- 1Department of Pharmacy, Inner Mongolia Medical University, Hohhot, China
- 2Inner Mongolia Hospital of Traditional Chinese Medicine, Hohhot, China
- 3Baotou Centre Hospital, Baotou, China
- 4Pharmaceutical Laboratory, Inner Mongolia Institute of Traditional Chinese Medicine, Hohhot, China
- 5Inner Mongolia Key Laboratory of Characteristic Geoherbs Resources Protection and Utilization, Baotou Medical College, Baotou, China
Immune dysfunction, which is responsible for the development of human diseases including cancer, is caused by a variety of factors. Therefore, regulation of the factors influencing the immune response is a potentially effective strategy to counter diseases. Presently, several immune adjuvants are used in clinical practice to enhance the immune response and host defense ability; however, synthetic drugs can exert negative side effects. Thus, the search for natural products of plant origin as new leads for the development of potent and safe immune boosters is gaining considerable research interest. Plant-based functional foods have been shown to exert several immunomodulatory effects in humans; therefore, the application of new agents to enhance immunological and specific host defenses is a promising approach. In this comprehensive review, we have provided an up-to-date report on the use as well as the known and potential mechanisms of bioactive compounds obtained from plant-based functional foods as natural immune boosters. Plant-based bioactive compounds promote immunity through multiple mechanisms, including influencing the immune organs, cellular immunity, humoral immunity, nonspecific immunity, and immune-related signal transduction pathways. Enhancement of the immune response in a natural manner represents an excellent prospect for disease prevention and treatment and is worthy of further research and development using approaches of modern science and technology.
Introduction
The immune system is one of the most complex biological systems in the body. It is a multifaceted and sophisticated network of specialized organs, cells, proteins, and chemicals, and plays an essential role in conferring protection against various pathogens (such as bacteria, viruses, and fungi), and cancer cells (Carr and Maggini, 2017). It is well known that host immunity is constituted by innate (non-specific) and adaptive (specific) immunity (Figure 1) (Orlowsky and Kraus, 2015; Nicholson, 2016). Immune system dysfunction renders an organism sensitive to pathogens, which can lead to the development of diseases, such as allergic diseases, rheumatoid arthritis, and inflammatory bowel diseases (Williams et al., 2017b; Ding et al., 2018; García et al., 2020). Since immunity is fundamental to the health of the host and plays an important role in preventing diseases, increased research efforts have been engaged at improving immune function. Currently, the clinical application of immunomodulators mainly includes immune adjuvants, such as aluminum hydroxide, Freund’s adjuvant, and albumen adjuvants (Yu et al., 2016; Cronkite and Strutt, 2018). However, these adjuvants have been reported to cause local stimulation, tissue damage, and carcinogenesis. Additionally, neither aluminum hydroxide nor Freund’s adjuvant can induce a strong cellular immune response (Chen et al., 2019). Although albumen adjuvants have recently been used as the primary treatment strategy for improving the immune function of individuals, their application is limited by their high costs and side effects (Chen and Zhan, 2019). It is therefore necessary to explore natural, safer, and more effective adjuvants that can enhance immune systems function by activating immune cells and by modulating immune molecules.
Plant-based functional foods are derived from natural or processed plant foods that contain known or unknown bioactive components (Kumar et al., 2018). In recent years, they have been extensively consumed because of their high bioactive components and health benefits (Mohamad et al., 2020). Notably, growing evidence from pre-clinical research has shown that plant-based functional foods can reduce the risk of developing various disorders, such as diabetes, cancer, cardiovascular disease, hyperlipidemia, and hyperuricemia (Andrea et al., 2017; Gong et al., 2019; Mehmood et al., 2019; Gong et al., 2020; Jiang et al., 2020). Owing to a growing awareness of the capabilities of plant-based functional foods to combat diseases, studies on immune boosters based on plant-based functional foods have received substantial attention, and an increased number of individuals continue to choose plant-based functional foods to improve immune system functions (Davoodvandi et al., 2019; Shafabakhsh et al., 2019). For example, previous studies have reported that active polysaccharides obtained from Ganoderma may evoke an immune defense response against tumor growth, viruses, bacteria, and fungal pathogens via modulation of lymphocytes and myeloid cells, thereby indicating a potential application for the modulation of the host immune system (Ren et al., 2020). Furthermore, astragalus, ginseng, and Ophiocordyceps sinensis have also been reported to exhibit appreciable immune enhancement effects (Chen and Zhan, 2019; Huang et al., 2019; Kim et al., 2019; Chen Z. et al., 2020). In general, the active ingredients of plant functional foods that enhance immunity mainly include polysaccharides, saponins, flavonoids, alkaloids. This review highlights the biological components of plant-based functional foods with immune-boosting effects and their utility in immune-enhancing applications, including those in the development of new treatment strategies for diseases.
Bioactive Components of Plant-Based Functional Foods and Their Immune-Boosting Effects
Polysaccharides
Polysaccharides are a class of natural macromolecules consisting of glycosidically linked carbohydrate monomers (Ferreira et al., 2015). Polysaccharides obtained from natural sources are known to exhibit various biological activities, includg immune regulatory, anti-tumor, and anti-inflammatory activities (Liu M. et al., 2018; Meng et al., 2018). Indeed, the immune-enhancing effects of polysaccharides have garnered considerable attention in recent years because of their low toxicity and few side effects (Huang et al., 2020). Recently, polysaccharides have been shown to possess immune-boosting effects in vitro and in vivo as evidenced by the promotion of immune organ development and the secretion of immune-related molecules. Furthermore, while polysaccharides can promote the activation of the antigen-specific immune system, they can also enhance the innate immune functions of the body, which renders them an ideal potential adjuvant (Sun B. N. et al., 2018). The immune-enhancing effects of the polysaccharides in plant-based functional foods and their mechanisms are summarized in Table 1.
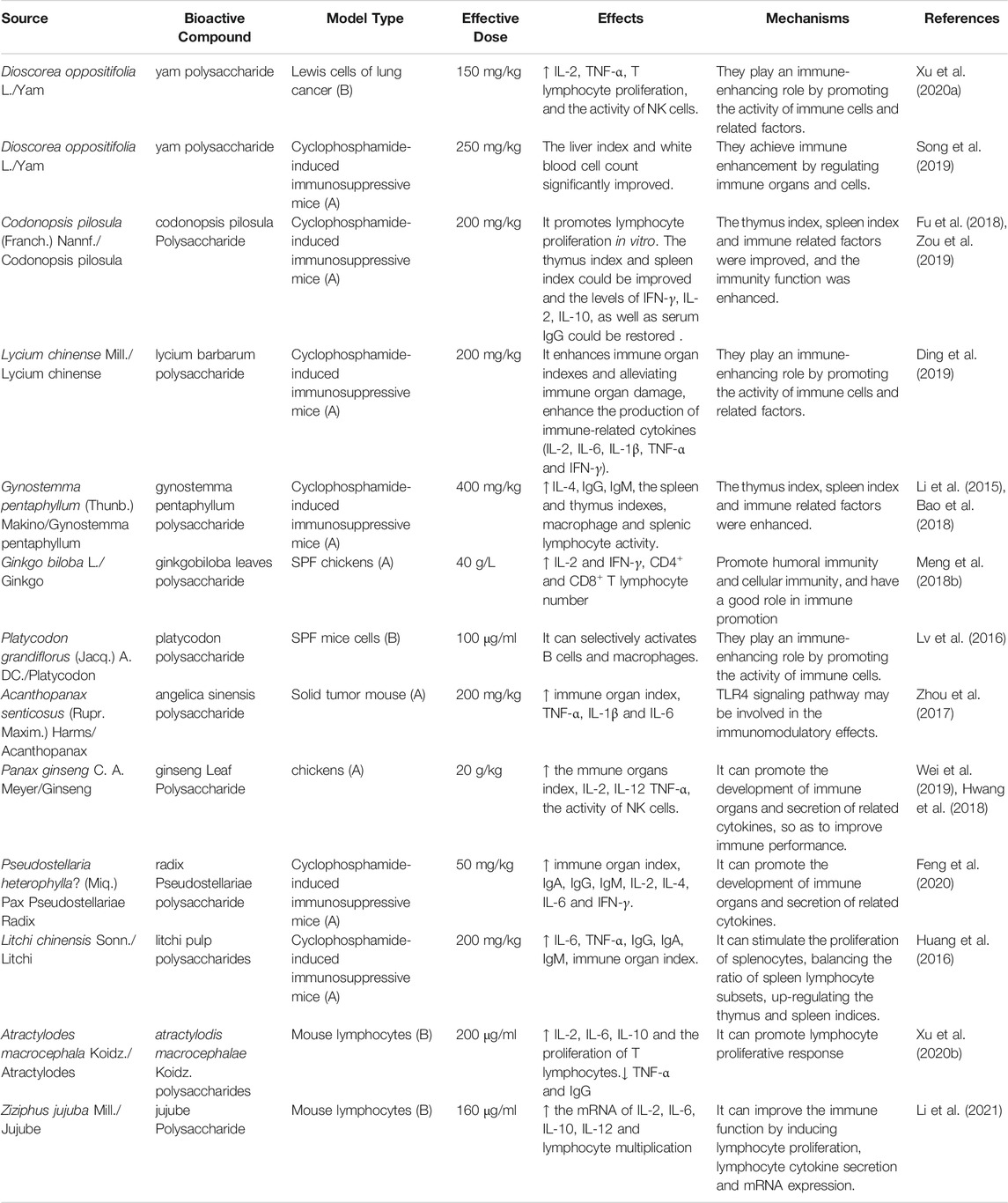
TABLE 1. Experiment and mechanism of polysaccharides bioactive components from plant-based functional foods on immune enhancement.
Extracted mulberry (Morus alba L.) leaf polysaccharides (MLP) exhibit a notable potential for improving immunity. More specifically, MLP can markedly improve the transformation rate of splenic lymphocytes and cytokines and notably increase the thymus index (Xue et al., 2015). Recently, Chen et al. studied the immune enhancement effects of MLP in vitro and demonstrated that, at concentrations of 125 and 250 μg/ml MLP, spleen B and T lymphocyte proliferation was promoted compared with control treatments (p < 0.05). For the in vivo experiments, chickens immunized with the Newcastle disease vaccine, were orally administered with MLP (4 and 8 g/kg); MLP markedly improved the Newcastle disease-associated serum antibody titer and serum IgA concentrations in tracheal and jejunal wash fluids (p < 0.05) (Chen et al., 2019). Another study evaluated the effects of MLP on various immune functions, including serum immunoglobulins and cytokines, in addition to lymphocyte proliferation in weanling pigs. These reports indicated that the thymus and spleen indices in the MLP groups (0.6 and 1.2 g/kg) were noticeably greater (p < 0.05) than those in the control group. Moreover, MLP supplementation elevated the levels of the serum cytokines interleukin (IL)-1, IL-2, IL-6, IL-8, and interferon (IFN)-γ, and increased the lymphocyte transformation rate (Zhao X. J. et al., 2019). These results suggest that MLP can markedly enhance immunity, and therefore can be used as an immune-enhancing drug candidate.
