- 1Department of Pharmacy, University of Pisa, Pisa, Italy
- 2Department of Pharmacy, University of Naples “Federico II”, Naples, Italy
The development of GPCR (G-coupled protein receptor) allosteric modulators has attracted increasing interest in the last decades. The use of allosteric modulators in therapy offers several advantages with respect to orthosteric ones, as they can fine-tune the tissue responses to the endogenous agonist. Since the discovery of the first A1 adenosine receptor (AR) allosteric modulator in 1990, several efforts have been made to develop more potent molecules as well as allosteric modulators for all adenosine receptor subtypes. There are four subtypes of AR: A1, A2A, A2B, and A3. Positive allosteric modulators of the A1 AR have been proposed for the cure of pain. A3 positive allosteric modulators are thought to be beneficial during inflammatory processes. More recently, A2A and A2B AR allosteric modulators have also been disclosed. The A2B AR displays the lowest affinity for its endogenous ligand adenosine and is mainly activated as a consequence of tissue damage. The A2B AR activation has been found to play a crucial role in chronic obstructive pulmonary disease, in the protection of the heart from ischemic injury, and in the process of bone formation. In this context, allosteric modulators of the A2B AR may represent pharmacological tools useful to develop new therapeutic agents. Herein, we provide an up-to-date highlight of the recent findings and future perspectives in the field of orthosteric and allosteric A2B AR ligands. Furthermore, we compare the use of orthosteric ligands with positive and negative allosteric modulators for the management of different pathological conditions.
Introduction
G-protein-coupled receptors (GPCRs) are a large family of membrane receptors that mediate the response to several extracellular stimuli. Thus, several efforts have been made to discover molecules acting on GPCRs that represent about one-fourth of marketed drugs approved in 2019 by the FDA (Salmaso and Jacobson, 2020). Among the GPCR family, Adenosine receptors (ARs) are mainly involved in the sensing of tissue damage rather than homeostatic regulators under physiological conditions (Xiao et al., 2019). Four subtypes of ARs (A1, A2A, A2B, and A3) mediate the response to the increase of extracellular adenosine concentrations in response to stressors (Chen et al., 2013). Adenosinergic pathways possess a dual face: on one side, elevated adenosine concentration restores an energy imbalance; on the other side, chronic exposure to high adenosine levels can switch to the promotion of pathological conditions such as uncontrolled inflammation, cytokine release, and fibrosis (Borea et al., 2017).
Particularly, the A2B AR has emerged as a possible therapeutic target in several physio-pathological conditions, including asthma (Cicala and Ialenti, 2013), colitis (Kolachala et al., 2008), cancer (Gao and Jacobson, 2019), cardiovascular, and metabolic disorders (Effendi et al., 2020). However, among all the ARs, the A2B subtype is the least characterized from a pharmacological point of view owing to the lack of X-ray structure and to its low affinity for the prototypic standard ligands commonly used to study ARs. Of note, the four AR subtypes, the A1, A2A, A2B, and A3, share a highly conserved binding (the “orthosteric” one) site of the endogenous agonist adenosine challenging the design of selective agonists. The design and development of positive (PAMs) as well as negative (NAMs) allosteric modulators binding to a less conserved, and topographically distinct site have emerged as an attractive strategy. Allosteric modulators can modulate the effects of the endogenous ligand in its site of production evidencing their ability to be spatially and temporally more selective than the orthosteric ones (Changeux and Christopoulos, 2016; Gregory et al., 2019; Congreve et al., 2020).
Biological Effects of the A2B AR
The coupling of the A2B AR with Gs causes, within the cells, the activation of adenylate cyclase with a consequent increase of cAMP levels. The increase of cAMP concentrations activates the protein kinase A (PKA) and Epac leading to the phosphorylation of cAMP response element-binding protein (CREB) and extracellular signal-regulated kinases (ERK) (Schulte and Fredholm, 2003; Giacomelli et al., 2018). Furthermore, the A2B AR is coupled with Gq11 that mediates the activation of Phospholipase C (PLC) to increase the levels of 1,4,5-inositol triphosphate (IP3)/diacylglycerol (DAG), leading to activation of Protein kinase C (PKC) and increase of Ca2+ levels (Figure 1) (Ciruela et al., 2010).
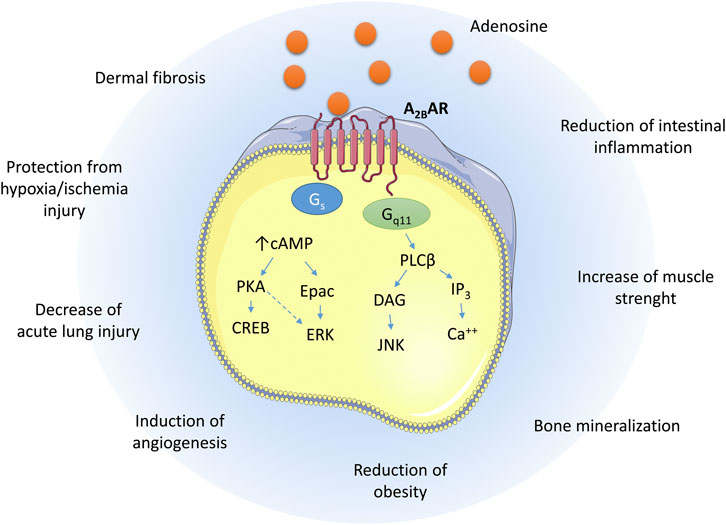
FIGURE 1. Schematic representation of the A2B AR signaling pathways upon adenosine binding and the biological effects exerted in different tissues.
