- Department of General Surgery, Xinqiao Hospital, Army Medical University, Chongqing, China
During acute intestinal ischemia reperfusion (IR) injury, the intestinal epithelial barrier (IEB) function is often disrupted. Enteric glial cells (EGCs) play an important role in maintaining the integrity of IEB functions. However, how EGCs regulate IEB function under IR stimulation is unknown. The present study reveals that the adenosine A2A receptor (A2AR) is important for mediating the barrier-modulating roles of EGCs. A2AR knockout (KO) experiments revealed more serious intestinal injury in A2AR KO mice than in WT mice after IR stimulation. Moreover, A2AR expression was significantly increased in WT mice when challenged by IR. To further investigate the role of A2AR in IEB, we established an in vitro EGC-Caco-2 co-culture system. Hypoxia stimulation was used to mimic the process of in vivo IR. Treating EGCs with the CGS21680 A2AR agonist attenuated hypoxia-induced intestinal epithelium damage through up-regulating ZO-1 and occludin expression in cocultured Caco-2 monolayers. Furthermore, we showed that A2AR and metabotropic glutamate receptor 5 (mGluR5) combine to activate the PKCα-dependent pathway in conditions of hypoxia. This study shows, for the first time, that hypoxia induces A2AR-mGluR5 interaction in EGCs to protect IEB function via the PKCα pathway.
Introduction
Ischemia-reperfusion (IR) injury of the intestine is a fatal syndrome in abdominal surgeries involving aortic aneurysm, small bowel or liver transplantation, cardiopulmonary bypass, strangulated hernias, and neonatal necrotizing entero colitis (Mallick et al., 2004). Acute intestinal IR injury is one of the most important causes of disruption to the intestinal epithelial barrier (IEB), initiates the systemic inflammatory response syndrome, and leads to multiple organ disorders (Vollmar and Menger, 2011; Lu et al., 2012). For these reasons, increasing attention has been focused on the underlying mechanisms of intestinal IR and promising protective strategies.
Traditionally, enteric glial cells (EGCs), the most abundant cell type in the intestinal nervous system, have been proposed to provide trophic and supportive effects for enteric neurons (Aube et al., 2006; Ruhl, 2005). However, accumulating evidence reveals that EGCs also play an important role in the regulation of intestinal epithelial proliferation and the intestinal mucosal defense system (Neunlist et al., 2013; Cheadle et al., 2013). EGCs are responsible for enhanced gut permeability and barrier dysfunction in inflammatory bowel disease (IBD) (Zhang et al., 2010; Cabarrocas et al., 2003; von Boyen and Steinkamp, 2010; Neunlist et al., 2008). In transgenic mice, the conditional deletion of EGCs results in the development of fulminant intestinal inflammation with mucosal barrier breakdown (Bush et al., 1998). Previously, we demonstrated that EGCs enhance IEB functions in response to lipopolysaccharide (LPS) stimulation by inhibiting increased iNOS activity (Xiao et al., 2011). We also found that EGC-released glial-derived neurotrophic factor (GDNF) is closely involved in the IEB protective mechanisms of EGCs in acute IR stimulation (Xiao et al., 2014). However, the precise mechanisms by which EGCs regulate IEB in IR injury have not been elucidated.
The Adenosine A2A receptor (A2AR), one of four G protein–coupled adenosine receptors (including A1R, A2AR, A2BR, and A3R), binds adenosine and induces activation of adenylate cyclase, promoting cAMP synthesis and producing corresponding biological effects (Welihinda et al., 2016). A2AR is involved in the regulation of several physiological functions, including in the gastrointestinal system (Fornai et al., 2009). A2AR has diverse and important roles in the intestine, including gut motor functions, acetylcholine release, cholinergic contraction modulation, and enteric nervous system regulation (Duarte-Araújo et al., 2004; Antonioli et al., 2006; Fornai et al., 2009; Schriemer et al., 2016). DucoSchriemer et al. revealed that A2AR is a key regulator of terminal neuronal differentiation in GDNF-treated enteric neural crest cells (ENCCs) (Schriemer et al., 2016). However, there is relatively little information about the role of A2AR in EGCs. Therefore, this study was designed to investigate the role of A2AR in EGC-mediated IEB regulation.
Materials and Methods
Cell Culture and Co-Culture
Rat EGC/PK060399egfr (CRL-2690™) and human intestinal epithelial cells Caco-2 (HTB-37™) were obtained from the American Type Culture Collection. EGC/PK060399egfr and Caco-2 cells were grown in high glucose DMEM and MEM, respectively, supplemented with 10% FCS, 2 mM l-glutamine, and 100 U/100 μg/ml penicillin–streptomycin. Cells were incubated in a 5% CO2 humidified incubator at 37°C. The EGC-Caco-2 co-culture system was established as described previously by our laboratory (Xiao et al., 2014). Caco-2 cells were seeded on Millicell®filters (0.4 μm pore diameter; Millipore; Billerica, MA) at a density of 5 × 104 cells/cm2 for up to 4–5 days. EGCs were seeded at an equal density in 6 or 24 well tissue culture plates to avoid any possible direct cell contact with Caco-2 cells. During the co-culture period, half of the culture medium in the apical and basal compartments was changed daily.
Mice
Global A2AR homozygous KO mice (A2AR−/− mice) with C57BL/6J background were provided by Dr. Yuanguo Zhou (Research Institute of Surgery, Daping Hospital, Army Medical University, Chongqing, China). Specific pathogen-free wild-type (WT) C57BL/6J mice were purchased from the Laboratory Animal Center of the Army Medical University. All mice were housed and maintained in laminar flow cabinets under specific pathogen-free conditions.
In Vitro Hypoxia Experiments
For hypoxia experiments, cells were subjected to hypoxia in a CO2 incubator (Forma® Series II Water Jacketed CO2 Incubators; Thermo Scientific) with 94% nitrogen, 5% CO2, and 1% oxygen and incubated at 37°C for 6 h. Re-oxygenation was initiated by replacing the media and exposing the cell monolayers to 37°C plus 5% CO2for 1 h. Control cells were maintained at 37°C in an atmosphere with 5% CO2.