Astragalus polysaccharide (APS) has been proven to be non-toxic in long-term clinical trials (Huang et al., 2019). More importantly, APS exhibits a wide range of pharmacological effects related to immune system regulation, such as enhancing the immune organ index, promoting the proliferation of immune cells, stimulating the release of cytokines, and affecting the secretion of immunoglobulins and the conduction of immune signals (Zheng et al., 2020). Chen et al. used mouse macrophages (RAW264.7 cells) to study the effects of APS (0.1, 0.5, and 1.0 mg/ml) on their morphology and immune function after lipopolysaccharide (LPS) stimulation. Compared with the control group, treatment with 1.0 mg/ml APS markedly inhibited changes in macrophage morphology and the proliferation capacity caused by LPS stimulation; the activity of macrophage acid phosphatase was also significantly increased (p < 0.05). Moreover, at different concentrations, APS significantly alleviated the decrease in alkaline phosphatase activity (p < 0.05) and significantly reduced levels of the LPS-induced pro-inflammatory cytokines IL⁃1β and tumor necrosis factor (TNF-α; p < 0.05). After treatment with 1.0 mg/ml APS, toll-like receptor (TLR) 4, myeloid differentiation primary response gene 88 (MyD88), and nuclear factor-κB (NF-κB) mRNA expression levels in the macrophages were significantly reduced (p < 0.05). Therefore, APS can be used to improve the morphology of macrophages, to restore cell proliferation, to reduce the secretion of pro-inflammatory factors, and to attenuate the immune stress response by regulating the expression of genes related to the TLR4 signaling pathway (Chen Z. et al., 2020). Additionally, one clinical trial investigated the effect of APS injection on the inflammatory cell count and the levels of related factors in 196 patients with bronchial asthma. It was found that an APS injection combined with conventional treatment effectively reduced the inflammatory cell count. After 2 weeks of treatment, levels of IL-6, IL-8, IL-13 IL-17, and TNF-α, and inflammatory cell counts, were significantly lower than those of the control group; additionally, CD3+, CD4+, and CD4+/CD8+ levels were significantly higher than the control group (p < 0.05). The incidence of adverse reactions (two patients with sore throat cases) was 2.04%, which was lower than that in the control group (Qiu et al., 2018).
Thus, polysaccharides can exert their immune activity by activating immune cells. Owing to the complex structures of polysaccharides, they are less soluble in water, which affects their biological activity. Structural modification of polysaccharides may enhance their biological activities, and aid in determining the relationship between their structure and immune function.
Saponins
Saponins are a type of aglycone containing triterpenoids or steroids. They are widely found to possess health-promoting properties in functional foods (He et al., 2019). Extracted plant saponins have shown good performance in various biological studies, demonstrating anti-tumor and immune-enhancing regulatory properties (Gong et al., 2020). Pharmacological studies of a variety of saponin compounds have also shown that they exert immune enhancement effects (Rajput et al., 2007). As an example, the immunological enhancement of gypenosides, which are mainly distributed in Gynostemma pentaphyllum (Thunb.) Makino (Pang et al., 2017), was investigated for immunosuppression in mice. Previously, studies have shown that gypenosides can promote the development of rat immune organs as well as improve specific and non-specific immune functions (Ning et al., 2016). In a recent study, Dan et al. (2020) established a mouse model of immunodeficiency via induction with cyclophosphamide (80 mg/kg). Subsequently, gypenosides (60, 120, and 180 mg/kg) were provided orally for 30 days. Compared to the model group, the peripheral blood white blood cell count, organ index, and CD4+/CD8+ levels were significantly increased (p < 0.01). The effect was more evident in the high-dose gypenoside group. Additionally, gypenosides were found to markedly increase the expression levels of TNF-α, IFN-γ, IL-10, IL-6, and IL-2 in serum, and their mRNA expression in spleen lymphocytes. Thus, gypenosides can enhance the immune function in mice with immunosuppression induced by cyclophosphamide treatment.
Codonopsis pilosula (Franch.) Nannf. is recognized as a medicine food homology species (Jiang et al., 2016); furthermore, the total saponins of C. pilosula (TSCP) have been studied for their immune-enhancing effects in mice with immunosuppression induced by hydrocortisone treatment. Interestingly, oral administration of TSCP enhanced the immune function in a dose-dependent manner (50, 100, and 200 mg/kg) and significantly increased levels of serum IL-2 and IFN-γ, proliferation of spleen T cells, and the killing rate of natural killer (NK) cells (p < 0.05). This indicates that TSCP can antagonize the inhibitory effect of hydrocortisone on T cells and NK cells, thus enhancing the immune function in immunosuppressed mice. Furthermore, compared with the model group, TSCP significantly improved the half hemolysis value for serum hemolysin and the phagocytosis index in the immunosuppressed mice (p < 0.05) (Cao and Wang, 2019). The results indicate that TSCP can enhance the phagocytic activity of mononuclear macrophages and promote the production of antibodies to restore them to the normal level in immunosuppressed mice. These results indicate that TSCP can enhance immunomodulatory effects in terms of cellular immunity, humoral immunity, and non-specific immunity.
In summary, saponins can remarkably enhance immune function in various ways. However, saponins exhibit a hemolytic effect; therefore, the application dosage should be determined appropriately. Moreover, the mechanism of action of saponins relative to the signal pathway of the immune regulatory system warrants clarification. Additional research may help clarify the significance of saponins in food and drug development, which may provide a potential adjuvant for application in the future. The immune enhancement effects of the bioactive components of saponins and their structures are illustrated in Figure 2; experimental studies are summarized in Table 2.
Flavonoids
Flavonoids are an important group of bioactive secondary metabolites found widely in plants (Liu J. et al., 2018). They are also important natural bioactive ingredients in several plant-based functional foods (Jiang et al., 2020). In recent years, the immune-enhancing effects of flavonoids have attracted considerable attention. For example, soybean flavone, sea buckthorn flavone, and quercetin are known to exert good immune regulatory effects, and are highly effective natural immune enhancers, which can enhance cytotoxic T cells and the killing activities of NK cells, promote the release of cytokines, improve the immune organ index, enhance immune function, and promote the body's immune system (Rasouli and Jahanianet, 2015; Kamboh et al., 2016). Additionally, flavonoids can inhibit the production of pro-inflammatory cytokines by regulating NF-κB expression (Huang et al., 2018).
Hesperidin is a beneficial bioactive ingredient mainly found in citrus fruits (Tejada et al., 2018). A recent study investigated the effects of oral hesperidin on the systemic immune system of rats after completion of intense exercise regimens. Supplementation of hesperidin (200 mg/kg) significantly reduced the leukocytosis induced by intensive exercise, increased the cytotoxicity of NK cells, and increased the proportion of phagocytic monocytes and T helper cells in thymus, blood, and spleen. Additionally, cytokine (IL-6, IFN-γ) secretion in peritoneal macrophages decreased (p < 0.01) (Ruiz-Iglesias et al., 2020). Moreover, in vitro studies have shown that hesperidin can play an anti-inflammatory role by mediating immune-related pathways, namely the NF-κB pathway (Birsu Cincin et al., 2015).
Lonicera japonica is recognized as an edible and medicinal species (Zhang B. et al., 2019). In this context, L. Japonica flavone has been studied for its immunomodulation effects in mice with dexamethasone-induced immunosuppression. Compared with the model group, L. japonica flavone (400 mg/kg) significantly improved the organ indices (p < 0.01). This indicates that it exerts remarkable protective effects on the spleen and thymus. Moreover, the flavone significantly enhanced the activities of non-specific immune factors (alkaline and acid phosphatases) in serum. L. japonica flavone could significantly increase the content of superoxide dismutase, as well as reduce the activities of monoamine oxidase and malondialdehyde in spleen and thymus in immunosuppressed mice (p < 0.01) (Pi et al., 2015). Recently, a clinical trial was conducted to investigate the effect of honeysuckle soup combined with benzathine penicillin on serum IL-8 and TNF-α levels in patients with syphilis. The serum IL-8, and TNF-α levels were significantly lower than those in benzathine penicillin-treated patients (p < 0.05) (Ni and Zhu, 2020). Thus, this suggests that L. japonica extract exhibits good immunomodulatory effects.
In recent years, the immune-enhancing effect and mechanism of action of flavonoids have been studied extensively; however, their clinical application as immunoregulatory drugs is limited because of their poor pharmacokinetic profile (De Ferrars et al., 2014). Furthermore, the large number of active sites, relatively slow efficacies, and the lack of specificity and selectivity towards certain diseases has limited the clinical application of flavonoids. It is believed that a gradual elucidation of the immune enhancement effect and mechanism of action of flavonoids will lay a foundation for the preliminary study of clinical immune enhancement applications. The immune enhancement effects of flavonoids from plant-based functional foods are summarized in Table 3; their structures are illustrated in Figure 3.
Alkaloids
Alkaloids are nitrogenous compounds other than proteins, peptides, amino acids, and vitamin B that mainly exist in the plant kingdom (Song and Jiang, 2017). Owing to their complex structures and remarkable biological activities, their roles in immune enhancement should not be ignored (Jiang et al., 2020). Indeed, it is known that alkaloids play an immune-enhancing role by regulating the proliferation of thymic and splenic lymphocytes and the secretion of cytokine (Zhou et al., 2020). The immune enhancement of alkaloid structures is illustrated in Figure 4.
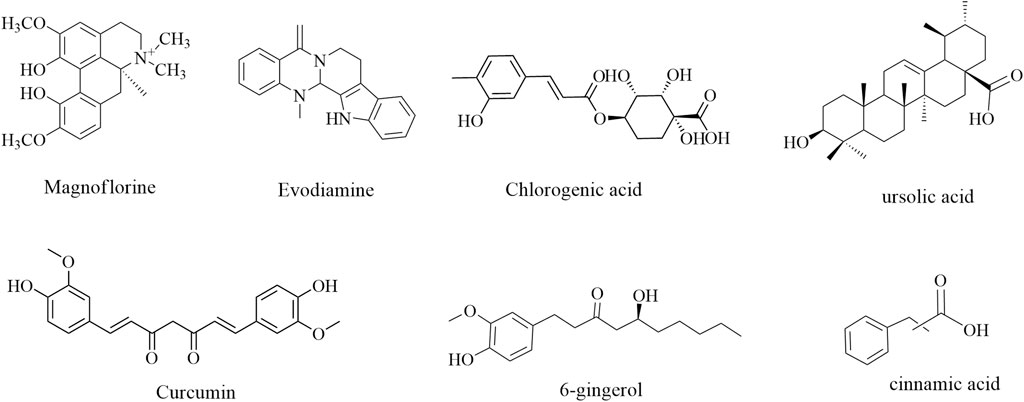
FIGURE 4. The immune enhancement effects of alkaloids and other components in plant-based functional food.
