expressions in vascular systems and prominent roles in inflammations, increasing attentions have been paid to the roles of VAP-1/SSAO in atherosclerosis, a chronic vascular inflammation that eventually drives clinical cardiovascular events. Clinical studies have demonstrated a potential value of soluble VAP-1 (sVAP-1) for the diagnosis and prognosis of cardiovascular diseases. Recent findings revealed that VAP-1 is expressed in atherosclerotic plaques and treatment with VAP-1 inhibitors alleviates the progression of atherosclerosis. This review will focus on the roles of VAP-1/SSAO in the progression of atherosclerotic lesions and therapeutic potentials of VAP-1 inhibitors for cardiovascular diseases.
Introduction
Cardiovascular diseases (CVD) are the leading cause of death worldwide. Most CVD patients die of ischemic heart disease and ischemic stroke (Zhang et al., 2020). The pathological basis of these diseases is atherosclerosis, and the subsequent induction of vascular stenosis or thrombosis leads to clinical acute cardiovascular events. Ongoing pathological studies indicate that atherosclerosis is a chronic inflammatory reaction of the vascular wall in response to dyslipidemia and endothelial distress involving the infiltration of leukocytes and activations of resident vascular cells (Frostegård, 2013). The recruitment of immune cells into atherosclerotic lesions requires the expression of adhesion molecules on endothelial cells. Vascular adhesion protein 1 (VAP-1), encoded by the AOC3 (amine oxidase copper-containing 3) gene, is an endothelial adhesion molecule with amine oxidase activity (Valente et al., 2008). The enzymatic activity of VAP-1 is highly sensitive to inhibition by semicarbazide (SCZ), and VAP-1 thus belongs to a family of semicarbazide-sensitive amine oxidase (SSAO). More and more evidence shows that VAP-1/SSAO is implicated in vascular diseases, probably due to the induction of leukocyte trafficking and vascular damage (Boomsma et al., 2005). This article reviews the involvement of VAP-1/SSAO in atherosclerotic diseases, including stroke and coronary artery disease (CAD), and discusses its role in atherosclerosis as well as the therapeutic potential of VAP-1 inhibitors for CVD.
VAP-1: An Inducible Adhesion Molecule in Inflammation
VAP-1/SSAO is expressed in the vascular system, especially on the surface of endothelial cells, and regulates the adhesion and migration of circulating immune cells (Salmi and Jalkanen, 2019). A variety of in-vitro binding experiments have shown that bindings of lymphocytes, monocytes, and granulocytes to high endothelial venules (HEVs) and/or blood vessels in different tissues are partially dependent on the expression levels of VAP-1 (Salmi et al., 1997a; Salmi et al., 1997b; Salmi et al., 1998; Jaakkola et al., 2000). Real-time imaging shows that VAP-1 mediates the slow-rolling, firm adhesion and migration of leukocytes in blood vessels of lymphoid tissues and inflammatory sites (Stolen et al., 2005). Despite lowly expressed in a subset of venules of normal nonlymphatic tissues like skin, liver, and hearts, VAP-1 is abundant in HEVs of peripheral lymph nodes where the sialic acid residues of VAP-1 interact with counter-receptors on lymphocytes and thereby mediate their homing (Salmi and Jalkanen, 1996; Smith et al., 1998). During inflammation, endothelial VAP-1 is upregulated and its enzymatic activity is indispensable for leukocyte extravasation through endothelium (Salmi and Jalkanen, 2005). Accordingly, mice with Aoc3 deficiency or mutant Aoc3 lacking functional SSAO activity have defects in leukocyte migrations (Noonan et al., 2013). Moreover, inactivation of VAP-1/SSAO by specific inhibitors or neutralizing antibodies significantly reduces a variety of acute and chronic inflammatory diseases in experimental animals (Salmi and Jalkanen, 2019). The enzymatic actions of VAP-1 on leukocyte trafficking appear to be mediated by the production of hydrogen peroxide (Salmi and Jalkanen, 2019). Notably, apart from impaired leukocyte infiltration into inflammatory sites, decreased homing of immune cells to lymphatic organs due to VAP-1 deficiency/inactivation may also attenuate inflammations by inhibiting their activation and proliferation (Koskinen et al., 2007).
Although highly expressed, VAP-1 in both smooth muscle cells (SMCs) and adipocytes does not mediate lymphocyte binding (Jaakkola et al., 1999; Boomsma et al., 2005; Bour et al., 2009). In SMCs, VAP-1 is enriched in caveolae of the plasma membrane, but its physiological functions remain unknown (Salmi and Jalkanen, 2019). In contrast, the expression of VAP-1 is upregulated in adipocytes during differentiation or after incubation with the inflammatory cytokine TNF-α (Abella et al., 2004). In adipocytes, VAP-1 colocalizes with glucose transporter GLUT4 in an endosomal compartment, and its substrates stimulate the recruitment of GLUT4 to the plasma membrane, thereby promoting the uptake of glucose (Enrique-Tarancón et al., 1998; Yu et al., 2004). These insulin-like effects (i.e., increased glucose uptake and lipogenesis) of SSAO substrates on adipocytes require hydrogen peroxide generated in VAP-1’s enzymatic reactions (Zorzano et al., 2003). The potentials of adipose VAP-1 in the management of blood glucose/lipids and obesity thus have received special attentions. Interestingly, deletion of VAP-1 or expression of mutated VAP-1 lacking SSAO activity favors fat deposits in mice on regular chow diet without affecting food intake and glucose handling (Jargaud et al., 2020).