Intestinal Ischemia/Reperfusion Model
Male mice (8–10 weeks old) were fasted for 12 h and were free to drink water prior to surgery. The animals were intraperitoneally injected with 40 mg/kg of pentobarbital anesthesia and an aseptic laparotomies dioventral line was placed. The following specific surgical procedures were performed as previously described (Xiao et al., 2014).
Western Blot Analysis
Cells and tissues were lyzed in cold RIPA buffer for 30 min and centrifuged at 13,000× g for 30 min at 4°C. Protein concentration was determined using a BCA assay reagent (Beyotime). The primary antibodies used were rabbit anti-ZO-1 (1:800), rabbit anti-Occludin (1:1000), mouse anti-A2AR (1:500), rabbit anti-PKCα (1:1000), rabbit anti-Na+/K+ ATPase (1:1000), and rabbit anti-GAPDH (1:1,000). Protein expression was measured in optical density units and normalized to GAPDH expression.
Immunofluorescence Staining
The small intestine tissues were embedded with OCT compound (Tissue-Tek, Sakura Finetek, Torrance, CA, United States). Consecutive frozen sections (5 μm in thickness) were obtained and fixed in 4% paraformaldehyde for 20 min at room temperature. After 30 min pre-incubation with a blocking solution containing 5% bovine serum albumin, sections were incubated overnight at 4°C with primary antibody against GFAP (Abcam), A2AR (Abcam), or ZO-1 (Abcam). After washing in PBS, sections were incubated with fluorescence-conjugated secondary antibodies at 37°C for 1 h. After washing in PBS, sections were incubated with DAPI nuclear stain solution for 5 min. All images were obtained using a TCS-SP5 confocal microscope (Leica, Germany).
Coimmunoprecipitation
Cells were harvested and lyzed in standard immunoprecipitation (IP) buffer containing either 1% 3-[(3-cholamidopropyl) dimethylammonio] propanesulfonic acid (Chaps) or 1% Triton X-100 (for ERAD substrates), or 2% digitonin (for ERAD machinery) for 1 h on ice. Cells were centrifuged at 16,000 × g for 10 min, and the supernatant was used for immunoprecipitation experiments. Co-IP was performed using protein A-agarose beads (EMD Millipore) with anti-A2AR (Santa Cruz Biotechnology, Inc.), anti-mGluR5 antibodies (Cell signaling), or anti-D2R antibodies (Santa Cruz Biotechnology, Inc.) following the usual method.
Transepithelial Electrical Resistance (TER) and Permeability Measurements
The TER of cells was determined via a Millipore electric resistance system (ERS-2; Millipore). Caco-2 cell monolayers were grown in Millicell inserts (0.33 cm2 area, 0.4 μm pore diameter, and 6.5 mm diameter) and the culture medium was replaced before TER measurement. To calculate the actual resistance of the cell monolayer, the mean resistance of filters without cells was subtracted from the monolayer measurement, and the difference between the filter and monolayer areas was corrected.
The intestinal mucosa TER was measured by Ussing chambers (Physiologic Instruments, San Diego, CA). The excised intestinal tissues were bathed in 5 ml Krebs buffer (110.0 mM NaCl, 3.0 mMCaCl2, 5.5 mM KCl, 1.4 mM KH2PO4, 29.0 mM NaHCO3, and 1.2 mM MgCl2, pH 7.4)on both the mucosal and serosal sides. The TER was measured as previously described (Liu et al., 2018).
Statistical Analysis
All experimental data are shown as the Mean ± SD. Statistical significance was determined by unpaired two-tailed Student t test analysis using GraphPad Prism version 7.0 software (San Diego, CA). If not otherwise stated, all experiments included three independent replications in triplicate. p < 0.05 was considered statistically significant.
Results
Acute IR Treatment Significantly Activated A2AR Expression in Intestinal Mucosa EGC
To study the effect of A2AR on EGC, we first examined the expression of A2AR in different pathological conditions. Lipopolysaccharide (LPS) and hypoxia treatments were used to stimulate EGCs in vitro. As shown in Figure 1A, A2AR expression dramatically increased in EGCs following LPS and hypoxia stimulation, with the effect of hypoxia being obvious than that of LPS. Therefore, hypoxia treatment was used to study the effect of A2AR. As demonstrated previously, GFAP is a specific marker of activated glial cells (Xiao et al., 2014). A2AR and GFAP immunofluorescent staining colocalization were used to observe changes in A2AR expression in EGCs. Acute IR-treated mice showed a moderate decrease in GFAP-positive intestinal EGCs compared to sham-treated mice (Figure 1B). However, the A2AR levels increased significantly in GFAP-positive EGCs after IR treatment. Together, these results indicate that hypoxia stimulation can activate the A2AR-mediated signaling pathway in mucosal EGCs in the intestine.
