- 1Key Laboratory of Basic Pharmacology of Ministry of Education and Joint International Research Laboratory of Ethnomedicine of Ministry of Education, Zunyi Medical University, Zunyi, China
- 2Key Laboratory of Basic Pharmacology of Guizhou Province, Zunyi Medical University, Zunyi, Guizhou, China
- 3Department of Clinical Pharmacotherapeutics, School of Pharmacy, Zunyi Medical University, Zunyi, China
Trilobatin (TLB) is an effective component from Lithocarpus polystachyrus Rehd. Our previous study revealed that TLB protected against oxidative injury in neuronal cells by AMPK/Nrf2/SIRT3 signaling pathway. However, whether TLB can delay aging remains still a mystery. Therefore, the present study was designed to investigate the possible longevity-enhancing effect of TLB, and further to explore its underlying mechanism in Caenorhabditis elegans (C. elegans). The results showed that TLB exerted beneficial effects on C. elegans, as evidenced by survival rate, body movement assay and pharynx-pumping assay. Furthermore, TLB not only significantly decreased ROS and MDA levels, but also increased anti-oxidant enzyme activities including CAT and SOD, as well as its subtypes SOD2 andSOD3, but not affect SOD1 activity, as evidenced by heat and oxidative stress resistance assays. Whereas, the anti-oxidative effects of TLB were almost abolished in SKN1, Sir2.3, and DAF16 mutant C. elegans. Moreover, TLB augmented the fluorescence intensity of DAF16: GFP, SKN1:GFP, GST4:GFP mutants, indicating that TLB increased the contents of SKN1, SIRT3 and DAF16 due to fluorescence intensity of these mutants, which were indicative of these proteins. In addition, TLB markedly increased the protein expressions of SKN1, SIRT3 and DAF16 as evidenced by ELISA assay. However, its longevity-enhancing effect were abolished in DAF16, Sir2.3, SKN1, SOD2, SOD3, and GST4 mutant C. elegans than those of non-TLB treated controls. In conclusion, TLB effectively prolongs lifespan of C. elegans, through regulating redox homeostasis, which is, at least partially, mediated by SKN1/SIRT3/DAF16 signaling pathway.
Introduction
Aging, a sophisticated biological process, is characterized as a gradual recession in biological functions at the molecular, organelle, tissue, even the entire organism level (Liu and Li, 2020; Zhang et al., 2020). Aging is considered as a greatest risk factor for vast of the mortality and morbidity in the world, due to it results in multiple increment incidence rates of cardiovascular disease, cerebrovascular disease, cancer, and neurodegenerative disease (Majidinia et al., 2019). Since the world population is aging at an accelerated pace, aging-related disorders aggravate the burden in economic terms and the suffering in human. Emerging evidence indicates that hesitate aging per se will be potential to simultaneously delay aging-related disorders (Gomes et al., 2020). However, the detailed mechanisms of aging are complex. Emerging evidence manifests that oxidative stress and mitochondrial dysfunction play imperative roles in aging (Park et al., 2020). Concomitant reduction in antioxidant capacity and/or increase in oxidative stress, which is referred to as the imbalance between the antioxidant enzyme system and production of reactive oxygen species (ROS). ROS is termed as a heterogeneous population of biologically active intermediation, deriving from the erobic metabolism as by-products and display a dual role in biology (Zhang et al., 2019). That is, ROS exhibits beneficial role in normal physiological conditions, while, superabundant ROS is a saboteur to aging or aging-related disorders. Of note, recent researches suggest that the silent information regulator 2 (SIR2) family is a highly conserved nicotinamide adenine dinucleotide-binding catalytic domain and involves in the cellular events mediating aging-related diseases (Talarowska et al., 2019; Hallows, et al., 2011). Sirtuin 3 (SIRT3) is the vital mitochondrial protein deacetylase of SIR2 family and it is known as an emerging mediator of mitochondrial responses to oxidative stress or aging, as well as modulates cell survival (Quan et al., 2020). Thus, the SIRT3 is expected to be a promising target for prophylaxis and treatment of aging.
Recent reports demonstrate that traditional Chinese medicine (TCM) or folk medicines and their active compounds exhibit effective anti-aging activities, such as resveratrol and curcumin (Kumar et al., 2018; Li et al., 2018). It is interesting to note that trilobatin (Figures 1A,B), a natural bioflavonoid derived from the leaves of Lithocarpus polystachyus Rehd, exerts multiple curative effects such as hypoglycemic effect, antiviral effect (Lei et al., 2018; Yin et al., 2018). Intriguingly, our previous study revealed that TLB effectively protected against oxidative injury in neuronal PC12 cells through regulating mitochondrial ROS homeostasis mediated by AMPK/NRF2/SIRT3 signaling pathway (Gao et al., 2018). However, whether TLB can anti-aging remains still unknown. Therefore, the aim of this study was designed to investigate the effect of TLB on aging in C. elegans and further to explore its potential mechanisms.
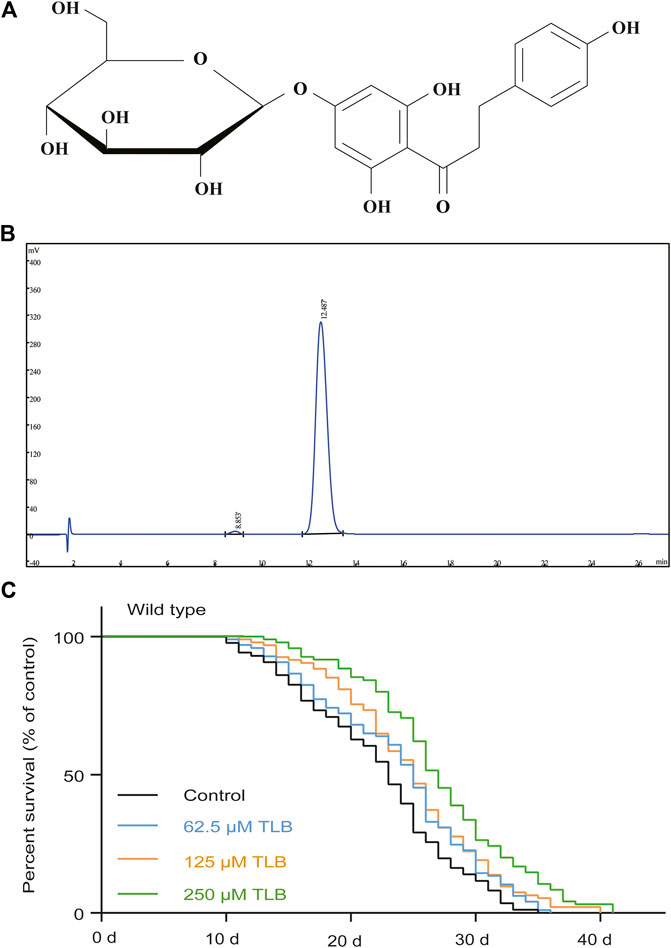
FIGURE 1. TLB extended lifespan under normal cultivate condition at 20°C in wild type C. elegans. (A) The structure of TLB. (B) The high-performance liquid chromatography of TLB. Wild type C. elegans were treated with or without 62.5, 125, and 250 μM TLB. (C) The Kaplan-Meier survival curves of wild type C. elegans (n = 86–97). Data were presented as mean ± SEM.