Soluble VAP-1: A Potential Biomarker of CVD
Soluble VAP-1 (sVAP-1) in circulation is produced by proteolytic cleavage of its membrane-bound form (Abella et al., 2004; Stolen et al., 2004b), and plasma levels of sVAP-1 vary in different species (Boomsma et al., 2003). In humans, plasma sVAP-1 concentrations are nicely correlated to SSAO activities in both healthy controls and patients with various diseases (Boomsma et al., 2003; Aalto et al., 2012). Data obtained in mice specifically expressing human VAP-1 (hVAP-1) in different cells revealed that plasma sVAP-1 is mainly derived from endothelial cells under both physiological and inflammatory conditions, with some contributions of adipocytes and SMCs (Göktürk et al., 2003; Stolen et al., 2004b). However, whether the same holds true in humans remains to be resolved. Physiologically, organs like livers, kidneys, and legs are unlikely to be the major source of plasma sVAP-1 in humans, as concentrations of sVAP-1 in arteries and veins of these organs are comparable (Boomsma et al., 2003). We speculate that a substantial amount of sVAP-1 could be produced by HEVs of lymphatic organs, where matrix metalloproteinases (MMPs) required for transendothelial migration of lymphocytes may cut off the membrane-bound VAP-1. In chronic inflammatory liver diseases, higher levels of sVAP-1 in hepatic veins vs. portal veins indicate that inflammation may trigger the production of sVAP-1 by local endothelial and parenchymal cells in inflammatory organs (Kurkijärvi et al., 2000). Conversely, surgical correction of coronary atherosclerotic lesions leads to the lowering of elevated plasma SSAO activities (Boomsma et al., 2003).
Elevated circulating sVAP-1 levels are evident in patients with all kinds of CVD including CAD, arterial stiffness, aortic stenosis, hypertension with echocardiographic alterations, chronic/congestive heart failure, and ischemic/hemorrhagic stroke (Table 1) (Chen et al., 2015). Consistent elevation of plasma sVAP-1 also reflects the severity of congestive/chronic heart failure and calcified aortic stenosis (Boomsma et al., 1997; Boomsma et al., 2000; Altug Cakmak et al., 2015). The importance of VAP-1/SSAO for calcification of human aortic valve is also evidenced by increased VAP-1 expression in the vicinity of calcified aortic valve zones and the decreased calcification of human valvular interstitial cells by SSAO inhibition (Mercier et al., 2020). Histological examinations demonstrated that VAP-1 is highly expressed in vessels of ischemic hearts with dense leukocyte infiltrations (Jaakkola et al., 2000). This contrasts with the reduction of VAP-1 expression in vessels of ischemic brain (Airas et al., 2008), probably due to endothelial VAP-1 shedding by microglial activation and matrix protease generation in acute ischemic stroke (del Zoppo et al., 2007). Interestingly, higher basal plasma activity of VAP-1/SSAO in the acute phase of ischemic stroke predicts the increased incidence of intracranial hemorrhage after intravenous infusion of tissue plasminogen activator (tPA) to restore brain perfusion (Hernandez-Guillamon et al., 2010). Likewise, neurological outcomes of intracerebral hemorrhage are predicted by plasma VAP-1 activities as well (Hernandez-Guillamon et al., 2012). In addition, sVAP-1 is associated with major adverse cardiovascular events (MACE) and mortality in people aged >50 without prior MACE and in patients with type II diabetes (Li et al., 2011; Aalto et al., 2014).
Clinical studies have demonstrated that serum sVAP-1 is a biomarker for atherosclerotic cardiovascular diseases (Table 1). Notably, the associations of sVAP-1 with cardiovascular risk factors and subclinical atherosclerosis are influenced by age, sex, and glucose. In Finnish subjects aged from 30 to 45, men had slightly higher levels of sVAP-1 than women, while opposite changes were observed in Chinese aged >40 (Aalto et al., 2012; Chen et al., 2016). As estrogen levels decline with age in females, higher concentrations of sVAP-1 correspond to low levels of estradiol in Chinese women. Moreover, the use of oral contraceptives in Finnish young women had a negative correlation to sVAP-1 activity, which may explain why levels of sVAP-1 were low and not correlated with age in Finnish women. It thus appears that chronic low-grade inflammations with aging and menopause lead to the elevation of sVAP-1 in women. Interestingly, a negative correlation of sVAP-1 with body mass index (BMI) has been observed in both Finish young and Chinese elder women, suggesting the increase of sVAP-1 may prevent the obesity in females. This anti-obese effect of VAP-1 seems to be estradiol-independent as they are negatively correlated. Rather, it is more likely to be attributed to the release of adipocyte VAP-1, which not only rises sVAP-1 but also reduces its insulin-mimic effects on the differentiation and lipogenesis of adipocytes. Why this effect is absent in males and whether androgen interferes with the generation of sVAP-1 are still perplexing. Notably, in young Finnish, but not elder Chinese, women, sVAP-1 represents an independent determinant of carotid intima-media thickness (IMT) and plaques. By contrast, despite no correlation with glucose in normoglycemic persons, sVAP-1 shows a strong positive association with type 1 and 2 diabetes, and independently determines carotid atherosclerotic plaques in hyperglycemic Chinese (Karádi et al., 2002; Aalto et al., 2012; Chen et al., 2016). Together, all these findings indicate that VAP-1 is involved in the pathogenesis of atherosclerosis.
VAP-1: An Important Player in Atherosclerosis
Atherosclerosis is a vascular inflammation caused by intima lipid accumulation and endothelial cell dysfunction. In humans, fatty streaks appear in the thoracic and abdominal aortas early in childhood, and start to develop in coronary arteries in the second decade (McGill et al., 2000). In healthy aortas, endothelium does not express VAP-1 and the high expression of VAP-1 is confined to SMCs in media (Salmi et al., 1993). Similarly, in-vivo VAP-1 antibody labeling experiments showed the absence of VAP-1 in the aortas of healthy C57BL/6N mice. However, the expression of VAP-1 is induced in endothelial cells lining the atherosclerotic plaques of LDLr−/−ApoB100/100 mice on 4-month Western-type diet (WTD), indicating the involvement of endothelial VAP-1 in leukocyte recruitment to atherosclerotic lesions (Silvola et al., 2016) (Figure 1). Accordingly, contents of activated macrophages in lesions were correlated with the local intensity of VAP-1 in PET imaging using [68Ga]DOTA-Siglec-9 (Silvola et al., 2016). Given the enrichment of VAP-1 in caveolae of the plasma membrane of SMCs (Salmi and Jalkanen, 2019), it is conceivable that the synthetic phenotype switch of SMCs, which reduces the formation of caveolae (Thyberg, 2002), will result in decreased VAP-1 expression in atherosclerotic lesions. Nonetheless, effects of hyperlipidemia and local vascular inflammatory reactions on VAP-1 expression in vascular SMCs are still unknown.