Although there are no A2B AR ligands currently in clinical evaluation, several pre-clinical data have been reported to support their use to treat different conditions such as acute lung injury, ischemia, vascular leakage, metabolic disorders, and bone defects (Jacobson et al., 2019; Carluccio et al., 2020; Gnad et al., 2020) (Table 1). The A2B AR is highly expressed in the respiratory tract and its modulation has been related to the pathogenesis of chronic obstructive pulmonary disease (COPD) and pulmonary fibrosis (Zhong et al., 2005; Cronstein, 2011; Giacomelli et al., 2018). The A2B AR has been proposed as a potential target in acute lung injury (ALI): in fact, the administration of aerosolized BAY-60-6583 1 attenuates pulmonary edema and diminishes lung inflammation (Hoegl et al., 2015). The A2B AR expression has been related to the activation of the hypoxia-inducible factor in different cell types such as endothelial cells (Feoktistov et al., 2004), lung (Eckle et al., 2014), liver cancer cells (Kwon et al., 2019), intestinal epithelial cells (Kong et al., 2006). Its activation has been reported to be useful in the treatment of ischemic injury in different tissues such as the intestines (Hart et al., 2011), heart (Ni et al., 2018) and brain (Coppi et al., 2020).
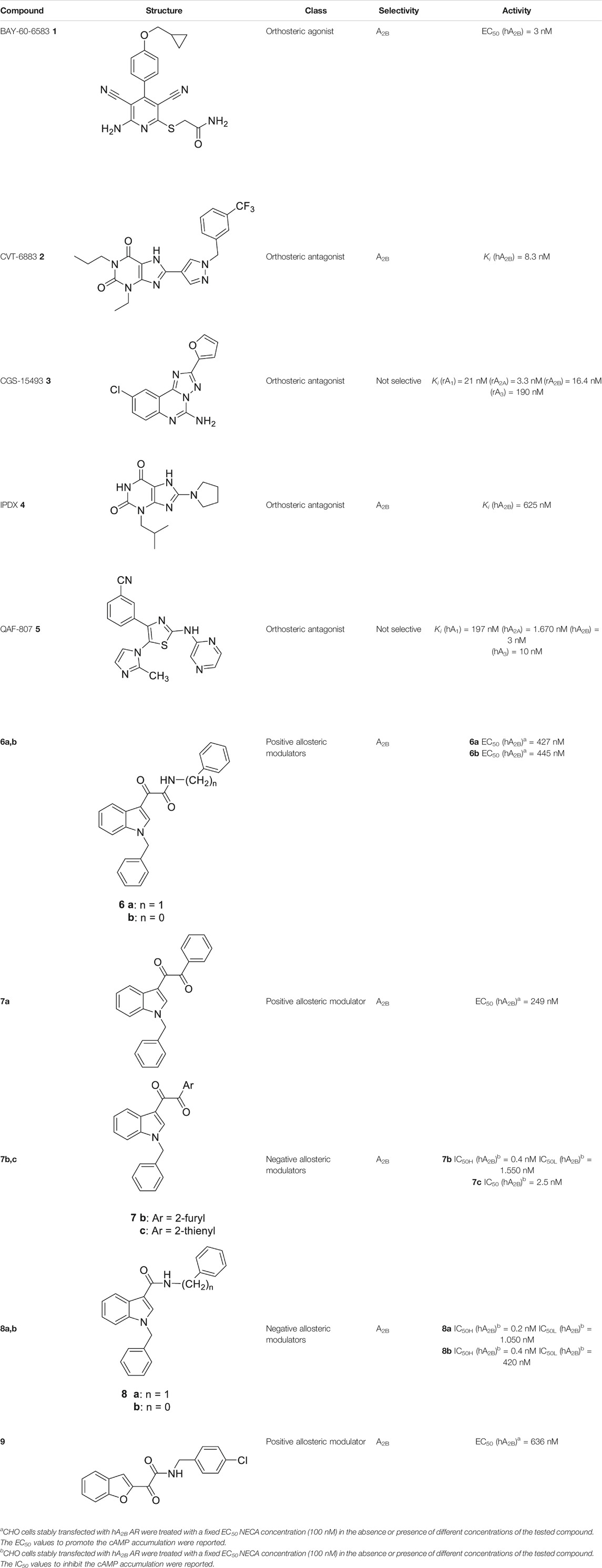
TABLE 1. Representative orthosteric ligands in pre-clinical and clinical trials and allosteric modulators of A2B AR.