Materials and Methods
Chemicals and Reagents
TLB (purity ≥98%) was purchased from Guangdong Kedi Medical Technology Corporation, and TLB was dissolved in dimethyl sulfoxide (DMSO) for storage to 1 mM and diluted in PBS. The final concentration of DMSO in the media was less than 1‰ (v/v). Agar powder (A8190), yeast extract (LP0021), Tryptone (LP0042), DMSO (D8371), cholesterol (C8280) and glycerol (G8190) were purchased from Solarbio (Beijing, China). ROS ELISA assay kit (RJ21993), MDA ELISA assay kit (RJ25505), SOD ELISA assay kit (RJ21986), SOD1 ELISA assay kit (RJ25669), SOD2 ELISA assay kit (RJ25670), SOD3 ELISA assay kit (RJ25671), glutathione (GSH) ELISA assay kit (RJ21991), CAT ELISA assay kit (RJ25506), and glutathione peroxidase (GSH-Px) ELISA assay kit (RJ25510) were purchased from Shanghai Ren Jie Biotechnology (Shanghai, China). SIRT3 ELISA kit (KD15803), FOXO3 ELISA kit (KD20035), and NRF2 ELISA kit (KD14201) were purchased from Guangzhou Kedi Biotechnology (Guangzhou, China) (-) -tetramisole hydrochloride (SLBN8309V) was purchased from Sigma-Aldrich (St Louis, MO, United States). Paraquat (FY-C10104076) was purchased from Nantong Feiyu Biotechnology (Jiangsu, China).
C. elegans Culture
All strains were afforded by Caenorhabditis Genetics Center (CGC, University of Minnesota, United States). The strains were used in this experiment as follow: Bristol N2 (wild type, WT); DA1116 eat2 (ad1116) II, RB654 sir2.3 (ok444) X, EU1 skn1 (zu67) IV, CF1038 daf16 (mu86) I, CF1139 muls61 (DAF16:GFP), CL2166 dvIs19 III (GST4:GFP), KN259 huIs33 (SOD3:GFP), LD1008 ldEx9 (SKN1:GFP), and TJ375 gpIs1 (heat shock protein 16.2:GFP, HSP16.2:GFP). C. elegans were maintained on nematode growth medium (NGM) with Escherichia coli OP50 at 20°C.
Lifespan Experiment
All age-synchronized strains were cultivated for 2-3 generations on fresh NGM plates at 20°C before used for lifespan analysis. Thereafter, late L4 larvae or young adults were shifted to 35 mm NGM plates with or without different concentrations of TLB (62.5, 125, and 250 μM) containing live or heat-inactivated E. coli OP50 (65°C for 1 h). The L4 C. elegans were defined as a start time point (d 0) for lifespan assay. C. elegans that failed to response to a mechanical stimulation were scored as dead when they failed to response to a mechanical stimulation (with a platinum wire (Huang et al., 2017). Since 250 μM TLB exhibited the best lifespan prolonged effect on lifespan, it was selected as the optimal concentration in following experiments.
Heat Stress and Oxidative Stress Resistance Assays
The effects of TLB on thermo-tolerance and oxidative stress in C. elegans were determined using thermo-tolerance assay and oxidative stress tolerance assay, respectively. In brief, the age-synchronized late L4 larvae or young adult C. elegans were shifted onto plates with or without TLB (250 μM) for 72 h. Thereafter, the adult C. elegans were incubated at 35°C for 2 h or treated with 10 mM paraquat at 20°C for all the life of C. elegans to determine the effects of TLB on heat stress and oxidative stress, respectively. The survival rates of C. elegans were recorded once per day as previous study (Zheng et al., 2017).
Determination of Progeny Production, Body Movement and Pharynx-Pumping
The breeding experiment was proceeded as previous study (Shen et al., 2018). In brief, C. elegans were cultured on plates with or without TLB (250 μM). Next, the parents of C. elegans were removed to fresh plates per day until reproduction ceased, and the hatched C. elegans were recorded each day. Moreover, body movement assay was performed as described previously (Yang et al., 2015). Briefly, age-synchronized late L4 larvae or young C. elegans were bred on NGM agar plates with or without 250 μM TLB at 20°C for 3, 6, and 9 days, then C. elegans were shifted to fresh plates. Thereafter, C. elegans body movement were counted with times of sinusoidal motion during 20 s. In addition, the effects of TLB on food intake in C. elegans were determined using counting the times of the pharynx contraction for 20 s.
Measurement of ROS and MDA Levels
C. elegans were raised in the absence or presence of 250 μM TLB for 72 h as mentioned above. Then, C. elegans were cultivated in NGM plates containing 10 mM paraquat and C. elegans homogenate was measured using ROS ELISA assay and lipid peroxidation MDA ELISA assay. In brief, C. elegans were harvested and rinsed thrice with ddH2O. The total protein WT C. elegans or mutant C. elegans were extracted and centrifuged for 10 min at 10,000 × g, 4°C. Then levels of MDA and ROS were detected by ELISA kits under the manufacturer’s instructions.
Measurement of Antioxidant Enzyme Activities
Briefly, C. elegans were treated as mentioned above. The total protein of C. elegans was extracted and centrifuged for 10 min at 10,000 × g, 4°C, then the supernatant was collected. Thereafter, activities of SOD, SOD1, SOD2, SOD3, CAT, GSH, and GSH-Px were detected using appropriate ELISA kits according to the manufacturer’s protocol.
Measurement of Expressions of SKN1, SIRT3, and DAF16
C. elegans were raised in the absence or presence of 250 μM TLB for 72 h as mentioned above. The total protein of C. elegans was extracted and centrifuged for 10 min at 10,000 × g, 4°C, then the supernatant was collected. Thereafter, expressions of SKN1, SIRT3, and DAF16 were detected using appropriate ELISA kits according to the manufacturer’s protocol.
Determination of Fluorescence in Transgenic C. elegans
The age-synchronized late L4 larvae or young transgenic adult C. elegans including CF1139 (DAF16:GFP), CL2166 (GST4:GFP), KN259 (SOD3:GFP), LD1008 (SKN1:GFP), TJ375 (HSP16.2:GFP) were raised on NGM dishes with or without 250 μM TLB for 72 h at 20°C. Thereafter, TJ375 mutants were treated by heat stress at 35°C for 2 h, and the fluorescence of C. elegans were observed under a fluorescence microscopy (Olympus BX53 + DP80, Olympus, Japanese) at wavelength with excitation/emission (360/420 nm) filters after the transgenic mutants were anesthetized using (-) -tetramisole hydrochloride (5 mM). The fluorescence of transgenic C. elegans were quantified using the Image Pro Plus 6.0 software.
Determination of Lipofuscin in C. elegans
The effects of TLB on aging in C. elegans were evaluated by detecting lipofuscin level. Briefly, C. elegans were treated with or without TLB for 5 or 10 days as mentioned above. Then C. elegans were randomly selected and washed with M9 buffer for three times and then anesthetized with 5 mM levamisole as described in previously study (Yang et al., 2018). The intestinal spontaneous fluorescence of C. elegans were observed using fluorescence microscopy (Olympus BX53 + DP80, Olympus, Japanese) at wavelength with excitation/emission (360/420 nm) filters. The fluorescence of C. elegans were quantified using the Image Pro Plus 6.0 software.
Statistical Analyses
The data were confirmed and conducted by using Graphpad Prism version 6.0 (Graphpad software, Inc. San Diego, United States). All data were expressed as mean ± SEM and analyzed with the SPSS 18.0 software (SPSS, Inc. Chicago, IL, United States). Besides, the data of lifespan experiments and stress resistance experiments were performed using the Kaplan–Meier survival analysis. All results were verified by at least three independent experiments. p < 0.05 was considered statistically significant.
Results
TLB Prolonged Longevity in C. elegans Under Normal Cultivate Condition
In order to confirm whether TLB can prolong the lifespan, the WT C. elegans were treated with different concentrations (62.5, 125, and 250 μM) of TLB. The results indicated that TLB (62.5, 125, and 250 μM) markedly prolonged the mean longevity by 7.7, 11.7, and 22.1% in C. elegans than those of non-TLB treated controls, respectively (Figure 1B, Table 1). These findings demonstrated that TLB led to a prominent increasement of lifespan in a concentration-dependent manner.