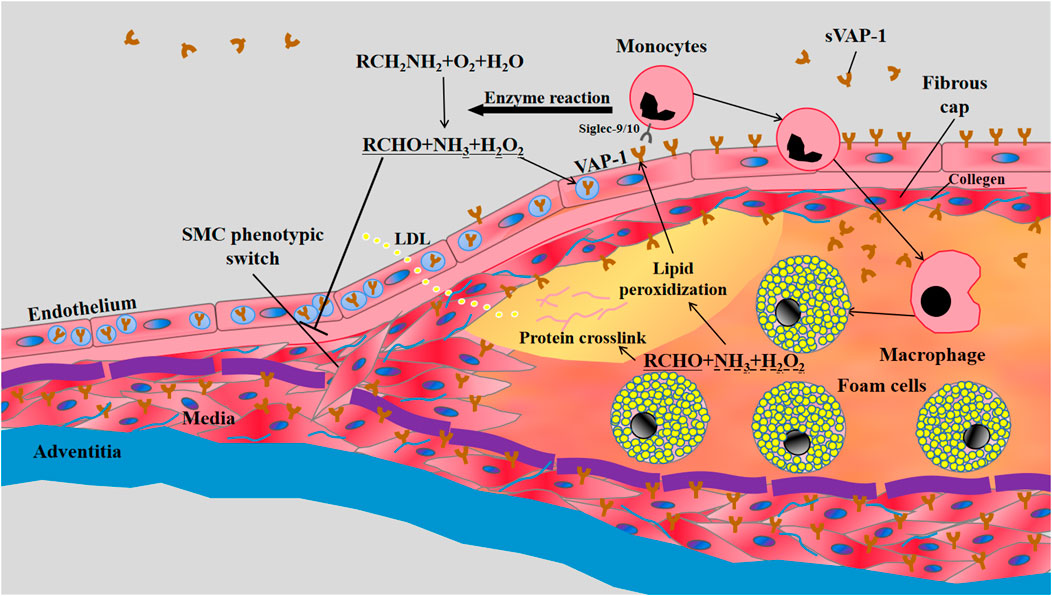
FIGURE 1. The involvement of VAP-1 in atherosclerosis. LDL retention and endothelial dysfunction are the essential initiators of atherosclerosis. The vascular inflammation induces the translocation of VAP-1 onto the luminal surface of endothelium, which subsequently interacts with Siglec-9/10 (sialic acid-binding immunoglobulin-like lectin 9/10) to mediate the infiltrations of monocytes into atherosclerotic lesions. Meanwhile, enzymatic products of VAP-1, such as H2O2, may further increase the expression of VAP-1 on endothelium and the subsequent transmigration of monocytes. The inflammatory early lesions stimulate the transformation of SMCs in media from the quiescent “contractile” phenotype state to the active “synthetic” state with increased proliferation, migration, and collagen synthesis. VAP-1 in the plasma membrane of SMCs may inhibit their phenotypic switch by its enzymatic products and thereby limit the growth of fibrous cap composed of SMCs and collagens. VAP-1 thus represents a pathological factor for plaque instability characterized by thin fibrous cap overlying a large lipid core rich in mon/macrophage-derived foam cells.
The role of VAP-1 in atherosclerosis was first disclosed in transgenic mice overexpressing hVAP-1 on endothelial cells (Stolen et al., 2004a). Compared to control counterparts, endothelial hVAP-1 overexpression did not induce more lesion formations in aortas. Instead, numbers of lesions in transgenic mice were significantly reduced, but the size of each lesion was larger than that in control mice. VAP-1/SSAO in arterial wall may generate hydrogen peroxide, aldehyde, and ammonium. These products are involved in lipid peroxidization, advanced glycation end products (AGEs) generation and protein crosslinking, all of which may lead to endothelial activation and VAP-1 induction. Moreover, higher levels of aorta SSAO and increased turnover of its substrate methylamine were correlated with increased atherosclerosis susceptibility in C57BL/6 mice (Yu and Deng, 1998). VAP-1/SSAO enzymatic reaction is thus thought to be atherogenic by facilitating leukocyte infiltration and vascular damage (Figure 1).
