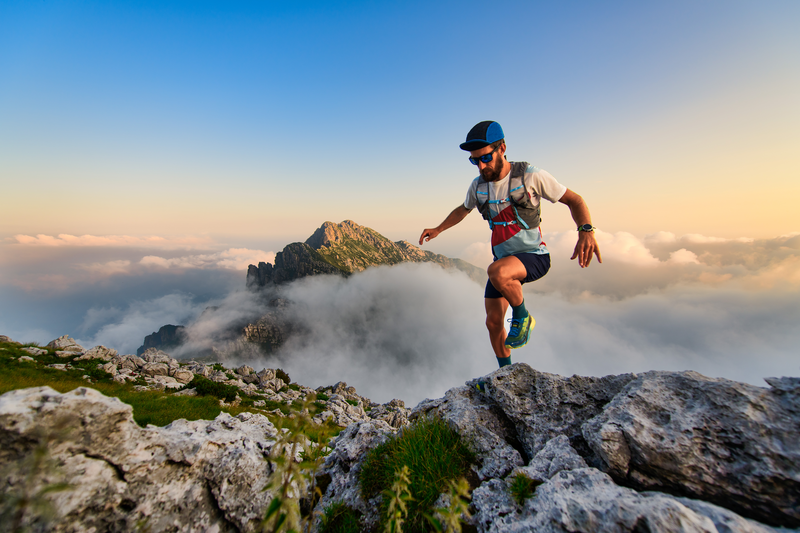
95% of researchers rate our articles as excellent or good
Learn more about the work of our research integrity team to safeguard the quality of each article we publish.
Find out more
ORIGINAL RESEARCH article
Front. Pharmacol. , 26 April 2021
Sec. Ethnopharmacology
Volume 12 - 2021 | https://doi.org/10.3389/fphar.2021.659954
Panax ginseng C. A. Mey (PGCAM) is a herbaceous perennial belonging to the Araliaceae family, mainly including Mountain-Cultivated Ginseng (MCG) and Garden Ginseng (GG) on the market. We aimed to establish a rapid, accurate and effective method to distinguish 15-year-old MCG and GG using ultra-performance liquid chromatography-quadrupole time-of-flight-tandem mass spectrometry (UPLC-QTOF-MS/MS), and also explored the pharmacological mechanisms of the main components using the Integrative Pharmacology-based Network Computational Research Platform of Traditional Chinese Medicine (TCMIP V2.0; http://www.tcmip.cn/). Altogether, 23 potential quality markers were characterized to distinguish 15-year-old MCG and GG, including ginsenosides Ra2, Rg1, and Ra1, and malonyl-ginsenoside Ra3, etc. The contents of 19 constituents (mainly protopanaxadiol-type) were higher in MCG compared with that in GG, and four constituents (mainly carbohydrate compounds) were higher in GG. The 105 putative targets corresponding to 23 potential quality markers were mainly involved in 30 pathways, which could be divided into 10 models, such as immune regulation, systems (metabolic, nervous, cardiovascular, reproductive), blood-pressure regulation, as well as antitumor, antiaging, antibacterial and anti-inflammatory effects. Furthermore, the potential quality markers of MCG and GG could inhibit the proliferation of breast cancer by regulating the mRNA expression of PSA, S6K, MDM2, and P53 genes by acting on AR, MTOR, PI3K and other targets. The Integrative Pharmacology Strategy may provide an efficient way to identify chemical constituents and explore the pharmacological actions of TCM formulations.
Panax ginseng C. A. Mey (PGCAM) is an herbaceous perennial belonging to the Araliaceae family. Its dried root and rhizome (Panax ginseng C. A. Mey. Radix et Rhizoma) have been used as a herbal medicine in China and some Asian countries for thousands of years (Mancuso and Santangelo, 2017), and have immunomodulation, antifatigue, antiaging, and anticancer effects (Shi et al., 2019). PGCAM was firstly recorded in Shennong’s Classic of Materia Medica (Liu et al., 2013), during which all PGCAM grew wild. However, with the development of society, wild-growing PGCAM could not meet human requirements. To solve the problem, farmers began to study and explore suitable cultivation methods for PGCAM. There are four types of PGCAM according to different cultivation methods: wild-growing ginseng, transplanted ginseng, mountain-cultivated ginseng (MCG) and garden ginseng (GG) (Yang et al., 2013). MCG and GG are recorded in the Pharmacopoeia of the People’s Republic of China from 2005 edition. MCG and GG have become the main varieties on the market.
MCG refers to the seeds of PGCAM germinated and grown in tall mountains and dense forests for 10–20 years (Zhu et al., 2018), so also called “Lin-Xia-Shan-Shen”. If MCG grows long enough, its quality and efficacy become almost the same as that of wild PGCAM. GG refers to PGCAM that planted in a garden and harvested after 4–6 years. In general, the quality of MCG is higher than that of GG, which has a stronger pharmacological effect (Kim et al., 2020). The price of PGCAM is directly proportional to the grown period: the longer the period, the higher the price. Therefore, adulteration or falsification of PGCAM has always been a serious problem in the commercial market.
To solve this problem, researchers have proposed different identification strategies for PGCAM. With the continuous development of new technologies, ultra-performance liquid chromatography-quadrupole time-of-flight-tandem mass spectrometry (UPLC-QTOF-MS/MS) has been widely used in the analysis of chemical components of PGCAM (Wu et al., 2018), multiple compounds have been identified, including ginsenosides, polysaccharides, fatty acids, volatile oils and amino acids (Liang et al., 2016). Ginsenosides, as the main components of PGCAM, show important pharmacological activities during the treatment of cardiovascular diseases (Kim, 2018). In addition, Prof. Xu and his colleague selected 12 chemical components to distinguish GG4–7 years and MCG15 years: ginsenoside Ra3/isomer, gypenoside XVII, quinquenoside R1, ginsenoside Ra7, notoginsenoside Fe, ginsenoside Ra2, ginsenoside Rs6/Rs7, malonyl ginsenoside Rc, malonyl ginsenoside Rb1, malonyl ginsenoside Rb2, palmitoleic acid, and ethyl linoleate (Xu et al., 2016). However, the characteristic components and pharmacological effects of MCG and GG grown for 15 years have not been reported. Therefore, we aimed to employ the Integrative Pharmacology Strategy to characterize the differential component between the two types of Ginseng and explore their pharmacological effects in vitro. Integrative Pharmacology Strategy (IPS) pays attention to the interactions between Chinese prescriptions and the organism from multiple levels and multiple aspects, its research content mainly includes component analysis, network pharmacology analysis and pharmacological experiment verification (Xu and Yang, 2014). The IPS systematically analyzes the interaction between TCM formulations and the organism from multiple levels and multiple aspects to form a new mode of research of TCM. To practice this strategy better, we established Integrative Pharmacology-based Network Computational Research Platform of Traditional Chinese Medicine (TCMIP V2.0; www.tcmip.cn/), which comprising five databases and seven functional modules (Xu et al., 2019).