TLB Increased Body Movement and Attenuated Pharynx-Pumping Rate or Lipofuscin but Not Affected the Propagation of C. elegans
The results showed that TLB did not affect the total progeny number of C. elegans (Figure 2A). Moreover, TLB markedly augmented the movement ratio of C. elegans than that of non-TLB treated controls (Figure 2B). Afterward, we detected the times of pharyngeal pumping to evaluate the food intake of C. elegans, the results showed that TLB markedly reduced the times of pharyngeal pumping when C. elegans were cultured both in live or heat-inactivated OP50 (Figure 2C), indicating that TLB reduced the decline of pharyngeal pumping rate accompanied with aging. Of note, there was no significant difference on the times of pharyngeal pumping of C. elegans that cultured both in live or heat-inactivated OP50, and due to it is easier to observe the change of C. elegans in heat-inactivated OP50 than that in live OP50, the heat-inactivated OP50 was used in the following experiment. Furthermore, eat2 C. elegans, an abnormal pharyngeal pumping mutant C. elegans, was used to confirm the attenuative effect of TLB on pharyngeal pumping. Of note, the results suggested that longevity-enhancing effects of TLB on C. elegans was partly abolished in eat2 mutant C. elegans than that of non-TLB treated controls (Figure 2D). These findings suggested that beneficial effects of TLB on lifespan was partially dependent on food intake, and there were probably other factors that still needed further explore. Intriguingly, TLB also dramatically attenuated the endogenous lipofuscin than that of non-TLB treated controls (Figures 2E,F), which further indicated that TLB played an active role in delaying aging.
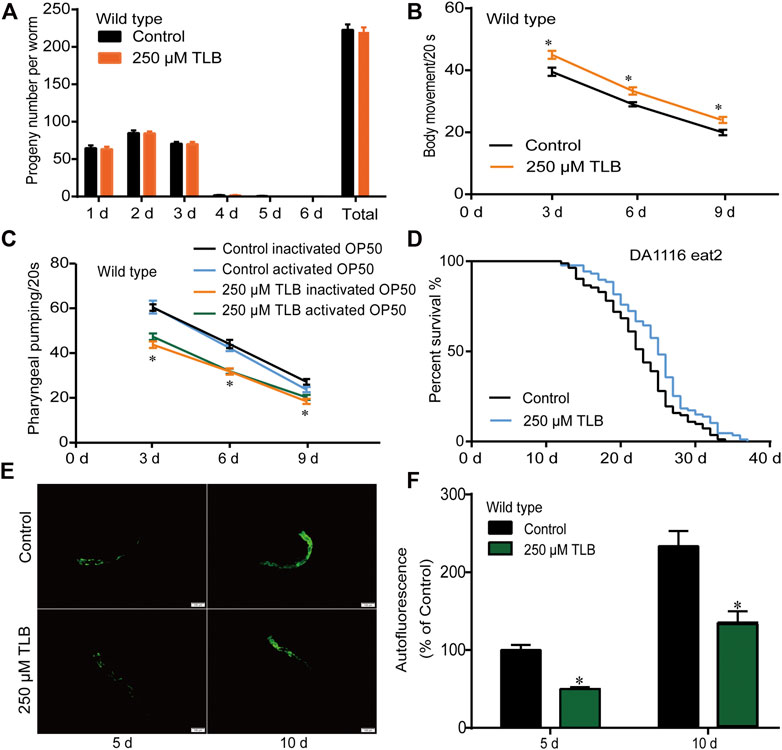
FIGURE 2. TLB changed behaviors in aging C. elegans. (A) The number of progeny per day and the total number of progeny of C. eleganss treated with 250 μM TLB at the onset of age-synchronized late L4 or young larvae C. elegans (n = 10). (B) Age-synchronized late L4 larvae or young C. elegans were bred on NGM agar plates with or without 250 μM TLB at 20 °C for 3, 6, and 9 days, C. elegans body movement were counted with times of sinusoidal motion during 20 s (n = 20). (C) The effects of TLB on food intake in C. elegans were determined the times of the pharynx contraction for 20 sat 20°C for 3, 6, and 9 days (n = 10). p values were calculated by independent-samples t test. (D) The survival curves of eat2 (DA1116) C. elegans raised on 0 and 250 μM TLB at 20°C (n = 82–87). The lifespan was analyzed by the Kaplan-Meier test, and p values were calculated by the log-rank test. (E) Representive lipofuscin autofluorescence image of 0 and 250 μM TLB C. elegans on the 5 and 10 days of adulthood. The fluorescence of C. elegans were observed under a fluorescence microscopy. (F) The mean pixel fluorescence of transgenic C. elegans were quantified using the Image Pro Plus 6.0 software. Magnification × 100; scale bar, 100 μm (n = 20). Data were presented as mean ± SEM. *p < 0.05 vs. non-TLB treated controls.
TLB Enhanced the Lifespan in C. elegans Under Heat Stress Condition
The results showed that the mean lifespan of C. elegans treated with TLB was markedly augmented than that of non-TLB treated controls, which manifested that TLB observably heightened C. elegans resistance to thermal tolerance (Figure 3A). Analogously, HSP16.2 gene levels were also measured using transgenic strain TJ375 (HSP16.2:GFP). The results revealed that the HSP16.2 levels of C. elegans were enhanced observably by TLB treated than that of non-TLB treated controls (Figures 3B,C), manifesting that TLB significantly increased the capacity for resistance to thermal stress.
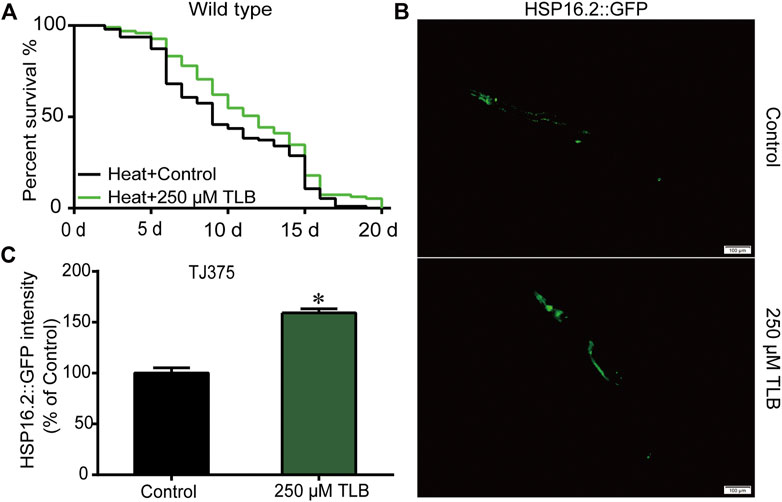
FIGURE 3. TLB reinforced the heat stress tolerance of C. elegans. Wild type C. elegans were treated with or without TLB under 35°C condition. (A) The Kaplan-Meier survival curves under 35°C condition (n = 94–95). (B) The HSP16.2 transgenic C. elegans were treated by heat stress at 35°C for 72 h, and the fluorescence of C. elegans were observed under a fluorescence microscopy. (C) The mean pixel fluorescence intensity of protein HSP16.2 were quantified using the Image Pro Plus 6.0 software (n = 20). Magnification × 100; scale bar, 100 μm. Data were presented as mean ± SEM. *p < 0.05 vs. non-TLB treated controls.
TLB Enhanced the Lifespan in C. elegans Under Oxidative Stress Condition
The results showed that TLB increased the lifespan than that of non-TLB treated controls after exposed to paraquat (Figure 4A). Furthermore, in order to explore the antioxidative effects of TLB, the levels of ROS and MDA, the antioxidative enzyme activities (GSH, GSH-Px, SOD, CAT) were determined. The results demonstrated that TLB decreased the levels of ROS and MDA than that of non-TLB treated controls after exposed to paraquat (Figures 4B,C). Additionally, TLB markedly strengthened CAT and SOD activities (p < 0.001) (Figures 4D,E). However, TLB did not affect GSH and GSH-Px activities (Figures 4I,J), while TLB significantly enhanced SOD2 and SOD3 activities, but not affected SOD1 activity (Figures 4F–H). In addition, the level of SOD3 was also quantified through utilizing transgenic strain KN259 (SOD3:GFP). The results showed that TLB significantly increased the GFP intensity of C. elegans than that of non-TLB controls (Figures 4K,L), indicating that TLB may be an activator of SOD, which could enhance the antioxidant ability. Whereas, the antioxidative effects of TLB were almost abolished in SKN1 (Figure 5), SIR2.3 (Figure 6) and DAF16 (Figure 7) mutant C. elegans. These findings suggested that longevity-enhancing effect of TLB on C. elegans, at least partly, through its anti-oxidant activities, indicating that TLB exerted a protective role on oxdative stress.