Recently, effects of SSAO inhibitors on the development/progression of atherosclerosis in rodents have been investigated, and findings in these pharmacological studies suggest a pathological role of VAP-1/SSAO activity in atherosclerosis (Supplementary Table 1). By using a classical SSAO inhibitor SCZ, we found that inactivation of VAP-1/SSAO promoted the stability of atherosclerotic lesions with less macrophages but more SMCs and collagen accumulations in LDLr−/− mice on WTD, albeit the ineffectiveness on plasma cholesterol levels and body weight (Zhang et al., 2016). Notably, beyond reducing leukocyte infiltration, VAP-1/SSAO inactivation promotes the synthetic phenotype switch of vascular SMCs, which enhances plaque stability, both in vivo and ex vivo. Moreover, stabilization of established lesions in LDLr−/− mice after lipid lowering was further enhanced by SCZ, accompanied by increased amounts of synthetic SMCs in lesions as well (Peng et al., 2016). These results indicate that the enzymatic products of VAP-1 may promote plaque vulnerability via SMCs (Figure 1). By contrast, in LDLr−/−ApoB100/100 mice on WTD, Sivola et al. demonstrated that 4-weeks treatment with JLP-1586, another VAP-1 inhibitor, had no effect on plaque size, body weight, or plasma lipid levels, but decreased the density of macrophages in atherosclerotic lesions (Silvola et al., 2016). In addition, Wang et al. found that ApoE−/− mice or rabbits treated with PXS-4728A, a specific SSAO inhibitor, exhibited reduced atheroma with less oxidative stress and endothelial dysfunction, and markedly alleviated plasma levels of lipids and glucose (Wang et al., 2018a; Wang et al., 2018b). Given that comparable LDL-C/glucose lowering by atorvastatin resulted in a similar reduction of lesions, it seems that the atheroprotective effect of PXS-4728A is largely attributed to the reduced levels of LDL-C/glucose. Recent studies indicate that hepatic inflammation and nonalcoholic fatty liver disease (NAFLD) may result in hyperglycemia and hyperlipidemia, respectively (Min et al., 2012; Okin and Medzhitov, 2016). VAP-1 mediates hepatic inflammation of NAFLD and its inhibition modifies hepatic steatosis (Weston et al., 2015; Shepherd et al., 2020). Whether, and to which extent, the amelioration of hepatic steatosis and inflammation contributes to LDL-C/glucose lowering of PXS-4728A remains to be investigated. Future investigations on high-fat/cholesterol diet fed mice with a combined deficiency of VAP-1 and LDLr−/− or ApoE−/− will provide direct evidence for the role of VAP-1 in cholesterol/glucose metabolism. Impacts of VAP-1 inhibitors on plasma LDL-C/glucose levels in VAP-1−/−ApoE−/− or VAP-1−/−LDLr−/− mice would help to clarify their target specificity. Given the different lipid/glucose metabolisms as well as inhibitor selectivity between small rodents and humans, LDL-C/glucose lowering effects of different VAP-1 inhibitors should also be tested/confirmed in non-rodent large animals and primates. Clearly, studies on atherosclerotic lesion development in total-body and/or cell-specific VAP-1 knockout mice are still lacking to further corroborate its roles in atherosclerosis.
VAP-1 Inhibitors: A Class of Potential Drugs for CVD
Obesity and diabetes are the major health risks associated with atherosclerotic CVD. Modulations of SSAO activity have been proven to be effective in management of (diabetic) obesity associated with low-grade adipose inflammation (Jargaud et al., 2020). Consequently, adipose inflammation and obesity are remarkably diminished by all kinds of SSAO inhibitors in obese and diabetic rodent models (Supplementary Table 1) (Papukashvili et al., 2020). Interestingly, despite a moderate and long-lasting elevation of serum glucose, the development of atherosclerotic lesions and obesity in diabetic KKAy mice was prevented by two different inhibitors, MDL-72974A, and aminoguanidine, indicating that simple hyperglycemia is not harmful for obesity and atherosclerosis in the absence of VAP-1/SSAO activity (Yu et al., 2002; Yu et al., 2004). However, the exact contribution of adipose SSAO inactivation to obesity-/diabetes-related vascular dysfunction and atherosclerotic lesion development remains unclear.
As outlined above, VAP-1/SSAO inhibitors may prevent adverse clinical events in CVD by reducing the lesion size/inflammation and/or increasing plaque stability. Moreover, VAP-1/SSAO inhibitors have shown beneficial effects on the treatment of CVD, such as stroke and myocardial infarction after onset, as well. In a rat embolic stroke model treated with tPA, SCZ prevented adverse effects caused by delayed tPA administration, leading to a smaller infarct volume (Hernandez-Guillamon et al., 2010). The administration of two VAP-1 inhibitors, LJP-1586, and SCZ, at 1 h after induction of intracerebral hemorrhage significantly reduced brain inflammation and edema as well as neurobehavioral deficits (Ma et al., 2011). Even at 6 h post-subarachnoid hemorrhage, administration of LJP-1586 improved the neurological outcome by 25% (Xu et al., 2014). Additionally, myocardial SSAO activity was increased in myocardial ischemia-reperfusion injury, and administration of SCZ, hydralazine, or LJP-1207, reduced the myocardial infarct size in vivo (Yang et al., 2011).
So far, clinical trials investigating hVAP-1-targeting therapeutics on CVD have not yet been initiated. Most inhibitors tested in preclinical studies could not proceed into clinical trials because of potential off-target toxicity or the appearance of better substitutions (Vakal et al., 2020). Nonetheless, PXS-4728A was safe and showed a long-lasting inhibitory effect in phase I and IIa clinical trials in NASH (non-alcoholic steatohepatitis). Unfortunately, this clinical investigation was reported to be discontinued due to the potential harmful interaction of PXS-4728A with a drug used by NASH patients (Vakal et al., 2020). Notably, a phase IIa trial of PXS-4728A in diabetic nephropathy is still ongoing. Analyzing its effect on atherosclerotic risk factors in these clinical studies will provide more insights into the therapeutic potentials of VAP-1 inhibition for CVD.
Conclusions and Future Perspectives
SSAO/VAP-1 is involved in the development of atherosclerosis. The enzymatic activity of VAP-1/SSAO facilitates the transendothelial migration of leukocytes, while the toxic products generated may inhibit the phenotypic switch of vascular SMCs. Extensive clinical studies have demonstrated the potential value of sVAP-1 in diagnosis and prognosis of CVD. Moreover, prevention of atherosclerosis and obesity, as well as the improvement of infarcts and injury after onset of CVD are evident in rodents treated with VAP-1 inhibitors. However, cell/stage-specific effects of VAP-1 and impacts of its inhibitors on atherosclerosis, as well as underlying mechanisms, warrant further investigations in rodents and non-rodent animals.
Author Contributions
HL and SD drafted the manuscript and generated the figures and tables; PN and XG discussed literatures; JW and YZ designed the work, drafted and revised the manuscript.