Therefore, we aimed to identify potential quality markers to distinguish 15-year-old MCG and GG using the UPLC-QTOF-MS/MS. Then, we employed the TCMIP V2.0 to carry out the network pharmacology analysis of the differential components contained in MCG and GG. Finally, we verified the results of network pharmacology in vitro. Our research will be a good application of Integrative Pharmacology Strategy.
Twelve batches of MCG and GG were collected from the cultivation areas in Huanren County (41.26°N, 125.36°E; Benxi City, Liaoning Province, China) in September 2017, and the voucher specimens were deposited in our lab. Sixty-six standards of PGCAM were purchased from Shanghai Standard Biotech (Shanghai, China) or isolated from the roots of PGCAM and Panax notoginseng C. A. Mey (Yang et al., 2016b). (Supplementary Table S1). HPLC-grade acetonitrile (CH3CN) and methanol were purchased from Thermo Fisher Scientific (Fair Lawn, NJ, United States). Formic acid (FA) was obtained from Sigma-Aldrich (Saint Louis, MO, United States). Ultra-pure water was prepared in-house using the Milli-Q™ system (Millipore, Bedford. MA, United States).
First, 50 mg of MCG powder and GG powder were soaked with 3 ml of 70% methanol (v/v), respectively, followed by ultrasound extraction for 60 min at 25°C. Then, the solution was centrifugated at 14,000 rpm for 10 min at room temperature after compensating with 70% methanol for the weight lost. The supernatant was transferred to a 5 ml volumetric flask and diluted to the scale mark. Finally, 1 mlwell-mixed liquid was centrifuged for 10 min, and supernatant were transferred to autosampler vials for analyses. Herbal samples were injected randomly. An equal volume of all test solutions was mixed to prepare the quality control (QC) sample, which was used to monitor the stability of the analytical system.
UPLC-MS was performed on an Acquity™ UPLC I-Class/Vion Ion Mobility Spectrometry (IMS)-QTOF system (Waters, Milford, MA, United States). The chromatographic separation was carried out on a BEH Shield RP18 column (2.1 × 100 mm, 1.7 µm) hyphenated with a VanGuard™ pre-column (2.1 × 50 mm, 1.7 µm; Waters) maintained at 35°C. The mobile phase consisted of 0.1% FA in CH3CN (A) and 0.1% FA in H2O (B). The optimized gradient program was: 0–2 min, 15–20% A; 2–7 min, 20–30% A; 7–17 min, 30–33% A; 17–20 min, 33–60% A; 20–22 min, 60–98% A; 22–24 min, 98–98% A. The flow rate was 0.3 ml/min and the injection volume was 3 μL. A 3-min re-equilibration time was set between successive injections. A “purge–wash–purge” cycle was set on the autosampler, with 10% CH3CN-H2O (v/v) as the purge solvent and 50% CH3CN-H2O as the wash solvent, to minimize the carry-over between injections.
The MS experiment performed on a Vion IMS-QTOF mass spectrometer in the negative electrospray ionization mode (Waters, Corp., Milford, United States). The LockSpray™ ion source was equipped under the following parameters: capillary voltage, 1.0 kV; cone voltage, 20 V; source offset, 80 V; source temperature, 120°C; desolvation gas temperature, 500°C; desolvation gas flow (N2), 800 L/h; cone gas flow (N2), 50 L/h. Default parameters were defined for the traveling-wave IMS separation. HDMSE data covered a m/z of 300–1,500 at 0.3 s per scan. The low collision energy was set at 6 eV and the high-energy ramp was 40–80 eV. Data acquisition was controlled by the UNIFI 1.9.3.0 software (Waters, Corp., Milford, United States). The accuracy error threshold was fixed at 10 ppm.
Raw HDMSE data were corrected with reference to m/z 554.2620 in the 12 batches of samples and QC by UNIFI 1.9.3.0. Then, preliminary data were processed automatically by Progenesis QI 2.1 software (Waters, Corp., Milford, CT, United States) [M-H]− and [M + FA-H]− were the main adduct ions in the negative mode. Efficient menu-guided processing, peak alignment and peak selection could generate a data matrix, including retention time (tR), m/z, normalized peak area, and collisioncross-secions (CCS). All the ion signals detected in each sample were normalized to the obtained value of the total ion count. The data matrix was filtered based on “80% rule” and “30% variation” (Li J. et al., 2019).
Processed data were subjected to principal component analysis (PCA) and orthogonal projections to latent structures discriminant analysis (OPLS-DA) using SIMCA-P 14.1 (Umetrics, Umea, Sweden). Potential quality markers were filtered out according to the variable importance for projection (VIP) values (VIP>1.5) and Student’s t-test (p < 0.05), which could be used distinguish between MCG and GG.
The mol. formats of potential quality markers were uploaded to TCMIP to predict the putative targets using the TCM target prediction and function analysis module (TTFM) of TCMIP according to the similarity of chemical structures to known drugs on the market. The Tanimoto Score was set at 0.7 (moderate similarity) to select constitute–putative target pairs.
To further explore the biological functions of the putative targets, the pathway–enrichment analyses was undertaken using the database for Annotation, Visualization, and Integrated Discovery (DAVID) v6.8 (https://david.ncifcrf.gov). Pathways with p < 0.05 were selected for further analyses.