Magnoflorine has been isolated from Magnolia officinalis Rehd. et Wils and shown to activate the NF-κB and phosphatidylinositol 3-kinase-protein kinase B (PI3K-Akt) signaling pathways by promoting the expression of MyD88 and TLR4 to enhance the immune function of macrophages stimulated by LPS. The results showed that magnoflorine (50 μg/ml) can enhance the upregulation of TNF-α, IL-1β. Additionally, magnoflorine treatments augmented the phosphorylation of extracellular signal-regulated kinase (ERK), c-Jun N-terminal kinase (JNK), and p38 MAPKs (Haque et al., 2018). Moreover, magnoflorine can also promote the proliferation of spleen cells induced by LPS in rats, enhance the secretion of TH1 and TH2 cytokines, and elevate the number of CD4+ T cells (Ahmad et al., 2015).
Evodiamine is the main active component of Euodia rutaecarpa (Juss) Benth, and has been shown to regulate mouse immunity (Song et al., 2015). Evodiamine inhibits the proliferation of thymic and splenic lymphocytes induced by concanavalin A (ConA) in mice. More specifically, the results of ELISA tests showed that the release of IL-2 and IL-12 in the spleen and thymus cells of mice treated with evodiamine was significantly lower than that in the control group (p < 0.05). Furthermore, analysis of reverse transcription polymerase chain reaction results showed that the mRNA levels of Bcl-12 and CDK2 in cells treated with 0.75 μmol/L evodiamine were significantly higher than those in the control group. Compared to that of the control group, treatment with 0.75 μmol/L evodiamine induced apoptosis of the thymic and splenic cells in mice (p < 0.05) (Song et al., 2008).
Although alkaloids have been shown to play an immunomodulatory role by promoting or by suppressing the activation or differentiation of immune cells and by regulating cytokine expression, a lack of clinical data implies that further studies should be conducted in the context of clinical trials and toxic doses.
Others
In addition to the above-mentioned bioactive ingredients, other components (e.g., terpenoids, essential oils, and organic acids) have also been found to exhibit immune enhancement effects. For example, 6-gingerol is the main component of ginger (Zingiber officinale Rosc.) The infiltration of CD4+/CD8+ T cells and B cells was increased, and the number of CD4+ T cells was decreased, in tumor-bearing mice after treatment with 6-gingerol (Fan et al., 2021). Moreover, the cinnamic acid present in cinnamon are known to elevate the levels of white blood cells (Cheng et al., 2017). The immune enhancement effects of others are summarized in Table 4; their structures are illustrated in Figure 4.
Mechanisms of the Immune-Enhancing Effects of Plant-Based Functional Foods
Influence on Immune Signal Transduction
The TLR/NF-κB Signaling Pathway
NF-κB is one of the key factors that regulates cell gene transcription (Figure 5). The IκB kinase (IKK) complex is activated to catalyze the phosphorylation of IκB and to establish interaction with NF-κB. Activated NF-κB is transported to the nucleus, where it directly initiates and regulates the transcription of genes involved in the immune response, and regulates the expression of cytokines and adhesion molecules (Li and Verma, 2002). NF-κB signaling is critical for the expression of inflammatory cytokines. When cells are exposed to inflammatory stimuli, the stimuli act by regulating inflammation through the IKK-IκB-NF-κB inflammatory cytokine pathway (Yang et al., 2017). Therefore, NF-κB can regulate inflammatory mediators, cytokines, and adhesion molecules, affecting the innate or acquired immune response, inflammatory response, tumor growth, and other biological functions in the body (Wang et al., 2019). The appropriate intervention of NF-κB signaling after inflammation may be important to reduce further inflammation damage and the induction of other diseases (Hou et al., 2021). Importantly, recent studies have shown that bioactive substances from plant-based functional foods can exert an immunomodulatory effect by inhibiting NF-κB activation, and therefore migration (Yang et al., 2017).
TLRs are a class of innate immune-related pattern recognition receptors (PRRs). TLRs can initiate innate immunity immediately upon infection by identifying the pathogen, and by initiating acquired immunity through signal transduction, which plays an important defensive role against a variety of microbes (Fitzgerald and Kagan, 2020). TLR signal transduction pathways can be divided into MyD88-dependent and non-MyD88-dependent pathways according to the different receptor proteins involved in the signal transduction process. MyD88-dependent pathways can be mainly divided into two types, namely the TLR-MyD88/IRAK-mitogen-activated protein kinase (MAPK) pathway and the TLR-MyD88/IRAK-NF-κB pathway (Fitzgerald and Kagan, 2020). More specifically, MyD88 establishes interaction with IRAK to recruit downstream signaling molecules to induce the activation of NF-κB and activator protein-1, and to secrete pro-inflammatory cytokines to activate innate immune responses. The non-MyD88-dependent approach mainly involves TLR3 and TLR4, which play important roles in the immune response (Bahramabadi et al., 2019). TLR4 is mainly expressed in macrophages, although it also plays a role in the recognition of LPS and certain endogenous heat shock proteins (Yang et al., 2017).
Studies have also shown that TLRs can be stimulated by components present in plant-based functional foods; NF-κB in the nucleus is activated, promoting cytokine secretion and up-regulating the expression of co-stimulating molecules, thereby playing an immune-enhancing role. For example, APS can confer protection against sepsis-induced cardiac dysfunction by inhibiting expression of the TLR4/NF-κB pathway (Xu et al., 2019).
MAPK Signaling Pathways
MAPKs are a group of threonine-serine protein kinases, which can respond to a wide range of extracellular stimuli; they constitute one of the most important signal transduction systems (Gong and Jiang, 2003). Under external stimulation, MAPK is activated via double site phosphorylation, promoting phosphorylation of transcription factors that enter the nucleus and regulating transcription of related genes (Wang J. T. et al., 2019). Three main MAPK family members are known, namely ERK, JNK, and p38MAPK, which play important roles in the regulation of cell proliferation, inflammation, apoptosis, and other signal transduction pathways (Cai et al., 2020). Dong et al. showed that hesperidin could selectively regulate the MAPK pathway to influence the cellular immune response. More specifically, hesperidin upregulates the expression and activation levels of p38MAPK and JNK, thereby enhancing cellular autonomic immunity (Dong et al., 2014). Additionally, sea buckthorn flavonoids can also inhibit the phosphorylation of p38 and the MAPK pathway of stress-activated protein kinase/JNK, thus reducing the immune inflammatory effect (Jiang et al., 2017). Licorice was shown to inhibit the expression of p38MAPK, ERK1/2, and JNK, and alleviated the immune inflammatory response in mice with myocardial fibrosis (Zhang Y. et al., 2016). These results suggest that active ingredients can play immune-enhancing roles by decreasing the phosphorylation levels of ERK, JNK, and p38MAPK.
Promotion of Immune Cytokine Production
Cytokines are small, low-molecular-weight proteins that have both autocrine and paracrine functions in immune cells, and are responsible for the regulation of the immune function of the body and participation in certain inflammatory reactions (Conlon et al., 2019). Immune cytokines not only affect the immune and hematopoietic systems, but also extensively affect the nervous, endocrine, and cardiovascular systems (McComb et al., 2019). Cytokines are classified according to their different functions, namely ILs, chemokines, lymphokines, IFNs, and the TNF family. As the most extensively secreted cytokine, ILs play an important role in the regulation of intercellular immunity and inflammation (McComb et al., 2019). Rapid advances in the therapeutic use of IL-2 and IL-10 against cancer have recently been achieved (Qiao and Fu, 2020). IFN is a subset of cytokines; they possess anti-viral, anti-tumor, and immune regulation abilities (McComb et al., 2019). The TNF family can be divided into two types, namely TNF-α and TNF-β, which can participate in immune regulation and inflammation (McComb et al., 2019). Among them, TNF-α is a class of dual action cytokines that play a dominant role in the regulation of inflammation (Wang et al., 2020d). Normally, cytokines play an important role in the maintenance of body homeostasis by regulating the body's immune response at a low level by controlling the development, differentiation, and function of immune cells (Zhang L. L. et al., 2020). On the contrary, a variety of pro-inflammatory cytokines are rapidly and considerably produced in body fluids, which can affect cell function, intracellular signaling pathways, and diseases (Du et al., 2021). Presently, plant-based functional foods are known to enhance immunity by promoting the production of cytokines. However, certain components in functional foods can inhibit the increase in cytokines caused by the inflammatory response, thereby protecting cells in the body (Zheng et al., 2020).
Wang et al. used RAW264.7 macrophages to study the anti-inflammatory and immunomodulatory effects of TFA. After a treatment period of 12 h, 10 μg/ml TFA significantly increased the secretion of IL-1β, IL-6, and TNF-α, and the mRNA levels of IL-1β, IL-6, and TNF-α in normal RAW264.7 cells. However, TFA (10, 25, and 100 μg/ml) inhibited the overexpression of IL-1β, IL-6, and TNF-α and their enhanced transcription levels in LPS-stimulated RAW2.7 cells in a dose-dependent manner. TFA enhances cytokine and mRNA levels under normal conditions, and inhibits the excessive release of proinflammatory cytokines and mRNAs under the stimulation of LPS, thus exerting its anti-inflammatory and immunological bimodulation effect (Wang M. et al., 2020).
Promotion of Innate Immunity Function
Innate immunity comprises a series of defense mechanisms that play crucial roles in the initiation and action of specific immunity. Thus far, various studies have found that the regulation of innate immune cells, such as NK cells, DCs, and macrophages, plays an immune-enhancing role (Liu C. H. et al., 2017).
Effects on NK Cells
NK cells are a type of immune cell closely related to responses during tumor formation, virus-associated infections, and immune regulation; they are the first line of defense in the human body (Russick et al., 2020). NK cells express several activation and inhibitory receptors, and secrete cytokines and chemokines to enable interaction with other immune cells (Vivier et al., 2018). Additionally, NK cells play a key role in tumor immune surveillance, generating a coordinated anti-tumor immune response through their cytotoxic effect function and their ability to interact with other immune cells (Morsink et al., 2020). Regulation of the activity of NK cells can strengthen the immune system against diseases.
Maatouk et al. investigated the ability of naringenin (5, 10, and 21 μg/ml) and heated naringenin (4, 6, and 8 μg/ml) to enhance NK activity against K562 myelogenous leukemia cells. Their findings revealed that naringenin and heated naringenin improved the NK cell lysis activity at concentrations of 5 and 6 μg/ml, respectively, whereby for heated naringenin, this enhancement was dose-dependent. Notably, naringenin treatment resulted in an enhanced NK cytotoxic activity against these target cells (Maatouk et al., 2016). Furthermore, Valentová et al. (2016) showed that rutin exposure also increased the killing activity of NK cells; rutin is commonly found in the normal human diet and is increasingly used in food supplements.
Effects on DCs
DCs are the most effective antigen-presenting cells in the innate immune system that play a vital role in both immune homeostasis and antitumor activity. As key immune sentinels, these cells initiate and regulate adaptive immune responses by integrating and transmitting a substantial number of afferent signals to lymphocytes (Ding et al., 2018). Additionally, a myriad bioactive components play immunomodulatory roles by promoting the development and maturation of DCs. Therefore, modulation of the DC activity to ameliorate autoimmune diseases may be effective (Lin et al., 2017; Lin et al., 2020).
Proanthocyanidins are found in dietary components (Kong et al., 2018). Williams et al. (2017a) suggested that proanthocyanidins could induce the formation of an anti-inflammatory phenotype in human DCs, resulting in the selective downregulation of the TH1 response in naive T cells. Furthermore, Zhang et al. (2015) showed that chrysin could inhibit the functional differentiation and maturation of DCs and improve the inflammatory response in experimental autoimmune encephalomyelitis.