Funding
This study was financially supported by the National Key Research and Development Program of China (2017YFB0403805) (JW); National Natural Science Foundation of China (81470564/81970371(YZ) and 31670888 (JW)); Key University Science Research Project of Jiangsu Province (20KJB320005) (HL); and a project funded by the Priority Academic Program Development of Jiangsu Higher Education Institutions (JW and YZ). The funders had no role in study design, data collection and analysis, decision to publish, or preparation of the article.
Conflict of Interest
The authors declare that the research was conducted in the absence of any commercial or financial relationships that could be construed as a potential conflict of interest.
Supplementary Material
The Supplementary Material for this article can be found online at: https://www.frontiersin.org/articles/10.3389/fphar.2021.679707/full#supplementary-material
References
Aalto, K., Havulinna, A. S., Jalkanen, S., Salomaa, V., and Salmi, M. (2014). Soluble Vascular Adhesion Protein-1 Predicts Incident Major Adverse Cardiovascular Events and Improves Reclassification in a Finnish Prospective Cohort Study. Circ. Cardiovasc. Genet. 7, 529–535. doi:10.1161/CIRCGENETICS.113.000543
Aalto, K., Maksimow, M., Juonala, M., Viikari, J., Jula, A., Kähönen, M., et al. (2012). Soluble Vascular Adhesion Protein-1 Correlates with Cardiovascular Risk Factors and Early Atherosclerotic Manifestations. Arterioscler Thromb. Vasc. Biol. 32, 523–532. doi:10.1161/ATVBAHA.111.238030
Abella, A., García-Vicente, S., Viguerie, N., Ros-Baró, A., Camps, M., Palacín, M., et al. (2004). Adipocytes Release a Soluble Form of VAP-1/SSAO by a Metalloprotease-dependent Process and in a Regulated Manner. Diabetologia 47, 429–438. doi:10.1007/s00125-004-1346-2
Airas, L., Lindsberg, P. J., Karjalainen-Lindsberg, M.-L., Mononen, I., Kotisaari, K., Smith, D. J., et al. (2008). Vascular Adhesion Protein-1 in Human Ischaemic Stroke. Neuropathol. Appl. Neurobiol. 34, 394–402. doi:10.1111/j.1365-2990.2007.00911.x
Altug Cakmak, H., Aslan, S., Erturk, M., Ornek, V., Rodi Tosu, A., Kemal Kalkan, A., et al. (2015). Assessment of the Relationship between Serum Vascular Adhesion Protein-1 (VAP-1) and Severity of Calcific Aortic Valve Stenosis. J. Heart Valve Dis. 24, 699–706. doi:10.1016/s0735-1097(16)32187-8
Boomsma, F., Hut, H., Bagghoe, U., van der Houwen, A., and van den Meiracker, A. (2005). Semicarbazide-sensitive Amine Oxidase (SSAO): from Cell to Circulation. Med. Sci. Monit. 11, RA122–6.
Boomsma, F., Bhaggoe, U. M., van der Houwen, A. M. B., and van den Meiracker, A. H. (2003). Plasma Semicarbazide-Sensitive Amine Oxidase in Human (Patho)physiology. Biochim. Biophys. Acta (Bba) - Proteins Proteomics 1647, 48–54. doi:10.1016/s1570-9639(03)00047-5
Boomsma, F., de Kam, P. J., Tjeerdsma, G., van den Meiracker, A. H., and van Veldhuisen, D. J. (2000). Plasma Semicarbazide-Sensitive Amine Oxidase (SSAO) Is an Independent Prognostic Marker for Mortality in Chronic Heart Failure. Eur. Heart J. 21, 1859–1863. doi:10.1053/euhj.2000.2176
Boomsma, F., van Veldhuisen, D. J., de Kam, P. J., Mosterd, A., Lie, K. I., et al. (1997). Man In't VeldPlasma Semicarbazide-Sensitive Amine Oxidase Is Elevated in Patients with Congestive Heart Failure. Cardiovasc. Res. 33, 387–391. doi:10.1016/s0008-6363(96)00209-x
Bour, S., Caspar-Bauguil, S., Iffiú-Soltész, Z., Nibbelink, M., Cousin, B., Miiluniemi, M., et al. (2009). Semicarbazide-sensitive Amine Oxidase/vascular Adhesion Protein-1 Deficiency Reduces Leukocyte Infiltration into Adipose Tissue and Favors Fat Deposition. Am. J. Pathol. 174, 1075–1083. doi:10.2353/ajpath.2009.080612
Carpéné, C., Boulet, N., Chaplin, A., and Mercader, J. (2019). Past, Present and Future Anti-obesity Effects of Flavin-Containing And/or Copper-Containing Amine Oxidase Inhibitors. Medicines 6, 9. doi:10.3390/medicines6010009
Chen, D.-W., Zhao, R.-M., Jin, Y., Zhang, J., Han, C., Jiang, S.-Q., et al. (2015). Plasma Soluble Vascular Adhesion Protein-1 Concentration Correlates with Arterial Stiffness: A Cross-Sectional Study. Arch. Gerontol. Geriatr. 61, 67–71. doi:10.1016/j.archger.2015.04.007
Chen, D. W., Jin, Y., Zhao, R. M., Long, L. J., Zhang, J., Han, C. L., et al. (2016). Age-, Sex- and Glucose-dependent Correlation of Plasma Soluble Vascular Adhesion Protein-1 Concentration with Cardiovascular Risk Factors and Subclinical Atherosclerosis. Eur. Rev. Med. Pharmacol. Sci. 20, 1544–1558.