MCF-7 cell line was kindly provided by Prof. Xiujie Wang (Institute of Genetics and Developmental Biology, Chinese Academy of Sciences). The MCF-7 cell line was cultured in DMEM high glucose medium (Thermo, Fair Lawn, NJ, United States) with 1% penicillin/streptomycin (Invitrogen, Carlsbad, CA, United States)and 10% fetal bovine serum (FBS; Sijiqing, Huzhou, Zhejiang, China) at 37°C in humid atmosphere with 5% CO2.
The extraction of MCG and GG was prepared according to Sample Preparation. Then, the methanol was volatilized and the residue was redissolved with sterile water. After filtered by 0.22 μm filter film, the herbal samples were stored in a refrigerator at 4°C. MCF-7 cell line was seeded in 96-well plates (5×103 cells/well) incubating at 37°C for 24 h. Various concentrations of MCG or GG were added to the wells and then incubated for 48 h. Cell proliferation was evaluated using a Cell Counting Kit-8 (CCK8) assay. The cells treated with various conditions were incubated with 10 μL CCK-8 solution (Dojindo, Kumamoto, Japan) for 90 min. Next the absorbance was measured by a Microplate Reader under 450 nm (Thermo, Fair Lawn, NJ, United States). All experiments were performed in triplicate and repeated four times. Data were presented as mean ± standard deviation (SD). A One-way ANOVA determined whether the results had statistical significance.
The total RNA was isolated from the cultured cells using Trizol (Tiangen, China). Then, cDNA was synthesized using a ReverTra Ace quantitative polymerase chain reaction (PCR) RT Master Mix Kit with gDNA Remover (TOYOBO Co., OSAKA, Japan) according to the manufacturer’s protocol. PCR product amplification was performed with SYBR Green Master Mix (Juhemei, China) on an Agilent Mx 3000P Real-Time PCR System (Applied Biosystems, CA). Primers were 5′-GAATCATCGGACTC AGGTACATC-3′ and 5′-TCTGTCTCACTAATTGCTCTCCT-3′ for MDM2; 5′-CAGCACATGACGGAGGTTGT-3′ and 5′-TCATCCAAATACTCCACACGC-3′ for P53; 5′-GTGAGGCAGGCGACTAATCAG-3′ and 5′-GTTCCCGTACC TGTTTGCAG-3′ for PSA; 5′-TTTGAGCTACTTCGGGTACTTGG-3′ and 5′-CGATGAAGGGATGCTTTACTTCC-3′ for S6K; 5′-CTGGAACGGTGAAGGT GACA-3′ and 5′-AAGGGACTTCCTGTAACAATGCA-3′ for BETA-ACTIN, and 40 PCR cycles (30 s denaturation at 95°C, 20 s annealing at 60°C, 30 s elongation at 72°C) were run. BETA-ACTIN was used as an internal standard. The relative mRNA levels were determined using the 2−ΔΔCt method.
All data were analyzed by SPSS 22.0 software (SPSS Inc., Chicago,IL, United States). Figures in this research were drawn with GraphPad Prism 8.0 software (GraphPad Prism, San Diego, CA, United States). The results were shown as mean ± standard deviation (SD). Significant differences between normally distributed gene expression data were determined by one-way analysis of variance (ANOVA). The significant difference was set at p < 0.05.
Multi-batch HDMSE data were processed by Progenesis QI, and generated a list comprising 1,023 ions (Supplementary Table S2). These ions were filtered based on “80% rule” and “30% variation” (Supplementary Table S3). Then, the remained 954 ions were subjected to PCA and OPLS-DA. MCG and GG groups were separated well in PCA score Plots (Figure1A), indicating the great difference in chemical profiles. To obtain better discrimination, they were subjected to OPLS-DA with R2X at 0.865 and Q2 at 0.897, especially in the component P1+3 direction (Figure 1B). The validity and predictability of OPLS-DA model was evaluated by 200-time permutation test (Figure 1C). The regression lines of R2 and Q2 decreased with decreased in the correlation coefficients between permuted and original response variables. The result indicated that the predictive OPLS-DA models did not overfit. S-plots were obtained to show responsibility of each ion for these variations more showed intuitively the contribution of differential ions on distinguishing between MCG and GG. Most of the ions clustered around the original point (Figure 1D), and only a few of them were scattered in the margin region. Just these few ions that representing chemical constituents contributed to the separation observed in the score plots. The ions that far away from the original point, always have higher value of VIP (Supplementary Figure S1). Therefore, 42 ions with VIP >1.5 and p < 0.05 (in the Student’s t-test) were selected as potential quality markers (Supplementary Table S4), 31 (73.8%) of which were higher in MG, and 11 (26.2%) were higher in GG. Nine representative compounds were illustrated in Figures 2A–I.
FIGURE 1. The untargeted metabolomics analysis based on multivariate statistical analysis of MCG and GG. (A): score plot of PCA; (B) score plot of OPLS-DA; (C) validation plots obtained from 200 permutation tests for the OPLS-DA models; (D) score plot of S-Plot.
FIGURE 2. Box charts illustrating the distribution difference of the nine marker compounds among MCG and GG. (A–F) can be the characteristic components of MCG. (G–I) can be the characteristic components of GG.
Sixty-six standard compounds and our house library were helped to identify the 23 potential quality markers. The base peak intensity chromatograms of MCG and GG corresponding to negative ion mode were shown in Figure 3. The 23 identified components with numbers on the peaks were classified as protopanaxadiol (PPD)-type sapogenins (M1, M3, M5, M6, M8, M11, M13, M17, M19, M20, M23, M24, M32, M33, M34, M42), Protopanaxatriol (PPT)-type sapogenins (M9, M12, M27, M28, M31) and carbohydrate compounds (M7, M15). The detailed information of the 23 components was listed in Table 1.
FIGURE 3. UHPLC/IM-QTOF-HDMSE of MCG and GG in the negative ion modes. MCG: the root and rhizome of Mountain-Cultivated Panax ginseng C. A. Mey; GG: the root and rhizome of Garden Panax ginseng C. A. Mey. (CG).
