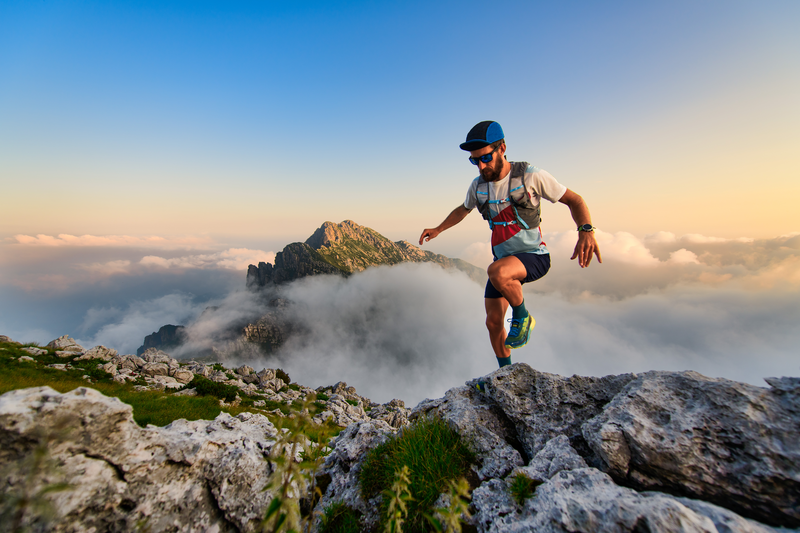
94% of researchers rate our articles as excellent or good
Learn more about the work of our research integrity team to safeguard the quality of each article we publish.
Find out more
ORIGINAL RESEARCH article
Front. Pharmacol. , 05 July 2021
Sec. Ethnopharmacology
Volume 12 - 2021 | https://doi.org/10.3389/fphar.2021.664607
Background:Triphala is a traditional polyherbal formula used in Indian Ayurvedic and Chinese Tibetan medicine. A wide range of biological activities have been attributed to Triphala, but the impact of various extraction methods on efficacy has not been determined.
Purpose: The study aimed to evaluate Triphala extracts obtained by various methods for their hepatoprotective effects and molecular mechanisms in a mouse model of carbon tetrachloride (CCl4)-induced liver injury.
Methods: HPLC fingerprinting was used to characterize the chemical characteristics of Triphala extracts obtained by (a) 0.5 h ultrasonication, (b) 2 h reflux, and (c) 4 h reflux. Hepatoprotective efficacy was evaluated in a mouse model of CCl4-induced liver damage. Serum levels of alanine transaminase (ALT) and aspartate aminotransferase (AST) were measured, as well as the liver antioxidant and inflammatory markers malondialdehyde superoxide dismutase glutathione peroxidase (GSH-Px), TNF-α, and IL-6. Gene and protein expression of Nrf-2 signaling components Nrf-2, heme oxygenase (HO-1), and NADPH Quinone oxidoreductase (NQO-1) in liver tissue were evaluated by real-time PCR and western blotting.
Results: Chemical analysis showed a clear difference in content between extracts produced by ultrasonic and reflux methods. The pharmacological analysis showed that all three Triphala extracts reduced ALT, AST, MDA, TNF-α, and IL-6 levels and increased SOD and GSH-Px. Triphala extracts also induced transcript and protein expression of Nrf-2, HO-1, and NQO-1.
Conclusion: Triphala extract prevents CCl4-induced acute liver injury. The ultrasonic extract of Triphala was most effective, suggesting that hepatoprotection may be related to the larger tannins via activation of Nrf-2 signaling.
As one of the largest metabolic organs in the human digestive system, the liver has functions of transformation, excretion, immunity, and detoxification and is susceptible to chemical liver injury caused by various pathogenic and stimulating factors. Aggravated liver cell damage, if left unchecked, can lead to hepatitis, liver fibrosis, or irreversible cirrhosis, which eventually leads to liver cancer (Gu and Manautou, 2012). Studies have shown that the primary pathogenic mechanism of chemical liver injury is the oxidative stress caused by the accumulation of reactive oxygen radicals in the liver and the resulting inflammatory response (Souza et al., 2018).
Triphala is a traditional polyherbal medicine comprised of Terminalia chebula Retz., Terminalia bellirica (Gaertn.) Roxb.., and Phyllanthus emblica Linn., each of which contains a variety of chemical substances with biological activity (Baliga et al., 2012; Zhao et al., 2015; Chen et al., 2019; Nigam et al., 2020). Triphala has a long history in Indian and Chinese traditional medicine as a complementary and alternative therapy for chronic diseases (Jaiswal et al., 2016; Prasad and Srivastava, 2020). Triphala is considered a multipurpose therapeutic drug with anti-inflammatory, analgesic, hypoglycemic, antibacterial, and antioxidant properties (Srikumar et al., 2006; Rasool and Sabina, 2007; Peterson et al., 2017; Luo et al., 2019). In traditional usage, Triphala is applied in the treatment of gastritis, hepatitis, colitis and other digestive diseases (Deep et al., 2005; Li et al., 2018a; Nariya et al., 2011; Olennikov et al., 2015; Rayudu and Raju, 2014; Wang et al., 2018). Triphala also has potential uses in the treatment of obesity and diabetes, as well as retinopathy and cardiovascular and cerebrovascular diseases (Saravanan et al., 2007; Gurjar et al., 2012; Kamali et al., 2012; Lu et al., 2012; Ganeshpurkar et al., 2015). Although Triphala is widely used throughout Asia, clinical safety data are lacking. In Thailand, a phase I clinical observational trial was performed in 20 healthy volunteers (10 male, 10 female) to verify the safety of Triphala. In that study, a water extract of Triphala had no obvious side effects (Phetkate et al., 2020). Pharmaceutical analyses have shown that Triphala is rich in saponins, terpenes, tannins, flavonoids, and phenolic acids (Avula et al., 2013). The hydrolyzed tannins in Triphala are considered the primary inducers of biological activity (Pawar et al., 2009; Russell et al., 2011).
Tannins are known to provide hepatoprotection (Olennikov et al., 2015). There are various research models for inducing liver injury in which Triphala and related plant medicinals have been tested for hepatoprotective activity. However, the preparation methods used in these studies vary and may confound the results (Table 1). In a previous study Huang et al. (2019), we found that the Phyllanthus emblica component of Triphala exhibited hydrolytic tannin conversion in heat- and reflux-mediated extraction, suggesting various extraction methods may yield extracts with variable biological activities. Published studies include the use of common hot and cold extraction methods for Triphala and related plants, but extraction temperature influences the chemical composition of Triphala.