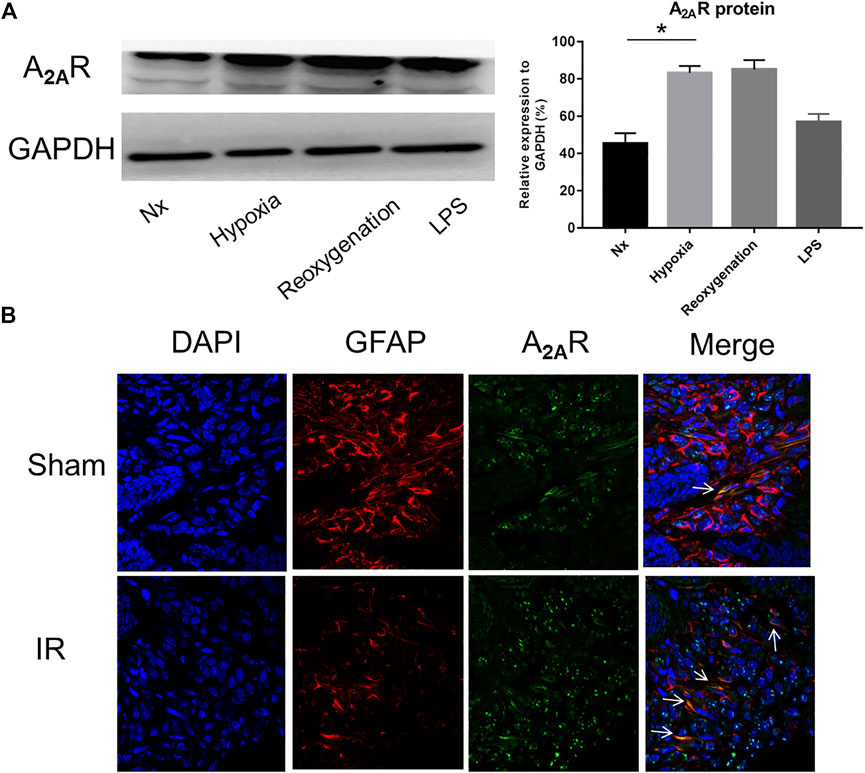
FIGURE 1. Expression of A2AR in enteric glial cells (EGCs) under hypoxia stimulation. (A) Western blot analysis shows a significant increase of A2AR protein level in cultured EGC cells after hypoxia and reoxygenation treatment. GAPDH was used as a standard for cellular protein input. (B) Immunofluorescence was used to detect A2AR expression in the small intestine under IR treatment. Red and green signals represent GFAP and A2AR, respectively. Results are expressed as mean ± SD (n = 4–6 mice/group) (*p < 0.05).
Activation of A2AR in EGCs Efficiently Prevents Barrier Dysfunction of Caco-2 Monolayers Under Hypoxia Stimulation
To further explore the role of A2AR in IEB modulation under acute hypoxia stimulation, we used an A2AR agonist and inhibitor separately in an in vitro EGC-Caco-2 co-culture system. The tight junctions (TJs) are primary determinants of IEB function (Lee, 2015). As shown in Figure 2A, western blot analysis revealed an almost 50% drop in ZO-1 and occludin expression in the hypoxia group and a similar reduction on ZO-1 and occludin expression in the A2AR inhibitor ZM241385 group. However, the A2AR agonist CGS21680 significantly prevented hypoxia-induced TJs destruction. Further study with immunocytochemistry (ICC) confirmed the western blot results (Figure 2B). Additionally, TER measurement analysis produced similar results. CGS21680 pretreatment effectively blocked TER decrease under hypoxia stimulation when compared to the ZM241385 pretreatment group (528.6 ± 18.11 Ω cm2 vs. 289.6 ± 12.63 Ω cm2 for the CGS21680 and ZM241385 pretreatment groups, respectively) (Figure 2C). Together, these results showed that A2AR plays a protective role in the EGC barrier-protecting effect on the IEC monolayer under hypoxia stimulation.
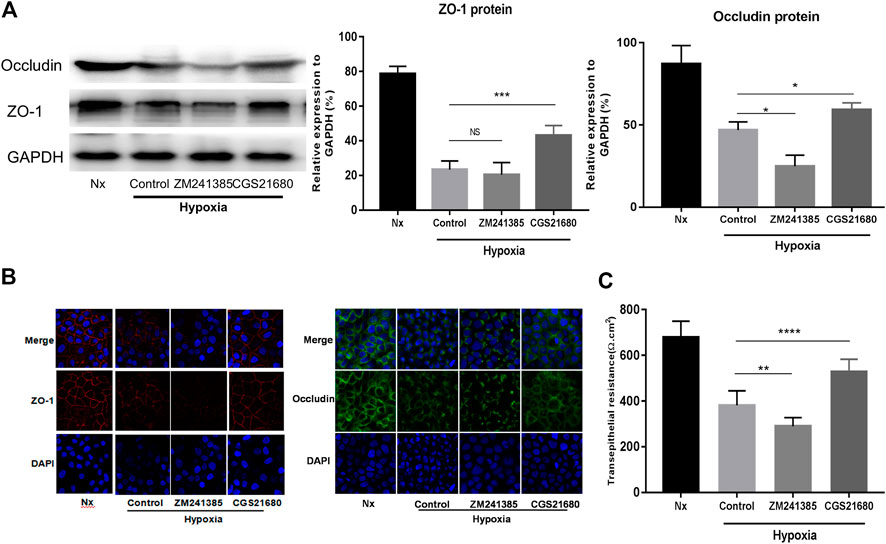
FIGURE 2. Activation of A2AR in enteric glial cells (EGCs) prevents Caco-2 monolayer barrier dysfunction under hypoxia stimulation. (A–C) EGCs were co-cultured with Caco-2 monolayers for 24 h and then treated with ZM241385 (1 μM) and CGS21680 (100 nM) for 6 h respectively under hypoxia conditions. (A) Western blot and (B) immunofluorescence analyses were used to detect ZO-1 and occludin expression. (C) The transepithelial electrical resistance (TER) of Caco-2 monolayers was determined to evaluate intestinal epithelial barrier (IEB) function. Results are expressed as mean ± SD. (**p < 0.01; ***p < 0.001; ****p < 0.0001; NS, not significant.)
A2AR Deficiency Aggravates IR-Induced IEB Injury
To further confirm the role of A2AR in the barrier protective of EGCs during hypoxia stimulation, A2AR KO mice were treated with IR. Western blot analysis of ZO-1 and occludin expression was assessed in the small intestine (Figure 3A). Acute IR treatment led to a substantial decrease in ZO-1 and occludin expression in A2AR KO mice compared to WT mice. Immunofluorescence analysis also revealed a similar reduction in ZO-1 and occludin expression in the intestinal mucosa after IR stimulation (Figure 3B). The functional impact of A2AR knockdown on tight junctions in the small intestine was further evaluated by determining the TER value using Ussing chambers. As shown in Figure 3E, intestinal I/R caused a marked TER decrease in A2AR KO mice (78.63 ± 3.407 Ω cm2) compared with WT mice (94.5 ± 3.151 Ω cm2). Histological examination of intestinal tissues revealed that IR-treated A2AR KO mice showed more increased intestinal villus fracturing and epithelial removal than did WT mice (Figures 3C,D). Together, these results strongly suggest that A2AR has a significant protective effect in IR-induced IEB injury.
