- 1Department of Physiology, Faculty of Medicine, Universiti Kebangsaan Malaysia, Cheras, Malaysia
- 2Department of Pharmacology, Faculty of Medicine, Universiti Kebangsaan Malaysia, Cheras, Malaysia
Exposure to cigarette smoke is an important risk factor for cardiovascular diseases. Nicotine is an addictive compound in cigarette smoke that triggers oxidative stress, which leads to vascular dysfunction. Piper sarmentosum Roxb. is a herb with antioxidant and vascular protective effects. This study evaluated the potential protective effect of the aqueous extract of P. sarmentosum leaf (AEPS) on vascular dysfunction in rats induced with prolonged nicotine administration. A total of 22 male Sprague-Dawley rats were divided into control (normal saline, oral gavage [p.o.]), nicotine (0.8 mg/kg/day nicotine, intraperitoneally [i.p.]), and nicotine + AEPS groups (250 mg/kg/day AEPS, p.o. + 0.8 mg/kg/day nicotine, i.p.). Treatment was given for 21 days. Thoracic aortae were harvested from the rats for the measurement of vasorelaxation, vascular nitric oxide (NO) level, and antioxidant level and the assessment of vascular remodeling. Rats treated with AEPS had improved vasorelaxation to endothelium-dependent vasodilator, acetylcholine (ACh), compared with the nicotine-induced rats (p < 0.05). The presence of endothelium increased the maximum relaxation of aortic rings in response to ACh. Compared with the nicotine group, AEPS enhanced vascular NO level (p < 0.001) and increased antioxidant levels as measured by superoxide dismutase activity (p < 0.05), catalase activity (p < 0.01), and reduced glutathione level (p < 0.05). No remarkable changes in aortic histomorphometry were detected. In conclusion, P. sarmentosum attenuates vascular endothelial dysfunction in nicotine-induced rats by improving vasorelaxation and enhancing vascular NO and antioxidant levels.
Introduction
Tobacco smoking contributes to eight million of annual deaths worldwide. More than seven million deaths are attributed to the direct effects of smoking, and more than 1.2 million premature deaths of adults and children due to exposure to secondhand smoke have been recorded annually (World Health Organization, 2019). Nicotine is the main ingredient in tobacco smoke that causes addiction (Hecht, 2003). In addition, tobacco smoking and exposure to nicotine increase the risk of cardiovascular diseases (CVD), such as atherosclerosis, ischemic heart disease, hypertension, and stroke (Son and Lee, 2020).
The effect of nicotine on the cardiovascular system is mediated by its binding to endogenous nicotinic acetylcholine receptors (nAChRs). NAChRs are expressed by vascular endothelial cells (EC) and vascular smooth muscle cells (VSMC); thus, ECs and VSMCs are the direct targets of nicotine-induced vascular dysfunction (Brüggmann et al., 2003; Moccia et al., 2004). The presentations of nicotine-induced vascular dysfunction include changes in vasoreactivity and vascular remodeling (Whitehead et al., 2021).
Nicotine impacts the survival, proliferation, migration, and matrix production of ECs and VSMCs and leads to vascular remodeling. Acute exposure to nicotine promotes the angiogenic response of the endothelium, whereas chronic exposure blunts pro-angiogenic response. Acute and chronic nicotine exposures lead to fibroblast proliferation, extracellular matrix deposition, VSMC proliferation and migration, and neointima formation (Cucina et al., 2000; Ng et al., 2007; Rodella et al., 2012). In addition, chronic nicotine infusion induces matrix metalloproteinase (MMP)-2 and MMP-9 expression and activity. MMPs cause elastin degradation in the aortic wall and irreversible aortic stiffening (Wagenhauser et al., 2018).
Concerning vascular reactivity, nicotine impairs vasorelaxation through endothelium-dependent and -independent mechanisms. The endothelium secretes various vasoactive substances that move to the underlying VSMC to induce vasoconstriction or vasorelaxation (Wright et al., 2004). Nicotine stimulates the release of the vasoconstrictor, endothelin-1, and inhibits the synthesis of the vasorelaxants, nitric oxide (NO) and prostacyclin, from the endothelium (Su and Wang, 1991; Toda and Toda, 2010; Durand and Gutterman, 2013). As for the endothelium-independent mechanisms, nicotine promotes vasoconstriction by activating voltage-gated potassium channels and enhancing VSMC response to norepinephrine (Mayhan and Sharpe, 2002; Olfert et al., 2018).
Oxidative stress is the underlying pathogenesis of nicotine-induced impaired vasorelaxation (Carnevale et al., 2016). Nicotine stimulates excessive reactive oxygen species (ROS) production and lowers antioxidant levels to cause oxidative stress (Tonnessen et al., 2000). Multiple studies suggested that excessive ROS contributes to the nicotine-induced impairment of NO-mediated, endothelium-dependent vasorelaxation (Jiang et al., 2006; Toda and Toda, 2010). Cells produce a system of endogenous antioxidants to neutralize ROS. Among these antioxidants are superoxide dismutase (SOD), catalase (CAT), and reduced glutathione (GSH) (Aguilar et al., 2016). Nicotine exposure reduces aortic SOD and GSH levels, and this finding is associated with the impairment of the endothelium-dependent vasorelaxation of the aorta (Zainalabidin et al., 2014).
Piper sarmentosum Roxb. is a herbaceous plant that is widely used in Chinese traditional medicine to treat fever, cough, and pleurisy. Pharmacologically, the herb has various vascular protective effects (Peungvicha et al., 1998; Mohd Zainudin et al., 2013). The aqueous extract of P. sarmentosum leaf (AEPS) has high antioxidant activity (Ismail et al., 2018) and can stimulate NO production in oxidative stress-induced endothelial cells (Ugusman et al., 2010). AEPS also reduces the formation of atherosclerotic lesions in hypercholesterolemic rabbits (Amran et al., 2010).
Even though AEPS has vascular protective effects in various experimental models, the potential of AEPS in attenuating nicotine-induced vascular dysfunction, including impaired vasorelaxation and vascular remodeling, has not been studied. Nicotine in tobacco smoke causes direct harm to smokers and, unfortunately, has harmful effects on passive smokers or people who are inadvertently exposed to environmental tobacco smoke (World Health Organization, 2019). We hypothesized that AEPS can protect against the vascular dysfunction caused by nicotine. Therefore, this study determined the effect of AEPS on vasorelaxation, vascular NO level, and antioxidant levels, as well as vascular remodeling, in nicotine-induced rats. Findings from this study will support the potential use of AEPS as a supplement to prevent nicotine-induced vascular dysfunction in people who are inadvertently exposed to tobacco smoke.