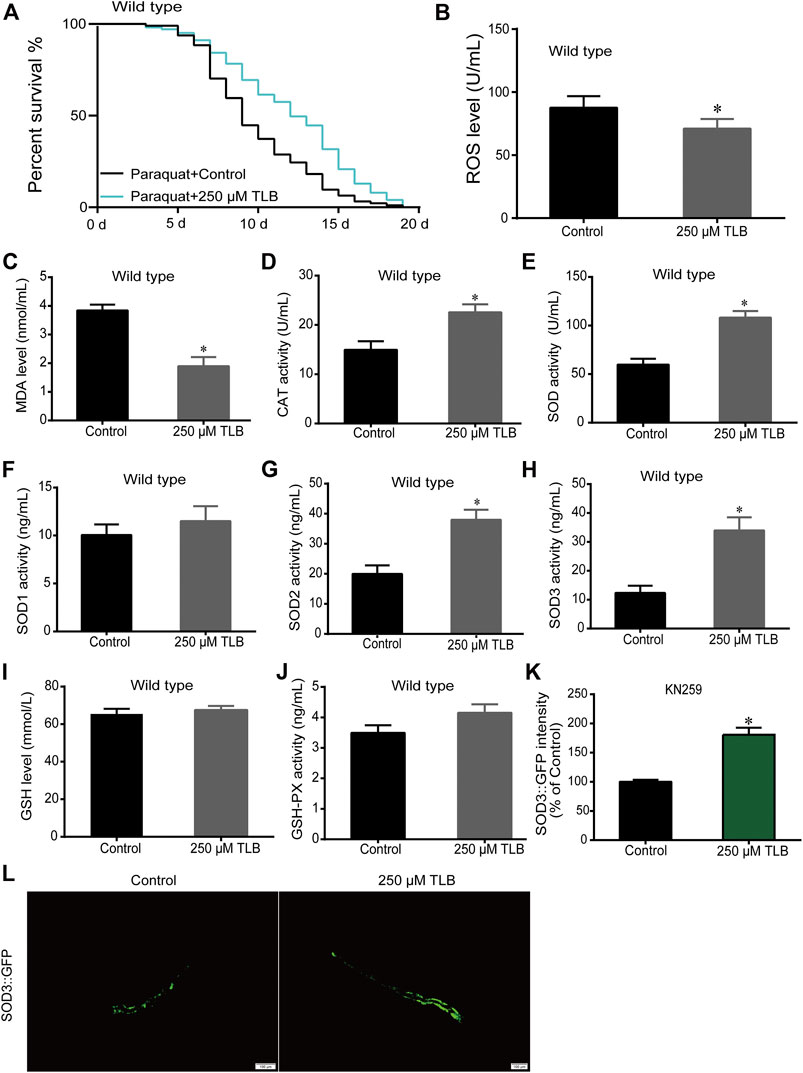
FIGURE 4. TLB reinforced the oxidative stress tolerance of C. elegans. Wild type C. elegans were treated with or without TLB after paraquat insult. (A) The Kaplan-Meier survival curves after paraquat insult. Wild type C. elegans were treated with TLB (n = 93–101), and p values were calculated by the log-rank test. C. elegans were raised in the absence or presence of 250 μM TLB for 72 h. The total protein of C. elegans was extracted and centrifuged for 10 min at 10,000 × g, 4°C, then the supernatant was detected using appropriate ELISA kits according to the manufacturer’s protocol.(B) ROS level (n = 1000); (C) MDA level (n = 1000); (D) CAT activity (n = 1000); (E) SOD activity (n = 1000); (F) SOD1 activity (n = 1000); (G) SOD2 activity (n = 1000); (H) SOD3 activity (n = 1000); (I) GSH level (n = 1000); (J) GSH-Px activity (n = 1000). (K) The SOD3 transgenic C. elegans were raised in the absence or presence of 250 μM TLB at 20°C for 72 h, and the fluorescence of C. elegans were observed under a fluorescence microscopy. (L)The mean pixel fluorescence intensity of protein SOD3 were quantified using the Image Pro Plus 6.0 software (n = 20). Magnification × 100; scale bar, 100 μm. Data were presented as mean ± SEM. *p < 0.05 vs. non-TLB treated controls.
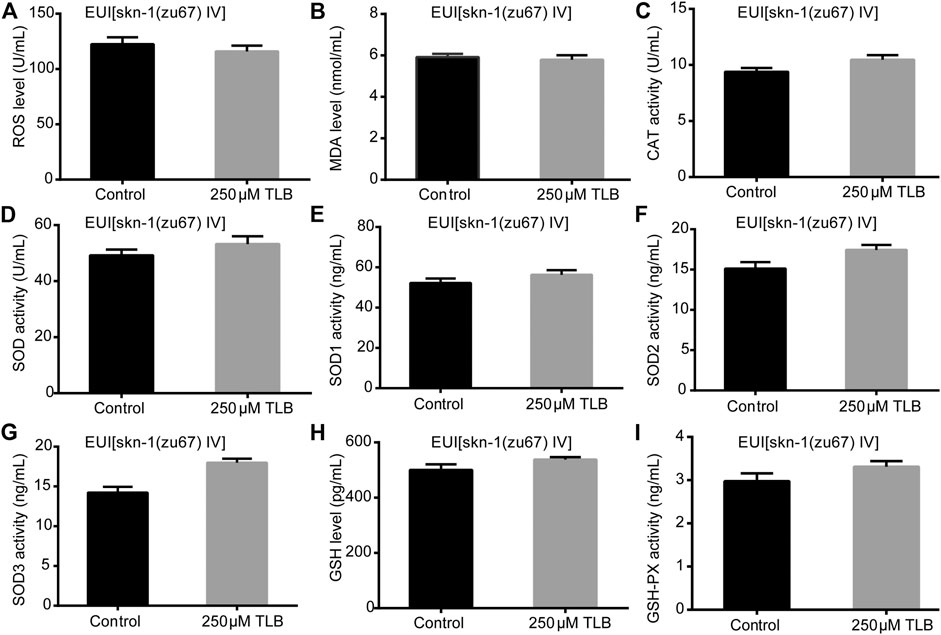
FIGURE 5. Anti-oxidant effects of TLB in SKN1 mutant C. elegans. C. elegans were raised in the absence or presence of 250 μM TLB for 72 h. The total protein of C. elegans was extracted and centrifuged for 10 min at 10,000 × g, 4°C, then the supernatant was detected using appropriate ELISA kits according to the manufacturer’s protocol. (A) ROS level; (B) MDA level; (C) CAT activity; (D) SOD activity; (E) SOD1 activity; (F) SOD2 activity; (G) SOD3 activity; (H) GSH activity; (I) GSH-Px activity. n = 1000. Data were presented as mean ± SEM.