In addition to the already known A2B AR activity, the A2B AR has been recently related to physiological processes and pathological conditions opening the way to new possible therapeuthic applications of the A2B AR ligands. In fact, the activity of the A2B AR has been linked to glucose homeostasis and insulin secretion and resistance (Merighi et al., 2015). Accordingly, it has been reported that the A2B AR is abundantly expressed in skeletal muscle (SKM) as well as brown adipose tissue (BAT), and its gene deletion in SKM cells causes sarcopenia, diminishes muscle strength, and reduces energy expenditure (Gnad et al., 2020). Similarly, the adipose tissue specific silencing exacerbates the age-related reduction of BAT. The same authors demonstrated that the receptor stimulation with the A2B AR agonist BAY-60-6583 1 ameliorates obesity (Gnad et al., 2020).
Elevated adenosine concentration is known to exert immunosuppressive action through activation of the ARs mainly by the A2A and A2B subtypes. Interestingly, the use of adenosine as A2A and A2B AR agonist has been proposed and tested as an effective treatment strategy for the recent COVID-19 (Correale et al., 2020; Falcone et al., 2020).
The role of the different ARs in orchestrating the mesenchymal stem cell (MSCs) differentiation has been the focus of several researches (Reviewed in Carluccio et al., 2020). The activation of the A1 AR promotes osteoclast differentiation reducing the MSC-osteoblast differentiation. Conversely, several evidence support the role played by the A2B AR in the adenosine-mediated commitment of MSC into osteoblast differentiation (Gharibi et al., 2011; Carroll et al., 2012; He et al., 2013; Mediero and Cronstein, 2013; Rao et al., 2015; Shih et al., 2019). The receptor activation promotes the expression of the osteogenic factor Runx2 and the phosphatase alkaline (ALP), favoring osteoblastogenesis (Gharibi et al., 2011). Adenosine levels raise up to micromolar concentrations during bone injury, thus triggering the activation of the A2B AR that is highly expressed in MSCs. Furthermore, the inflammation evoked during bone injury further enhances the anabolic responses evoked by A2B AR ligands (Daniele et al., 2017). These data support the role of the A2B AR as an interesting target in the treatment of bone defects such as osteoporosis. Accordingly, the A2B AR stimulation attenuates bone loss in ovariectomized mice, supporting its potential as a target for osteoporosis also consequent to estrogen deficiency (Shih et al., 2019).
Adenosine A2B AR Orthosteric vs Allosteric Ligands
It is beyond the scope of this perspective to review the whole available literature concerning A2B AR ligands. Instead, we have chosen to select a number of relevant (classes of) compounds to 1) offer a reliable overview of those drugs involved in both preclinical and clinical studies, and 2) briefly recapitulate the effects produced by a specific orthosteric or allosteric binding to the A2B AR.
The number of A2B AR agonists developed is the most limited among the ARs agonists, and no agonists for this AR subtype have yet entered clinical trials; nevertheless, some relevant A2B AR agonists have been proposed and subjected to preclinical studies. Among them, the most promising compound, the non-nucleoside agonist BAY-60-6583 1 (Table 1), showed to be useful in the treatment of acute lung injury as well as in cardiovascular diseases, such as atherosclerosis and coronary artery disorders (Eckle et al., 2008; Gao et al., 2014).
Conversely, several highly potent and selective A2B AR antagonists have been reported and some of these had entered in clinical trials in the past decades. CVT-6883 (2, Table 1, also known as GS-601), developed by Gilead along with various other compounds, such as, CGS15493 (3, Table 1, developed by Novartis), WO-00125210 (Bayer HealthCare Pharmaceuticals), IPDX (4, Table 1, Vanderbilt University), and ATL-907 (Adenosine Therapeutics) have entered phase I and II trials for the treatment of asthma (Chandrasekaran et al., 2019; Effendi et al., 2020). In addition, the dual A2B/A3 AR antagonist QAF-807 (5, Table 1, Novartis) had reached phase III clinical trial but it failed to attenuate PC20 AMP challenge as a marker of airway inflammation in mild asthmatic subjects (Wilson, 2008).
The discrepancy between the few drugs entered in clinical practice and the pivotal role of adenosinergic system in several pathological processes can be partially explained by the ubiquitous AR expression in almost all tissues, increasing the possibility of unwanted side effects. In this respect, small-molecules acting as allosteric modulators of the A2B AR might be worthy of investigation as they could represent a potential novel therapeutic strategy for pathological conditions characterized by an altered functionality of this AR subtype.