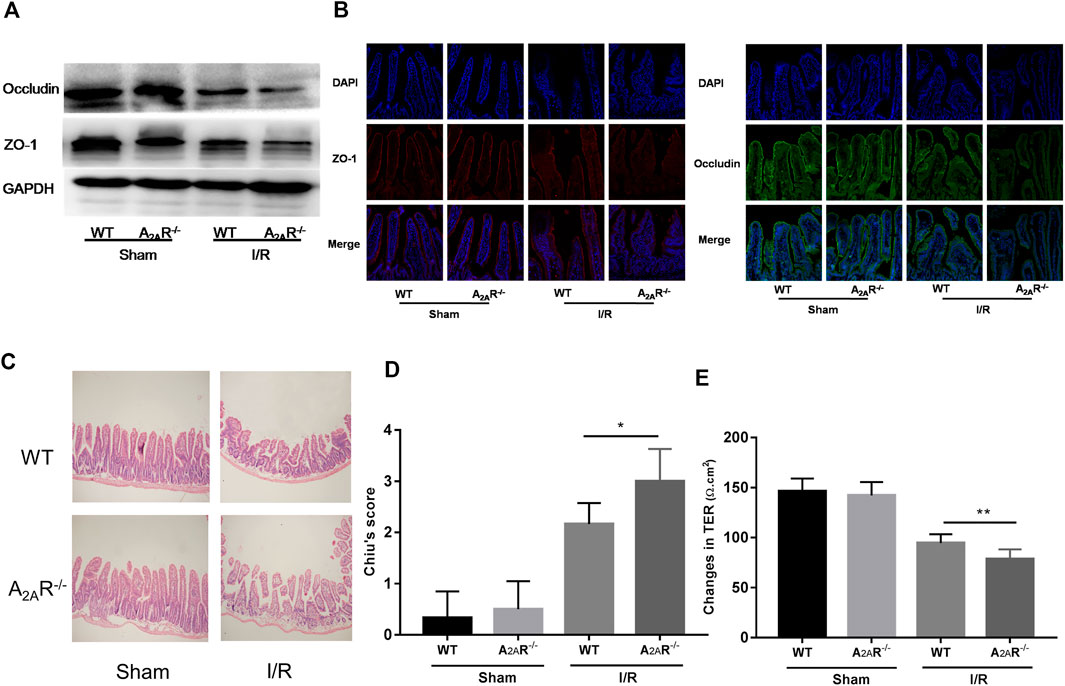
FIGURE 3. A2AR deficiency aggravates IR-induced intestinal epithelial barrier (IEB) injury. IEB function was assessed in IR-treated WT and A2AR−/− mice by (A) ZO-1 and occludin protein expression measured by western blot (B), ZO-1 and occludin protein expression measured by immunofluorescence, and (E) the small intestine TER value. The degree of intestinal damage was assessed in IR-treated WT and A2AR−/− mice by (C) hematoxylin and eosin staining, and (D) Chiu’s Score. Results are expressed as mean ± SD (n = 4–6 mice/group. *p < 0.05; **p < 0.01).
Hypoxia Induces the Interaction between A2AR and mGluR5
There are synergistic interactions between A2AR, mGluR5, and the dopamine D2 receptor (D2R) in central nervous system (CNS) related diseases (Ferraro et al., 2012; Fernández-Dueñas et al., 2013). However, whether this relationship exists in the enteric nervous system has yet to be determined. It has been suggested that in micro glial cells, A2AR combines with D2R in low glutamate concentration and combines with mGluR5 in high glutamate concentration (Dai et al., 2010; Beggiato et al., 2016). Therefore, we used high glutamate concentrations as a positive control. We studied the relationship among them under hypoxia in EGCs. When exploring the effect of oxygen concentration, the band corresponding to D2R was coimmunoprecipitated by anti-A2AR antibodies, and an A2AR band was coimmunoprecipitated by anti-D2R antibodies. Together, these results indicate that A2AR and D2R interact in normoxic but not in hypoxic conditions (Figure 4A). However, an opposite relationship was observed between A2AR and mGluR5. As shown in Figure 4B, the band corresponding to mGluR5 was coimmunoprecipitated by anti-A2AR antibodies, and an A2AR band was also coimmunoprecipitated by anti-mGluR5 antibodies. These results indicate that A2AR and D2R interact in hypoxia but not in normoxia, demonstrating that A2AR interacts with D2R under normoxic conditions and interacts with mGluR5 under hypoxic conditions.
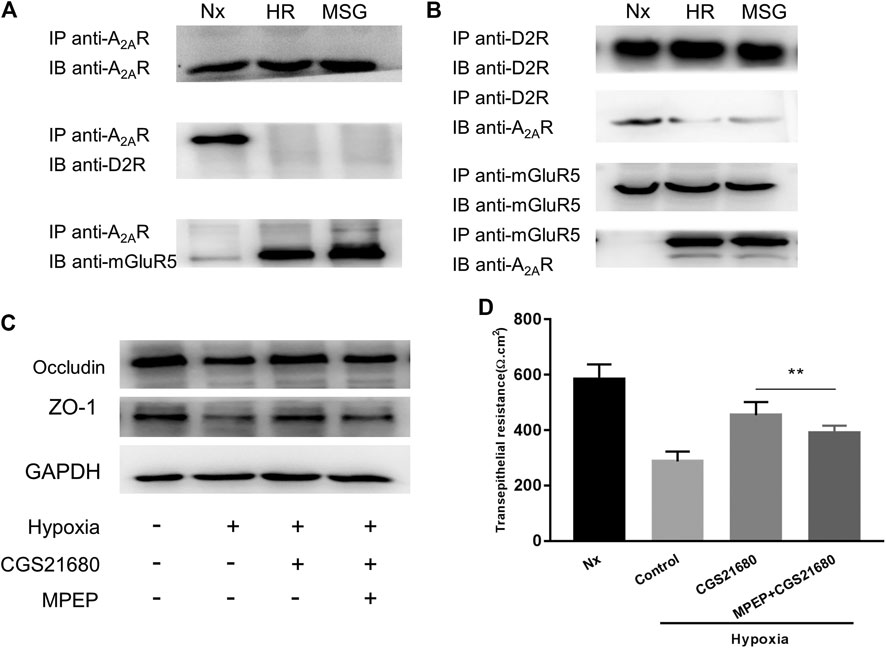
FIGURE 4. The protective effect of A2AR on the intestinal epithelial barrier (IEB) is mediated by combining with mGluR5. (A) Co-IP of A2AR and D2R or mGluR5 in enteric glial cells (EGCs) using an anti-A2AR precipitating antibody. (B) Interactions between A2AR and D2R or mGluR5 in EGCs were confirmed by Co-IP using anti- D2R or anti-mGluR5 precipitating antibodies. (C,D) EGCs were co-cultured with Caco-2 monolayers for 24 h and then treated with MPEP (100 μM) and CGS21680 (100 nM) for 6 h respectively under hypoxia conditions. (C) Western blot and (D) TER analysis were used to detect the function of IEB. Results are expressed as mean ± SD. (**p < 0.01).