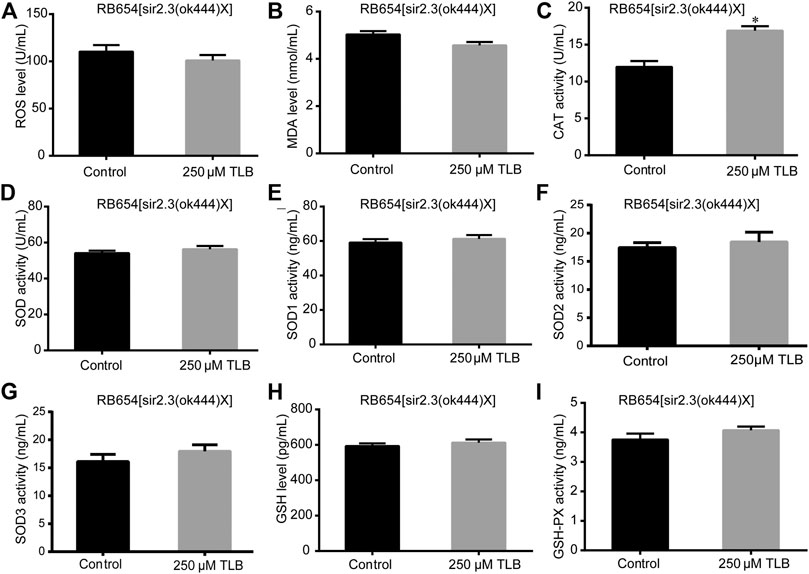
FIGURE 6. Anti-oxidant effects of TLB in Sir2.3 mutant C. elegans. C. elegans were raised in the absence or presence of 250 μM TLB for 72 h. The total protein of C. elegans was extracted and centrifuged for 10 min at 10,000 × g, 4°C, then the supernatant was detected using appropriate ELISA kits. (A) ROS level; (B) MDA level; (C) CAT activity; (D) SOD activity; (E) SOD1 activity; (F) SOD2 activity; (G) SOD3 activity; (H) GSH activity; (I) GSH-Px activity. n = 1000. Data were presented as mean ± SEM. *p < 0.05 vs. non-TLB treated controls.
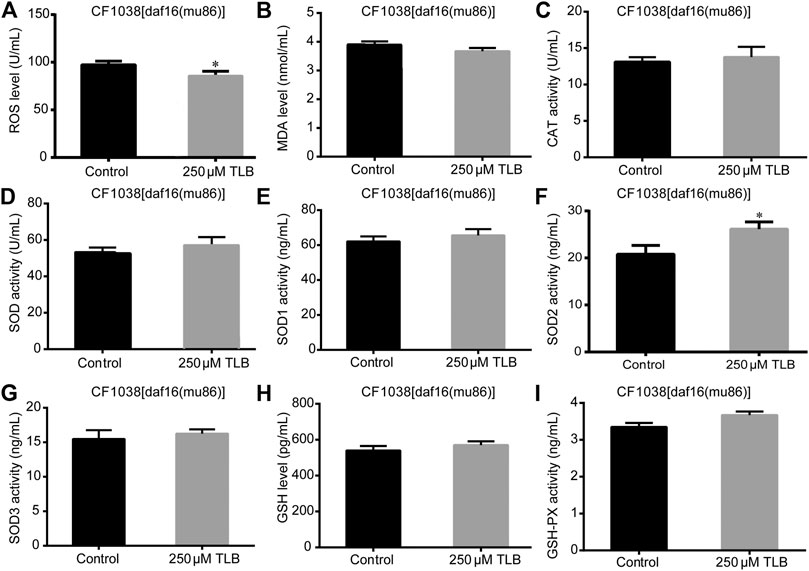
FIGURE 7. Anti-oxidant effects of TLB DAF16 mutant C. elegans. C. elegans were raised in the absence or presence of 250 μM TLB for 72 h. The total protein of C. elegans was extracted and centrifuged for 10 min at 10,000 × g, 4°C, then the supernatant was detected using appropriate ELISA kits. (A) ROS level; (B) MDA level; (C) CAT activity; (D) SOD activity; (E) SOD1 activity; (F) SOD2 activity; (G) SOD3 activity; (H) GSH activity; (I) GSH-Px activity. n = 1000. Data were presented as mean ± SEM. *p < 0.05 vs. non-TLB treated controls.
DAF16/SIRT3/SKN1 Signaling Pathway Was Involved in Longevity-Enhancing Effects of TLB in C. elegans
The results of ELISA assay further demonstrated that TLB increased the expressions of the nuclear localization of DAF16, SIRT3 and SKN1(Figures 8A–C), as well as, augmented the fluorescence intensity of DAF16:GFP, SKN1:GFP, GST4:GFP than those of non-TLB treated controls (Figures 9A–D) as evidenced by the fluorescence of GFP mutants. Furthermore, the longevity-enhancing effects of TLB were almost abolished in DAF16, Sir2.3, SKN1, SOD3, SOD2, and GST4 mutant C. elegans than those of WT C. elegans (Figures 9E–J).
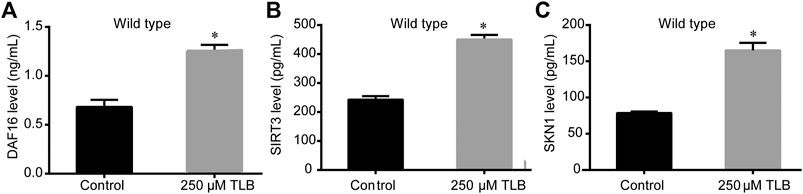
FIGURE 8. TLB increased the protein expressions of DAF16, SIRT3 and SKN1of WT C. elegans. C. elegans were raised in the absence or presence of 250 μM TLB for 72 h. The total protein of C. elegans was extracted and centrifuged for 10 min at 10,000 × g, at 4°C, then the supernatant was detected using appropriate ELISA kits. (A) DAF16 level (B) SIRT3 level (C) SKN1 level. (n = 1000). Data were presented as mean ± SEM. *p < 0.05 vs. non-TLB treated controls.
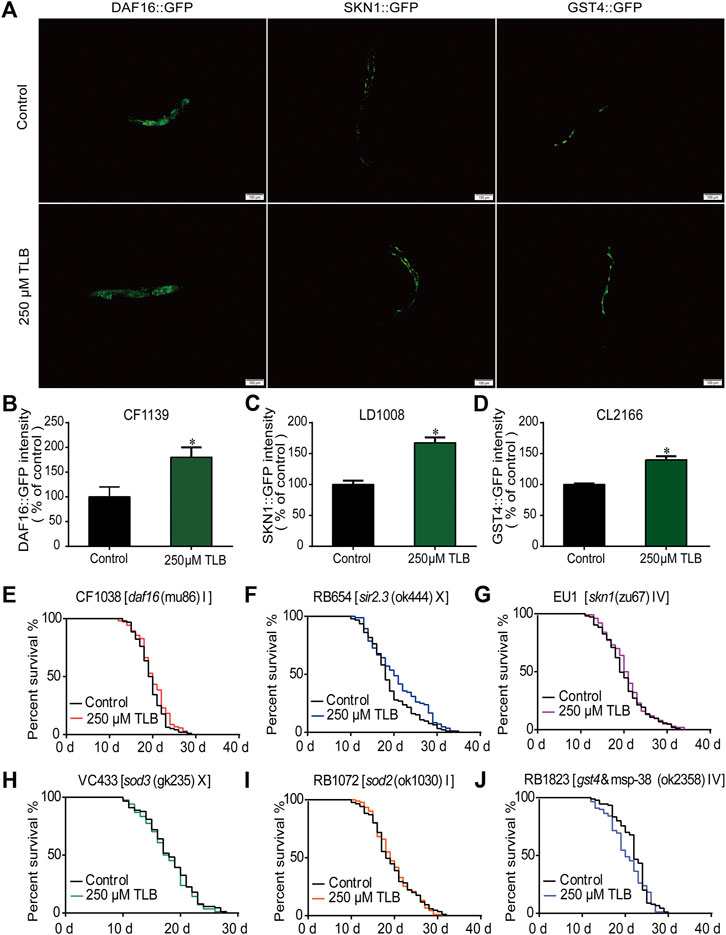
FIGURE 9. TLB extended lifespan via SKN1/SIRT3/DAF16 signaling pathway. Wild type or mutant type C. elegans were treated with or without 250 μM TLB for 72 h. (A) The mean pixel fluorescence intensity of proteins GST4, SKN1, and the nuclear localization of DAF16 (n = 20). The fluorescence of transgenic C. elegans were quantified using the Image Pro Plus 6.0 software. Magnification, 100 × scale bar, 100 μm (B–D) The quantization of levels of DAF16, SKN1 and GST4 (n = 20). All age-synchronized strains were transferred to NGM plates with or without 250 μM TLB at 20°C. Survival curves of (E)daf16 (mu86) (n = 96–104), (F)sir2.3 (ok444) X (n = 84–93), (G)skn1 (zu67) IV (n = 100–103), (H)sod3 (gk235) X (n = 83–89), (I)sod2 (ok1030) (n = 85–91), (J)gst4&msp-38 (ok2358) IV (n = 88–90). Data were presented as mean ± SEM. *p < 0.05 vs. non-TLB treated controls.