Effects on the Mononuclear Phagocyte System (MPS)
The MPS consists of immune effector cells distributed in blood, lymph, and tissues. Macrophages are key host defenses against pathogens and play an important role in the immune system, including antigen presentation, phagocytosis of pathogens, secretion of various cytokines, and activation of immune responses (Tao et al., 2020). Macrophages can phagocytize pathogens directly, but also indirectly attack pathogens through the release of cytotoxic molecules such as nitric oxide (NO) and secretion of cytokines, including TNF-α and IL-6, to perform immune functions (Zhang X. X. et al., 2016; Dong et al., 2019). Biologically active ingredients derived from plant-based functional foods can activate macrophages, enhance phagocytosis and antigen presentation, and promote the secretion of relevant active molecules (Lv et al., 2016). Thus, macrophages are ideal target cells for a variety of immunomodulatory and anti-inflammatory drugs.
Ginseng polysaccharide (GPS) is extracted from Panax ginseng C. A. Meyer (Zhao B. et al., 2019). In one study, the effects of GPS on the morphology and immune function of LPS-induced mouse macrophages (RAW264.7) were determined. The results showed that GPS significantly improved macrophage morphology and restored proliferation. Compared with the LPS group, treatment with 1 mg/ml GPS significantly increased macrophage acid phosphatase activity, while treatments with 0.5 and 1 mg/ml GPS significantly reduced alkaline phosphatase activity caused by LPS stimulation (Chen G. Y. et al., 2020). The active components of Platycodon grandiflorus are mainly saponins, among which platycodin D is the main saponin constituent (Ji et al., 2020). Li et al. (2019) explored the effect of platycodin D on the immune function of mouse macrophage phagocytosis using an MTT assay. Compared with the control group, platycodin D (25, 50, 75, and 100 μg/ml) treatment significantly promoted the proliferation of lymphocytes and enhanced macrophage phagocytosis (p < 0.01). The group treated with 50 μg/ml platycodin D exhibited a more significant effect than that of the other groups (p < 0.01). Furthermore, platycodin D treatment stimulated the secretion of TNF-α and IL-12 in macrophages, with 50 μg/ml being the optimal concentration. Overall, it was found that platycodin D enhanced the activity of mouse macrophages.
Enhanced Adaptive Immunity
Initial immune cells exhibit broad specificity against pathogens, whereas the adaptive immune system cells exhibit a highly specific immune response to a specific antigen (Wang et al., 2020d).
Enhanced Cellular Immunity
Cellular immunity is the immune response mediated by T lymphocytes (Nicholson, 2016). In cellular immunity, antigens are processed by antigen-presenting cells into peptides that bind to the major histocompatibility complex (MHC) and produce an activated T-cell receptor signal. The antigen binds to relevant receptors on the surface of T lymphocytes to produce a signal, and the T lymphocytes proliferate and differentiate rapidly with a few of them becoming sensitized lymphocytes. Among them, cytotoxic T cells (Tc) can lead to the rupture and death of exogenous cells, and T helper (Th) cells secrete cytokines such as ILs, which stimulate Tc and various phagocytes to accumulate around foreign cells and result in complete removal. Toward the end of this response, T suppressor (Ts) cells exhibit functions and halt the immune response by inhibiting the action of other lymphocytes (Nicholson, 2016; Wang and Lin, 2019). A series of studies have shown that plant-based functional foods can promote lymphocyte transformation and improve cellular immune function. (Yang et al., 2017).
As an example, lentinan has been shown to increase macrophage toxicity to metastatic tumors by regulating the function of immune cells such as T lymphocytes and macrophages at multiple levels (Ahn et al., 2017). More specifically, lentinan can promote T lymphocyte proliferation and enhance the T lymphocyte activity to improve the host body balance. Additionally, lentinan has been shown to stimulate T cells and improve survival in cancer patients (Wang J. T. et al., 2019).
Enhanced Humoral Immunity
Humoral immunity is mainly mediated by B cells, that is, plasma cells produce antibodies to protect the immune mechanism. The antibody titer reflects the affinity and immune response of the antibody to the antigen. The content of hemolysin in serum is an important indicator of humoral immune function (Xing et al., 2020). In this context, the active components of plant-based functional foods have been found to confer protection to the body by producing antibodies to accelerate lymphocytic phagocytosis and clearance by phagocytes (Yang et al., 2017).
The effect of polysaccharide extracted from the herb Gastrodia elata on humoral immunity in immunodeficient mice was studied following induction using cyclophosphamide (Li et al., 2016). After completion of a 10 days treatment, G. elata polysaccharide medium-dose (200 mg/kg) and high-dose groups (400 mg/kg) exhibited significantly increased serum IgA, IgG, and hemolysin levels (p < 0.01). Furthermore, the spleen index and thymus index in the high-dose polysaccharide group were increased, and serum IgM levels in the medium-dose polysaccharide group were significantly increased (p < 0.05). G. elata polysaccharide can alleviate the inhibitory effect of cyclophosphamide on humoral immune function in mice.
Promotion Effect on Immune Organs Function
The immune system is a defense network encompassing the entire body. Immune function is dependent on the immune organ to produce a considerable number of immune cells to regulate the immune response and to prevent the spread of infection (Figure 6; Yang et al., 2017; McComb et al., 2019). All blood cells originate from the same precursor hematopoietic stem cells, but their sites of maturation and residence differ, and the cells are mainly divided between the central immune organ and the peripheral immune organ (McComb et al., 2019). The thymus, as the primary immune organ, is mainly involved in the cellular immune response, whereas the spleen, as a peripheral immune organ, is involved in humoral immunity (Nicholson, 2016; Sun T. T. et al., 2018). Thus far, several studies have shown that components of plant-based functional foods can act on various immune organs, enhancing the immune response by increasing the organ weight, by improving the organ index (a reflection of a change in body immune function), and by promoting the development of partial visceral organs (Li X. Q. et al., 2020).
For example, Lycium barbarum polysaccharides (LBP) exhibit an enhancing effect on the immune organ index, which has been confirmed in a few studies (Hao et al., 2015; Zhao et al., 2015). Furthermore, Tang and He (2013) evaluated the effects of LBP on a D-galactose aging mouse model and found that the thymus index and spleen index were significantly increased in the LBP (3 g/kg) groups compared to those of the control (p < 0.01). Therefore, LBP can effectively protect the immune organs and enhance the immune ability of the body. Furthermore, Kamboh et al. (2016) investigated the immunomodulatory effects of hesperidin on LPS-induced broilers and showed that 20 mg/kg hesperidin treatment significantly increased the bursa and spleen index at 21 and 42 days compared to the control (p < 0.05). These results indicate that the bioactive components present in plant-based functional foods promote the growth and development of the immune organs and exhibit immune-enhancing effects.
Conclusion
Immune dysfunction in the body can be caused by a variety of factors. To address this issue, plant-based functional foods have received increased attention because of their extensive immune-enhancing properties. As a result, various studies have attempted to elucidate the cellular and molecular regulatory mechanisms and signaling pathways of immunoactive ingredients to determine the immune-enhancing effects of these active ingredients. Unlike current drugs, which are expensive and can result in a variety of side effects, plant-based functional foods result in fewer side effects, are stable, and tend to exhibit a lasting efficacy. With the discovery and utilization of functional foods, suitable candidates among the vast range of natural products are being identified and characterized in detail. However, the application of bioactive compounds as immune factors has certain limitations; thus, we herein propose the following points and recommendations: 1) Although certain plant-based functional foods have been reported to exhibit beneficial effects in enhancing immunity, their bioactive components have not been fully elucidated and identified; hence, this should serve as a major focus for further investigations. 2) Presently, it is difficult to correlate the structures and activities of complex bioactive constituents (e.g., polysaccharides); thus, a study of the relationship between the structures and efficacies of bioactive components from plant-based functional foods is necessary. 3) Various active ingredients derived from plant-based functional foods remain to be tested in clinical trials. In this context, it should be noted that the effects observed in animal models may differ from those in humans, rendering human clinical trials essential. 4) The in vivo environment is more complex than the in vitro environment; thus, additional in vivo studies should be performed to further elucidate the mechanisms underlying the immune-enhancing capabilities of bioactive components obtained from plant-based functional foods. The molecular mechanisms underlying the action of such compounds should be studied intensively. 5) A few reports on the immune enhancement effects of bioactive components from plant-based functional foods originate from poor-quality research attributed to inadequate methods. Therefore, high-quality studies of such bioactive components are warranted to establish their efficacy and to provide a more convincing theoretical basis for the synthesis of novel immune-boosting drugs.
Author Contributions
Conceptualization, ML, GZ, and LJ Writing—original draft preparation, LJ, YL Writing—review and editing, LJ Supervision, ML and GS All authors have read and agreed to the published version of the manuscript.
Funding
This research was funded by the National Natural Science Foundation of China (No. 81874336).
Conflict of Interest
The authors declare that the research was conducted in the absence of any commercial or financial relationships that could be construed as a potential conflict of interest.
Acknowledgments
I would like to thank Li Xue and Ji Mingyue for their suggestions on revision during this period. At the same time, I would like to thank Editage for helping with the English language.
