- 1Agricultural Bio-pharmaceutical Laboratory, Qingdao Agricultural University, Qingdao, China
- 2College of Veterinary Medicine, Xinjiang Agricultural University, Urumqi, China
- 3National Centre for Veterinary Drug Safety Evaluation, College of Veterinary Medicine, China Agricultural University, Beijing, China
Background: Klebsiella pneumonia has emerged as a critical pathogen causing severe clinical problems, such as pneumonia and sepsis. Meanwhile, intensified drug resistance induced by antibiotic therapy necessitates discovering novel and active molecules from Traditional Chinese Medicine (TCM) for treatment.
Methods and results: In this study, the isolated Bergenin monohydrate showed an anti-inflammatory effect in Klebsiella-infected mice. We initially investigated the anti-inflammatory effects and cytoprotection against oxidative stress in vitro and in vivo. Interestingly, a specific dose of Bm can effectively ameliorate lung injury and suppress the expression of inflammatory cytokines such as TNF-α, IL-6, IL-1β and PEG2. Moreover, Bm was also shown to reduced the levels of MPO, MDA and increased SOD and GSH activities. Moreover, we assessed the intracellular signaling molecules including p38, ERK, JNK, IκB, NF-κB-p65 by western blotting and verified through MAPK and NF-κB pathways inhibition experiments. These results reveal that Bm executed its effects via the classical MAPK signaling pathway and NF-κB pathway.
Conclusion: Given its underlying anti-inflammatory effect, Bm may be used as a promising therapeutic against Klebsiella-induced infection, thus providing a benefit for the future clinical therapy of pneumonia and medicine design.
Introduction
Klebsiella pneumoniae (Kp), a nonmotile, facultatively anaerobic, gram-negative, rod-shaped bacterium, can cause acute lung injury in humans and is considered an urgent health concern (Peteranderl et al., 2017; Zhang et al., 2018). For adults in which Kp was the primary isolate, it caused a mortality rate of up to 50% in respiratory tract infections, with only 52% survival (Zhang et al., 2018; Choby et al., 2020). Currently, antibiotic administration remains a major strategy for treating Kp infections in the clinic (Bengoechea and Sa Pessoa, 2019). However, Kp has increasingly survived antibiotic treatment due to resistance or tolerance to the drugs. (Chew et al., 2017; Hossan et al., 2018; Chang et al., 2020). Hence, ample research space exists for the development of an efficient treatment to control Kp infection (Turan et al., 2018; Yang et al., 2018; Avgerinos et al., 2020).
Traditional Chinese medicine (TCM) has thousands of years of history in Asian countries. TCM studies have contributed to pharmacodynamic and pharmacokinetic development by generations of practitioners and researchers (Poivre and Duez, 2017; Chan and Ng, 2020). To date, several Chinese medicinal monomers extracted from traditional Chinese medicinal herbs have been widely used to treat inflammatory diseases of the lungs (Fan et al., 2018). For example, xanthohumol was reported to alleviate acute lung bacterial infection by adjusting the AMPK/GSK3β-Nrf2 signaling pathways (Lv et al., 2017a). At the same time, apigenin displayed protective effects in mice with pneumococcal pneumonia through pneumolysin cytolytic activity (Song et al., 2016). Likewise, daphnetin exhibited protective activity against t-BHP-induced oxidative injury and mitochondrial dysfunction by upregulating the Nrf2 antioxidant signaling pathway and activating JNK and ERK (Lv et al., 2017b). A previous study reported that Adenostemma Lavenia extract displayed a strong alleviating effect on pulmonary congestion, pneumonia, bacterial infections of the respiratory tract, edema, and inflammation (Batubara et al., 2020). In 2020, another study revealed that another extract of traditional Chinese medicine, baicalein, shows a significant effect against drug-resistant S. aureus infections by inhibiting the coagulase activity of vWbp and thus might be a promising therapeutic drug for bacterial pneumonia (Zhang et al., 2020). Notably, most bacteria rarely generate drug resistance to traditional Chinese medicine due to the unique and special properties of the therapeutic approach (Liao et al., 2018; Chan and Ng, 2020). This new perspective of investigating monomers from TCM has already contributed to modern medicine and has become a promising area of further research (Wang et al., 2018; Newman, 2020).
Over the last few years, our laboratory has set out to screen effective TCM molecules to treat Kp infection. Intriguingly, we found that Bergenin monohydrate (Bm) has multiple biological activities, suggesting its anti-inflammatory and immunomodulatory properties. Here, we confirmed that Bm exhibits a protective effect against Kp-induced cell damage and was effective in Kp-induced mice. In addition, we uncovered the involvement of the NF-κB and MAPK signaling pathways in the activity of Bm. This study might provide a potential reference for exploring therapeutic candidates derived from Chinese medicinal herbs to treat Kp infection.
Materials and Methods
Bacterial Strain, Chemicals and Animals
The Kp strain was purchased from the China Center of Industrial Culture Collection (CICC-10870). Bergenin monohydrate was obtained from Chengdu Herbpurify Co. Ltd., Sichuan, China, with a certified purity of ≥98. RAW 264.7 cells and WI-38 cells were obtained from our laboratory, and MEM and DMEM were provided by Procell Life Science and Technology Co. Ltd., Wuhan, China. The assay kits and instructions were purchased from Jiancheng Bioengineering Institute, Nanjing, China. The NF-κB pathway inhibitor (BAY 11-7082) and MAPK inhibitor (SB203580) were purchased from Sigma (Sigma-Aldrich). IκB, p-IκB, p68, p-p65, ERK1/2, p-ERK1/2, p65, p-p65, JNK, and p-JNK antibodies (rabbit antibodies) for western blot analysis were purchased from Beijing Boaosen Biotechnology Co., Ltd. Secondary antibodies (IgG-HRP) were obtained from Zhongshan Jinqiao Biological Technology Co., Ltd. Unless otherwise specified, the other materials were from Sigma-Aldrich (United States).
A total of 90 six-week-old BALB/c mice weighing 18–20 g were used in this study. All mice were fed ad libitum, kept in a pathogen-free environment, and maintained in groups of 5 per cage in a specific pathogen-free (SPF) animal room. All animal experiments, including any relevant details, were performed under the applicable guidelines and regulations of Experimental Animal Care and were approved by the Animal Welfare and Research Ethics Committee (Approval Number: SYXK (SD) 20170005).
Determination of Bacterial Growth Curve and Preparation of Bacteria
The methods for bacterial growth and preparation were described in a previous study by our lab (Sun et al., 2019). Kp was strictly cultured in the microbial operation room following operating principles and cultured with graded Bm concentrations (0, 2.5, 5, 10 and 20 μg/ml) to the postexponential phase at 5% CO2, pH 7.0–7.4 and 37°C. Bacterial supernatant was collected for MTT, ROS. The rest of the Kp bacterial sample was utilized for cells experiments and animal experiment.
MIC Determination Assay
Double-gradient dilution was applied to obtain a series of Bm solution concentrations, which were then sequentially added to the wells of a 96-well culture plate (Liao et al., 2018). The Kp broth was cultured to logarithmic growth phase and diluted to 1 × 107 CFU/mL, and100 μL drops of the dilution were added to each well. Following incubation at 5% CO2, pH 7.0–7.4, and 37°C for 24 h, we observed the turbidity of the broth. Transparency of the samples indicated that bacterial growth was inhibited at the corresponding concentration of the drug, and the lowest drug concentration that could inhibit bacterial growth was obtained. This concentration was considered the minimum inhibitory concentration (MIC).
Cell Culture
After incubation in Dulbecco’s modified Eagle’s medium (DMEM) in 5% CO2 at 37°C, RAW 264.7 cells were cultured and adjusted to 2 × 104 cells/well, seeded into 96-well plates and incubated for 24 h. Bm was dissolved in DMEM. No significant treatment effect of Bm was found for Kp-infected RAW 264.7 cells in our experiments. Thus, RAW 264.7 cells were first administered Bm at different concentrations (final concentrations of 0, 2.5, 5, 10 and 20 μg/ml) and a positive control drug (dexamethasone, 100 μg/ml) for 1 h and then exposed to KP for 24 h.
