- 1Department of Cardiology, University of Heidelberg, Heidelberg, Germany
- 2DZHK (German Center for Cardiovascular Research), Partner Site Heidelberg/Mannheim, University of Heidelberg, Heidelberg, Germany
- 3HCR, Heidelberg Center for Heart Rhythm Disorders, University of Heidelberg, Heidelberg, Germany
- 4Clinic for Cardiology II: Electrophysiology, University Hospital Münster, Münster, Germany
- 5Department of Clinical Pharmacology and Pharmacoepidemiology, University of Heidelberg, Heidelberg, Germany
Atrial fibrillation (AF) is the most common sustained arrhythmia with a prevalence of up to 4% and an upwards trend due to demographic changes. It is associated with an increase in mortality and stroke incidences. While stroke risk can be significantly reduced through anticoagulant therapy, adequate treatment of other AF related symptoms remains an unmet medical need in many cases. Two main treatment strategies are available: rate control that modulates ventricular heart rate and prevents tachymyopathy as well as rhythm control that aims to restore and sustain sinus rhythm. Rate control can be achieved through drugs or ablation of the atrioventricular node, rendering the patient pacemaker-dependent. For rhythm control electrical cardioversion and pharmacological cardioversion can be used. While electrical cardioversion requires fasting and sedation of the patient, antiarrhythmic drugs have other limitations. Most antiarrhythmic drugs carry a risk for pro-arrhythmic effects and are contraindicated in patients with structural heart diseases. Furthermore, catheter ablation of pulmonary veins can be performed with its risk of intraprocedural complications and varying success. In recent years TASK-1 has been introduced as a new target for AF therapy. Upregulation of TASK-1 in AF patients contributes to prolongation of the action potential duration. In a porcine model of AF, TASK-1 inhibition by gene therapy or pharmacological compounds induced cardioversion to sinus rhythm. The DOxapram Conversion TO Sinus rhythm (DOCTOS)-Trial will reveal whether doxapram, a potent TASK-1 inhibitor, can be used for acute cardioversion of persistent and paroxysmal AF in patients, potentially leading to a new treatment option for AF.
1 Introduction
Atrial fibrillation (AF) is the most common sustained cardiac arrhythmia with a prevalence of up to 4% in modern western populations and 0.5–2% in Asians (Fuster et al., 2006; Zoni-Berisso et al., 2014; Zulkifly et al., 2018), indicating the increased AF risk of Whites compared to Asians, Hispanics, and Blacks (Dewland et al., 2013). Differences in genetics, environmental exposures, cardiac structures, and ascertainment of AF are discussed as possible reasons but so far without a definite answer (Nazeri et al., 2010; Dewland et al., 2013; Gillott et al., 2017; Lee et al., 2017; Ugowe et al., 2018). AF is a predominant disease of the elderly population, with prevalence rates of 10.0–17.0% for patients over the age of 80 compared to 0.1% under the age of 49 (Zoni-Berisso et al., 2014). In 2010, the number of people worldwide suffering from AF was estimated to range around 33.5 million (Chugh et al., 2014) with an increased prevalence in children of parents with AF (Fox et al., 2004; Øyen et al., 2012). Furthermore, 30–40% of patients undergoing cardiac surgery and even 10–20% of patients with non-cardiac surgery develop new on-set AF (Chebbout et al., 2018; Dobrev et al., 2019). As triggers, a combination of inflammation, cardiac ischemia, altered atrial cytosolic calcium handling, and sympathetic activation are discussed, whereas the underlying mechanisms of postoperative AF are not yet fully understood (Aguilar and Nattel, 2019; Dobrev et al., 2019; Fakuade et al., 2020).
AF is associated with an increase of overall mortality of 1.5-fold in men and 2-fold in women (Benjamin et al., 1998; Stewart et al., 2002; Andersson et al., 2013). It is connected with an almost 5-fold increase in stroke incidences (Wolf et al., 1991) and about 20–30% of all ischemic stroke patients suffer from AF (Henriksson et al., 2012). Therefore, stroke prevention represents a central aspect of AF therapy (Hindricks et al., 2020). However, while death by stroke can be greatly reduced by anticoagulant therapy (Hart et al., 1999, 2007; Ruff et al., 2014; Piazza et al., 2020) there is still a residual stroke rate of 1.5% per year (Kirchhof et al., 2013). Furthermore, cardiovascular death remains common in patients suffering from AF (Kotecha et al., 2014) with a death rate of about 3.5% per year (Kirchhof et al., 2013).
In AF therapy, there are two predominant treatment strategies: rhythm control that aims to restore and sustain sinus rhythm (SR) and rate control that modulates ventricular heart rate and prevents development of tachymyopathy (Hindricks et al., 2020). In the existing literature, there are many clinical trials, systematic reviews, and meta-analyses slightly favoring one strategy above the other but, in cumulative analyses, the outcome of both treatment strategies seems to be comparable and to depend largely on comorbidities and severity of AF (de Denus et al., 2005; Chatterjee et al., 2013; Al-Khatib et al., 2014; Kotecha and Kirchhof, 2014; Nattel et al., 2014; Conkbayir et al., 2019; Conte et al., 2019; de Vecchis et al., 2019; Willems et al., 2019). Very recently, however, Kirchhof et al. (2020) showed in the Early Treatment of Atrial Fibrillation for Stroke Prevention Trial (EAST-AFNET 4), that rhythm control might be associated with a lower risk of death for cardiovascular reasons than rate control in patients with early (less than 1 year) AF, suggesting that rhythm control could be superior at least in this patient population.
Although many therapeutic options are available, there is still an unmet need in AF therapy due to insufficient efficacy and cardiac as well as extracardiac side effects of current treatments, which may negatively influence their therapeutic benefit (Ravens, 2010, 2017). To satisfy this unmet treatment need the underlying causes of AF have become a focus of research, leading to recognition of the tandem of P domains in a weak inward rectifying K+ channel (TWIK)-related acid sensitive K+ channel 1 (TASK-1), a member of the two-pore-domain potassium (K2P) channel family (Schmidt et al., 2015, 2017; Ravens, 2017). The atrial-selective TASK-1 current plays an important role in the repolarization phase of the atrial action potential (AP) (Schmidt et al., 2015, 2017; Schmidt and Peyronnet, 2018). AF induced changes in the TASK-1 current lead to an altered action potential duration (APD), which can help maintain AF, therefore, showing an involvement of TASK-1 in the pathophysiology of AF (Schmidt et al., 2015, 2017). Taken into account its atrial-selective expression in the human heart, its upregulation in AF, and its contribution to AF related APD shortening, TASK-1 represents a promising target for the treatment of AF (Schmidt et al., 2015, 2017).
In the following review we provide an overview of the existing literature regarding the two predominant treatment strategies for AF as well as the current state of research on TASK-1 in the context of AF therapy.
2 Rate Control Therapy
One of the two main treatment strategies for AF is rate control therapy. Modulation of the ventricular heart rate prevents development of tachymyopathy and is often enough to improve AF-related symptoms (Hindricks et al., 2020). First-line therapy in acute and long-term rate control are beta-blockers (Jones et al., 2014; Hindricks et al., 2020). In SR patients with heart failure they achieve a reduction in all-cause mortality which however, has recently been questioned in AF patients (Kotecha et al., 2014). Usually, they are well tolerated by all ages and have a potential for symptomatic and functional improvements (Kotecha et al., 2014, 2016).
Alternatively, or in combination, if the desired heart rate is not achieved with monotherapy, non-dihydropyridine calcium channel blockers can be used (Hindricks et al., 2020). However, if administered in combination with beta-blockers, a careful monitoring of the heart rate via 24 h electrocardiogram (ECG) to check for bradycardia should be done (Hindricks et al., 2020). Verapamil and diltiazem slow heart rate and are able to reduce symptoms of AF (Ulimoen et al., 2013). They are, however, not safe in use for patients with heart failure (Goldstein et al., 1991; Elkayam, 1998).
Another option for rate control therapy are cardiac glycosides (Hindricks et al., 2020). They act through an inhibition of the Na+–K+-ATPase (Schatzmann, 1953; Glynn, 1957; Schatzmann and Räss, 1965). They have been in use for over 200 years and are still controversially discussed (Goldberger and Alexander, 2014). The Digitalis Investigation Group (1997) could show in a randomized trial, that digoxin did not reduce mortality in patients with heart failure but reduced the rate of hospitalization. In this trial, however, patients with AF were excluded. Several studies have observed an increased mortality in AF patients taking digoxin (Hallberg et al., 2007; Whitbeck et al., 2013; Turakhia et al., 2014; Charnigo et al., 2018). Interpretation of the results from cardiac glycoside studies is often difficult as Ziff et al. (2015) found out in their meta-analysis that patients with a prescription of digoxin have generally more co-morbidities than patients without digoxin, potentially explaining a selection bias toward higher all-cause mortality in the digoxin group. Consequently, digoxin is still recommended by the new European Society of Cardiology (ESC) guideline for the management of AF (Hindricks et al., 2020). As several of the mentioned studies could link digoxin toxicity to elevated plasma levels a stringent dose adjustment is necessary, especially in patients with renal impairment. In conjunction with the topic of this review, a blockade of the TASK-1 current (ITASK-1) has been demonstrated by our group (Schmidt et al., 2018).
If rate control is not sufficient, the use of amiodarone can be considered. Because of its many extracardiac adverse effects (Vorperian et al., 1997; Jafari-Fesharaki and Scheinman, 1998; Cohen-Lehman et al., 2010; Papiris et al., 2010) it should represent a last resort for rate control therapy.
The optimal target heart rate for AF patients is not clearly defined. Van Gelder et al. (2010) found in the Rate Control Efficacy in Permanent Atrial Fibrillation (RACE) II study that lenient rate control with a resting heart rate of less than 110 bpm is as effective as a strict rate control with a heart rate of less than 80 bpm at rest and less than 110 bpm during moderate exercise in regard to death through cardiovascular reasons, hospitalization for stroke, heart failure, systemic embolism, bleeding, and life-threatening arrhythmic events, at least for patients with preserved left ventricular ejection fraction. Furthermore, the lenient rate control was easier to achieve and non-inferior in the endpoint of quality of life (Van Gelder et al., 2010; Groenveld et al., 2011).
In case of an unsuccessful therapy with pharmacological rate control, an ablation of the atrioventricular (AV) node or His bundle may be performed. The overall mortality for this method is low (Queiroga et al., 2003) and quality of life improves (Lim et al., 2007). Of course, after ablation of the AV node, patients remain pacemaker-dependent for the rest of their life. To prevent tachymyopathy a biventricular pacing device should be preferred in patients with reduced left ventricular ejection fraction. Therefore, AV node ablation should only be considered after a failed pharmacological approach or in patients who already have a pacemaker implanted. It further represents a worthwhile option to improve the percentage of biventricular pacing in cardiac resynchronization therapy (CRT) patients with AF.
3 Rhythm Control Therapy
Rhythm control therapy is the second main treatment strategy for AF, aiming to restore and sustain SR. Cardioversion from AF to SR can be achieved by pharmacological or electrical means (Shea and Maisel, 2002). To help maintain SR, antiarrhythmic drugs (AADs), catheter ablation, or surgical ablation (if the patient undergoes heart surgery for coronary artery bypass graft or valve replacement) are available (Hindricks et al., 2020). Furthermore, for recent-onset AF in hemodynamically stable patients, Pluymaekers et al. (2019) showed in the Rate Control vs. Electrical Cardioversion Trial 7—Acute Cardioversion vs. Wait and See (RACE 7 ACWAS) that a wait-and-see approach was non-inferior to early cardioversion in achieving SR after 4 weeks. Early cardioversion has been defined as pharmacological cardioversion (pCV) as soon as possible after presentation at the emergency department, whereas, delayed cardioversion comprised solely of rate control therapy for the first 48 h followed by cardioversion if needed. As they observed spontaneous conversion in 69% of patients in the delayed cardioversion group, no further treatment was needed for most patients.
However, while the results from the RACE 7 ACWAS trial are in support of a wait-and-see approach as a further therapy option for recent-onset AF, there is still some debate about the merits of this approach (Botto and Tortora, 2020; Capucci and Compagnucci, 2020). Capucci and Compagnucci (2020) argued against wait-and-see and give for consideration that quality of life assessment, performed after 4 weeks, does not show the initial dissatisfaction of the patients in the delayed group after discharge from the emergency department. On the other hand, this may not be the case as in many patients rate control therapy is sufficient enough for symptom relief and therefore improvement of quality of life (Botto and Tortora, 2020). Another point is patient safety, as the risk for AF complications increases with duration and some studies indicate that longer AF episodes promote the inducibility and stability of AF (Capucci and Compagnucci, 2020). In contrast, however, while active cardioversion is generally considered as safe it still has several side effects and therefore the question remains if immediate treatment is acceptable for a condition that is likely to resolve spontaneously (Botto and Tortora, 2020). In the end, the choice will also depend on practical feasibility as for the wait-and-see approach access to prompt follow-up is necessary (Botto and Tortora, 2020; Capucci and Compagnucci, 2020).
3.1 Electrical Cardioversion
Zoll et al. (1956) performed the first successful external electrical cardioversion (eCV) of ventricular fibrillation in four human patients in 1955 and around 10 years later Lown et al. (1963) described eCV of AF in 50 patients. In eCV a synchronized electrical current is applied to the heart to restore SR. However, while it is an effective method (Mittal et al., 2000; Kirchhof et al., 2002, 2005; Morani et al., 2019), it requires sedation of the patient with, e.g., benzodiazepines or propofol (Notarstefano et al., 2007; Furniss and Sneyd, 2015; Morani et al., 2019) and therefore, normally a fasting period of 6 h before eCV is needed (Nattel and Opie, 2006). Furthermore, administration of oxygen and its control with pulse oximetry as well as an ECG monitoring are required. As a result, cardioversion has to be performed in a hospital or at least a similar setting in the presence of trained professionals (Furniss and Sneyd, 2015).
About 30% of patients have another AF episode within a three-month follow-up (De Simone et al., 2003; Loricchio et al., 2007; Xia et al., 2009). Some studies showed even higher recurrence rates of up to 43% after 30 days (Malouf et al., 2005; Zarauza et al., 2006). Therefore, many patients need more than one eCV (Botkin et al., 2003; Raitt et al., 2006). Potential side effects of this procedure are, in addition to the anesthetic risk, skin burns, heart block, prolonged sinus arrest, ventricular pro-arrhythmia, epidermal necrosis, and apnea (Pagan-Carlo et al., 1997; Kowey et al., 1998; Yava et al., 2012). However, while most studies show no relevant myocardial damage from external eCV (Georges et al., 1996; Neumayr et al., 1997; Greaves and Crake, 1998; Walcott et al., 2003; Piechota et al., 2007; Lobo et al., 2018), there is injury to the myocardium by internal eCV (Noro et al., 2016; Stieger et al., 2018).
3.2 Pharmacological Cardioversion
In 1918 Walter Frey proposed the pharmacological active substance quinidine for the conversion of AF to SR (Frey, 1918). Since then, many substances were tested for their anti-arrhythmic potency so that today several AADs are available for pCV (amiodarone, disopyramide, dofetilide, dronedarone, esmolol, flecainide, ibutilide, procainamide, propafenone, quinidine, sotalol, and vernakalant; see also Supplementary Table S1 for a full list of recommended AADs) (Boriani et al., 2004; Marinelli and Capucci, 2010; Schilling, 2010; January et al., 2014; Hindricks et al., 2020). Compared to eCV, pCV is not as successful in restoring SR (Chen et al., 2013) and is connected with longer hospitalization (Dankner et al., 2009; Cristoni et al., 2011; Bellone et al., 2012; Gitt et al., 2013; Crijns et al., 2014). However, because the patients do not need to be sedated, no fasting is required (Hindricks et al., 2020). The substances recommended by the ESC for acute pCV are summarized in Table 1. Another possible drug for acute cardioversion is dofetilide, which is not available in Europe (European Medicines Agency, 2004). Each drug comes with its own drawbacks and limitations (Hindricks et al., 2020).
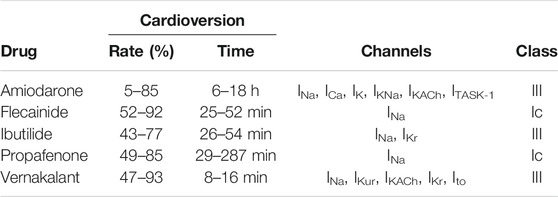
TABLE 1. Overview of recommended antiarrhythmic drugs for acute pharmacological cardioversion by the ESC with their rate and time of cardioversion, targets and class after Vaughan Williams.
In 1970 Miles Vaughan Williams proposed a system of four classes for the characterization of AADs after their mode of action (Vaughan Williams, 1984). The first class are sodium channel inhibitors which are divided in three subgroups according to their effect on APD and the strength of the channel block (Campbell and Vaughan Williams, 1983). Class Ia produces a moderate block and increased APD, class Ib a weak block and reduced APD, and class Ic a marked block and conserved APD (Lei et al., 2018). Class II drugs are comprised of β-blocker and reduce sino-atrial node pacing rates as well as slow AV node AP conduction (Dukes and Vaughan Williams, 1984). Class III drugs are potassium channel blockers that slow AP repolarization and prolong the effective refractory period (Lei et al., 2018). Lastly, Class IV drugs are calcium channel blockers which reduce heart rate and conduction, especially on the AV node and sino-atrial node (Lei et al., 2018).
3.2.1 Class Ic AADs
Flecainide and propafenone block fast sodium channel currents (INa) and belong to the class Ic AADs after Vaughan Williams (Cowan and Vaughan Williams, 1981; Vaughan Williams, 1984; Harrison, 1985; Roden and Woosley, 1986; Funck-Brentano et al., 1990; Wang et al., 1992). Flecainide has been shown to be successful in cardioversion of recent-onset AF in 52–92% of cases depending on the observation period. The mean time to cardioversion ranged from 110 to 190 min after oral administration and 25–52 min after intravenous infusion (Madrid et al., 1993; Reisinger et al., 1998; Alp et al., 2000; Martínez-Marcos et al., 2000; Khan, 2003; Reisinger et al., 2004; Balla et al., 2011; Bash et al., 2012). Flecainide has been shown to be superior to propafenone and amiodarone in both time and rate of cardioversion (Martínez-Marcos et al., 2000; Balla et al., 2011), similar to ibutilide (Reisinger et al., 2004), and inferior to vernakalant (Pohjantähti-Maaroos et al., 2019). The most common adverse events during a therapy with flecainide are dizziness (30%), visual disturbance (28%), headache (9%), and nausea (9%). Pro-arrhythmia has been observed in 7–8% of patients (Holmes and Heel, 1985).
Propafenone has a cardioversion rate of 49–85% in recent-onset AF, depending on the observation time point and the route of administration, and a mean time of cardioversion of 29–30 min after intravenous and 166–287 min after oral administration (Capucci et al., 1999; Madonia et al., 2000; Martínez-Marcos et al., 2000; Khan, 2001; Zhang et al., 2005; Balla et al., 2011; Conde et al., 2013). Studies comparing propafenone to other drugs showed an inferiority to vernakalant in both time and rate of cardioversion (Conde et al., 2013) as well as flecainide (Martínez-Marcos et al., 2000; Balla et al., 2011), to ibutilide in rate with a non-significant inferiority in time (Zhang et al., 2005) and superiority to amiodarone in rate and time (Martínez-Marcos et al., 2000; Balla et al., 2011). Adverse events observed under the treatment of propafenone mostly affect the cardiovascular, gastrointestinal and nervous system. The most common adverse effects are dizziness (12.5%), nausea/vomiting (10.7%), unusual taste (8.8%), constipation (7.2%), and fatigue (6.0%). The most frequent cardiac adverse events are pro-arrhythmia (4.7%), angina (4.6%), congestive heart failure (3.7%), and ventricular tachycardia (3.4%) (Capucci and Boriani, 1995).
Propafenone and flecainide should not be used in patients with structural heart diseases as they are associated with a high pro-arrhythmic risk and increase mortality in these cases as demonstrated for flecainide in the Cardiac Arrhythmia Suppression Trial (CAST) (Morganroth, 1987; Echt et al., 1991; Podrid and Anderson, 1996; Andrikopoulos et al., 2015; Tamargo et al., 2015; Hindricks et al., 2020). Furthermore, in most trials structural heart diseases have been an exclusion criterion (Khan, 2003).
3.2.2 Class III AADs
Amiodarone is the original representative of class III AADs as it leads to prolongation of the APD (Vaughan Williams, 1984; Harrison, 1985). It has a cardioversion rate for recent-onset AF of about 5–85% and a mean time to cardioversion of 6–18 h after intravenous application (Noc et al., 1990; Joseph and Ward, 2000; Martínez-Marcos et al., 2000; Kafkas et al., 2007; Balla et al., 2011; Camm et al., 2011; Savelieva et al., 2013). In studies directly comparing amiodarone against flecainide and propafenone as well as vernakalant it was inferior in both time and rate of cardioversion (Martínez-Marcos et al., 2000; Balla et al., 2011; Camm et al., 2011). Compared to ibutilide it has been inferior in time but the difference in rate has not been statistically significant (Kafkas et al., 2007). However, it is important to note that especially in studies comparing amiodarone against faster acting AADs the window of observation has an impact on the outcome. Some studies stopped after 90 min whereas the effect of amiodarone takes a longer time, resulting in low cardioversion rates of amiodarone compared to studies with a longer observation period (Martínez-Marcos et al., 2000; Balla et al., 2011; Camm et al., 2011).