In this study, we compared the hepatoprotective efficacy of Triphala extracts obtained by various methods. We established a mouse model of carbon tetrachloride (CCl4)-induced liver damage, then monitored for the presence of oxidative damage markers alanine transaminase (ALT) and aspartate aminotransferase (AST), as well as the liver antioxidant and inflammatory markers malondialdehyde (MDA), superoxide dismutase (SOD), glutathione peroxidase (GSH-Px), as well as inflammatory factors and Nrf2 signaling-related genes and proteins to explore the protective effect and mechanism of the various Triphala extracts. The results of this study will support future clinical applications of Triphala.
Terminalia chebula Retz., Terminalia bellirica (Gaertn.) Roxb. and Phyllanthus emblica Linn. were purchased from Zhongyong Pharmaceutical Co., Ltd. (Sichuan, China). All herbs were identified by Professor Jin Pei, deposited at the Chengdu University of TCM, and met Chinese Pharmacopoeia requirements (2015 Edition). Standards of Chebulic acid (CHB180831), Gallic acid (CHB171107), Punicalin (CHB190211), Catechin (CHB170301), Epigallocatechin gallate (CHB180307), Epicatechin (CHB180831), Corilagin (CHB190106), Gallocatechin gallate (CHB180327), 1,3,6-tri-O-galloylglucose (CHB191021), Epicatechin gallate (CHB170317), Ferulic acid (CHB 180201), Chebulagic acid (CHB190109), 1,2,3,4,6-O-penta-galloyl glucose (CHB190125), Chebulinic acid (CHB190124), and Ellagic acid (CHB170303) were purchased from Chengdu Chroma-Biotechnology Co., Ltd. (Chengdu, China), the purity of all standard products is ≥ 98%. CCl4 (20181010) was purchased from Tianjin Bodi Chemical Co., Ltd. (Tianjin, China), Dimethyl diphenyl bicarboxylate (DDB) (200603) was purchased from Bond Pharmaceuticals Group Co., Ltd. (Wenling, China). Test kits for ALT (20191003), AST (20191005), SOD (20191101), MDA (20191028), and GSH-Px (20191029) were obtained from Nanjing Jiancheng Bioengineering Institute (Nanjing, China). TNF-α (A28291045) and IL-6 (A20691132) were obtained by Multi Sciences Biotech Co., Ltd. (Hangzhou, China).
Plant materials were prepared in traditional proportions (3 Terminalia chebula: 2 Terminalia bellirica: 2.4 Phyllanthus emblica). Triphala is extracted in water solvent and extracted by ultrasonication for 0.5 h (U-0.5 h) at 20°C, reflux for 2 h (R-2 h) at 100°C, and reflux for 4 h (R-4 h) at 100°C. The solution concentration was 0.24 g/ml, and the extraction values of the three methods were 12.74%, 34.91%, and 41.30%, respectively. The extracts were filtered, and the filtrates were stored in a refrigerator at 4°C.
Kunming mice (30 ± 2 g) were supplied by Dashuo Laboratory Animal Co. Ltd. (Chengdu, China). The animals were housed at room temperature under a 12:12 light:dark schedule with food and water ad libitum. All experiments were performed in strict accordance with the recommendations of China’s “Guidelines for the Care and Use of Laboratory Animals.” The experimental protocol was approved by the Ethics Committee of the Affiliated Hospital of Chengdu University of TCM (Approval ID: 2018BL-002).
The animals were divided into nine groups, with an average of six mice per group. The experimental groups received one of the three Triphala extracts (U-0.5, R-2, and R-4 h), with treatments administered by gavage at 1.2 g/kg for the low-dose group (L) and 2.4 g/kg for the high-dose group (H). Dosages were calculated based on the clinical dosage of Triphala by the body surface area method. Positive controls received DDB (7.5 mg/kg) by gavage. Normal (N) and Model (M) controls were given the same volume of distilled water by gavage. All groups were treated intragastrically once daily for a week. Two hours after treatment on the last day, all mice but those in the N group were given 0.1% CCl4 vegetable oil solution (10 ml/kg body weight) by intraperitoneal injection, while the mice in the group N were merely given the same amount of vegetable oil. All animals were fasted overnight and sacrificed after 16 h. Blood and liver tissues were collected immediately. The collected blood was centrifuged at 4,000 rpm at 4°C for 10 min and stored at –20°C. The liver tissues were dissected and immediately rinsed with ice-cold saline. One portion was immediately refrigerated at –80°C, and the other was fixed with 4% paraformaldehyde for histopathological analysis.
The sample concentration is too high for liquid chromatography analysis, so samples were diluted 10-fold and analyzed by Shimadzu LC-20AT HPLC (Shimadzu Corporation, Kyoto, Japan) on Welchrom C18 columns (4.6 × 250 mm, 5 μm; Shanghai Yuexu Material Technology Co., Ltd., China). Detection conditions were as follows: wavelength 270 nm, column temperature was 25°C, mobile phase flow rate 1 ml min−1, injection volume 10 μL. The mobile phase was 0.2% aqueous phosphoric acid and methanol, adopting a gradient elution program of 5% of B at 0–6 min, 5%–7% of B at 6–15 min, 7%–15% of B at 15–20 min, 15%–21% of B at 20–25 min, 21%–22% of B at 25–41 min, 22%–28% of B at 41–47 min, 28%–32% of B at 47–55 min, 32%–37% of B at 55–61 min, 37%–38% of B at 61–62 min, 38%–39% of B at 62–67 min, 39%–45% of B at 67–70 min, 45%–65% of B at 70–80 min, 65%–5% of B at 80–90 min (Huang et al., 2018).
The analysis was performed on ultra-high performance liquid chromatography coupled with quadrupole-orbitrap high resolution mass (UPLC-Q-Orbitrap HRMS) (Thermo Fisher, United States). Chromatographic separation was carried out at 30°C on Thermo Scientific Accucore C18 (2.1 mm × 100 mm, 2.6 μm). The mobile phase consisted of (A) water with 0.1% formic acid and (B) methanol. The gradient was as follows: 0–25 min, 5% B isocratic; 25–30 min, 5–95% B linear; 30–35 min, 50% B isocratic. The flow rate was 0.3 ml/min. The MS acquisition was performed using both positive and negative ionization mode. The heated electrospray ionization parameters as follows: sheath gas flow 35 arb (arbitrary units), auxiliary gas flow 10 arb, spray voltage 3.0 kV for positive ionization and negative ionization, capillary temperature 320°C, probe heater temperature 350°C, the ion scanning range is m/z 100–1500.
Under the above conditions, the chemical constituents of the three extracts of Triphala were qualitatively analyzed. Use Xcalibur 3.0 software to process the total ion chromatogram of the sample in positive and negative ion mode, match the measured spectrum with the mzCloud and mzVault network databases, and then combine the precise relative molecular mass of the target component, reference substances, MassBank, and Human Metabolome database (HMDB), PubMed, ChemSpider and related references, for manual identification and identification.