Measurement of ROS Production
Cultured RAW 264.7 cells were adjusted to 2 × 108 cells/well and incubated in 6-well plates for 24 h. After that, the cells were treated with Bm at different concentrations (0, 2.5, 5, 10 and 20 μg/ml) in DMEM for 1 h. Then, the cells were infected with Kp for 24 h and washed twice with PBS. Subsequently, 50 μL DCFH-DA was added to the cells for 30 min without light. DCF fluorescence intensities were detected spectrophotometrically at excitation and emission wavelengths of 485 and 535 nm, respectively.
Animal Experiment and Pathological Indexes
Ninety BALB/c mice were kept under SPF conditions and randomly divided into six groups:control group (saline only, 0.2 ml), Kp group (1 × 107 CFU, 0.2 ml), Kp + Bm (75, 150 or 300 μg/kg, 0.2 ml) groups, Kp + DEX (100 μg/ml, 0.2 ml) group, Bm was diluted in saline. In the mouse model of Kp lung infection, Kp was administered intranasally, and the mice were treated with Bm via intraperitoneal injection 1 h before Kp infection. After the experiments, all the mice were sacrificed via carbon dioxide asphyxiation. Afterward, lung tissues were rinsed and fixed for further analysis, bronchoalveolar lavage fluid (BALF) was prepared for the following experiments. All vitro experiments were repeated at least three times, and all animal experiments were repeated twice for under identical conditions.
After euthanasia of mice by CO2 asphyxiation, the same lung tissues and BALF were collected as described in previous work (Liao et al., 2018). The levels of the representative inflammatory cytokines TNF-α, IL-6, IL-1β and PEG2 in BALF were then determined using specific ELISA kits.
Right lung tissues were excised from each mouse 48 h after Kp treatment. Then, we homogenized the lung tissues and dissolved them in extraction buffer for analysis of MPO, MDA, SOD and GSH contents. MPO and MDA contents were measured using assay kits to determine neutrophil accumulation and lipid peroxidation in lung tissues. BALF samples were centrifuged for 10 min at 4°C. The supernatant was then discarded, and cell pellets were resuspended in PBS. Finally, cells were stained using the Wright-Giemsa method, and the number of neutrophils, macrophages and total cells was determined. Lung tissue samples were homogenized in 10 ml normal saline. The homogenate was centrifuged at 2,500× g for 10 min, and the supernatant was collected. Counts of Kp organisms were obtained after plating serial dilutions of lung homogenates on Mueller-Hinton agar. After 24 h of incubation at 5% CO2 and 37°C, the colonies were counted and recorded.
Histopathological Evaluation
Left lung tissues were excised 48 h after Kp infection, and histopathological examination was performed. Briefly, lung tissues from the mice were immersed in standard 10% neutral buffered formalin, dissected into 5 μm-thick sections, fixed, and then visualized via hematoxylin and eosin (H&E) staining. Images were acquired under a light microscope (Olympus, Japan). Comparison of the staining results was performed by research team members who were blinded to the grouping information.
Western Blotting
Harvesting of protein lysates from RAW 264.7 cells and mouse lung tissues was performed according to the present study. Then, the same quantity of proteins (30 µg/lane) were subjected to electrophoresis in a 12% polyacrylamide gel, transferred to a PVDF membrane, and blocked with 5% FBS at room temperature for 1 h. The blots were then separately incubated with primary murine anti-human β-actin (1:5,000), IκB (1:1,000), phospho-IκB (1:1,000), p68 (1:1,000), phospho-p68 (1:1,000), p65 (1:1,000), phospho-p65 (1:1,000), ERK1/2 (1:1,000), and phospho-ERK1/2 (1:1,000) antibodies overnight at 4°C. β-Actin was used as an endogenous control and for normalization. The membranes were washed with TBST for 10 min and then incubated with anti-mouse IgG secondary antibody (1:100) for 30 min at room temperature. Finally, the membranes were visualized using an enhanced chemiluminescence (ECL) agent.
Verification of Pathway Inhibition in WI 38 Cells
To verify that Bm attenuates the inflammatory response via the MAPK and NF-κB pathways in WI 38 cells, we cultured WI 38 cells in MEM containing 10% FBS, 1% P/S and 1% GlutaMax in an atmosphere of 95% air and 5% CO2. When the density of the WI 38 cells increased to 80%, we seeded them into 96-well plates at 1 × 105 cells/well, incubated them for 24 h, and added SB203580 and BAY 11–7,082 at two different doses (10 and 20 μmol/L). After 1 h, 10 μg/ml Bm was added and incubated with the cells for another 1 h, and then, the plate was exposed to Kp for 24 h. The cytotoxic effects of these two pathway inhibitors were determined.
Statistical Analysis
All the data were collected and analyzed using SPSS 22.0 software (IBM). The experimental groups were compared via one-way analysis of variance (ANOVA), and an LSD test was applied for multiple comparisons. In the western blotting analysis, densitometry analyses were quantified with ImageJ software. Statistical significance was defined as “*” for p < 0.05 and “**” for p < 0.01.
Results
Bergenin Monohydrate Rescues Cell Growth and ROS Production in Klebsiella pneumonia-Infected Cells
The MIC of Bm was greater than 256 μg/ml, which indicated that Bm had no considerable effect on Kp growth (Supplementary Figure S1). In addition, growth curve assays (Figure 1A) showed no apparent difference between the groups, suggesting that Bm did not directly inhibit Kp growth. The results of MTT assays to measure cell viability indicated that 2.5, 5, 10 and 20 μg/ml Bm had no toxicity toward RAW 264.7 cells. (Figure 1B). Moreover, Kp significantly reduced cell viability, confirming successful modeling.
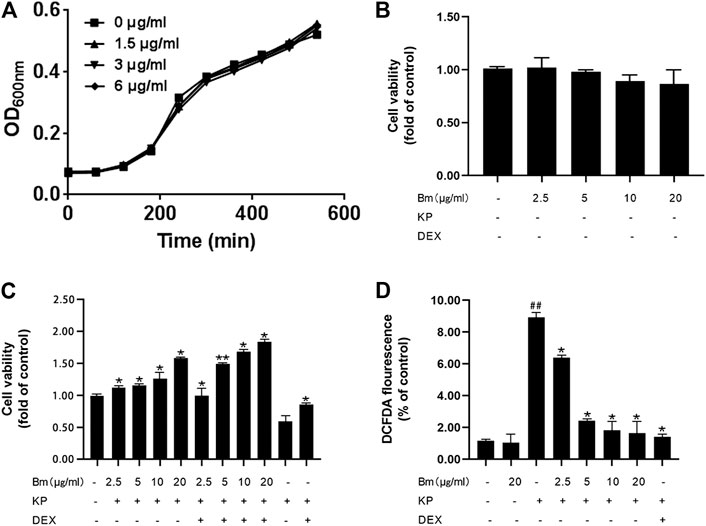
FIGURE 1. Effect of Bergenin monohydrate on Klebsiella pneumonia-infected RAW264.7 cell viability and ROS production (A) Viable cell count growth curves (B) Cell viability without Klebsiella pneumonia infection (C) Cell viability with both Klebsiella pneumonia infection and Bergenin monohydrate protection (D) ROS production with both Klebsiella pneumonia infection and Bergenin monohydrate protection. All data are presented as the means ± SEM (n = 5). ##p< 0.01 vs the Control group; *p < 0.05 and **p < 0.01 vs the Klebsiella pneumonia group.