Amiodarone is a multi-channel blocker, inhibiting inward sodium (INa) and calcium channel currents (ICa) as well as voltage- and ligand-gated potassium channel currents (IK, IKNa, IKACh) (Kodama et al., 1999) and the two-pore domain potassium channel TASK-1 (ITASK-1) (Gierten et al., 2010). There are numerous side effects described in the literature, whereas hypothyroidism is the most common with a rate of up to 22%. (Amiodarone Trials Meta-Analysis Investigators, 1997; Kodama et al., 1999; Goldschlager et al., 2007). Another serious adverse effect is pulmonary toxicity (Wolkove and Baltzan, 2009) with dose-dependent incidence rates of about 2–6% (Dusman et al., 1990; Goldschlager et al., 2007). Other side effects are a blue discoloration and photosensitivity of the skin, photophobia, halo visions as well as optic neuropathy, gastrointestinal side effects, bradycardia, AV-block, and pro-arrhythmia (Goldschlager et al., 2007; Camm and Savelieva, 2008). However, most of these side effects are mainly observed during prolonged therapy and not in the setting of acute cardioversion (Singh, 1996; Wolkove and Baltzan, 2009).
To reduce the side effects of amiodarone, the substance has been modified (Nattel and Singh, 1999). Removal of the two iodine atoms and the addition of a methanesulfonyl group results in dronedarone, which has similar electrophysiological effects as amiodarone (Sun et al., 1999, 2002). It is a multi-channel blocker acting on potassium (IKr, IKs, IK1, IKACh, ITASK-1, ISUS), sodium (INa), calcium (ICa), and hyperpolarization-activated cyclic nucleotide-gated channels (If) (Thomas et al., 2003; Kathofer et al., 2005; Bogdan et al., 2011; Schweizer et al., 2011; Schmidt et al., 2012).
In the ATHENA trial (A placebo-controlled, double-blind, parallel arm Trial to assess the efficacy of dronedarone 400 mg bid for the prevention of cardiovascular Hospitalization or death from any cause in patiENts with Atrial fibrillation/atrial flutter) a decrease in hospitalization due cardiovascular events or death in patients with AF that are treated with dronedarone has been found (Hohnloser et al., 2008, 2009). However, contrary to the expectations the PALLAS (Permanent Atrial Fibrillation Outcome Study Using Dronedarone on Top of Standard Therapy) trial had to be stopped for safety reasons because patients with permanent AF in risk of major vascular events had an increased rate of heart failure, stroke, and death of cardiovascular reasons under treatment with dronedarone compared to placebo (Connolly et al., 2011). It also increased mortality in patients with symptomatic heart failure and severe left ventricular systolic dysfunction (Køber et al., 2008). Today, dronedarone is not indicated for the use in acute cardioversion of AF (European Medicines Agency, 2019).
Further class III AADs are the methanesulfonamide derivatives ibutilide and dofetilide (Murray, 1998; Mounsey and DiMarco, 2000). Ibutilide prolongs the APD through blockade of the rapid component of cardiac delayed rectifier potassium currents (IKr) and the activation of slow delayed inward sodium currents (INa) (Murray, 1998; Glatter et al., 2001). It is associated with a risk for torsades de pointes of about 5% (Kowey et al., 1996; Nattel and Singh, 1999; Gowda et al., 2003; Nair et al., 2011). The cardioversion rate of ibutilide in recent-onset AF is reported to be around 43–77% and the mean time to cardioversion after intravenous infusion 26–54 min (Gowda et al., 2003; Kafkas et al., 2007; Simon et al., 2017; Vogiatzis et al., 2017). Kafkas et al. (2007) showed in their study that ibutilide is superior in cardioversion time to amiodarone with no significant difference in cardioversion rate and Zhang et al. (2005) showed a superiority to propafenone in cardioversion rate but not time. It is similar to flecainide (Reisinger et al., 2004) and inferior to vernakalant (Simon et al., 2017; Vogiatzis et al., 2017) in time and rate of cardioversion.
Dofetilide inhibits the rapid component of the delayed rectifier potassium current (IKr) (Mounsey and DiMarco, 2000; Roukoz and Saliba, 2007). Similar to ibutilide it has a risk for torsades de pointes of 0.3–10.5% (Falk et al., 1997; Nørgaard et al., 1999; Bianconi et al., 2000; Lenz and Hilleman, 2000). The rate of cardioversion is between 12.5 and 53% depending on drug dose and the duration of AF and the mean time to cardioversion is 40–55 min (Suttorp et al., 1992; Sedgwick et al., 1995; Falk et al., 1997; Nørgaard et al., 1999; Bianconi et al., 2000). It is safe for patients after myocardial infarction as well as congestive heart failure and left ventricular systolic dysfunctions (Shenasa and Shenasa, 2016).
Vernakalant is effective in conversion of recent-onset AF with a rate of 47–70% in the first 90 min after intravenous infusion, in small, non-randomized trials up to 93%. The median time to cardioversion is around 8–16 min (Roy et al., 2004, 2008; Kowey et al., 2009; Camm et al., 2011; Bash et al., 2012; Conde et al., 2013; Savelieva et al., 2013; Torp-Pedersen et al., 2013; Carbajosa et al., 2017; Simon et al., 2017; Vogiatzis et al., 2017; Kossaify, 2019; Pohjantähti-Maaroos et al., 2019). It is superior to ibutilide (Simon et al., 2017; Vogiatzis et al., 2017), propafenone (Conde et al., 2013), amiodarone (Camm et al., 2011) and flecainide (Pohjantähti-Maaroos et al., 2019) in both cardioversion rate and time to cardioversion. However, the effect on AF with a duration of more than seven days is reduced (Torp-Pedersen et al., 2013).
Vernakalant blocks sodium currents (INa) in a frequency and voltage-dependent manner. At normal heart rates and membrane resting potentials the inhibition is low but increases with faster rates and less negative potentials as seen in the atria during AF compared to the ventricle. Furthermore, vernakalant blocks atrial-specific ultra-rapid delayed rectifier potassium currents (IKur), acetylcholine-activated atrial potassium currents (IKACh) and transient outward potassium currents (Ito). An inhibition of the rapid component of cardiac delayed rectifier potassium currents (IKr) has been observed in vitro but this effect seems to be too small to have therapeutic relevance. Taken together, this leads to an almost atrial-selective effect with no ventricular pro-arrhythmic effects (Fedida et al., 2005; Fedida, 2007). Serious adverse effects of vernakalant are hypotension and AV-block (Kowey et al., 2009; Savelieva et al., 2013; Torp-Pedersen et al., 2013). It is safe in use for patients with a history of ischemic heart disease as long as they have no hypotension or severe aortic stenosis (Savelieva et al., 2013; Torp-Pedersen et al., 2013) but the risk for adverse effects may be higher in patients with heart failure (Savelieva et al., 2013).
3.3 Catheter Ablation
An alternative to drug treatment is catheter ablation (Hindricks et al., 2020). This method is based on Haissaguerre et al. (1998) who described triggers for paroxysmal AF in the pulmonary veins and the termination of AF through their ablation by radio-frequency. Pulmonary vein isolation (PVI) is superior to AADs in maintaining SR with a comparable rate of complications (Cosedis Nielsen et al., 2012; Mont et al., 2014). However, only about 40% of patients are free from AF after a six year follow-up period and about 60% needed more than one procedure (Sorgente et al., 2012). Isolation of pulmonary veins can be achieved via radio frequency ablation or cryoballoon ablation. Kuck et al. (2016) compared both methods and found that cryo-ablation is non-inferior to radio-frequency ablation. However, the primary safety endpoint of death, cerebrovascular events, and serious treatment-related adverse events has been reached in over 10% of patients in both ablation groups (Kuck et al., 2016). To test if catheter ablation is superior to pharmacological drug therapy, the Catheter Ablation vs. Anti-arrhythmic Drug Therapy for Atrial Fibrillation (CABANA) trial has been initiated. The primary endpoint of the study was total mortality, disabling stroke, serious bleeding, or cardiac arrest (Packer et al., 2018). The trial shows no significant reduction of this endpoint with catheter ablation compared to anti-arrhythmic drug therapy. However, major limitations of this trial are a lower than expected event rate and treatment crossovers that require consideration when interpreting the results (Packer et al., 2019).
A similar trial but with a narrower patient population is the Catheter Ablation vs. Standard Conventional Treatment in Patients with Left Ventricular Dysfunction and Atrial Fibrillation (CASTLE-AF) trial that compared catheter ablation to pharmacological standard therapy in patients with AF and left ventricular dysfunction. The primary endpoint was the composite of all-cause mortality or worsening of heart failure requiring unplanned hospitalization (Marrouche and Brachmann, 2009). The results of the trial are a significant reduction in all-cause mortality and rate of hospitalizations for worsening of heart failure in the ablation group (13.4%) compared to the medication group (25.0%) (Marrouche et al., 2018). This trial, however, comes with a couple of possible pitfalls such as (I) missing data on the quality of rate control in patients in the medication group, (II) the late appearing effect in the all-cause mortality where only about 50% of the patients remained in the follow-up, (III) significant differences in patient characteristics and, (IV) the fact that some sub-groups like patients including an ejection fraction of less than 25% did not appear to have a benefit from ablation. Furthermore, all patients had symptomatic AF with previously failed AAD therapy or were intolerant or unwilling to take AADs (Bono and Kirchhof, 2018).
Catheter ablation has potential periprocedural complications such as pericarditis, pericardial effusion, cardiac tamponade, stroke, pulmonary vein stenosis, severe esophageal injury leading to atrioesophageal fistula, phrenicus lesions, and pacemaker dependency (Sacher et al., 2006; Cosedis Nielsen et al., 2012; Mont et al., 2014; Han et al., 2017; Kapur et al., 2017; Yetter et al., 2017; Hindricks et al., 2020). These severe adverse events occur in up to 7% of patients and up to 3% will even have life-threatening complication whereof less than 0.2% die (Dagres et al., 2009; Cappato et al., 2010; Gupta et al., 2013).
Electrical and pharmacological cardioversion as well as catheter ablation all have their own drawbacks and limitations as mentioned above. Especially the risk of potentially life-threatening ventricular arrhythmia is a significant limitation for the use of AADs. Therefore, there is a need for new potential targets and drugs for the treatment of AF (Ravens, 2010; Schmidt et al., 2011). In this regard the identification of atrial selective drug targets would be essential to overcome ventricular side effects of AAD therapy (Peyronnet and Ravens, 2019). In the following, this review will focus on the TASK-1 channel, a potassium channel that shows atrial specific expression and is associated with a characteristic electrical remodeling of atrial tissue in AF (Schmidt et al., 2015).
4 Electrical Remodeling
Electrical remodeling is part of the cardiac response to functional and structural stressors with the initial aim of maintaining and compensating cardiac performance (Cutler et al., 2011). However, over time, this compensation mechanism turns maladaptive and leads to arrhythmia and pump failure (Cutler et al., 2011). This process could be shown in the atrium of goats with artificially induced AF resulting in a shortening of the atrial effective refractory period (AERP), a reversion of its physiological rate adaptation, and an increase in rate, inducibility and stability of AF (Wijffels et al., 1995). For this phenomena Wijffels et al. (1995) coined the phrase “Atrial fibrillation begets atrial fibrillation” as they could show that AF induced remodeling leads to a progression of AF.
The alterations in cardiac electrical function summarized as electrical remodeling are the subject of current research and not yet completely understood (Nattel et al., 2020). Altered calcium handling through the reduction of the L-type calcium channel current (ICaL) has been observed (Christ et al., 2004) as well as alterations in potassium channel currents, for example upregulation of the inward-rectifier potassium channel Kir2.1 and therefore increase of IK1 (Gaborit et al., 2005), decrease of the transient outward potassium current Ito (Nattel et al., 2007), suppression of the ultrarapid delayed rectifier current IKur (Nattel et al., 2008), an increase of the slow delayed rectifier current IKs (Caballero et al., 2010), and a decreased expression of Small-conductance Ca2+-activated K+ (SK) channels (Yu et al., 2012). Beside changes in ion-channel expression there are also alterations in other cellular structures. For example, the expression of gap junction protein Conexin43 is suppressed in AF (Bikou et al., 2011).
To describe all the molecular alterations that take place in electrical remodeling during AF would be beyond the scope of this review. Therefore, we will focus on TASK-1, a newly identified potassium channel that is associated with a typical upregulation during AF.
5 Two-Pore-Domain Potassium Channels
Hodgkin and Huxley already proposed the presence of leak currents in 1952 (Hodgkin and Huxley, 1952) but it took another 40 years to identify the K2P channels as being responsible for this instantaneous and non-inactivating current, making them the newest identified group of potassium channels (Duprat et al., 1997; Lesage and Lazdunski, 2000; Goldstein et al., 2001, 2005). Until today, the channel family comprises 15 members with a common structure element of two pore-forming (P) loops and four transmembrane domains (M) per subunit (Ketchum et al., 1995; Goldstein et al., 2005). On the cell membrane they assemble as hetero- and homodimers and are regulated by a variety of different stimuli such as signaling lipids, temperature, pressure, pH-level, and a number of pharmaceutical substances (Lesage and Lazdunski, 2000; Goldstein et al., 2001; Lesage, 2003; Dedman et al., 2009; Feliciangeli et al., 2015). However, they are relatively insensitive to typical potassium channel blockers such as tetraethylammonium, 4-aminopyridine, cesium, and barium ions (Lesage, 2003). The different members of the family show either a weak inward (Lesage et al., 1996), open (Duprat et al., 1997), or outward rectification (Maingret et al., 2002).
K2P channels are involved in ion homeostasis, hormone secretion, cell development, and excitability. Therefore, these channels play a role in many physiological and pathological mechanism such as vascular and pulmonary hypertension, cardiac arrhythmia, nociception, neuroprotection, taste and temperature sensing, anesthesia, apoptosis, carcinogenesis, and depression (Patel and Honore, 2001; Bayliss and Barrett, 2008; Enyedi and Czirják, 2010; Lloyd et al., 2011; Williams et al., 2013; Feliciangeli et al., 2015; Kim and Kang, 2015).
5.1 TASK-1 in Atrial Fibrillation
TASK-1 is a member of the K2P channel family and represents a promising target for AF therapy (Limberg et al., 2011; Schmidt et al., 2015, 2017). It is predominantly expressed in the human atria (Schmidt et al., 2015, 2017). Higher mRNA expression levels in the left atrium (14-fold) and right atrium (16-fold) of humans compared to human left ventricular tissue samples were measured (Schmidt et al., 2015). Furthermore, it was shown that atrial tissue undergoes an electrical remodeling process during AF—indicating an atrial cardiomyopathy—that leads to a strong upregulation of TASK-1 mRNA and protein levels, which are observed on a functional level as well (Barth et al., 2005; Schotten et al., 2011; Schmidt et al., 2015).
Furthermore, the current density of TASK-1 in isolated human atrial cardiomyocytes was 3 times higher in patients with chronic AF compared to SR. In paroxysmal AF patients, an increase by 1.5-fold was observed (Schmidt et al., 2015). Inhibition of TASK-1 currents in isolated human atrial cardiomyocytes of patients with chronic AF leads to a prolongation of the APD of up to 30% (Schmidt et al., 2015). In combination of these two facts it could be shown that an upregulation of TASK-1 leads to a shortening of the APD in patients with chronic AF (Schmidt et al., 2015, 2017). This could be verified by the application of A293, a specific TASK-1 inhibitor. Whereas, A293 had almost no effect on the APD of atrial cardiomyocytes from patients with SR or paroxysmal AF, it has been able to prolong it in patients with chronic AF to a duration very similar to SR (Schmidt et al., 2015). Taken together, the TASK-1 current participates predominantly in the atrial APD shortening of patients with AF. This property indicates a promising new anti-arrhythmic target to inhibit AF.
These experimental findings have been verified in a computational model of a human atrial cardiomyocyte. For this purpose, the model from Grandi et al. (2011) has been modified to include the TASK-1 currents. The model also reproduced that shortening of the APD in chronic AF through higher expression of TASK-1 has pro-arrhythmic effects (Schmidt et al., 2015, 2017). In contrast to AF patients, a downregulation of TASK-1 has been observed in patients with severe impairment of left ventricular function, leading to the assumption that a therapy with TASK-1 inhibitors would have a smaller effect on patients with paroxysmal AF and severely impaired left ventricular function compared to patients with a normal left ventricular function (Schmidt et al., 2015, 2017).
In a porcine animal model it could be shown that pigs with artificially induced AF via atrial burst stimulation by an implanted pacemaker using a biofeedback pacing algorithm developed similar upregulation of TASK-1 and shortening of the APD as seen in human patients. Pigs were treated with an adeno-associated viral vector carrying anti–TASK-1-siRNA which was injected in both atria and led to a reduced expression of TASK-1. This treatment led to a prolongation of the APD and reduction of TASK-1 current in the siRNA group compared to AF control pigs and to a significant reduction in AF burden (Schmidt et al., 2019b).
Liang et al. (2014) showed that loss-of-function mutations of TASK-1 lead to increased susceptibility to develop AF in the presence of pro-arrhythmic stimuli and Harleton et al. (2015, 2013) observed a phosphorylation dependent TASK-1 dysregulation in a canine model of AF, further underlining its role in AF.
While these data clearly show the role of TASK-1 in the pathophysiology of AF, this is not the case for the other TASK channels (Rinné et al., 2015; Schmidt et al., 2015). For example, tandem of P domains in a weak inward rectifying K+ channel (TWIK)-related acid sensitive K+ channel 3 (TASK-3) that has long been known as a non-cardiac channel (Putzke et al., 2007; Schmidt et al., 2015), has been found in expression analysis of human atrial auricles (Rinné et al., 2015). However, in patch-clamp experiments, performed on isolated rat cardiomyocytes, no functional evidence for TASK-3 currents could be obtained (Putzke et al., 2007). Rinné et al. (2015) confirmed these results in human cardiomyocytes, finding only TASK-1 currents and intermediate currents between TASK-1 and TASK-3, concluding that TASK-3 forms heterodimers with TASK-1 in human cardiomyocytes. Compared to TASK-1 the cardiac expression of TASK-3 was shown to be substantially lower, therefore, the role of TASK-3 in the atrial AP remains to be seen (Rinné et al., 2015; Schmidt et al., 2015). Finally, for the other TASK channels there is no data available showing an involvement in the atrial AP, suggesting that they are unsuitable targets for AF therapy (Gurney and Manoury, 2009; Schmidt and Peyronnet, 2018; Peyronnet and Ravens, 2019).
Beside TASK-1, other atrial-selective channels have been proposed as possible drug targets in the therapy of AF (Ravens, 2017). The first such candidate was the voltage-gated outward-rectifying K+ channel Kv1.5 (Fedida et al., 1993). Courtemanche et al. (1999) predicted a prolongation of the atrial APD through inhibition of Kv1.5 in a mathematical AF model. However, experimental validation led to mixed results depending strongly on AF induced electrical remodeling (Wettwer et al., 2004; Ravens, 2017; Schmidt and Peyronnet, 2018). In human SR samples an inhibition of Kv1.5 shortened the atrial APD, possibly resulting in the maintenance of reentrant excitation (Loose et al., 2014). Contrary, in AF samples a prolongation of the atrial APD was observed upon Kv1.5 inhibition (Loose et al., 2014). However, since Kv1.5 is—in contrast to TASK-1— downregulated in chronic AF patients, the possible therapeutic effect of Kv1.5 inhibition is in question (Van Wagoner et al., 1997; Schmidt and Peyronnet, 2018). Therefore, in accordance with these data, no clinical studies that show a definite reduction of AF burden have been reported (Ravens, 2017; Schmidt and Peyronnet, 2018).
Another group of channels discussed in the context of atrial-selectivity are the SK channels (Ravens, 2017). However, while SK 1 and SK 2 seem to be more abundant expressed in the atria, SK 3 is uniformly expressed in the atrium and ventricle, question if SK channels are really atrial-selective (Tuteja et al., 2005). Furthermore, the role of SK channels in modifying the shape of the atrial AP in AF patients remains far from clear as both, AF-related up- and downregulation have been observed (Skibsbye et al., 2014; Ravens, 2017; Schmidt and Peyronnet, 2018). Therefore, while inhibition of SK channels is expected to prolong the APD and consecutively protect against re-entry (Diness et al., 2010), pro-arrhythmic events have also been observed (Hsueh et al., 2013). Taken together, these data question the possible usage of SK channel blockers in AF therapy (Ravens, 2017; Schmidt and Peyronnet, 2018).
In conclusion, the TASK-1 channel represents a promising target for future therapy of AF since it is atrial-specific expressed, which reduces the risk of pro-arrhythmic effects in the ventricle, and has a strong influence on atrial APD in AF patients (Ravens, 2010; Schmidt et al., 2014b, 2015).
