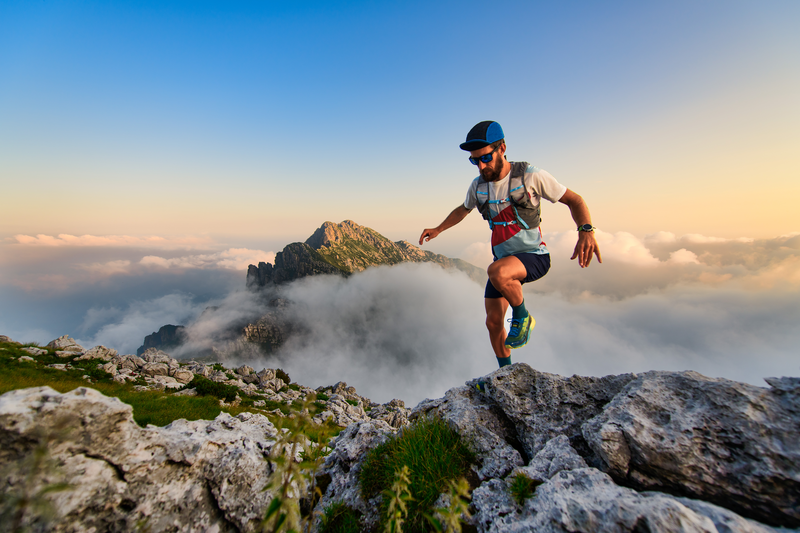
94% of researchers rate our articles as excellent or good
Learn more about the work of our research integrity team to safeguard the quality of each article we publish.
Find out more
MINI REVIEW article
Front. Pharmacol. , 13 May 2021
Sec. Experimental Pharmacology and Drug Discovery
Volume 12 - 2021 | https://doi.org/10.3389/fphar.2021.666664
The current pandemic caused by SARS-CoV2 and named COVID-19 urgent the need for novel lead antiviral drugs. Recently, United States Food and Drug Administration (FDA) approved the use of remdesivir as anti-SARS-CoV-2. Remdesivir is a natural product-inspired nucleoside analogue with significant broad-spectrum antiviral activity. Nucleosides analogues from marine sponge including spongouridine and spongothymidine have been used as lead for the evolutionary synthesis of various antiviral drugs such as vidarabine and cytarabine. Furthermore, the marine sponge is a rich source of compounds with unique activities. Marine sponge produces classes of compounds that can inhibit the viral cysteine protease (Mpro) such as esculetin and ilimaquinone and human serine protease (TMPRSS2) such as pseudotheonamide C and D and aeruginosin 98B. Additionally, sponge-derived compounds such as dihydrogracilin A and avarol showed immunomodulatory activity that can target the cytokines storm. Here, we reviewed the potential use of sponge-derived compounds as promising therapeutics against SARS-CoV-2. Despite the reported antiviral activity of isolated marine metabolites, structural modifications showed the importance in targeting and efficacy. On that basis, we are proposing a novel structure with bifunctional scaffolds and dual pharmacophores that can be superiorly employed in SARS-CoV-2 infection.
The current outbreak caused by the novel coronavirus (SARS-CoV-2) and designated COVID-19 by the World Health Organization (WHO), spread aggressively worldwide (Li et al., 2020). As of today, there is no safe and effective drug available for SARS-CoV-2 and the efficacy of available antiviral drugs is still controversial. Therefore, there is an urgent need for the design and development of novel treatment and therapeutic strategies to combat SARS-CoV-2 and possibly other emergent future viruses. Recently, remdesivir was approved by FDA as an anti-SARS-CoV-2. The anti-SARS-CoV-2 activity of remdesivir was proven following a randomized study at ten hospitals in Hubei, China (Wang et al., 2020). Patients receiving remdesivir showed clinical improvement when compared to placebo (Simonis et al., 2021).
Remdesivir is a prodrug that is once entered the cell converted to a triphosphate nucleoside analogue with significant inhibition activity against viral RNA-dependent RNA polymerase (RdRp) (Eastman et al., 2020). Remdesivir was originally developed by Gilead Sciences in collaboration with the United States. Centers for Disease Control and Prevention (CDC) and the United States. Army Medical Research Institute of Infectious Diseases (USAMRIID) (Eastman et al., 2020). Remdesivir is a nucleoside analogue, a class of drugs, that was only developed after being found in sea sponges. Sponges are known for the unusual nucleoside properties (Laffoley et al., 2020). FDA has also approved ocean-derived drugs for HIV, herpes, and now for COVID-19. Although over 34,000 marine natural products have been discovered with great potential to improve human life and health, this represents only 3% of the ocean’s natural sources (Laffoley et al., 2020). Therefore, the essential role of ocean-derived drugs as anti-SARS-CoV-2 are highlighted.