Furthermore, Bm protected RAW 264.7 cells from Kp infection (Figure 1C), and the protective efficacy increased with increasing Bm concentration. In addition, Bm enhanced the dexamethasone effect, which was most pronounced at 20 μg/ml. DCF fluorescence intensities were detected with a multidetection reader to calculate the percentage of DCF fluorescence in the control (Figure 1D). As indicated, the Kp-infected RAW 264.7 cells displayed higher ROS production than the Bm groups and the blank control group (p < 0.01). Notably, the ROS production in RAW 264.7 cells reached nearly the same level as that in the blank group at 20 μg/ml Bm, which was lower than that in the dexamethasone treatment group and even lower than that in the dexamethasone combined with Bm treatment group.
Bergenin Monohydrate Treatment Significantly Decreased the Klebsiella pneumonia Content
Histological changes in lung tissues were assessed by H&E staining (Figure 2A). There were no signs of structural damage in the lung tissue after 300 μg/kg Bm treatment (Figures 2A,b). The Kp group (Figures 2A,c) had the thickest alveolar walls, red blood cells were found in the alveolar cavity, and the capillary walls were distinctly expanded. Compared with the Kp group, the Bm-treated groups (Figures 2A,e,f) showed effectively alleviated lung tissue injury, including less accumulation of inflammatory cells, thinner alveolar walls and reduced alveolar hemorrhage. All changes occurred in a dose-dependent manner. In particular, the lung tissue status in the high-dose Bm treatment group (Figures 2A,f) was similar to that in the dexamethasone group (Figures 2A,g) and tended to be close to that in the blank group. Edema status was also evaluated by examining pulmonary bacterial content in lung tissues. As shown in Figure 2B, the Kp content in the Kp group was substantially higher than in the other groups, while groups pretreated with Bm at 75, 150, and 300 μg/kg showed reduced Kp content in the lungs by 4.76, 4.48, and 4.32 L g CFU/g, respectively. These findings indicate that Bm had significant effects in lowering the amount of Kp bacteria within the lungs.
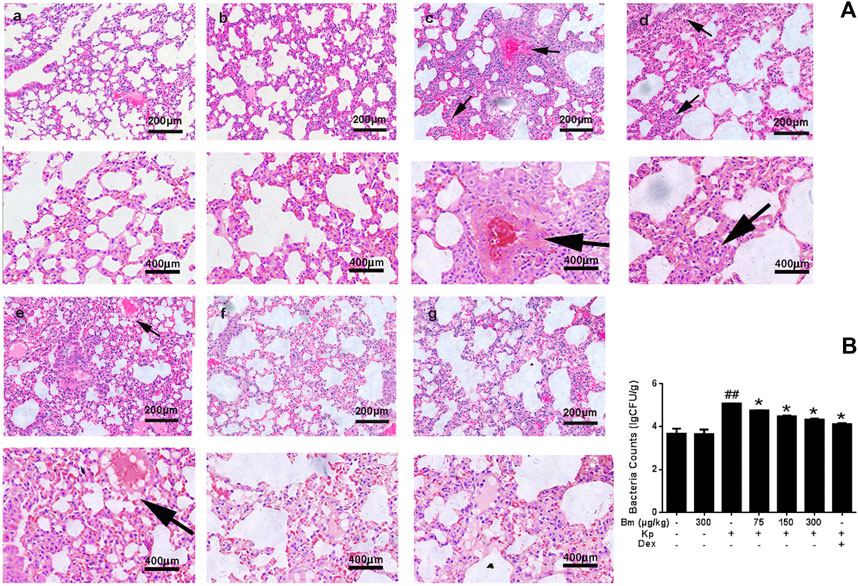
FIGURE 2. Characterization of lungs from the mouse model (A) Lung tissue structure was observed with H&E staining: a) blank control group, b) Bergenin monohydrate treatment group (300 μg/kg), c) Klebsiella pneumonia-infected group, e-f) Klebsiella pneumonia-infected mice treated with Bergenin monohydrate (75, 150, 300 μg/kg), g) Dex group (black arrows: pathological changes) (B) Lung bacterial content changes after Klebsiella pneumonia infection. ##p < 0.01 vs the Control group; *p < 0.05 and **p < 0.01 vs the Klebsiella pneumonia group; $p < 0.05 vs the Dex group.
ELISA measurement of TNF-α, IL-6, IL-1β and PEG2 contents after Kp treatment for 48 h.
As indicated in Figures 3A-D, TNF-α, IL-6, IL-1β and PEG2 contents in BALF were significantly increased after Kp administration compared with the control group. After treatment with Bm (75, 150, and 300 μg/kg), TNF-α, IL-6, IL-1β, and PEG2 levels in Kp-induced pneumonia mice exhibited a downward trend in a dose-dependent manner.
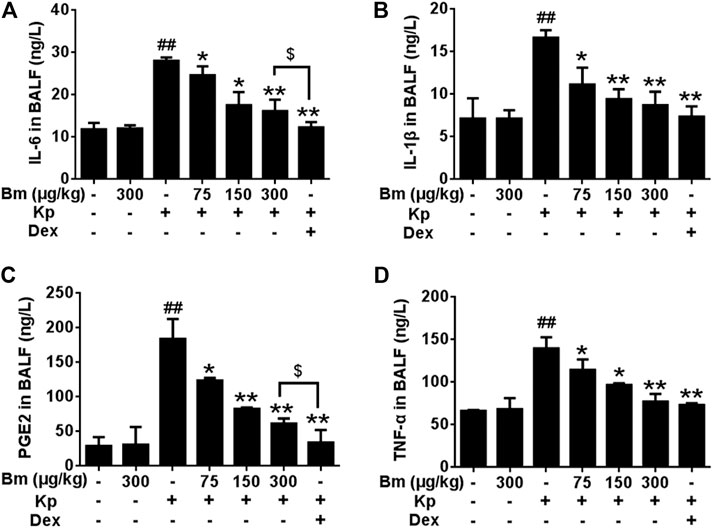
FIGURE 3. Effects of Bergenin monohydrate on IL-1β (A), IL-6 (B), PEG2 (C) and TNF-α (D) production and secretion in BALF from Klebsiella pneumonia-induced pneumonia mice. All data are presented as the means ± SEM (n = 5). ##p < 0.01 vs the Control group; *p < 0.05 and **p < 0.01 vs the Klebsiella pneumonia group; $p < 0.05 vs the Dex group.
Effects of Bergenin Monohydrate on Cell Counts in Klebsiella pneumonia-Infected Mice
As illustrated in Figures 4A-C, Kp-infected mice showed increased total cells, macrophages, and neutrophils compared with the control group. Strikingly, after treatment with Bm at three doses (75, 150, and 300 μg/kg), cell numbers were effectively reduced in the mice.
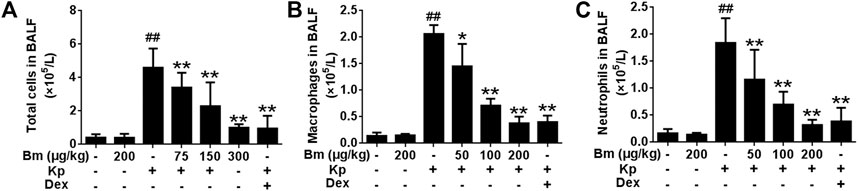
FIGURE 4. Effects of Bergenin monohydrate on the number of total cells, neutrophils and macrophages after Klebsiella pneumonia infection (A) Statistics of total cells in BALF (B) Statistics of macrophages in BALF (C) Statistics of neutrophils in BALF. All data are presented as the means ± SEM (n = 5). ##p < 0.01 vs the Control group; *p < 0.05 and **p < 0.01 vs the Klebsiella pneumonia group.
Bm treatment restored the MPO, MDA, SOD and GSH levels in Kp-infected mice.
As shown in Figures 5A-D, Kp significantly elevated MPO and MDA levels compared with levels in the other groups. Groups pretreated with Bm at 75, 150, and 300 μg/kg showed decreased MPO levels by 4.07, 3.07, and 1.52 U/g, respectively, and decreased MDA levels by 3.20, 2.02, and 0.80 nmol/mg, respectively. Meanwhile, Kp dramatically reduced the levels of SOD and GSH. Groups pretreated with Bm exhibited increased SOD levels by 31.22, 45.40, and 52.65 U/mg, respectively, and increased GSH levels by 160.46, 183.46, and 208.41 μmol/L, respectively.