Materials and Methods
Preparation and Analysis of Aqueous extract of Piper sarmentosum Roxb. leaf
P. sarmentosum leaves were supplied by Herbagus Sdn. Bhd., Penang, Malaysia and identified by a plant taxonomist in the Herbarium of Universiti Kebangsaan Malaysia (UKM; specimen voucher number: UKMB40240). AEPS was prepared according to a previous method (Ugusman et al., 2011). Fresh P. sarmentosum leaves were sun-dried and ground into powder. The powder was mixed with distilled water in a ratio of 1:10 (w/v) and heated at 80°C for 3 h. The extract was filtered and concentrated repeatedly and then freeze-dried and kept at 4°C. Liquid chromatography–mass spectrometry (LCMS)–Orbitrap full-scan analysis was conducted to identify the compounds in AEPS, and the results have been published previously (Sundar et al., 2019).
Animals and Study Design
The study was approved by the Animal Ethics Committee of UKM (approval code: PP/FISIO/2018/AZIZAH/26-SEPT./957-SEPT.-2018-SEPT.-2019). Twenty-two male Sprague-Dawley rats (250–300 g) were obtained from the Animal Resource Unit of UKM. Each rat was kept in a cage and maintained under standard conditions of a 12 h light and 12 h dark cycle. The rats were fed on a standard rat chow diet with water ad libitum. The rats were randomly divided into three groups (n = 6–8 per group): the control group was given normal saline; the nicotine group was given 0.8 mg/kg/day nicotine (Tokyo Chemical Industry, Japan) intraperitoneally (i.p.); and the AEPS group was fed with 250 mg/kg/day AEPS by oral gavage (p.o.) 30 min before treatment with 0.8 mg/kg/day nicotine (i.p.). Treatment was continued daily for 21 days. The dosage and duration of nicotine treatment mimic the exposure of a chronic light smoker and has been proven to cause vascular dysfunction in a previous study (Moon et al., 2014). The dose of AEPS was chosen based on the optimal dose that improved vasorelaxation in nicotine-induced rats (Supplementary Figure S1, Supplementary Table S1). On day 22, the rats were terminally anaesthetized with intravenous injection of ketamine and xylazine cocktail (0.2 ml/kg BW). The thoracic aortae were then harvested and cleaned from the surrounding fat and connective tissues. Parts of the fresh aortic tissues were immediately used for wire myography. The remaining aortic tissues were used for vascular NO, antioxidant, and histological analyses.
Measurement of Mean Systolic Blood Pressure
MSBP was measured in conscious rats on day 21 by using the CODA II™ Non-Invasive Blood Pressure System (Kent Scientific Corporation, United States). MSBP values were then used for aortic morphometry analysis.
Wire Myography
The thoracic aortae were cut into 2 mm rings. Some rings were endothelium-denuded, whereas others had intact endothelium. The endothelium was removed by gently rubbing the interior of the vessel around a wire, and removal was confirmed by the lack of a vasodilator response to 10–6 M acetylcholine (ACh; Tokyo Chemical Industry, Japan). The aortic rings were mounted on two stainless steel pins in a four-channel wire myograph (Danish Myo Technology, United States). The vessels were bathed in physiological Krebs solution with the following composition: 118 mM NaCl, 4.7 mM KCl, 11 mM glucose, 1.2 mM MgSO4, 25 mM NaHCO3, 1.03 mM KH2PO4, and 2.5 mM CaCl2. Then, the vessels were gassed continuously with 95% O2 and 5% CO2 at 37°C. The aortic rings were set to an optimum tension of 1 g and allowed to equilibrate for at least 30 min before use (Almabrouk et al., 2018). After the calibration, the viability of the aortic rings was tested by adding 40 mM KCl. The vessels were then contracted with 10–6 M phenylephrine (Sigma, United States) before starting experiments. The cumulative concentration–relaxation curves to the endothelium-dependent vasodilator, ACh (10–9–10–6 M), and the endothelium-independent vasodilator, sodium nitroprusside (SNP; 10–9–10–6 M; Sigma, United States), added at 3 min intervals were constructed. Power-Lab Data Acquisition System (ADInstruments, Australia) was used to measure and record the changes in vessel tension. Vasorelaxation data were expressed as the percentage loss of phenylephrine-induced contraction.
Preparation of Tissue Lysates
Aortic tissue lysates were prepared based on previous methods (Zainalabidin et al., 2014). The tissues were weighed and crushed into powder form using a mortar and pestle in liquid nitrogen. Then, phosphate-buffered saline (PBS; 0.1 M, pH 7.4) was added to the tissue powder in a ratio of 1:9 (w/v) and centrifuged for 10 min at 4°C. The protein concentration in the aortic tissue lysates was measured using Bradford assay (Bradford, 1976).
Measurement of Vascular Nitric Oxide Level
The concentration of NO in the aortic tissue lysates was measured indirectly using Nitrite/Nitrate Colorimetric Assay Kit (Sigma, United States) according to the manufacturer’s instructions. The whole aorta was used for this assay without specifically isolating the endothelial layer. The principle of this assay is based on the measurement of total nitrite in the samples. Nitrate reductase was used to reduce nitrate to nitrite. Total nitrite was measured at 540 nm after the addition of Griess reagent.
Measurement of Vascular Antioxidant Levels
SOD activity in the aortic tissue lysates was measured as described previously (Beyer and Fridovich, 1987). Briefly, the tissue lysates were mixed with SOD substrate containing PBS–EDTA, riboflavin, L-methionine, Triton-X, and nitro blue tetrazolium (NBT). SOD activity in the samples was determined on the basis of one unit of an enzyme that inhibited 50% of NBT reduction and expressed as unit per milligram protein. CAT activity in the aortic samples was tested on the basis of a previous method (Aebi, 1984). The samples were mixed into H2O2, and the disappearance of H2O2 was measured spectrophotometrically at 240 nm. GSH level in the aortic tissue lysates was measured as described previously (Ellman, 1959). The samples were mixed with 5,5-dithio-bis-[2-nitrobenzoic acid] for 15 min. Subsequently, the absorbance values of the samples were measured at 415 nm using a microplate reader.
Histological Analysis
Thoracic aortae were fixed in 10% formalin, dehydrated, and embedded in paraffin wax. Aortic sections were cut on a rotary microtome and stained with hematoxylin and eosin. The sections were photographed using an Olympus SZ61TR-TP051000 microscope (Olympus, Japan), and the images were analyzed using Life Science Olympus cellSens Standard software (Olympus, Japan). The morphometric parameters of the aorta, including intima–media thickness (IMT), intima–media area (IMA), lumen diameter (d), circumference wall tension (CWT), and tensile stress (TS), were measured based on previous protocols (Zainalabidin et al., 2014). The four quadrants of the aortic lumen at 0°, 90°, 180°, and 270° between the layers of the tunica media and tunica intima were measured, and the average readings were recorded as the IMT value. Lumen area (α) was calculated by drawing a circular line over the tunica intima layer. Lumen diameter (d) was calculated using the formula
Statistical Analysis
The data were analyzed using GraphPad Prism software version 7. The results are presented as mean ± standard error of the mean (SEM). The myography data were analyzed using two-way ANOVA followed by Tukey’s post hoc test. For other data, unpaired t-test was used to compare the means. p < 0.05 was considered statistically significant.