The first selective PAM, PD81,723, has been reported in 1990 for the A1 AR subtype (Bruns and Fergus, 1990). Since then, many research groups have performed extensive structure-activity relationship studies and reported several PAMs of different AR subtypes (Valant et al., 2012; Jacobson et al., 2018; Deb et al., 2019). Less has been reported regarding A2A and A2B AR allosteric modulators. In 2013, our research group serendipitously has discovered the first class of A2B AR allosteric modulators (Taliani et al., 2013). Specifically, adopting the strategy of designing AR antagonists starting from benzodiazepine receptor (BzR) ligands, the indol-3-ylglyoxylamide scaffold, previously exploited by us to develop BzR ligands (Da Settimo et al., 1996; Da Settimo et al., 1998; Primofiore et al., 2001; Primofiore et al., 2006; Primofiore et al., 2007; Taliani et al., 2009; Cosimelli et al., 2012; Salerno et al., 2012), has been structurally modified, providing a series of 1-benzyl-3-ketoindoles (6a-b, 7a-c, 8a-b, Table 1). These compounds possess two structural features characterizing most of the A2B AR antagonists: three lipophilic moieties linked to a heterocyclic ring and a group capable to establish hydrogen bonds.
No compounds show significant binding affinities toward A1, A2A,, and A3 ARs, except for 7a and 7b, which display moderate A1 AR affinity (sub-micromolar Ki values). Quite surprisingly, the new compounds act as selective human A2B AR modulators in a stably transfected cell line. In particular, 6a,b and 7a behave as PAMs of the A2B AR in functional assay by increasing the efficacy but not the potency of the A2B AR agonists (NECA, BAY 60-6583, adenosine) in stimulating cAMP accumulation. These compounds have been deeply investigated using competitive and kinetic binding experiments resulting in the hypothesis that they favor the receptor active state without altering the orthosteric site. Compounds 7b,c and 8a,b act as NAMs of the A2B AR by decreasing both efficacy and potency of agonists. Similar to the PAMs, the activity of these compounds have been also investigated with binding experiments. They probably interfer with receptor-Gs protein coupling and, consequently, with the agonist functional response favoring the receptor uncoupled state (Trincavelli et al., 2014).
The positive or negative profile of these compounds seems to be correlated to small structural differences. However, the limited number of compounds allowed to delineate only preliminary structure-activity relationships. In brief, compounds 6a, 6b, 8a, and 8b act as PAMs or NAMs depending on the nature of the linker between the indole nucleus and the lipophilic side chain, i.e. glyoxylamide for 6 and carboxamide for 8. Conversely, for compounds 7 the interaction with the protein strictly depends on the nature of the pendant aromatic ring: 7a with a phenyl ring is a PAM while 7b and 7c, featuring a furyl or a thienyl ring, respectively, are NAMs.
Based on the evidence that the A2B AR is the principal AR subtype implicated in MSC differentiation to osteoblasts and bone formation (Gharibi et al., 2011), the A2B AR PAM 6b has been selected for its specificity toward the A2B AR and evaluated for its effect on the agonist-mediated MSC differentiation to osteoblasts. Compound 6b potentiates the effects of either adenosine and synthetic orthosteric A2B AR agonists (NECA and BAY 60-6583 1) in promoting MSC differentiation to osteoblasts in vitro (Trincavelli et al., 2014b) by increasing the osteogenic marker expression and by favoring osteoblast mineralization. In addition, in the early stage of differentiation, 6b potentiates the physiological and A2B agonist-mediated reduction of IL-6 levels that in turn is necessary to support the differentiation process in MSCs. On the contrary, in the late differentiation phase, 6b improves the physiological and A2B agonist-mediated IL-6 increase, important to ensure a pro-survival effect in osteoblasts. These results provide the basis for a prospective therapeutic use of selective A2B AR PAMs in bone diseases.
Very recently, Barresi et al. (2021), to expand the knowledge about the pharmacophoric requirements for A2B AR allosteric modulation, have reported a series of novel derivatives chemically related to 6–8. Compounds from this library exhibit different degrees of similarity with the indoles 6, 7, and 8, including novel indole-based derivatives bearing various substitutions at 1- and/or 3-positions, and derivatives characterized by different aromatic heterocycles in place of indole. Interestingly, structure-activity relationships in terms of matrix mineralization stimulation activity in MSCs (either in the presence or in the absence of the agonist BAY60-6583 1) suggest that the indole nucleus and N1-arylalkyl group do not represent key pharmacophoric elements for a compound acting as A2B AR PAM. In this study compound 9 (Table 1), which possesses a peculiar chemical structure with respect to the reference N1-benzyl substituted indoles 6–8, has been identified to represent a novel lead structure for the development of novel A2B AR PAMs potentially acting as anti-osteoporosis agents.
Discussion
Allosteric modulation is a fundamental mechanism in biology and the development of allosteric ligand on GPCRs is a fast-growing field. Allosteric modulators have the potential to inhibit, activate, or maintain the signaling of the GPCR receptor allowing their modulation based on the physiological requirement without blocking endogenous ligand binding. The research on this field has delivered hundred of candidates in the pipeline and also FDA-approved therapies.