Nucleosides are the building block of nucleic acid, which consist of nucleobases linked to sugar moiety (Seley-Radtke and Yates, 2018). Nucleosides are involved in vital biological activities including the formation of nucleotides (Seley-Radtke and Yates, 2018). A variety of nucleosides analogues with unique chemical structures was isolated from marine sponge and showed significant antiviral activities (Anjum et al., 2016). The isolated nucleosides analogues were incorporated for the design and development of antiviral drugs following structural modifications in the sugar moiety and/ or the nucleobase (Chien et al., 2020). Initially, nucleosides analogues such as spongouridine and spongothymidine, isolated from Cryptotethya sponge, were investigated for antiviral activity (Bergmann and Feeney, 1951). Replacement of ribose sugar by arabinose paved the basic root for the development of FDA-approved vidarabine (ara-A) and cytarabine (ara-C) (Figure 1).
FIGURE 1. Nucleotide analogues inhibitors (NIs). (A) Development of the first NIs. (B) Nucleotides analogues as potent antiviral against SARS-CoV-2.
Nucleoside analogues were privileged as scaffold for the design and development of nucleotide (Table 1; Figure 1) and nucleoside analogue inhibitors (NIs) (Supplementary Figure S1; Supplementary Table S1). Nucleosides analogues were employed in the treatment of viral infections including in particular coronavirus (Pruijssers and Denison, 2019). NIs are known as broad-spectrum inhibitors of RdRp (Shannon et al., 2020a). RdRp showed high structural conservation among coronaviruses Aftab et al. (2020), thus considered an attractive target for the development of various antiviral drugs (Table 1; Figure 1; Supplementary Table S1; Supplementary Figure S1). Mycalisine A, and B are nucleosides analogues isolated from the marine sponge Mycale sp. 1985 and employed as scaffold for the development of NIs following structure modification by the inclusion of CN group (Kato et al., 1985; Bhakuni and Rawat, 2005). Mycalisine A inspired the synthesis of remdesivir.
Remdesivir, a nucleotide analogue with 1-ribose and CN substitution, showed interesting antiviral activity by exhibiting dual inhibition activity against RdRp and exonuclease proteins (Shannon et al., 2020a; Zhang et al., 2020a). In addition, 2-methyl cytidine and EIDD-2801, modified cytidine analogues Zandi et al. (2020), inhibited SARS-CoV-2 replication Jena (2020), Sheahan et al. (2020) with no toxicity on Vero cells (Yosief et al., 1998). Furthermore, computational modelling of ilimaquinone Surti et al. (2020) and its adenosine analogues, asmarine B (Kim et al., 2009), showed potential inhibition activity against SARS-CoV-2 (Božić et al., 2010).
The data highlighted that metabolites derived from marine sponge can be promising RdRp inhibitors following minor structural modifications. These modifications can include the change in the sugar moiety, and the addition of substituents such as cyano, fluoride, and methyl groups. Interestingly, the inclusion of the side chain with cyano group in the remdesivir enhanced the compound bioavailability and overcame the resistance mechanism by the viral exonuclease. Furthermore, modification of ilimaquinone by the inclusion of adenosine enhanced the activity of the original natural compound100-fold. These data indicate that modification in compounds obtained from the marine sponge is necessary for focused targeting, enhancement of bioavailability and activity, and overcome resistance mechanism, despite the potential activity of the original compounds. Importantly, compounds with superior dual activity are those sharing nucleotide or nucleoside along with sugar as scaffold such as avinosol (Diaz-Marrero et al., 2006).
Mpro is a critical protease required during the viral replication (Du et al., 2004). Consequently, its inhibition can stop the production of viral particles (Grum-Tokars et al., 2008). Further, Mpro showed no genetics homology with the human genome making it an attractive target in the development of safer antiviral drugs (Abd El-Mordy et al., 2020).
Based on several computational simulation studies in addition to molecular docking and molecular dynamics studies, variable natural marine compounds were suggested as inhibitors to SARS-CoV-2 Mpro. Figure 2A summarized different classes of compounds derived from the marine sponge with potential Mpro inhibition activity (Golda and Pyrc, 2008; Khan et al., 2020).
FIGURE 2. Sponge-derived compounds with potent targets. (A) Anti-Mpro compounds derived from marine sponge. (B) Anti-TMPRSS2 compounds derived from marine sponge.
Coumarine derivatives such as esculetin-4-carboxylic acid methyl ester and esculetin-4-carboxylic acid ethyl ester isolated from the marine sponge, Axinella cf. corrugate, showed effective inhibition activity to SARS-coronavirus Mpro (Lira et al., 2007) at IC50 = 46 μM (Coelho et al., 2020). Molecular docking also indicated their effective interaction with SARS-CoV-2 Mpro (Vijayaraj et al., 2020). Molecular docking study also showed the potential interaction of naphthalene derivative, hamigeran-b, isolated from the marine sponge, Hamigera tarangaensis, with the Mpro of SARS-CoV and SARS-CoV-2 (Vijayaraj et al., 2020). Similarly, chimyl alcohol (1-O-hexadecylglycerol), isolated from Desmapsamma anchorata sponge (Quijano et al., 1994), showed potential inhibition activity to SARS-CoV-2 by binding to Mpro (Khan et al., 2020).
Ilimaquinone is a bioactive sesquiterpene isolated from the Hippospongia metachromia sponge (Surti et al., 2020). Computational modelling indicated the potential inhibitory activity of the compound against SARS-CoV-2 proteases (Surti et al., 2020). Virtual screening and ADMET studies indicated that terpenoid T3, isolated from the marine sponge, Cacospongia mycofijiensis, can exhibit potential inhibition activity against SARS CoV-2 Mpro (Sepay et al., 2020).