5.2 TASK-1 in Pulmonary Hypertension
Besides the heart, the TASK-1 channel is expressed in the liver, placenta, prostate, kidney, lung, pancreas, ovary, small intestine, and brain (Lesage and Lazdunski, 2000; Williams et al., 2013). Therefore, it is involved in a variety of physiological and pathophysiological processes. For example, TASK-1 plays a role in pulmonary hypertension (PH). PH is defined as an elevation of the mean pulmonary arterial pressure of more than 20 mmHg and a pulmonary vascular resistance greater or equal 3 Wood (Simonneau et al., 2019). It is a progressive disease that leads to right ventricular failure and ultimately to death (Humbert et al., 2004; Gaine and McLaughlin, 2017) with a 3-years survival of 68% (Hurdman et al., 2012). PH is caused by pathological remodeling of the pulmonary arteries, primarily through the excessive proliferation of pulmonary arterial smooth muscle cells (PASMCs). This leads to vasoconstriction in conjunction with an increase of pulmonary vascular resistance, followed by destruction of small-resistance pulmonary arteries and arterioles, and ends in right ventricular failure (Crosswhite and Sun, 2014; Thompson and Lawrie, 2017).
Potassium channels play an important role in PH, as decreased potassium channel activity in PASMCs increases resistance to apoptosis, cell proliferation and vasoconstriction leading to vascular remodeling (Boucherat et al., 2015; Olschewski et al., 2017). Olschewski et al. (2006) showed in isolated primary PASMCs that a knockdown of TASK-1 leads to depolarization of the cells and therefore to a higher pulmonary muscle tone. Ma et al. (2013) described six heterozygous miss-sense mutations of TASK-1, leading to loss of function, causing PH in patients. Navas Tejedor et al. (2017) found two homozygous mutations of TASK-1 in a Spanish patient cohort associated with a severe and early form of PH. Cunningham et al. (2019) could show that these two mutations lead to severely reduced TASK-1 channel current compared to wild type. While the guanylate cyclase activator, riociguat, a novel treatment for PH, enhances TASK-1 wild type channel current, it had no effect on the mutated channels (Cunningham et al., 2019).
Whether pharmacological inhibition of TASK-1 can also lead to PH is still not finally clarified (Olschewski et al., 2016; Schmidt et al., 2016; Wiedmann et al., 2021). Olschewski et al. (2016) give for consideration that endothelin-1, a strong endogenous inhibitor of the TASK-1 channel, can induce PH as well as dasatinib, a src tyrosine kinase inhibitor that inactivates the TASK-1 channel (Tang et al., 2009; Montani et al., 2012; Seyler et al., 2012; Nagaraj et al., 2013). As these data were not validated in in vivo animal models or human studies, the net effect of TASK-1 current on pulmonary muscle tone was largely unknown (Schmidt et al., 2016). Very recently, however, our group published some preliminary data on the hemodynamic effect of TASK-1 inhibition in a pig model. A mild increase in pulmonary artery pressure was observed upon acute TASK-1 inhibition (Wiedmann et al., 2021). Therefore, this potential side effect has to be considered in the development of TASK-1 inhibitors for future treatment of AF (Schmidt et al., 2016).
5.3 TASK-1 in Sleep Apnea
In addition to the involvement of TASK-1 in the pathophysiology of AF and PH, it also plays a role in sleep apnea. Sleep apnea is defined as pauses of breathing for more than 10 s with a stop of the airflow at nose and mouth of more than five times per hour and a partial neurological arousal (Guilleminault et al., 1976; Costanzo et al., 2015). There are three types of sleep apnea, obstructive sleep apnea (OSA), which originates in the obstruction of the upper airways (Laratta et al., 2017), central sleep apnea (CSA) with a temporary withdrawal of the brainstem-driven respiratory drive resulting in a stop of the activity of the respiratory muscle and therefore the airflow (Costanzo et al., 2015), and a mixed form of both (Gastaut et al., 1966).
OSA has a prevalence of 46% in the German population over the age of 20 years, whereas, only 6% suffer from OSA syndrome (Fietze et al., 2019). Most common symptoms are daytime sleepiness, unrefreshing sleep, and fatigue (Laratta et al., 2017). OSA is connected with an increased risk of myocardial infarction, congestive heart failure, coronary revascularization procedures, and cardiovascular death (Redline et al., 2010; Hla et al., 2015). Furthermore it is associated with insulin resistance, therapy-resistant hypertension and AF (Bradley and Floras, 2003; Somers et al., 2008; Digby and Baranchuk, 2012; Hohl et al., 2014; Jullian-Desayes et al., 2015).
CSA has a high prevalence in patients with heart failure of about 30–40% (Javaheri, 2006; Oldenburg et al., 2007; Macdonald et al., 2008). The symptoms of CSA are similar to OSA with insomnia, fatigue, and sometimes daytime sleepiness (Costanzo et al., 2015). One of the risk factors for CSA is AF besides male sex, lower ejection fraction, waking hypocapnia, higher New York Heart Association functional class, and higher B-type natriuretic peptide levels (Javaheri et al., 1998; Sin et al., 1999; Oldenburg et al., 2007; Calvin et al., 2011; Costanzo et al., 2015).
The detection of pH and blood gas is an essential part of the feedback control required for spontaneous breathing (Feldman et al., 2003). The sensing of O2 occurs in the carotid bodies in the periphery whereas CO2 is mainly measured by central chemoreceptors in the brainstem (Richerson, 1995; Nattie, 1999; Ballantyne and Scheid, 2000; Feldman et al., 2003). The CO2 central chemoreceptor (CCR) has a high sensitivity for the partial pressure of carbon dioxide (pCO2), as a rise of only 1 mmHg in pCO2 increases ventilation by 20–30% (Feldman et al., 2003). So far there has been no evidence of a direct sensing of CO2; in contrast CO2 sensing occurs through measuring of the pH-level (Feldman et al., 2003; Jiang et al., 2005). There are many proteins sensitive to changes in pH, for example low resistance gap junctions (Dean et al., 2001, 2002; Solomon et al., 2001; Solomon and Dean, 2002), inward rectifier potassium channels (Zhu et al., 2000; Jiang et al., 2001), pH-sensitive membrane ion-transport proteins (Putnam, 2001; Wiemann and Bingmann, 2001), and TASK channels (Bayliss et al., 2001; Washburn et al., 2002). Inhibition of TASK-1 by extracellular acidosis leads to depolarization of the membrane and increases excitability of the cell (Wang et al., 2008). Furthermore, Mulkey et al. (2004) could show that neither inwardly rectifying nor calcium-activated potassium channels but background potassium channels are responsible for the chemosensitivity of cells in the retrotrapezoid nucleus of rats. In addition, it has been shown by Patel et al. (1999) that halothane and isoflurane, two volatile anesthetics, activate TASK channel current. Furthermore, Takahashi et al. (2005) observed that halothane reduces the response to hypoxia, as hypoxia leads to an inhibition of TASK-channel currents, further suggesting a role of TASK in the chemosensing of the carotid body. However, Mulkey et al. (2007) suggested a few years later, that there had to be some other potassium channel, beside TASK-channels, responsible for the central respiratory chemosensitivity. While the involvement of TASK-1 may not be critical for the central respiratory chemosensitivity, it appears to be required for the pH sensing by the carotid body (Trapp et al., 2008; Guyenet et al., 2010). Nevertheless, an involvement of TASK-1 in ventilatory control via modulation of the respiratory output reacting to chemical stimuli seems likely (Wang et al., 2008).
OSA as well as CSA result from an unstable chemical control system, therefore leading to instability of the ventilation control (Hudgel et al., 1998; Younes et al., 2001; Wang et al., 2008). Wang et al. (2008) could show that TASK-1 plays a role in spontaneous sleep apnea in rats. They found a positive relation between TASK-1 protein content in the brainstem and the spontaneous sleep apnea index. They suggested an elevation of TASK-1 expression in the brainstem may lead to an increased occurrence of sleep apnea due to unstable respiratory control. This might be due to an augmented sensitivity of the CCR via heightened TASK-1 expression, resulting in an over-sensing of pCO2 changes, leading to an over-correction of the negative-feedback-loop and therefore to sleep apnea or other breathing disorders (Khoo et al., 1982; Khoo, 2000; Wang et al., 2008).
However, TASK-1 does not only assemble as homodimers but also as heterodimers with TASK-3. They share about 62% of their amino acid sequence (Rajan et al., 2000; Berg et al., 2004). Therefore, there still is some debate about whether only TASK-1 and heterodimers of TASK-1 and TASK-3 are involved in the breathing regulation, as it seems to be the case in rodents (Trapp et al., 2008; Wang et al., 2008) or if homodimers of TASK-3 are involved as was recently suggested by Cunningham et al. (2019).
Because the TASK-1 channel is involved in many pathological processes, a modulation of the channel by drugs could be a promising strategy against AF, sleep apnea or drug-induced ventilatory depression, sleep disorders, neurodegenerative disorders, major depression, and/or malignancy (Chokshi et al., 2015; Kiper et al., 2015; Wiedmann et al., 2016).
5.4 Inhibitors of TASK-1
Many inhibitors of TASK-1 have been originally developed as inhibitors of the voltage-gated potassium channel Kv1.5 (Kiper et al., 2015). Kv1.5 has been proposed as a promising target for the therapy of AF because it is expressed in the atrium but not the ventricle and its inhibition prolongs the atrial AP but does not influence ventricular repolarization (Decher et al., 2004, 2006). It plays a critical role in the AP repolarization of human atrial myocytes as it contributes to the delayed rectifier sustained outward current (IKsus) (Fedida et al., 1993; Wang et al., 1993). In this context TASK-1 has been discussed as a target for AF as it contributes to IKsus as well, even though to a lesser extent than Kv1.5 (Limberg et al., 2011; Kiper et al., 2015).
Furthermore, it could be shown that the Kv1.5 blocker AVE0118 is effective against sleep apnea in a pig model of upper airway collapse induced through negative pressure (Wirth et al., 2013). However, it has been found that the Kv1.5 blockers A1899 (also known as S20951), A293 (also known as A1231), and S9947 are much more potent TASK-1 inhibitors (Knobloch et al., 2002, 2004; Putzke et al., 2007; Streit et al., 2011).
TASK-1 binds its inhibitors highly selective, with strong affinity and extremely low washout rates. Recently, Rödström et al. (2020) have been able to solve the structure of human TASK-1 by X-ray crystallography. They could show that high-affinity inhibitors are trapped in a vestibule below the selectivity filter by an X-gate, leading to the above mentioned low washout rates (Rödström et al., 2020). The entrapment has to be considered when interpreting data from pharmacokinetic-pharmacodynamic studies as inhibitors will remain bound and active for a longer time than predicted by these studies.
Given the role of TASK-1 in AF, it is not surprising that both AVE0118 as well as A293 among others have been reported as being effective against AF in animal models (Knobloch et al., 2002, 2004; Wirth et al., 2007). (For a complete list of all TASK-1 inhibitors tested in animal or human studies for their possible use in AF treatment see Table 2.) It has been shown that the porcine variant of TASK-1 is pharmacologically and functionally very similar to the human variant as they share about 97% of their identity as well as their selective expression in the atria (Schmidt et al., 2014a; Wiedmann et al., 2020). Therefore, our group tested A293 in a porcine model of paroxysmal AF. In this model AF was induced via right atrial burst pacing by implanted pacemakers. We could show in patch-clamp experiments on isolated cardiomyocytes from pigs with paroxysmal AF that inhibition of TASK-1 via application of A293 leads to prolongation of the APD. Furthermore, we observed that intravenous administration of A293 led to a significant prolongation of the atrial effective refractive period. We have also been able to restore sinus rhythm after elicitation of AF episodes by application of A293 with cardioversion times of about 3 min (Wiedmann et al., 2020). Similar results could be observed in an animal model of persistent AF (Wiedmann et al., 2021). To sum up, blockage of TASK-1 currents by A293 leads to anti-arrhythmic effects and acute cardioversion in vivo (Wiedmann et al., 2020, 2021).
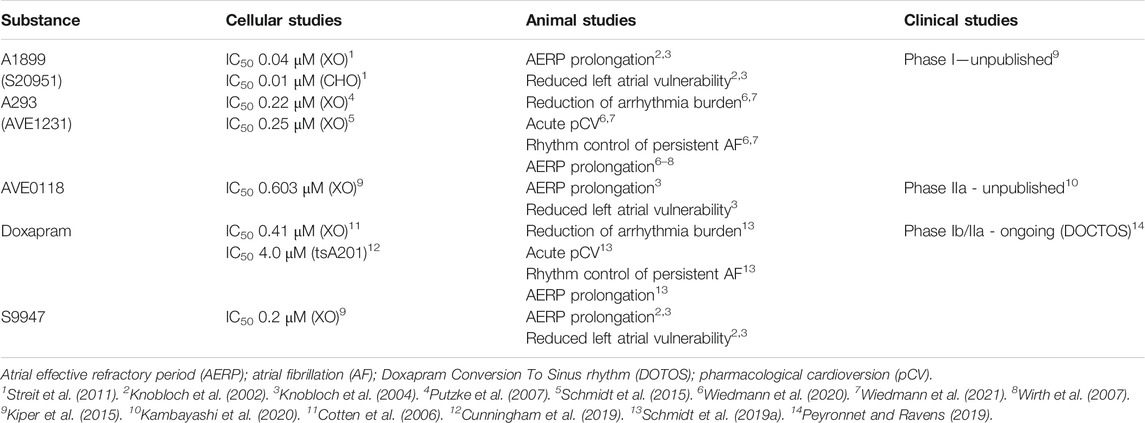
TABLE 2. List of TASK-1 inhibitors that have been tested in animal or human studies for their possible use in atrial fibrillation treatment. For IC50 value measurement the humanTASK-1 channel has been heterogeneously expressed in Xenopus laevis oocytes (XO), chinese hamster ovarian cells (CHO) or human embryonic kidney cells (tsA201). For the IC50 measurements of doxapram in X. leavis oocytes ratTASK-1 has been used, no measurements with hu manTASK-1 in oocytes are published.
5.4.1 Doxapram
Another potent TASK-1 inhibitor is doxapram (Cotten et al., 2006). It has been developed in the 1960s for use in humans and animals as a drug to stimulate breathing and activate the carotid body (Lunsford et al., 1964). Doxapram is used as a breathing stimulant in patients with moderate to severe ventilatory failure to reverse hypercapnia and hypoxemia (Edwards and Leszczynski, 1967; Yost, 2006) as well as in patients with chronic obstructive pulmonary disease (Moser et al., 1973). In a clinical case study doxapram has been proposed as treatment for OSA (Houser and Schlueter, 1978; Yost, 2006). Furthermore, doxapram arouses patients after anesthesia and drug-induced central nervous system depression because of barbiturates, volatile anesthetics, nitrous oxide, and benzodiazepines (Evers et al., 1965; Riddell and Robertson, 1978; Rappolt Sr et al., 1980; Yost, 2006) and has been used for the treatment of post-operative shivering (Singh et al., 1993). Another indication for doxapram is apnea in newborns (Henderson-Smart and Steer, 2004; Mathew, 2011).
Administration of doxapram leads to an elevation of tidal volume as well as a marginal increase of respiratory rate (Winnie and Collins, 1966). Doxapram acts on different parts of the respiratory control system in a concentration-dependent manner. It modulates peripheral as well as central sites (Yost, 2006). Nishino et al. (1982) showed that doxapram acts on peripheral chemoreceptors in the carotid body. After sectioning the carotid sinus and aortic nerve in cats the ventilatory effect of doxapram has been abolished for doses as high as 6 mg/kg (Mitchell and Herbert, 1975; Nishino et al., 1982). Some residual ventilatory effect has been observed after denervation of peripheral chemoreceptors with even higher doses of doxapram, leading to the assumption of a secondary site of action probably in the central nervous system (Mitchell and Herbert, 1975; Wilkinson et al., 2010; Golder et al., 2013).
Doxapram is a very efficient TASK inhibitor with a half-maximal effective concentration of 410 nM for ratTASK-1, 37 μM for ratTASK-3 and 9 μM for a heterodimer of both in Xenopus laevis oocytes (Cotten et al., 2006). These values are within the range of therapeutic doxapram concentrations; therefore, it is plausible to assume that TASK-1 and TASK-3 present in glomus cells of the carotid body are mediating the ventilatory effects of doxapram (Yost, 2006; O’Donohoe et al., 2018). Inhibition of TASK-1 and TASK-3 depolarizes the membrane, followed by calcium influx through voltage-gated calcium channels, leading to secretion of neurotransmitters. These neurotransmitters cause excitation of afferent nerves terminating in respiratory and cardiovascular control centers in the brainstem, resolving respiratory failure induced by narcotics or central nervous system depressants (Buckler, 2007; Peers et al., 2010; Golder et al., 2013; O’Donohoe et al., 2018).
Interestingly, Cunningham et al. (2020) could show recently that doxapram inhibits both humanTASK-1 and humanTASK-3 equipotently (4.0 μM for TASK-1 vs. 2.5 μM for TASK-3), suggesting a possible reason for the differences observed between rodent models and humans. Furthermore, they could show that the two enantiomers of the racemate doxapram have different effects on TASK channels. Whereas, the (+)-enantiomer (GAL-054) has a higher inhibitory effect on humanTASK-1 (1.6 μM) and humanTASK-3 (1.4 μM), the effect of the (–)-enantiomer (GAL-053) is much reduced with 336 μM (humanTASK-1) and 286 μM (humanTASK-3) (Cunningham et al., 2020). Furthermore, Golder et al. (2012a, b) showed in rats that the adverse events such as arrhythmias, seizures, and death were only observed with GAL-053, whereas, the respiratory stimulant properties were connected with administration of GAL-054. However, GAL-054 leads to hypertension in rats with an increase in blood pressure of 15–20% above baseline (Golder et al., 2013).
Today, doxapram is rarely applied because of the introduction of safer and shorter-acting anesthetic drugs that have no need for an arousing breathing stimulant drug. Even its application in patients with respiratory insufficiency is almost obsolete as there are other substances and techniques available (Yost, 2006). However, in recent years there has been work by our group to investigate doxapram as a new drug for rhythm control in AF and help this substance to experience a renaissance.
Similar to A293, we administered doxapram in a porcine model for paroxysmal and persistent AF to test its efficacy in restoring and sustaining SR. The induction of AF was achieved by intermittent atrial burst pacing via implanted pacemakers. An AV-node ablation was performed to prevent tachymyopathy and an integrated biofeedback-algorithm prevented pacing during episodes of AF to promote the development of endogenous AF. A significant reduction of AF-burden has been observed in pigs under daily doxapram treatment. Furthermore, doxapram could be applied for cardioversion of paroxysmal AF in pigs (Schmidt et al., 2019a). Taken together, doxapram appears to be a promising drug for the treatment of AF.
However, being data from a large-animal model, these findings need to be verified in a human trial. Therefore, the DOxapram Conversion TO Sinus rhythm (DOCTOS)-Trial (EudraCT No: 2018-002979-17) has been initiated (Peyronnet and Ravens, 2019). Only patients with paroxysmal or persistent, non-valvular AF will be included in this study. The primary aim is to investigate the anti-arrhythmic potential of doxapram for acute cardioversion from AF to SR after intravenous application. Secondary aims are finding the ideal dose for cardioversion, to measure the time to cardioversion as well as the time to recurrence of AF within 7 days of cardioversion, to determine the doxapram pharmacokinetics after one or two equal consecutive bolus doses and lastly to gather data on the safety and tolerability of doxapram (EU Clinical Trials Register, 2018). A positive outcome of the DOCTOS-Trial would be a further step toward doxapram as a possible new treatment option for AF patients.
6 Conclusion
To conclude this review, there are many different options available to treat patients with AF, but none of them is without limitations and drawbacks. Therefore, it is essential to look for further ways to treat these patients. Underlying atrial cardiomyopathy mechanisms have to be elucidated to decide the best therapeutic strategy for each patient. A new option could become the inhibition of the TASK-1 potassium channel. Therefore, we are awaiting with interest the results of the DOCTOS trial and to answer the question: Is doxapram a new treatment option for AF? However, more scientific insights in AF are the key to develop successful and personalized treatment strategies for patients.
Author Contributions
MK, AB, FW, and CS contributed conception and design of the article; MK wrote the first draft of the manuscript; AB, FW, and CS wrote sections of the manuscript. All authors contributed to manuscript revision, read, and approved the submitted version.
Funding
This work was supported by research grants from the University of Heidelberg, Faculty of Medicine, Heidelberg, Germany (Rahel Goitein-Straus Scholarship and Olympia-Morata Scholarship to CS); from the German Center for Cardiovascular Research, Berlin, Germany (DZHK) (Excellence Grant to C.S.; Excellence Program: Postdoc Start-up Grant to FW); from the German Cardiac Society (DGK), Dusseldorf, Germany (Research Scholarship DGK082018 to FW; Otto-Hess Fellowship to FW; from the German Heart Foundation/German Foundation of Heart Research, Frankfurt, Germany (F/15/18 to FW, F/41/15 to CS, Kaltenbach Scholarship to AB and FW); from the Joachim-Herz Foundation, Hamburg, Germany (Addon-Fellowship for Interdisciplinary Life Science to FW); and from the German Research Foundation (DFG), Bonn, Germany (SCHM 3358/1-1 to CS, and SFB 1425 to CS).