Among the GPCRs, ARs have attracted considerable attention in drug developmemt. During the last fifty decades, several efforts have been made to develop small molecules acting as agonists, antagonists or allosteric enhancers of ARs. Most of these molecules failed in clinical trials and only three are currently approved for human use: adenosine, Regadenoson and Istradefylline. However, increasing evidence demonstrates the biological role played by ARs, particularly by the A2B subtype, in pathological conditions. The A2B AR is expressed under stress conditions in almost all human tissues, and exhibits a low affinity for its endogenous agonist adenosine. Based on the tissue, the nature of the stimuli and the time of exposure, the A2B AR can mediate positive or negative effects. Thus, in the last decade, the research has been focused, on one hand, on a deeper knowledge of the molecular mechanism evoked by the A2B AR activation in different pathological conditions; on the other hand, on the development of small molecules able to selectively bind to this receptor subtype. New A2B AR ligands are currently being developed and the discovery of allosteric modulators represents an attractive research topic. The use of a GPCR orthosteric agonist can always lead to undesired effects due to its activation in other tissues. The limitation of the use of an AR orthosteric agonists and antagonists can not be completely overcome by the use of an allosteric modulator. Of note, the use of an A2B AR agonist can lead to its activation in all the tissue (also where a low amount of adenosine is present) promoting undesired side effects. Conversely, allosteric modulators may represent a more physiologic alternative to orthosteric agonist and antagonist as they promote site-specific and event-specific responses mainly in damaged tissues, where adenosine is massively released. Thus, the development of potent and selective A2B AR PAMs can open the way to a new application of AR ligand in clinical practice likely reducing the side effects with respect to those potentially caused by orthosteric agonists.
Data Availability Statement
The original contributions presented in the study are included in the article/Supplementary Material, further inquiries can be directed to the corresponding authors.
Author Contributions
EB, CG, GG, ST, and MT conceived and wrote the manuscript. EB and CG prepared the images. CM, FS, GG, and MT edited and reviewed the final version of the article. All authors have read and agreed to the published version of the manuscript.
Conflict of Interest
The authors declare that the research was conducted in the absence of any commercial or financial relationships that could be construed as a potential conflict of interest.
References
Barresi, E., Giacomelli, C., Marchetti, L., Baglini, E., Salerno, S., Greco, G., et al. (2021). Novel positive allosteric modulators of A2B adenosine receptor acting as bone mineralisation promoters. J. Enzyme Inhib. Med. Chem. 36, 286–294. doi:10.1080/14756366.2020.1862103
Borea, P. A., Gessi, S., Merighi, S., Vincenzi, F., and Varani, K. (2017). Pathological overproduction: the bad side of adenosine. Br. J. Pharmacol. 174, 1945–1960. doi:10.1111/bph.13763
Bruns, R. F., and Fergus, J. H. (1990). Allosteric enhancement of adenosine A1 receptor binding and function by 2-amino-3-benzoylthiophenes. Mol. Pharmacol. 38, 939–949.
Carluccio, M., Ziberi, S., Zuccarini, M., Giuliani, P., Caciagli, F., Di Iorio, P., et al. (2020). Adult mesenchymal stem cells: is there a role for purine receptors in their osteogenic differentiation? Purinergic Signal. 16, 263–287. doi:10.1007/s11302-020-09703-4
Carroll, S. H., Wigner, N. A., Kulkarni, N., Johnston-Cox, H., Gerstenfeld, L. C., and Ravid, K. (2012). A2B adenosine receptor promotes mesenchymal stem cell differentiation to osteoblasts and bone formation in vivo. J. Biol. Chem. 287, 15718–15727. doi:10.1074/jbc.M112.344994
Chandrasekaran, B., Samarneh, S., Jaber, A. M. Y., Kassab, G., and Agrawal, N. (2019). Therapeutic potentials of A2B adenosine receptor ligands: current status and perspectives. Curr. Pharm. Des. 25, 2741–2771. doi:10.2174/1381612825666190717105834
Changeux, J. P., and Christopoulos, A. (2016). Allosteric modulation as a unifying mechanism for receptor function and regulation. Cell 166, 1084–1102. doi:10.1016/j.cell.2016.08.015