Several classes of compounds derived from marine sponge were proposed as potential SARS-CoV-2 Mpro inhibitors based on computational analysis; however, these data need to be further validated by both enzymatic activity and in vitro assays. On the other hand, terpenoid moiety is shared between most marine compounds proposed with Mpro inhibition activity including T3, ilimaquinone, and hamigeran-b (Figure 2A), indicating its potential involvement in the inhibition activity of Mpro.
TMPRSS2 is a human serine protease enzyme used by the virus for its activation and cell entry. Pseudotheonamide C and D, isolated from the Theonella swinhoei sponge, showed potent inhibitory activity against serine protease (Figure 2B) (Nakao et al., 1999). Similarly, aeruginosin 98B, isolated from the marine sponge Microcystis aeruginosa, showed inhibitory activity against serine protease (Ersmark et al., 2008). Pseudotheonamide C and D and aeruginosin 98B contain guanidino group that mimics the arginine substrate of the enzyme (Figure 2B) (Buchanan et al., 2008).
Structure-based modelling indicated that both pseudotheonamide and aeruginosin may also show potent inhibitory activity against SARS-CoV-2 Mpro (Gentile et al., 2020). However, more biological activity studies are still required. This is considered bifunctional activity since they inhibit both cysteine (Mpro) and serine (TMPRSS2) proteases.
The aforementioned data can be of great benefit to fight against SARS-CoV-2 once the biological activity of the compounds is validated.
SARS-CoV-2 infection stimulates the host immune responses in two phases, the initial phase during the viral invasion, and the severe stage when a massive cytokine and chemokine storm takes place including the overproduction of IL-1, IL-6, IL-8, IL-17, CCL-2, TNF-ɑ, G-CSF, IP-10, MCP-1, and MIP and exhaustion of T cells. Therefore, strategies to boost the immune system at the earlier stage (mild condition) and those to modulate or suppress the cytokine storm at a later stage (severe condition) are required to manage SARS-CoV-2 infection (Niloufar et al., 2020).
An enormous array of molecules isolated from marine sponge showed the ability to boost innate immunity at the early infection stage or to control the cytokine storm and the excessive inflammation at the late severe infection stage (Table 2; Supplementary Figure S2). Avarol produced from the Disidea avara sponge was reported to boost the humoral immune response upon exposure to viral infection (Müller et al., 1985). Lectin is an immuno-stimulant that was isolated from the marine sponge Pellina semitubulosa (Engel et al., 1992). Lectin has a hexamer polypeptide chain covalently linked via a disulfide bond that can enhance the production of IL-1 and IL-2 at 0.3 and 10.0 pg/ml.
TABLE 2. Summary of compounds isolated from different marine sponges and showed immunomodulatory activity.
Identification of immunosuppressive molecules from marine sponge was initially reported in the 1980s when two compounds, 4-α-methyl-5 α-cholest-8-en-3 β-ol and 4,5-dibromo-2-pyrrolic acid were isolated from Agelas flabelliformis Carter (Agelasidae) (Gunasekera et al., 1989). Octa-peptide hymenistatin I, isolated from Hymeniacidon sp. Sponge, demonstrated humoral and cellular immunosuppressive activity (Pettit et al., 1990). Contignasterol, produced from Petrosia contignata sponge, is a histamine-release inhibitor, which is more likely downregulating the production of IL-6 (Takei et al., 1994; Han, 2020). Puupehedione is a sesquiterpene quinone that has been isolated from several marine sponges such as Verongida sp. and Hyrtios sp. and showed the ability to modulate the immune response of T-cells (Hamann et al., 1993; Nasu et al., 1995).
Eryloside E, isolated from Erylus goffrilleri sponge, demonstrated specific immunosuppressive activity at IC50 1.3 μg/ml (Gulavita et al., 1994). Pateamine A was isolated from Mycale sp. and showed selective inhibition activity on the production of IL-2 (Romo et al., 1998; Costela-Ruiz et al., 2020). Similarly, the pyrrole-imidazole alkaloid taurodispacamide A, isolated from the marine sponge Agelas oroides, showed inhibitory activity to IL-2 production (Fattorusso and Taglialatela-Scafati, 2000). Several immunosuppressive compounds such as 3-polyoxygenated sterol were isolated from the marine sponge Disidea sp. (Supplementary Figure S3) de Almeida Leone et al. (2000) that can block the activity of IL-8, a cytokine responsible for the development of acute respiratory distress syndrome (Tang et al., 2020).
Greve et al. (2007) showed that the Ianthella quadrangulata sponge produces the polyketide iso-iantheran A, which is capable to activate the P2Y11 receptor Greve et al. (2007), a regulator of human immune responses (Ledderose et al., 2020). Terpene dihydrogracilin A is a potent IL-6 inhibitor that was isolated from the Dendrilla membranosa sponge (Ciaglia et al., 2017).
The data described here indicated that several metabolites derived from marine sponge showed promising immunomodulatory activity. Some of these compounds shared structural similarity including the terpenoid and/ or the sugar moieties (Supplementary Figure S2).