Characteristic MS/MS features of the ginsenoside Ra2 were observed in the MS2 spectrum, including m/z 1209.6271 ([M-H]−), 1077.5858 ([M-H-Xyl]−), 945.5432 ([M-H-Xyl-Ara]−), 783.4898 ([M-H-Xyl-Ara-Glc]−), 621.4375 ([M-H-Xyl-Ara-2Glc]−), and 459.3874 ([PPD-H]−) (Figure 4). M1 (ginsenoside Ra2, tR, 11.25 min, chemical formula, C58H98O26), M6 (notoginsenoside K, tR, 18.07 min, chemical formula, C48H82O18), M24 (ginsenoside Ra3, tR, 11.61 min, chemical formula, C59H100O27), M33 (ginsenoside Ra1, tR, 13.12 min, chemical formula, C58H98O26), M34 (malonyl-floralginsenoside Rd5, tR, 18.82 min, chemical formula, C51H84O21) were identified as PPD-type sapogenins compared with the reference compounds (Supplementary Table S1). Taking ginsenoside Ra2 as an example, the fragmentation pattern of panaxadiol saponins was analyzed.
Taking ginsenoside Rg1 as an example, the fragmentation pattern of panaxatriol saponins was analyzed. Characteristic MS/MS features of ginsenoside Rg1 were observed in the MS2 spectrum, including m/z 799.4859 ([M-H]−), 637.4334 ([M-H-Glc]−), 475.3788 ([M-H-2Glc]−), and 391.2841 ([M-H-2Glc-C6H12]−) (Figure 4). M9 (ginsenoside Rg1, tR, 9.54 min, chemical formula, C42H72O14), M12 (notoginsenoside S, tR, 9.62 min, chemical formula, C63H106O30), M27 (notoginsenoside Rt, tR, 6.90 min, chemical formula, C44H74O15), M31 (malonyl-floralginsenoside Re1, tR, 6.81 min, chemical formula, C51H84O21) were identified as PPT-type sapogenins compared with the reference compounds (Supplementary Table S1).
A total of 105 putative targets were predicated based on 23 primarily identified compounds (Tanimoto score ≥0.7) using TTFM of TCMIP v2.0 (Supplementary Table S5). Which were mainly involved in 30 pathways after functional analysis DAVID 6.8 (Supplementary Table S6). The network of interactions between 23 components, the corresponding 105 putative targets and 30 pathways was visualized using Cytoscape v3.7.1 (Boston, MA, United States) as show in Figure 5. The 30 pathways were related to 10 function modules, such as metabolic system, nervous system, cardiovascular system, immune-regulation system and reproductive system. Among them, there were 12 immune pathways (fc epsilon RI signaling pathway; leukocyte transendothelial migration; non-small cell lung cancer; pathways in cancer; acute myeloid leukemia; toll-like receptor signaling pathway; small cell lung cancer; apoptosis; prostate cancer; natural killer cell mediated cytotoxicity; glioma; mammalian target of rapamycin signaling pathway (mTOR) signaling pathway), nine metabolic pathways (starch and sucrose metabolism; oxidative phosphorylation; type II diabetes mellitus (DM); insulin signaling pathway; galactose metabolism; tricarboxylic acid cycle (TCA cycle); glycolysis/gluconeogenesis; fructose and mannose metabolism; amino sugar and nucleotide sugar metabolism), four nervous system pathways (Alzheimer’s disease; Parkinson’s disease; Huntington’s disease; erbB signaling pathway), there cardiovascular system pathways (cardiac muscle contraction; contraction of vascular smooth muscle; aldosterone-regulated sodium reabsorption) and two reproductive-system pathways (progesterone-mediated oocyte maturation; Oocyte meiosis).
FIGURE 5. Illustration of the relevance among marker compounds for distinguishing MCG and GG, their target, pathways and therapeutic effects. Blue nodes represent characteristic constituents of MCG. Green nodes represent characteristic constituents of GG. Orange nodes refer to the targets; Purple nodes represent the pathways; Yellow nodes refer to therapeutic effects. Red nodes represent the effect of ginseng.
The enrichment analysis above showed that 23 components played more importment roles in tumor pathway, which indicated that the anticancer effect of MCG was stronger than GG. Therefore, we selected MCF-7 cell line to verify the results. The CCK-8 assay showed that MCG or GG did not significantly inhibit the proliferation of MCF-7 cell line when the concentration was lower than 4 mg/ml. When the concentration of MCG or GG was 8 mg/ml, the survival rate of MCG group was only 11.8%, and GG group was 21.99%, which indicated that the concentration of 4–8 mg/ml was the range of MCG and GG to inhibit the proliferation of MCF-7 cell line (Supplementary Figure S2). Thus, the concentrations of 4, 5, 6, 7, and 8 mg/ml were set to explore the inhibitory effect of MCG and GG on MCF-7 cell line. When the extract concentration was 4 mg/ml, the survival rate of MCF-7 cell line was significantly inhibited by MCG compared with GG (Figure 6A).
FIGURE 6. In vitro pharmacodynamics experiments and RT-PCR detection of key effector genes in MCG and GG. (A): Results of in vitro pharmacodynamics experiments of MCG and GG. (B–F): Results of RT-PCR detection of key effector genes in MCG and GG. “*”: p < 0.05; “**”: p < 0.01; “***”: p < 0.001; “****”: p < 0.0001. (F):Mechanism of anti-breast cancer effect of quality markers of MCG.
Therefore, MG and GG at 4 mg/ml were selected to subject to RT-PCR detection. The mRNA expression of PSA, MDM2 and S6K genes in the group of MCG was significantly down-regulated compared with that of control group, and the degree of down regulation was stronger than that of GG. Conversely, the mRNA expression of P53 gene was significantly up-regulated, and the degree stronger than that of GG (Figures 6B–E).