del Zoppo, G. J., Milner, R., Mabuchi, T., Hung, S., Wang, X., Berg, G. I., et al. (2007). Microglial Activation and Matrix Protease Generation during Focal Cerebral Ischemia. Stroke 38 (2 Suppl. l), 646–651. doi:10.1161/01.STR.0000254477.34231.cb
Enrique-Tarancón, G., Marti, L., Morin, N., Lizcano, J., Unzeta, M., Sevilla, L., et al. (1998). Role of Semicarbazide-Sensitive Amine Oxidase on Glucose Transport and GLUT4 Recruitment to the Cell Surface in Adipose Cells. J. Biol. Chem. 273, 8025–8032. doi:10.1074/jbc.273.14.8025
Frostegård, J. (2013). Immunity, Atherosclerosis and Cardiovascular Disease. BMC. Med. 11, 117. doi:10.1186/1741-7015-11-117
Göktürk, C., Nilsson, J., Nordquist, J., Kristensson, M., Svensson, K., Söderberg, C., et al. (2003). Overexpression of Semicarbazide-Sensitive Amine Oxidase in Smooth Muscle Cells Leads to an Abnormal Structure of the Aortic Elastic Laminas. Am. J. Pathol. 163, 1921–1928. doi:10.1016/S0002-9440(10)63550-X
Hernandez-Guillamon, M., Garcia-Bonilla, L., Solé, M., Sosti, V., Parés, M., Campos, M., et al. (2010). Plasma VAP-1/SSAO Activity Predicts Intracranial Hemorrhages and Adverse Neurological Outcome after Tissue Plasminogen Activator Treatment in Stroke. Stroke 41, 1528–1535. doi:10.1161/STROKEAHA.110.584623
Hernandez-Guillamon, M., Solé, M., Delgado, P., García-Bonilla, L., Giralt, D., Boada, C., et al. (2012). VAP-1/SSAO Plasma Activity and Brain Expression in Human Hemorrhagic Stroke. Cerebrovasc. Dis. 33, 55–63. doi:10.1159/000333370
Jaakkola, K., Jalkanen, S., Kaunismäki, K., Vänttinen, E., Saukko, P., Alanen, K., et al. (2000). Vascular Adhesion Protein-1, Intercellular Adhesion Molecule-1 and P-Selectin Mediate Leukocyte Binding to Ischemic Heart in Humans. J. Am. Coll. Cardiol. 36, 122–129. doi:10.1016/s0735-1097(00)00706-3
Jaakkola, K., Kaunismäki, K., Tohka, S., Yegutkin, G., Vänttinen, E., Havia, T., et al. (1999). Human Vascular Adhesion Protein-1 in Smooth Muscle Cells. Am. J. Pathol. 155, 1953–1965. doi:10.1016/S0002-9440(10)65514-9
Jargaud, V., Bour, S., Tercé, F., Collet, X., Valet, P., Bouloumié, A., et al. (2020). Obesity of Mice Lacking VAP-1/SSAO by Aoc3 Gene Deletion Is Reproduced in Mice Expressing a Mutated Vascular Adhesion Protein-1 (VAP-1) Devoid of Amine Oxidase Activity. J. Physiol. Biochem. 77, 141–154. doi:10.1007/s13105-020-00756-y
Karádi, I., Mészáros, Z., Csányi, A., Szombathy, T., Hosszúfalusi, N., Romics, L., et al. (2002). Serum Semicarbazide-Sensitive Amine Oxidase (SSAO) Activity Is an Independent Marker of Carotid Atherosclerosis. Clinica Chim. Acta 323, 139–146. doi:10.1016/s0009-8981(02)00189-4
Koskinen, K., Nevalainen, S., Karikoski, M., Hänninen, A., Jalkanen, S., and Salmi, M. (2007). VAP-1-deficient Mice Display Defects in Mucosal Immunity and Antimicrobial Responses: Implications for Antiadhesive Applications. J. Immunol. 179, 6160–6168. doi:10.4049/jimmunol.179.9.6160
Kurkijärvi, R., Yegutkin, G. G., Gunson, B. K., Jalkanen, S., Salmi, M., and Adams, D. H. (2000). Circulating Soluble Vascular Adhesion Protein 1 Accounts for the Increased Serum Monoamine Oxidase Activity in Chronic Liver Disease. Gastroenterology 119, 1096–1103. doi:10.1053/gast.2000.18163
Li, H.-Y., Jiang, Y.-D., Chang, T.-J., Wei, J.-N., Lin, M.-S., Lin, C.-H., et al. (2011). Serum Vascular Adhesion Protein-1 Predicts 10-year Cardiovascular and Cancer Mortality in Individuals with Type 2 Diabetes. Diabetes 60, 993–999. doi:10.2337/db10-0607
Li, H.-Y., Lin, M.-S., Wei, J.-N., Hung, C. S., Chiang, F.-T., Lin, C.-H., et al. (2009). Change of Serum Vascular Adhesion Protein-1 after Glucose Loading Correlates to Carotid Intima-Medial Thickness in Non-diabetic Subjects. Clinica Chim. Acta 403, 97–101. doi:10.1016/j.cca.2009.01.027
Ma, Q., Manaenko, A., Khatibi, N. H., Chen, W., Zhang, J. H., and Tang, J. (2011). Vascular Adhesion Protein-1 Inhibition Provides Antiinflammatory protection after an Intracerebral Hemorrhagic Stroke in Mice. J. Cereb. Blood Flow Metab. 31, 881–893. doi:10.1038/jcbfm.2010.167
Maciorkowska, D., Zbroch, E., and Malyszko, J. (2015). Circulating Renalase, Catecholamines, and Vascular Adhesion Protein 1 in Hypertensive Patients. J. Am. Soc. Hypertens. 9, 855–864. doi:10.1016/j.jash.2015.08.002
McGill, H. C., McMahan, C. A., Herderick, E. E., Tracy, R. E., Malcom, G. T., Zieske, A. W., et al. (2000). Effects of Coronary Heart Disease Risk Factors on Atherosclerosis of Selected Regions of the Aorta and Right Coronary Artery. Arterioscler Thromb. Vasc. Biol. 20, 836–845. doi:10.1161/01.atv.20.3.836
Mercier, N., Pawelzik, S.-C., Pirault, J., Carracedo, M., Persson, O., Wollensack, B., et al. (2020). Semicarbazide-Sensitive Amine Oxidase Increases in Calcific Aortic Valve Stenosis and Contributes to Valvular Interstitial Cell Calcification. Oxidative Med. Cell Longevity 2020, 1–9. doi:10.1155/2020/5197376
Min, H.-K., Kapoor, A., Fuchs, M., Mirshahi, F., Zhou, H., Maher, J., et al. (2012). Increased Hepatic Synthesis and Dysregulation of Cholesterol Metabolism Is Associated with the Severity of Nonalcoholic Fatty Liver Disease. Cel Metab. 15, 665–674. doi:10.1016/j.cmet.2012.04.004
Noonan, T., Lukas, S., Peet, G. W., Pelletier, J., Panzenbeck, M., Hanidu, A., et al. (2013). The Oxidase Activity of Vascular Adhesion Protein-1 (VAP-1) Is Essential for Function. Am. J. Clin. Exp. Immunol. 2, 172–185.