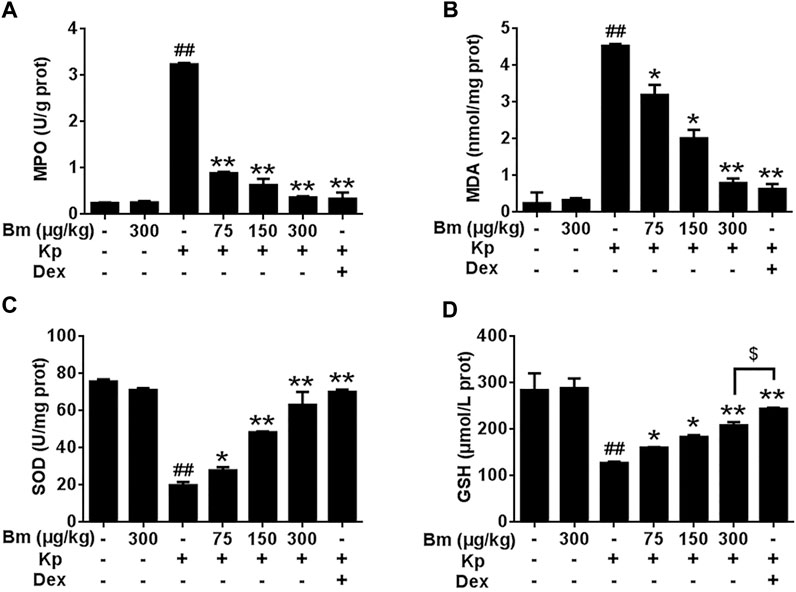
FIGURE 5. Effects of Bergenin monohydrate on MPO (A), MDA (B), GSH (C) and SOD (D) levels in lung tissues after Klebsiella pneumonia infection. *p < 0.05 and **p < 0.01 indicate significant differences compared with the Klebsiella pneumonia only group. All data are presented as the means ± SEM (n = 5 in each group). ##p < 0.01 vs the Control group; *p < 0.05 and **p < 0.01 vs the Klebsiella pneumonia group; $p < 0.05 vs the Dex group.
Bergenin Monohydrate Inhibited Kp-Induced NF-κB and MAPK Signaling Pathway Activation
We first examined the effect of a MAPK pathway inhibitor (SB203580) and NF-κB pathway inhibitor (BAY 11-7082) on WI38 cell viability. SB203580 and BAY 11-7082 at concentrations up to 20 µM barely altered cell viability, but at concentrations above 20 μM, they were cytotoxic (Figure 6A). Within safety thresholds, both the MAPK pathway inhibitor and NF-κB pathway inhibitor significantly reduced the protective effect of Bm against Kp infection in WI38 cells. The inhibitory effect of high concentrations was more robust than that of low concentrations (Figure 6B). Among them, the cell survival rate was even lower than that in the blank control group when 20 µM MAPK pathway inhibitor and NF-κB pathway inhibitor were added. However, without inhibitor interference, the cell survival rate was the highest, and Bm showed a relatively noticeable therapeutic effect.
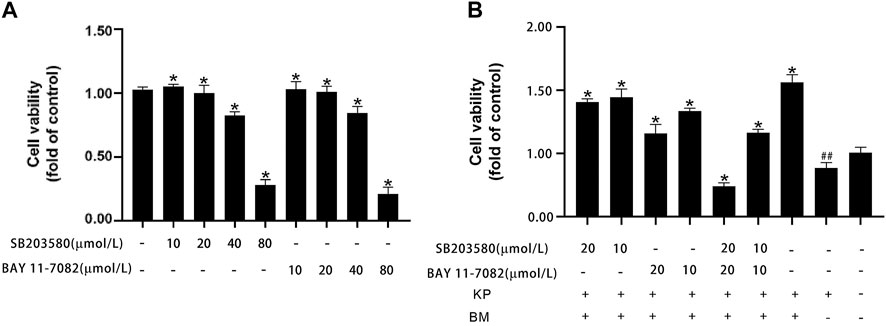
FIGURE 6. Effects of Bergenin monohydrate on the MAPK and NF-κB signaling pathways (A) MAPK and NF-κB pathway inhibitors were cytotoxic to WI 38 cells at higher concentrations (B) Cell viability of Bergenin monohydrate-treated and Klebsiella pneumonia-infected WI 38 cells after treatment with different doses of MAPK and NF-κB pathway inhibitors. ##p < 0.01 vs the Control group; *p < 0.05 and **p < 0.01 vs the Klebsiella pneumonia group.
As shown in the western blotting results, compared with the control and Bm groups after infection with Kp, the Kp group displayed higher levels of p38, ERK, and JNK phosphorylation (Figures 7A,B). There was an obvious dose-dependent effect among the groups in which pretreatment with Bm drastically suppressed phosphorylation of the three MAPK subfamilies p38, ERK, and JNK. Likewise, we examined IκB and NF-κB p65 subunits. We found that the Kp group displayed higher levels of IκB and NF-κB p65 phosphorylation than the other groups. In addition, in vivo studies confirmed a similar trend in p38, ERK, and JNK phosphorylation as observed in the in vitro experiments (Figures 8A,B). Hence, Bm efficiently inhibited the MAPK and NF-κB signaling pathways while attenuating the inflammatory response in Kp mice.
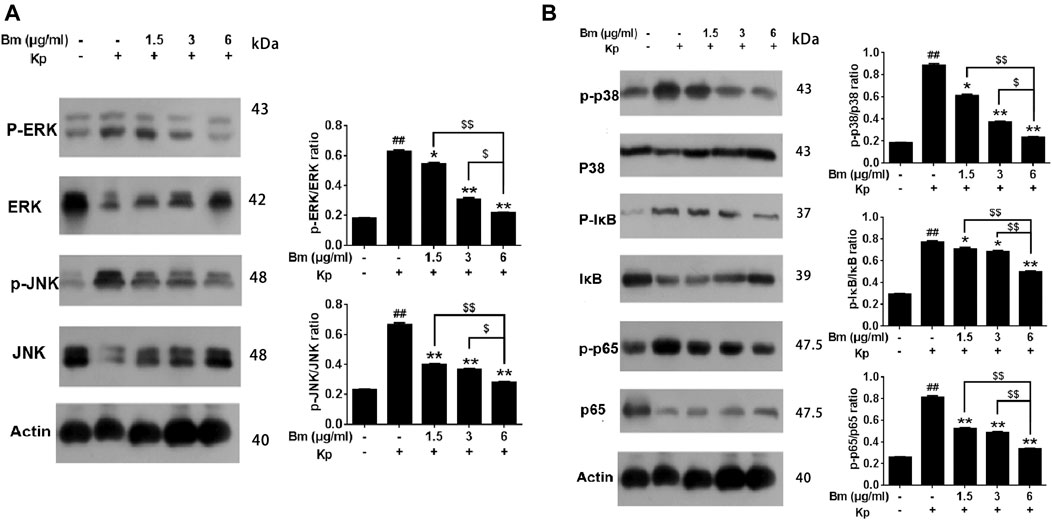
FIGURE 7. Effects of Bergenin monohydrate on the NF-κB and MAPK pathways in Klebsiella pneumonia-infected RAW264.7 cells determined by western blotting. Effects of Bergenin monohydrate on the expression of ERK, P38 and JNK in the MAPK pathway in RAW264.7 cells. Effects of Bergenin monohydrate on the expression of IκB/P65 in the NF-κB pathway in RAW264.7 cells (A) Effects on MAPK pathways in Klebsiella pneumonia-infected RAW264.7 cells (B) Effects on NF-κB pathways in Klebsiella pneumonia-infected RAW264.7 cells. All data are presented as the means ± SEM (n = 5 in each group). ##p < 0.01 vs the Control group; *p < 0.05 and **p < 0.01 vs the Klebsiella pneumonia group; $p < 0.05 and $$p < 0.01 vs the high-dose group.