Chen, J. F., Eltzschig, H. K., and Fredholm, B. B. (2013). Adenosine receptors as drug targets—what are the challenges? Nat. Rev. Drug Discov. 12, 265–286. doi:10.1038/nrd3955
Cicala, C., and Ialenti, A. (2013). Adenosine signaling in airways: toward a promising antiasthmatic approach. Eur. J. Pharmacol. 714, 522–525. doi:10.1016/j.ejphar.2013.06.033
Ciruela, F., Albergaria, C., Soriano, A., Cuffí, L., Carbonell, L., Sánchez, S., et al. (2010). Adenosine receptors interacting proteins (ARIPs): behind the biology of adenosine signaling. Biochim. Biophys. Acta 1798, 9–20. doi:10.1016/j.bbamem.2009.10.016
Congreve, M., de Graaf, C., Swain, N. A., and Tate, C. G. (2020). Impact of GPCR structures on drug discovery. Cell 181, 81–91. doi:10.1016/j.cell.2020.03.003
Coppi, E., Dettori, I., Cherchi, F., Bulli, I., Venturini, M., Lana, D., et al. (2020). A2B adenosine receptors: when outsiders may become an attractive target to treat brain ischemia or demyelination. Int. J. Mol. Sci. 21, 9697. doi:10.3390/ijms21249697
Correale, P., Caracciolo, M., Bilotta, F., Conte, M., Cuzzola, M., Falcone, C., et al. (2020). Therapeutic effects of adenosine in high flow 21% oxygen aereosol in patients with Covid19-pneumonia. PLoS One 15, e0239692. doi:10.1371/journal.pone.0239692
Cosimelli, B., Cosconati, S., Marinelli, L., Novellino, E., and Greco, G. (2012). From the pharmacophore to the homology model of the benzodiazepine receptor: the indolyglyoxylamides affair. Curr. Top. Med. Chem. 12, 321–332. doi:10.2174/156802612799078702
Cronstein, B. N. (2011). Adenosine receptors and fibrosis: a translational review. F1000 Biol. Rep. 3, 21. doi:10.3410/B3-21
Da Settimo, A., Primofiore, G., Da Settimo, F., Marini, A. M., Novellino, E., Greco, G., et al. (1998). N’-Phenylindol-3-ylglyoxylohydrazide derivatives: synthesis, structure-activity relationships, molecular modeling studies, and pharmacological action on brain benzodiazepine receptors. J. Med. Chem. 41, 3821–3830. doi:10.1021/jm9800301
Da Settimo, A., Primofiore, G., Da Settimo, F., Marini, A. M., Novellino, E., Greco, G., et al. (1996). Synthesis, structure-activity relationships, and molecular modeling studies of N-(indol-3-ylglyoxylyl)benzylamine derivatives acting at the benzodiazepine receptor. J. Med. Chem. 39, 5083–5091. doi:10.1021/jm960240i
Daniele, S., Natali, L., Giacomelli, C., Campiglia, P., Novellino, E., Martini, C., et al. (2017). Osteogenesis is improved by low tumor necrosis factor alpha concentration through the modulation of gs-coupled receptor signals. Mol. Cell Biol. 37, e00442–16. doi:10.1128/MCB.00442-16
Deb, P. K., Deka, S., Borah, P., Abed, S. N., and Klotz, K. N. (2019). Medicinal chemistry and therapeutic potential of agonists, antagonists and allosteric modulators of A1 adenosine receptor: current status and perspectives. Curr. Pharm. Des. 25, 2697–2715. doi:10.2174/1381612825666190716100509
Eckle, T., Grenz, A., Laucher, S., and Eltzschig, H. K. (2008). A2B adenosine receptor signaling attenuates acute lung injury by enhancing alveolar fluid clearance in mice. J. Clin. Invest. 118, 3301–3315. doi:10.1172/JCI34203
Eckle, T., Kewley, E. M., Brodsky, K. S., Tak, E., Bonney, S., Gobel, M., et al. (2014). Identification of hypoxia-inducible factor HIF-1A as transcriptional regulator of the A2B adenosine receptor during acute lung injury. J. Immunol. 192, 1249–1256. doi:10.4049/jimmunol.1100593
Effendi, W. I., Nagano, T., Kobayashi, K., and Nishimura, Y. (2020). Focusing on adenosine receptors as a potential targeted therapy in human diseases. Cells 9, 785. doi:10.3390/cells9030785
Falcone, C., Caracciolo, M., Correale, P., Macheda, S., Vadalà, E. G., La Scala, S., et al. (2020). Can adenosine fight COVID-19 acute respiratory distress syndrome? J. Clin. Med. 9, 3045. doi:10.3390/jcm9093045
Feoktistov, I., Ryzhov, S., Zhong, H., Goldstein, A. E., Matafonov, A., Zeng, D., et al. (2004). Hypoxia modulates adenosine receptors in human endothelial and smooth muscle cells toward an A2B angiogenic phenotype. Hypertension 44, 649–654. doi:10.1161/01.HYP.0000144800.21037.a5
Gao, Z.-G., and Jacobson, K. A. (2019). A2B adenosine receptor and cancer. Int. J. Mol. Sci. 20, 5139. doi:10.3390/ijms20205139
Gao, Z. G., Balasubramanian, R., Kiselev, E., Wei, Q., and Jacobson, K. A. (2014). Probing biased/partial agonism at the G protein-coupled A2B adenosine receptor. Biochem. Pharmacol. 90, 297–306. doi:10.1016/j.bcp.2014.05.008