Conflict of Interest
FW, HK, and CS have filed a patent application for KCNK3-based gene therapy for cardiac arrhythmia. FW, WH, HK, and CS have filed a patent application for pharmacological TASK-1 inhibition in treatment of atrial arrhythmia. The remaining authors have reported that they have no relationships relevant to the content of this paper to disclose.
Supplementary Material
The Supplementary Material for this article can be found online at: https://www.frontiersin.org/articles/10.3389/fphar.2021.638445/full#supplementary-material.
Abbreviation:
AAD, antiarrhythmic drug; AERP, atrial effective refractory period; AF, atrial fibrillation; AP, action potential; APD, action potential duration; AV, atrioventricular; CCR, CO2 central chemoreceptor; CRT, cardiac resynchronization therapy; CSA, central sleep apnea; ECG, electrocardiogram; eCV, electrical cardioversion; ESC, European Society of Cardiology; K2P, two-pore-domain potassium; OSA, obstructive sleep apnea; PASMC, pulmonary arterial smooth muscle cell; pCO2, partial pressure of carbon dioxide; pCV, pharmacological cardioversion; PH, pulmonary hypertension; PVI, pulmonary vein isolation; SK, Small-conductance Ca2+-activated K+; SR, sinus rhythm; TASK-1, tandem of P domains in a weak inward rectifying K+ channel (TWIK)-related acid sensitive K+ channel 1; TASK-3, tandem of P domains in a weak inward rectifying K+ channel (TWIK)-related acid sensitive K+ channel 3
References
Aguilar, M., and Nattel, S. (2019). Postoperative atrial fibrillation after noncardiac surgery: maybe not so Benign after all. Can. J. Cardiol. 35, 1423–1425. doi:10.1016/j.cjca.2019.08.013
Al-Khatib, S. M., LaPointe, N. M. A., Chatterjee, R., Crowley, M. J., Dupre, M. E., Kong, D. F., et al. (2014). Rate- and rhythm-control therapies in patients with atrial fibrillation: a systematic review. Ann. Intern. Med. 160, 760–773. doi:10.7326/M13-1467
Alp, N., Bell, J., and Shahi, M. (2000). Randomised double blind trial of oral versus intravenous flecainide for the cardioversion of acute atrial fibrillation. Heart 84, 37–40. doi:10.1136/heart.84.1.37
Amiodarone Trials Meta-Analysis Investigators (1997). Effect of prophylactic amiodarone on mortality after acute myocardial infarction and in congestive heart failure: meta-analysis of individual data from 6500 patients in randomised trials. Lancet 350, 1417–1424. doi:10.1016/S0140-6736(97)05281-1
Andersson, T., Magnuson, A., Bryngelsson, I.-L., Frøbert, O., Henriksson, K. M., Edvardsson, N., et al. (2013). All-cause mortality in 272 186 patients hospitalized with incident atrial fibrillation 1995–2008: a Swedish nationwide long-term case–control study. Eur. Heart J. 34, 1061–1067. doi:10.1093/eurheartj/ehs469
Andrikopoulos, G. K., Pastromas, S., and Tzeis, S. (2015). Flecainide: current status and perspectives in arrhythmia management. World J. Cardiol. 7, 76–85. doi:10.4330/wjc.v7.i2.76
Balla, I., Petrela, E., and Kondili, A. (2011). Pharmacological conversion of recent atrial fibrillation: a randomized, placebo-controlled study of three antiarrhythmic drugs. Anatol J. Cardiol. 11, 600–606. doi:10.5152/akd.2011.162
Ballantyne, D., and Scheid, P. (2000). Mammalian brainstem chemosensitive neurones: linking them to respiration in vitro. J. Physiol. 525, 567–577. doi:10.1111/j.1469-7793.2000.00567.x
Barth, A. S., Merk, S., Arnoldi, E., Zwermann, L., Kloos, P., Gebauer, M., et al. (2005). Reprogramming of the human atrial transcriptome in permanent atrial fibrillation: expression of a ventricular-like genomic signature. Circ. Res. 96, 1022–1029. doi:10.1161/01.RES.0000165480.82737.33
Bash, L. D., Buono, J. L., Davies, G. M., Martin, A., Fahrbach, K., Phatak, H., et al. (2012). Systematic review and meta-analysis of the efficacy of cardioversion by vernakalant and comparators in patients with atrial fibrillation. Cardiovasc. Drugs Ther. 26, 167–179. doi:10.1007/s10557-012-6374-4
Bayliss, D. A., and Barrett, P. Q. (2008). Emerging roles for two-pore-domain potassium channels and their potential therapeutic impact. Trends Pharmacol. Sci. 29, 566–575. doi:10.1016/j.tips.2008.07.013
Bayliss, D. A., Talley, E. M., Sirois, J. E., and Lei, Q. (2001). TASK-1 is a highly modulated pH-sensitive ‘leak'K+ channel expressed in brainstem respiratory neurons. Respir. Physiol. 129, 159–174. doi:10.1016/S0034-5687(01)00288-2
Bellone, A., Etteri, M., Vettorello, M., Bonetti, C., Clerici, D., Gini, G., et al. (2012). Cardioversion of acute atrial fibrillation in the emergency department: a prospective randomised trial. Emerg. Med. J. 29, 188–191. doi:10.1136/emj.2010.109702
Benjamin, E. J., Wolf, P. A., D'Agostino, R. B., Silbershatz, H., Kannel, W. B., and Levy, D. (1998). Impact of atrial fibrillation on the risk of death: the Framingham heart study. Circulation 98, 946–952. doi:10.1161/01.CIR.98.10.946
Berg, A. P., Talley, E. M., Manger, J. P., and Bayliss, D. A. (2004). Motoneurons express heteromeric TWIK-related acid-sensitive K+ (TASK) channels containing TASK-1 (KCNK3) and TASK-3 (KCNK9) subunits. J. Neurosci. 24, 6693–6702. doi:10.1523/JNEUROSCI.1408-04.2004
Bianconi, L., Castro, A., Dinelli, M., Alboni, P., Pappalardo, A., Richiardi, E., et al. (2000). Comparison of intravenously administered dofetilide versus amiodarone in the acute termination of atrial fibrillation and flutter. a multicentre, randomized, double-blind, placebo-controlled study. Eur. Heart J. 21, 1265–1273. doi:10.1053/euhj.1999.203
Bikou, O., Thomas, D., Trappe, K., Lugenbiel, P., Kelemen, K., Koch, M., et al. (2011). Connexin 43 gene therapy prevents persistent atrial fibrillation in a porcine model. Cardiovasc. Res. 92, 218–225. doi:10.1093/cvr/cvr209
Bogdan, R., Goegelein, H., and Ruetten, H. (2011). Effect of dronedarone on Na+, Ca2+ and HCN channels. Naunyn Schmiedebergs Arch. Pharmacol. 383, 347–356. doi:10.1007/s00210-011-0599-9
Bono, J. d., and Kirchhof, P. (2018). Is there a CASTLE-AF on the hill?. Eur. Heart J. 39, 1324–1325. doi:10.1093/eurheartj/ehy172
Boriani, G., Diemberger, I., Biffi, M., Martignani, C., and Branzi, A. (2004). Pharmacological cardioversion of atrial fibrillation. Drugs 64, 2741–2762. doi:10.2165/00003495-200464240-00003
Botkin, S. B., Dhanekula, L. S., and Olshansky, B. (2003). Outpatient cardioversion of atrial arrhythmias: efficacy, safety, and costs. Am. Heart J. 145, 233–238. doi:10.1067/mhj.2003.112
Botto, G. L., and Tortora, G. (2020). Is delayed cardioversion the better approach in recent-onset atrial fibrillation? Yes. Intern. Emerg. Med. 15, 1–4. doi:10.1007/s11739-019-02225-x
Boucherat, O., Chabot, S., Antigny, F., Perros, F., Provencher, S., and Bonnet, S. (2015). Potassium channels in pulmonary arterial hypertension. Eur. Respir. J. 46, 1167–1177. doi:10.1183/13993003.00798-2015
Bradley, T. D., and Floras, J. S. (2003). Sleep apnea and heart failure: Part I: obstructive sleep apnea. Circulation 107, 1671–1678. doi:10.1161/01.CIR.0000061757.12581.15
Buckler, K. J. (2007). TASK-like potassium channels and oxygen sensing in the carotid body. Respir. Physiol. Neurobiol. 157, 55–64. doi:10.1016/j.resp.2007.02.013
Caballero, R., de la Fuente, M. G., Gómez, R., Barana, A., Amorós, I., Dolz-Gaitón, P., et al. (2010). In humans, chronic atrial fibrillation decreases the transient outward current and ultrarapid component of the delayed rectifier current differentially on each atria and increases the slow component of the delayed rectifier current in both. J. Am. Coll. Cardiol. 55, 2346–2354. doi:10.1016/j.jacc.2010.02.028
Calvin, A. D., Somers, V. K., Van Der Walt, C., Scott, C. G., and Olson, L. J. (2011). Relation of natriuretic peptide concentrations to central sleep apnea in patients with heart failure. Chest 140, 1517–1523. doi:10.1378/chest.10-2472
Camm, A. J., Capucci, A., Hohnloser, S. H., Torp-Pedersen, C., Van Gelder, I. C., Mangal, B., et al. (2011). A randomized active-controlled study comparing the efficacy and safety of vernakalant to amiodarone in recent-onset atrial fibrillation. J. Am. Coll. Cardiol. 57, 313–321. doi:10.1016/j.jacc.2010.07.046
Camm, A. J., and Savelieva, I. (2008). New antiarrhythmic drugs for atrial fibrillation: focus on dronedarone and vernakalant. J. Interv. Card Electrophysiol. 23, 7–14. doi:10.1007/s10840-008-9269-3
Campbell, T. J., and Vaughan Williams, E. M. (1983). Voltage-and time-dependent depression of maximum rate of depolarisation of Guinea-pig ventricular action potentials by two new antiarrhythmic drugs, flecainide and lorcainide. Cardiovasc. Res. 17, 251–258. doi:10.1093/cvr/17.5.251
Cappato, R., Calkins, H., Chen, S.-A., Davies, W., Iesaka, Y., Kalman, J., et al. (2010). Updated worldwide Survey on the methods, efficacy, and safety of catheter ablation for human atrial fibrillation. Circ. Arrhythm Electrophysiol. 3, 32–38. doi:10.1161/CIRCEP.109.859116
Capucci, A., and Boriani, G. (1995). Propafenone in the treatment of cardiac arrhythmias. Drug Saf. 12, 55–72. doi:10.2165/00002018-199512010-00005
Capucci, A., and Compagnucci, P. (2020). Is delayed cardioversion the better approach in recent-onset atrial fibrillation? No. Intern. Emerg. Med. 15, 5–7. doi:10.1007/s11739-019-02224-y
Capucci, A., Villani, G. Q., Aschieri, D., and Piepoli, M. (1999). Safety of oral propafenone in the conversion of recent onset atrial fibrillation to sinus rhythm: a prospective parallel placebo-controlled multicentre study. Int. J. Cardiol. 68, 187–196. doi:10.1016/S0167-5273(98)00363-5
Carbajosa, J. D., Cosin-Sales, J., Pérez-Durá, M. J., Noceda, J., Urtubia-Palacios, A., Hernandez-Sori, N., et al. (2017). Vernakalant in hospital emergency practice: safety and effectiveness. Emergencias 29, 397–402.
Charnigo, R., Khairy, P., Guo, J., Shohoudi, A., and Elayi, C. S. (2018). Use of digoxin in atrial fibrillation: one step further in the mortality controversy from the affirm study. Pacing Clin. Electrophysiol. 41, 713–719. doi:10.1111/pace.13350
Chatterjee, S., Sardar, P., Lichstein, E., Mukherjee, D., and Aikat, S. (2013). Pharmacologic rate versus rhythm-control strategies in atrial fibrillation: an updated comprehensive review and meta-analysis. Pacing Clin. Electrophysiol. 36, 122–133. doi:10.1111/j.1540-8159.2012.03513.x
Chebbout, R., Heywood, E., Drake, T., Wild, J., Lee, J., Wilson, M., et al. (2018). A systematic review of the incidence of and risk factors for postoperative atrial fibrillation following general surgery. Anaesthesia 73, 490–498. doi:10.1111/anae.14118
Chen, W. S., Gao, B. R., Chen, W. Q., Li, Z. Z., Xu, Z. Y., Zhang, Y. H., et al. (2013). Comparison of pharmacological and electrical cardioversion in permanent atrial fibrillation after prosthetic cardiac valve replacement: a prospective randomized trial. J. Int. Med. Res. 41, 1067–1073. doi:10.1177/0300060513489800
Chokshi, R. H., Larsen, A. T., Bhayana, B., and Cotten, J. F. (2015). Breathing stimulant compounds inhibit TASK-3 potassium channel function likely by binding at a common site in the channel pore. Mol. Pharmacol. 88, 926–934. doi:10.1124/mol.115.100107
Christ, T., Boknik, P., Wohrl, S., Wettwer, E., Graf, E., Bosch, R., et al. (2004). L-type Ca2+ current downregulation in chronic human atrial fibrillation is associated with increased activity of protein phosphatases. Circulation 110, 2651–2657. doi:10.1161/01.CIR.0000145659.80212.6A
Chugh, S. S., Havmoeller, R., Narayanan, K., Singh, D., Rienstra, M., Benjamin, E. J., et al. (2014). Worldwide epidemiology of atrial fibrillation: a Global burden of disease 2010 study. Circulation 129, 837–847. doi:10.1161/CIRCULATIONAHA.113.005119
Cohen-Lehman, J., Dahl, P., Danzi, S., and Klein, I. (2010). Effects of amiodarone therapy on thyroid function. Nat. Rev. Endocrinol. 6, 34–41. doi:10.1038/nrendo.2009.225
Conde, D., Costabel, J. P., Aragon, M., Lambardi, F., Klein, A., Corrales Barbosa, A., et al. (2013). Propafenone versus vernakalant for conversion of recent-onset atrial fibrillation. Cardiovasc. Ther. 31, 377–380. doi:10.1111/1755-5922.12036
Conkbayir, C., Yigit, Z., Hural, R., Ugurlucan, M., Oztas, D. M., Okcun, B., et al. (2019). Do restoring and maintaining sinus rhythm have a Beneficial effect on secondary prevention of stroke in patients with atrial fibrillation? A Pilot study. Angiology 70, 916–920. doi:10.1177/0003319719856455
Connolly, S. J., Camm, A. J., Halperin, J. L., Joyner, C., Alings, M., Amerena, J., et al. (2011). Dronedarone in high-risk permanent atrial fibrillation. N. Engl. J. Med. 365, 2268–2276. doi:10.1056/NEJMoa1109867
Conte, S. M., Florisson, D. S., De Bono, J. A., Davies, R. A., and Newcomb, A. E. (2019). Management of atrial fibrillation after cardiac surgery. Intern. Med. J. 49, 656–658. doi:10.1111/imj.14281
Cosedis Nielsen, J., Johannessen, A., Raatikainen, P., Hindricks, G., Walfridsson, H., Kongstad, O., et al. (2012). Radiofrequency ablation as initial therapy in paroxysmal atrial fibrillation. N. Engl. J. Med. 367, 1587–1595. doi:10.1056/NEJMoa1113566
Costanzo, M. R., Khayat, R., Ponikowski, P., Augostini, R., Stellbrink, C., Mianulli, M., et al. (2015). Mechanisms and clinical consequences of untreated central sleep apnea in heart failure. J. Am. Coll. Cardiol. 65, 72–84. doi:10.1016/j.jacc.2014.10.025
Cotten, J. F., Keshavaprasad, B., Laster, M. J., Eger, E. I., and Yost, C. S. (2006). The ventilatory stimulant doxapram inhibits TASK tandem pore (K) potassium channel function but does not affect Minimum Alveolar anesthetic concentration. Anesth. Analg. 102, 779–785. doi:10.1213/01.ane.0000194289.34345.63
Courtemanche, M., Ramirez, R. J., and Nattel, S. (1999). Ionic targets for drug therapy and atrial fibrillation-induced electrical remodeling: insights from a mathematical model. Cardiovasc. Res. 42, 477–489. doi:10.1016/S0008-6363(99)00034-6
Cowan, J. C., and Vaughan Williams, E. M. (1981). Characterization of a new oral antiarrhythmic drug, flecainide (r818). Eur. J. Pharmacol. 73, 333–342. doi:10.1016/0014-2999(81)90234-X
Crijns, H. J., Weijs, B., Fairley, A.-M., Lewalter, T., Maggioni, A. P., Martín, A., et al. (2014). Contemporary real life cardioversion of atrial fibrillation: results from the multinational RHYTHM-AF study. Int. J. Cardiol. 172, 588–594. doi:10.1016/j.ijcard.2014.01.099
Cristoni, L., Tampieri, A., Mucci, F., Iannone, P., Venturi, A., Cavazza, M., et al. (2011). Cardioversion of acute atrial fibrillation in the short observation unit: comparison of a protocol focused on electrical cardioversion with simple antiarrhythmic treatment. Emerg. Med. J. 28, 932–937. doi:10.1136/emj.2009.083196
Crosswhite, P., and Sun, Z. (2014). Molecular mechanisms of pulmonary arterial remodeling. Mol. Med. 20, 191–201. doi:10.2119/molmed.2013.00165
Cunningham, K. P., Holden, R. G., Escribano-Subias, P. M., Cogolludo, A., Veale, E. L., and Mathie, A. (2019). Characterization and regulation of wild-type and mutant TASK-1 two pore domain potassium channels indicated in pulmonary arterial hypertension. J. Physiol. 597, 1087–1101. doi:10.1113/JP277275
Cunningham, K. P., MacIntyre, D. E., Mathie, A., and Veale, E. L. (2020). Effects of the ventilatory stimulant, doxapram on human TASK-3 (KCNK9, K2P9.1) channels and TASK-1 (KCNK3, K2P3.1) channels. Acta Physiol. 228, e13361. doi:10.1111/apha.13361
Cutler, M. J., Jeyaraj, D., and Rosenbaum, D. S. (2011). Cardiac electrical remodeling in health and disease. Trends Pharmacol. Sci. 32, 174–180. doi:10.1016/j.tips.2010.12.001
Dagres, N., Hindricks, G., Kottkamp, H., Sommer, P., Gaspar, T., Bode, K., et al. (2009). Complications of atrial fibrillation ablation in a high-volume center in 1,000 procedures: still cause for concern?. J. Cardiovasc. Electrophysiol. 20, 1014–1019. doi:10.1111/j.1540-8167.2009.01493.x
Dankner, R., Shahar, A., Novikov, I., Agmon, U., Ziv, A., and Hod, H. (2009). Treatment of stable atrial fibrillation in the emergency department: a population-based comparison of electrical direct-current versus pharmacological cardioversion or conservative management. Cardiology 112, 270–278. doi:10.1159/000151703
de Denus, S., Sanoski, C. A., Carlsson, J., Opolski, G., and Spinler, S. A. (2005). Rate vs rhythm control in patients with atrial fibrillation: a meta-analysis. Arch. Intern. Med. 165, 258–262. doi:10.1001/archinte.165.3.258
De Simone, A., De Pasquale, M., De Matteis, C., Canciello, M., Manzo, M., Sabino, L., et al. (2003). Verapamil plus antiarrhythmic drugs reduce atrial fibrillation recurrences after an electrical cardioversion (VEPARAF Study). Eur. Heart J. 24, 1425–1429. doi:10.1016/S0195-668X(03)00311-7
de Vecchis, R., Soreca, S., and Ariano, C. (2019). Ablation, rate or rhythm control strategies for patients with atrial fibrillation: how do they affect mid-term clinical outcomes? A retrospective cohort study. Minerva Cardioangiol. 67, 272–279. doi:10.23736/S0026-4725.19.04877-1
Dean, J. B., Ballantyne, D., Cardone, D. L., Erlichman, J. S., and Solomon, I. C. (2002). Role of gap junctions in CO chemoreception and respiratory control. Am. J. Physiol. Lung Cell Mol. Physiol. 283, L665–L670. doi:10.1152/ajplung.00142.2002
Dean, J. B., Kinkade, E. A., and Putnam, R. W. (2001). Cell–cell coupling in CO/H+-excited neurons in brainstem slices. Respir. Physiol. 129, 83–100. doi:10.1016/S0034-5687(01)00284-5
Decher, N., Kumar, P., Gonzalez, T., Pirard, B., and Sanguinetti, M. C. (2006). Binding site of a novel Kv1. 5 blocker: a “foot in the door” against atrial fibrillation. Mol. Pharmacol. 70, 1204–1211. doi:10.1124/mol.106.026203
Decher, N., Pirard, B., Bundis, F., Peukert, S., Baringhaus, K.-H., Busch, A. E., et al. (2004). Molecular basis for kv1. 5 channel block. J. Biol. Chem. 279, 394–400. doi:10.1074/jbc.M307411200
Dedman, A., Sharif-Naeini, R., Folgering, J. H., Duprat, F., Patel, A., and Honoré, E. (2009). The mechano-gated K channel TREK-1. Eur. Biophys. J. 38, 293–303. doi:10.1007/s00249-008-0318-8
Dewland, T. A., Olgin, J. E., Vittinghoff, E., and Marcus, G. M. (2013). Incident atrial fibrillation among Asians, Hispanics, Blacks, and Whites. Circulation 128, 2470–2477. doi:10.1161/CIRCULATIONAHA.113.002449
Digby, G. C., and Baranchuk, A. (2012). Sleep apnea and atrial fibrillation; 2012 update. Curr. Cardiol. Rev. 8, 265–272. doi:10.2174/157340312803760811
Digitalis Investigation Group (1997). The effect of digoxin on mortality and morbidity in patients with heart failure. N. Engl. J. Med. 336, 525–533. doi:10.1056/NEJM199702203360801
Diness, J. G., Sørensen, U. S., Nissen, J. D., Al-Shahib, B., Jespersen, T., Grunnet, M., et al. (2010). Inhibition of small-conductance ca2+-activated k+ channels terminates and protects against atrial fibrillation. Circ. Arrhythm Electrophysiol. 3, 380–390. doi:10.1161/CIRCEP.110.957407
Dobrev, D., Aguilar, M., Heijman, J., Guichard, J.-B., and Nattel, S. (2019). Postoperative atrial fibrillation: mechanisms, manifestations and management. Nat. Rev. Cardiol. 16, 417–436. doi:10.1038/s41569-019-0166-5
Dukes, I., and Vaughan Williams, E. (1984). Effects of selective alpha 1-, alpha 2-, beta 1-and beta 2-adrenoceptor stimulation on potentials and contractions in the rabbit heart. J. Physiol. 355, 523–546. doi:10.1113/jphysiol.1984.sp015436
Duprat, F., Lesage, F., Fink, M., Reyes, R., Heurteaux, C., and Lazdunski, M. (1997). TASK, a human background K+ channel to sense external pH variations near physiological pH. EMBO J. 16, 5464–5471. doi:10.1093/emboj/16.17.5464
Dusman, R. E., Stanton, M. S., Miles, W. M., Klein, L. S., Zipes, D. P., Fineberg, N. S., et al. (1990). Clinical features of amiodarone-induced pulmonary toxicity. Circulation 82, 51–59. doi:10.1161/01.CIR.82.1.51
Echt, D. S., Liebson, P. R., Mitchell, L. B., Peters, R. W., Obias-Manno, D., Barker, A. H., et al. (1991). Mortality and morbidity in patients receiving encainide, flecainide, or placebo: the Cardiac Arrhythmia Suppression Trial. N. Engl. J. Med. 324, 781–788. doi:10.1056/NEJM199103213241201
Edwards, G., and Leszczynski, S. (1967). A double-blind trial of five respiratory stimulants in patients in acute ventilatory failure. Lancet 290, 226–229. doi:10.1016/S0140-6736(67)92296-9
Elkayam, U. (1998). Calcium channel blockers in heart failure. Cardiology 89, 38–46. doi:10.1159/000047278
Enyedi, P., and Czirják, G. (2010). Molecular background of leak K+ currents: two-pore domain potassium channels. Physiol. Rev. 90, 559–605. doi:10.1152/physrev.00029.2009
EU Clinical Trials Register (2018). Doctos trial (DOxapram conversion TO sinus rhythm study)—EudraCT number: 2018-002979-17. Available at: .https://www.clinicaltrialsregister.eu/ctr-search/trial/2018-002979-17/DE (Accessed Auguts, 28, 2019).