Results
Effect of Aqueous Extract of Piper sarmentosum Roxb. Leaf on Vasorelaxation
The endothelium-intact aortic rings of rats treated with AEPS had higher relaxation response to ACh compared with the nicotine group (p < 0.05). The endothelium-intact aortic rings of nicotine-induced rats showed lower relaxation response to ACh compared with the control group (p < 0.05) (Figure 1A). Besides, the endothelium-denuded aortic rings of rats treated with AEPS had higher relaxation response to ACh compared with the nicotine group (p < 0.001). Endothelium-denuded aortic rings of nicotine-induced rats showed lower relaxation response to ACh compared with the control group (p < 0.01, Figure 1B). The maximum relaxation (Rmax) of the vessels with ACh was remarkably higher in endothelium-intact vessels than in endothelium-denuded vessels in all groups (Table 1). No remarkable difference was observed in vasorelaxation toward endothelium-independent vasodilator, SNP, in all groups (Figures 1C,D). The presence or absence of endothelium also did not affect the maximum relaxation to SNP in all groups (Table 1).
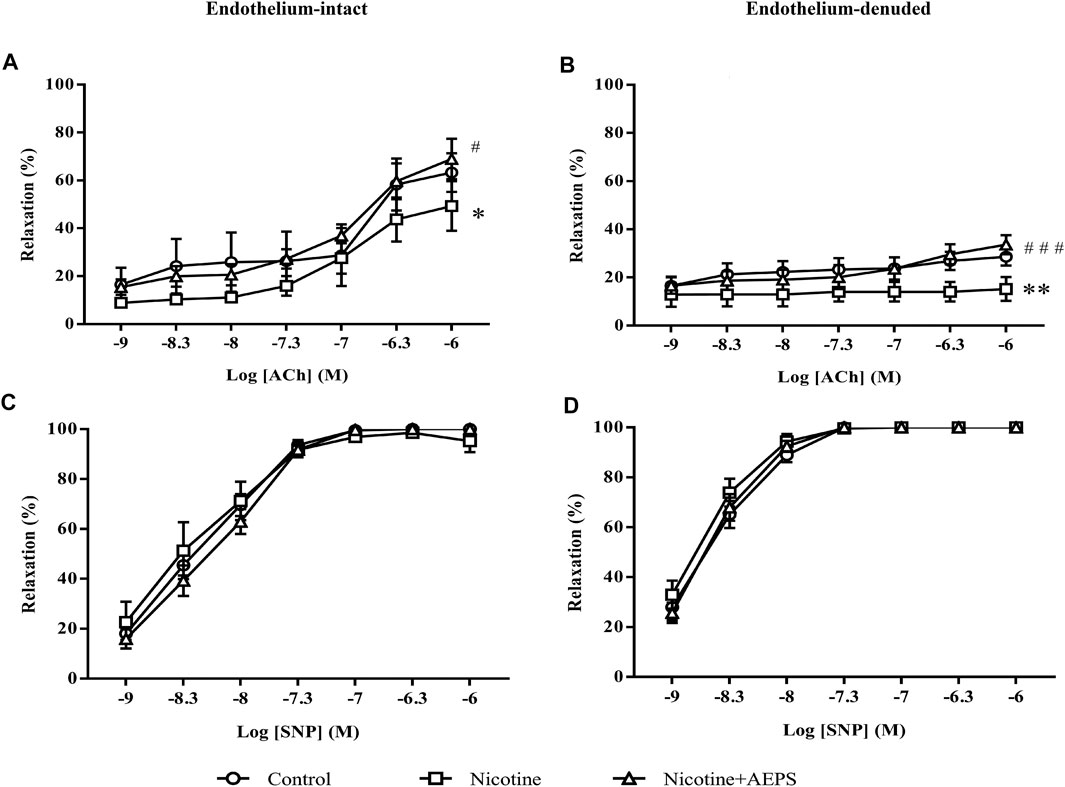
FIGURE 1. Concentration-relaxation curves to the endothelium-dependent vasodilator ACh (A, B) and endothelium-independent vasodilator SNP (C, D) in rat aortic rings from each group. The values are given as mean ± SEM, n = 6–8 for each group. *p < 0.05, **p < 0.01 compared with the control group; #p < 0.05, ###p < 0.001 compared with the nicotine group. (See Table 1 for comparison of maximum relaxation among the groups).
Effect of Aqueous Extract of Piper sarmentosum Roxb. Leaf on Vascular Nitric Oxide Level
Rats treated with AEPS had significantly higher vascular NO level compared with nicotine-administered rats (p < 0.001). However, nicotine administration did not cause any remarkable change in vascular NO level compared with the control (Figure 2).
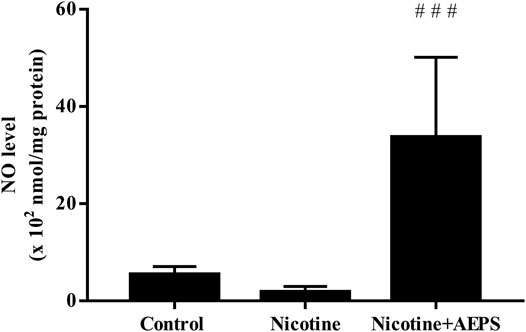
FIGURE 2. Level of nitric oxide (NO) in rat aortic tissues. The values are given as mean ± SEM, n = 6–8 for each group. ###p < 0.001 compared with the nicotine group.
Effect of Aqueous Extract of Piper sarmentosum Roxb. Leaf on Vascular Antioxidant Levels
Treatment with AEPS significantly improved vascular antioxidant levels as shown by the enhanced SOD activity (p < 0.05), CAT activity (p < 0.05), and GSH level (p < 0.05) compared with the nicotine group. Nicotine significantly reduced vascular SOD activity (p < 0.05) but not CAT activity and GSH levels compared with the control group (Figures 3A–C).
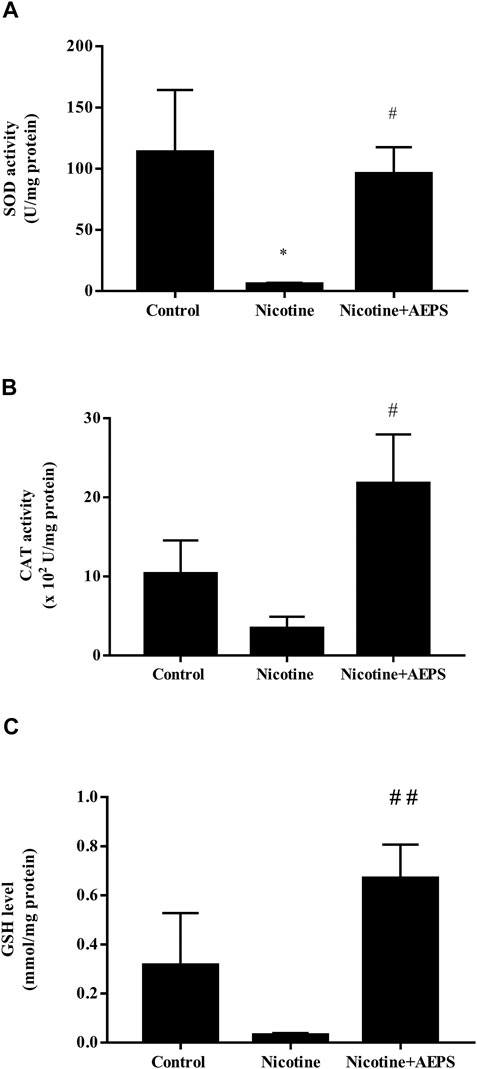
FIGURE 3. Level of antioxidants (A) superoxide dismutase (SOD) activity (B) catalase (CAT) activity and (C) reduced glutathione (GSH) level in rat aortic tissues from each group. The values are given as mean ± SEM, n = 6–8 for each group. *p < 0.05 compared with the control group, #p < 0.05, ##p < 0.01 compared with the nicotine group.