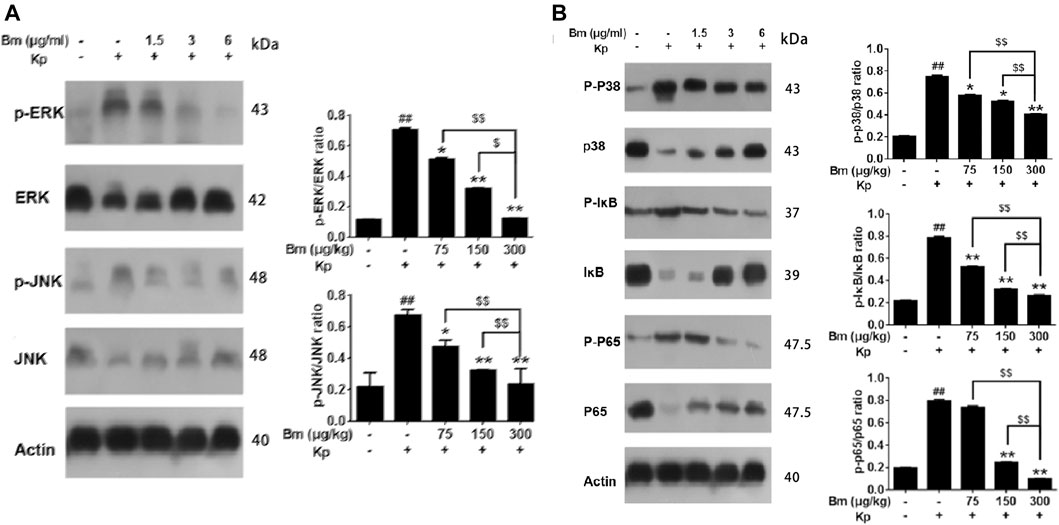
FIGURE 8. Effects of Bergenin monohydrate (Bm) on the NF-κB and MAPK pathways in Klebsiella pneumonia-infected mice determined by western blotting. Effects of Bergenin monohydrate on the expression of ERK, P38 and JNK in the MAPK pathway in Klebsiella pneumonia-infected mice. Effects of Bergenin monohydrate on the expression of IκB and P65 in the NF-κB pathway in Klebsiella pneumonia-infected mice (A) Effects on MAPK pathways in Klebsiella pneumonia-infected mice (B) Effects on NF-κB pathways in Klebsiella pneumonia-infected mice. All data are presented as the means ± SEM (n = 5 in each group). ##p < 0.01 vs the Control group; *p < 0.05 and **p < 0.01 vs the Klebsiella pneumonia group; $p < 0.05 and $$p < 0.01 vs the high-dose group.
Discussion
Klebsiella pneumonia is a crucial type of bacteria in the genus Klebsiella and accounts for more than 95% of Klebsiella infections (Raetz et al., 1991; Geetha et al., 2020; Ota et al., 2020). Kp infection is acknowledged to be severe and challenging to treat, causing a high mortality rate. In the past several years, antibiotics have been used to clear up this pneumonia, posing significant challenges due to multidrug resistance (Bengoechea and Sa Pessoa, 2019). Increasing antibiotic resistance necessitates the development of new drugs produced from bioactive natural products owing to their broad-spectrum pharmacological activity and low toxicity (Mohr, 2016; Pilehvar et al., 2016; Dodds, 2017). TCM monomers and their derivatives are believed to be very helpful in resolution of inflammatory responses, and their bioactive function and mechanism have been explored in recent years. As a representative TCM monomer, Bergenia purpurascens Qi Q., et al., 2018 which is a potent compound extracted from Bergenia purpurascens, has been demonstrated to exert numerous pharmacological effects, including antibacterial, analgesic, anti-inflammatory and antitumor activities (Fisher et al., 2017; Wu et al., 2017).
In the present study, both in vitro and in vivo experiments showed that Bm can suppress the expression of major inflammatory cytokines in Kp-infected cells and ameliorate lung injury in Kp-infected mice. ROS measurements in RAW 264.7 cells revealed a dose-dependent protective effect of Bm. Histological changes and pulmonary bacterial count results indicated that Bm reduced Kp bacteria levels while improving lung tissue histopathology. Bm is the main medicinal component of Ardisia japonica, and a large number of studies have shown that Ardisia japonica can be employed clinically to treat lung injury diseases, such as tuberculosis (Wang et al., 2016; Choi et al.,2004). In addition, Bm can prevent damage to the spleen and thymus by enhancing cellular immunity and cellular antioxidative activity (Qi et al., 2018) and promote anti-inflammatory and immunoregulatory effects. These results are consistent with our cell and animal experiment results. In the initial inflammatory process induced by Kp, lung tissue damage induces neutrophil infiltration, and one significant index of neutrophil infiltration is MPO Lv et al., 2017 MDA is produced by cell membranes and can be used to reflect free radical metabolism and the state of cells attacked by free radicals (Reichmann et al., 2018). GSH and SOD are key indicators of oxidative stress, and their ratio reflects the redox state of the cellular environment and, consequently, is a cellular health measure (Petrov et al., 2016). In our study, treatment with Bm caused a dose-dependent decrease in MPO and MDA and an increase in SOD and GSH levels, thereby protecting cells against oxidative insult by Kp. As a side observation, the therapeutic effect of Bm was consistent with the pathway inhibition experiment results in human fibroblasts (WI38 cells), heralding the possibility of effective therapy for human Klebsiella pneumonia.
TNF-α, IL-6, IL-1β and PEG2 proinflammatory cytokines, including TNF, IL-1, IL-6 and IL-8, are small molecular peptides that can regulate a variety of physiological functions and increase the expression of proinflammatory cytokines (Fann et al., 2018). These important cytokines are interrelated and involved in regulating the inflammatory response (Yang et al., 2020). IL-6 and IL-1β are involved in the inflammatory response in organs and tissues of mammals and are generally known as triggers of pathologic pain (Hudock et al., 2020). In 2020, IL-1β, IL-6, and other proteins were found to be correlated with pulmonary inflammation with extensive lung involvement, as seen in COVID-19 patients. TNF-α improves the levels of PEG2 and bradykinin, which are also thought to influence the TNF-α/TNF receptor signaling pathway and promote the development of pulmonary endothelial cell injury (Nishida et al., 2020). In 2018, a study reported that knockdown of TNF-α alleviated the severity of lung injury; thus, TNF-α might be a key target in injury and repair related to the lung (Qi et al., 2018). In the present study, the ELISA results indicated that TNF-α, IL-6, IL-1β and PEG2 levels in Kp-induced pneumonia mice were downregulated during Bm treatment, suggesting that Bm attenuates intestinal inflammation by decreasing the levels of these cytokines in lung tissues and cells.