European Medicines Agency (2004). EMEA/CPMP/1504/04: Public statement on Tikosyn (dofetilide). Available at: https://www.ema.europa.eu/en/documents/public-statement/public-statement-tikosyn-dofetilide-voluntary-withdrawal-marketing-authorisation-european-union_en.pdf (Accessed May, 03, 2020).
European Medicines Agency (2019). EMEA/H/C/001043—R/0042: Multaq: EPAR—Product Information. Available at: https://www.ema.europa.eu/en/documents/product-information/multaq-epar-product-information_en.pdf (Accessed May, 03, 2020).
Evers, W., Malik, K., and Dobkin, A. B. (1965). Influence of doxapram hydrochloride on recovery from Thiopental anaesthesia. Can J. Anaesth. 12, 281. doi:10.1007/BF03004143
Fakuade, F. E., Steckmeister, V., Seibertz, F., Gronwald, J., Kestel, S., Menzel, J., et al. (2020). Altered atrial cytosolic calcium handling contributes to the development of postoperative atrial fibrillation. Cardiovasc. Res. 12, 33. doi:10.1093/cvr/cvaa162
Falk, R. H., Pollak, A., Singh, S. N., and Friedrich, T. (1997). Intravenous dofetilide, a class III antiarrhythmic agent, for the termination of sustained atrial fibrillation or flutter. J. Am. Coll. Cardiol. 29, 385–390. doi:10.1016/S0735-1097(96)00506-2
Fedida, D., Orth, P. M., Chen, J. Y., Lin, S., Plouvier, B., Jung, G., et al. (2005). The mechanism of atrial antiarrhythmic action of RSD1235. J. Cardiovasc. Electrophysiol. 16, 1227–1238. doi:10.1111/j.1540-8167.2005.50028.x
Fedida, D. (2007). Vernakalant (RSD1235): a novel, atrial-selective antifibrillatory agent. Expert Opin. Invest Drugs 16, 519–532. doi:10.1517/13543784.16.4.519
Fedida, D., Wible, B., Wang, Z., Fermini, B., Faust, F., Nattel, S., et al. (1993). Identity of a novel delayed rectifier current from human heart with a cloned K+ channel current. Circ. Res. 73, 210–216. doi:10.1161/01.RES.73.1.210
Feldman, J. L., Mitchell, G. S., and Nattie, E. E. (2003). Breathing: rhythmicity, plasticity, chemosensitivity. Annu. Rev. Neurosci. 26, 239–266. doi:10.1146/annurev.neuro.26.041002.131103
Feliciangeli, S., Chatelain, F. C., Bichet, D., and Lesage, F. (2015). The family of K channels: salient structural and functional properties. J. Physiol. 593, 2587–2603. doi:10.1113/jphysiol.2014.287268
Fietze, I., Laharnar, N., Obst, A., Ewert, R., Felix, S. B., Garcia, C., et al. (2019). Prevalence and association analysis of obstructive sleep apnea with gender and age differences–Results of SHIP-Trend. J. Sleep Res. 28, e12770. doi:10.1111/jsr.12770
Fox, C. S., Parise, H., D’Agostino Sr, R. B., Lloyd-Jones, D. M., Vasan, R. S., Wang, T. J., et al. (2004). Parental atrial fibrillation as a risk factor for atrial fibrillation in offspring. JAMA 291, 2851–2855. doi:10.1001/jama.291.23.2851
Frey, W. (1918). Ueber vorhofflimern beim menschen und seine beseitigung durch chiniden. Berl klin Wschr 55, 417–419.
Funck-Brentano, C., Kroemer, H. K., Lee, J. T., and Roden, D. M. (1990). Propafenone. N. Engl. J. Med. 322, 518–525. doi:10.1056/NEJM199002223220806
Furniss, S. S., and Sneyd, J. R. (2015). Safe sedation in modern cardiological practice. Heart 101, 1526–1530. doi:10.1136/heartjnl-2015-307656
Fuster, V., Rydén, L. E., Cannom, D. S., Crijns, H. J., Curtis, A. B., Ellenbogen, K. A., et al. (2006). ACC/AHA/ESC 2006 guidelines for the management of patients with atrial fibrillation: a report of the American College of Cardiology/American heart association Task Force on practice guidelines and the European Society of Cardiology Committee for practice guidelines (Writing Committee to Revise the 2001 guidelines for the management of patients with atrial fibrillation). J. Am. Coll. Cardiol. 48, e149–e249. doi:10.1016/j.jacc.2006.07.018
Gaborit, N., Steenman, M., Lamirault, G., Le Meur, N., Le Bouter, S., Lande, G., et al. (2005). Human atrial ion channel and transporter subunit gene-expression remodeling associated with valvular heart disease and atrial fibrillation. Circulation 112, 471–481. doi:10.1161/CIRCULATIONAHA.104.506857
Gaine, S., and McLaughlin, V. (2017). Pulmonary arterial hypertension: tailoring treatment to risk in the current era. Eur. Respir. Rev. 26, 170095. doi:10.1183/16000617.0095-2017
Gastaut, H., Tassinari, C., and Duron, B. (1966). Polygraphic study of the episodic diurnal and nocturnal (hypnic and respiratory) manifestations of the pickwick syndrome. Brain Res. 1, 167–186. doi:10.1016/0006-8993(66)90117-X
Georges, J.-L., Spentchian, M., Caubel, C., Collignon, I., Schwob, J., Livarek, B., et al. (1996). Time course of troponin I, myoglobin, and cardiac enzyme release after electrical cardioversion. Am. J. Cardiol. 78, 825–827. doi:10.1016/S0002-9149(96)00430-4
Gierten, J., Ficker, E., Bloehs, R., Schweizer, P. A., Zitron, E., Scholz, E., et al. (2010). The human cardiac K3.1 (TASK-1) potassium leak channel is a molecular target for the class III antiarrhythmic drug amiodarone. Naunyn Schmiedebergs Arch. Pharmacol. 381, 261–270. doi:10.1007/s00210-009-0454-4
Gillott, R. G., Willan, K., Kain, K., Sivananthan, U. M., and Tayebjee, M. H. (2017). South asian ethnicity is associated with a lower prevalence of atrial fibrillation despite greater prevalence of established risk factors: a population-based study in bradford metropolitan district. Europace 19, 356–363. doi:10.1093/europace/euw010
Gitt, A. K., Smolka, W., Michailov, G., Bernhardt, A., Pittrow, D., and Lewalter, T. (2013). Types and outcomes of cardioversion in patients admitted to hospital for atrial fibrillation: results of the German rhythm-af study. Clin. Res. Cardiol. 102, 713–723. doi:10.1007/s00392-013-0586-x
Glatter, K. A., Dorostkar, P. C., Yang, Y., Lee, R. J., Van Hare, G. F., Keung, E., et al. (2001). Electrophysiological effects of ibutilide in patients with accessory pathways. Circulation 104, 1933–1939. doi:10.1161/hc4101.097538
Glynn, I. (1957). The action of cardiac glycosides on sodium and potassium movements in human red cells. J. Physiol. 136, 148–173. doi:10.1113/jphysiol.1957.sp005749
Goldberger, Z. D., and Alexander, G. C. (2014). Digitalis use in contemporary clinical practice: refitting the foxglove. JAMA Intern. Med. 174, 151–154. doi:10.1001/jamainternmed.2013.10432
Golder, F. J., Gruber, R. B., Baby, S. M., Puskovic, V., Ideo, C. M., Peng, S., et al. (2012a). Enantiomeric separation of doxapram reveals a superior respiratory stimulant, GAL-054. FASEB J. 26, 894–922. doi:10.1096/fasebj.26.1_supplement.894.22
Golder, F. J., Gruber, R. B., Puskovic, V., Peng, S., Dax, S. L., MacIntyre, D. E., et al. (2012b). Enantiomeric separation of doxapram reveals a superior respiratory stimulant, GAL-054. FASEB J. 26, 704–726. doi:10.1096/fasebj.26.1_supplement.704.26
Golder, F. J., Hewitt, M. M., and McLeod, J. F. (2013). Respiratory stimulant drugs in the post-operative setting. Respir. Physiol. Neurobiol. 189, 395–402. doi:10.1016/j.resp.2013.06.010
Goldschlager, N., Epstein, A. E., Naccarelli, G. V., Olshansky, B., Singh, B., Collard, H. R., et al. (2007). A practical guide for clinicians who treat patients with amiodarone: 2007. Heart Rhythm 4, 1250–1259. doi:10.1016/j.hrthm.2007.07.020
Goldstein, R. E., Boccuzzi, S. J., Cruess, D., and Nattel, S. (1991). Diltiazem increases late-onset congestive heart failure in postinfarction patients with early reduction in ejection fraction. Circulation 83, 52–60. doi:10.1161/01.CIR.83.1.52
Goldstein, S. A., Bayliss, D. A., Kim, D., Lesage, F., Plant, L. D., and Rajan, S. (2005). International Union of Pharmacology. LV. Nomenclature and molecular relationships of two-P potassium channels. Pharmacol. Rev. 57, 527–540. doi:10.1124/pr.57.4.12
Goldstein, S. A., Bockenhauer, D., O’Kelly, I., and Zilberberg, N. (2001). Potassium leak channels and the KCNK family of two-P-domain subunits. Nat. Rev. Neurosci. 2, 175. doi:10.1038/35058574
Gowda, R. M., Punukollu, G., Khan, I. A., Wilbur, S. L., Vasavada, B. C., and Sacchi, T. J. (2003). Ibutilide for pharmacological cardioversion of atrial fibrillation and flutter: impact of race on efficacy and safety. Am. J. Ther. 10, 259–263. doi:10.1097/00045391-200307000-00005
Grandi, E., Pandit, S. V., Voigt, N., Workman, A. J., Dobrev, D., Jalife, J., et al. (2011). Human atrial action potential and ca2+ model: sinus rhythm and chronic atrial fibrillation. Circ. Res. 109, 1055–1066. doi:10.1161/CIRCRESAHA.111.253955
Greaves, K., and Crake, T. (1998). Cardiac troponin T does not increase after electrical cardioversion for atrial fibrillation or atrial flutter. Heart 80, 226–228. doi:10.1136/hrt.80.3.226
Groenveld, H. F., Crijns, H. J., Van den Berg, M. P., Van Sonderen, E., Alings, A. M., Tijssen, J. G., et al. (2011). The effect of rate control on quality of life in patients with permanent atrial fibrillation: data from the RACE II (Rate Control Efficacy in Permanent Atrial Fibrillation II) study. J. Am. Coll. Cardiol. 58, 1795–1803. doi:10.1016/j.jacc.2011.06.055
Guilleminault, C., Tilkian, A., and Dement, W. C. (1976). The sleep apnea syndromes. Annu. Rev. Med. 27, 465–484. doi:10.1146/annurev.me.27.020176.002341
Gupta, A., Perera, T., Ganesan, A., Sullivan, T., Lau, D. H., Roberts-Thomson, K. C., et al. (2013). Complications of catheter ablation of atrial fibrillation: a systematic review. Circ. Arrhythm Electrophysiol. 6, 1082–1088. doi:10.1161/CIRCEP.113.000768
Gurney, A., and Manoury, B. (2009). Two-pore potassium channels in the cardiovascular system. Eur. Biophys. J. 38, 305. doi:10.1007/s00249-008-0326-8
Guyenet, P. G., Stornetta, R. L., and Bayliss, D. A. (2010). Central respiratory chemoreception. J. Comp. Neurol. 518, 3883–3906. doi:10.1002/cne.22435
Haissaguerre, M., Jaïs, P., Shah, D. C., Takahashi, A., Hocini, M., Quiniou, G., et al. (1998). Spontaneous initiation of atrial fibrillation by ectopic beats originating in the pulmonary veins. N. Engl. J. Med. 339, 659–666. doi:10.1056/NEJM199809033391003
Hallberg, P., Lindbäck, J., Lindahl, B., Stenestrand, U., and Melhus, H. (2007). Digoxin and mortality in atrial fibrillation: a prospective cohort study. Eur. J. Clin. Pharmacol. 63, 959–971. doi:10.1007/s00228-007-0346-9
Han, H.-C., Ha, F. J., Sanders, P., Spencer, R., Teh, A. W., O’donnell, D., et al. (2017). Atrioesophageal fistula: clinical presentation, procedural characteristics, diagnostic investigations, and treatment outcomes. Circ. Arrhythm Electrophysiol. 10, e005579. doi:10.1161/CIRCEP.117.005579
Harleton, E., Besana, A., Chandra, P., Danilo, P., Rosen, T. S., Rosen, M. R., et al. (2015). TASK-1 current is inhibited by phosphorylation during human and canine chronic atrial fibrillation. Am. J. Physiol. Heart Circ. Physiol. 308, H126. doi:10.1152/ajpheart.00614.2014
Harleton, E., Besana, A., Comas, G. M., Danilo, P., Rosen, T. S., Argenziano, M., et al. (2013). Ability to induce atrial fibrillation in the peri-operative period is associated with phosphorylation-dependent inhibition of TWIK protein-related acid-sensitive potassium channel 1 (TASK-1). J. Biol. Chem. 288, 2829–2838. doi:10.1074/jbc.M112.404095
Harrison, D. C. (1985). Antiarrhythmic drug classification: new science and practical applications. Am. J. Cardiol. 56, 185–187. doi:10.1016/0002-9149(85)90591-0
Hart, R. G., Benavente, O., McBride, R., and Pearce, L. A. (1999). Antithrombotic therapy to prevent stroke in patients with atrial fibrillation: a meta-analysis. Ann. Intern. Med. 131, 492–501. doi:10.7326/0003-4819-131-7-199910050-00003
Hart, R. G., Pearce, L. A., and Aguilar, M. I. (2007). Meta-analysis: antithrombotic therapy to prevent stroke in patients who have nonvalvular atrial fibrillation. Ann. Intern. Med. 146, 857–867. doi:10.7326/0003-4819-146-12-200706190-00007
Henderson-Smart, D. J., and Steer, P. A. (2004). Doxapram treatment for apnea in preterm infants. Cochrane Database Syst. Rev. 11, CD000074. doi:10.1002/14651858.CD000074.pub2
Henriksson, K. M., Farahmand, B., Åsberg, S., Edvardsson, N., and Terént, A. (2012). Comparison of cardiovascular risk factors and survival in patients with ischemic or hemorrhagic stroke. Int. J. Stroke 7, 276–281. doi:10.1111/j.1747-4949.2011.00706.x
Hindricks, G., Potpara, T., Dagres, N., Arbelo, E., Bax, J. J., Blomström-Lundqvist, C., et al. (2020). 2020 ESC Guidelines for the diagnosis and management of atrial fibrillation developed in collaboration with the European Association of Cardio-Thoracic Surgery (EACTS). Eur. Heart J. 14, 1–125. doi:10.1093/eurheartj/ehaa612
Hla, K. M., Young, T., Hagen, E. W., Stein, J. H., Finn, L. A., Nieto, F. J., et al. (2015). Coronary heart disease incidence in sleep disordered breathing: the Wisconsin sleep cohort study. Sleep 38, 677–684. doi:10.5665/sleep.4654
Hodgkin, A. L., and Huxley, A. F. (1952). A quantitative description of membrane current and its application to conduction and excitation in nerve. J. Physiol. 117, 500–544. doi:10.1113/jphysiol.1952.sp004764
Hohl, M., Linz, B., Bohm, M., and Linz, D. (2014). Obstructive sleep apnea and atrial arrhythmogenesis. Curr. Cardiol. Rev. 10, 362–368. doi:10.2174/1573403x1004140707125137
Hohnloser, S. H., Connolly, S. J., Crijns, H. J., Page, R. L., Seiz, W., and Torp-Petersen, C. (2008). Rationale and design of ATHENA: a placebo-controlled, double-blind, parallel arm Trial to assess the efficacy of dronedarone 400 mg bid for the prevention of cardiovascular Hospitalization or death from any cause in patiENts with Atrial fibrillation/atrial flutter. J. Cardiovasc. Electrophysiol. 19, 69–73. doi:10.1111/j.1540-8167.2007.01016.x
Hohnloser, S. H., Crijns, H. J., Van Eickels, M., Gaudin, C., Page, R. L., Torp-Pedersen, C., et al. (2009). Effect of dronedarone on cardiovascular events in atrial fibrillation. N. Engl. J. Med. 360, 668–678. doi:10.1056/NEJMoa0803778
Holmes, B., and Heel, R. (1985). Flecainide. A preliminary review of its pharmacodynamic properties and therapeutic efficacy. Drugs 29, 1–33. doi:10.2165/00003495-198529010-00001
Houser, W. C., and Schlueter, D. P. (1978). Prolonged doxapram infusion in obesity-hypoventilation syndrome. JAMA 239, 340–341. doi:10.1001/jama.1978.03280310072026