The aforementioned classes of sponge-derived compounds provided an insight into pharmacophores with shared structures that can be employed in the development of novel scaffold with potent antiviral activity and improved efficacy against SARS-CoV-2. A promising strategy as indicated in Figure 3 is by designing NIs that target both SARS-CoV-2 RdRp and exonuclease (Pruijssers and Denison, 2019) as shown in remdesivir, in addition to the inclusion of other pharmacophores that target other viral proteins (Khater et al., 2020). In that respect, marine nucleosides analogues and peptidomimetics can inhibit the viral RdRp, and Mpro, respectively, while guanine derivatives can inhibit the human TMPRSS2 (Buchanan et al., 2008). Furthermore, the addition of terpenoid moiety can be of great benefit as an immunomodulator. On that basis, we are proposing a conjugated structure as indicated in Figure 3 with bifunctional scaffolds and pharmacophore features with the ability to target essential SARS-CoV-2 proteins.
All authors involved in developing the idea, designing the manuscript, writing the draft and final version. SS supervise the data collection and writing process.
The authors declare that the research was conducted in the absence of any commercial or financial relationships that could be construed as a potential conflict of interest.
The authors acknowledge the generous support from Sandooq Al-Watan and University of Sharjah to SS.
The Supplementary Material for this article can be found online at: https://www.frontiersin.org/articles/10.3389/fphar.2021.666664/full#supplementary-material
Abd El-Mordy, F. M., El-Hamouly, M. M., Ibrahim, M. T., El-Rheem, G. A., Aly, O. M., Abd El-kader, A. M., et al. (2020). Inhibition of SARS-CoV-2 Main Protease by Phenolic Compounds from Manilkara Hexandra (Roxb.) Dubard Assisted by Metabolite Profiling and In Silico Virtual Screening. RSC Adv. 10, 32148–32155. doi:10.1039/d0ra05679k
Abuo-Rahma, G. E.-D. A., Mohamed, M. F. A., Ibrahim, T. S., Shoman, M. E., Samir, E., and Abd El-Baky, R. M. (2020). Potential Repurposed SARS-CoV-2 (COVID-19) Infection Drugs. RSC Adv. 10, 26895–26916. doi:10.1039/d0ra05821a
Aftab, S. O., Ghouri, M. Z., Masood, M. U., Haider, Z., Khan, Z., Ahmad, A., et al. (2020). Analysis of SARS-CoV-2 RNA-dependent RNA Polymerase as a Potential Therapeutic Drug Target Using a Computational Approach. J. translational Med. 18, 1–15. doi:10.1186/s12967-020-02439-0
Anjum, K., Abbas, S. Q., Shah, S. A. A., Akhter, N., Batool, S., and Hassan, S. S. u. (2016). Marine Sponges as a Drug Treasure. Biomolecules Ther. 24, 347–362. doi:10.4062/biomolther.2016.067
Bergmann, W., and Feeney, R. J. (1951). Contributions to the Study of Marine Products. Xxxii. The Nucleosides of Sponges. I.1. J. Org. Chem. 16, 981–987. doi:10.1021/jo01146a023
Bhakuni, D., and Rawat, D. (2005). Bioactive Marine Nucleosides. Bioactive Mar. Nat. Prod., 208–234. doi:10.1007/1-4020-3484-9_8
Božić, T., Novaković, I., Gašić, M. J., Juranić, Z., Stanojković, T., Tufegdžić, S., et al. (2010). Synthesis and Biological Activity of Derivatives of the Marine Quinone Avarone. Eur. J. Med. Chem. 45, 923–929. doi:10.1016/j.ejmech.2009.11.033
Brown, A. J., Won, J. J., Graham, R. L., Dinnon, K. H., Sims, A. C., Feng, J. Y., et al. (2019). Broad Spectrum Antiviral Remdesivir Inhibits Human Endemic and Zoonotic Deltacoronaviruses with a Highly Divergent RNA Dependent RNA Polymerase. Antivir. Res. 169, 104541. doi:10.1016/j.antiviral.2019.104541
Buchanan, M. S., Carroll, A. R., Wessling, D., Jobling, M., Avery, V. M., Davis, R. A., et al. (2008). Clavatadine A, A Natural Product with Selective Recognition and Irreversible Inhibition of Factor XIa. J. Med. Chem. 51, 3583–3587. doi:10.1021/jm800314b
Chien, M., Anderson, T. K., Jockusch, S., Tao, C., Li, X., Kumar, S., et al. (2020). Nucleotide Analogues as Inhibitors of SARS-CoV-2 Polymerase, a Key Drug Target for COVID-19. J. Proteome Res. 19, 4690-4697. doi:10.1021/acs.jproteome.0c00392
Ciaglia, E., Malfitano, A., Laezza, C., Fontana, A., Nuzzo, G., Cutignano, A., et al. (2017). Immuno-Modulatory and Anti-inflammatory Effects of Dihydrogracilin A, a Terpene Derived from the Marine Sponge Dendrilla Membranosa. Int J Mol Sci. 18, 1643. doi:10.3390/ijms18081643