Every two days throughout the experiment, the mice were weighed, and changes were noted. Dissected mouse livers were weighed, and the liver index was calculated as liver index = liver mass (g)/mouse body weight (g) × 100%.
Serum levels of ALT and AST were determined using standard kits according to the manufacturer’s instructions.
The liver tissue stored in 4% paraformaldehyde solution at 4°C was embedded in paraffin, then sliced by microtome, stained by hematoxylin and eosin (H&E), and finally examined under an optical microscope (Nikon eclipse ci, Japan).
Hepatic levels of MDA, SOD, GSH-Px, TNF-α, and IL-6 were determined according to the manufacturer’s instructions of standard assay kits.
Total RNA was extracted from mouse liver using Trizol (Invitrogen Life Technologies), followed by reverse transcription to cDNA (Applied Biological Materials Inc.) and PCR amplification (Shanghai Hongshi Medical Technology Co., Ltd.). Amplification conditions were: initial denaturation at 95°C for 10 min, followed by 40 cycles of denaturation at 95 °C for 15 s, annealing at 60°C for 15 s, and extension for 60 s. Amplification targets were the Nrf-2, NQO-1, and HO-1 genes, with primers described in Supplementary Table S1. Relative expression was determined by the 2−ΔΔCT method.
Liver tissue was weighed, washed 2–3 times with ice-cold PBS, mixed with a 10-fold volume of RIPA, homogenized on ice, centrifuged at 12,000 g for 10 min, and the supernatant collected. Protein concentration was determined by the BCA method. Total protein (15 μL) was separated by 10% SDS-PAGE, then transferred to a PVDF membrane (Millipore Corporation, United States) overnight at 25 V. The membrane was sealed in 5% defatted milk/TBST for 1 h and incubated with primary antibody overnight at 4°C. The membrane was rinsed three times with TBST at room temperature, incubated with secondary antibody at room temperature for 30 min, then washed three times with TBST. Protein bands were detected by ECL, and the images were collected with a chemiluminescence imaging system (Shanghai Clinx Scientific Instrument Co., Ltd.). Grayscale analysis was performed with the on-instrument ChemiScope software.
Data analysis was performed using SPSS 21.0 statistical analysis software with results expressed as mean ± standard deviation. Analysis of variance (ANOVA) and LSD t-tests were used to compare multiple groups. Significance was defined as p < 0.05.
HPLC chromatograms are shown in Figure 1A. Through the comparison of reference substance, we carried out quantitative analysis of 15 components, as shown in Table 2. We compared the differences of 9 components with large changes in peak area during the decoction of Triphala (Figure 1B). Multivariate statistical methods were used to analyze the fingerprint data, and SIMCA-P 13.0 software was used to perform PCA analysis. The PCA score chart shows differences between the products of the three extraction methods, particularly between the ultrasonic and reflux methods (Figure 1C). Orthogonal projections to latent structures discriminant (OPLS-DA) and S-plot analysis were used to find differences in chemical markers. The S-plot is a loading profile that depicts the influence of variables on biomarker selection. Among all the 186 variables in the S-plot, we identified 10 chemical markers that differed most between the Triphala preparations (Figure 1D). The retention times for these markers were 15.230, 56.484, 54.018, 8.614, 79.821, 60.271, 32.466, 12.475, 64.938, and 76.437. By comparison with the reference substance’s retention time, nine of the components were determined to be gallic acid, 1,3,6-tri-O-galloylglucose, corilagin, chebulic acid, ellagic acid, epicatechin gallate, catechin, chebulagic acid, and chebulinic acid. These 9 identified actives have a wide range of biological activities (Table 3). The component most reduced by extraction is hydrolyzed tannin (chebulinic and chebulagic acid), while tannin hydrolysate was greatly increased (corilagin, gallic acid, chebulic acid, and ellagic acid).
FIGURE 1. (A) HPLC of Triphala in different extraction process (1. Chebulic acid, 2. Gallic acid, 3. Punicalin 4. Catechin, 5. Epigallocatechin gallate, 6. Epicatechin, 7. Corilagin, 8. Gallocatechin gallate, 9.1,3,6-tri-O-galloylglucose, 10. Epicatechin gallate, 11. Ferulic acid, 12. Chebulagic acid, 13. 1,2,3,4,6-penta-O-galloyl glucose, 14. Chebulinic acid, 15. Ellagic acid). (B) The variation of peak area of composition in different decoction time. (C) PCA score of extracts of Triphala. (D) S-plot of extracts of Triphala.
Chebulinic acid decreased most dramatically during the decoction of Triphala, likely due to its chemical structure. Chebulinic acid is formed by the condensation of small molecule tannins and glucose through five ester bonds. It is not stable under heat and easily decomposes into one molecule of chebulic acid and one molecule of 1,3,6-Tri-O-galloyl glucose, which is then decomposed into three molecules of gallic acid and one molecule of glucose. Chebulagic acid is also unstable under heated conditions and is hydrolyzed to produce one molecule of chebulic acid and one molecule of corilagin. Corilagin is composed of a galloyl, a hexahydroxybiphenoyl (HHDP) and a glucosyl moiety by three ester bonds. When heated, it will continue to hydrolyze to produce gallic acid, ellagic acid, and glucose. A schematic diagram of the hydrolysis of chebulinic and chebulagic acid is shown in Figure 2. Triphala also contains catechins, condensed tannins that undergo condensation reactions and precipitate, thus reducing the final extract’s content.
Through the UPLC-Q-Orbitrap HRMS, a total of 106 compounds were identified in the three extracts of Triphala, among which 76 were common components, as shown in Table 4. The results showed that Triphala mainly contained tannins, phenolic acids, flavonoids, alkaloid sugars and glycosides, vitamins, amino acids, fatty acids, organic acids, and a small amount of coumarins, terpenoids, sterols, lignin.
There was no significant difference in animal weights between groups (Supplementary Table S2). Treatments yielded no adverse reactions, including nausea, vomiting, and loss of appetite. The dosages chosen had no significant impact on physiological growth and had no obvious toxic side effects.
The liver index is an important indicator of pathological changes caused by liver injury (Figure 3). Compared with the N group, the model groups’ liver index increased significantly (p < 0.01), indicating that hepatotoxicity was successfully modeled. Compared with the M group, the liver index of the treatment group was reduced. Group DDB and U-0.5 h differed significantly (p < 0.01), and the high dose R-2 h also differed significantly (p < 0.01). The results show that all Triphala extracts alleviated liver damage in mice, but the ultrasonic extraction method was most effective.
FIGURE 3. Comparison of mouse liver index (
Compared with the N group, ALT and AST levels in group M were significantly increased (p < 0.01; Figure 4A,B). In contrast, all mice treated with U-0.5, R-2, and the high dose R-4 h exhibited significantly reduced ALT and AST (p < 0.01). However, mice treated with a low dose of the 4-h reflux extract showed a slight but insignificant decrease in enzyme activity (p > 0.05).