PGCAM as a herbal nutritional supplement is well known for its extensive pharmacological effects (Nguyen and Nguyen, 2019). MCG and GG are the main PGCAM varieties on the market to meet the huge demand of people (Yang et al., 2013) (Shen et al., 2019). At present, ginsenoside is the main marker for identification of different species of MCG and GG. For example, ginsenosides can be used to distinguish different planting methods and different years of ginseng (Cui et al., 2013; Ruan et al., 2018). As natural plants, the environment and duration of growth affect the quality and price of PGCAM directly. (Xu et al., 2016; Zhu et al., 2018). Many studies have been undertaken to characterize the chemical profiles of PGCAM cultivated in different environments for various durations, which could help distinguish diverse samples (Chang et al., 2016; Chang et al., 2017). Hence, we aimed to employ integrative pharmacology strategy to characterize the differential components between the two types of Ginseng and explore their pharmacological effects by network pharmacology and verified in vitro.
Altogether, 23 chemical components were identified to distinguish the two sets of samples, and nine of them were identified according to use of reference compounds. The characteristic fragments of PPD-saponins were m/z 459.38 and m/z 375.29. These data could be used to identify unknown marker compounds belonging to this type, including M14, M16, M18, M26, M29, M30, M36, M39, and M41. Ginsenosides were the main characteristic components of the MCG group. Carbohydrates were the main characteristic components of the GG group. The peak area of PPD-type saponins in the MCG group was higher than that of GG group, and the peak area of carbohydrate compounds was higher in the GG group. The results showed that ginsenoside Ra2 and sucrose could not only distinguish 15 years old MCG and GG, but also 10–20 years old MCG and 4–6 years old GG (Xu et al., 2016). Ginsenoside Rg1 can not only distinguish the 15-year-old forest ginseng and garden ginseng, but also distinguish the ginseng of different ages, different planting sites and different slope directions (Zhu et al., 2021). In conclusion, some of the quality markers in the same region can distinguish ginseng under different conditions. It shows that our research results have certain universality.
To explore further the pharmacological activities of these marker components, components–targets–pathways network was constructed. We found that the marker components could regulate immune, metabolic, nervous–system, cardiovascular–system and reproductive–system pathways. Among them, immunomodulatory and metabolic regulatory pathways were the main pathways regulated by marker components during the treatment of cancer and diabetes mellitus.
The pharmacological effects of marker components were investigated further. Ginsenoside Rg1 has been found to: 1) protect the heart from cardiovascular diseases (Xu et al., 2018); 2) impact the neuroendocrine system for treatment of depression (Mou et al., 2017); 3) inhibit inflammation and apoptosis (Guo et al., 2019); 4) possess neuroprotective properties (Li G. et al., 2019); 5) enhance gene expression and oxidative muscle metabolism in muscles (Jeong et al., 2019); 6) protect against diabetic nephropathy (DN) by reducing oxidative stress (Du et al., 2018). Hence, Rg1 can regulate the cardiovascular system, nervous system and immune system. Sucrose can reduce procedural pain from single events, including heel lancing, venipuncture and intramuscular injection, which shows that sucrose can regulate the nervous system without side effects. (Stevens et al., 2016). Ginsenoside Ra1 is the main active component of ginseng used for immune regulation (Liu et al., 2019). Hence, ginsenosides Ra1 can affect the cardiovascular system and regulate the immune system. Notoginsenoside K has been shown to exhibit immunologic-adjuvant activities on the humoral immune responses of ICR mice against ovalbumin (Sun et al., 2005; Qin et al., 2006). Ginsenosides Ra1, notoginsenoside K, and ginsenoside Rg1 can affect the cardiovascular system, immune regulation, nervous system, and have anti-inflammatory and antioxidant effects. Sucrose has a protective effect upon HepG2 cells and fibroblasts (Sim et al., 2020), which suggests that sucrose has an antioxidant effect. Maltose can enhance the anti-melanogenic activity (Bin et al., 2017). The results showed that the pharmacological activities of potential quality markers were consistent with those reported in the literature. Seven malonylginsenosides (malonyl-ginsenoside Ra1, malonyl-ginsenoside Ra2, malonyl-ginsenoside Ra3, malonyl-floralginsenoside Rd, malonyl-floralginsenoside Rd6, malonyl-floralginsenoside Re1 and malonyl-floralginsenoside Rd5) were identified as the PPD-type markers of MCG and GG. However, reports on the pharmacologic effects and mechanism of action of malonyl saponins are lacking, which needed further study.
Network pharmacology pathway enrichment showed that the cancer pathways enriched by quality markers were more abundant. Therefore, we used MCF-7 cell line to evaluate the anticancer effect of MCG and GG. The results showed that the anticancer effect of MCG was better than GG, which was consistent with the published data (Kim et al., 2020). The main genes involve in anticancer effect of MCG and GG are PIK3CA, PIK3CG, MTOR and AR, which are also the key genes of PI3K/Akt/mTOR pathway. Among them, the quality markers acting on PIK3CA, PIK3CG and AR were malonyl -ginsenoside Ra3, malonyl-floralginsenoside Rd, malonyl -ginsenoside Ra1, malonyl-floralginsenoside Rd6, malonyl -ginsenoside Ra2, malonyl-floralginsenoside Re1, malonyl-floralginsenoside Rd5, notoginsenoside Rt, notoginsenoside S, notoginsenoside T, notoginsenoside K, ginsenoside Ra1, ginsenoside Ra2, ginsenoside Ra3, Ginsenoside Rg1. The potential quality markers acting on MTOR were malonyl -ginsenoside Ra3, malonyl-floralginsenoside Rd, malonyl -ginsenoside Ra1, malonyl-floralginsenoside Rd6, malonyl -ginsenoside Ra2, malonyl-floralginsenoside Re1, malonyl-floralginsenoside Rd5, which were common quality markers of PIK3CA, PIK3CG, MTOR. Except Notoginsenoside Rt, the content of the remaining 14 quality markers in MCG was higher than GG. In conclusion, the 14 quality markers of MCG play a better role in anticancer by acting on PI3K/Akt/mTOR pathway while the data of mRNA expression of PIK3CA, PIK3CG, MTOR, AR in the MCF-7 cell samples treated by MCG and GG showed that there was no significant difference between ginseng treatment group and control group. However, we found that downstream genes of the PI3K pathway play an important role in cancer therapy through literature research. In addition, MDM2 has been shown to be abnormally upregulated due to gene amplification, increased transcription, and enhanced translation, leading to enhanced degradation and reduction of P53 activity in some human tumors (Konopleva et al., 2020)and therefore, many drugs/compounds have been developed for reactivation of P53 gene by inhibiting MDM2 interaction with P53 in order to treat cancer (Gupta et al., 2019). Moreover, MTOR pathway plays an important role in the treatment of human cancer. mTORC1 mediates its function via its downstream targets 40 S ribosomal S6 kinases (S6K) (Sridharan and Basu, 2020). Prostate specific antigen (PSA) is a serine protease produced by prostate epithelial cells and prostate cancer (PCA), which can be regulated by AR. The increase of PSA level can be used as a diagnostic marker of tumor or cancer (Balk et al., 2003). The RT-PCR data showed that mRNA expression of MDM2, S6K, PSA were significantly decreased, and the mRNA expression of P53 gene was increased in MCG compared with GG. In summary, the results show that the quality markers of MCG can reduce the survival rate of breast cancer cells better by acting on PIK3CG, PIK3CA, mTOR, AR genes and co-regulating the effector genes MDM2, P53, PSA, S6K (Figure 6F).