To explore further pathway mechanisms, we investigated whether Bm regulates the MAPK and NF-κB signaling pathways to execute protective and anti-inflammatory actions. Western blotting and ELISA results revealed that the levels of the proinflammatory cytokines TNF-α, IL-6, IL-1β and PEG2; the MAPK subfamilies p38, ERK, and JNK; and the NF-κB subfamilies IκB and NF-κB-p65 exhibited a downward trend in a dose-dependent manner with Bm treatment after Kp infection. Both the MAPK and NF-κB signaling pathways are regarded as classical and significant anti-inflammatory pathways (Sun, 2017; Kim et al., 2019; Ali et al., 2020; Ren et al., 2020). The pathway inhibition experiments in the present study confirmed the involvement of the MAPK signaling pathway and NF-kappaB signaling pathway in the activity of Bm against Kp, and good agreement among pathway inhibition experiments and ELISA and western blotting results was found. MAPKs are a family of serine/threonine protein kinases that mediate fundamental biological processes, signal transduction and cell responses and phosphorylate various substrate proteins, including proinflammatory transcription factors; thus, MAPKs are potential targets for anti-inflammatory therapeutics (Liang et al., 2014). In mammals, The MAPK family includes the extracellular signal-regulated kinase (ERK), Jun N-terminal kinase (JNK) and p38 pathways (Zhao et al., 2020). Together with the findings obtained in this study, we found that after Kp infection, Bm could regulate the MAPK pathway by inactivating JNK, ERK and p38. NF-κB is another prominent transcription factor involved in various functions affecting cellular behavior (Victor et al., 2005) and is considered the central mediator of the inflammatory response and a leading participant in normal immune processes. NF-κB is activated by the MAPK pathway to regulate antimicrobial and inflammatory responses. As one of the downstream ERK/MAPK signaling pathway pathways, NF-kB plays an important role in inflammatory processes (Baldwin, 1996; Urbano et al., 2014; Soleimani et al., 2019). In the nucleus, activated NF-kB is phosphorylated as a transcription factor of inflammatory genes (Kim et al., 2019; Mishra et al., 2020; Soleimani et al., 2020). IκB is a negative regulator of the NF-κB pathway, and activation of IκB promotes NF-κB p65 translocation to the cell nucleus. (Qi et al., 2018; Xu et al., 2019). Moreover, several previous studies have illustrated that activated NF-κB regulates the expression of genes such as IL-1β, IL-6, and TNF-α and promotes the occurrence, development and pathogenesis of autoimmune diseases, chronic infections and cancer (Nold-Petry et al., 2015; Stassi et al., 2017; Wilson et al., 2020). The results of this study are consistent with those of the above studies, and similarly, our study provides an experimental basis for application of Bm, potentially leading to discovery of other effects and side effects.
The study had a few limitations. First of all, Bm has not been found an obvious inhibition effect for Kp growth and protective effect for Kp-infected Raw cells, but in animal experiments, it demonstrated a good anti-inflammatory effect. This phenomenon attracted our attention. By reviewing the available literature, we have found that conditions in vitro are unable to mimic that in vivo environment with hypoxia, hypoglycemia, and other metabolic changes (Su et al., 2019), although some compound didn’t show an inhibitory effect on pathogens in vitro, they can actually inhibit the growth of pathogens by changing the microenvironment in vivo (Liu et al., 2017). However, the specific mechanisms require further investigation. Moreover, the influence of Bm for MAPK and NF-κB signaling pathways were confirmed, but the mechanisms underlying the effects of Bm in other signaling pathways are not completely understood and require further investigation.
In conclusion, our results demonstrated a dose-dependent protective role of Bm in Kp-infected cells and mice through modulation of inflammatory cytokine expression. Bm exerted anti-inflammatory action by regulating Iκ-Bα and p65 phosphorylation and regulated the MAPK signaling pathway by inhibiting p38, ERK, and JNK phosphorylation after Kp infection. Thus, our findings provide evidence that Bm may be a promising therapeutic agent for Kp prophylaxis.
Data Availability Statement
The raw data supporting the conclusions of this article will be made available by the authors, without undue reservation.
Ethics Statement
The animal study was reviewed and approved by the Animal Welfare and Research Ethics Committee of Qingdao Agriculture University (Approved Number: SYXK (SD) 20170005).
Author Contributions
QT and QW contributed equally to this article and shared the first authorship. QT and QW contributed to the writing of this manuscript; ZS and SK performed the experiments and analyzed data; QT and YF designed this study and analyzed data; QT, ZH oversaw the execution of this study and contributed to the writing and revision of this manuscript. All authors reviewed the manuscript.
Funding
This work was supported by The National Key Research and Development Plan (grant number: 2016YFD0501007), and the Beijing Municipal Science and Technology Project (grant number: Z191100001619001).
Conflict of Interest
The authors declare that the research was conducted in the absence of any commercial or financial relationships that could be construed as a potential conflict of interest.
Supplementary Material
The Supplementary Material for this article can be found online at: https://www.frontiersin.org/articles/10.3389/fphar.2021.651664/full#supplementary-material
References
Ali, F. E. M., Saad Eldien, H. M., Mostafa, N. A. M., Almaeen, A. H., Marzouk, M. R. A., Eid, K. M., et al. (2020). The Impact of Royal Jelly against Hepatic Ischemia/Reperfusion-Induced Hepatocyte Damage in Rats: The Role of Cytoglobin, Nrf-2/HO-1/COX-4, and P38-MAPK/NF-κB-p65/TNF-α Signaling Pathways. Cmp 14, 88–100. doi:10.2174/1874467213666200514223829
Avgerinos, E. D., Kostopanagiotou, G., Kostopanagiotou, K., Kopanakis, N., Andreadou, I., Lekka, M., et al. (2020). Corrigendum to “Intestinal Preconditioning Ameliorates Ischemia-Reperfusion Induced Acute Lung Injury in Rats: An Experimental Study”. J. Surg. Res. 246 (2), 629–301. doi:10.1016/j.jss.2017.11.042
Baldwin, A. S. (1996). THE NF-κB and IκB PROTEINS: New Discoveries and Insights. Annu. Rev. Immunol. 14, 649–681. doi:10.1146/annurev.immunol.14.1.649
Batubara, I., Astuti, R. I., Prastya, M. E., Ilmiawati, A., Maeda, M., Suzuki, M., et al. (2020). The Antiaging Effect of Active Fractions and Ent-11α-Hydroxy-15-Oxo-Kaur-16-En-19-Oic Acid Isolated from Adenostemma Lavenia (L.) O. Kuntze at the Cellular Level. Antioxidants 9 (8), 719. doi:10.3390/antiox9080719