Coelho, C., Gallo, G., Campos, C. B., Hardy, L., and Würtele, M. (2020). Biochemical Screening for SARS-CoV-2 Main Protease Inhibitors. PLOS ONE. 15, e0240079. doi:10.1371/journal.pone.0240079
Costela-Ruiz, V. J., Illescas-Montes, R., Puerta-Puerta, J. M., Ruiz, C., and Melguizo-Rodríguez, L. (2020). SARS-CoV-2 Infection: The Role of Cytokines in COVID-19 Disease. Cytokine Growth Factor. Rev. 54, 62–75. doi:10.1016/j.cytogfr.2020.06.001
de Almeida Leone, P., Redburn, J., Hooper, J. N. A., and Quinn, R. J. (2000). PolyoxygenatedDysideaSterols that Inhibit the Binding of [I125] IL-8 to the Human Recombinant IL-8 Receptor Type A. J. Nat. Prod. 63, 694–697. doi:10.1021/np9904657
Diaz-Marrero, A. R., Austin, P., Van Soest, R., Matainaho, T., Roskelley, C. D., Roberge, M., et al. (2006). Avinosol, A Meroterpenoid-Nucleoside Conjugate with Antiinvasion Activity Isolated from the Marine SpongeDysideasp. Org. Lett. 8, 3749–3752. doi:10.1021/ol061333p
Du, Q.-S., Wang, S.-Q., Zhu, Y., Wei, D.-Q., Guo, H., Sirois, S., et al. (2004). Polyprotein Cleavage Mechanism of SARS CoV Mpro and Chemical Modification of the Octapeptide. Peptides. 25, 1857–1864. doi:10.1016/j.peptides.2004.06.018
Eastman, R. T., Roth, J. S., Brimacombe, K. R., Simeonov, A., Shen, M., Patnaik, S., et al. (2020). Remdesivir: A Review of its Discovery and Development Leading to Emergency Use Authorization for Treatment of COVID-19. ACS Cent. Sci. 6, 672–683. doi:10.1021/acscentsci.0c00489
Engel, M., Bachmann, M., Schröder, H. C., Rinkevich, B., Kljajic, Z., Uhlenbruck, G., et al. (1992). A Novel Galactose- and Arabinose-specific Lectin from the Sponge Pellina Semitubulosa: Isolation, Characterization and Immunobiological Properties. Biochimie. 74, 527–537. doi:10.1016/0300-9084(92)90150-d
Ersmark, K., Del Valle, J. R., and Hanessian, S. (2008). Chemistry and Biology of the Aeruginosin Family of Serine Protease Inhibitors. Angew. Chem. Int. Ed. 47, 1202–1223. doi:10.1002/anie.200605219
Fattorusso, E., and Taglialatela-Scafati, O. (2000). Two Novel Pyrrole-Imidazole Alkaloids from the Mediterranean Sponge Agelas Oroides. Tetrahedron Lett. 41, 9917–9922. doi:10.1016/s0040-4039(00)01764-0
Ferrándiz, M. L., Sanz, M. J., Bustos, G., Payá, M., Alcaraz, M. J., and De Rosa, S. (1994). Avarol and Avarone, Two New Anti-inflammatory Agents of Marine Origin. Eur. J. Pharmacol. 253, 75–82. doi:10.1016/0014-2999(94)90759-5
Garcia, J. V., Wahl, A., Gralinski, L., Johnson, C., Yao, W., Kovarova, M., et al. (2020). Acute SARS-CoV-2 Infection Is Highly Cytopathic, Elicits a Robust Innate Immune Response and Is Efficiently Prevented by EIDD-2801. Res. Sq, rs.3.rs-80404. doi:10.21203/rs.3.rs-80404/v1
Gentile, D., Patamia, V., Scala, A., Sciortino, M. T., Piperno, A., and Rescifina, A. (2020). Putative Inhibitors of SARS-CoV-2 Main Protease from a Library of Marine Natural Products: A Virtual Screening and Molecular Modeling Study. Mar. Drugs. 18, 225. doi:10.3390/md18040225
Golda, A., and Pyrc, K. (2008). Recent Antiviral Strategies against Human Coronavirus-Related Respiratory Illnesses. Curr. Opin. Pulm. Med. 14, 248–253. doi:10.1097/mcp.0b013e3282f7646f
Gordon, C. J., Tchesnokov, E. P., Woolner, E., Perry, J. K., Feng, J. Y., Porter, D. P., et al. (2020). Remdesivir Is a Direct-Acting Antiviral that Inhibits RNA-dependent RNA Polymerase from Severe Acute Respiratory Syndrome Coronavirus 2 with High Potency. J. Biol. Chem. 295, 6785–6797. doi:10.1074/jbc.ra120.013679
Greve, H., Meis, S., Kassack, M. U., Kehraus, S., Krick, A., Wright, A. D., et al. (2007). New Iantherans from the Marine Sponge Ianthella Quadrangulata: Novel Agonists of the P2Y11 Receptor. J. Med. Chem. 50, 5600–5607. doi:10.1021/jm070043r
Grum-Tokars, V., Ratia, K., Begaye, A., Baker, S. C., and Mesecar, A. D. (2008). Evaluating the 3C-like Protease Activity of SARS-Coronavirus: Recommendations for Standardized Assays for Drug Discovery. Virus. Res. 133, 63–73. doi:10.1016/j.virusres.2007.02.015
Gulavita, N. K., Wright, A. E., Kelly-Borges, M., Longley, R. E., Yarwood, D., and Sills, M. A. (1994). Eryloside E from an Atlantic Sponge Erylus Goffrilleri. Tetrahedron Lett. 35, 4299–4302. doi:10.1016/s0040-4039(00)73338-7