Discussion
The dominating findings of this study revealed that: 1) TLB hindered natural aging of C. elegans; 2) TLB not only effectually reduced production of ROS and MDA, but also improved antioxidant enzymes and thermal tolerance in C. elegans; 3) TLB prolonged longevity of C. elegans, at least partly, through activating DAF16/SIRT3/SKN1 signaling pathway (Figure 10).
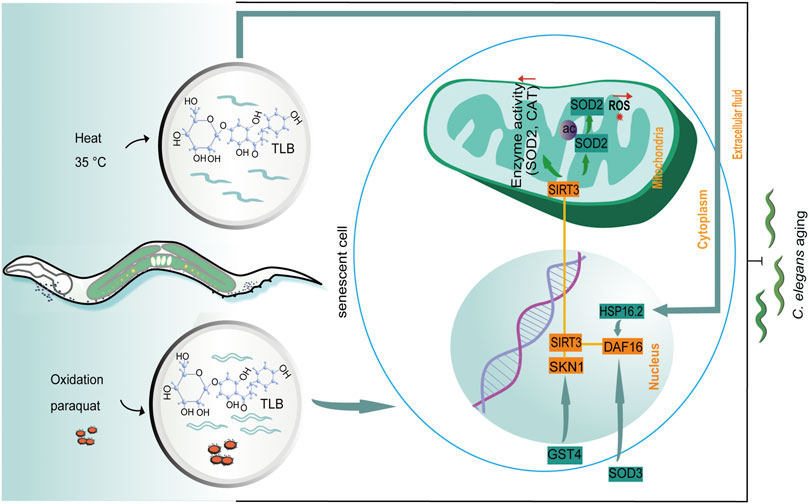
FIGURE 10. The schematic presentation illuminating proposed mechanisms that TLB prolonged lifespan in C. elegans. Excessive ROS production is caused by paraquat and 35°C leading to aging in C. elegans. TLB attenuates overproduction of ROS and improves the anti-oxidative ability through mediating the SKN1/SIRT3/DAF16 axis.
Accumulating evidence suggests that heat shock and oxidative stress are vital reasons of aging (Wang et al., 2016; Huang et al., 2017), which is a dominating dangerous factor for multitudinous diseases, including cancer, diabetes, multiple neurodegenerative disorders, and eventually, these changes result in living organism gradually potential to die (Edifizi and Schumacher, 2017). The senility course in C. elegans is rather conserved and displays resemblances to humans in many aspects such as movement, memorial capacities, immunologic function, generative rate, and cumulation of detrimental metabolites like lipofuscin. Especially, due to its characteristics of short lifespan, morphological simplicity, undemanding maintenance, and genetic manipulation, C. elegans was widely used to the effects of compound on lifespan (Goh et al., 2018). Our findings showed that TLB markedly prolonged the lifespan of C. elegans under normal or stress environment, which suggested that TLB effectively prolonged lifespan. Notably, lifespan is widely considered closely related with reproduction, the locomotion rate, food intake and lipofuscin accumulation in multiple organisms containing C. elegan (Wan et al., 2017; Seo et al., 2018). Our results further revealed that TLB augmented body movement rate of C. elegans, but it had no effect on reproduction. Since dietary restriction with reduced food intake delays aging and increases lifespan in C. elegans. Of note, TLB significantly decreased food intake rate, which might be contribute to the anti-aging effect of TLB.
Interestingly, TLB also mitigated the level of lipofuscin, an indicator of aging in numerous organisms (Evangelou and Gorgoulis, 2017), which further confirmed that TLB exerted longevity-enhancing effects in C. elegans. Of note, HSPs are a class of heat stress proteins with highly conserved structure and function. HSP generated to help proteins fold into a natural conformation under stress conditions such as high temperature, thus protected the organism and prolonged lifespan. Among them, HSP16.2 is considered as a sensitive anti-stress marker and acted as a crucial role in organism to assist correctly proteins fold (Jattujan et al., 2018; Ungvari et al., 2019). The results suggested that TLB markedly increased the HSP16.2 level and its downstream SOD3, suggesting that the longevity-enhancing effect of TLB were, at least partly, due to its anti-stress response. Furthermore, paraquat was used to generate excessive ROS and MDA to mimic oxidative damage (Moldogazieva et al., 2019), and the results demonstrated that TLB significantly decreased the levels of ROS and MDA. Moreover, it is reported that SOD has an activity of transforming intracellular superoxide anion into hydrogen peroxide (H2O2), and GSH-Px is responsible for scavenging the formed H2O2. CAT could effectively convert H2O2 to H2O and O2 (Wang D. et al., 2018). Our findings showed that TLB apparently heightened antioxidant enzyme activities of SOD and CAT, but not affected the GSH level and the GSH-Px activity, which indicated that TLB might promote the elimination of superoxide anion and H2O2 through mediating SOD and CAT activities. Of note, the SOD family is composed of three isotypes: SOD1, SOD2, and SOD3. SOD1, SOD2, and SOD3 catalyze the superoxide anion conversion to H2O2. Among them, SOD1 is mainly located in cytosolic, SOD2 and SOD3 are located in mitochondrial matrix and extracellular space, respectively (Wert et al., 2018). Notably, previous study has reported that both SOD1 and SOD2 effectively inhibit oxidative stress and SOD3 more likely contributes to prolong lifespan. Moreover, SOD2 and SOD3 are also involved in a ROS signal that functions in intercellular crosstalk and mediates longevity (Parascandolo and Laukkanen, 2019). Our results showed that TLB significantly enhanced SOD2 and SOD3 activities, but not affected SOD1 activity, which suggested that TLB might mainly eliminated the ROS of mitochondrial matrix and extracellular space of cells, but not the ROS of cytosolic. These findings suggested that the anti-oxidative effect of TLB mainly due to eliminating excessive ROS in mitochondria and promoting the lifespan-related SOD2 and SOD3 in C. elegans.
Emerging evidence suggests that SOD2 is the downstream of SIRT3, which is located in mitochondria and attenuated mitochondrial ROS (Katwal et al., 2018). In our previous study, a major upstream of SIRT3 that has been proved is NRF2, which is encoded as SKN1 gene on C. elegans (Gao et al., 2018; D'Amora et al., 2018). Our results demonstrated that TLB upregulated the protein expressions of SKN1 and SIRT3, thereby exerting its anti-oxidantive effect; whereas, the anti-oxidantive effect of TLB were almost eliminated in SKN1 and SIRT3 mutant C. elegans, which indicated that NRF2/SIRT3 signaling pathway was involved in the anti-aging effects of TLB in C. elegans. In addition, SOD3 is known as a downstream gene of DAF16, which is an ortholog of the FOXO gene in C. elegans (Wang et al., 2017). Our results showed that TLB significantly increased DAF16 level, and the beneficial effect almost was abolished in DAF16 mutant C. elegans. Since FOXO3 is the downstream of SIRT3(Wang L.-F. et al., 2018), thus it is reasonably assumed that SKN1/SIRT3/DAF16 signaling was involved in the longevity-enhancing effect of TLB in C. elegans; whereas, the mechanistic details of how TLB effect the DAF16, SIRT3, and SKN1 expression will be further explored in our next story.