FIGURE 4. Comparison of serum levels of ALT (A) and AST (B) (
As in Figure 4C, in the normal group, the hepatic lobules were clear in structure, the cords were neatly arranged, and the hepatocytes were rich in cytoplasm and normal in morphology. In the model group, the hepatic lobule structure was destroyed, most hepatocytes were swollen, many hepatocytes were steatotic and necrotic, and many inflammatory cells were infiltrated. In contrast, the liver tissues of the DDB group were nearly normalized; the degree of the hepatocellular lesion was relatively mild. In the U-0.5 h treatment group, the dose dependence was not significant, the hepatic lobule structure was clear, the hepatocyte cytoplasm was abundant, the morphological structure was normal, and a small amount of hepatocyte necrosis, nuclear fragmentation, or dissolution was seen at the edge of the local tissue. In the R-2 h treatment group, the dose dependence was significant. In the high-dose group, the hepatic lobule structure was clear, more hepatocytes showed mild degeneration, and smaller round vacuoles were seen in the cytoplasm. In the low-dose group, a large amount of hepatocyte necrosis, nuclear fragmentation, and dissolution was seen around the central vein and the junction area, and a small amount of hepatocyte balloon-like degeneration was seen at the edge of the necrotic focus. The cells were swollen, the nuclei were centered, and the cytoplasm was vacuolated. In the R-4 h treatment group, the degree of liver cell damage was similar to the model group. The hepatic lobule structure was destroyed, and many inflammatory cells were infiltrated.
Compared with the N group, MDA levels in the model group were significantly increased. The levels of SOD and GSH-Px were significantly reduced (p < 0.01; Figure 5), indicating that the liver tissue experienced intense oxidative stress and mounted a lipid peroxidation response. Compared with the M group, MDA levels in the different treatment groups were significantly reduced (p < 0.01), the levels of SOD were significantly increased (p < 0.01), and the levels of GSH-Px were also significantly increased (p < 0.05 or 0.01). Thus, we conclude that Triphala improved the antioxidant response, relieving liver damage caused by CCl4.
FIGURE 5. Comparison of liver levels of MDA (A), SOD (B) and GSH-Px (C) (
Compared with the N group, IL-6 and TNF-α levels in the model group were significantly increased (p < 0.01; Figure 6). Compared with the M group, the levels of TNF-α were significantly reduced in the treatment groups (p < 0.01). IL-6 levels were significantly reduced in the U-0.5 and H-R-2 h groups (p < 0.01) and significantly differed in the L-R-2 and R-4 h groups (p < 0.05). Thus, the ultrasonic extract of Triphala provided a greater anti-inflammatory effect.
FIGURE 6. Comparison of liver levels of IL-6 (A), TNF-α (B) (
The expression levels of Nrf-2, HO-1, and NQO-1 mRNA in the nuclei of liver tissues in the treatment groups were significantly increased in the treatment groups (Figure 7). Specifically, Nrf-2, HO-1, and NQO-1 mRNA expression was highest in the group treated with the ultrasonic Triphala extract (p < 0.01). CCl4 also promotes the expression of these genes.
FIGURE 7. Relative expression of Nrf-2 (A), HO-1 (B) and NQO-1 (C) mRNA (
Expression levels of Nrf-2, HO-1, and NQO-1 protein in the nuclei of liver tissues significantly increased in the treatment groups (Figure 8), with no substantial difference between extraction methods. CCl4 also promotes the expression of these proteins.
FIGURE 8. Western blot detection of Nrf-2 signaling pathway and related proteins (A), Relative expression of Nrf-2 (B), HO-1 (C) and NQO-1 (D) protein (
Liver disease carries high morbidity and mortality worldwide. Reactive oxygen species (ROS) play a pivotal role in the occurrence and development of chronic liver disease (Kuriakose et al., 2017). Plants are rich in antioxidant ingredients, which are important in reducing the pathogenesis of oxidative stress due to their free radical scavenging effect (Firuzi et al., 2011). Nevertheless, before plants are used as therapies in modern medical systems, they need to be systematically verified and screened. Triphala has been used as a traditional Ayurvedic medicine for centuries and has been shown to have great potential to promote antioxidant activity (Prasad and Srivastava, 2020). The compound is known to scavenge free radicals, restore antioxidant enzyme and non-enzyme levels, reduces lipid peroxidation, and has good therapeutic prospects for liver diseases. As the main active ingredient of Triphala, tannin is also the main substance of antioxidant activity.
CCl4 is widely used in the preparation of liver injury models and is one of the commonly used chemical drugs to verify the hepatoprotective activity of plant-based drugs. The oxidative damage caused by CCl4 is a good model for screening anti-plant drugs for liver protection activity (Nada et al., 2010). Free radicals (∙CCl3) are the active metabolites of CCl4, mainly related to liver damage caused by CCl4 (Wu et al., 2007), reacting with oxygen to form trichloromethyl peroxide radical (CCl3OO∙), which initiates a chain reaction of lipid peroxidation and attacks and destroys polyunsaturated fatty acids, especially those related to phospholipids (Szymonik-Lesiuk et al., 2003; Ranawat et al., 2010).
This study examined three Triphala extracts for protective activity with DDB as a positive control drug. The results show that the three tested extracts have a protective effect on damaged liver cells, but the most protective was the ultrasonic extraction, which significantly reduced serum ALT and AST and MDA in liver tissues increased SOD and GSH-Px activities. These results show that Triphala can better improve free radical scavenging and reduce cell damage caused by free radicals. In addition, CCl4-induced oxygen free radicals can produce Kupffer cells, which mediate the liver inflammatory response by inducing TNF-α and interleukin (Yu et al., 2014). Current research shows that Triphala can reduce the overexpression of TNF-α and IL-6 in CCl4-induced mouse liver tissues, inhibit inflammation, and provide a hepatoprotective effect.
The Nrf-2 signaling pathway is one of the body’s most important signaling pathways to cope with oxidative stress injury. It can increase the antioxidant level by up-regulating antioxidant proteins in liver cells (Li et al., 2015). Under normal conditions, Nrf-2 is in a state of inhibition. When free radicals attack the body, Nrf-2 enters the nucleus and activates heme oxygenase-1 (HO-1) and phosphoramidite adenine dinucleotide quinone oxidoreductase-1 (NQO-1), further catalyzing heme degradation and eliminating free radicals from the body (Hseu et al., 2012). This study has shown that Triphala can significantly increase both transcript and protein expression of Nrf-2, HO-1, and NQO-1 in damaged liver tissues, regulates the Nrf-2 signaling pathway, and improves the performance of the body’s antioxidant system.