mGluR5 Inhibition Attenuates the Protective Effect of A2AR on the IEB from Hypoxia Induced Damage
To confirm that A2AR regulates the IEB via a mGluR5-dependent pathway, we tested the responsiveness of the IEB to 100 μM of the MPEP selective mGluR5 antagonist under hypoxia. Western blot analysis of ZO-1 and occludin expression revealed that treatment with MPEP significantly inhibited CGS21680-mediated activation of ZO-1 and occludin expression after hypoxia induced damage (Figure 4C). Similar results were observed in TER measurements: CGS21680 pretreatment effectively prevented the decrease of TER from hypoxia stimulation, while, MPEP blocked CGS21680-induced potentiation (454.3 ± 15.7 Ω cm2 vs. 391 ± 8.441 Ω cm2) (Figure 4D). Together, these results indicate that the protective effect of A2ARon the IEB after hypoxia stimulation is dependent on mGluR5.
The PKCα Signaling Pathway Is Required for the Protective Function of A2AR on IEB
The PKCα signaling pathway is associated with an A2AR–mGluR5 interaction-associated proinflammatory effect (Dai et al., 2013; Li et al., 2017). Therefore, we next tested whether PKCα is required for A2AR-mediated IEB protection. PKC family isoforms can translocate to multiple subcellular localizations in response to hypoxia in different cell lines (Yu et al., 2015). Consistent with this, western blots showed increased PKCα expression in the membrane after hypoxia stimulation in EGCs (Figure 5A). These results were confirmed by immunocytochemistry analysis showing that PKCα translocates from the cytoplasm to the cell membrane in EGCs after hypoxia stimulation (Figure 5B). We then used PKCα inhibitor, chelerythrine chloride, to observe whether the protective effect of CGS21680 on IEB was affected. As shown in Figure 5C, chelerythrine chloride significantly reduced ZO-1 and occludin protein levels in CGS21680-pretreated EGCs. As expected, chelerythrine chloride pretreatment also blocked CGS21680-promoted TER (533 ± 24.2 Ω cm2 vs. 390.9 ± 24.14 Ω cm2) (Figure 5D). Together, these results suggest that A2AR exerts its protective effects on IEB via the PKCα signaling pathway.
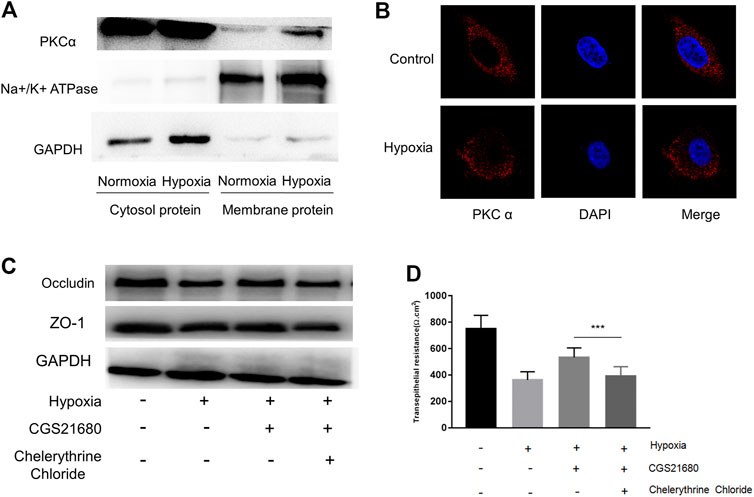
FIGURE 5. The protective effect of A2AR on IEB is PKCα dependent. PKCα expression was assessed in different enteric glial cells (EGCs) fractions. (A) Membrane and cytosol proteins were respectively extracted to detect PKCα change under hypoxia. (B) Immunofluorescence was used to visualize PKCα distribution under hypoxia. (C,D) EGCs were co-cultured with Caco-2 monolayers for 24 h, then treated with chelerythrine chloride (10 μM) and CGS21680 (100 nM) for 6 h under hypoxic conditions. (C) Western blot and (D) TER analysis were used to detect the function of IEB. Results are expressed as mean ± SD. (***p < 0.001).
Discussion
Our research has previously demonstrated that EGCs enhance IEB functions under acute intestinal injury (Xiao et al., 2014). In this context, our data provide the first evidence that EGCs protect IEB by activating A2AR. The A2AR agonist significantly improved the barrier functions of Caco-2 monolayers following exposure to HR stimulation. Moreover, in A2AR KO mice, intestinal tissue damage was accelerated, including the structural and mucosal barrier defects, following intestinal I/R. We found that A2AR combines with mGluR5 under hypoxic conditions to exert a protective effect on IEB. This data also shows that A2AR and mGluR5 combine to activate the PKCα-dependent signaling pathway. Together, these results show that A2AR plays a critical role in the barrier protective mechanism of EGCs under acute intestinal hypoxia stimulation.