Okin, D., and Medzhitov, R. (2016). The Effect of Sustained Inflammation on Hepatic Mevalonate Pathway Results in Hyperglycemia. Cell 165, 343–356. doi:10.1016/j.cell.2016.02.023
Papukashvili, D., Rcheulishvili, N., and Deng, Y. (2020). Attenuation of Weight Gain and Prevention of Associated Pathologies by Inhibiting SSAO. Nutrients 12, 184. doi:10.3390/nu12010184
Peng, Y., Wang, J., Zhang, M., Niu, P., Yang, M., Yang, Y., et al. (2016). Inactivation of Semicarbazide-Sensitive Amine Oxidase Stabilizes the Established Atherosclerotic Lesions via Inducing the Phenotypic Switch of Smooth Muscle Cells. PloS one 11, e0152758. doi:10.1371/journal.pone.0152758
Salmi, M., Hellman, J., and Jalkanen, S. (1998). The Role of Two Distinct Endothelial Molecules, Vascular Adhesion Protein-1 and Peripheral Lymph Node Addressin, in the Binding of Lymphocyte Subsets to Human Lymph Nodes. J. Immunol. 160, 5629–5636.
Salmi, M., and Jalkanen, S. (2005). Cell-surface Enzymes in Control of Leukocyte Trafficking. Nat. Rev. Immunol. 5, 760–771. doi:10.1038/nri1705
Salmi, M., and Jalkanen, S. (1996). Human Vascular Adhesion Protein 1 (VAP-1) Is a Unique Sialoglycoprotein that Mediates Carbohydrate-dependent Binding of Lymphocytes to Endothelial Cells. J. Exp. Med. 183, 569–579. doi:10.1084/jem.183.2.569
Salmi, M., and Jalkanen, S. (2019). Vascular Adhesion Protein-1: A Cell Surface Amine Oxidase in Translation. Antioxid. Redox Signaling 30, 314–332. doi:10.1089/ars.2017.7418
Salmi, M., Kalimo, K., and Jalkanen, S. (1993). Induction and Function of Vascular Adhesion Protein-1 at Sites of Inflammation. J. Exp. Med. 178, 2255–2260. doi:10.1084/jem.178.6.2255
Salmi, M., Rajala, P., and Jalkanen, S. (1997a). Homing of Mucosal Leukocytes to Joints. Distinct Endothelial Ligands in Synovium Mediate Leukocyte-Subtype Specific Adhesion. J. Clin. Invest. 99, 2165–2172. doi:10.1172/JCI119389
Salmi, M., Tohka, S., Berg, E. L., Butcher, E. C., and Jalkanen, S. (1997b). Vascular Adhesion Protein 1 (VAP-1) Mediates Lymphocyte Subtype-specific, Selectin-independent Recognition of Vascular Endothelium in Human Lymph Nodes. J. Exp. Med. 186, 589–600. doi:10.1084/jem.186.4.589
Shepherd, E. L., Karim, S., Newsome, P. N., and Lalor, P. F. (2020). Inhibition of Vascular Adhesion Protein-1 Modifies Hepatic Steatosis In Vitro and In Vivo. Wjh 12, 931–948. doi:10.4254/wjh.v12.i11.931
Silvola, J. M. U., Virtanen, H., Siitonen, R., Hellberg, S., Liljenbäck, H., Metsälä, O., et al. (2016). Leukocyte Trafficking-Associated Vascular Adhesion Protein 1 Is Expressed and Functionally Active in Atherosclerotic Plaques. Sci. Rep. 6, 35089. doi:10.1038/srep35089
Stolen, C. M., Madanat, R., Marti, L., Kari, S., Yegutkin, G. G., Sariola, H., et al. (2004a). Semicarbazide Sensitive Amine Oxidase Overexpression Has Dual Consequences: Insulin Mimicry and Diabetes-like Complications. FASEB J. 18, 702–704. doi:10.1096/fj.03-0562fje
Stolen, C. M., Marttila-Ichihara, F., Koskinen, K., Yegutkin, G. G., Turja, R., Bono, P., et al. (2005). Absence of the Endothelial Oxidase AOC3 Leads to Abnormal Leukocyte Traffic In Vivo. Immunity 22, 105–115. doi:10.1016/j.immuni.2004.12.006
Stolen, C. M., Yegutkin, G. G., Kurkijärvi, R., Bono, P., Alitalo, K., and Jalkanen, S. (2004b). Origins of Serum Semicarbazide-Sensitive Amine Oxidase. Circ. Res. 95, 50–57. doi:10.1161/01.RES.0000134630.68877.2F
Thyberg, J. (2002). Caveolae and Cholesterol Distribution in Vascular Smooth Muscle Cells of Different Phenotypes. J. Histochem. Cytochem. 50 (2), 185–195. doi:10.1177/002215540205000206
Vakal, S., Jalkanen, S., Dahlström, K. M., and Salminen, T. A. (2020). Human Copper-Containing Amine Oxidases in Drug Design and Development. Molecules 25 (6), 1293. doi:10.3390/molecules25061293
Valente, T., Solé, M., and Unzeta, M. (2008). SSAO/VAP-1 Protein Expression during Mouse Embryonic Development. Dev. Dyn. 237, 2585–2593. doi:10.1002/dvdy.21682
Wang, S.-H., Yu, T.-Y., Hung, C.-S., Yang, C.-Y., Lin, M.-S., Su, C.-Y., et al. (2018a). Inhibition of Semicarbazide-Sensitive Amine Oxidase Reduces Atherosclerosis in Cholesterol-Fed New Zealand White Rabbits. Sci. Rep. 8, 9249. doi:10.1038/s41598-018-27551-6