Hsueh, C.-H., Chang, P.-C., Hsieh, Y.-C., Reher, T., Chen, P.-S., and Lin, S.-F. (2013). Proarrhythmic effect of blocking the small conductance calcium activated potassium channel in isolated canine left atrium. Heart Rhythm 10, 891–898. doi:10.1016/j.hrthm.2013.01.033
Hudgel, D. W., Gordon, E. A., Thanakitcharu, S., and Bruce, E. N. (1998). Instability of ventilatory control in patients with obstructive sleep apnea. Am. J. Respir. Crit. Care Med. 158, 1142–1149. doi:10.1164/ajrccm.158.4.9712105
Humbert, M., Sitbon, O., and Simonneau, G. (2004). Treatment of pulmonary arterial hypertension. N. Engl. J. Med. 351, 1425–1436. doi:10.1056/NEJMra040291
Hurdman, J., Condliffe, R., Elliot, C., Davies, C., Hill, C., Wild, J., et al. (2012). ASPIRE registry: assessing the Spectrum of Pulmonary hypertension Identified at a REferral centre. Eur. Respir. J. 39, 945–955. doi:10.1183/09031936.00078411
Jafari-Fesharaki, M., and Scheinman, M. M. (1998). Adverse effects of amiodarone. Pacing Clin. Electrophysiol. 21, 108–120. doi:10.1111/j.1540-8159.1998.tb01068.x
January, C. T., Wann, L. S., Alpert, J. S., Calkins, H., Cigarroa, J. E., Cleveland, J. C., et al. (2014). 2014 AHA/ACC/HRS guideline for the management of patients with atrial fibrillation: a report of the American College of Cardiology/American heart association Task Force on practice guidelines and the heart rhythm Society. J. Am. Coll. Cardiol. 64, e1–e76. doi:10.1016/j.jacc.2014.03.022
Javaheri, S., Parker, T., Liming, J., Corbett, W., Nishiyama, H., Wexler, L., et al. (1998). Sleep apnea in 81 ambulatory male patients with stable heart failure: types and their prevalences, consequences, and presentations. Circulation 97, 2154–2159. doi:10.1161/01.CIR.97.21.2154
Javaheri, S. (2006). Sleep disorders in systolic heart failure: a prospective study of 100 male patients. the final report. Int. J. Cardiol. 106, 21–28. doi:10.1016/j.ijcard.2004.12.068
Jiang, C., Rojas, A., Wang, R., and Wang, X. (2005). CO central chemosensitivity: why are there so many sensing molecules?. Respir. Physiol. Neurobiol. 145, 115–126. doi:10.1016/j.resp.2004.07.005
Jiang, C., Xu, H., Cui, N., and Wu, J. (2001). An alternative approach to the identification of respiratory central chemoreceptors in the brainstem. Respir. Physiol. 129, 141–157. doi:10.1016/S0034-5687(01)00301-2
Jones, C., Pollit, V., Fitzmaurice, D., and Cowan, C. (2014). The management of atrial fibrillation: summary of updated NICE guidance. The BMJ 348, g3655. doi:10.1136/bmj.g3655
Joseph, A. P., and Ward, M. R. (2000). A prospective, randomized controlled trial comparing the efficacy and safety of sotalol, amiodarone, and digoxin for the reversion of new-onset atrial fibrillation. Ann. Emerg. Med. 36, 1–9. doi:10.1067/mem.2000.107655
Jullian-Desayes, I., Joyeux-Faure, M., Tamisier, R., Launois, S., Borel, A.-L., Levy, P., et al. (2015). Impact of obstructive sleep apnea treatment by continuous positive airway pressure on cardiometabolic biomarkers: a systematic review from sham CPAP randomized controlled trials. Sleep Med. Rev. 21, 23–38. doi:10.1016/j.smrv.2014.07.004
Kafkas, N. V., Patsilinakos, S. P., Mertzanos, G. A., Papageorgiou, K. I., Chaveles, J. I., Dagadaki, O. K., et al. (2007). Conversion efficacy of intravenous ibutilide compared with intravenous amiodarone in patients with recent-onset atrial fibrillation and atrial flutter. Int. J. Cardiol. 118, 321–325. doi:10.1016/j.ijcard.2006.07.017
Kambayashi, R., Hagiwara-Nagasawa, M., Ichikawa, T., Goto, A., Chiba, K., Nunoi, Y., et al. (2020). Analysis of electropharmacological effects of AVE0118 on the atria of chronic atrioventricular block dogs: characterization of anti-atrial fibrillatory action by atrial repolarization-delaying agent. Heart Vessels 35, 1316–1322. doi:10.1007/s00380-020-01612-1
Kapur, S., Barbhaiya, C., Deneke, T., and Michaud, G. F. (2017). Esophageal injury and atrioesophageal fistula caused by ablation for atrial fibrillation. Circulation 136, 1247–1255. doi:10.1161/CIRCULATIONAHA.117.025827
Kathofer, S., Thomas, D., and Karle, C. A. (2005). The novel antiarrhythmic drug dronedarone: comparison with amiodarone. Cardiovasc. Drug Rev. 23, 217–230. doi:10.1111/j.1527-3466.2005.tb00167.x
Ketchum, K. A., Joiner, W. J., Sellers, A. J., Kaczmarek, L. K., and Goldstein, S. A. (1995). A new family of outwardly rectifying potassium channel proteins with two pore domains in tandem. Nature 376, 690–695. doi:10.1038/376690a0
Khan, I. A. (2003). Oral loading single dose flecainide for pharmacological cardioversion of recent-onset atrial fibrillation. Int. J. Cardiol. 87, 121–128. doi:10.1016/S0167-5273(02)00467-9
Khan, I. A. (2001). Single oral loading dose of propafenone for pharmacological cardioversion of recent-onset atrial fibrillation. J. Am. Coll. Cardiol. 37, 542–547. doi:10.1016/S0735-1097(00)01116-5
Khoo, M. C. (2000). Determinants of ventilatory instability and variability. Respir. Physiol. 122, 167–182. doi:10.1016/S0034-5687(00)00157-2
Khoo, M., Kronauer, R. E., Strohl, K. P., and Slutsky, A. S. (1982). Factors inducing periodic breathing in humans: a general model. J. Appl. Physiol. 53, 644–659. doi:10.1152/jappl.1982.53.3.644
Kim, D., and Kang, D. (2015). Role of K channels in stimulus-secretion coupling. Pflugers Arch. 467, 1001–1011. doi:10.1007/s00424-014-1663-3
Kiper, A. K., Rinné, S., Rolfes, C., Ramírez, D., Seebohm, G., Netter, M. F., et al. (2015). Kv1. 5 blockers preferentially inhibit TASK-1 channels: TASK-1 as a target against atrial fibrillation and obstructive sleep apnea?. Pflugers Arch. 467, 1081–1090. doi:10.1007/s00424-014-1665-1
Kirchhof, P., Breithardt, G., Camm, A. J., Crijns, H. J., Kuck, K.-H., Vardas, P., et al. (2013). Improving outcomes in patients with atrial fibrillation: rationale and design of the Early treatment of Atrial fibrillation for Stroke prevention Trial. Am. Heart J. 166, 442–448. doi:10.1016/j.ahj.2013.05.015
Kirchhof, P., Camm, A. J., Goette, A., Brandes, A., Eckardt, L., Elvan, A., et al. (2020). Early rhythm-control therapy in patients with atrial fibrillation. N. Engl. J. Med. 13, 33. doi:10.1056/NEJMoa2019422
Kirchhof, P., Eckardt, L., Loh, P., Weber, K., Fischer, R.-J., Seidl, K.-H., et al. (2002). Anterior-posterior versus anterior-lateral electrode positions for external cardioversion of atrial fibrillation: a randomised trial. Lancet 360, 1275–1279. doi:10.1016/S0140-6736(02)11315-8
Kirchhof, P., Mönnig, G., Wasmer, K., Heinecke, A., Breithardt, G., Eckardt, L., et al. (2005). A trial of self-adhesive patch electrodes and hand-held paddle electrodes for external cardioversion of atrial fibrillation (MOBIPAPA). Eur. Heart J. 26, 1292–1297. doi:10.1093/eurheartj/ehi160
Knobloch, K., Brendel, J., Peukert, S., Rosenstein, B., Busch, A. E., and Wirth, K. J. (2002). Electrophysiological and antiarrhythmic effects of the novel I channel blockers, S9947 and S20951, on left vs. right pig atrium in vivo in comparison with the I blockers dofetilide, azimilide, d,l-sotalol and ibutilide. Naunyn Schmiedebergs Arch. Pharmacol. 366, 482–487. doi:10.1007/s00210-002-0599-x
Knobloch, K., Brendel, J., Rosenstein, B., Bleich, M., Busch, A. E., and Wirth, K. J. (2004). Atrial-selective antiarrhythmic actions of novel I vs. I, I, and I class Ic drugs and beta blockers in pigs. Med. Sci. Monit. 10, BR221–BR228.
Køber, L., Torp-Pedersen, C., McMurray, J. J., Gøtzsche, O., Lévy, S., Crijns, H., et al. (2008). Increased mortality after dronedarone therapy for severe heart failure. N. Engl. J. Med. 358, 2678–2687. doi:10.1056/NEJMoa0800456
Kodama, I., Kamiya, K., and Toyama, J. (1999). Amiodarone: ionic and cellular mechanisms of action of the most promising class III agent. Am. J. Cardiol. 84, 20–28. doi:10.1016/S0002-9149(99)00698-0
Kossaify, A. (2019). Vernakalant in atrial fibrillation: a relatively new weapon in the armamentarium against an old enemy. Drug Target Insights 13, 1–7. doi:10.1177/1177392819861114
Kotecha, D., Holmes, J., Krum, H., Altman, D. G., Manzano, L., Cleland, J. G., et al. (2014). Efficacy of beta blockers in patients with heart failure plus atrial fibrillation: an individual-patient data meta-analysis. Lancet 384, 2235–2243. doi:10.1016/S0140-6736(14)61373-8
Kotecha, D., and Kirchhof, P. (2014). Rate and rhythm control have comparable effects on mortality and stroke in atrial fibrillation but better data are needed. BMJ Evid. Based Med. 19, 222–223. doi:10.1136/ebmed-2014-110062
Kotecha, D., Manzano, L., Krum, H., Rosano, G., Holmes, J., Altman, D. G., et al. (2016). Effect of age and sex on efficacy and tolerability of β blockers in patients with heart failure with reduced ejection fraction: individual patient data meta-analysis. The BMJ 353, i1855. doi:10.1136/bmj.i1855
Kowey, P. R., Dorian, P., Mitchell, L. B., Pratt, C. M., Roy, D., Schwartz, P. J., et al. (2009). Vernakalant hydrochloride for the rapid conversion of atrial fibrillation after cardiac surgery: a randomized, double-blind, placebo-controlled trial. Circ. Arrhythm Electrophysiol. 2, 652–659. doi:10.1161/CIRCEP.109.870204
Kowey, P. R., Marinchak, R. A., Rials, S. J., and Filart, R. A. (1998). Acute treatment of atrial fibrillation. Am. J. Cardiol. 81, 16C–22C. doi:10.1016/S0002-9149(98)00182-9
Kowey, P. R., VanderLugt, J. T., and Luderer, J. R. (1996). Safety and risk/benefit analysis of ibutilide for acute conversion of atrial fibrillation/flutter. Am. J. Cardiol. 78, 46–52. doi:10.1016/S0002-9149(96)00566-8
Kuck, K.-H., Brugada, J., Fürnkranz, A., Metzner, A., Ouyang, F., Chun, K. J., et al. (2016). Cryoballoon or Radiofrequency ablation for paroxysmal atrial fibrillation. N. Engl. J. Med. 374, 2235–2245. doi:10.1056/NEJMoa1602014
Laratta, C. R., Ayas, N. T., Povitz, M., and Pendharkar, S. R. (2017). Diagnosis and treatment of obstructive sleep apnea in adults. CMAJ 189, E1481. doi:10.1503/cmaj.170296
Lee, J.-Y., Kim, T.-H., Yang, P.-S., Lim, H. E., Choi, E.-K., Shim, J., et al. (2017). Korean atrial fibrillation network genome-wide association study for early-onset atrial fibrillation identifies novel susceptibility loci. Eur. Heart J. 38, 2586–2594. doi:10.1093/eurheartj/ehx213
Lei, M., Wu, L., Terrar, D. A., and Huang, C. L.-H. (2018). Modernized classification of cardiac antiarrhythmic drugs. Circulation 138, 1879–1896. doi:10.1161/CIRCULATIONAHA.118.035455
Lenz, T. L., and Hilleman, D. E. (2000). Dofetilide, a new class III antiarrhythmic agent. Pharmacotherapy 20, 776–786. doi:10.1592/phco.20.9.776.35208
Lesage, F., Guillemare, E., Fink, M., Duprat, F., Lazdunski, M., Romey, G., et al. (1996). TWIK-1, a ubiquitous human weakly inward rectifying K+ channel with a novel structure. EMBO J. 15, 1004–1011. doi:10.1002/j.1460-2075.1996.tb00437.x
Lesage, F., and Lazdunski, M. (2000). Molecular and functional properties of two-pore-domain potassium channels. Am. J. Physiol. Ren. Physiol 279, F793–F801. doi:10.1152/ajprenal.2000.279.5.F793
Lesage, F. (2003). Pharmacology of neuronal background potassium channels. Neuropharmacology 44, 1–7. doi:10.1016/S0028-3908(02)00339-8
Liang, B., Soka, M., Christensen, A. H., Olesen, M. S., Larsen, A. P., Knop, F. K., et al. (2014). Genetic variation in the two-pore domain potassium channel, TASK-1, may contribute to an atrial substrate for arrhythmogenesis. J. Mol. Cell Cardiol 67, 69–76. doi:10.1016/j.yjmcc.2013.12.014
Lim, K.-T., Davis, M. J., Powell, A., Arnolda, L., Moulden, K., Bulsara, M., et al. (2007). Ablate and pace strategy for atrial fibrillation: long-term outcome of AIRCRAFT trial. Europace 9, 498–505. doi:10.1093/europace/eum091
Limberg, S. H., Netter, M. F., Rolfes, C., Rinné, S., Schlichthörl, G., Zuzarte, M., et al. (2011). TASK-1 channels may modulate action potential duration of human atrial cardiomyocytes. Cell Physiol Biochem 28, 613–624. doi:10.1159/000335757
Lloyd, E. E., Crossland, R. F., Phillips, S. C., Marrelli, S. P., Reddy, A. K., Taffet, G. E., et al. (2011). Disruption of K6.1 produces vascular dysfunction and hypertension in mice. Hypertension 58, 672–678. doi:10.1161/HYPERTENSIONAHA.111.175349
Lobo, R., Jaffe, A. S., Cahill, C., Blake, O., Abbas, S., Meany, T. B., et al. (2018). Significance of high-sensitivity troponin T after elective external direct current cardioversion for atrial fibrillation or atrial flutter. Am. J. Cardiol. 121, 188–192. doi:10.1016/j.amjcard.2017.10.009
Loose, S., Mueller, J., Wettwer, E., Knaut, M., Ford, J., Milnes, J., et al. (2014). Effects of I blocker MK-0448 on human right atrial action potentials from patients in sinus rhythm and in permanent atrial fibrillation. Front Pharmacol. 5, 26. doi:10.3389/fphar.2014.00026
Loricchio, M. L., Cianfrocca, C., Pasceri, V., Bianconi, L., Auriti, A., Calo, L., et al. (2007). Relation of C-reactive protein to long-term risk of recurrence of atrial fibrillation after electrical cardioversion. Am. J. Cardiol. 99, 1421–1424. doi:10.1016/j.amjcard.2006.12.074
Lown, B., Perlroth, M. G., Kaidbey, S., Abe, T., and Harken, D. E. (1963). Cardioversion of atrial fibrillation: a report on the treatment of 65 episodes in 50 patients. N. Engl. J. Med. 269, 325–331. doi:10.1056/NEJM196308152690701
Lunsford, C. D., Cale, A. D., Ward, J. W., Franko, B. V., and Jenkins, H. (1964). 4-(β-Substituted ethyl)-3, 3-diphenyl-2-pyrrolidinones. A new Series of CNS stimulants. J. Med. Chem. 7, 302–310. doi:10.1021/jm00333a012
Ma, L., Roman-Campos, D., Austin, E. D., Eyries, M., Sampson, K. S., Soubrier, F., et al. (2013). A novel channelopathy in pulmonary arterial hypertension. N. Engl. J. Med. 369, 351–361. doi:10.1056/NEJMoa1211097
Macdonald, M., Fang, J., Pittman, S. D., White, D. P., and Malhotra, A. (2008). The current prevalence of sleep disordered breathing in congestive heart failure patients treated with beta-blockers. J. Clin. Sleep Med. 4, 38–42.
Madonia, S., De Simone, M., Brai, G., Gozzo, D., Gristina, A., Luciano, L., et al. (2000). Intravenous versus oral initial load of propafenone for conversion of recent-onset atrial fibrillation in the emergency room: a randomized trial. Ital. Heart J. 1, 475–479.