EGCs are involved in the regulation of IEB function. However, the precise mechanisms by which EGCs function in the regulation of IEB remain unclear. Increasing evidence indicates that EGCs and astrocytes share morphological features and electrophysiological properties and express similar proteins, including GFAP and S100β, leading to the idea that EGCs might share many features of the central nervous system astrocytes (Le Berre-Scoul et al., 2017). The similarities between EGCs and astrocytes indicate that these two glial cell types may regulate barrier functions through common molecular mechanisms (Jiang et al., 2005; Xiao et al., 2014). It is reported that primary cell cultures of either astrocytes or enteric glia can induce barrier properties across endothelia and epithelia (Savidge et al., 2007). Jiang Set al. previously reported that implantation of enteric glia accelerates normal spinal cord vasculature repair processes at the site of injury and promotes functional blood-brain barrier (BBB) induction (Jiang et al., 2005). Additionally, glia promote blood-brain barrier-like properties in peripheral sites including blood-ocular barriers in the eye, the perineurium of peripheral nerves, and the blood myenteric plexus barrier in the gut (Gershon and Bursztajn, 1978; Savidge et al., 2007). Our previous LPS and hypoxia reperfusion stimulation studies showed that EGCs can effectively alleviate IEB damage (Xiao et al., 2011; Xiao et al., 2014). Therefore, we explored the mechanism by which EGCs protect the IEB.
A2AR activation is closely related to a variety of neurological diseases and is an important component of the adenosine signaling pathway (Stone et al., 2009). Recently, many studies have suggested that A2AR also plays an important protective role in enteritis (Warren et al., 2012; Antonioli et al., 2018). However, there is relatively little information about the role of A2AR in intestinal IR damage. A2AR inactivation can prevent IR by regulating the inflammatory response and excitotoxic cascades in the brain, kidney, lung, and blood vessels (Vincent and Okusa, 2015; Li et al., 2013; Mohamed et al., 2012; Mohamed et al., 2016; Gui et al., 2009; Cunha, 2005). Due to the similarities between the brain and the intestine, we speculate that A2AR may also have protective effects on intestinal IR damage. Our data shows that IEB damage is accelerated in A2AR KO mice. However, because there are no mice with selective inactivation of EGCs-derived A2AR, we cannot comprehensively show that EGCs protect IEB via the A2AR pathway. We exposed an in vitro EGC-Caco-2 co-culture system to hypoxia treatment to detect the role of A2AR in EGCs. Our results show that activation of A2AR in EGCs prevents damage to the IEB during hypoxia.
To clarify the mechanism by which A2AR influences IEB functions under acute intestinal epithelium hypoxia injury, we explored how A2AR works in the brain. Functional A2AR-mGluR5 heteromeric complexes have been reported in the central nervous system (Beggiato et al., 2016; Temido-Ferreira et al., 2018). Beggiato et al. found that A2AR and mGlu5R interact synergistically to modulate D2R-mediated control of striatopallidal GABA neurons (Beggiato et al., 2016). Additionally, Dai et al. reported that A2AR-mGluR5 interplay is critical for the proinflammatory effect in bone marrow-derived cells (BMDCs) after acute lung injury (Dai et al., 2013). Consistent with our expectation, we observed that A2AR combined with mGluR5 in EGCs suffering from hypoxia.
mGluR5 is a G-protein-coupled receptor that exerts its physiological roles through intracellular chemical-messenger signaling cascades (Power et al., 2016). In general, mGluR5 represents a promising target for studying neuro-protective agents of potential application in neurodegenerative diseases (Li et al., 2017). However, little data exists supporting the function of mGluR5 in the intestine, especially in relation to its role in IEB regulation. In the intestinal mucosa, mGluR5 is only observed in EGCs (Nasser et al., 2007). EGCs are involved in the occurrence of inflammatory bowel disease through c-Fos and ERK1/2 phosphorylation induced by mGluR5 (Nasser et al., 2007). In the present study, we demonstrated that mGluR5 plays a key role in the protection of IEB by A2AR. The proinflammatory effect of mGluR5 is not mediated by PKC signaling, but instead uses the PKA pathway (Dai et al., 2013). Giaroni et al. reported that the PKCα antagonist significantly inhibits intestinal mucosal injury induced by IR (Giaroni et al., 2011). These studies provide further support for our results that A2AR protects the IEB by a PKCα dependent pathway.
Taken together, our results suggest a model for A2AR in the maintenance of intestinal barrier function. Upon intestinal hypoxia injury, A2AR combines with mGluR5 to protect IEB function via the PKCα pathway in EGCs (Figure 6). Although there are complex interactions between A2AR and mGluR5 that remain to be fully understood, our findings are important for a better understanding of the role of EGCs in regulating IEB. Additionally, these findings offer new insight into the clinical use of A2AR modulators for IR-induced intestinal injury.
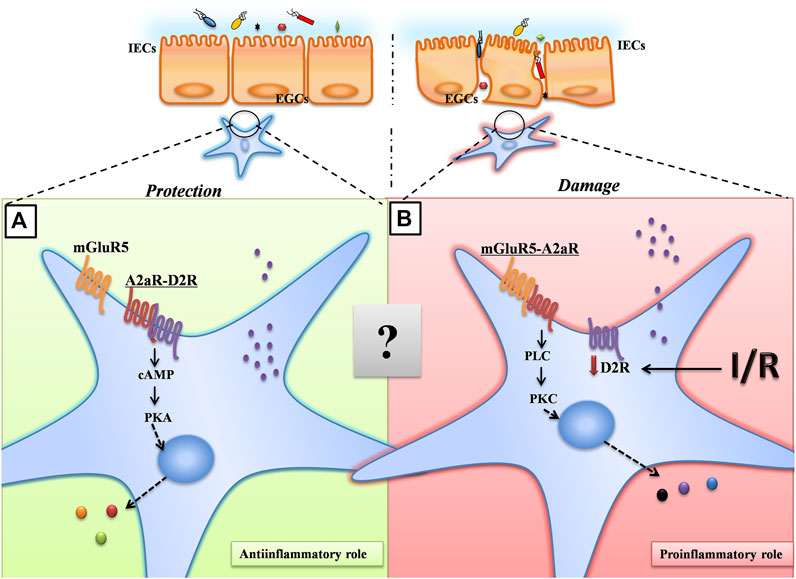
FIGURE 6. A proposed model for A2AR in the maintenance of intestinal barrier function. Under normoxic conditions, A2AR combines with D2R to maintain the normal physiological activities of EGCs through the PKA signaling pathway. Under hypoxic conditions, A2AR combines with mGluR5 to protect IEB function via the PKCα pathway in EGCs.