Wang, S.-H., Yu, T.-Y., Tsai, F.-C., Weston, C. J., Lin, M.-S., Hung, C.-S., et al. (2018b). Inhibition of Semicarbazide-Sensitive Amine Oxidase Reduces Atherosclerosis in Apolipoprotein E-Deficient Mice. Translational Res. 197, 12–31. doi:10.1016/j.trsl.2018.03.001
Weston, C. J., Shepherd, E. L., Claridge, L. C., Rantakari, P., Curbishley, S. M., Tomlinson, J. W., et al. (2015). Vascular Adhesion Protein-1 Promotes Liver Inflammation and Drives Hepatic Fibrosis. J. Clin. Invest. 125, 501–520. doi:10.1172/JCI73722
Xu, H.-L., Garcia, M., Testai, F., Vetri, F., Barabanova, A., Pelligrino, D. A., et al. (2014). Pharmacologic Blockade of Vascular Adhesion Protein-1 Lessens Neurologic Dysfunction in Rats Subjected to Subarachnoid Hemorrhage. Brain Res. 1586, 83–89. doi:10.1016/j.brainres.2014.08.036
Yang, W., Li, H., Luo, H., and Luo, W. (2011). Inhibition of Semicarbazide-Sensitive Amine Oxidase Attenuates Myocardial Ischemia-Reperfusion Injury in an In Vivo Rat Model. Life Sci. 88, 302–306. doi:10.1016/j.lfs.2010.12.003
Yu, P. H., and Deng, Y. L. (1998). Endogenous Formaldehyde as a Potential Factor of Vulnerability of Atherosclerosis: Involvement of Semicarbazide-Sensitive Amine Oxidase-Mediated Methylamine Turnover. Atherosclerosis 140, 357–363. doi:10.1016/s0021-9150(98)00142-7
Yu, P. H., Wang, M., Fan, H., Deng, Y., and Gubisne-Haberle, D. (2004). Involvement of SSAO-Mediated Deamination in Adipose Glucose Transport and Weight Gain in Obese Diabetic KKAy Mice. Am. J. Physiology-Endocrinology Metab. 286, E634–E641. doi:10.1152/ajpendo.00272.2003
Yu, P., Wang, M., Deng, Y., Fan, H., and Shira-Bock, L. (2002). Involvement of Semicarbazide-Sensitive Amine Oxidase-Mediated Deamination in Atherogenesis in KKAy Diabetic Mice Fed with High Cholesterol Diet. Diabetologia 45, 1255–1262. doi:10.1007/s00125-002-0903-9
Zhang, L., Li, Q., Han, X., Wang, S., Li, P., Ding, Y., et al. (2020). Associations of Socioeconomic Factors with Cause-specific Mortality and burden of Cardiovascular Diseases: Findings from the Vital Registration in Urban Shanghai, China, during 1974-2015. BMC Public Health 20, 1291. doi:10.1186/s12889-020-09390-1
Zhang, M., Liu, L., Zhi, F., Niu, P., Yang, M., Zhu, X., et al. (2016). Inactivation of Semicarbazide-Sensitive Amine Oxidase Induces the Phenotypic Switch of Smooth Muscle Cells and Aggravates the Development of Atherosclerotic Lesions. Atherosclerosis 249, 76–82. doi:10.1016/j.atherosclerosis.2016.03.039
Zorzano, A., Abella, A., Marti, L., Carpéné, C., Palacín, M., and Testar, X. (2003). Semicarbazide-sensitive Amine Oxidase Activity Exerts Insulin-like Effects on Glucose Metabolism and Insulin-Signaling Pathways in Adipose Cells. Biochim. Biophys. Acta (Bba) - Proteins Proteomics 1647, 3–9. doi:10.1016/s1570-9639(03)00039-6
Keywords: VAP-1, inflammation, atherosclerosis, myocardial infarction, stroke
Citation: Li H, Du S, Niu P, Gu X, Wang J and Zhao Y (2021) Vascular Adhesion Protein-1 (VAP-1)/Semicarbazide-Sensitive Amine Oxidase (SSAO): A Potential Therapeutic Target for Atherosclerotic Cardiovascular Diseases. Front. Pharmacol. 12:679707. doi: 10.3389/fphar.2021.679707
Received: 12 March 2021; Accepted: 28 June 2021;
Published: 08 July 2021.
Edited by:
Jian Gao, Second Affiliated Hospital of Dalian Medical University, ChinaReviewed by:
Pengfei Zuo, Zhongda Hospital, Southeast University, ChinaYanan Wang, Xi’an Jiaotong University Health Science Center, China
Sheng Wang, Fifth People’s Hospital of Suzhou, China
Xiang-An Li, University of Kentucky, United States
Copyright © 2021 Li, Du, Niu, Gu, Wang and Zhao. This is an open-access article distributed under the terms of the Creative Commons Attribution License (CC BY). The use, distribution or reproduction in other forums is permitted, provided the original author(s) and the copyright owner(s) are credited and that the original publication in this journal is cited, in accordance with accepted academic practice. No use, distribution or reproduction is permitted which does not comply with these terms.
*Correspondence: Jun Wang, andhbmc3OUBzdWRhLmVkdS5jbg==; Ying Zhao, eXpoYW9Ac3VkYS5lZHUuY24=
†These authors have contributed equally to this work