Effect of Aqueous Extract of Piper sarmentosum Roxb. Leaf on Vascular Remodeling
The aorta from the control and AEPS groups exhibited normal histology as indicated by the regular arrangement of the elastic lamella in the tunica media layer. The nicotine group displayed disorganized tunica media layer with increased interlamellar space (Figure 4). However, the morphometric analysis of the aorta, including IMT, IMA, d, CWT, and TS, showed no remarkable changes in all groups, even though the nicotine group showed an increasing trend in IMT (Table 2).
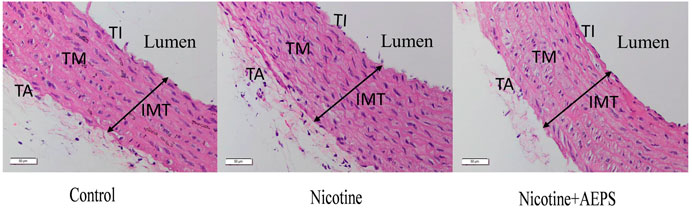
FIGURE 4. Representative images of haematoxylin and eosin-stained sections of the aortic wall from each group of rats. Control and nicotine + AEPS groups showed normal histological appearance. The nicotine group displayed disorganized tunica media layer (n = 6-8 per group, ×400 magnification, scale bar: 50 µm). IMT, intima-media thickness; TI, tunica intima; TM, tunica media; TA, tunica adventitia.
Discussion
This study showed that AEPS attenuated nicotine-induced vascular endothelial dysfunction as AEPS improved endothelium-dependent vasorelaxation in rats administered with nicotine. Exposure to nicotine impaired endothelium-dependent vasorelaxation to ACh. Impaired endothelium-dependent vasorelaxation is a functional characteristic of vascular endothelial dysfunction (Daiber et al., 2017). The effects of nicotine and AEPS on vasorelaxation were endothelium dependent because of the remarkable reduction in vasorelaxation in response to ACh but not in response to SNP. Besides, the maximum vasorelaxation to ACh was substantially higher in endothelium-intact vessels.
ACh works by binding to M3 receptors on the endothelium, which leads to calcium release and endothelial nitric oxide synthase (eNOS) activation. eNOS converts L-arginine to NO, which diffuses from the ECs into the adjacent layer of smooth muscle to cause vasorelaxation (Sandoo et al., 2010). By contrast, SNP is a NO donor that acts directly on VSMCs to cause vasorelaxation. The response to SNP is not dependent on the production of NO by ECs (Si et al., 2017).
This study demonstrated that AEPS supplementation to nicotine-induced rats increased vascular NO level. NO is a potent vasodilator; thus, the improved vasorelaxation observed in rats treated with AEPS is most likely contributed by the enhanced NO levels. AEPS also increases NO levels in spontaneously hypertensive rats and L-NG-nitro arginine methyl ester-induced hypertensive rats (Zainudin et al., 2015; Alwi et al., 2018). AEPS stimulates the synthesis of NO by increasing mRNA expression, protein level, and eNOS activity in ECs (Ugusman et al., 2010). Besides, antioxidants can also protect NO from degradation by free radicals and thus maintain the bioavailability of NO (Ugusman et al., 2010). The antioxidant activity of AEPS is well established (Zainudin et al., 2015; Wang et al., 2017; Yeo et al., 2018) and may also contribute to the enhanced NO level.
Impaired vasorelaxation due to nicotine exposure has been linked to increased vascular oxidative stress (Si et al., 2017). In a previous study, nicotine reduced the relaxation of rat’s aortic rings and increased the level of oxidative stress marker, malondialdehyde (Zainalabidin et al., 2014). Oxidative stress reduces endothelial NO production by deactivating eNOS (Ugusman et al., 2010). Nicotine deactivates eNOS by reducing the availability of eNOS essential cofactor, tetrahydrobiopterin (BH4). BH4 deficiency causes eNOS to become unpaired and unable to produce NO (Forstermann and Munzel, 2006; Li et al., 2018). Apart from NO, nicotine reduces other endothelium-dependent vasodilators, such as prostaglandins and prostacyclin (Tonnessen et al., 2000). The reduction of NO and other endothelium-dependent vasodilator results in impaired vasorelaxation. However, we did not measure the level of other endothelium-dependent vasodilators in this study.
Nonetheless, in this study, nicotine did not cause a remarkable reduction in NO level. Even though the NO level in nicotine-treated rats was not substantially reduced, the nicotine group had a NO reduction trend. We used the whole aorta for the measurement of NO level without specifically isolating the endothelial layer. This factor might contribute to the non-remarkability of the results. Nevertheless, previous studies related to the effects of nicotine on NO level have shown various inconsistent results. A study showed that nicotine does not cause any change in NO (Moon et al., 2014). Another study showed that nicotine reduces NO level by deactivating eNOS and stimulating the generation of superoxide anions that degrade NO (Kathuria et al., 2013). By contrast, a previous study showed that nicotine increases NO levels by increasing intracellular calcium, which in turn activates eNOS to produce NO (Ijomone et al., 2014). These inconsistent results may be contributed by the difference in the availability of eNOS cofactors, such as BH4 and NADPH, which are important for eNOS activity (Ugusman et al., 2010), as well as the oxidative degradation of NO by superoxide anion (Tonnessen et al., 2000).
In view of the relationship of nicotine with oxidative stress and NO level, which contributes to vascular endothelial dysfunction, SOD activity, CAT activity, and GSH levels in the aortic tissues were measured. The results showed that supplementation with AEPS enhanced SOD and CAT activities, as well as GSH level, in the aorta of nicotine-induced rats. This result suggests a protective effect of AEPS against oxidative stress induced by nicotine. The results align with previous findings that showed that AEPS enhances the mRNA expression of SOD, CAT, and glutathione peroxidase (GPX) in ECs exposed to H2O2 (Ugusman et al., 2011).