Data Availability Statement
The raw data supporting the conclusions of this article will be made available by the authors, without undue reservation.
Ethics Statement
The animal study was reviewed and approved by Animal Ethics Committee of the Army Medical University.
Author Contributions
LS and XL contributed equally to this work. LS and XL conceived the study and analyzed the data. HG, SC, XF, and CZ performed the research. HY and WX wrote the manuscript.
Funding
This research was supported by grants from the National Natural Science Foundation of China (NSFC 81770524 and NSFC 81470803 to WX), and the Program of Changjiang Scholars and Innovative Research (IRT 13050 to HY).
Conflict of Interest
The authors declare that the research was conducted in the absence of any commercial or financial relationships that could be construed as a potential conflict of interest.
Acknowledgments
We thank Professor Yuanguo Zhou for providing the A2AR−/− mice.
References
Antonioli, L., El-Tayeb, A., Pellegrini, C., Fornai, M., Awwad, O., Giustarini, G., et al. (2018). Anti-inflammatory effect of a novel locally acting A2A receptor agonist in a rat model of oxazolone-induced colitis. Purinergic Signal. 14 (1), 27–36. doi:10.1007/s11302-017-9591-2
Antonioli, L., Fornai, M., Colucci, R., Ghisu, N., Blandizzi, C., and Del Tacca, M. (2006). A2a receptors mediate inhibitory effects of adenosine on colonic motility in the presence of experimental colitis. Inflamm. Bowel Dis. 12 (2), 117–122. doi:10.1097/01.mib.0000198535.13822.a9
Aube, A.-C., Cabarrocas, J., Bauer, J., Philippe, D., Aubert, P., Doulay, F., et al. (2006). Changes in enteric neurone phenotype and intestinal functions in a transgenic mouse model of enteric glia disruption. Gut 55 (5), 630–637. doi:10.1136/gut.2005.067595
Beggiato, S., Tomasini, M. C., Borelli, A. C., Borroto-Escuela, D. O., Fuxe, K., Antonelli, T., et al. (2016). Functional role of striatal A2A, D2, and mGlu5 receptor interactions in regulating striatopallidal GABA neuronal transmission. J. Neurochem. 138 (2), 254–264. doi:10.1111/jnc.13652
Bush, T. G., Savidge, T. C., Freeman, T. C., Cox, H. J., Campbell, E. A., Mucke, L., et al. (1998). Fulminant jejuno-ileitis following ablation of enteric glia in adult transgenic mice. Cell 93 (2), 189–201. doi:10.1016/s0092-8674(00)81571-8
Cabarrocas, J., Savidge, T. C., and Liblau, R. S. (2003). Role of enteric glial cells in inflammatory bowel disease. Glia 41 (1), 81–93. doi:10.1002/glia.10169
Cheadle, G. A., Costantini, T. W., Lopez, N., Bansal, V., Eliceiri, B. P., and Coimbra, R. (2013). Enteric glia cells attenuate cytomix-induced intestinal epithelial barrier breakdown. PLoS One 8 (7), e69042. doi:10.1371/journal.pone.0069042
Cunha, R. A. (2005). Neuroprotection by adenosine in the brain: from A1 receptor activation to A2A receptor blockade. Purinergic Signal. 1 (2), 111–134. doi:10.1007/s11302-005-0649-1
Dai, S.-S., Wang, H., Yang, N., An, J.-H., Li, W., Ning, Y.-L., et al. (2013). Plasma glutamate-modulated interaction of A2AR and mGluR5 on BMDCs aggravates traumatic brain injury-induced acute lung injury. J. Exp. Med. 210 (4), 839–851. doi:10.1084/jem.20122196
Dai, S. S., Zhou, Y. G., Li, W., An, J. H., Li, P., Yang, N., et al. (2010). Local glutamate level dictates adenosine A2A receptor regulation of neuroinflammation and traumatic brain injury. J. Neurosci. 30 (16), 5802–5810. doi:10.1523/jneurosci.0268-10.2010
Duarte-Araújo, M., Nascimento, C., Alexandrina Timóteo, M., Magalhães-Cardoso, T., and Correia-de-Sá, P. (2004). Dual effects of adenosine on acetylcholine release from myenteric motoneurons are mediated by junctional facilitatory A2A and extrajunctional inhibitory A1 receptors. Br. J. Pharmacol. 141 (6), 925–934. doi:10.1038/sj.bjp.0705697
Fernández-Dueñas, V., Gómez-Soler, M., Morató, X., Núñez, F., Das, A., Kumar, T. S., et al. (2013). Dopamine D2 receptor-mediated modulation of adenosine A2A receptor agonist binding within the A2AR/D2R oligomer framework. Neurochem. Int. 63 (1), 42–46. doi:10.1016/j.neuint.2013.04.006
Ferraro, L., Beggiato, S., Tomasini, M. C., Fuxe, K., Antonelli, T., and Tanganelli, S. (2012). A2A/D2 receptor heteromerization in a model of Parkinson’s disease. Focus on striatal aminoacidergic signaling. Brain Res. 1476, 96–107. doi:10.1016/j.brainres.2012.01.032
Fornai, M., Antonioli, L., Colucci, R., Ghisu, N., Buccianti, P., Marioni, A., et al. (2009). A1and A2areceptors mediate inhibitory effects of adenosine on the motor activity of human colon. Neurogastroenterol. Motil. 21 (4), 451–466. doi:10.1111/j.1365-2982.2008.01213.x
Gershon, M. D., and Bursztajn, S. (1978). Properties of the enteric nervous system: limitation of access of intravascular macromolecules to the myenteric plexus and muscularis externa. J. Comp. Neurol. 180 (3), 467–487. doi:10.1002/cne.901800305