Madrid, A., Moro, C., Marin-Huerta, E., Mestre, J., Novo, L., and Costa, A. (1993). Comparison of flecainide and procainamide in cardioversion of atrial fibrillation. Eur. Heart J. 14, 1127–1131. doi:10.1093/eurheartj/14.8.1127
Maingret, F., Honoré, E., Lazdunski, M., and Patel, A. J. (2002). Molecular basis of the voltage-dependent gating of TREK-1, a mechano-sensitive K+ channel. Biochem. Biophys. Res. Commun. 292, 339–346. doi:10.1006/bbrc.2002.6674
Malouf, J. F., Kanagala, R., Al Atawi, F. O., Rosales, A. G., Davison, D. E., Murali, N. S., et al. (2005). High Sensitivity C-Reactive Protein: a novel predictor for recurrence of atrial fibrillation after successful cardioversion. J. Am. Coll. Cardiol. 46, 1284–1287. doi:10.1016/j.jacc.2005.06.053
Marinelli, A., and Capucci, A. (2010). Pharmacologic conversion of atrial fibrillation and atrial flutter. Card Electrophysiol. Clin. 2, 393–407. doi:10.1016/j.ccep.2010.06.002
Marrouche, N. F., Brachmann, J., Andresen, D., Siebels, J., Boersma, L., Jordaens, L., et al. (2018). Catheter ablation for atrial fibrillation with heart failure. N. Engl. J. Med. 378, 417–427. doi:10.1056/NEJMoa1707855
Marrouche, N. F., and Brachmann, J. (2009). Catheter ablation versus standard Conventional treatment in patients with left ventricular dysfunction and atrial fibrillation (CASTLE-AF)-Study design. Pacing Clin. Electrophysiol. 32, 987–994. doi:10.1111/j.1540-8159.2009.02428.x
Martínez-Marcos, F. J., García-Garmendia, J. L., Ortega-Carpio, A., Fern ández Gómez, J. M., Santos, J. M., and Camacho, C. (2000). Comparison of intravenous flecainide, propafenone, and amiodarone for conversion of acute atrial fibrillation to sinus rhythm. Am. J. Cardiol. 86, 950–953. doi:10.1016/S0002-9149(00)01128-0
Mathew, O. (2011). Apnea of prematurity: pathogenesis and management strategies. J. Perinatol 31, 302–310. doi:10.1038/jp.2010.126
Mitchell, R. A., and Herbert, D. A. (1975). Potencies of doxapram and hypoxia in stimulating carotid-body chemoreceptors and ventilation in anesthetized cats. Anesthesiology 42, 559–566. doi:10.1097/00000542-197505000-00009
Mittal, S., Ayati, S., Stein, K., Schwartzman, D., Cavlovich, D., Tchou, P., et al. (2000). Transthoracic cardioversion of atrial fibrillation: comparison of rectilinear biphasic versus damped sine wave monophasic shocks. Circulation 101, 1282–1287. doi:10.1161/01.CIR.101.11.1282
Mont, L., Bisbal, F., Hernandez-Madrid, A., Perez-Castellano, N., Viñolas, X., Arenal, A., et al. (2014). Catheter ablation vs. antiarrhythmic drug treatment of persistent atrial fibrillation: a multicentre, randomized, controlled trial (SARA study). Eur. Heart J. 35, 501–507. doi:10.1093/eurheartj/eht457
Montani, D., Bergot, E., Günther, S., Savale, L., Bergeron, A., Bourdin, A., et al. (2012). Pulmonary arterial hypertension in patients treated by dasatinib. Circulation 125, 2128–2137. doi:10.1161/CIRCULATIONAHA.111.079921
Morani, G., Borio, G., Bolzan, B., and Ribichini, F. L. (2019). Safety and efficacy of a cardiologist-only approach to deep sedation for electrical cardioversion. J. Cardiovasc. Med. 20, 16–22. doi:10.2459/JCM.0000000000000731
Morganroth, J. (1987). Risk factors for the development of proarrhythmic events. Am. J. Cardiol. 59, E32–E37. doi:10.1016/0002-9149(87)90199-8
Moser, K. M., Luchsinger, P. C., Adamson, J. S., McMahon, S. M., Schlueter, D. P., Spivack, M., et al. (1973). Respiratory stimulation with intravenous doxapram in respiratory failure: a double-blind cooperative study. N. Engl. J. Med. 288, 427–431. doi:10.1056/NEJM197303012880901
Mounsey, J. P., and DiMarco, J. P. (2000). Dofetilide. Circulation 102, 2665–2670. doi:10.1161/01.CIR.102.21.2665
Mulkey, D. K., Stornetta, R. L., Weston, M. C., Simmons, J. R., Parker, A., Bayliss, D. A., et al. (2004). Respiratory control by ventral surface chemoreceptor neurons in rats. Nat. Neurosci. 7, 1360–1369. doi:10.1038/nn1357
Mulkey, D. K., Talley, E. M., Stornetta, R. L., Siegel, A. R., West, G. H., Chen, X., et al. (2007). TASK channels determine pH sensitivity in select respiratory neurons but do not contribute to central respiratory chemosensitivity. J. Neurosci. 27, 14049–14058. doi:10.1523/JNEUROSCI.4254-07.2007
Nagaraj, C., Tang, B., Bálint, Z., Wygrecka, M., Hrzenjak, A., Kwapiszewska, G., et al. (2013). Src tyrosine kinase is crucial for potassium channel function in human pulmonary arteries. Eur. Respir. J. 41, 85–95. doi:10.1183/09031936.00211811
Nair, M., George, L. K., and Koshy, S. K. (2011). Safety and efficacy of ibutilide in cardioversion of atrial flutter and fibrillation. J. Am. Board Fam. Med. 24, 86–92. doi:10.3122/jabfm.2011.01.080096
Nattel, S., Burstein, B., and Dobrev, D. (2008). Atrial remodeling and atrial fibrillation: mechanisms and implications. Circ. Arrhythm Electrophysiol. 1, 62–73. doi:10.1161/CIRCEP.107.754564
Nattel, S., Guasch, E., Savelieva, I., Cosio, F. G., Valverde, I., Halperin, J. L., et al. (2014). Early management of atrial fibrillation to prevent cardiovascular complications. Eur. Heart J. 35, 1448–1456. doi:10.1093/eurheartj/ehu028
Nattel, S., Heijman, J., Zhou, L., and Dobrev, D. (2020). Molecular basis of atrial fibrillation pathophysiology and therapy: a translational perspective. Circ. Res. 127, 51–72. doi:10.1161/CIRCRESAHA.120.316363
Nattel, S., Maguy, A., Le Bouter, S., and Yeh, Y.-H. (2007). Arrhythmogenic ion-channel remodeling in the heart: heart failure, myocardial infarction, and atrial fibrillation. Physiol. Rev. 87, 425–456. doi:10.1152/physrev.00014.2006
Nattel, S., and Opie, L. H. (2006). Controversies in atrial fibrillation. Lancet 367, 262–272. doi:10.1016/S0140-6736(06)68037-9
Nattel, S., and Singh, B. N. (1999). Evolution, mechanisms, and classification of antiarrhythmic drugs: focus on class III actions. Am. J. Cardiol. 84, 11–19. doi:10.1016/S0002-9149(99)00697-9
Nattie, E. (1999). CO, brainstem chemoreceptors and breathing. Prog. Neurobiol. 59, 299–331. doi:10.1016/S0301-0082(99)00008-8
Navas Tejedor, P., Tenorio Castaño, J., Palomino Doza, J., Arias Lajara, P., Gordo Trujillo, G., López Meseguer, M., et al. (2017). An homozygous mutation in KCNK3 is associated with an aggressive form of hereditary pulmonary arterial hypertension. Clin. Genet. 91, 453–457. doi:10.1111/cge.12869
Nazeri, A., Razavi, M., Elayda, M. A., Lee, V.-V., Massumi, A., and Wilson, J. M. (2010). Race/ethnicity and the incidence of new-onset atrial fibrillation after isolated coronary artery bypass surgery. Heart Rhythm 7, 1458–1463. doi:10.1016/j.hrthm.2010.06.037
Neumayr, G., Hagn, C., Gänzer, H., Friedrich, G., Pechlaner, C., Joannidis, M., et al. (1997). Plasma levels of troponin T after electrical cardioversion of atrial fibrillation and flutter. Am. J. Cardiol. 80, 1367–1369. doi:10.1016/S0002-9149(97)00686-3
Nishino, T., Mokashi, A., and Lahiri, S. (1982). Stimulation of carotid chemoreceptors and ventilation by doxapram in the cat. J. Appl. Physiol. 52, 1261–1265. doi:10.1152/jappl.1982.52.5.1261
Noc, M., Stajer, D., and Horvat, M. (1990). Intravenous amiodarone versus verapamil for acute conversion of paroxysmal atrial fibrillation to sinus rhythm. Am. J. Cardiol. 65, 679–680. doi:10.1016/0002-9149(90)91053-9
Nørgaard, B. L., Wachtell, K., Christensen, P. D., Madsen, B., Johansen, J. B., Christiansen, E. H., et al. (1999). Efficacy and safety of intravenously administered dofetilide in acute termination of atrial fibrillation and flutter: a multicenter, randomized, double-blind, placebo-controlled trial. Am. Heart J. 137, 1062–1069. doi:10.1016/S0002-8703(99)70363-7
Noro, M., Zhu, X., Enomoto, Y., Oikawa, Y., Tatsunami, H., Ishii, R., et al. (2016). Decreased defibrillation threshold and minimized myocardial damage with left axilla implantable cardioverter defibrillator implantation. Circ. J. 80, 878–886. doi:10.1253/circj.CJ-15-1258
Notarstefano, P., Pratola, C., Toselli, T., Baldo, E., and Ferrari, R. (2007). Sedation with midazolam for electrical cardioversion. Pacing Clin. Electrophysiol. 30, 608–611. doi:10.1111/j.1540-8159.2007.00720.x
Oldenburg, O., Lamp, B., Faber, L., Teschler, H., Horstkotte, D., and Töpfer, V. (2007). Sleep-disordered breathing in patients with symptomatic heart failure a contemporary study of prevalence in and characteristics of 700 patients. Eur. J. Heart Fail 9, 251–257. doi:10.1016/j.ejheart.2006.08.003
Olschewski, A., Chandran, N., and Olschewski, H. (2016). Letter by Olschewski et al Regarding Article,”Upregulation of K3.1 K+ Current Causes Action Potential Shortening in Patients With Chronic Atrial Fibrillation”. Circulation 133, e439. doi:10.1161/CIRCULATIONAHA.115.018800
Olschewski, A., Li, Y., Tang, B., Hanze, J., Eul, B., Bohle, R. M., et al. (2006). Impact of TASK-1 in human pulmonary artery smooth muscle cells. Circ. Res. 98, 1072–1080. doi:10.1161/01.RES.0000219677.12988.e9
Olschewski, A., Veale, E. L., Nagy, B. M., Nagaraj, C., Kwapiszewska, G., Antigny, F., et al. (2017). TASK-1 (KCNK3) channels in the lung: from cell biology to clinical implications. Eur. Respir. J. 50, 1700754. doi:10.1183/13993003.00754-2017
Øyen, N., Ranthe, M. F., Carstensen, L., Boyd, H. A., Olesen, M. S., Olesen, S.-P., et al. (2012). Familial aggregation of lone atrial fibrillation in young persons. J. Am. Coll. Cardiol. 60, 917–921. doi:10.1016/j.jacc.2012.03.046
O’Donohoe, P. B., Huskens, N., Turner, P. J., Pandit, J. J., and Buckler, K. J. (2018). A1899, PK-THPP, ML365, and Doxapram inhibit endogenous TASK channels and excite calcium signaling in carotid body type-1 cells. Physiol. Rep. 6, e13876. doi:10.14814/phy2.13876
Packer, D. L., Mark, D. B., Robb, R. A., Monahan, K. H., Bahnson, T. D., Moretz, K., et al. (2018). Catheter ablation versus antiarrhythmic drug therapy for atrial fibrillation (CABANA) trial: study rationale and design. Am. Heart J. 199, 192–199. doi:10.1016/j.ahj.2018.02.015
Packer, D. L., Mark, D. B., Robb, R. A., Monahan, K. H., Bahnson, T. D., Poole, J. E., et al. (2019). Effect of catheter ablation vs antiarrhythmic drug therapy on mortality, stroke, bleeding, and cardiac arrest among patients with atrial fibrillation: the CABANA randomized clinical trial. JAMA 321, 1261–1274. doi:10.1001/jama.2019.0693
Pagan-Carlo, L. A., Stone, M. S., and Kerber, R. E. (1997). Nature and determinants of skin “burns” after transthoracic cardioversion. Am. J. Cardiol. 79, 689–691. doi:10.1016/S0002-9149(96)00845-4
Papiris, S. A., Triantafillidou, C., Kolilekas, L., Markoulaki, D., and Manali, E. D. (2010). Amiodarone. Drug Saf. 33, 539–558. doi:10.2165/11532320-000000000-00000
Patel, A., and Honore, E. (2001). Molecular physiology of oxygen-sensitive potassium channels. Eur. Respir. J. 18, 221–227. doi:10.1183/09031936.01.00204001
Patel, A. J., Honoré, E., Lesage, F., Fink, M., Romey, G., and Lazdunski, M. (1999). Inhalational anesthetics activate two-pore-domain background k+ channels. Nat. Neurosci. 2, 422–426. doi:10.1038/8084
Peers, C., Wyatt, C. N., and Evans, A. M. (2010). Mechanisms for acute oxygen sensing in the carotid body. Respir. Physiol. Neurobiol. 174, 292–298. doi:10.1016/j.resp.2010.08.010
Peyronnet, R., and Ravens, U. (2019). Atria-selective antiarrhythmic drugs in need of alliance partners. Pharmacol. Res. 145, 104262. doi:10.1016/j.phrs.2019.104262
Piazza, G., Hurwitz, S., Galvin, C. E., Harrigan, L., Baklla, S., Hohlfelder, B., et al. (2020). Alert-based computerized decision support for high-risk hospitalized patients with atrial fibrillation not prescribed anticoagulation: a randomized, controlled trial (AF-ALERT). Eur. Heart J. 41, 1086–1096. doi:10.1093/eurheartj/ehz526
Piechota, W., Gielerak, G., Ryczek, R., Kazmierczak, A., Bejm, J., and Piechota, W. (2007). Cardiac troponin I after external electrical cardioversion for atrial fibrillation as a marker of myocardial injury - a preliminary report. Kardiol Pol. 65, 664–669.
Pluymaekers, N. A., Dudink, E. A., Luermans, J. G., Meeder, J. G., Lenderink, T., Widdershoven, J., et al. (2019). Early or delayed cardioversion in recent-onset atrial fibrillation. N. Engl. J. Med. 380, 1499–1508. doi:10.1056/NEJMoa1900353
Podrid, P. J., and Anderson, J. L. (1996). Safety and tolerability of long-term propafenone therapy for supraventricular tachyarrhythmias. Am. J. Cardiol. 78, 430–434. doi:10.1016/S0002-9149(96)00332-3
Pohjantähti-Maaroos, H., Hyppölä, H., Lekkala, M., Sinisalo, E., Heikkola, A., and Hartikainen, J. (2019). Intravenous vernakalant in comparison with intravenous flecainide in the cardioversion of recent-onset atrial fibrillation. Eur. Heart J. Acute Cardiovasc. Care 8, 114–120. doi:10.1177/2048872617728558
Putnam, R. W. (2001). Intracellular pH regulation of neurons in chemosensitive and nonchemosensitive areas of brain slices. Respir. Physiol. 129, 37–56. doi:10.1016/S0034-5687(01)00281-X
Putzke, C., Wemhöner, K., Sachse, F. B., Rinné, S., Schlichthörl, G., Li, X. T., et al. (2007). The acid-sensitive potassium channel TASK-1 in rat cardiac muscle. Cardiovasc. Res. 75, 59–68. doi:10.1016/j.cardiores.2007.02.025
Queiroga, A., Marshall, H., Clune, M., and Gammage, M. (2003). Ablate and pace revisited: long term survival and predictors of permanent atrial fibrillation. Heart 89, 1035–1038. doi:10.1136/heart.89.9.1035
Raitt, M. H., Volgman, A. S., Zoble, R. G., Charbonneau, L., Padder, F. A., O’Hara, G. E., et al. (2006). Prediction of the recurrence of atrial fibrillation after cardioversion in the atrial fibrillation follow-up investigation of rhythm management (AFFIRM) study. Am. Heart J. 151, 390–396. doi:10.1016/j.ahj.2005.03.019
Rajan, S., Wischmeyer, E., Liu, G. X., Preisig-Müller, R., Daut, J., Karschin, A., et al. (2000). TASK-3, a novel tandem pore domain acid-sensitive K+ channel an extracellular histidine as pH sensor. J. Biol. Chem. 275, 16650–16657. doi:10.1074/jbc.M000030200
Rappolt Sr, R. T., Gay, G. R., Decker, W. J., and Inaba, D. S. (1980). NAGD regimen for the coma of drug-related overdose. Ann. Emerg. Med. 9, 357–363. doi:10.1016/S0196-0644(80)80112-0
Ravens, U. (2017). Atrial-selective K+ channel blockers: potential antiarrhythmic drugs in atrial fibrillation? Can J. Physiol. Pharmacol. 95, 1313–1318. doi:10.1139/cjpp-2017-0024
Ravens, U. (2010). Novel pharmacological approaches for antiarrhythmic therapy. Naunyn Schmiedebergs Arch. Pharmacol. 3, 187–193. doi:10.1007/s00210-009-0487-8
Redline, S., Yenokyan, G., Gottlieb, D. J., Shahar, E., O’Connor, G. T., Resnick, H. E., et al. (2010). Obstructive sleep apnea—Hypopnea and incident stroke: the sleep heart health study. Am. J. Respir. Crit. Care Med. 182, 269–277. doi:10.1164/rccm.200911-1746OC
Reisinger, J., Gatterer, E., Heinze, G., Wiesinger, K., Zeindlhofer, E., Gattermeier, M., et al. (1998). Prospective comparison of flecainide versus sotalol for immediate cardioversion of atrial fibrillation. Am. J. Cardiol. 81, 1450–1454. doi:10.1016/S0002-9149(98)00223-9
Reisinger, J., Gatterer, E., Lang, W., Vanicek, T., Eisserer, G., Bachleitner, T., et al. (2004). Flecainide versus ibutilide for immediate cardioversion of atrial fibrillation of recent onset. Eur. Heart J. 25, 1318–1324. doi:10.1016/j.ehj.2004.04.030
Richerson, G. (1995). Response to CO of neurons in the rostral ventral medulla in vitro. J. Neurophysiol. 73, 933–944. doi:10.1152/jn.1995.73.3.933
Riddell, P., and Robertson, G. (1978). Use of doxapram as an arousal agent in outpatient general anaesthesia. Br. J. Anaesth. 50, 921–924. doi:10.1093/bja/50.9.921
Rinné, S., Kiper, A. K., Schlichthörl, G., Dittmann, S., Netter, M. F., Limberg, S. H., et al. (2015). TASK-1 and TASK-3 may form heterodimers in human atrial cardiomyocytes. J. Mol. Cell Cardiol 81, 71–80. doi:10.1016/j.yjmcc.2015.01.017
Roden, D. M., and Woosley, R. L. (1986). Flecainide. N. Engl. J. Med. 315, 36–41. doi:10.1056/NEJM198607033150106
Rödström, K. E., Kiper, A. K., Zhang, W., Rinné, S., Pike, A. C., Goldstein, M., et al. (2020). A lower X-gate in TASK channels traps inhibitors within the vestibule. Nature 582, 443–447. doi:10.1038/s41586-020-2250-8
Roukoz, H., and Saliba, W. (2007). Dofetilide: a new class III antiarrhythmic agent. Expert Rev. Cardiovasc. Ther. 5, 9–19. doi:10.1586/14779072.5.1.9
Roy, D., Pratt, C. M., Torp-Pedersen, C., Wyse, D. G., Toft, E., Juul-Moller, S., et al. (2008). Vernakalant hydrochloride for rapid conversion of atrial fibrillation. Circulation 117, 1518–1525. doi:10.1161/CIRCULATIONAHA.107.723866
Roy, D., Rowe, B. H., Stiell, I. G., Coutu, B., Ip, J. H., Phaneuf, D., et al. (2004). A randomized, controlled trial of RSD1235, a novel anti-arrhythmic agent, in the treatment of recent onset atrial fibrillation. J. Am. Coll. Cardiol. 44, 2355–2361. doi:10.1016/j.jacc.2004.09.021
Ruff, C. T., Giugliano, R. P., Braunwald, E., Hoffman, E. B., Deenadayalu, N., Ezekowitz, M. D., et al. (2014). Comparison of the efficacy and safety of new oral anticoagulants with warfarin in patients with atrial fibrillation: a meta-analysis of randomised trials. Lancet 383, 955–962. doi:10.1016/S0140-6736(13)62343-0
Sacher, F., Monahan, K. H., Thomas, S. P., Davidson, N., Adragao, P., Sanders, P., et al. (2006). Phrenic nerve injury after atrial fibrillation catheter ablation: characterization and outcome in a multicenter study. J. Am. Coll. Cardiol. 47, 2498–2503. doi:10.1016/j.jacc.2006.02.050
Savelieva, I., Graydon, R., and Camm, A. J. (2013). Pharmacological cardioversion of atrial fibrillation with vernakalant: evidence in support of the ESC Guidelines. Europace 16, 162–173. doi:10.1093/europace/eut274
Schatzmann, H. (1953). Herzglykoside als hemmstoffe für den aktiven kalium und natrium-transport durch die erythrocytemembran. Helv. Physiol. Pharmacol. Acta 11, 346–354.
Schatzmann, H., and Räss, B. (1965). Inhibition of the active na-k-transport and na-k-activated membrane atp-ase of erythrocyte stroma by ouabain. Helv. Physiol. Pharmacol. Acta 65, C47–C49.