Abbreviations
APS, Astragalus polysaccharide; GPS, ginseng polysaccharide; LBP, Lycium barbarum polysaccharides; TSCP, total saponins of Codonopsis pilosula; MLP, mulberry leaf polysaccharides; LPS, lipopolysaccharide; ConA, concanavalin A; NO, nitric oxide; CD3, cluster of differentiation 3; DCs, dendritic cells; NK, natural killer; IL, interleukin; INF, interferon; TNF, tumor necrosis factor; TLR, toll-like receptor; ERK, extracellular signal-regulated kinase; IKK, IκB kinase; JNK, c-Jun N-terminal kinase; MAPK, mitogen-activated protein kinase; MPS, mononuclear phagocyte system; NF-κB, nuclear factor-κB; PRRs, pattern recognition receptors; MyD88, myeloid differentiation primary response gene 88; PI3K-Akt, phosphatidylinositol 3-kinase-protein kinase B
References
Ahmad, W., Jantan, I., Kumolosasi, E., and Bukhari, S. N. (2015). Immunostimulatory effects of the standardized extract of tinospora crispa on innate immune responses in wistar kyoto rats. Drug Des. Devel Ther. 9, 2961–2973. doi:10.2147/DDDT.S85405
Ahn, H., Jeon, E., Kim, J. C., Kang, S. G., Yoon, S. I., Ko, H. J., et al. (2017). Lentinan from shiitake selectively attenuates AIM2 and non-canonical inflammasome activation while inducing pro-inflammatory cytokine production. Sci. Rep. 7, 314. doi:10.1038/s41598-017-01462-4
Andrea, K., Patrik, S., Peter, K., Pavol, Z., Sona, U., Martin, K., et al. (2017). Are plant-based functional foods better choice against cancer than single phytochemicals? a critical review of current breast cancer research. Biomed. Pharmacother. 96, 1465–1477. doi:10.1016/j.biopha.2017.11.134
Bahramabadi, R., Dabiri, S., Iranpour, M., and Kazemi Arababadi, M. (2019). TLR4: an important molecule participating in either anti-human papillomavirus immune responses or development of its related cancers. Viral Immunol. 32, 417–423. doi:10.1089/vim.2019.0061
Bao, F. X., Tao, L. X., and Zhang, H. Y. (2018). Research progress on pharmacological effects of Gynostemma pentaphyllum active ingredients. Chin. J. New Drugs Clin. Rem. 37, 11–17. doi:10.14109/j.cnki.xyylc.2018.01.003
Bi, S. C., Ma, X. D., Wu, Y., Cui, X. M., and Hu, S. H. (2019). Oral administration of ginsenoside Rg1 enhances gut mucosal immunity in chickens. Chin. J. Vet. Sci. 39, 2215–2221. doi:10.16303/j.cnki.1005-4545.2019.11.21
Birsu Cincin, Z., Unlu, M., Kiran, B., Sinem Bireller, E., Baran, Y., and Cakmakoglu, B. (2015). Anti-proliferative, apoptotic and signal transduction effects of hesperidin in non-small cell lung cancer cells. Cel. Oncol. (Dordr) 38 (3), 195–204. doi:10.1007/s13402-015-0222-z
Cai, G., Sun, K., Xia, S., Feng, Z., Zou, H., Gu, J., et al. (2020). Decrease in immune function and the role of mitogen-activated protein kinase (MAPK) overactivation in apoptosis during T lymphocytes activation induced by zearalenone, deoxynivalenol, and their combinations. Chemosphere 255, 126999. doi:10.1016/j.chemosphere.2020.126999
Cao, F. H., and Wang, Y. P. (2019). Effect of total saponins of Codonopsis pilosula nanoemulsion on immunologic function of mice. J. Northwest A&F Univ. (Nat. Sci. Edit) 47 (05), 125–131. doi:10.13207/j.cnki.jnwafu.2019.05.016
Carr, A., and Maggini, S. (2017). Vitamin C and immune function. Nutrients 9 (11), 1211. doi:10.3390/nu9111211
Chai, Z., Zhang, J. J., Sun, S. J., Wei, K. Z., Yan, J. L., and Wei, J. Z. (2018). Study on effects of anti-aging and immune regulation of Polygala saponins.China J. Trad. Chin. Med. Pharm. 33, 704–707. CNKI:SUN:BXYY.0.2018-02-083
Chen, Q. Y., and Zhan, J. H. (2019). The immunomodulatory effect of traditional chinese medicine and its research progress. Jiangxi Med. J. 54, 181–184. doi:10.3969/j.issn.1006-2238.2019.2.032
Chen, H. Z., Luo, H. N., Quan, B. Y., and Yi, C. X. (2017a). Effect of Rg3 on the expression of CD 80/86 and cellular immune function in patients with nasopharyngeal carcinoma after radiotherapy. Jilin J. Trad. Chin. Med. 37 (12), 1211–1214. doi:10.13463/j.cnki.jlzyy.2017.12.007
Chen, M. Z., Li, Y., Chen, W., and He, W. (2017b). Regulating effect of total momordicoside to immune in IgA nephropathy of rats. J. Liaoning Univ. Trad. Chin. Med. 19 (07), 46–49. doi:10.13194/j.issn.1673-842x.2017.07.011
Chen, X. L., Sheng, Z. C., Qiu, S. L., Yang, H. F., Jia, J. P., Wang, J., et al. (2019). Purification, characterization and in vitro and in vivo immune enhancement of polysaccharides from mulberry leaves. PLoS One 14, e0208611. doi:10.1371/journal.pone.0208611
Chen, G. Y., Han, G. J., Zhang, L. L., Li, H., and Yang, C. M. (2020). Immune regulation of ginseng polysaccharide on lipopolysaccharide-stimulated mouse macrophages. Chin. J. Anim. Sci. 1-11. doi:10.19556/j.0258-7033.20200323-10
Chen, Z., Liu, L., Gao, C., Chen, W., Vong, C. T., Yao, P., et al. (2020). Astragali radix (Huangqi): a promising edible immunomodulatory herbal medicine. J. Ethnopharmacol. 258, 112895. doi:10.1016/j.jep.2020.112895
Cheng, C. Y., and Yeh, C. C. (2019). Adaptive immunoregulation of luteolin and chlorogenic acid in lipopolysaccharide-induced interleukin-10 expression. Ci Ji Yi Xue Za Zhi. 32, 186–192. doi:10.4103/tcmj.tcmj_23_19
Cheng, Q., Guo, S, S., Ding, B. Y., Li, Y. H., and Xia, Y. (2017). Effects of dietary coated-cinnamon on non-specific immun function of broiler chickens. Chin. Poult. 39 (18), 28–33. doi:10.16372/j.issn.1004-6364.2017.18.006
Chi, X., Bi, S., Xu, W., Zhang, Y., Liang, S., and Hu, S. (2017). Oral administration of tea saponins to relive oxidative stress and immune suppression in chickens. Poult. Sci. 96, 3058–3067. doi:10.3382/ps/pex127
Conlon, K. C., Miljkovic, M. D., and Waldmann, T. A. (2019). Cytokines in the treatment of cancer. J. Interferon Cytokine Res. 39, 6–21. doi:10.1089/jir.2018.0019
Cronkite, D. A., and Strutt, T. M. (2018). The regulation of inflammation by innate and adaptive Lymphocytes. J. Immunol. Res. 2018, 1467538. doi:10.1155/2018/1467538
Dan, B., Li, L. Y., He, J., and Chen, X. H. (2020). Immunoregulatory effects of gypenosides on cyclophosphamide-induced immunosuppression in mice. Northwest Pharm. J. 35, 680–684. doi:10.3969/j.issn.1004-2407.2020.05.011
Davoodvandi, A., Sahebnasagh, R., Mardanshah, O., Asemi, Z., Nejati, M., Shahrzad, M. K., et al. (2019). Medicinal plants as natural polarizers of macrophages: phytochemicals and pharmacological effects. Curr. Pharm. Des. 25 (30), 3225–3238. doi:10.2174/1381612825666190829154934
De Ferrars, R. M., Czank, C., Zhang, Q., Botting, N. P., Kroon, P. A., Cassidy, A., et al. (2014). The pharmacokinetics of anthocyanins and their metabolites in humans. Br. J. Pharmacol. 171 (13), 3268–3282. doi:10.1111/bph.12676
Ding, S. J., Jiang, H. M., and Fang, J. (2018). Regulation of immune function by polyphenols. J. Immunol. Res., 1–8. doi:10.1155/2018/1264074
Ding, Y., Yan, Y., Chen, D., Ran, L., Mi, J., Lu, L., et al. (2019). Modulating effects of polysaccharides from the fruits of Lycium barbarum on the immune response and gut microbiota in cyclophosphamide-treated mice. Food Funct. 10, 3671–3683. doi:10.1039/c9fo00638a
Dong, W., Wei, X., Zhang, F., Hao, J., Huang, F., Zhang, C., et al. (2014). A dual character of flavonoids in influenza a virus replication and spread through modulating cell-autonomous immunity by MAPK signaling pathways. Sci. Rep. 4, 7237. doi:10.1038/srep07237
Dong, Z., Zhang, M. M., Li, H. X., Zhan, Q. P., Lai, F. R., and Wu, H. (2019). Structural characterization and immunomodulatory activity of a novel polysaccharide from Pueraria lobata (Willd.) ohwi root. Int. J. Biol. Macromol. 154, 1556–1564. doi:10.1016/j.ijbiomac.2019.11.040
Du, F. Y., Xue, G. J., Liu, Z. B., and Chen, G. L. (2021). Research advances of cytokine storm and therapeutics. Chin. J. Med. Chem. 31 (01), 39–54. doi:10.14142/j.cnki.cn21-1313/r.2021.01.005
Fan, Q. L., Li, H., Jiang, S. Q., Li, L., and Ye, J. L. (2021). Effect of capsaicin, gingerol, allicin, and essential oil on growth performance, carcass performance, antioxidant and immune function of broiler chickens. Feed Industry 42 (02), 7–12. doi:10.13302/j.cnki.fi.2021.02.002
Feng, D., Hao, S. Y., Fu, Y., Wang, X. H., Du, X. H., and Liu, H. R. (2020). Effect of Radix Pseudostellariae polysaccharide injection on immune function of immunosuppressive mice. J. Trad. Chin. Vet. Med. 39, 74–77. doi:10.13823/j.cnki.jtcvm.2020.03.019
Ferreira, S. S., Passos, C. P., Madureira, P., Vilanova, M., and Coimbra, M. A. (2015). Structure-function relationships of immunostimulatory polysaccharides: a review. Carbohydr. Polym. 132, 378–396. doi:10.1016/j.carbpol.2015.05.079
Fitzgerald, K. A., and Kagan, J. C. (2020). Toll-like receptors and the control of immunity. Cell 180, 1044–1066. doi:10.1016/j.cell.2020.02.041
Fu, Y. P., Feng, B., Zhu, Z. K., Feng, X., Chen, S. F., Li, L. X., et al. (2018). The polysaccharides from Codonopsis pilosula modulates the immunity and intestinal microbiota of cyclophosphamide-treated immunosuppressed mice. Molecules 23, 1801. doi:10.3390/molecules23071801
García, M. J., Pascual, M., Del Pozo, C., Díaz-González, A., Castro, B., Rasines, L., et al. (2020). Impact of immune-mediated diseases in inflammatory bowel disease and implications in therapeutic approach. Sci. Rep. 10 (1), 10731. doi:10.1038/s41598-020-67710-2
Gong, X. W., and Jiang, Y. (2003). The structural basis of biological function of mitogen-activated protein kinases. Chin. J. Biochem. Mol. Biol. 19 (1), 5–11.