Gharibi, B., Abraham, A. A., Ham, J., and Evans, B. A. (2011). Adenosine receptor subtype expression and activation influence the differentiation of mesenchymal stem cells to osteoblasts and adipocytes. J. Bone Miner. Res. 26, 2112–2124. doi:10.1002/jbmr.424
Giacomelli, C., Daniele, S., Romei, C., Tavanti, L., Neri, T., Piano, I., et al. (2018). The A2B adenosine receptor modulates the epithelial- mesenchymal transition through the balance of cAMP/PKA and MAPK/ERK pathway activation in human epithelial lung cells. Front. Pharmacol. 9, 54. doi:10.3389/fphar.2018.00054
Gnad, T., Navarro, G., Lahesmaa, M., Reverte-Salisa, L., Copperi, F., Cordomi, A., et al. (2020). Adenosine/A2B receptor signaling ameliorates the effects of aging and counteracts obesity. Cell Metab. 32, 56–70.e7. doi:10.1016/j.cmet.2020.06.006
Gregory, K. J., Bridges, T. M., Gogliotti, R. G., Stauffer, S. R., Noetzel, M. J., Jones, C. K., et al. (2019). In Vitro to in Vivo translation of allosteric modulator concentration-effect relationships: implications for drug discovery. ACS Pharmacol. Transl. Sci. 2, 442–452. doi:10.1021/acsptsci.9b00062
Hart, M. L., Grenz, A., Gorzolla, I. C., Schittenhelm, J., Dalton, J. H., and Eltzschig, H. K. (2011). Hypoxia-inducible factor-1α-dependent protection from intestinal ischemia/reperfusion injury involves ecto-5’-nucleotidase (CD73) and the A2B adenosine receptor. J. Immunol. 186, 4367–4374. doi:10.4049/jimmunol.0903617
He, W., Mazumder, A., Wilder, T., and Cronstein, B. N. (2013). Adenosine regulates bone metabolism via A1, A2A, and A2B receptors in bone marrow cells from normal humans and patients with multiple myeloma. FASEB J. 27, 3446–3454. doi:10.1096/fj.13-231233
Hoegl, S., Brodsky, K. S., Blackburn, M. R., Karmouty-Quintana, H., Zwissler, B., and Eltzschig, H. K. (2015). Alveolar epithelial A2B adenosine receptors in pulmonary protection during acute lung injury. J. Immunol. 195, 1815–1824. doi:10.4049/jimmunol.1401957
Jacobson, K. A., Merighi, S., Varani, K., Borea, P. A., Baraldi, S., Aghazadeh Tabrizi, M., et al. (2018). A3 adenosine receptors as modulators of inflammation: from medicinal chemistry to therapy. Med. Res. Rev. 38, 1031–1072. doi:10.1002/med.21456
Jacobson, K. A., Tosh, D. K., Jain, S., and Gao, Z. G. (2019). Historical and current adenosine receptor agonists in preclinical and clinical development. Front. Cell Neurosci. 13, 124. doi:10.3389/fncel.2019.00124
Kolachala, V., Ruble, B., Vijay-Kumar, M., Wang, L., Mwangi, S., Figler, H., et al. (2008). Blockade of adenosine A2B receptors ameliorates murine colitis. Br. J. Pharmacol. 155, 127–137. doi:10.1038/bjp.2008.227
Kong, T., Westerman, K. A., Faigle, M., Eltzschig, H. K., and Colgan, S. P. (2006). HIF-dependent induction of adenosine A2B receptor in hypoxia. FASEB J. 20, 2242–2250. doi:10.1096/fj.06-641910.1096/fj.06-6419com
Kwon, J. H., Lee, J., Kim, J., Jo, Y. H., Kirchner, V. A., Kim, N., et al. (2019). HIF-1α regulates A2B adenosine receptor expression in liver cancer cells. Exp. Ther. Med. 18, 4231–4240. doi:10.3892/etm.2019.8081
Mediero, A., and Cronstein, B. N. (2013). Adenosine and bone metabolism. Trends Endocrinol. Metab. 24, 290–300. doi:10.1016/j.tem.2013.02.001
Merighi, S., Borea, P. A., and Gessi, S. (2015). Adenosine receptors and diabetes: focus on the A2B adenosine receptor subtype. Pharmacol. Res. 99, 229–236. doi:10.1016/j.phrs.2015.06.015
Ni, Y., Liang, D., Tian, Y., Kron, I. L., French, B. A., and Yang, Z. (2018). Infarct-sparing effect of adenosine A2B receptor agonist is primarily due to its action on splenic leukocytes via a PI3K/Akt/IL-10 pathway. J. Surg. Res. 232, 442–449. doi:10.1016/j.jss.2018.06.042
Primofiore, G., Da Settimo, F., Marini, A. M., Taliani, S., La Motta, C., Simorini, F., et al. (2006). Refinement of the benzodiazepine receptor site topology by structure-activity relationships of new N-(heteroarylmethyl)indol-3-ylglyoxylamides. J. Med. Chem. 49, 2489–2495. doi:10.1021/jm0511841
Primofiore, G., Settimo, F., Taliani, S., Marini, A. M., Novellino, E., Greco, G., et al. (2001). Novel N-(arylalkyl)indol-3-ylglyoxylylamides targeted as ligands of the benzodiazepine receptor: synthesis, biological evaluation, and molecular modeling analysis of the structure-activity relationships. J. Med. Chem. 44, 2286–2297. doi:10.1021/jm010827j