We characterized, for the first time, 23 chemical components to distinguish 15-year-old MCG and GG, which corresponding to 105 putative targets and 30 pathways. In addition, the 23 components inhibit the proliferation of breast cancer (MCF-7 cells) by regulating the mRNA expression of PSA, S6K, MDM2 and P53, in which MG exhibited stronger effects than that of GG. Integrative Pharmacology Strategy undoubtedly provide an efficient way to identify chemical constituents and explore the pharmacological actions of TCM formulations.
The original contributions presented in the study are included in the article/Supplementary Material, further inquiries can be directed to the corresponding authors.
HX and LH designed the study. SL and PW drafted the manuscript. SL and WY analyzed the chemical constituents in PGCAM. SL analyzed of network pharmacology. SL, CZ, and LZ carried out anticancer pharmacodynamics experiment and RT-PCR detection. JZ and YQ established the UNIFI database of PGCAM. The other authors participated in the design of the study and performed the statistical analysis All authors read and approved the final version of the manuscript.
This work was supported by grants from the National Key Research and Development Program of China (2017YFC1702303, 2017YFC1702104), National Natural Science Foundation of China (81830111, 81774201), National Science and Technology Major Project of China (2019ZX09201005–001–003), the Youth Innovation Team of Shaanxi Universities and Shaanxi Provincial Science and Technology Department Project (2016SF-378), and the Fundamental Research Funds for the Central Public Welfare Research Institutes (ZXKT17058). These funding agencies had no role in the study design, collection, analyses, or interpretation of data, writing of the report, or the decision to submit the manuscript for publication.
The authors declare that the research was conducted in the absence of any commercial or financial relationships that could be construed as a potential conflict of interest.
We thank JZ in Liaoning Shangyao Good Nurse Pharmaceutical Co., Ltd. for his help on the suppling the 15 years of MCG and GG samples.
The Supplementary Material for this article can be found online at: https://www.frontiersin.org/articles/10.3389/fphar.2021.659954/full#supplementary-material.
Balk, S. P., Ko, Y.-J., and Bubley, G. J. (2003). Biology of prostate-specific antigen. J Clin Oncol. 21, 383–391. doi:10.1200/jco.2003.02.083
Bin, B.-H., Kim, S. T., Bhin, J., Byoun, K., Lee, T. R., and Cho, E.-G. (2017). The synergistic effect of maltose enhances the anti-melanogenic activity of acarbose. Arch. Dermatol. Res. 309, 217–223. doi:10.1007/s00403-017-1717-4
Chang, X. W., Wang, B. R., Wang, T., Li, D. K., Zhao, Y., Zhang, Y. L., et al. (2016). [Plant metabolomics approach for age discrimination of mountain cultivated ginseng using UPLC-Q-TOF/MS]. Zhongguo Zhong Yao Za Zhi. 41, 3609–3614. doi:10.4268/cjcmm20161918
Chang, X., Zhang, J., Li, D., Zhou, D., Zhang, Y., Wang, J., et al. (2017). Nontargeted metabolomics approach for the differentiation of cultivation ages of mountain cultivated ginseng leaves using UHPLC/QTOF-MS. J. Pharm. Biomed. Anal. 141, 108–122. doi:10.1016/j.jpba.2017.04.009
Cui, L. L., Fu, S. F., Wang, Y. P., Zhao, J. H., and Yao, C. L. (2013). Comparison of saponin content of Panax ginseng from different habitats and different years in Jilin Province. J. Jilin Agric. Univ. 35, 427–432.