The results also demonstrated that nicotine decreased SOD activity, but no considerable differences in CAT activity and GSH level were found. Nicotine stimulates the production of superoxide anion by increasing the expression of NADPH oxidase 4 (NOX4) (Hua et al., 2010). NOX4 is the major source of superoxide anion in blood vessels (Ugusman et al., 2011). SOD is an antioxidant enzyme that acts as the first defense to neutralize superoxide anions into H2O2 (Zainalabidin et al., 2016). The excessive usage of SOD to neutralize the superoxide anions produced in response to nicotine has led to reduced SOD activity (Moon et al., 2014; Zainalabidin et al., 2014). Additionally, CAT transforms H2O2 to oxygen and water, whereas GSH is needed for GPX to convert H2O2 into water (Holben and Smith, 1999). In the present study, nicotine did not reduce the levels of CAT and GSH. This result is probably related to the action of SOD as the first antioxidant enzyme that neutralizes excessive free radicals before the actions of CAT and GSH start (Zainalabidin et al., 2016).
Collectively, this study showed that AEPS attenuates nicotine-induced vascular endothelial dysfunction by improving vasorelaxation and increasing vascular NO and antioxidant levels. The antioxidant activity of AEPS is often associated with its flavonoid content (Lee et al., 2014). Based on the LCMS analysis, the AEPS used in this study contains flavonoids, such as quercetin, naringenin, and vitexin (Sundar et al., 2019). Quercetin and naringenin are potent antioxidants that stimulate endothelial NO production (Yamamoto and Oue, 2006; Qin et al., 2016). Quercetin improves endothelium-dependent vasorelaxation by stimulating eNOS activity and increasing NO bioavailability in endothelial cells (Shen et al., 2012). Another flavonoid found in AEPS, naringenin, restores ACh-induced vasorelaxation in the aorta of diabetic rats. Naringenin reduces diabetic vascular endothelial dysfunction by downregulating oxidative stress and inflammation (Ren et al., 2016). In addition, vitexin improves aortic relaxation to ACh in chronic myocardial ischemia/reperfusion injury rat model (Che et al., 2016). Vitexin activates eNOS via the PI3K/Akt signaling pathway and can therefore regulate NO level (Cui et al., 2019). The present study used crude AEPS and not its purified active compound; thus, we were unable to pinpoint the specific compound of the extract that mediated the positive effects. However, the protective effects of AEPS on vascular endothelial dysfunction are probably mediated by the abovementioned potential flavonoids.
Apart from impaired vasorelaxation, abnormal vascular remodeling is one of the markers of chronic vascular dysfunction (Zainalabidin et al., 2014). AEPS treatment maintained the normal histological features of the aorta, whereas nicotine disorganized the tunica media layer and increased the interlamellar space. However, the morphometric analysis of the aorta showed no remarkable changes in all groups, even though IMT tend to increase in the nicotine group. The effect of nicotine on vascular remodeling is closely related to its dose and duration (Li et al., 2017). The i.p. injection of 0.6 mg/kg/day nicotine for 28 days causes an increment in IMT and CWT and a narrowing of the aortic lumen in rats (Zainalabidin et al., 2014). Nicotine infusion at 20 mg/kg/day for 40 days results in elastin fragmentation and increased stiffness in mouse aorta (Wagenhauser et al., 2018). The dose and duration of nicotine administration in this study were 0.8 mg/kg/day and 21 days, respectively. The duration of nicotine administration in this study is probably not long enough to cause remarkable structural changes in the aortic wall. Besides, we did not incorporate a special stain, such as the Verhoeff–van Gieson stain, to delineate the elastic fibers in the aortic wall for a better morphological assessment. This factor might also affect our morphometric analysis of the aorta.
Another limitation of this study is the absence of a treatment control group. This study reported the findings from three experimental groups, namely, control, nicotine, and nicotine + AEPS groups. A treatment control group that consists of AEPS alone should be included to affirm the safety and exclude any potential adverse effects of AEPS on healthy vasculature. Nonetheless, our previous studies that incorporated a treatment control group with AEPS alone showed no adverse effect on healthy vasculature in in vitro and in vivo levels. For instance, AEPS up to 300 μg/ml concentration did not reduce endothelial cell viability (Sundar et al., 2019). In rats, treatment with 500 mg/kg/day AEPS for 28 days did not cause any remarkable change in blood pressure (Alwi et al., 2018; Ugusman et al., 2020; Azmi et al., 2021). Besides, rat aorta displayed normal histology under light and electron microscopic examinations following AEPS treatment (Thent et al., 2012a; Thent et al., 2012b). Overall, previous studies showed that AEPS has no adverse effects on healthy vasculature.
Conclusion
P. sarmentosum attenuates nicotine-induced vascular endothelial dysfunction by enhancing vasorelaxation, vascular NO, and antioxidant levels. Thus, P. sarmentosum may be beneficial to prevent the vascular endothelial dysfunction caused by nicotine exposure. However, the molecular mechanism underlying the protective effect of P. sarmentosum on nicotine-induced vascular endothelial dysfunction needs further investigation. Furthermore, this study is an in vivo animal study that investigated some fundamental effects of AEPS on nicotine-induced vascular endothelial dysfunction. Further studies are required to identify and isolate the active compounds in AEPS responsible for the positive effects, as well as to explore their mechanisms of action. In addition, clinical trials that incorporate sufficient sample size and thorough methodology are needed to confirm our conclusions on the efficacy and safety of AEPS as a supplement for nicotine-induced vascular endothelial dysfunction in humans.
Data Availability Statement
The original contributions presented in the study are included in the article/Supplementary Material, further inquiries can be directed to the corresponding author.
Ethics Statement
The animal study was reviewed and approved by Universiti Kebangsaan Malaysia Animal Ethics Committee.
Author Contributions
Conceptualization, AA, AH, NS, and AU; methodology, MM, FJ, and AU; formal analysis, MM, FJ and AU; writing: original draft preparation, MM; writing: review and editing, AA, AH, NS, FJ, and AU.; supervision, AA, AH, NS, and AU; funding acquisition, AA, AH, NS, and AU. All authors read and approved the final manuscript.
Funding
This research was funded by Ministry of Higher Education Malaysia Fundamental Research Grant Scheme (FRGS/1/2019/SKK08/UKM/02/8) and Universiti Kebangsaan Malaysia Medical Centre Fundamental grant (FF-2018-403).
Conflict of Interest
The authors declare that the research was conducted in the absence of any commercial or financial relationships that could be construed as a potential conflict of interest.
Acknowledgments
The authors want to thank Datu Agasi Mohd Kamal, Firdaus Azmi, and all the medical lab technologists in Physiology Department, UKM, for their technical assistance. We also want to extend our gratitude to Satirah Zainal Abidin for her valuable input on the histological interpretation of the aorta.