Gong, X., Ji, M. Y., Xu, J. P., Zhang, C. H., and Li, M. H. (2019). Hypoglycemic effects of bioactive ingredients from medicine food homology and medicinal health food species used in China. Crit. Rev. Food Sci. Nutr. 60, 2303–2326. doi:10.1080/10408398.2019.1634517
Gong, X., Li, X., Xia, Y., Xu, J. F., Li, Q. Y., Zhang, C. H., et al. (2020). Effects of phytochemicals from plant-based functional foods on hyperlipidemia and their underpinning mechanisms. Trends Food Sci. Technol. 103, 304–320. doi:10.1016/j.tifs.2020.07.026
Hao, W. L., Chen, Z. B., Zhao, R., and Bo, L. (2015). Effect of Lycium barbarum polysaccharide on immune function and anti-fatigue of sub-healthy mice. Chin. J. Biologicals. 28, 693–697. doi:10.13200/j.cnki.cjb.000954
Haque, M. A., Jantan, I., Harikrishnan, H., and Abdul Wahab, S. M. (2018). Magnoflorine enhances LPS-activated pro-inflammatory responses via MyD88-dependent pathways in U937 macrophages. Planta Med. 84 (17), 1255–1264. doi:10.1055/a-0637-9936
He, Y., Hu, Z., Li, A., Zhu, Z., Yang, N., Ying, Z., et al. (2019). Recent advances in biotransformation of saponins. Molecules 24, 2365. doi:10.3390/molecules24132365
Hou, D. R., Liu, Z., Cui, S. T., and Ma, J. (2021). Tanshinone Ⅱ-A inhibited LPS-induced cell inflammation by regulating the TLR4/IκBα/NFκB signaling pathway. Chin. Pharmacol. Bull. 37 (2), 210–214. doi:10.3969/j.issn.1001-1978.2021.02.012
Huang, F., Zhang, R., Liu, Y., Xiao, J., Liu, L., Wei, Z., et al. (2016). Dietary litchi pulp polysaccharides could enhance immunomodulatory and antioxidant effects in mice. Int. J. Biol. Macromol. 92, 1067–1073. doi:10.1016/j.ijbiomac.2016.08.021
Huang, W., Li, M. L., Xia, M. Y., and Shao, J. Y. (2018). Fisetin-treatment alleviates airway inflammation through inhbition of MyD88/NF-κB signaling pathway. Int. J. Mol. Med. 42 (1), 208–218. doi:10.3892/ijmm.2018.3582
Huang, H., Luo, S. H., Huang, D. C., Cheng, S. J., Cao, C. J., and Chen, G. T. (2019). Immunomodulatory activities of proteins from Astragalus membranaceus waste. J. Sci. Food Agr. 99, 4174–4181. doi:10.1002/jsfa.9650
Huang, Q., Li, L. Y., Liu, Q. Q., and Wang, Z. (2020). Advances in immunoregulation effects of Ganoderma lucidum polysaccharide and/or Polyporus umbellatus polysaccharide. Food Sci. 41, 275–282. doi:10.7506/spkx1002-6630-20190813-149
Hwang, S. H., Shin, M. S., Yoon, T. J., and Shin, K. S. (2018). Immunoadjuvant activity in mice of polysaccharides isolated from the leaves of Panax ginseng C. Int. J. Biol. Macromolecules 107, 2695–2700. doi:10.1016/j.ijbiomac.2017.10.160
Ji, M. Y., Bo, A., Yang, M., Xu, J. F., Jiang, L. L., Zhou, B. C., et al. (2020). The pharmacological effects and health benefits of Platycodon grandiflorus-a medicine food homology species. Foods 9, 142. doi:10.3390/foods9020142
Jia, Z., Chen, A., Wang, C., He, M., Xu, J., Fu, H., et al. (2019). Amelioration effects of kaempferol on immune response following chronic intermittent cold-stress. Res. Vet. Sci. 125, 390–396. doi:10.1016/j.rvsc.2019.08.012
Jiang, Y. P., Liu, Y. F., Guo, Q. L., Xu, C. B., Zhu, C. G., and Shi, J. G. (2016). Sesquiterpene glycosides from the roots of Codonopsis pilosula. Acta Pharm. Sin. B. 6, 46–54. doi:10.1016/j.apsb.2015.09.007
Jiang, F., Guan, H., Liu, D., Wu, X., Fan, M., and Han, J. (2017). Flavonoids from sea buckthorn inhibit the lipopolysaccharide-induced inflammatory response in RAW264.7 macrophages through the MAPK and NF-κB pathways. Food Funct. 8 (3), 1313–1322. doi:10.1039/c6fo01873d
Jiang, L. L., Gong, X., Ji, M. Y., Wang, C. C., Wang, J. H., and Li, M. H. (2020). Bioactive compounds from plant-based functional foods: a promising choice for the prevention and management of hyperuricemia. Foods 9 (8), 973. doi:10.3390/foods9080973
Kamboh, A. A., Hang, S. Q., Khan, M. A., and Zhu, W. Y. (2016). In vivo immunomodulatory effects of plant flavonoids in lipopolysaccharide-challenged broilers. Animal 10, 1619–1625. doi:10.1017/S1751731116000562
Kim, J. H., Doo, E. H., Jeong, M., Kim, S., Lee, Y. Y., Yang, J., et al. (2019). Enhancing immunomodulatory function of Red Ginseng through fermentation using bifidobacterium animalis subsp. lactis LT 19-2. Nutrients 11 (7), 1481. doi:10.3390/nu11071481
Kong, N., Liu, C., Yang, F. H., Zhang, T. Y., Wang, B. K., and Gao, H. J. (2018). Effects of procyanidins on immune function in cyclophosphamide-induced immunosuppression mice. Tianjin Med. J. 46, 1291–1294. doi:10.11958/20181203
Kumar, A., Mosa, K. A., Ji, L., Kage, U., Dhokane, D., Karre, S., et al. (2018). Metabolomics-assisted biotechnological interventions for developing plant-based functional foods and nutraceuticals. Crit. Rev. Food Sci. Nutr. 58 (11), 1791–1807. doi:10.1080/10408398.2017.1285752
Li, Q., and Verma, I. M. (2002). NF-kappaB regulation in the immune system. Nat. Rev. Immunol. 2 (10), 725–734. doi:10.1038/nri910
Li, X. B., Wang, C., Chen, Y. L., Zhan, J. P., Xie, Z. L., and Zhang, Y. T. (2015). Effect on spleen immune function of Gynostemma pentaphyllum polysaccharide to immunosuppressed mice induced by cyclophosphamide. Lishizhen Med. Materia Med. Res. 26, 2308–2310. doi:10.3969/j.issn.1008-0805.2015.10.002
Li, X. B., Zhan, J. P., Zhang, Y. T., Xie, Z. L., Zhu, Y. Q., Chen, Y., et al. (2016). Effect of polysaccharide from Gastrodia elata B1 on humoral immune function in immunosuppressed mice induced by cyclophosphamide. Chin. J. Gerontol. 36 (5), 1027–1028. doi:10.3969/j.issn.1005-9202.2016.05.002
Li, L. K., Luo, Q. H., Huang, C., Chen, X. L., Chen, P., Li, Y. F., et al. (2017). Effects of soy isoflavones on expression of IL-2IL-4TNF-α and INF-γ in male rats' spleen. Acta Agri. Zhejiangensis 29 (9), 1458–1464. doi:10.3969/j.issn.1004-1524.2017.09.06
Li, J. S., Feng, H. H., Wang, M., and Yu, Y. (2019). Effect of platycodin D on lymphocyte and macrophage immune function of mice. J. Northwest A&F Univ. (Nat Sci. Ed). 47, 39–44. doi:10.13207/j.cnki.jnwafu.2019.01.005
Li, X., Yang, K., Yu, Y., Qiu, Z. Y., and Li, J. S. (2020). Effect of onion quercetin on immune organ index, phagocytic function of macrophages and secretion cytokine in chickens. Feed Res. 43 (03), 33–38. doi:10.13557/j.cnki.issn1002-2813.2020.03.009
Li, X. Q., Yang, W. W., and Li, S. Z. (2020). Research progress on the immune function of active ingredients in food. J. Shenyang Med. Coll. 22, 277–288.
Li, F. J., Li, J. S., Wang, Y. L., Jin, X., Li, X., and Yu, Y. (2021). Research on the immunoregulatory effect of jujube polysaccharide on lymphocyte in mice. Sci. Technol. Cereals. Oils Foods 29, 141–147. doi:10.16210/j.cnki.1007-7561.2021.01.019
Liao, Y., Tan, R. Z., Li, J. C., Liu, T. T., Zhong, X., Yan, Y., et al. (2020). Isoliquiritigenin attenuates UUO-induced renal inflammation and fibrosis by inhibiting mincle/Syk/NF-kappa B signaling pathway. Drug Des. Devel. Ther. 14, 1455–1468. doi:10.2147/DDDT.S243420
Lin, W. Q., Wang, W. T., Wang, D. L., and Ling, W. H. (2017). Quercetin protects against atherosclerosis by inhibiting dendritic cell activation. Mol. Nutr. Food Res. 61, 1700031. doi:10.1002/mnfr.201700031
Lin, Y. T., Chen, L. L., and Hu, X. F. (2020). A review of the types, functions and related diseases of immune cells. Biol. Teach 45, 77–80. CNKI:SUN:SWJX.0.2020-04-037
Liu, B. C., Qiu, Y., Zhao, R. D., Han, X., Yun, F. Y., Tui, X., et al. (2017a). Digital gene expression profiling of dendritic cells treated with Seabuckthorn favones. Chin. J. Microbiol. Immunol. 37, 840–848. doi:10.3760/cma.j.issn.0254-5101.2017.11.007
Liu, C. H., Liu, H., and Ge, B. (2017). Innate immunity in tuberculosis: host defense vs pathogen evasion. Cell Mol. Immunol. 14, 963–975. doi:10.1038/cmi.2017.88
Liu, Y. F., Xue, X. X., Li, Z. Y., Wang, J. P., and Zhang, Y. J. (2017c). Effect of apigenin on dendritic cells maturation and function in murine splenocytes. Acta Pharm. Sin. 52(3), 397–402. doi:10.16438/j.0513-4870.2016-0949
Liu, J., Wang, X., Yong, H., Kan, J., and Jin, C. (2018). Recent advances in flavonoid-grafted polysaccharides: synthesis, structural characterization, bioactivities and potential applications. Int. J. Biol. Macromol. 116, 1011–1025. doi:10.1016/j.ijbiomac.2018.05.149
Liu, M., Li, S. S., Wang, X. X., Zhu, Y. F., Zhang, J. J., Liu, H., et al. (2018). Characterization, anti-oxidation and anti-inflammation of polysaccharides by Hypsizygus marmoreus against LPS-induced toxicity on lung. Int. J. Biol. Macromol. 111, 121–128. doi:10.1016/j.ijbiomac.2018.01.010
Lv, X. C., Zhang, L. S., and Wang, F. J. (2016). Recent advances on immune regulation of herbal polysaccharide. Acta Univ. Trad. Med. Sinensis Pharmacologiaeque Shanghai 30, 97–101. doi:10.16306/j.1008-861x.2016.03.022
Lv, J., Gao, Y., Li, C., Yang, L. F., and Zhao, B. N. (2020). Effect of american ginseng saponins on enhancing immunity based on zebrafish model organisms. Chin. Trad. Herbal Drugs 51 (14), 3728–3733. doi:10.7501/j.issn.0253-2670.2020.14.016
Ma, H. X., and Bai, W. M. (2019). Efficacy of ginsenoside Rh2 combined with chemotherapy for lung cancer and its effect on tumor markers and immune function. Med. J. Air Force 35 (05), 406–409. doi:10.3969/j.issn.2095-3402.2019.05.012