Gunasekera, S. P., Cranick, S., and Longley, R. E. (1989). Immunosuppressive Compounds from a Deep Water Marine Sponge, Agelas Flabelliformis. J. Nat. Prod. 52, 757–761. doi:10.1021/np50064a015
Hamann, M. T., Scheuer, P. J., and Kelly-Borges, M. (1993). Biogenetically Diverse, Bioactive Constituents of a Sponge, Order Verongida: Bromotyramines and Sesquiterpene-Shikimate Derived Metabolites. J. Org. Chem. 58, 6565–6569. doi:10.1021/jo00076a012
Han, G. (2020). Histamine Antagonists to Temper the Cytokine Overproduction in Gastrointestinal Cells Infected by SARS-CoV-2. Basel: MDPI AG
Iwu, M. M., Okunji, C. O., Tchimene, M., and Sokomba, E. (2020). Antiviral Activity of Andrographolide against Ebola Virus, Dengue fever and SARS coronavirus. Research Square. doi:10.21203/rs.3.rs-24311/v1
Jena, N. (2020). Identification of Potent Drugs and Antiviral Agents for the Treatment of the SARS-CoV-2 Infection. ChemRxiv
Kato, Y., Fusetani, N., Matsunaga, S., and Hashimoto, K. (1985). Bioactive Marine Metabolites IX. Mycalisines A and B, Novel Nucleosides Which Inhibit Cell Division of Fertilized Starfish Eggs, from the Marine Sponge Sp. Tetrahedron Lett. 26, 3483–3486. doi:10.1016/s0040-4039(00)98670-2
Khalili, J. S., Zhu, H., Mak, N. S. A., Yan, Y., and Zhu, Y. (2020). Novel Coronavirus Treatment with Ribavirin: Groundwork for an Evaluation Concerning COVID‐19. J. Med. Virol. 92, 740-746. doi:10.1002/jmv.25798
Khan, M. T., Ali, A., Wang, Q., Irfan, M., Khan, A., Zeb, M. T., et al. (2020). Marine Natural Compounds as Potents Inhibitors against the Main Protease of SARS-CoV-2-A Molecular Dynamic Study. J. Biomol. Struct. Dyn., 1–11. doi:10.1080/07391102.2020.1769733
Khater, S., Dasgupta, N., and Das, G. (2020). Combining SARS-CoV-2 Proofreading Exonuclease and RNA-dependent RNA Polymerase Inhibitors as a Strategy to Combat COVID-19: A High-Throughput in Silico Screen. OSF Preprints
Kim, B. G., Chun, T. G., Lee, H.-Y., and Snapper, M. L. (2009). A New Structural Class of S-Adenosylhomocysteine Hydrolase Inhibitors. Bioorg. Med. Chem. 17, 6707–6714. doi:10.1016/j.bmc.2009.07.061
Laffoley, D., Baxter, J. M., Amon, D. J., Claudet, J., Hall-Spencer, J. M., Grorud-Colvert, K., et al. (2020). Evolving the Narrative for Protecting a Rapidly Changing Ocean. Aquat. Conserv. 1–23 doi:10.1002/aqc.3512
Ledderose, C., Bromberger, S., Slubowski, C. J., Sueyoshi, K., Aytan, D., Shen, Y., et al. (2020). The Purinergic Receptor P2Y11 Choreographs the Polarization, Mitochondrial Metabolism, and Migration of T Lymphocytes. Sci. Signaling. 13, eaba3300. doi:10.1126/scisignal.aba3300
Lee, K., Kim, D.-E., Jang, K.-S., Kim, S.-J., Cho, S., and Kim, C. (2017). Gemcitabine, a Broad-Spectrum Antiviral Drug, Suppresses Enterovirus Infections through Innate Immunity Induced by the Inhibition of Pyrimidine Biosynthesis and Nucleotide Depletion. Oncotarget. 8, 115315–115325. doi:10.18632/oncotarget.23258
Li, H., Shinde, P. B., Lee, H. J., Yoo, E. S., Lee, C.-O., Hong, J., et al. (2009). Bile Acid Derivatives from a Sponge-Associated Bacterium Psychrobacter Sp. Arch. Pharm. Res. 32, 857–862. doi:10.1007/s12272-009-1607-1
Li, Q., Guan, X., Wu, P., Wang, X., Zhou, L., Tong, Y., et al. (2020). Early Transmission Dynamics in Wuhan, China, of Novel Coronavirus-Infected Pneumonia. N. Engl. J. Med. 382, 1199–1207. doi:10.1056/nejmoa2001316
Lira, S. P. d., Seleghim, M. H. R., Williams, D. E., Marion, F., Hamill, P., Jean, F., et al. (2007). A SARS-Coronovirus 3CL Protease Inhibitor Isolated from the Marine Sponge Axinella Cf. Corrugata: Structure Elucidation and Synthesis. J. Braz. Chem. Soc. 18, 440–443. doi:10.1590/s0103-50532007000200030
Momattin, H., Al-Ali, A. Y., and Al-Tawfiq, J. A. (2019). A Systematic Review of Therapeutic Agents for the Treatment of the Middle East Respiratory Syndrome Coronavirus (MERS-CoV). Trav. Med. Infect. Dis. 30, 9–18. doi:10.1016/j.tmaid.2019.06.012
Müller, W. E., Maidhof, A., Zahn, R. K., Schröder, H. C., Gasić, M. J., Heidemann, D., et al. (1985). Potent Antileukemic Activity of the Novel Cytostatic Agent Avarone and its Analogues In Vitro and In Vivo. Cancer Res. 45, 4822–4826.