Primofiore, G., Taliani, S., Da Settimo, F., Marini, A. M., La Motta, C., Simorini, F., et al. (2007). Novel N-substituted indol-3-ylglyoxylamides probing the LDi and L1/L2 lipophilic regions of the benzodiazepine receptor site in search for subtype-selective ligands. J. Med. Chem. 50, 1627–1634. doi:10.1021/jm0607707
Rao, V., Shih, Y. R., Kang, H., Kabra, H., and Varghese, S. (2015). Adenosine signaling mediates osteogenic differentiation of human embryonic stem cells on mineralized matrices. Front. Bioeng. Biotechnol. 3, 185. doi:10.3389/fbioe.2015.00185
Salerno, S., Da Settimo, F., Taliani, S., Simorini, F., La Motta, C., Fornaciari, G., et al. (2012). Medicinal chemistry of indolylglyoxylamide GABAA/BzR high affinity ligands: identification of novel anxiolytic/non sedative agents. Curr. Top. Med. Chem. 12, 286–311. doi:10.2174/156802612799078847
Salmaso, V., and Jacobson, K. A. (2020). Purinergic signaling: impact of GPCR structures on rational drug design. ChemMedChem 15, 1958–1973. doi:10.1002/cmdc.202000465
Schulte, G., and Fredholm, B. B. (2003). The G(s)-coupled adenosine A2B receptor recruits divergent pathways to regulate ERK1/2 and p38. Exp. Cell Res. 290, 168–176. doi:10.1016/s0014-4827(03)00324-0
Shih, Y. V., Liu, M., Kwon, S. K., Iida, M., Gong, Y., Sangaj, N., et al. (2019). Dysregulation of ectonucleotidase-mediated extracellular adenosine during postmenopausal bone loss. Sci. Adv. 5, eaax1387. doi:10.1126/sciadv.aax1387
Taliani, S., Cosimelli, B., Da Settimo, F., Marini, A. M., La Motta, C., Simorini, F., et al. (2009). Identification of anxiolytic/nonsedative agents among indol-3-ylglyoxylamides acting as functionally selective agonists at the gamma-aminobutyric acid-A (GABAA) alpha2 benzodiazepine receptor. J. Med. Chem. 52, 3723–3734. doi:10.1021/jm9001154
Taliani, S., Trincavelli, M. L., Cosimelli, B., Laneri, S., Severi, E., Barresi, E., et al. (2013). Modulation of A2B adenosine receptor by 1-Benzyl-3-ketoindole derivatives. Eur. J. Med. Chem. 69, 331–337. doi:10.1016/j.ejmech.2013.09.001
Trincavelli, M. L., Daniele, S., Giacomelli, C., Taliani, S., Da Settimo, F., Cosimelli, B., et al. (2014b). Osteoblast differentiation and survival: a role for A2B adenosine receptor allosteric modulators. Biochim. Biophys. Acta 1843, 2957–2966. doi:10.1016/j.bbamcr.2014.09.013
Trincavelli, M. L., Giacomelli, C., Daniele, S., Taliani, S., Cosimelli, B., Laneri, S., et al. (2014). Allosteric modulators of human A2B adenosine receptor. Biochim. Biophys. Acta 1840, 1194–1203. doi:10.1016/j.bbagen.2013.12.021
Valant, C., Aurelio, L., Devine, S. M., Ashton, T. D., White, J. M., Sexton, P. M., et al. (2012). Synthesis and characterization of novel 2-amino-3-benzoylthiophene derivatives as biased allosteric agonists and modulators of the adenosine A1 receptor. J. Med. Chem. 55, 2367–2375. doi:10.1021/jm201600e
Wilson, C. N. (2008). Adenosine receptors and asthma in humans. Br. J. Pharmacol. 155, 475–486. doi:10.1038/bjp.2008.361
Xiao, C., Liu, N., Jacobson, K. A., Gavrilova, O., and Reitman, M. L. (2019). Physiology and effects of nucleosides in mice lacking all four adenosine receptors. PLoS Biol. 17, e3000161. doi:10.1371/journal.pbio.3000161
Keywords: adenosine receptors, allosteric modulators, A2B receptor, mesenchymal stromal cells, bone healing
Citation: Barresi E, Martini C, Da Settimo F, Greco G, Taliani S, Giacomelli C and Trincavelli ML (2021) Allosterism vs. Orthosterism: Recent Findings and Future Perspectives on A2B AR Physio-Pathological Implications. Front. Pharmacol. 12:652121. doi: 10.3389/fphar.2021.652121
Received: 11 January 2021; Accepted: 12 February 2021;
Published: 24 March 2021.
Edited by:
Francesco Caciagli, University of Studies G.d’Annunzio Chieti and Pescara, ItalyReviewed by:
Loredana Cappellacci, University of Camerino, ItalyCeline Valant, Monash University, Parkville campus, Australia
Copyright © 2021 Barresi, Martini, Da Settimo, Greco, Taliani, Giacomelli and Trincavelli. This is an open-access article distributed under the terms of the Creative Commons Attribution License (CC BY). The use, distribution or reproduction in other forums is permitted, provided the original author(s) and the copyright owner(s) are credited and that the original publication in this journal is cited, in accordance with accepted academic practice. No use, distribution or reproduction is permitted which does not comply with these terms.
*Correspondence: Sabrina Taliani, c2FicmluYS50YWxpYW5pQHVuaXBpLml0; Chiara Giacomelli, Y2hpYXJhLmdpYWNvbWVsbGlAdW5pcGkuaXQ=