Maatouk, M., Elgueder, D., Mustapha, N., Chaaban, H., Bzéouich, I. M., Loannou, I., et al. (2016). Effect of heated naringenin on immunomodulatory properties and cellular antioxidant activity. Cel. Stress Chaperone 21, 1101–1109. doi:10.1007/s12192-016-0734-0
Maatouk, M., Mustapha, N., Mokdad-Bzeouich, I., Chaaban, H., Abed, B., Iaonnou, I., et al. (2017). Thermal treatment of luteolin-7-O-β-glucoside improves its immunomodulatory and antioxidant potencies. Cel. Stress Chaperones 22, 775–785. doi:10.1007/s12192-017-0808-7
Manzoni, A. G., Passos, D. F., Leitemperger, J. W., Storck, T. R., Doleski, P. H., Jantsch, M. H., et al. (2020). Hyperlipidemia-induced lipotoxicity and immune activation in rats are prevented by curcumin and rutin. Int. Immunopharmacol. 81, 106217. doi:10.1016/j.intimp.2020.106217
McComb, S., Thiriot, A., Akache, B., Krishnan, L., and Stark, F. (2019). Introduction to the immune system. Metho. Mol. Biol. 2024, 1–24. doi:10.1007/978-1-4939-9597-4_110.1007/978-1-62703-589-7_1
Mehmood, A., Zhao, L., Wang, C. T., Nadeem, M., Raza, A., Ali, N., et al. (2019). Management of hyperuricemia through dietary polyphenols as a natural medicament: a comprehensive review. Crit. Rev. Food Sci. Nutr. 59 (9), 1433–1455. doi:10.1080/10408398.2017.1412939
Meng, F. C., Li, Q., Qi, Y. M., He, C. W., Wang, C. M., and Zhang, Q. W. (2018a). Characterization and immunoregulatory activity of two polysaccharides from the root of Ilex asprella. Carbohydr. Polym. 197, 9–16. doi:10.1016/j.carbpol.2018.05.066
Meng, X. Y., Chu, Z. F., Zang, J., Zhang, H., Wang, S. J., Wei, K., et al. (2018b). Immunomodulatory functions of Ginkgobiloba leaves polysaccharide on vvIBDV vaccine. Chin. J. Vet. Sci. 39, 640–645. doi:10.16303/j.cnki.1005-4545.2019.04.07
Mohamad, N. E., Romli, M. F., Alitheen, N. B., Abu, N., Yeap, S. K., Lim, K. L., et al. (2020). Apoptosis and metastasis inhibitory potential of pineapple vinegar against mouse mammary gland cells in vitro and in vivo. Nutr. Metab. (Lond) 16, 49. doi:10.1186/s12986-019-0380-5
Morsink, M. A. J., Willemen, N. G. A., Leijten, J., Bansal, R., and Shin, S. R. (2020). Immune organs and immune cells on a chip: an overview of biomedical applications. Micromachines 11, 849. doi:10.3390/mi11090849
Nafees, S., Rashid, S., Ali, N., Hasan, S. K., and Sultana, S. (2015). Rutin ameliorates cyclophosphamide induced oxidative stress and inflammation in Wistar rats: role of NFκB/MAPK pathway. Chem. Biol. Interact. 231, 98–107. doi:10.1016/j.cbi.2015.02.021
Ni, C. N., and Zhu, J. T. (2020). Clinical efficacy of honeysuckle soup combined with benzathine penicillin for syphilis patients and the effects on the serum IL-8 and TNF-α levels. Chin. J. Hum. Sex. 29 (6), 105–107. doi:10.3969/j.issn.1672-1993.2020.06.030
Ning, Y. C., Qiao, H. X., Pan, C. M., and Zhang, X. J. (2016). Effects of fermented gypenosides on immune function in mice. Hubei Agr. Sci. 55, 2304–2307. doi:10.14088/j.cnki.issn0439-8114.2016.09.038
Orlowsky, E. W., and Kraus, V. B. (2015). The role of innate immunity in osteoarthritis: when our first line of defense goes on the offensive. J. Rheumatol. 42 (3), 363–371. doi:10.3899/jrheum.140382
Pang, M. X., Fang, Y. Y., Chen, S. H., Zhu, X. X., Shan, C. W., Su, J., et al. (2017). Gypenosides inhibits xanthine oxidoreductase and ameliorates urate excretion in hyperuricemic rats induced by high cholesterol and high fat food (Lipid Emulsion). Med. Sci. Monit. 23, 1129–1140. doi:10.12659/msm.903217
Pi, J. H., Tan, J., Hu, C. T., and Xiang, D. B. (2015). Effects of Lonicera Japonica flavone on immunomodulation in mice. Chin. J. Appl. Physiol. 31, 89–92. doi:10.13459/j.cnki.cjap.2015.01.026
Qiao, J., and Fu, Y. X. (2020). Cytokines that target immune killer cells against tumors. Cel Mol. Immunol. 17 (7), 722–727. doi:10.1038/s41423-020-0481-0
Qin, F., Zhu, S. Y., Cheng, D. R., Chen, Y. Y., Zuo, W. Y., Wang, A. P., et al. (2017). Study on the hemolytic activity and immune activity of S-6 in vitro. Chin. J. Vet. Parasitol. 25 (04), 45–49. CNKI:SUN:ZSJB.0.2017-04-009
Qiu, Z. W., Gao, W., Wu, J., Gou, S. D., and Lu, M. Z. (2018). Effect of Astragalus polysaccharide injection on inflammatory cell count and levels of related factors in bronchial lavage fluid or sputum of patients with asthma. J. Prev. Med. Chin. PLA. 36, 746–749. doi:10.13704/j.cnki.jyyx.2018.06.015
Rajput, Z. I., Hu, S. H., Xiao, C. W., and Arijo, A. G. (2007). Adjuvant effects of saponins on animal immune responses. J. Zhejiang Univ. Sci. B. 8 (3), 153–161. doi:10.1631/jzus.2007.B0153
Rasouli, E., and Jahanian, R. (2015). Improved performance and immunological responses as the result of dietary genistein supplementation of broiler chicks. Animal 9, 1473–1480. doi:10.1017/S1751731115000853
Ren, L., Zhang, J., and Zhang, T. H. (2020). Immunomodulatory activities of polysaccharides from Ganoderma on immune effector cells. Food Chem. 340, 127933. doi:10.1016/j.foodchem.2020.127933
Ruan, M., Yu, B., and Zhou, F. (2021). Effect of iso-astragaloside Ⅵ on immunologic function of lymphocytes in vitro and dendritic cells. Chin. Trad. Herbal Med. 52, 196–202. doi:10.7501/j.issn.0253-2670.2021.01.023
Ruiz-Iglesias, P., Estruel-Amades, S., Camps-Bossacoma, M., Massot-Cladera, M., Franch, À., Pérez-Cano, F. J., et al. (2020). Influence of hesperidin on systemic immunity of rats following an intensive training and exhausting exercise. Nutrients 12 (5), 1291. doi:10.3390/nu12051291
Russick, J., Joubert, P. E., Gillard-Bocquet, M., Torset, C., Meylan, M., Petitprez, F., et al. (2020). Natural killer cells in the human lung tumor microenvironment display immune inhibitory functions. J. Immunother. Cancer 8, e001054. doi:10.1136/jitc-2020-001054
Shafabakhsh, R., Pourhanifeh, M. H., Mirzaei, H. R., Sahebkar, A., Asemi, Z., and Mirzaei, H. (2019). Targeting regulatory T cells by curcumin: a potential for cancer immunotherapy. Pharmacol. Res. 147, 104353. doi:10.1016/j.phrs.2019.104353
Song, D. X., and Jiang, J. G. (2017). Hypolipidemic components from medicine food homology species used in China: pharmacological and health effects. Arch. Med. Res. 48, 569–581. doi:10.1016/j.arcmed.2018.01.004
Song, C. Y., Deng, L., Hu, H. Y., and Zhang, X. M. (2008). Effects of evodiamine on immune function in mice. J. Chin. Mater. Med. 31, 885–888. doi:10.3321/j.issn:1001-4454.2008.06.030
Song, Y., Li, J., Cheng, Y., Lin, Z., He, B. Y., and Wang, C. Y. (2015). Lowering effect of evodiamine dispersible tablets on uric acid in chickens. Chin. J. New Drug. 24, 1057–1060.
Song, J. J., Fan, J. C., Wang, Y., and Zheng, X. Z. (2019). The protective effect of Chinese yam polysaccharide on spleen injury in mice induced by cyclophosphamide. Asia-pacific trad. Med 15, 15–17. doi:10.11954/ytctyy.201902003
Sun, R., Zhang, J. W., Liang, H., Wang, H. L., Zhang, S. P., and Zhi, F. (2017). Effect of ginseng saponin for serum immune factors in rats with type Ⅲ prostatitis. J. Sichuan Traditio. Chin. Med. 35 (02), 54–56. CNKI:SUN:SCZY.0.2017-02-023
Sun, B. N., Yu, S., Zhao, D. Y., Guo, S. H., Wang, X. H., and Zhao, K. (2018). Polysaccharides as vaccine adjuvants. Vaccine 36, 5226–5234. doi:10.1016/j.vaccine.2018.07.040
Sun, T. T., Gao, Y. H., Sun, Z., Lou, Y. J., and Zhou, H. Z. (2018). Research advances of Lycium barbarum polysaccharides. Chin. J. Vet. Drug 52, 75–80. doi:10.11751/ISSN.1002-1280.2018.12.12
Tang, T., and He, B. X. (2013). Treatment of D-galactose induced mouse aging with Lycium barbarum polysaccharides and its mechanism study. Afr. J. Tradit. Complet. 10, 12–17. doi:10.4314/ajtcam.v10i4.3
Tao, S., Zhao, Z., Zhang, X., Guan, X., Wei, J., Yuan, B., et al. (2020). The role of macrophages during breast cancer development and response to chemotherapy. Clin. Transl. Oncol. 22, 1938–1951. doi:10.1007/s12094-020-02348-0
Tejada, S., Pinya, S., Martorell, M., Capó, X., Tur, J. A., Pons, A., et al. (2018). Potential anti-inflammatory effects of hesperidin from the genus citrus. Curr. Med. Chem. 25 (37), 4929–4945. doi:10.2174/0929867324666170718104412
Valentová, K., Šíma, P., Rybková, Z., Křížan, J., Malachová, K., and Křen, V. (2016). Antimutagenic and immunomodulatory properties of quercetin glycosides. J. Sci. Food Agric. 96, 1492–1499. doi:10.1002/jsfa.7251
Vivier, E., Artis, D., Colonna, M., Diefenbach, A., Di Santo, J. P., Eberl, G., et al. (2018). Innate lymphoid cells: 10 years on. Cell 174, 1054–1066. doi:10.1016/j.cell.2018.07.017
Wang, X., and Lin, Z. (2019). Immunomodulating effect of Ganoderma (lingzhi) and possible mechanism. Adv. Exp. Med. Biol. 1182, 1–37. doi:10.1007/978-981-32-9421-9_1
Wang, Z. H., Lei, M. S., Peng, S., Zheng, Y., Shi, L. J., and Peng, M. J. (2016). Effects of flavonoid and flavone from eucommia ulmoides Oliv.on proliferation of splenocytes and production of IL-2 and IFN-γ in mice. Nat. Product Res. Develop. 28 (04), 514–518+489. doi:10.16333/j.1001-6880.2016.4.008
Wang, H., Chen, X. Y., Jing, J., Liu, X., Tian, C. L., and Liu, M. C. (2019). Progress on regulation effects of flavonoids on immune-related signaling pathways. Prog. Vet. Med 40 (12), 102–105. doi:10.16437/j.cnki.1007-5038.2019.12.021