Supplementary Material
The Supplementary Material for this article can be found online at: https://www.frontiersin.org/articles/10.3389/fphar.2021.667102/full#supplementary-material
References
Aguilar, T. a. F., Navarro, B. C. H., and Perez, J. a. M. (2016). Endogenous Antioxidants: A Review of Their Role in Oxidative Stress. London, UK: IntechOpen. doi:10.5772/65715
Almabrouk, T. A. M., White, A. D., Ugusman, A. B., Skiba, D. S., Katwan, O. J., Alganga, H., et al. (2018). High Fat Diet Attenuates the Anticontractile Activity of Aortic PVAT via a Mechanism Involving AMPK and Reduced Adiponectin Secretion. Front. Physiol. 9, 1–14. doi:10.3389/fphys.2018.00051
Alwi, N. a. N. M., Zakaria, Z., Karim, A. a. H., Nordin, N. a. M. M., and Ugusman, A. (2018). Antihypertensive Effect of Piper Sarmentosum in L-NAME-Induced Hypertensive Rats. Sains Malays. 47, 2421–2428. doi:10.17576/jsm-2018-4710-18
Amran, A. A., Zakaria, Z., Othman, F., Das, S., Raj, S., and Nordin, N.-A. M. (2010). Aqueous Extract of Piper Sarmentosum Decreases Atherosclerotic Lesions in High Cholesterolemic Experimental Rabbits. Lipids Health Dis. 9, 44–46. doi:10.1186/1476-511X-9-44
Azmi, M. F., Aminuddin, A., Jamal, J. A., A Hamid, A., and Ugusman, A. (2021). Quantified Piper Sarmentosum Roxb. Leaves Aqueous Leaf Extract and its Antihypertensive Effect in Dexamethasone-Induced Hypertensive Rats. Sains Malaysiana. 50, 171–179. doi:10.17576/jsm-2021-5001-17
Beyer, W. F., and Fridovich, I. (1987). Assaying for Superoxide Dismutase Activity: Some Large Consequences of Minor Changes in Conditions. Anal. Biochem. 161, 559–566. doi:10.1016/0003-2697(87)90489-1
Bradford, M. M. (1976). A Rapid and Sensitive Method for the Quantitation of Microgram Quantities of Protein Utilizing the Principle of Protein-Dye Binding. Anal. Biochem. 72, 248–254. doi:10.1006/abio.1976.999910.1016/0003-2697(76)90527-3
Brüggmann, D., Lips, K. S., Pfeil, U., Haberberger, R. V., and Kummer, W. (2003). Rat Arteries Contain Multiple Nicotinic Acetylcholine Receptor α-subunits. Life Sci. 72, 2095–2099. doi:10.1016/s0024-3205(03)00067-5
Carnevale, R., Sciarretta, S., Violi, F., Nocella, C., Loffredo, L., Perri, L., et al. (2016). Acute Impact of Tobacco vs Electronic Cigarette Smoking on Oxidative Stress and Vascular Function. Chest. 150, 606–612. doi:10.1016/j.chest.2016.04.012
Che, X., Wang, X., Zhang, J., Peng, C., Zhen, Y., Shao, X., et al. (2016). Vitexin Exerts Cardioprotective Effect on Chronic Myocardial Ischemia/reperfusion Injury in Rats via Inhibiting Myocardial Apoptosis and Lipid Peroxidation. Am. J. Transl Res. 8, 3319–3328.
Cucina, A., Sapienza, P., Corvino, V., Borrelli, V., Mariani, V., Randone, B., et al. (2000). Nicotine-induced Smooth Muscle Cell Proliferation Is Mediated through bFGF and TGF-Β1. Surgery. 127, 316–322. doi:10.1067/msy.2000.104249
Cui, Y.-h., Zhang, X.-q., Wang, N.-d., Zheng, M.-d., and Yan, J. (2019). Vitexin Protects against Ischemia/reperfusion-Induced Brain Endothelial Permeability. Eur. J. Pharmacol. 853, 210–219. doi:10.1016/j.ejphar.2019.03.015
Daiber, A., Steven, S., Weber, A., Shuvaev, V. V., Muzykantov, V. R., Laher, I., et al. (2017). Targeting Vascular (Endothelial) Dysfunction. Br. J. Pharmacol. 174, 1591–1619. doi:10.1111/bph.13517
Durand, M. J., and Gutterman, D. D. (2013). Diversity in Mechanisms of Endothelium-dependent Vasodilation in Health and Disease. Microcirculation. 20, 239–247. doi:10.1111/micc.12040
Ellman, G. L. (1959). Tissue Sulfhydryl Groups. Arch. Biochem. Biophys. 82, 70–77. doi:10.1016/0003-9861(59)90090-6
Förstermann, U., and Münzel, T. (2006). Endothelial Nitric Oxide Synthase in Vascular Disease: from Marvel to Menace. Circulation. 113, 1708–1714. doi:10.1161/CIRCULATIONAHA.105.602532
Hecht, S. S. (2003). Tobacco Carcinogens, Their Biomarkers and Tobacco-Induced Cancer. Nat. Rev. Cancer 3, 733–744. doi:10.1038/nrc1190
Holben, D. H., and Smith, A. M. (1999). The Diverse Role of Selenium within Selenoproteins. J. Am. Diet. Assoc. 99, 836–843. doi:10.1016/S0002-8223(99)00198-4
Hua, P., Feng, W., Ji, S., Raij, L., and Jaimes, E. A. (2010). Nicotine Worsens the Severity of Nephropathy in Diabetic Mice: Implications for the Progression of Kidney Disease in Smokers. Am. J. Physiology-Renal Physiol. 299, F732–F739. doi:10.1152/ajprenal.00293.2010
Ijomone, O. M., Olaibi, O. K., and Nwoha, P. U. (2014). Effects of Chronic Nicotine Administration on Body Weight, Food Intake and Nitric Oxide Concentration in Female and Male Rats. Pathophysiology 21, 185–190. doi:10.1016/j.pathophys.2014.08.003
Ismail, S. M., Chua, K. H., Aminuddin, A., and Ugusman, A. (2018). Piper Sarmentosum as an Antioxidant: A Systematic Review. Sains Malaysiana. 47, 2359–2368. doi:10.1016/j.jtumed.2018.01.00310.17576/jsm-2018-4710-12
Jiang, D.-J., Jia, S.-J., Yan, J., Zhou, Z., Yuan, Q., and Li, Y.-J. (2006). Involvement of DDAH/ADMA/NOS Pathway in Nicotine-Induced Endothelial Dysfunction. Biochem. Biophysical Res. Commun. 349, 683–693. doi:10.1016/j.bbrc.2006.08.115
Kathuria, S., Mahadevan, N., and Balakumar, P. (2013). Possible Involvement of PPARγ-Associated eNOS Signaling Activation in Rosuvastatin-Mediated Prevention of Nicotine-Induced Experimental Vascular Endothelial Abnormalities. Mol. Cel Biochem. 374, 61–72. doi:10.1007/s11010-012-1505-6
Lee, J. H., Cho, S., Paik, H. D., Choi, C. W., Nam, K. T., Hwang, S. G., et al. (2014). Investigation on Antibacterial and Antioxidant Activities, Phenolic and Flavonoid Contents of Some Thai Edible Plants as an Alternative for Antibiotics. Asian Australas. J. Anim. Sci. 27, 1461–1468. doi:10.5713/ajas.2013.13629