Bengoechea, J. A., and Sa Pessoa, J. (2019). Klebsiella Pneumoniaeinfection Biology: Living to Counteract Host Defences. FEMS Microbiol. Rev. 43 (2), 123–144. doi:10.1093/femsre/fuy043
Chan, H. H. L., and Ng, T. (2020). Traditional Chinese Medicine (TCM) and Allergic Diseases. Curr. Allergy Asthma Rep. 20 (11), 67. doi:10.1007/s11882-020-00959-9
Chang, H.-J., Kim, Y.-H., Kang, Y.-H., Choi, M.-H., and Lee, J.-H. (2020). Antioxidant and Antibacterial Effects of Medicinal Plants and Their Stick-type Medicinal Concentrated Beverages. Food Sci. Biotechnol. 29 (10), 1413–1423. doi:10.1007/s10068-020-00793-9
Chew, K. L., Lin, R. T. P., and Teo, J. W. P. (2017). Klebsiella pneumoniae in Singapore: Hypervirulent Infections and the Carbapenemase Threat. Front. Cel. Infect. Microbiol. 7, 515. doi:10.3389/fcimb.2017.00515
Choby, J. E., Howard‐Anderson, J., and Weiss, D. S. (2020). HypervirulentKlebsiella Pneumoniae- Clinical and Molecular Perspectives. J. Intern. Med. 287 (3), 283–300. doi:10.1111/joim.13007
Dodds, D. R. (2017). Antibiotic Resistance: A Current Epilogue. Biochem. Pharmacol. 134, 139–146. doi:10.1016/j.bcp.2016.12.005
Fan, X., Li, N., Wang, X., Zhang, J., Xu, M., Liu, X., et al. (2018). Protective Immune Mechanisms of Yifei Tongluo, a Chinese Herb Formulation, in the Treatment of Mycobacterial Infection. PLoS One 13 (9), e0203678. doi:10.1371/journal.pone.0203678
Fann, D. Y.-W., Lim, Y.-A., Cheng, Y.-L., Lok, K.-Z., Chunduri, P., Baik, S.-H., et al. (2018). Evidence that NF-κB and MAPK Signaling Promotes NLRP Inflammasome Activation in Neurons Following Ischemic Stroke. Mol. Neurobiol. 55 (2), 1082–1096. doi:10.1007/s12035-017-0394-9
Fisher, R. A., Gollan, B., and Helaine, S. (2017). Persistent Bacterial Infections and Persister Cells. Nat. Rev. Microbiol. 15 (8), 453–464. doi:10.1038/nrmicro.2017.42
Geetha, P. V., Aishwarya, K. V. L., Mariappan, S., and Sekar, U. (2020). Fluoroquinolone Resistance in Clinical Isolates of Klebsiella Pneumoniae. J. Lab. Physicians 12 (2), 121–125. doi:10.1055/s-0040-1716478
Hossan, M. S., Jindal, H., Maisha, S., Samudi Raju, C., Devi Sekaran, S., Nissapatorn, V., et al. (2018). Antibacterial Effects of 18 Medicinal Plants Used by the Khyang Tribe in Bangladesh. Pharm. Biol. 56 (1), 201–208. doi:10.1080/13880209.2018.1446030
Hudock, K. M., Collins, M. S., Imbrogno, M., Snowball, J., Kramer, E. L., Brewington, J. J., et al. (2020). Neutrophil Extracellular Traps Activate IL-8 and IL-1 Expression in Human Bronchial Epithelia. Am. J. Physiology-Lung Cell Mol. Physiol. 319 (1), L137–L147. doi:10.1152/ajplung.00144.2019
Kim, H. N., Kim, J. D., Yeo, J. H., Son, H.-J., Park, S. B., Park, G. H., et al. (2019). Heracleum Moellendorffii Roots Inhibit the Production of Pro-inflammatory Mediators through the Inhibition of NF-κB and MAPK Signaling, and Activation of ROS/Nrf2/HO-1 Signaling in LPS-Stimulated RAW264.7 Cells. BMC Complement. Altern. Med. 19 (1), 310. doi:10.1186/s12906-019-2735-x
Liang, D., Li, F., Fu, Y., Cao, Y., Song, X., Wang, T., et al. (2014). Thymol Inhibits LPS-Stimulated Inflammatory Response via Down-Regulation of NF-κB and MAPK Signaling Pathways in Mouse Mammary Epithelial Cells. Inflammation 37 (1), 214–222. doi:10.1007/s10753-013-9732-x
Liao, L.-y., He, Y.-f., Li, L., Meng, H., Dong, Y.-m., Yi, F., et al. (2018). A Preliminary Review of Studies on Adaptogens: Comparison of Their Bioactivity in TCM with that of Ginseng-like Herbs Used Worldwide. Chin. Med. 13, 57. doi:10.1186/s13020-018-0214-9
Liu, S., Liu, B., Luo, Z.-Q., Qiu, J., Zhou, X., Li, G., et al. (2017). The Combination of Osthole with Baicalin Protects Mice from Staphylococcus aureus Pneumonia. World J. Microbiol. Biotechnol. 33 (1), 11. doi:10.1007/s11274-016-2162-9
Lv, H., Liu, Q., Wen, Z., Feng, H., Deng, X., and Ci, X. (2017a). Xanthohumol Ameliorates Lipopolysaccharide (LPS)-induced Acute Lung Injury via Induction of AMPK/GSK3β-Nrf2 Signal axis. Redox Biol. 12, 311–324. doi:10.1016/j.redox.2017.03.001
Lv, H., Liu, Q., Zhou, J., Tan, G., Deng, X., and Ci, X. (2017b). Daphnetin-mediated Nrf2 Antioxidant Signaling Pathways Ameliorate Tert-Butyl Hydroperoxide (T-Bhp)-Induced Mitochondrial Dysfunction and Cell Death. Free Radic. Biol. Med. 106, 38–52. doi:10.1016/j.freeradbiomed.2017.02.016
Mishra, R., Sehring, I., Cederlund, M., Mulaw, M., and Weidinger, G. (2020). NF-κB Signaling Negatively Regulates Osteoblast Dedifferentiation during Zebrafish Bone Regeneration. Dev. Cell 52 (2), 167–182. doi:10.1016/j.devcel.2019.11.016
Mohr, K. I. (2016). History of Antibiotics Research. Curr. Top. Microbiol. Immunol. 398, 237–272. doi:10.1007/82_2016_499
Newman, D. J. (2020). Modern Traditional Chinese Medicine: Identifying, Defining and Usage of TCM Components. Adv. Pharmacol. 87, 113–158. doi:10.1016/bs.apha.2019.07.001
Nishida, K., Watanabe, H., Miyahisa, M., Hiramoto, Y., Nosaki, H., Fujimura, R., et al. (2020). Systemic and Sustained Thioredoxin Analogue Prevents Acute Kidney Injury and Its-Associated Distant Organ Damage in Renal Ischemia Reperfusion Injury Mice. Sci. Rep. 10 (1), 20635. doi:10.1038/s41598-020-75025-5
Nold-Petry, C. A., Lo, C. Y., Rudloff, I., Elgass, K. D., Li, S., Gantier, M. P., et al. (2015). IL-37 Requires the Receptors IL-18Rα and IL-1R8 (SIGIRR) to Carry Out its Multifaceted Anti-inflammatory Program upon Innate Signal Transduction. Nat. Immunol. 16 (4), 354–365. doi:10.1038/ni.3103
Ota, K., Kaku, N., and Yanagihara, K. (2020). Efficacy of Meropenem and Amikacin Combination Therapy against Carbapenemase-Producing Klebsiella pneumoniae Mouse Model of Pneumonia. J. Infect. Chemother. 26 (12), 1237–1243. doi:10.1016/j.jiac.2020.07.002
Peteranderl, C., Sznajder, J. I., Herold, S., and Lecuona, E. (2017). Inflammatory Responses Regulating Alveolar Ion Transport during Pulmonary Infections. Front. Immunol. 8, 446. doi:10.3389/fimmu.2017.00446
Petrov, D., Daura, X., and Zagrovic, B. (2016). Effect of Oxidative Damage on the Stability and Dimerization of Superoxide Dismutase 1. Biophysical J. 110 (7), 1499–1509. doi:10.1016/j.bpj.2016.02.037
Pilehvar, S., Gielkens, K., Trashin, S. A., Dardenne, F., Blust, R., and De Wael, K. (2016). (Electro)Sensing of Phenicol Antibiotics-A Review. Crit. Rev. Food Sci. Nutr. 56 (14), 2416–2429. doi:10.1080/10408398.2013.845140
Poivre, M., and Duez, P. (2017). Biological Activity and Toxicity of the Chinese Herb Magnolia Officinalis Rehder and E. Wilson (Houpo) and its Constituents. J. Zhejiang Univ. Sci. B 18 (3), 194–214. doi:10.1631/jzus.B1600299
Qi, Q., Dong, Z., Sun, Y., Li, S., and Zhao, Z. (2018). Protective Effect of Bergenin against Cyclophosphamide-Induced Immunosuppression by Immunomodulatory Effect and Antioxidation in Balb/c Mice. Molecules 23 (10), 2668. doi:10.3390/molecules23102668