Nakao, Y., Masuda, A., Matsunaga, S., and Fusetani, N. (1999). Pseudotheonamides, Serine Protease Inhibitors from the Marine SpongeTheonella Swinhoei1. J. Am. Chem. Soc. 121, 2425–2431. doi:10.1021/ja9831195
Nasu, S. S., Yeung, B. K. S., Hamann, M. T., Scheuer, P. J., Kelly-Borges, M., and Goins, K. (1995). Puupehenone-related Metabolites from Two Hawaiian Sponges, Hyrtios Spp. J. Org. Chem. 60, 7290–7292. doi:10.1021/jo00127a039
Niloufar, Y., Amene, S., and Nima, R. (2020). Anosmia: a Missing Link in the Neuroimmunology of Coronavirus Disease 2019 (COVID-19). Rev. Neurosciences. 31, 691–701. doi:10.1515/revneuro-2020-0039
Pankiewicz, K. W. (2000). Fluorinated Nucleosides. Carbohydr. Res. 327, 87–105. doi:10.1016/s0008-6215(00)00089-6
Pettit, G. R., Clewlow, P. J., Dufresne, C., Doubek, D. L., Cerny, R. L., and Rützler, K. (1990). Antineoplastic Agents. 193. Isolation and Structure of the Cyclic Peptide Hymenistatin 1. Can. J. Chem. 68, 708–711. doi:10.1139/v90-110
Pruijssers, A. J., and Denison, M. R. (2019). Nucleoside Analogues for the Treatment of Coronavirus Infections. Curr. Opin. Virol. 35, 57–62. doi:10.1016/j.coviro.2019.04.002
Quijano, L., Cruz, F., Navarrete, I., Gómez, P., and Rios, T. (1994). Alkyl Glycerol Monoethers in the Marine spongeDesmapsamma Anchorata. Lipids. 29, 731–734. doi:10.1007/bf02538919
Romo, D., Rzasa, R. M., Shea, H. A., Park, K., Langenhan, J. M., Sun, L., et al. (1998). Total Synthesis and Immunosuppressive Activity of (−)-Pateamine A and Related Compounds: Implementation of a β-Lactam-Based Macrocyclization. J. Am. Chem. Soc. 120, 12237–12254. doi:10.1021/ja981846u
Seley-Radtke, K. L., and Yates, M. K. (2018). The Evolution of Nucleoside Analogue Antivirals: A Review for Chemists and Non-chemists. Part 1: Early Structural Modifications to the Nucleoside Scaffold. Antivir. Res. 154, 66–86. doi:10.1016/j.antiviral.2018.04.004
Sepay, N., Sekar, A., Halder, U. C., Alarifi, A., and Afzal, M. (2020). Anti-COVID-19 Terpenoid from Marine Sources: a Docking, ADMET and Molecular Dynamics Study. J. Mol. Struct. 1228, 129433. doi:10.1016/j.molstruc.2020.129433
Shannon, A., Le, N. T.-T., Selisko, B., Eydoux, C., Alvarez, K., Guillemot, J.-C., et al. (2020a). Remdesivir and SARS-CoV-2: Structural Requirements at Both Nsp12 RdRp and Nsp14 Exonuclease Active-Sites. Antiviral Res. 178, 104793. doi:10.1016/j.antiviral.2020.104793
Shannon, A., Selisko, B., Huchting, J., Touret, F., Piorkowski, G., Fattorini, V., et al. (2020b). Rapid Incorporation of Favipiravir by the Fast and Permissive Viral RNA Polymerase Complex Results in SARS-CoV-2 Lethal Mutagenesis. Nat. Commun. 11, 1–9. doi:10.1038/s41467-020-18463-z
Sheahan, T. P., Sims, A. C., Zhou, S., Hill, C., Leist, S. R., Schaefer, A., et al. (2020). An orally bioavailable broad-spectrum antiviral inhibits SARS-CoV-2 in human airway epithelial cell cultures and multiple coronaviruses in mice. Sci. Translational Med. 12, eabb5883. doi:10.1126/scitranslmed.abb5883
Simonis, A., Theobald, S. J., Fätkenheuer, G., Rybniker, J., and Malin, J. J. (2021). A Comparative Analysis of Remdesivir and Other Repurposed Antivirals against SARS-CoV-2. EMBO Mol. Med. 13, e13105. doi:10.15252/emmm.202013105
Surti, M., Patel, M., Adnan, M., Moin, A., Ashraf, S. A., Siddiqui, A. J., et al. (2020). Ilimaquinone (Marine Sponge Metabolite) as a Novel Inhibitor of SARS-CoV-2 Key Target Proteins in Comparison with Suggested COVID-19 Drugs: Designing, Docking and Molecular Dynamics Simulation Study. RSC Adv. 10, 37707–37720. doi:10.1039/d0ra06379g
Takei, M., Burgoyne, D. L., and Andersen, R. J. (1994). Effect of Contignasterol on Histamine Release Induced by Anti-immunoglobuline from Rat Peritoneal Mast Cells. J. Pharm. Sci. 83, 1234–1235. doi:10.1002/jps.2600830909