Li, J., Liu, S., Cao, G., Sun, Y., Chen, W., Dong, F., et al. (2018). Nicotine Induces Endothelial Dysfunction and Promotes Atherosclerosis via GTPCH1. J. Cel Mol Med. 22, 5406–5417. doi:10.1111/jcmm.13812
Li, W., Du, D.-Y., Liu, Y., Jiang, F., Zhang, P., and Li, Y.-T. (2017). Long-term Nicotine Exposure Induces Dysfunction of Mouse Endothelial Progenitor Cells. Exp. Ther. Med. 13, 85–90. doi:10.3892/etm.2016.3916
Mayhan, W. G., and Sharpe, G. M. (2002). Acute and Chronic Treatment with Nicotine Impairs Reactivity of Arterioles in Response to Activation of Potassium Channels. J. Cardiovasc. Pharmacol. 39, 695–703. doi:10.1097/00005344-200205000-00010
Moccia, F., Frost, C., Berra-Romani, R., Tanzi, F., and Adams, D. J. (2004). Expression and Function of Neuronal Nicotinic ACh Receptors in Rat Microvascular Endothelial Cells. Am. J. Physiology-Heart Circulatory Physiol. 286, H486–H491. doi:10.1152/ajpheart.00620.2003
Mohd Zainudin, M., Zakaria, Z., Megat Mohd Nordin, N. A., and Othman, F. (2013). Does Oral Ingestion ofPiper sarmentosumCause Toxicity in Experimental Animals? Evidence-Based Complement. Altern. Med. 2013, 1–9. doi:10.1155/2013/705950
Moon, H. K., Kang, P., Lee, H. S., Min, S. S., and Seol, G. H. (2014). Effects of 1,8-cineole on Hypertension Induced by Chronic Exposure to Nicotine in Rats. J. Pharm. Pharmacol. 66, 688–693. doi:10.1111/jphp.12195
Ng, M. K. C., Wu, J., Chang, E., Wang, B.-y., Katzenberg-Clark, R., Ishii-Watabe, A., et al. (2007). A central Role for Nicotinic Cholinergic Regulation of Growth Factor-Induced Endothelial Cell Migration. Arterioscler Thromb Vasc Biol. 27, 106–112. doi:10.1161/01.ATV.0000251517.98396.4a
Olfert, I. M., Devallance, E., Hoskinson, H., Branyan, K. W., Clayton, S., Pitzer, C. R., et al. (2018). Chronic Exposure to Electronic Cigarettes Results in Impaired Cardiovascular Function in Mice. J. Appl. Physiol. 124, 573–582. doi:10.1152/japplphysiol.00713.2017
Peungvicha, P., S. Thirawarapan, S., Temsiririrkkul, R., Watanabe, H., Kumar Prasain, J., and Kadota, S. (1998). Hypoglycemic Effect of the Water Extract of Piper Sarmentosum in Rats. J. Ethnopharmacology. 60, 27–32. doi:10.1016/s0378-8741(97)00127-x
Qin, W., Ren, B., Wang, S., Liang, S., He, B., Shi, X., et al. (2016). Apigenin and Naringenin Ameliorate PKCβII-Associated Endothelial Dysfunction via Regulating ROS/caspase-3 and NO Pathway in Endothelial Cells Exposed to High Glucose. Vasc. Pharmacol. 85, 39–49. doi:10.1016/j.vph.2016.07.006
Ren, B., Qin, W., Wu, F., Wang, S., Pan, C., Wang, L., et al. (2016). Apigenin and Naringenin Regulate Glucose and Lipid Metabolism, and Ameliorate Vascular Dysfunction in Type 2 Diabetic Rats. Eur. J. Pharmacol. 773, 13–23. doi:10.1016/j.ejphar.2016.01.002
Rodella, L. F., Rossini, C., Favero, G., Foglio, E., Loreto, C., and Rezzani, R. (2012). Nicotine-induced Morphological Changes in Rat Aorta: the Protective Role of Melatonin. Cells Tissues Organs. 195, 252–259. doi:10.1159/000324919
Sandoo, A., Veldhuijzen Van Zanten, J. J. C. S., Metsios, G. S., Carroll, D., and Kitas, G. D. (2010). The Endothelium and its Role in Regulating Vascular Tone. Open Cardiovasc Med J. 4, 302–312. doi:10.2174/1874192401004010302
Shen, Y., Croft, K. D., Hodgson, J. M., Kyle, R., Lee, I.-L. E., Wang, Y., et al. (2012). Quercetin and its Metabolites Improve Vessel Function by Inducing eNOS Activity via Phosphorylation of AMPK. Biochem. Pharmacol. 84, 1036–1044. doi:10.1016/j.bcp.2012.07.016
Si, L. Y.-N., Kamisah, Y., Ramalingam, A., Lim, Y. C., Budin, S. B., and Zainalabidin, S. (2017). Roselle Supplementation Prevents Nicotine-Induced Vascular Endothelial Dysfunction and Remodelling in Rats. Appl. Physiol. Nutr. Metab. 42, 765–772. doi:10.1139/apnm-2016-0506
Son, Y.-J., and Lee, H.-J. (2020). Association between Persistent Smoking after a Diagnosis of Heart Failure and Adverse Health Outcomes: A Systematic Review and Meta-Analysis. Tob. Induc. Dis. 18, 1–11. doi:10.18332/tid/116411
Su, Y. C., and Wang, D. X. (1991). Effects of Cigarette Smoking, Hypoxia and Vasoactive Mediators on the Production of PGI2 and TXA2 in Cultured Pulmonary Artery Endothelial Cells. J. Tongji Med. Univ. 11, 6–9. doi:10.1007/bf02893179
Sundar, U. M., Ugusman, A., Chua, H. K., Latip, J., and Aminuddin, A. (2019). Piper Sarmentosum Promotes Endothelial Nitric Oxide Production by Reducing Asymmetric Dimethylarginine in Tumor Necrosis Factor-α-Induced Human Umbilical Vein Endothelial Cells. Front. Pharmacol. 10, 1–14. doi:10.3389/fphar.2019.01033
Thent, Z. C., Lin, T. S., Das, S., and Zakaria, Z. (2012a). Histological Changes in the Heart and the Proximal Aorta in Experimental Diabetic Rats Fed with Piper Sarmentsoum. Afr. J. Tradit Complement. Altern. Med. 9, 396–404.
Thent, Z. C., Seong Lin, T., Das, S., and Zakaria, Z. (2012b). Effect ofPiper sarmentosumExtract on the Cardiovascular System of Diabetic Sprague-Dawley Rats: Electron Microscopic Study. Evidence-Based Complement. Altern. Med. 2012, 1–9. doi:10.1155/2012/628750
Toda, N., and Toda, H. (2010). Nitric Oxide-Mediated Blood Flow Regulation as Affected by Smoking and Nicotine. Eur. J. Pharmacol. 649, 1–13. doi:10.1016/j.ejphar.2010.09.042
Tonnessen, B. H., Severson, S. R., Hurt, R. D., and Miller, V. M. (2000). Modulation of Nitric-Oxide Synthase by Nicotine. J. Pharmacol. Exp. Ther. 295, 601–606.