Raetz, C. R. H., Ulevitch, R. I., Wright, S. D., Sibley, C. H., Ding, A., and Nathan, C. F. (1991). Gram‐negative Endotoxin: an Extraordinary Lipid with Profound Effects on Eukaryotic Signal Transduction 1. FASEB j. 5 (12), 2652–2660. doi:10.1096/fasebj.5.12.1916089
Reichmann, D., Voth, W., and Jakob, U. (2018). Maintaining a Healthy Proteome during Oxidative Stress. Mol. Cel 69 (2), 203–213. doi:10.1016/j.molcel.2017.12.021
Ren, Q., Guo, F., Tao, S., Huang, R., Ma, L., and Fu, P. (2020). Flavonoid Fisetin Alleviates Kidney Inflammation and Apoptosis via Inhibiting Src-Mediated NF-κB P65 and MAPK Signaling Pathways in Septic AKI Mice. Biomed. Pharmacother. 122, 109772. doi:10.1016/j.biopha.2019.109772
Soleimani, A., Pashirzad, M., Avan, A., Ferns, G. A., Khazaei, M., and Hassanian, S. M. (2019). Role of the Transforming Growth Factor‐β Signaling Pathway in the Pathogenesis of Colorectal Cancer. J. Cel Biochem 120 (6), 8899–8907. doi:10.1002/jcb.28331
Soleimani, A., Rahmani, F., Ferns, G. A., Ryzhikov, M., Avan, A., and Hassanian, S. M. (2020). Role of the NF-κB Signaling Pathway in the Pathogenesis of Colorectal Cancer. Gene 726, 144132. doi:10.1016/j.gene.2019.144132
Song, M., Li, L., Li, M., Cha, Y., Deng, X., and Wang, J. (2016). Apigenin Protects Mice from Pneumococcal Pneumonia by Inhibiting the Cytolytic Activity of Pneumolysin. Fitoterapia 115, 31–36. doi:10.1016/j.fitote.2016.09.017
Stassi, A. F., Baravalle, M. E., Belotti, E. M., Rey, F., Gareis, N. C., Díaz, P. U., et al. (2017). Altered Expression of Cytokines IL-1α, IL-6, IL-8 and TNF-α in Bovine Follicular Persistence. Theriogenology 97, 104–112. doi:10.1016/j.theriogenology.2017.04.033
Su, X., Shen, Z., Yang, Q., Sui, F., Pu, J., Ma, J., et al. (2019). Vitamin C Kills Thyroid Cancer Cells through ROS-dependent Inhibition of MAPK/ERK and PI3K/AKT Pathways via Distinct Mechanisms. Theranostics 9 (15), 4461–4473. doi:10.7150/thno.35219
Sun, S.-C. (2017). The Non-canonical NF-κB Pathway in Immunity and Inflammation. Nat. Rev. Immunol. 17 (9), 545–558. doi:10.1038/nri.2017.52
Sun, Z., Li, Q., Hou, R., Sun, H., Tang, Q., Wang, H., et al. (2019). Kaempferol-3-O-glucorhamnoside Inhibits Inflammatory Responses via MAPK and NF-κB Pathways In Vitro and In Vivo. Toxicol. Appl. Pharmacol. 364, 22–28. doi:10.1016/j.taap.2018.12.008
Turan, I., Sayan Ozacmak, H., Ozacmak, V. H., Barut, F., and Ozacmak, I. D. (2018). The Effects of S-Nitrosoglutathione on Intestinal Ischemia Reperfusion Injury and Acute Lung Injury in Rats: Roles of Oxidative Stress and NF-κB. Tissue and Cell 52, 35–41. doi:10.1016/j.tice.2018.03.012
Urbano, P., Soccol, V., and Feijo Azevedo, V. (2014). Apoptosis and the FLIP and NF-Kappa B Proteins as Pharmacodynamic Criteria for Biosimilar TNF-Alpha Antagonists. Btt 8, 211–220. doi:10.2147/BTT.S57253
Victor, V., Rocha, M., Esplugues, J., and Fuente, M. (2005). Role of Free Radicals in Sepsis: Antioxidant Therapy. Cpd 11 (24), 3141–3158. doi:10.2174/1381612054864894
Wang, J., Wong, Y.-K., and Liao, F. (2018). What Has Traditional Chinese Medicine Delivered for Modern Medicine? Expert Rev. Mol. Med. 20, e4. doi:10.1017/erm.2018.3
Wang, Y., Qi, Q., Li, A., Yang, M., Huang, W., Xu, H., et al. (2016). Immuno-enhancement Effects of Yifei Tongluo Granules on Cyclophosphamide-Induced Immunosuppression in Balb/c Mice. J. Ethnopharmacology 194, 72–82. doi:10.1016/j.jep.2016.08.046
Wilson, J. G., Simpson, L. J., Ferreira, A.-M., Rustagi, A., Roque, J., Asuni, A., et al. (2020). Cytokine Profile in Plasma of Severe COVID-19 Does Not Differ from ARDS and Sepsis. JCI Insight 5 (17). doi:10.1172/jci.insight.140289
Wu, X., Tan, S., Xing, Y., Pu, Q., Wu, M., and Zhao, J. X. (2017). Graphene Oxide as an Efficient Antimicrobial Nanomaterial for Eradicating Multi-Drug Resistant Bacteria In Vitro and In Vivo. Colloids Surf. B: Biointerfaces 157, 1–9. doi:10.1016/j.colsurfb.2017.05.024
Xu, R., Zhang, F., Chai, R., Zhou, W., Hu, M., Liu, B., et al. (2019). Exosomes Derived from Pro‐inflammatory Bone Marrow‐derived Mesenchymal Stem Cells Reduce Inflammation and Myocardial Injury via Mediating Macrophage Polarization. J. Cel Mol. Med. 23 (11), 7617–7631. doi:10.1111/jcmm.14635
Yang, B., Zhang, Z., Yang, Z., Ruan, J., Luo, L., Long, F., et al. (2020). Chanling Gao Attenuates Bone Cancer Pain in Rats by the IKKβ/NF-κB Signaling Pathway. Front. Pharmacol. 11, 525. doi:10.3389/fphar.2020.00525
Yang, Z., Zhang, X. R., Zhao, Q., Wang, S. L., Xiong, L. L., Zhang, P., et al. (2018). Knockdown of TNF-α A-lleviates A-cute L-ung I-njury in R-ats with I-ntestinal I-schemia and R-eperfusion I-njury by U-pregulating IL-10 E-xpression. Int. J. Mol. Med. 42 (2), 926–934. doi:10.3892/ijmm.2018.3674
Zhang, H., Luan, Y., Jing, S., Wang, Y., Gao, Z., Yang, P., et al. (2020). Baicalein Mediates Protection against Staphylococcus Aureus-Induced Pneumonia by Inhibiting the Coagulase Activity of vWbp. Biochem. Pharmacol. 178, 114024. doi:10.1016/j.bcp.2020.114024
Zhang, L., Zhang, Y.-J., Chen, J., Huang, X.-L., Fang, G.-S., Yang, L.-J., et al. (2018). The Association of HLA-B27 and Klebsiella pneumoniae in Ankylosing Spondylitis: A Systematic Review. Microb. Pathogenesis 117, 49–54. doi:10.1016/j.micpath.2018.02.020
Zhao, M., Guo, D.-L., Liu, G.-H., Fu, X., Gu, Y.-C., Ding, L.-S., et al. (2020). Antifungal Halogenated Cyclopentenones from the Endophytic Fungus Saccharicola Bicolor of Bergenia Purpurascens by the One Strain-Many Compounds Strategy. J. Agric. Food Chem. 68 (1), 185–192. doi:10.1021/acs.jafc.9b06594
Keywords: Klebsiella pneumoniae, Bergenin monohydrate, anti-inflammatory, MAPK pathways, NF-κB pathways
Citation: Tang Q, Wang Q, Sun Z, Kang S, Fan Y and Hao Z (2021) Bergenin Monohydrate Attenuates Inflammatory Response via MAPK and NF-κB Pathways Against Klebsiella pneumonia Infection. Front. Pharmacol. 12:651664. doi: 10.3389/fphar.2021.651664
Received: 10 January 2021; Accepted: 19 April 2021;
Published: 04 May 2021.
Edited by:
Jie Xu, Fudan University, ChinaReviewed by:
Haichong Wu, Zhejiang University, ChinaKangfeng Jiang, Yunnan Agricultural University, China
Soromou Lanan Wassy, Higher Institute of Sciences and Veterinary Medicine, Dalaba, Guinea
Gan Zhao, University of Pennsylvania, United States
Copyright © 2021 Tang, Wang, Sun, Kang, Fan and Hao. This is an open-access article distributed under the terms of the Creative Commons Attribution License (CC BY). The use, distribution or reproduction in other forums is permitted, provided the original author(s) and the copyright owner(s) are credited and that the original publication in this journal is cited, in accordance with accepted academic practice. No use, distribution or reproduction is permitted which does not comply with these terms.
*Correspondence: Zhihui Hao, aGFvemhpaHVpQGNhdS5lZHUuY24=
†These authors have contributed equally to this work and share first authorship