In summary, the three tested Triphala extracts have a hepatoprotective effect, but there were clear differences in efficacy between preparations. The ultrasonic preparation of Triphala was most effective, suggesting that macromolecular substances mediate the protective effect against liver injury, and the loss of macromolecular substances to hydrolysis reduces hepatoprotective potency. During the hydrolysis of Triphala, small molecules such as gallic and ellagic acid increase, but studies have found that gallic acid and ellagic acid exhibit poor absorption, low bioavailability, and easy saturation. When the content is saturated, the increase in content has little effect on the efficacy (Seeram et al., 2004; Ahmed et al., 2018). Structure-activity analysis has shown a large number of phenolic hydroxyl groups in the molecular structure of chebulagic and chebulinic acid, suggesting significant antioxidant activity. Therefore, treatment with botanical medicines rich in hydrolyzed tannin must be prepared in a way that optimizes efficacy. There are various preparation processes, but extraction methods should be controlled to meet the consistency requirements for the biological activities of medications. This study’s most significant finding is that low-temperature extraction is essential to retaining bioactive hydrolyzed tannins and improving Triphala’s hepatoprotective efficacy.
The raw data supporting the conclusions of this article will be made available by the authors, without undue reservation, to any qualified researcher.
The animal study was reviewed and approved by Ethics Committee of the Affiliated Hospital of Chengdu University of TCM.
Revision of the manuscript was done by XW and DZ. XW, CL, FR, and WL performed the experiments. Acquisition and analysis of experimental data were carried out by XW. YH, HH, gave some advice for improving the paper. Study concept and design were the responsibility of JL and LH.
This research was funded by the National Natural Science Foundation of China (81973493), Xinglin Scholar Research Premotion Project of Chengdu University of TCM (CXTD2018006), Sichuan Science and Technology Program (2021YFN0100), Sanajon Pharmaceutical Group-Chengdu University of TCM Joint Laboratory Project (2019-YF04-00086-JH).
SF was employed by Sanajon Pharmaceutical Group.
The remaining authors declare that the research was conducted in the absence of any commercial or financial relationships that could be construed as a potential conflict of interest.
The authors would like to acknowledge JS (Innovative Institute of Chinese Medicine and Pharmacy, Chengdu University of Traditional Chinese Medicine), for the assistance with experimental platform.
The Supplementary Material for this article can be found online at: https://www.frontiersin.org/articles/10.3389/fphar.2021.664607/full#supplementary-material.
Ahmadi-Naji, R., Heidarian, E., and Ghatreh-Samani, K. (2017). Evaluation of the Effects of the Hydroalcoholic Extract of Terminalia Chebula Fruits on Diazinon-Induced Liver Toxicity and Oxidative Stress in Rats. Avicenna J. Phytomed 7 (5), 454–466.
Ahmed, H., Galal, A. F., Shalby, A. B., Abd-Rabou, A. A., and Mehaya, F. M. (2018). Improving Anti-cancer Potentiality and Bioavailability of Gallic Acid by Designing Polymeric Nanocomposite Formulation. Asian Pac. J. Cancer Prev. 19 (11), 3137–3146. doi:10.31557/apjcp.2018.19.11.3137
Avula, B., Wang, Y.-H., Wang, M., Shen, Y.-H., and Khan, I. (2013). Simultaneous Determination and Characterization of Tannins and Triterpene Saponins from the Fruits of Various Species of Terminalia and Phyllantus Emblica Using a UHPLC-UV-MS Method: Application to Triphala. Planta Med. 79 (2), 181–188. doi:10.1055/s-0032-1328089
Baliga, M. S., Meera, S., Mathai, B., Rai, M. P., Pawar, V., and Palatty, P. L. (2012). Scientific Validation of the Ethnomedicinal Properties of the Ayurvedic Drug Triphala: a Review. Chin. J. Integr. Med. 18 (12), 946–954. doi:10.1007/s11655-012-1299-x
Chakraborty, D., and Verma, R. (2010). Ameliorative Effect of Emblica Officinalis Aqueous Extract on Ochratoxin-Induced Lipid Peroxidation in the Kidney and Liver of Mice. Int. J. Occup. Med. Environ. Health. 23 (1), 63–73. doi:10.2478/v10001-010-0009-4
Chaphalkar, R., Apte, K. G., Talekar, Y., Ojha, S. K., and Nandave, M. (2017). Antioxidants ofPhyllanthus emblicaL. Bark Extract Provide Hepatoprotection against Ethanol-Induced Hepatic Damage: A Comparison with Silymarin. Oxidative Med. Cell Longevity. 2017, 1. doi:10.1155/2017/3876040
Chen, Y., Zhou, G., Ma, B., Tong, J., and Wang, Y. (2019). Active Constituent in the Ethyl Acetate Extract Fraction of Terminalia Bellirica Fruit Exhibits Antioxidation, Antifibrosis, and Proapoptosis Capabilities In Vitro. Oxidative Med. Cell Longevity. 2019, 1. doi:10.1155/2019/5176090
Choi, M.-K., Kim, H.-G., Han, J.-M., Lee, J.-S., Lee, J. S., Chung, S. H., et al. (2015). Hepatoprotective Effect ofTerminalia Chebulaagainstt-BHP-Induced Acute Liver Injury in C57/BL6 Mice. Evidence-Based Complement. Altern. Med. 2015, 1. doi:10.1155/2015/517350
Choubey, S., Goyal, S., Varughese, L. R., Kumar, V., Sharma, A. K., and Beniwal, V. (2018). Probing Gallic Acid for its Broad Spectrum Applications. Mini Rev Med Chem. 18 (15), 1283–1293. doi:10.2174/1389557518666180330114010
Deep, G., Dhiman, M., Rao, A. R., and Kale, R. K. (2005). Chemopreventive Potential of Triphala (A Composite Indian Drug) on Benzo(a)pyrene Induced Forestomach Tumorigenesis in Murine Tumor Model System. J. Exp. Clin. Cancer Res. 24 (4), 555–563.