Wang, J. T., Wang, H. C., and Liu, L. (2019). Progress in research on structure-function relationship of lentinan. Food Sci. 40, 363–369. doi:10.7506/spkx10026630-20181016-162
Wang, Q., Li, H. R., Li, P. Y., Xiao, J. B., and Wang, B. L. (2019b). Experimental study on effects of notoginsenoside R1 on cardiac function, lung tissue injury and immune imbalance in rats with traumatic shock. Med. J. West Chin. 21, 1826–1836. doi:10.3969/j.issn.1672-3511.2019.12.005
Wang, L., Lu, W., Ding, Y., and Qiao, F. F. (2020a). Effect of pueraria injection combined with ribavirin on immune function and inflammatory factors in children with viral myocarditis. Int. Med. Health Guid. News 26, 697–700. doi:10.3760/cma.j.issn.1007-1245.2020.05.030
Wang, M., Guo, Z., Zhou, H. Y., Ge, B. J., Wang, Z., Li, H. T., et al. (2020). Study on the bi-directional regulation of anti-inflammatory immune response by total flavonoids of Astragalus in macrophages RAW264.7. Chin. J. Prev. Vet. Med. 42 (8), 822–829. doi:10.3969/j.issn.1008-0589.201912014
Wang, Y., Fu, X., Zhang, C., and Ji, Y. T. (2020c). Regulatory effect of curcumin on expression to relevant cytokines in colonic tissue about colitis mice. J. Tianjin Univ. Trad. Chin. Med. 39 (06), 686–689. doi:10.11656/j.issn.1673-9043.2020.06.18
Wang, Y., Zhang, Q., Chen, Y., Liang, C. L., Liu, H., Qiu, F., et al. (2020d). Antitumor effects of immunity-enhancing traditional Chinese medicine. Biomed. Pharmacother. 121, 109570. doi:10.1016/j.biopha.2019.109570
Wei, X. C., Chen, T., Sun, J. J., Luo, J. Y., Ni, Y. C., Shu, G., et al. (2019). Effects of ginseng leaf polysaccharide on immune performance of yuehuang chickens during 1 to 28 Days of age. Chin. J. Anim. Nutr. 31, 2330–2339. doi:10.3969/j.issn.1006-267x.2019.05.040
Williams, A. R., Klaver, E. J., Laan, L. C., Ramsay, A., Fryganas, C., Difborg, R., et al. (2017a). Co-operative suppression of inflammatory responses in human dendritic cells by plant proanthocyanidins and products from the parasitic nematode Trichuris suis. Immunology 150, 312–328. doi:10.1111/imm.12687
Williams, A. R., Krych, L., Fauzan Ahmad, H., Nejsum, P., Skovgaard, K., Nielsen, D. S., et al. (2017b). A polyphenol-enriched diet and Ascaris suum infection modulate mucosal immune responses and gut microbiota composition in pigs. Plos. One 12 (10), e0186546. doi:10.1371/journal.pone.0186546
Xing, J., Zhang, Z. Q., Luo, K. K., Tang, X. Q., Sheng, X. Z., and Zhan, W. B. (2020). T and B lymphocytes immune responses in flounder (Paralichthys olivaceus) induced by two forms of outer membrane protein K from Vibrio anguillarum: subunit vaccine and DNA vaccine. Mol. Immunol. 118, 40–51. doi:10.1016/j.molimm.2019.12.002
Xu, X. Y., Rui, S. Z., Chen, C., Zhang, G. C., Li, Z., Wang, J. H., et al. (2019). Protective effects of astragalus polysaccharide nanoparticles on septic cardiac dysfunction through inhibition of TLR4/NF-kB signaling pathway. Int. J. Biol. Macromol. 153, 977–985. doi:10.1016/j.ijbiomac.2019.10.227
Xu, Y. Z., Pang, H. G., Li, H. Y., and Fan, J. C. (2020a). Research on anti-tumor and immunomodulatory effects of Yam polysaccharides on tumor mice. J. Med. Forum 41 (8–10), 15. CNKI:SUN:HYYX.0.2020-09-003
Xu, W., Fang, S. J., Guan, R., Zhang, C. R., and Hu, S. H. (2020b). Immunomodulatory effect of Atractylodis macrocephalae Koidz. Polysaccharides on mouse lymphocytes. Chin. J. Immunol. 36, 1573–1577. doi:10.3969/j.issn.1000-484X.2020.13.007
Xu, H., Niu, Y., Hong, W., Liu, W., Zuo, X., Bao, X., et al. (2020c). Development of a water-in-oil-in-water adjuvant for foot-and-mouth disease vaccine based on ginseng stem-leaf saponins as an immune booster. Comp. Immunol. Microbiol. Infect. Dis. 71, 101499. doi:10.1016/j.cimid.2020.101499
Xue, M., Sun, H., Cao, Y., Wang, G., Meng, Y., Wang, D., et al. (2015). Mulberry leaf polysaccharides modulate murine bone-marrow-derived dendritic cell maturation. Hum. Vaccin. Immunother. 11, 946–950. doi:10.1080/21645515.2015.1011977
Yang, J., Sha, J. D., Gao, X., Lin, S. T., Sun, T. T., Tian, C., et al. (2017). Immune regulation function of flavonoids and its mechanisms. Chin. J. Anim. Nutr. 29, 4295–4300. doi:10.3969/j.issn.1006-267x.2017.12.008
Yu, X. C., Zhang, L. S., and Wang, F. J. (2016). Recent advances on immune regulation of herbal polysaccharide. Acta Univ. Trad. Med. Sinensis Pharmacologiaeque Shanghai. 30, 97–101. doi:10.16306/j.1008-861x.2016.03.022
Zhang, K., Ge, Z. Z., Xue, Z. Q., Huang, W. J., Mei, M., Zhang, Q., et al. (2015). Chrysin suppresses human CD14(+) monocyte-derived dendritic cells and ameliorates experimental autoimmune encephalomyelitis. J. Neuroimmunol. 288, 13–20. doi:10.1016/j.jneuroim.2015.08.017
Zhang, Y., Zhang, L., Zhang, Y., Xu, J. J., Sun, L. L., and Li, S. Z. (2016). The protective role of liquiritin in high fructose-induced myocardial fibrosis via inhibiting NF-κB and MAPK signaling pathway. Biomed. Pharmacother. 84, 1337–1349. doi:10.1016/j.biopha.2016.10.036
Zhang, X. X., Yao, K. N., Ren, L. H., Chen, T., and Yao, D. G. (2016). Protective effect of Astragalus polysaccharide on endothelial progenitor cells injured by thrombin. Int. J. Biol. Macromol. 82, 711–718. doi:10.1016/j.ijbiomac.2015.09.051
Zhang, L., Wang, S., Liu, Z., Zhang, L., Wang, S., and Wang, B. (2017). Procyanidin, a kind of biological flavonoid, induces protective anti-tumor immunity and protects mice from lethal B16F10 challenge. Int. Immunopharmacol. 47, 251–258. doi:10.1016/j.intimp.2017.04.007
Zhang, B., Nan, T. G., Xin, J., Zhan, Z. L., Kang, L. P., Yuan, Y., et al. (2019). Development of a colloidal gold-based lateral flow dipstick immunoassay for rapid detection of chlorogenic acid and luteoloside in Flos Lonicerae Japonicae. J. Pharm. Biomed. Anal. 170, 83–88. doi:10.1016/j.jpba.2019.03.035
Zhang, L. H., Wu, T. T., Zhao, L. G., Xiao, W., Zhang, S. H., Wang, C. Y., et al. (2019b). Advances in studies on anticancer activity of flavonoids from ginkgo biloba extract. Chin. Phar. J. 54 (06), 444–449. doi:10.11669/cpj.2019.06.003
Zhang, L. L., Wang, Y., and Liu, L. (2020). Research progress of cytokines and inflammatory immune diseases. Pharm. Clin. Res. 28 (03), 202–205. doi:10.13664/j.cnki.pcr.2020.03.011
Zhang, Y., Zhong, X. M., Yang, J., Dong, J. L., and Tian, L. (2020b). Effect of puerarin on the expression of inflammatory cytokines in gouty arthritis mice. Immunol. J. 36 (10), 903–907+913. doi:10.13431/j.cnki.immunol.j.20200140
Zhao, R., Hao, W. L., Ma, B. L., and Chen, Z. B. (2015). Improvement effect of Lycium barbarum polysaccharide on subhealth mice. Iran J. Basic Med. Sci. 18, 1245–1252. doi:10.22038/IJBMS.2015.6281
Zhao, B., Lv, C., and Lu, J. (2019). Natural occurring polysaccharides from Panax ginseng C. A. meyer: a review of isolation, structures, and bioactivities. Int. J. Biol. Macromol. 133, 324–336. doi:10.1016/j.ijbiomac.2019.03.229
Zhao, X. J., Yang, R. L., Bi, Y., Bilal, M., Kuang, Z., Iqbal, H. M. N., et al. (2019). Effects of dietary supplementation with mulberry (Morus alba L.) leaf polysaccharides on immune parameters of weanling pigs. Animals (Basel) 10, 35. doi:10.3390/ani10010035
Zheng, Y. J., Ren, W. Y., Zhang, L. N., Zhang, Y. M., Liu, D. L., and Liu, Y. Q. (2020). A review of the pharmacological action of Astragalus polysaccharide. Front. Pharmacol. 11, 349. doi:10.3389/fphar.2020.00349
Zhou, L. J., Long, T. T., Zhou, X., and Bao, Y. X. (2017). Immunomodulatory effects of Acanthopanax Senticosus polysaccharides on lewis tumor-bearing mice through TLR4 signaling pathway. Chin. J. Immunol. 33, 849–853. doi:10.3969/j.issn.1000-484X.2017.06.009
Zhou, L. H., Yao, T., Guo, A. L., Lin, J. J., Pan, S. Q., and Chang, Y. M. (2020). Progress in the study of two-way immune regulation of traditional Chinese medicine in recent ten years. J. Basic Chin. Med. 26, 1016–1033.
Keywords: immune system, plant-based functional food, immune booster, disease treatment, bioactive compound
Citation: Jiang L, Zhang G, Li Y, Shi G and Li M (2021) Potential Application of Plant-Based Functional Foods in the Development of Immune Boosters. Front. Pharmacol. 12:637782. doi: 10.3389/fphar.2021.637782
Received: 04 December 2020; Accepted: 15 February 2021;
Published: 20 April 2021.
Edited by:
Haroon Khan, Abdul Wali Khan University Mardan, PakistanReviewed by:
Subhalakshmi Ghosh, Independent Researcher, Kolkata, IndiaHamed Mirzaei, Kashan University of Medical Sciences, Iran
Hammad Ullah, University of Naples Federico II, Italy
Copyright © 2021 Jiang, Zhang, Li, Shi and Li. This is an open-access article distributed under the terms of the Creative Commons Attribution License (CC BY). The use, distribution or reproduction in other forums is permitted, provided the original author(s) and the copyright owner(s) are credited and that the original publication in this journal is cited, in accordance with accepted academic practice. No use, distribution or reproduction is permitted which does not comply with these terms.
*Correspondence: Guirong Shi, 13327195228@163.com; Minhui Li, prof_liminhui@yeah.net
†These authors have contributed equally to this work