Of note, emerging evidence reveals that there are close relationship between polyphenol compounds, TCM, redox status and the vitagene network, which encodes for heat shock proteins, the thioredoxin and the sirtuin protein systems, and its possible biological relevance in neuroprotection (Wang Y. et al., 2018). Moreover, preconditioning signal leading to cellular protection through hormesis, terms as a dose response phenomenon characterized by a low dose stimulation and a high dose inhibition, is an important redox dependent aging-associated neurodegenerative/neuroprotective issue (Calabrese et al., 2010; Calabrese et al., 2018; Brunetti et al., 2020; Rosa et al., 2020; Siracusa et al., 2020). We speculated that TLB might be a promising natural radical scavenger for the prophylaxis and neuroprotective effects, thereby exerting an anti-aging effect. To this regard, the pharmacokinetic and pharmacodynamics functions and hormetic properties of TLB in other aging animal models such as SAMP8 mice will be further elucidated.
Taken together, the present study demonstrates that, for the first time, TLB effectively prolongs lifespan of C. elegans, at least partially via modulating SKN1/SIRT3/DAF16 signaling pathway. These findings raise the possibility that TLB may be a potential and effective agent against aging or aging-related diseases.
Data Availability Statement
The original contributions presented in the study are included in the article/Supplementary Material, further inquiries can be directed to the corresponding author.
Ethics Statement
The animal study was reviewed and approved by the Ethics Committee of Zunyi Medicial University.
Author Contributions
QG and JS designed the experiments. NL, XL, and Y-LS performed the experiments. FL analyzed the data. NL wrote the manuscript. J-MG and Y-QH revised the manuscript.
Funding
This work was supported by Natural Science Foundation of China (Grant No. 81760727), National key R & D plan for Research on modernization of Traditional Chinese Medicine (Grant No. 2017YFC1702005), Post subsidy project of State key R & D plan in social development field (Grant No. SQ2017YFC170204–05), the “hundred” level of high-level innovative talents in Guizhou Province (Grant No. QKHRCPT 20165,684), Program for Changjiang Scholars and Innovative Research Team in University, China (Grant No. IRT_17R113), and Program for Outstanding Youth of Zunyi Medical University (Grant No. 15zy-002).
Conflict of Interest
The authors declare that the research was conducted in the absence of any commercial or financial relationships that could be construed as a potential conflict of interest.
References
Brunetti, G., Di Rosa, G., Scuto, M., Leri, M., Stefani, M., Schmitz-Linneweber, C., et al. (2020). Healthspan maintenance and prevention of Parkinson's-like phenotypes with hydroxytyrosol and oleuropein aglycone in C. elegans. Ijms 21, 2588. doi:10.3390/ijms21072588
Calabrese, V., Cornelius, C., Dinkova-Kostova, A. T., Calabrese, E. J., Mattson, M. P., and Mattson, M. P. (2010). Cellular stress responses, the hormesis paradigm, and vitagenes: novel targets for therapeutic intervention in neurodegenerative disorders. Antioxid. Redox Signal. 13, 1763–1811. doi:10.1089/ars.2009.3074
Calabrese, V., Santoro, A., Monti, D., Crupi, R., Di Paola, R., Latteri, S., et al. (2018). Aging and Parkinson's Disease: inflammaging, neuroinflammation and biological remodeling as key factors in pathogenesis. Free Radic. Biol. Med. 115, 80–91. doi:10.1016/j.freeradbiomed.2017.10.379
D'Amora, D. R., Hu, Q., Pizzardi, M., and Kubiseski, T. J. (2018). BRAP-2 promotes DNA damage induced germline apoptosis in C. elegans through the regulation of SKN-1 and AKT-1. Cell Death Differ. 25, 1276–1288. doi:10.1038/s41418-017-0038-7
Edifizi, D., and Schumacher, B. (2017). Omics approaches for identifying physiological adaptations to genome instability in aging. Ijms 18, 2329. doi:10.3390/ijms18112329
Evangelou, K., and Gorgoulis, V. G. (2017). Sudan black B, the specific histochemical stain for lipofuscin: a novel method to detect senescent cells. Methods Mol. Biol. 1534, 111–119. doi:10.1007/978-1-4939-6670-7_10
Gao, J., Liu, S., Xu, F., Liu, Y., Lv, C., Deng, Y., et al. (2018). Trilobatin protects against oxidative injury in neuronal PC12 cells through regulating mitochondrial ROS homeostasis mediated by AMPK/Nrf2/Sirt3 signaling pathway. Front. Mol. Neurosci. 11, 267. doi:10.3389/fnmol.2018.00267
Goh, G. Y. S., Winter, J. J., Bhanshali, F., Doering, K. R. S., Lai, R., Lee, K., et al. (2018). NHR-49/HNF4 integrates regulation of fatty acid metabolism with a protective transcriptional response to oxidative stress and fasting. Aging cell 17, e12743. doi:10.1111/acel.12743
Gomes, A. P., Ilter, D., Low, V., Endress, J. E., Fernández-García, J., Rosenzweig, A., et al. (2020). Age-induced accumulation of methylmalonic acid promotes tumour progression. Nature 585, 283–287. doi:10.1038/s41586-020-2630-0
Hallows, W. C., Yu, W., Smith, B. C., Devires, M. K., Ellinger, J. J., Someya, S., et al. (2011). Sirt3 promotes the urea cycle and fatty acid oxidation during dietary restriction. Mol. Cel 41, 139–149. doi:10.1016/j.molcel.2011.01.002
Huang, X.-B., Mu, X.-H., Wan, Q.-L., He, X.-M., Wu, G.-S., and Luo, H.-R. (2017). Aspirin increases metabolism through germline signalling to extend the lifespan of Caenorhabditis elegans. PLoS One 12, 499–506. doi:10.1371/journal.pone.0184027
Jattujan, P., Chalorak, P., Siangcham, T., Sangpairoj, K., Nobsathian, S., Poomtong, T., et al. (2018). Holothuria scabra extracts possess anti-oxidant activity and promote stress resistance and lifespan extension in Caenorhabditis elegans. Exp. Gerontol. 110, 158–171. doi:10.1016/j.exger.2018.06.0010.1016/j.exger.2018.06.006
Katwal, G., Baral, D., Fan, X., Weiyang, H., Zhang, X., Ling, L., et al. (2018). SIRT3 a major player in attenuation of hepatic ischemia-reperfusion injury by reducing ROS via its downstream mediators: SOD2, CYP-D, and HIF-1α. Oxidative Med. Cell Longevity 2018, 1. doi:10.1155/2018/2976957
Kumar, S. S. D., Houreld, N. N., and Abrahamse, H. (2018). Therapeutic potential and recent advances of curcumin in the treatment of aging-associated diseases. Molecules 23, 835. doi:10.3390/molecules23040835
Lei, L., Huang, B., Liu, A., Lu, Y.-J., Zhou, J.-L., Zhang, J., et al. (2018). Enzymatic production of natural sweetener trilobatin from citrus flavanone naringin using immobilised α-l -rhamnosidase as the catalyst. Int. J. Food Sci. Technol. 53, 2097–2103. doi:10.1111/ijfs.13796
Li, Y.-R., Li, S., and Lin, C.-C. (2018). Effect of resveratrol and pterostilbene on aging and longevity. Biofactors 44, 69–82. doi:10.1002/biof.1400
Liu, R.-M., and Liu, G. (2020). Cell senescence and fibrotic lung diseases. Exp. Gerontol. 132, 110836. doi:10.1016/j.exger.2020.110836
Majidinia, M., Karimian, A., Alemi, F., Yousefi, B., and Safa, A. (2020). Targeting miRNAs by polyphenols: novel therapeutic strategy for aging. Biochem. Pharmacol. 173, 113688. doi:10.1016/j.bcp.2019.113688