Ugusman, A., Md Fadze, N. F., Hamid, A. A., Asmawi, Z., and Aminuddin, A. (2020). Piper Sarmentosum Attenuates Dexamethasone-Induced Hypertension by Stimulating Endothelial Nitric Oxide Synthase. J. Res. Pharm. 24, 1–9. doi:10.35333/jrp.2020.122
Ugusman, A., Zakaria, Z., Hui, C. K., and Megat Mohd Nordin, N. A. (2011). Piper Sarmentosum Inhibits ICAM-1 and Nox4 Gene Expression in Oxidative Stress-Induced Human Umbilical Vein Endothelial Cells. BMC Complement. Altern. Med. 11, 1–8. doi:10.1186/1472-6882-11-31
Ugusman, A., Zakaria, Z., Hui, C. K., and Nordin, N. A. M. M. (2010). Piper Sarmentosum Increases Nitric Oxide Production in Oxidative Stress: a Study on Human Umbilical Vein Endothelial Cells. Clinics. 65, 709–714. doi:10.1590/S1807-59322010000700010
Wagenhäuser, M. U., Schellinger, I. N., Yoshino, T., Toyama, K., Kayama, Y., Deng, A., et al. (2018). Chronic Nicotine Exposure Induces Murine Aortic Remodeling and Stiffness Segmentation-Implications for Abdominal Aortic Aneurysm Susceptibility. Front. Physiol. 9, 1–11. doi:10.3389/fphys.2018.01459
Wang, D. F., Zhou, L. L., Zhou, H. L., Hou, G. Y., Zhou, X., and Li, W. (2017). Effects ofPiper Sarmentosumextract on the Growth Performance, Antioxidant Capability and Immune Response in Weaned Piglets. J. Anim. Physiol. Anim. Nutr. 101, 105–112. doi:10.1111/jpn.12517
Whitehead, A. K., Erwin, A. P., and Yue, X. (2021). Nicotine and Vascular Dysfunction. Acta Physiol. 231, 1–13. doi:10.1111/apha.13631
Wright, J. L., Tai, H., and Churg, A. (2004). Cigarette Smoke Induces Persisting Increases of Vasoactive Mediators in Pulmonary Arteries. Am. J. Respir. Cel Mol Biol 31, 501–509. doi:10.1165/rcmb.2004-0051OC
Yamamoto, Y., and Oue, E. (2006). Antihypertensive Effect of Quercetin in Rats Fed with a High-Fat High-Sucrose Diet. Biosci. Biotechnol. Biochem. 70, 933–939. doi:10.1271/bbb.70.933
Yeo, E. T. Y., Wong, K. W. L., See, M. L., Wong, K. Y., Gan, S. Y., and Chan, E. W. L. (2018). Piper Sarmentosum Roxb. Confers Neuroprotection on Beta-Amyloid (Aβ)-Induced Microglia-Mediated Neuroinflammation and Attenuates Tau Hyperphosphorylation in SH-Sy5y Cells. J. Ethnopharmacology 217, 187–194. doi:10.1016/j.jep.2018.02.025
Zainalabidin, S., Budin, S. B., Ramalingam, A., and Lim, Y. C. (2014). Aortic Remodelling in Chronic Nicotine-Administered Rat. Korean J. Physiol. Pharmacol. 18, 411–418. doi:10.4196/kjpp.2014.18.5.411
Zainalabidin, S., Shahidin, S. N. F. S. N., and Budin, S. B. (2016). Hibiscus sabdariffa Linn.(Roselle) Protects against Nicotine-Induced Heart Damage in Rats. Sains Malays 45, 207–21.
Zainudin, M. M., Zakaria, Z., and Nordin, N. a. M. M. (2015). The Use of Piper Sarmentosum Leaves Aqueous Extract (Kadukmy™) as Antihypertensive Agent in Spontaneous Hypertensive Rats. BMC Complement. Altern. Med. 15, 1–10. doi:10.1186/s12906-015-0565-z
Glossary
ACh acetylcholine
AEPS aqueous extract of Piper sarmentosum Roxb. leaf
BH4 tetrahydrobiopterin
BW body weight
CAT catalase
CVD cardiovascular diseases
CWT circumference wall tension
EC endothelial cell
EDTA ethylenediaminetetraacetic acid
eNOS endothelial nitric oxide synthase
GPX glutathione peroxidase
GSH reduced glutathione
H&E hematoxylin and eosin
H2O2 hydrogen peroxide
i.p. intraperitoneally
IMA intima-media area
IMT intima-media thickness
LCMS liquid chromatography mass spectrometry
MMP matrix metalloproteinase
mRNA messenger ribonucleic acid
MSBP mean systolic blood pressure
NAChR nicotinic acetylcholine receptor
NADPH nicotinamide adenine dinucleotide phosphate
NBT nitro blue tetrazolium
NO nitric oxide
NOX4 NADPH oxidase 4
p.o. oral gavage
PBS phosphate-buffered saline
Rmax maximum relaxation
SEM standard error of the mean
SNP sodium nitroprusside
SOD superoxide dismutase
TS tensile stress
UKM Universiti Kebangsaan Malaysia
VSMC vascular smooth muscle cell
w/v weight/volume
Keywords: nicotine, nitric oxide, oxidative stress, vascular remodeling, vascular endothelial dysfunction, Piper sarmentosum Roxb.
Citation: Md. Salleh MFRR, Aminuddin A, Hamid AA, Salamt N, Japar Sidik FZ and Ugusman A (2021) Piper sarmentosum Roxb. Attenuates Vascular Endothelial Dysfunction in Nicotine-Induced Rats. Front. Pharmacol. 12:667102. doi: 10.3389/fphar.2021.667102
Received: 12 February 2021; Accepted: 02 June 2021;
Published: 14 June 2021.
Edited by:
Mas Rizky A.A Syamsunarno, Padjadjaran University, IndonesiaReviewed by:
Leonardo Borges, State University of Goiás, BrazilMaizura Mohd Zainudin, International Islamic University Malaysia, Malaysia
Copyright © 2021 Md. Salleh, Aminuddin, Hamid, Salamt, Japar Sidik and Ugusman. This is an open-access article distributed under the terms of the Creative Commons Attribution License (CC BY). The use, distribution or reproduction in other forums is permitted, provided the original author(s) and the copyright owner(s) are credited and that the original publication in this journal is cited, in accordance with accepted academic practice. No use, distribution or reproduction is permitted which does not comply with these terms.
*Correspondence: Azizah Ugusman, ZHIuYXppemFoQHBwdWttLnVrbS5lZHUubXk=