Derosa, G., Maffioli, P., and Sahebkar, A. (2016). Ellagic Acid and its Role in Chronic Diseases. Adv. Exp. Med. Biol. 928, 473–479. doi:10.1007/978-3-319-41334-1_20
Firuzi, O., Miri, R., Tavakkoli, M., and Saso, L. (2011). Antioxidant Therapy: Current Status and Future Prospects. Curr Med Chem. 18 (25), 3871–3888. doi:10.2174/092986711803414368
Fu, B., Zeng, Q., Zhang, Z., Qian, M., Chen, J., Dong, W., et al. (2019). Epicatechin Gallate Protects HBMVECs from Ischemia/Reperfusion Injury through Ameliorating Apoptosis and Autophagy and Promoting Neovascularization. Oxidative Med. Cell Longevity. 2019, 1. doi:10.1155/2019/7824684
Ganeshpurkar, A., Jain, S., and Agarwal, S. (2015). Experimental Studies on Glycolytic Enzyme Inhibitory and Antiglycation Potential of Triphala. Ayu. 36 (1), 96–100. doi:10.4103/0974-8520.169000
Gong, J., Li, L., Lin, Y. x., Xiao, D., Liu, W., Zou, B. r., et al. (2020). Simultaneous Determination of Gallic Acid, Methyl Gallate, and 1,3,6‐tri‐ O ‐galloyl‐ β ‐ D ‐glucose from Turkish Galls in Rat Plasma Using Liquid Chromatography‐tandem Mass Spectrometry and its Application to Pharmacokinetics Study. Biomed. Chromatogr. 34 (10). doi:10.1002/bmc.4916
Gu, X., and Manautou, J. E. (2012). Molecular Mechanisms Underlying Chemical Liver Injury. Expert Rev. Mol. Med. 14, e4. doi:10.1017/s1462399411002110
Gurjar, S., Pal, A., and Kapur, S. (2012). Triphala and its Constituents Ameliorate Visceral Adiposity from a High-Fat Diet in Mice with Diet-Induced Obesity. Altern. Ther. Health Med. 18 (6), 38–45.
Hseu, Y.-C., Chou, C.-W., Senthil Kumar, K. J., Fu, K.-T., Wang, H.-M., Hsu, L.-S., et al. (2012). Ellagic Acid Protects Human Keratinocyte (HaCaT) Cells against UVA-Induced Oxidative Stress and Apoptosis through the Upregulation of the HO-1 and Nrf-2 Antioxidant Genes. Food Chem. Toxicol. 50 (5), 1245–1255. doi:10.1016/j.fct.2012.02.020
Huang, C.-Z., Tung, Y.-T., Hsia, S.-M., Wu, C.-H., and Yen, G.-C. (2017). The Hepatoprotective Effect of Phyllanthus Emblica L. Fruit on High Fat Diet-Induced Non-alcoholic Fatty Liver Disease (NAFLD) in SD Rats. Food Funct. 8 (2), 842–850. doi:10.1039/c6fo01585a
Huang, H.-Z., Zhao, S.-Y., Ke, X.-M., Lin, J.-Z., Huang, S.-S., Xu, R.-C., et al. (2018). Study on the Stability Control Strategy of Triphala Solution Based on the Balance of Physical Stability and Chemical Stabilities. J. Pharm. Biomed. Anal. 158, 247–256. doi:10.1016/j.jpba.2018.06.008
Huang, H. Z., Wei, X. C., Lin, J. Z., Tan, P., Fan, S. H., Zhang, D. K., et al. (2019). Tannin Transformation during the Reflux Process of Phyllanthus Emblica L. And Discussion of the Content Determination Method in Chinese Pharmacopoeia. Chin. Pharm. J. 54 (7), 581–587. doi:CNKI:SUN:ZGYX.0.2019-07-011
Jadon, A., Bhadauria, M., and Shukla, S. (2007). Protective Effect of Terminalia Belerica Roxb. And Gallic Acid against Carbon Tetrachloride Induced Damage in Albino Rats. J. Ethnopharmacology. 109 (2), 214–218. doi:10.1016/j.jep.2006.07.033
Jaiswal, Y., Liang, Z., and Zhao, Z. (2016). Botanical Drugs in Ayurveda and Traditional Chinese Medicine. J. Ethnopharmacology. 194, 245–259. doi:10.1016/j.jep.2016.06.052
Jayesh, K., Helen, L. R., Vysakh, A., Binil, E., and Latha, M. S. (2017). In vivo toxicity Evaluation of Aqueous Acetone Extract of Terminalia Bellirica (Gaertn.) Roxb. Fruit. Regul. Toxicol. Pharmacol. 86, 349–355. doi:10.1016/j.yrtph.2017.04.002
Jayesh, K., Helen, L. R., Vysakh, A., Binil, E., and Latha, M. S. (2019). Protective Role of Terminalia Bellirica (Gaertn.) Roxb Fruits against CCl4 Induced Oxidative Stress and Liver Injury in Rodent Model. Ind. J. Clin. Biochem. 34 (2), 155–163. doi:10.1007/s12291-017-0732-8
Kamali, S. H., Khalaj, A. R., Hasani-Ranjbar, S., Esfehani, M. M., Kamalinejad, M., Soheil, O., et al. (2012). Efficacy of 'Itrifal Saghir', a Combination of Three Medicinal Plants in the Treatment of Obesity; A Randomized Controlled Trial. DARU J. Pharm. Sci. 20 (1), 33. doi:10.1186/2008-2231-20-33
Kuriakose, J., Lal Raisa, H., Vysakh, V., Eldhose, B., and M S, L. (2017). Terminalia Bellirica (Gaertn.) Roxb. Fruit Mitigates CCl4 Induced Oxidative Stress and Hepatotoxicity in Rats. Biomed. Pharmacother. 93, 327–333. doi:10.1016/j.biopha.2017.06.080
Li, Q., Cheng, Y., Bi, M., Lin, H., Chen, Y., Zou, Y., et al. (2015). Effects of N-Butylphthalide on the Activation of Keap1/Nrf-2 Signal Pathway in Rats after Carbon Monoxide Poisoning. Environ. Toxicol. Pharmacol. 40 (1), 22–29. doi:10.1016/j.etap.2015.05.009
Li, Q., Li, H.-J., Xu, T., Du, H., Huan Gang, C.-L., Fan, G., et al. (2018a). Natural Medicines Used in the Traditional Tibetan Medical System for the Treatment of Liver Diseases. Front. Pharmacol. 9, 29. doi:10.3389/fphar.2018.00029
Li, X., Deng, Y., Zheng, Z., Huang, W., Chen, L., Tong, Q., et al. (2018b). Corilagin, a Promising Medicinal Herbal Agent. Biomed. Pharmacother. 99, 43–50. doi:10.1016/j.biopha.2018.01.030
Lu, K., Chakroborty, D., Sarkar, C., Lu, T., Xie, Z., Liu, Z., et al. (2012). Triphala and its Active Constituent Chebulinic Acid Are Natural Inhibitors of Vascular Endothelial Growth Factor-A Mediated Angiogenesis. PLoS One. 7 (8), e43934. doi:10.1371/journal.pone.0043934
Lu, Y., Yan, H., Teng, S., and Yang, X. (2019). A Liquid Chromatography-Tandem Mass Spectrometry Method for Preclinical Pharmacokinetics and Tissue Distribution of Hydrolyzable Tannins Chebulinic Acid and Chebulagic Acid in Rats. Biomed. Chromatogr. 33 (3), e4425. doi:10.1002/bmc.4425