Du, N., Xu, Z., Gao, M., Liu, P., Sun, B., and Cao, X. (2018). Combination of Ginsenoside Rg1 and Astragaloside IV reduces oxidative stress and inhibits TGF-β1/Smads signaling cascade on renal fibrosis in rats with diabetic nephropathy. Drug Des Devel Ther. Vol. 12, 3517–3524. doi:10.2147/dddt.s171286
Guo, X., Zhang, J., Liu, M., and Zhao, G. C. (2019). Protective effect of ginsenoside Rg1 on attenuating anti-GBM glomerular nephritis by activating NRF2 signalling. Artif. Cell Nanomedicine, Biotechnol. 47, 2972–2979. doi:10.1080/21691401.2019.1640712
Gupta, A., Shah, K., Oza, M. J., and Behl, T. (2019). Reactivation of p53 gene by MDM2 inhibitors: a novel therapy for cancer treatment. Biomed. Pharmacother. 109, 484–492. doi:10.1016/j.biopha.2018.10.155
Jeong, H.-J., So, H.-K., Jo, A., Kim, H.-B., Lee, S.-J., Bae, G.-U., et al. (2019). Ginsenoside Rg1 augments oxidative metabolism and anabolic response of skeletal muscle in mice. J. Ginseng Res. 43, 475–481. doi:10.1016/j.jgr.2018.04.005
Kim, J.-H. (2018). Pharmacological and medical applications of Panax ginseng and ginsenosides: a review for use in cardiovascular diseases. J. Ginseng Res. 42, 264–269. doi:10.1016/j.jgr.2017.10.004
Kim, J., Yoo, J. M., Kim, J. S., Kim, S. G., Park, J. E., Seok, Y. M., et al. (2020). Anticancer effect of mountain ginseng on human breast cancer: comparison with farm-cultivated ginseng. Evid. Based Complement. Alternat Med. 2020, 2584783. doi:10.1155/2020/2584783
Konopleva, M., Martinelli, G., Daver, N., Papayannidis, C., Wei, A., Higgins, B., et al. (2020). MDM2 inhibition: an important step forward in cancer therapy. Leukemia. 34, 2858–2874. doi:10.1038/s41375-020-0949-z
Li, G., Zhang, N., Geng, F., Liu, G., Liu, B., Lei, X., et al. (2019). High-throughput metabolomics and ingenuity pathway approach reveals the pharmacological effect and targets of Ginsenoside Rg1 in Alzheimer's disease mice. Sci. Rep. 9, 7040. doi:10.1038/s41598-019-43537-4
Li, J., Zuo, T., Zhang, C., Li, W., Wang, H., Hu, Y., et al. (2019). Simultaneous profiling and holistic comparison of the metabolomes among the flower buds of Panax ginseng, Panax quinquefolius, and Panax notoginseng by UHPLC/IM-QTOF-HDMS(E)-Based metabolomics analysis. Molecules 24, 2188. doi:10.3390/molecules24112188
Liang, S., Xu, X.-W., Zhao, X.-F., Hou, Z.-G., Wang, X.-H., and Lu, Z.-B. (2016). Two new fatty acids esters were detected in ginseng stems by the application of azoxystrobin and the increasing of antioxidant enzyme activity and ginsenosides content. Pestic. Biochem. Physiol. 134, 63–72. doi:10.1016/j.pestbp.2016.04.005
Liu, J., Nile, S. H., Xu, G., Wang, Y., and Kai, G. (2019). Systematic exploration of Astragalus membranaceus and Panax ginseng as immune regulators: insights from the comparative biological and computational analysis. Phytomedicine 153077. doi:10.1016/j.phymed.2019.153077
Liu, Z., Li, W., Li, X., Zhang, M., Chen, L., Zheng, Y.-N., et al. (2013). Antidiabetic effects of malonyl ginsenosides from Panax ginseng on type 2 diabetic rats induced by high-fat diet and streptozotocin. J. Ethnopharmacology 145, 233–240. doi:10.1016/j.jep.2012.10.058
Mancuso, C., and Santangelo, R. (2017). Panax ginseng and Panax quinquefolius : from pharmacology to toxicology. Food Chem. Toxicol. 107, 362–372. doi:10.1016/j.fct.2017.07.019
Mou, Z., Huang, Q., Chu, S.-F., Zhang, M.-J., Hu, J.-F., Chen, N.-H., et al. (2017). Antidepressive effects of ginsenoside Rg1 via regulation of HPA and HPG axis. Biomed. Pharmacother. 92, 962–971. doi:10.1016/j.biopha.2017.05.119
Nguyen, N. H., and Nguyen, C. T. (2019). Pharmacological effects of ginseng on infectious diseases. Inflammopharmacol 27, 871–883. doi:10.1007/s10787-019-00630-4
Qin, F., Ye, Y.-P., and Sun, H.-X. (2006). Haemolytic activity and adjuvant effect of notoginsenoside K from the roots ofPanax notoginseng. Chem Biodivers. 3, 1144–1152. doi:10.1002/cbdv.200690116
Ruan, X. J., Gao, Y. G., Zhao, Y., Zang, P., He, Z. M., Zhu, H. Y., et al. (2018). Effects of forest type, origin, age and slope aspect on the content of 20 kinds of saponins in Panax ginseng. Food Sci. 39, 195–202.
Shen, L., Xu, J., Hu, H. Y., Hu, X., Zhang, Y. K., Li, X. W., et al. (2019). [Correlative analysis of agronomic traits and quality of Panax ginseng saponins cultivation in farmland]. Zhongguo Zhong Yao Za Zhi. 44, 5124–5128. doi:10.19540/j.cnki.cjcmm.20191009.106
Shi, Z.-Y., Zeng, J.-Z., and Wong, A. S. T. (2019). Chemical structures and pharmacological profiles of ginseng saponins. Molecules 24, 2443. doi:10.3390/molecules24132443
Sim, U., Sung, J., Lee, H., Heo, H., Jeong, H. S., and Lee, J. (2020). Effect of calcium chloride and sucrose on the composition of bioactive compounds and antioxidant activities in buckwheat sprouts. Food Chem. 312, 126075. doi:10.1016/j.foodchem.2019.126075
Sridharan, S., and Basu, A. (2020). Distinct roles of mTOR targets S6K1 and S6K2 in breast cancer. Int. J. Mol. Sci. 21, 1199. doi:10.3390/ijms21041199
Stevens, B., Yamada, J., Ohlsson, A., Haliburton, S., and Shorkey, A. (2016). Sucrose for analgesia in newborn infants undergoing painful procedures. Cochrane Database Syst. Rev. 7, Cd001069. doi:10.1002/14651858.CD001069.pub5
Sun, H., Ye, Y., and Pan, Y. (2005). Immunological-adjuvant saponins from the roots ofPanax notoginseng. Chem Biodivers. 2, 510–515. doi:10.1002/cbdv.200590032
Wang, H.-P., Zhang, Y.-B., Yang, X.-W., Zhao, D.-Q., and Wang, Y.-P. (2016). Rapid characterization of ginsenosides in the roots and rhizomes of Panax ginseng by UPLC-DAD-QTOF-MS/MS and simultaneous determination of 19 ginsenosides by HPLC-ESI-MS. J. Ginseng Res. 40, 382–394. doi:10.1016/j.jgr.2015.12.001
Wu, W., Jiao, C., Li, H., Ma, Y., Jiao, L., and Liu, S. (2018). LC-MS based metabolic and metabonomic studies ofPanax ginseng. Phytochem. Anal. 29, 331–340. doi:10.1002/pca.2752
Xu, H.-Y., Zhang, Y.-Q., Liu, Z.-M., Chen, T., Lv, C.-Y., Tang, S.-H., et al. (2019). ETCM: an encyclopaedia of traditional Chinese medicine. Nucleic Acids Res. 47, D976–d982. doi:10.1093/nar/gky987
Xu, H. Y., and Yang, H. J. (2014). [Integrative pharmacology: new paradigm of modernization of Chinese medicine]. Zhongguo Zhong Yao Za Zhi 39, 357–362.