Schilling, R. J. (2010). Cardioversion of atrial fibrillation: the use of antiarrhythmic drugs. Heart 96, 333–338. doi:10.1136/hrt.2008.155812
Schmidt, C., Kisselbach, J., Schweizer, P. A., Katus, H. A., and Thomas, D. (2011). The pathology and treatment of cardiac arrhythmias: focus on atrial fibrillation. Vasc. Health Risk Manag. 7, 193–202. doi:10.2147/VHRM.S10758
Schmidt, C., and Peyronnet, R. (2018). Voltage-gated and stretch-activated potassium channels in the human heart. Herzschr Elektrophys 29, 36–42. doi:10.1007/s00399-017-0541-z
Schmidt, C., Wiedmann, F., Beyerdorf, C., Zhao, Z., Lan, H., El-Battrawy, I., et al. (2019a). V1506 - a promising novelty for antiarrhythmic therapy: doxapram inhibition of TASK-1 K+ channels suppresses atrial fibrillation in a large animal model. Clin. Res. Cardiol. 108. doi:10.1007/s00392-019-01435-9
Schmidt, C., Wiedmann, F., Beyersdorf, C., Zhao, Z.-h., El-Battrawy, I., Lan, H., et al. (2019b). Genetic ablation of tandem of P domains in a weak inward rectifying K+ channel–related acid-sensitive K+ channel-1 (K3.1) K+ channels suppresses atrial fibrillation and prevents electrical remodeling. Circ. Arrhythm Electrophysiol. 12, e007465. doi:10.1161/CIRCEP.119.007465
Schmidt, C., Wiedmann, F., Gaubatz, A.-R., Ratte, A., Katus, H. A., and Thomas, D. (2018). New targets for old drugs: cardiac glycosides inhibit atrial-specific K2P3.1 (TASK-1) channels. J. Pharmacol. Exp. Ther. 365, 614–623. doi:10.1124/jpet.118.247692
Schmidt, C., Wiedmann, F., Langer, C., Tristram, F., Anand, P., Wenzel, W., et al. (2014a). Cloning, functional characterization, and remodeling of K3.1 (TASK-1) potassium channels in a porcine model of atrial fibrillation and heart failure. Heart Rhythm 11, 1798–1805. doi:10.1016/j.hrthm.2014.06.020
Schmidt, C., Wiedmann, F., Schweizer, P. A., Becker, R., Katus, H. A., and Thomas, D. (2012). Novel electrophysiological properties of dronedarone: inhibition of human cardiac two-pore-domain potassium (K) channels. Naunyn Schmiedebergs Arch. Pharmacol. 385, 1003–1016. doi:10.1007/s00210-012-0780-9
Schmidt, C., Wiedmann, F., Schweizer, P. A., Katus, H. A., and Thomas, D. (2014b). Inhibition of cardiac two-pore-domain K+ (K) channels–an emerging antiarrhythmic concept. Eur. J. Pharmacol. 738, 250–255. doi:10.1016/j.ejphar.2014.05.056
Schmidt, C., Wiedmann, F., Voigt, N., Zhou, X.-B., Heijman, J., Lang, S., et al. (2016). Response to Letter regarding Article,”Upregulation of K3.1 K+ current causes action potential shortening in patients with chronic atrial fibrillation”. Circulation 133, e440–e441. doi:10.1161/CIRCULATIONAHA.115.020662
Schmidt, C., Wiedmann, F., Voigt, N., Zhou, X.-B., Heijman, J., Lang, S., et al. (2015). Upregulation of K2P3. 1 K+ current causes action potential shortening in patients with chronic atrial fibrillation. Circulation 132, 82–92. doi:10.1161/CIRCULATIONAHA.114.012657
Schmidt, C., Wiedmann, F., Zhou, X.-B., Heijman, J., Voigt, N., Ratte, A., et al. (2017). Inverse remodelling of K3.1 K+ channel expression and action potential duration in left ventricular dysfunction and atrial fibrillation: implications for patient-specific antiarrhythmic drug therapy. Eur. Heart J. 38, 1764–1774. doi:10.1093/eurheartj/ehw559
Schotten, U., Verheule, S., Kirchhof, P., and Goette, A. (2011). Pathophysiological mechanisms of atrial fibrillation: a translational appraisal. Physiol. Rev. 91, 265–325. doi:10.1152/physrev.00031.2009
Schweizer, P. A., Becker, R., Katus, H. A., and Thomas, D. (2011). Dronedarone: current evidence for its safety and efficacy in the management of atrial fibrillation. Drug Des. Devel Ther. 5, 27–39. doi:10.2147/DDDT.S10315
Sedgwick, M. L., Lip, G., Rae, A. P., and Cobbe, S. M. (1995). Chemical cardioversion of atrial fibrillation with intravenous dofetilide. Int. J. Cardiol. 49, 159–166. doi:10.1016/0167-5273(95)02284-4
Seyler, C., Duthil-Straub, E., Zitron, E., Gierten, J., Scholz, E., Fink, R., et al. (2012). TASK1 (K K+ channel inhibition by endothelin-1 is mediated through Rho kinase-dependent phosphorylation. Br. J. Pharmacol. 165, 1467–1475. doi:10.1111/j.1476-5381.2011.01626.x
Shea, J. B., and Maisel, W. H. (2002). Cardioversion. Circulation 106, e176–e178. doi:10.1161/01.CIR.0000040586.24302
Shenasa, F., and Shenasa, M. (2016). Dofetilide: electrophysiologic effect, efficacy, and safety in patients with cardiac arrhythmias. Card Electrophysiol. Clin. 8, 423–436. doi:10.1016/j.ccep.2016.02.006
Simon, A., Niederdoeckl, J., Skyllouriotis, E., Schuetz, N., Herkner, H., Weiser, C., et al. (2017). Vernakalant is superior to ibutilide for achieving sinus rhythm in patients with recent-onset atrial fibrillation: a randomized controlled trial at the emergency department. Europace 19, 233–240. doi:10.1093/europace/euw052
Simonneau, G., Montani, D., Celermajer, D. S., Denton, C. P., Gatzoulis, M. A., Krowka, M., et al. (2019). Haemodynamic definitions and updated clinical classification of pulmonary hypertension. Eur. Respir. J. 53, 1801913. doi:10.1183/13993003.01913-2018
Sin, D. D., Fitzgerald, F., Parker, J. D., Newton, G., Floras, J. S., and Bradley, T. D. (1999). Risk factors for central and obstructive sleep apnea in 450 men and women with congestive heart failure. Am. J. Respir. Crit. Care Med. 160, 1101–1106. doi:10.1164/ajrccm.160.4.9903020
Singh, B. N. (1996). Antiarrhythmic actions of amiodarone: a profile of a paradoxical agent. Am. J. Cardiol. 78, 41–53. doi:10.1016/S0002-9149(96)00452-3
Singh, P., Dimitriou, V., Mahajan, R., and Crossley, A. (1993). Double-blind comparison between doxapram and pethidine in the treatment of postanaesthetic shivering. Br. J. Anaesth. 71, 685–688. doi:10.1093/bja/71.5.685
Skibsbye, L., Poulet, C., Diness, J. G., Bentzen, B. H., Yuan, L., Kappert, U., et al. (2014). Small-conductance calcium-activated potassium (SK) channels contribute to action potential repolarization in human atria. Cardiovasc. Res. 103, 156–167. doi:10.1093/cvr/cvu121
Solomon, I. C., and Dean, J. B. (2002). Gap junctions in CO-chemoreception and respiratory control. Respir. Physiol. Neurobiol. 131, 155–173. doi:10.1016/S1569-9048(02)00090-3
Solomon, I. C., Halat, T. J., El-Maghrabi, M. R., and O’Neal, M. H. (2001). Localization of connexin26 and connexin32 in putative CO-chemosensitive brainstem regions in rat. Respir. Physiol. 129, 101–121. doi:10.1016/S0034-5687(01)00299-7
Somers, V. K., White, D. P., Amin, R., Abraham, W. T., Costa, F., Culebras, A., et al. (2008). Sleep apnea and cardiovascular disease: an American heart association/American College of Cardiology Foundation scientific statement from the American heart association Council for high blood pressure research professional Education Committee, Council on clinical Cardiology, stroke Council, and Council on cardiovascular Nursing. J. Am. Coll. Cardiol. 52, 686–717. doi:10.1016/j.jacc.2008.05.002
Sorgente, A., Tung, P., Wylie, J., and Josephson, M. E. (2012). Six year follow-up after catheter ablation of atrial fibrillation: a palliation more than a true cure. Am. J. Cardiol. 109, 1179–1186. doi:10.1016/j.amjcard.2011.11.058
Stewart, S., Hart, C. L., Hole, D. J., and McMurray, J. J. (2002). A population-based study of the long-term risks associated with atrial fibrillation: 20-year follow-up of the Renfrew/Paisley study. Am. J. Med. 113, 359–364. doi:10.1016/S0002-9343(02)01236-6
Stieger, P., Rana, O. R., Saygili, E., Zazai, H., Rauwolf, T., Genz, C., et al. (2018). Impact of internal and external electrical cardioversion on cardiac specific enzymes and inflammation in patients with atrial fibrillation and heart failure. J. Cardiol. 72, 135–139. doi:10.1016/j.jjcc.2018.01.016
Streit, A. K., Netter, M. F., Kempf, F., Walecki, M., Rinné, S., Bollepalli, M. K., et al. (2011). A specific two-pore domain potassium channel blocker Defines the structure of the TASK-1 open pore. J. Biol. Chem. 286, 13977–13984. doi:10.1074/jbc.M111.227884
Sun, W., Sarma, J. S., and Singh, B. N. (2002). Chronic and acute effects of dronedarone on the action potential of rabbit atrial muscle preparations: comparison with amiodarone. J. Cardiovasc. Pharmacol. 39, 677–684.
Sun, W., Sarma, J. S., and Singh, B. N. (1999). Electrophysiological effects of dronedarone (sr33589), a noniodinated benzofuran derivative, in the rabbit heart: comparison with amiodarone. Circulation 100, 2276–2281. doi:10.1161/01.CIR.100.22.2276
Suttorp, M. J., Polak, P. E., van’t Hof, A., Rasmussen, H. S., Dunselman, P. H., and Kingma, J. H. (1992). Efficacy and safety of a new selective class III antiarrhythmic agent dofetilide in paroxysmal atrial fibrillation or atrial flutter. Am. J. Cardiol. 69, 417–419. doi:10.1016/0002-9149(92)90247-v
Takahashi, T., Osanai, S., Nakano, H., Ohsaki, Y., and Kikuchi, K. (2005). Doxapram stimulates the carotid body via a different mechanism than hypoxic chemotransduction. Respir. Physiol. Neurobiol. 147, 1–9. doi:10.1016/j.resp.2005.01.005
Tamargo, J., Le Heuzey, J.-Y., and Mabo, P. (2015). Narrow therapeutic index drugs: a clinical pharmacological consideration to flecainide. Eur. J. Clin. Pharmacol. 71, 549–567. doi:10.1007/s00228-015-1832-0
Tang, B., Li, Y., Nagaraj, C., Morty, R. E., Gabor, S., Stacher, E., et al. (2009). Endothelin-1 inhibits background two-pore domain channel TASK-1 in primary human pulmonary artery smooth muscle cells. Am. J. Respir. Cell Mol Biol 41, 476–483. doi:10.1165/rcmb.2008-0412OC
Thomas, D., Kathöfer, S., Zhang, W., Wu, K., Wimmer, A.-B., Zitron, E., et al. (2003). Acute effects of dronedarone on both components of the cardiac delayed rectifier K+ current, HERG and KvLQT1/minK potassium channels. Br. J. Pharmacol. 140, 996–1002. doi:10.1038/sj.bjp.0705502
Thompson, A. R., and Lawrie, A. (2017). Targeting vascular remodeling to treat pulmonary arterial hypertension. Trends Mol. Med. 23, 31–45. doi:10.1016/j.molmed.2016.11.005
Torp-Pedersen, C., Camm, A. J., Butterfield, N. N., Dickinson, G., and Beatch, G. N. (2013). Vernakalant: conversion of atrial fibrillation in patients with ischemic heart disease. Int. J. Cardiol. 166, 147–151. doi:10.1016/j.ijcard.2011.10.108
Trapp, S., Aller, M. I., Wisden, W., and Gourine, A. V. (2008). A role for TASK-1 (KCNK3) channels in the chemosensory control of breathing. J. Neurosci. 28, 8844–8850. doi:10.1523/JNEUROSCI.1810-08.2008
Turakhia, M. P., Santangeli, P., Winkelmayer, W. C., Xu, X., Ullal, A. J., Than, C. T., et al. (2014). Increased mortality associated with digoxin in contemporary patients with atrial fibrillation: findings from the TREAT-AF study. J. Am. Coll. Cardiol. 64, 660–668. doi:10.1016/j.jacc.2014.03.060
Tuteja, D., Xu, D., Timofeyev, V., Lu, L., Sharma, D., Zhang, Z., et al. (2005). Differential expression of small-conductance Ca2+-activated K+ channels SK1, SK2, and SK3 in mouse atrial and ventricular myocytes. Am. J. Physiol. Heart Circ. Physiol. 289, H2714–H2723. doi:10.1152/ajpheart.00534.2005
Ugowe, F. E., Jackson, L. R., and Thomas, K. L. (2018). Racial and ethnic differences in the prevalence, management, and outcomes in patients with atrial fibrillation: a systematic review. Heart rhythm 15, 1337–1345. doi:10.1016/j.hrthm.2018.05.019
Ulimoen, S. R., Enger, S., Carlson, J., Platonov, P. G., Pripp, A. H., Abdelnoor, M., et al. (2013). Comparison of four single-drug regimens on ventricular rate and arrhythmia-related symptoms in patients with permanent atrial fibrillation. Am. J. Cardiol. 111, 225–230. doi:10.1016/j.amjcard.2012.09.020
Van Gelder, I. C., Groenveld, H. F., Crijns, H. J., Tuininga, Y. S., Tijssen, J. G., Alings, A. M., et al. (2010). Lenient versus strict rate control in patients with atrial fibrillation. N. Engl. J. Med. 362, 1363–1373. doi:10.1056/NEJMoa1001337
Van Wagoner, D. R., Pond, A. L., McCarthy, P. M., Trimmer, J. S., and Nerbonne, J. M. (1997). Outward K+ current densities and Kv1. 5 expression are reduced in chronic human atrial fibrillation. Circ. Res. 80, 772–781. doi:10.1161/01.RES.80.6.772
Vaughan Williams, E. (1984). A classification of antiarrhythmic actions reassessed after a decade of new drugs. J. Clin. Pharmacol. 24, 129–147. doi:10.1002/j.1552-4604.1984.tb01822.x
Vogiatzis, I., Papavasiliou, E., Dapcevitch, I., Pittas, S., and Koulouris, E. (2017). Vernakalant versus ibutilide for immediate conversion of recent-onset atrial fibrillation. Hippokratia 21, 67–73. doi:10.1093/ehjci/eux141.025
Vorperian, V. R., Havighurst, T. C., Miller, S., and January, C. T. (1997). Adverse effects of low dose amiodarone: a meta-analysis. J. Am. Coll. Cardiol. 30, 791–798. doi:10.1016/S0735-1097(97)00220-9
Walcott, G. P., Killingsworth, C. R., and Ideker, R. E. (2003). Do clinically relevant transthoracic defibrillation energies cause myocardial damage and dysfunction?. Resuscitation 59, 59–70. doi:10.1016/S0300-9572(03)00161-8
Wang, J., Zhang, C., Li, N., Su, L., and Wang, G. (2008). Expression of TASK-1 in brainstem and the occurrence of central sleep apnea in rats. Respir. Physiol. Neurobiol. 161, 23–28. doi:10.1016/j.resp.2007.11.008
Wang, Z., Fermini, B., and Nattel, S. (1993). Sustained depolarization-induced outward current in human atrial myocytes. Evidence for a novel delayed rectifier K+ current similar to Kv1. 5 cloned channel currents. Circ. Res. 73, 1061–1076. doi:10.1161/01.res.73.6.1061
Wang, Z., Pagé, P., and Nattel, S. (1992). Mechanism of flecainide’s antiarrhythmic action in experimental atrial fibrillation. Circ. Res. 71, 271–287. doi:10.1161/01.RES.71.2.271
Washburn, C. P., Sirois, J. E., Talley, E. M., Guyenet, P. G., and Bayliss, D. A. (2002). Serotonergic raphe neurons express TASK channel transcripts and a TASK-like pH-and halothane-sensitive K+ conductance. J. Neurosci. 22, 1256–1265. doi:10.1523/JNEUROSCI.22-04-01256.2002
Wettwer, E., Hála, O., Christ, T., Heubach, J. F., Dobrev, D., Knaut, M., et al. (2004). Role of I in controlling action potential shape and contractility in the human atrium: influence of chronic atrial fibrillation. Circulation 110, 2299–2306. doi:10.1161/01.CIR.0000145155.60288.71
Whitbeck, M. G., Charnigo, R. J., Khairy, P., Ziada, K., Bailey, A. L., Zegarra, M. M., et al. (2013). Increased mortality among patients taking digoxin—analysis from the AFFIRM study. Eur. Heart J. 34, 1481–1488. doi:10.1093/eurheartj/ehs348
Wiedmann, F., Beyersdorf, C., Zhou, X.-B., Kraft, M., Foerster, K. I., El-Battrawy, I., et al. (2021). The experimental TASK-1 potassium channel inhibitor A293 can Be Employed for rhythm control of persistent atrial fibrillation in a translational large animal model. Front Physiol. 11, 629241. doi:10.3389/fphys.2020.629421
Wiedmann, F., Beyersdorf, C., Zhou, X., Büscher, A., Kraft, M., Nietfeld, J., et al. (2020). Pharmacologic TWIK-related acid-sensitive K+ channel (TASK-1) potassium channel inhibitor A293 Facilitates acute cardioversion of paroxysmal atrial fibrillation in a porcine large animal model. J. Am. Heart Assoc. 9, e015751. doi:10.1161/JAHA.119.015751
Wiedmann, F., Schmidt, C., Lugenbiel, P., Staudacher, I., Rahm, A.-K., Seyler, C., et al. (2016). Therapeutic targeting of two-pore-domain potassium (K) channels in the cardiovascular system. Clin. Sci. 130, 643–650. doi:10.1042/CS20150533
Wiemann, M., and Bingmann, D. (2001). Ventrolateral neurons of medullary organotypic cultures: intracellular pH regulation and bioelectric activity. Respir. Physiol. 129, 57–70. doi:10.1016/S0034-5687(01)00282-1
Wijffels, M. C., Kirchhof, C. J., Dorland, R., and Allessie, M. A. (1995). Atrial fibrillation begets atrial fibrillation: a study in awake chronically instrumented goats. Circulation 92, 1954–1968. doi:10.1161/01.CIR.92.7.1954
Wilkinson, K. A., Huey, K., Dinger, B., He, L., Fidone, S., and Powell, F. L. (2010). Chronic hypoxia increases the gain of the hypoxic ventilatory response by a mechanism in the central nervous system. J. Appl. Physiol. 109, 424–430. doi:10.1152/japplphysiol.01311.2009
Willems, S., Meyer, C., de Bono, J., Brandes, A., Eckardt, L., Elvan, A., et al. (2019). Cabins, castles, and constant hearts: rhythm control therapy in patients with atrial fibrillation. Eur. Heart J. 40, 3793–3799. doi:10.1093/eurheartj/ehz782
Williams, S., Bateman, A., and O’Kelly, I. (2013). Altered expression of two-pore domain potassium (K) channels in cancer. PLoS One 8, e74589. doi:10.1371/journal.pone.0074589
Winnie, A. P., and Collins, V. J. (1966). The search for a pharmacologic ventilator. Acta Anaesthesiol Scand. 10, 63–71. doi:10.1111/j.1399-6576.1966.tb00993.x
Wirth, K. J., Brendel, J., Steinmeyer, K., Linz, D. K., Rütten, H., and Gögelein, H. (2007). In vitro and in vivo effects of the atrial selective antiarrhythmic compound AVE1231. J. Cardiovasc. Pharmacol. 49, 197–206. doi:10.1097/FJC.0b013e318032002f
Wirth, K. J., Steinmeyer, K., and Ruetten, H. (2013). Sensitization of upper airway mechanoreceptors as a new pharmacologic principle to treat obstructive sleep apnea: investigations with AVE0118 in anesthetized pigs. Sleep 36, 699–708. doi:10.5665/sleep.2630
Wolf, P. A., Abbott, R. D., and Kannel, W. B. (1991). Atrial fibrillation as an independent risk factor for stroke: the Framingham study. Stroke 22, 983–988. doi:10.1161/01.STR.22.8.983
Wolkove, N., and Baltzan, M. (2009). Amiodarone pulmonary toxicity. Can Respir. J. 16, 43–48. doi:10.1155/2009/282540
Xia, W., Yin, Z., Li, J., Song, Y., and Qu, X. (2009). Effects of rosuvastatin on asymmetric dimethylarginine levels and early atrial fibrillation recurrence after electrical cardioversion. Pacing Clin. Electrophysiol. 32, 1562–1566. doi:10.1111/j.1540-8159.2009.02554.x
Yava, A., Koyuncu, A., Tosun, N., and Kiliç, S. (2012). Effectiveness of local cold application on skin burns and pain after transthoracic cardioversion. Emerg. Med. J. 29, 544–549. doi:10.1136/emj.2010.098053
Yetter, E., Brazg, J., Del Valle, D., Mulvey, L., and Dickman, E. (2017). Delayed cardiac tamponade: a rare but life-threatening complication of catheter ablation. Am. J. Emerg. Med. 35, 803. doi:10.1016/j.ajem.2016.11.041
Yost, C. S. (2006). A new look at the respiratory stimulant doxapram. CNS Drug Rev. 12, 236–249. doi:10.1111/j.1527-3458.2006.00236.x
Younes, M., Ostrowski, M., Thompson, W., Leslie, C., and Shewchuk, W. (2001). Chemical control stability in patients with obstructive sleep apnea. Am. J. Respir. Crit. Care Med. 163, 1181–1190. doi:10.1164/ajrccm.163.5.2007013
Yu, T., Deng, C., Wu, R., Guo, H., Zheng, S., Yu, X., et al. (2012). Decreased expression of small-conductance Ca2+-activated K+ channels SK1 and SK2 in human chronic atrial fibrillation. Life Sci. 90, 219–227. doi:10.1016/j.lfs.2011.11.008
Zarauza, J., Lera, M. J. R., Álvarez, C. F., Hernando, J. P., Ceballos, B., Gutiérrez, B., et al. (2006). Relationship between C-reactive protein level and early recurrence of atrial fibrillation after electrical cardioversion. Rev. Esp Cardiol. 59, 125–129. doi:10.1016/S1885-5857(06)60120-5
Zhang, H., Guo, J., Fang, Q., Zheng, Y., Sun, Y., Zhu, W., et al. (2005). Immediate cardioversion of atrial fibrillation and atrial flutter lasting less than 90 days by ibutilide versus propafenone: a multicenter study. Zhonghua yi xue za zhi 85, 798–801. doi:10.1111/j.1368-5031.2005.00705.x
Zhu, G., Liu, C., Qu, Z., Chanchevalap, S., Xu, H., and Jiang, C. (2000). CO inhibits specific inward rectifier K+ channels by decreases in intra-and extracellular pH. J. Cell Physiol 183, 53–64. doi:10.1002/(SICI)1097-4652(200004)183:1¡53::AID-JCP7¿3.0.CO;2-R
Ziff, O. J., Lane, D. A., Samra, M., Griffith, M., Kirchhof, P., Lip, G. Y., et al. (2015). Safety and efficacy of digoxin: systematic review and meta-analysis of observational and controlled trial data. The BMJ 351, h4451. doi:10.1136/bmj.h4451
Zoll, P. M., Linenthal, A. J., Gibson, W., Paul, M. H., and Norman, L. R. (1956). Termination of ventricular fibrillation in man by externally applied electric countershock. N. Engl. J. Med. 254, 727–732. doi:10.1056/NEJM195604192541601
Zoni-Berisso, M., Lercari, F., Carazza, T., and Domenicucci, S. (2014). Epidemiology of atrial fibrillation: European perspective. Clin. Epidemiol. 6, 213–220. doi:10.2147/CLEP.S47385
Keywords: atrial fibrillation, anti-arrhythmic drugs, TASK-1, Cardioversion, KCNK3
Citation: Kraft M, Büscher A, Wiedmann F, L’hoste Y, Haefeli WE, Frey N, Katus HA and Schmidt C (2021) Current Drug Treatment Strategies for Atrial Fibrillation and TASK-1 Inhibition as an Emerging Novel Therapy Option. Front. Pharmacol. 12:638445. doi: 10.3389/fphar.2021.638445
Received: 06 December 2020; Accepted: 21 January 2021;
Published: 04 March 2021.
Edited by:
Michael Curtis, King’s College London, United KingdomReviewed by:
Bin-Nan Wu, Kaohsiung Medical University, TaiwanOscar Casis, Universidad del País Vasco UPV/EHU, Spain
Copyright © 2021 Kraft, Büscher, Wiedmann, L’hoste, Haefeli, Frey, Katus and Schmidt. This is an open-access article distributed under the terms of the Creative Commons Attribution License (CC BY). The use, distribution or reproduction in other forums is permitted, provided the original author(s) and the copyright owner(s) are credited and that the original publication in this journal is cited, in accordance with accepted academic practice. No use, distribution or reproduction is permitted which does not comply with these terms.
*Correspondence: Constanze Schmidt, Constanze.Schmidt@med.uni-heidelberg.de