Luo, C., Xu, X., Wei, X., Feng, W., Huang, H., Liu, H., et al. (2019). Natural Medicines for the Treatment of Fatigue: Bioactive Components, Pharmacology, and Mechanisms. Pharmacol. Res. 148, 104409. doi:10.1016/j.phrs.2019.104409
Mahesh, R., Bhuvana, S., and Hazeena Begum, V. M. (2009). Effect ofTerminalia Chebulaaqueous Extract on Oxidative Stress and Antioxidant Status in the Liver and Kidney of Young and Aged Rats. Cell Biochem. Funct. 27 (6), 358–363. doi:10.1002/cbf.1581
M.S., C.-Y., Peng, W.-H., Cheng, H.-Y., Chen, F.-N., Lai, M.-T., and Chiu, T.-H. (2006). Hepatoprotective Effect of Phyllanthus in Taiwan on Acute Liver Damage Induced by Carbon Tetrachloride. Am. J. Chin. Med. 34 (3), 471–482. doi:10.1142/S0192415X06004004
Munawar, T. M., Surya Prakash, D. V., and Vangalapati, M. (2019). Development of Response Surface Methodology for Optimization of Parameters and Quantitative Analysis of Chebulinic Acid from Composition of Medicinal Herbs by HPLC. Saudi J. Biol. Sci. 26 (7), 1809–1814. doi:10.1016/j.sjbs.2018.02.013
Nada, S. A., Omara, E. A., Abdel-Salam, O. M. E., and Zahran, H. G. (2010). Mushroom Insoluble Polysaccharides Prevent Carbon Tetrachloride-Induced Hepatotoxicity in Rat. Food Chem. Toxicol. 48 (11), 3184–3188. doi:10.1016/j.fct.2010.08.019
Nakano, E., Kamei, D., Murase, R., Taki, I., Karasawa, K., Fukuhara, K., et al. (2019). Anti-inflammatory Effects of New Catechin Derivatives in a Hapten-Induced Mouse Contact Dermatitis Model. Eur. J. Pharmacol. 845, 40–47. doi:10.1016/j.ejphar.2018.12.036
Nariya, M. B., Shukla, V. J., Ravishankar, B., and Jain, S. M. (2011). Comparison of Gastroprotective Effects of Triphala Formulations on Stress-Induced Ulcer in Rats. Indian J. Pharm. Sci. 73 (6), 682–687. doi:10.4103/0250-474x.100252
Nigam, M., Mishra, A. P., Adhikari‐Devkota, A., Dirar, A. I., Hassan, M. M., Adhikari, A., et al. (2020). Fruits of Terminalia Chebula Retz.: A Review on Traditional Uses, Bioactive Chemical Constituents and Pharmacological Activities. Phytotherapy Res. 34, 2518. doi:10.1002/ptr.6702
Olennikov, D., Kashchenko, N., and Chirikova, N. (2015). In Vitro Bioaccessibility, Human Gut Microbiota Metabolites and Hepatoprotective Potential of Chebulic Ellagitannins: A Case of Padma Hepaten Formulation. Nutrients. 7 (10), 8456–8477. doi:10.3390/nu7105406
Panchabhai, T. S., Ambarkhane, S. V., Joshi, A. S., Samant, B. D., and Rege, N. N. (2008). Protective Effect ofTinospora Cordifolia, Phyllanthus Emblica and Their Combination against Antitubercular Drugs Induced Hepatic Damage: an Experimental Study. Phytother. Res. 22 (5), 646–650. doi:10.1002/ptr.2356
Pawar, V., Lahorkar, P., and Anantha Narayana, D. (2009). Development of a RP-HPLC Method for Analysis ofTriphala Curnaand its Applicability to Test Variations inTriphala Curnapreparations. Indian J. Pharm. Sci. 71 (4), 382–386. doi:10.4103/0250-474x.57286
Peterson, C. T., Denniston, K., and Chopra, D. (2017). Therapeutic Uses of Triphala in Ayurvedic Medicine. J. Altern. Complement. Med. 23 (8), 607–614. doi:10.1089/acm.2017.0083
Phetkate, P., Kummalue, T., Rinthong, P.-o., Kietinun, S., and Sriyakul, K. (2020). Study of the Safety of Oral Triphala Aqueous Extract on Healthy Volunteers. J. Integr. Med. 18 (1), 35–40. doi:10.1016/j.joim.2019.10.002
Prasad, S., and Srivastava, S. K. (2020). Oxidative Stress and Cancer: Chemopreventive and Therapeutic Role of Triphala. Antioxidants. 9 (1), 72. doi:10.3390/antiox9010072
Ranawat, L., Bhatt, J., and Patel, J. (2010). Hepatoprotective Activity of Ethanolic Extracts of Bark of Zanthoxylum Armatum DC in CCl4 Induced Hepatic Damage in Rats. J. Ethnopharmacology. 127 (3), 777–780. doi:10.1016/j.jep.2009.10.019
Rasool, M., and Sabina, E. P. (2007). Antiinflammatory Effect of the Indian Ayurvedic Herbal Formulation Triphala on Adjuvant-Induced Arthritis in Mice. Phytother. Res. 21 (9), 889–894. doi:10.1002/ptr.2183
Rayudu, V., and Raju, A. B. (2014). Effect of Triphala on Dextran Sulphate Sodium-Induced Colitis in Rats. Ayu 35 (3), 333–338. doi:10.4103/0974-8520.153787
Russell, L. H., Mazzio, E., Badisa, R. B., Zhu, Z. P., Agharahimi, M., Millington, D. J., et al. (2011). Differential Cytotoxicity of Triphala and its Phenolic Constituent Gallic Acid on Human Prostate Cancer LNCap and Normal Cells. Anticancer Res. 31 (11), 3739–3745.
Saravanan, S., Srikumar, R., Manikandan, S., Jeya Parthasarathy, N., and Sheela Devi, R. (2007). Hypolipidemic Effect of Triphala in Experimentally Induced Hypercholesteremic Rats. Yakugaku Zasshi. 127 (2), 385–388. doi:10.1248/yakushi.127.385
Sarkar, R., Hazra, B., and Mandal, N. (2015). Amelioration of Iron Overload-Induced Liver Toxicity by a Potent Antioxidant and Iron chelator,Emblica officinalisGaertn. Toxicol. Ind. Health. 31 (7), 656–669. doi:10.1177/0748233713483195
Sarkar, R., Hazra, B., and Mandal, N. (2012). Reducing Power and Iron Chelating Property of Terminalia Chebula (Retz.) Alleviates Iron Induced Liver Toxicity in Mice. BMC Complement. Altern. Med. 12, 144. doi:10.1186/1472-6882-12-144
Sayed, S., Ahsan, N., Kato, M., Ohgami, N., Rashid, A., and Akhand, A. A. (2015). Protective Effects of phyllanthus Emblica Leaf Extract on Sodium Arsenite-Mediated Adverse Effects in Mice. Nagoya J. Med. Sci. 77 (1-2), 145–153.