Tang, Y., Liu, J., Zhang, D., Xu, Z., Ji, J., and Wen, C. (2020). Cytokine Storm in COVID-19: The Current Evidence and Treatment Strategies. Front. Immunol. 11, 1708. doi:10.3389/fimmu.2020.01708
Tong, S., Su, Y., Yu, Y., Wu, C., Chen, J., Wang, S., et al. (2020). Ribavirin Therapy for Severe COVID-19: a Retrospective Cohort Study. Int. J. Antimicrob. Agents 56, 106114. doi:10.1016/j.ijantimicag.2020.106114
Vijayaraj, R., Altaff, K., Rosita, A. S., Ramadevi, S., and Revathy, J. (2020). Bioactive Compounds from Marine Resources against Novel Corona Virus (2019-nCoV): In Silico Study for Corona Viral Drug. Nat. Product. Res., 1–5. doi:10.1080/14786419.2020.1791115
Wang, Y., Anirudhan, V., Du, R., Cui, Q., and Rong, L. (2021). RNA‐dependent RNA Polymerase of SARS‐CoV‐2 as a Therapeutic Target. J. Med. Virol. 93, 300–310. doi:10.1002/jmv.26264
Wang, Y., Zhang, D., Du, G., Du, R., Zhao, J., Jin, Y., et al. (2020). Remdesivir in Adults with Severe COVID-19: a Randomised, Double-Blind, Placebo-Controlled, Multicentre Trial. The Lancet 395, 1569–1578. doi:10.1016/s0140-6736(20)31022-9
Yosief, T., Rudi, A., Stein, Z., Goldberg, I., Gravalos, G. M. D., Schleyer, M., et al. (1998). Asmarines A-C; Three Novel Cytotoxic Metabolites from the Marine Sponge Raspailia Sp. Tetrahedron Lett. 39, 3323–3326. doi:10.1016/s0040-4039(98)00481-x
Zandi, K., Amblard, F., Musall, K., Downs-Bowen, J., Kleinbard, R., Oo, A., et al. (2020). Repurposing Nucleoside Analogs for Human Coronaviruses. Antimicrob. Agents Chemother. 65, e01652–20. doi:10.1128/AAC.01652-20
Zhang, P., Bao, B., Dang, H. T., Hong, J., Lee, H. J., Yoo, E. S., et al. (2009). Anti-inflammatory Sesquiterpenoids from a Sponge-Derived FungusAcremoniumsp. J. Nat. Prod. 72, 270–275. doi:10.1021/np8006793
Zhang, L., Zhang, D., Yuan, C., Wang, X., Li, Y., Jia, X., et al. (2020a). Role of 1'-Ribose Cyano Substitution for Remdesivir to Effectively Inhibit Both Nucleotide Addition and Proofreading in SARS-CoV-2 Viral RNA Replication. bioRxiv. doi:10.1039/d0cp05948j
Keywords: COVID-19, SARS-CoV-2, marine sponge, nucleoside analogues, MPRO, immunomodulators
Citation: Hamoda AM, Fayed B, Ashmawy NS, El-Shorbagi A-NA, Hamdy R and Soliman SSM (2021) Marine Sponge is a Promising Natural Source of Anti-SARS-CoV-2 Scaffold. Front. Pharmacol. 12:666664. doi: 10.3389/fphar.2021.666664
Received: 11 February 2021; Accepted: 28 April 2021;
Published: 13 May 2021.
Edited by:
Salvatore Salomone, University of Catania, ItalyCopyright © 2021 Hamoda, Fayed, Ashmawy, El-Shorbagi, Hamdy and Soliman. This is an open-access article distributed under the terms of the Creative Commons Attribution License (CC BY). The use, distribution or reproduction in other forums is permitted, provided the original author(s) and the copyright owner(s) are credited and that the original publication in this journal is cited, in accordance with accepted academic practice. No use, distribution or reproduction is permitted which does not comply with these terms.
*Correspondence: Rania Hamdy, cmFuaWFfaGFtZHkyMDAwQHlhaG9vLmNvbQ==; Sameh S. M. Soliman, c3NvbGltYW5Ac2hhcmphaC5hYy5hZQ==
Disclaimer: All claims expressed in this article are solely those of the authors and do not necessarily represent those of their affiliated organizations, or those of the publisher, the editors and the reviewers. Any product that may be evaluated in this article or claim that may be made by its manufacturer is not guaranteed or endorsed by the publisher.
Research integrity at Frontiers
Learn more about the work of our research integrity team to safeguard the quality of each article we publish.