Moldogazieva, N. T., Mokhosoev, I. M., Mel’nikova, T. I., Porozov, Y. B., and Terentiev, A. A. (2019). Oxidative stress and advanced lipoxidation and glycation end products (ALEs and AGEs) in aging and age-related diseases. Oxid. Med. Cell. Longev. 2019, 3085756. doi:10.1155/2019/3085756
Parascandolo, A., and Laukkanen, M. O. (2019). Carcinogenesis and reactive oxygen species signaling: interaction of the NADPH oxidase NOX1-5 and superoxide dismutase 1-3 signal transduction pathways. Antioxid. Redox Signal. 30, 443–486. doi:10.1089/ars.2017.7268
Park, J.-H., Burgess, J. D., Faroqi, A. H., Demeo, N. N., Fiesel, F. C., Springer, W., et al. (2020). Alpha-synuclein-induced mitochondrial dysfunction is mediated via a sirtuin 3-dependent pathway. Mol. Neurodegener. 15, 5. doi:10.1186/s13024-019-0349-x
Quan, Y., Park, W., Jin, J., Kim, W., Park, S. K., and Kang, K. P. (2020). Sirtuin 3 activation by honokiol decreases unilateral ureteral obstruction-induced renal inflammation and fibrosis via regulation of mitochondrial dynamics and the renal NF-κB-TGF-β1/Smad signaling pathway. Ijms 21, 402. doi:10.3390/ijms21020402
Rosa, G. D., Brunetti, G., Scuto, M., Salinaro, A. T., Calabrese, E. J., Crea, R., et al. (2020). Healthspan enhancement by olive polyphenols in C. elegans wild type and Parkinson's models. Int. J. Mol. Sci. 21, 3839. doi:10.3390/ijms21113893
Seo, Y., Kingsley, S., Walker, G., Mondoux, M. A., and Tissenbaum, H. A. (2018). Metabolic shift from glycogen to trehalose promotes lifespan and healthspan in Caenorhabditis elegans. Proc. Natl. Acad. Sci. U.S.A. 115, E2791–E2800. doi:10.1073/pnas.1714178115
Shen, P., Kershaw, J. C., Yue, Y., Wang, O., Kim, K.-H., Mcclements, D. J., et al. (2018). Effects of conjugated linoleic acid (CLA) on fat accumulation, activity, and proteomics analysis in Caenorhabditis elegans. Food Chem. 249, 193–201. doi:10.1016/j.foodchem.2018.01.017
Siracusa, R., Scuto, M., Fusco, R., Fusco, R., Trovato, A., Ontario, M. L., et al. (2020). Anti-inflammatory and anti-oxidant activity of hidrox in rotenone-induced Parkinson's disease in mice. Antioxidants 9, 824. doi:10.3390/antiox9090824
Talarowska, M., Filip, M., Szemraj, J., and Gałecki, P. (2019). Does education level protect us from rapid ageing? Sirtuin expression versus age and level of education. Neuro. Endocrinol. Lett. 40, 93–98. doi:10.3389/fphar.2019.01178
Ungvari, Z., Tarantini, S., Nyúl-Tóth, Á., Kiss, T., Yabluchanskiy, A., Csipo, T., et al. (2019). Nrf2 dysfunction and impaired cellular resilience to oxidative stressors in the aged vasculature: from increased cellular senescence to the pathogenesis of age-related vascular diseases. GeroScience 41, 727–738. doi:10.1007/s11357-019-00107-w
Wan, Q.-L., Shi, X., Liu, J., Ding, A.-J., Pu, Y.-Z., Li, Z., et al. (2017). Metabolomic signature associated with reproduction-regulated aging in Caenorhabditis elegans. Aging 9, 447–474. doi:10.18632/aging.101170
Wang, D., Calabrese, E. J., Lian, B., Lin, Z., and Calabrese, V. (2018). Hormesis as a mechanistic approach to understanding herbal treatments in traditional Chinese medicine. Pharmacol. Ther. 184, 42–50. doi:10.1016/j.pharmthera.2017.10.013
Wang, L.-F., Huang, C.-C., Xiao, Y.-F., Guan, X.-H., Wang, X.-N., Cao, Q., et al. (2018). CD38 deficiency protects heart from high fat diet-induced oxidative stress via activating sirt3/FOXO3 pathway. Cell Physiol. Biochem. 48, 2350–2363. doi:10.1159/000492651
Wang, Q., Huang, Y., Qin, C., Liang, M., Mao, X., Li, S., et al. (2016). Bioactive peptides from angelica sinensis protein hydrolyzate delay senescence in Caenorhabditis elegansthrough antioxidant activities. Oxid. Med. Cell. Longev. 2016, 8956981. doi:10.1155/2016/8956981
Wang, S., Zhang, C., Niyazi, S., Zheng, L., Li, J., Zhang, W., et al. (2017). A novel cytoprotective peptide protects mesenchymal stem cells against mitochondrial dysfunction and apoptosis induced by starvation via Nrf2/Sirt3/FoxO3a pathway. J. Transl. Med. 15, 33. doi:10.1186/s12967-017-1144-5
Wang, Y., Branicky, R., Noë, A., and Hekimi, S. (2018). Superoxide dismutases: dual roles in controlling ROS damage and regulating ROS signaling. J. Cell Biol. 217, 1915–1928. doi:10.1083/jcb.201708007
Wert, K. J., Velez, G., Cross, M. R., Wagner, B. A., Teoh-Fitzgerald, M. L., Buettner, G. R., et al. (2018). Extracellular superoxide dismutase (SOD3) regulates oxidative stress at the vitreoretinal interface. Free Radic. Biol. Med. 124, 408–419. doi:10.1016/j.freeradbiomed.2018.06.024
Yang, J., Wan, Q.-L., Mu, Q.-Z., Wu, C.-F., Ding, A.-J., Yang, Z.-L., et al. (2015). The lifespan-promoting effect of otophylloside B in Caenorhabditis elegans. Nat. Prod. Bioprospect. 5, 177–183. doi:10.1007/s13659-015-0064-4
Yang, Z.-Z., Yu, Y.-T., Lin, H.-R., Liao, D.-C., Cui, X.-H., and Wang, H.-B. (2018). Lonicera japonica extends lifespan and healthspan in Caenorhabditis elegans. Free Radic. Biol. Med. 129, 310–322. doi:10.1016/j.freeradbiomed10.1016/j.freeradbiomed.2018.09.035
Yin, S., Zhang, X., Lai, F., Liang, T., Wen, J., Lin, W., et al. (2018). Trilobatin as an HIV-1 entry inhibitor targeting the HIV-1 Gp41 envelope. FEBS Lett. 592, 2361–2377. doi:10.1002/1873-346810.1002/1873-3468.13113
Zhang, C., He, L.-J., Zhu, Y.-B., Fan, Q.-Z., Miao, D.-D., Zhang, S.-P., et al. (2019). Piperlongumine inhibits akt phosphorylation to reverse resistance to cisplatin in human non-small cell lung cancer cells via ROS regulation. Front. Pharmacol. 10, 1178. doi:10.3389/fphar.2019.01178
Zhang, W., Qu, J., Liu, G.-H., and Belmonte, J. C. I. (2020). The ageing epigenome and its rejuvenation. Nat. Rev. Mol. Cell Biol. 21, 137–150. doi:10.1038/s41580-019-0204-5
Keywords: trilobatin, anti-aging, Caenorhabditis elegans, SKN1, SIRT3, DAF16
Citation: Li N, Li X, Shi Y-L, Gao J-M, He Y-Q, Li F, Shi J-S and Gong Q-H (2021) Trilobatin, a Component from Lithocarpus polystachyrus Rehd., Increases Longevity in C. elegans Through Activating SKN1/SIRT3/DAF16 Signaling Pathway. Front. Pharmacol. 12:655045. doi: 10.3389/fphar.2021.655045
Received: 18 January 2021; Accepted: 15 March 2021;
Published: 15 April 2021.
Edited by:
Abdur Rauf, University of Swabi, PakistanReviewed by:
Yunjiao Chen, South China Agricultural University, ChinaVittorio Calabrese, University of Catania, Italy
Anees Ahmed Khalil, University of Lahore, Pakistan
Copyright © 2021 Li, Li, Shi, Gao, He, Li, Shi and Gong. This is an open-access article distributed under the terms of the Creative Commons Attribution License (CC BY). The use, distribution or reproduction in other forums is permitted, provided the original author(s) and the copyright owner(s) are credited and that the original publication in this journal is cited, in accordance with accepted academic practice. No use, distribution or reproduction is permitted which does not comply with these terms.
*Correspondence: Qi-Hai Gong, gqh@zmu.edu.cn