Xu, X.-f., Cheng, X.-l., Lin, Q.-h., Li, S.-s., Jia, Z., Han, T., et al. (2016). Identification of mountain-cultivated ginseng and cultivated ginseng using UPLC/oa-TOF MSE with a multivariate statistical sample-profiling strategy. J. Ginseng Res. 40, 344–350. doi:10.1016/j.jgr.2015.11.001
Xu, Z.-M., Li, C.-B., Liu, Q.-L., Li, P., and Yang, H. (2018). Ginsenoside Rg1 prevents doxorubicin-induced cardiotoxicity through the inhibition of autophagy and endoplasmic reticulum stress in mice. Int J Mol Sci. 19, 3658. doi:10.3390/ijms19113658
Yang, W.-z., Ye, M., Qiao, X., Liu, C.-f., Miao, W.-j., Bo, T., et al. (2012). A strategy for efficient discovery of new natural compounds by integrating orthogonal column chromatography and liquid chromatography/mass spectrometry analysis: its application in Panax ginseng, Panax quinquefolium and Panax notoginseng to characterize 437 potential new ginsenosides. Analytica Chim. Acta 739, 56–66. doi:10.1016/j.aca.2012.06.017
Yang, W., Qiao, X., Li, K., Fan, J., Bo, T., Guo, D.-A., et al. (2016a). Identification and differentiation of Panax ginseng, Panax quinquefolium, and Panax notoginseng by monitoring multiple diagnostic chemical markers. Acta Pharmaceutica Sinica B. 6, 568–575. doi:10.1016/j.apsb.2016.05.005
Yang, W., Zhang, J., Yao, C., Qiu, S., Chen, M., Pan, H., et al. (2016b). Method development and application of offline two-dimensional liquid chromatography/quadrupole time-of-flight mass spectrometry-fast data directed analysis for comprehensive characterization of the saponins from Xueshuantong Injection. J. Pharm. Biomed. Anal. 128, 322–332. doi:10.1016/j.jpba.2016.05.035
Yang, X. B., Yang, X. W., and Liu, J. X. (2013). Study on ginsenoside in the roots and rhizomes of Panax ginseng. Mod. Chin. Med. 15, 349–358.
Zhang, C., Zuo, T., Wang, X., Wang, H., Hu, Y., Li, Z., et al. (2019). Integration of data-dependent acquisition (DDA) and data-independent high-definition MSE (HDMSE) for the comprehensive profiling and characterization of multicomponents from Panax japonicus by UHPLC/IM-QTOF-MS. Molecules. 24, 2708. doi:10.3390/molecules24152708
Zhu, H., Lin, H., Tan, J., Wang, C., Wang, H., Wu, F., et al. (2018). UPLC-QTOF/MS-Based nontargeted metabolomic analysis of mountain- and garden-cultivated ginseng of different ages in northeast China. Molecules 24, 33. doi:10.3390/molecules24010033
Zhu, L., Luan, X., Yuan, Y., Dou, D., and Huang, L. (2021). The characteristics of ginsenosides and oligosaccharides in mountain‐ and garden‐cultivated ginseng. J. Sci. Food Agric. 101, 1491–1498. doi:10.1002/jsfa.10762
Zuo, T. T., Zhang, C. X., Li, W. W., Wang, H. D., Hu, Y., Yang, W. Z., et al. (2019). Offline two-dimensional liquid chromatography coupled with ion mobility-quadrupole time-of-flight mass spectrometry enabling four-dimensional separation and characterization of the multicomponents from white ginseng and red ginseng. J. Pharm. Anal. 10, 597-609. doi:10.1016/j.jpha.2019.11.001
Keywords: mountain-cultivated ginseng, garden ginseng, UHPLC/IM-QTOF-HDMSE, metabolomics analysis, TCMIP V2.0
Citation: Li S, Wang P, Yang W, Zhao C, Zhang L, Zhang J, Qin Y, Xu H and Huang L (2021) Characterization of the Components and Pharmacological Effects of Mountain-Cultivated Ginseng and Garden Ginseng Based on the Integrative Pharmacology Strategy. Front. Pharmacol. 12:659954. doi: 10.3389/fphar.2021.659954
Received: 28 January 2021; Accepted: 26 March 2021;
Published: 26 April 2021.
Edited by:
Ruiwen Zhang, University of Houston, United StatesReviewed by:
Pei Luo, Macau University of Science and Technology, MacauCopyright © 2021 Li, Wang, Yang, Zhao, Zhang, Zhang, Qin, Xu and Huang. This is an open-access article distributed under the terms of the Creative Commons Attribution License (CC BY). The use, distribution or reproduction in other forums is permitted, provided the original author(s) and the copyright owner(s) are credited and that the original publication in this journal is cited, in accordance with accepted academic practice. No use, distribution or reproduction is permitted which does not comply with these terms.
*Correspondence: Haiyu Xu, aHl4dUBpY21tLmFjLmNu; Luqi Huang, aHVhbmdsdXFpMDFAMTI2LmNvbQ==
†These authors have contributed equally to this work
Disclaimer: All claims expressed in this article are solely those of the authors and do not necessarily represent those of their affiliated organizations, or those of the publisher, the editors and the reviewers. Any product that may be evaluated in this article or claim that may be made by its manufacturer is not guaranteed or endorsed by the publisher.
Research integrity at Frontiers
Learn more about the work of our research integrity team to safeguard the quality of each article we publish.