Seeram, N. P., Lee, R., and Heber, D. (2004). Bioavailability of Ellagic Acid in Human Plasma after Consumption of Ellagitannins from Pomegranate (Punica Granatum L.) Juice. Clinica Chim. Acta. 348 (1-2), 63–68. doi:10.1016/j.cccn.2004.04.029
Sharma, A., and Sharma, K. K. (2011). Chemoprotective Role of Triphala against 1,2-dimethylhydrazine Dihydrochloride Induced Carcinogenic Damage to Mouse Liver. Ind. J. Clin. Biochem. 26 (3), 290–295. doi:10.1007/s12291-011-0138-y
Singh, D. P., and Mani, D. (2015). Protective Effect of Triphala Rasayana against Paracetamol-Induced Hepato-Renal Toxicity in Mice. J. Ayurveda Integr. Med. 6 (3), 181–186. doi:10.4103/0975-9476.146553
Singh, D., Yadav, E., Falls, N., Kumar, V., Singh, M., and Verma, A. (2019). Phytofabricated Silver Nanoparticles of Phyllanthus Emblica Attenuated Diethylnitrosamine-Induced Hepatic Cancer via Knock-Down Oxidative Stress and Inflammation. Inflammopharmacol. 27 (5), 1037–1054. doi:10.1007/s10787-018-0525-6
Sobeh, M., Mahmoud, M. F., Hasan, R. A., Abdelfattah, M. A. O., Osman, S., Rashid, H.-O., et al. (2019). Chemical Composition, Antioxidant and Hepatoprotective Activities of Methanol Extracts from Leaves ofTerminalia belliricaandTerminalia sericea(Combretaceae). PeerJ 7, e6322. doi:10.7717/peerj.6322
Souza, C. F., Baldissera, M. D., Descovi, S. N., Zeppenfeld, C. C., Garzon, L. R., da Silva, A. S., et al. (2018). Serum and Hepatic Oxidative Damage Induced by a Diet Contaminated with Fungal Mycotoxin in Freshwater Silver Catfish Rhamdia quelen: Involvement on Disease Pathogenesis. Microb. Pathogenesis. 124, 82–88. doi:10.1016/j.micpath.2018.08.041
Srikumar, R., Parthasarathy, N. J., Manikandan, S., Narayanan, G. S., and Sheeladevi, R. (2006). Effect of Triphala on Oxidative Stress and on Cell-Mediated Immune Response against Noise Stress in Rats. Mol. Cel Biochem. 283 (1-2), 67–74. doi:10.1007/s11010-006-2271-0
Szymonik-Lesiuk, S., Czechowska, G., Stryjecka-Zimmer, M., SLomka, M., MAldro, A., CeliNski, K., et al. (2003). Catalase, Superoxide Dismutase, and Glutathione Peroxidase Activities in Various Rat Tissues after Carbon Tetrachloride Intoxication. J. Hepato-Biliary-Pancreatic Surg. 10 (4), 309–315. doi:10.1007/s00534-002-0824-5
Tatiya, A., Sutar, M., Surana, S., and Gamit, N. (2012). Hepatoprotective Effect of Poly Herbal Formulation against Various Hepatotoxic Agents in Rats. Phcog Res. 4 (1), 50–56. doi:10.4103/0974-8490.91040
Wang, M., Li, Y., and Hu, X. (2018). Chebulinic Acid Derived from Triphala Is a Promising Antitumour Agent in Human Colorectal Carcinoma Cell Lines. BMC Complement. Altern. Med. 18 (1), 342. doi:10.1186/s12906-018-2412-5
Wu, Y., Yang, L., Wang, F., Wu, X., Zhou, C., Shi, S., et al. (2007). Hepatoprotective and Antioxidative Effects of Total Phenolics from Laggera Pterodonta on Chemical-Induced Injury in Primary Cultured Neonatal Rat Hepatocytes. Food Chem. Toxicol. 45 (8), 1349–1355. doi:10.1016/j.fct.2007.01.011
Yoo, H.-J., Hong, C.-O., Ha, S. K., and Lee, K.-W. (2020). Chebulic Acid Prevents Methylglyoxal-Induced Mitochondrial Dysfunction in INS-1 Pancreatic β-Cells. Antioxidants. 9 (9), 771. doi:10.3390/antiox9090771
Yu, H., Zheng, L., Yin, L., Xu, L., Qi, Y., Han, X., et al. (2014). Protective Effects of the Total Saponins from Dioscorea Nipponica Makino against Carbon Tetrachloride-Induced Liver Injury in Mice through Suppression of Apoptosis and Inflammation. Int. Immunopharmacology. 19 (2), 233–244. doi:10.1016/j.intimp.2014.01.019
Keywords: Triphala, extraction process, CCl4-induced acute liver injury, Nrf-2 signaling pathway, hepatoprotective effects, bioactivity consistency
Citation: Wei X, Luo C, He Y, Huang H, Ran F, Liao W, Tan P, Fan S, Cheng Y, Zhang D, Lin J and Han L (2021) Hepatoprotective Effects of Different Extracts From Triphala Against CCl4-Induced Acute Liver Injury in Mice. Front. Pharmacol. 12:664607. doi: 10.3389/fphar.2021.664607
Received: 05 February 2021; Accepted: 06 April 2021;
Published: 05 July 2021.
Edited by:
Juei-Tang Cheng, Chang Jung Christian University, TaiwanReviewed by:
Guang-Bo Ge, Shanghai University of Traditional Chinese Medicine, ChinaCopyright © 2021 Wei, Luo, He, Huang, Ran, Liao, Tan, Fan, Cheng, Zhang, Lin and Han. This is an open-access article distributed under the terms of the Creative Commons Attribution License (CC BY). The use, distribution or reproduction in other forums is permitted, provided the original author(s) and the copyright owner(s) are credited and that the original publication in this journal is cited, in accordance with accepted academic practice. No use, distribution or reproduction is permitted which does not comply with these terms.
*Correspondence: Dingkun Zhang, NDY1NzkwNjQzQHFxLmNvbQ==; Junzhi Lin, NTgyMDk3MDEzQHFxLmNvbQ==; Li Han, aGFubGl5eEAxNjMuY29t
Disclaimer: All claims expressed in this article are solely those of the authors and do not necessarily represent those of their affiliated organizations, or those of the publisher, the editors and the reviewers. Any product that may be evaluated in this article or claim that may be made by its manufacturer is not guaranteed or endorsed by the publisher.
Research integrity at Frontiers
Learn more about the work of our research integrity team to safeguard the quality of each article we publish.