Giaroni, C., Zanetti, E., Giuliani, D., Oldrini, R., Marchet, S., Moro, E., et al. (2011). Protein kinase C modulates NMDA receptors in the myenteric plexus of the Guinea pig ileum during in vitro ischemia and reperfusion. Neurogastroenterol. Motil. 23 (2), e91–e103. doi:10.1111/j.1365-2982.2010.01644.x
Gui, L., Duan, W., Tian, H., Li, C., Zhu, J., Chen, J.-F., et al. (2009). Adenosine A2A receptor deficiency reduces striatal glutamate outflow and attenuates brain injury induced by transient focal cerebral ischemia in mice. Brain Res. 1297, 185–193. doi:10.1016/j.brainres.2009.08.050
Jiang, S., Khan, M. I., Lu, Y., Werstiuk, E. S., and Rathbone, M. P. (2005). Acceleration of blood-brain barrier formation after transplantation of enteric glia into spinal cords of rats. Exp. Brain Res. 162 (1), 56–62. doi:10.1007/s00221-004-2119-3
Le Berre-Scoul, C., Chevalier, J., Oleynikova, E., Cossais, F., Talon, S., Neunlist, M., et al. (2017). A novel enteric neuron-glia coculture system reveals the role of glia in neuronal development. J. Physiol. 595 (2), 583–598. doi:10.1113/jp271989
Lee, S. H. (2015). Intestinal permeability regulation by tight junction: implication on inflammatory bowel diseases. Intest Res. 13 (1), 11–18. doi:10.5217/ir.2015.13.1.11
Li, C., Chai, S., Ju, Y., Hou, L., Zhao, H., Ma, W., et al. (2017). Pu-erh tea protects the nervous system by inhibiting the expression of metabotropic glutamate receptor 5. Mol. Neurobiol. 54 (7), 5286–5299. doi:10.1007/s12035-016-0064-3
Li, J., Zhao, Z., He, X., Zeng, Y-J., and Dai, S.-S. (2013). Sinomenine protects against lipopolysaccharide-induced acute lung injury in mice via adenosine A(2A) receptor signaling. PLoS One 8 (3), e59257. doi:10.1371/journal.pone.0059257
Liu, Z., Li, L., Chen, W., Wang, Q., Xiao, W., Ma, Y., et al. (2018). Aryl hydrocarbon receptor activation maintained the intestinal epithelial barrier function through Notch1 dependent signaling pathway. Int. J. Mol. Med. 41 (3), 1560–1572. doi:10.3892/ijmm.2017.3341
Lu, Y.-Z., Wu, C.-C., Huang, Y.-C., Huang, C.-Y., Yang, C.-Y., Lee, T.-C., et al. (2012). Neutrophil priming by hypoxic preconditioning protects against epithelial barrier damage and enteric bacterial translocation in intestinal ischemia/reperfusion. Lab. Invest. 92 (5), 783–796. doi:10.1038/labinvest.2012.11
Mallick, I. H., Yang, W., Winslet, M. C., and Seifalian, A. M. (2004). Ischemia-reperfusion injury of the intestine and protective strategies against injury. Dig. Dis. Sci. 49 (9), 1359–1377. doi:10.1023/b:ddas.0000042232.98927.91
Mohamed, R. A., Agha, A. M., Abdel-Rahman, A. A., and Nassar, N. N. (2016). Role of adenosine A2A receptor in cerebral ischemia reperfusion injury: signaling to phosphorylated extracellular signal-regulated protein kinase (pERK1/2). Neuroscience 314, 145–159. doi:10.1016/j.neuroscience.2015.11.059
Mohamed, R. A., Agha, A. M., and Nassar, N. N. (2012). SCH58261 the selective adenosine A2A receptor blocker modulates ischemia reperfusion injury following bilateral carotid occlusion: role of inflammatory mediators. Neurochem. Res. 37 (3), 538–547. doi:10.1007/s11064-011-0640-x
Nasser, Y., Keenan, C. M., Ma, A. C., McCafferty, D.-M., and Sharkey, K. A. (2007). Expression of a functional metabotropic glutamate receptor 5 on enteric glia is altered in states of inflammation. Glia 55 (8), 859–872. doi:10.1002/glia.20507
Neunlist, M., Van Landeghem, L., Bourreille, A., and Savidge, T. (2008). Neuro-glial crosstalk in inflammatory bowel disease. J. Intern. Med. 263 (6), 577–583. doi:10.1111/j.1365-2796.2008.01963.x
Neunlist, M., Van Landeghem, L., Mahé, M. M., Derkinderen, P., des Varannes, S. B., and Rolli-Derkinderen, M. (2013). The digestive neuronal-glial-epithelial unit: a new actor in gut health and disease. Nat. Rev. Gastroenterol. Hepatol. 10 (2), 90–100. doi:10.1038/nrgastro.2012.221
Power, E. M., English, N. A., and Empson, R. M. (2016). Are Type 1 metabotropic glutamate receptors a viable therapeutic target for the treatment of cerebellar ataxia? J. Physiol. 594 (16), 4643–4652. doi:10.1113/jp271153
Ruhl, A. (2005). Glial cells in the gut. Neurogastroenterol. Motil. 17 (6), 777–790. doi:10.1111/j.1365-2982.2005.00687.x
Savidge, T. C., Sofroniew, M. V., and Neunlist, M. (2007). Starring roles for astroglia in barrier pathologies of gut and brain. Lab. Invest. 87 (8), 731–736. doi:10.1038/labinvest.3700600
Schriemer, D., Sribudiani, Y., IJpma, A., Natarajan, D., MacKenzie, K. C., Metzger, M., et al. (2016). Regulators of gene expression in enteric neural crest cells are putative Hirschsprung disease genes. Develop. Biol. 416 (1), 255–265. doi:10.1016/j.ydbio.2016.06.004
Stone, T. W., Ceruti, S., and Abbracchio, M. P. (2009). Adenosine receptors and neurological disease: neuroprotection and neurodegeneration. Handb Exp. Pharmacol. (193), 535–587. doi:10.1007/978-3-540-89615-9_17
Temido-Ferreira, M., Ferreira, D. G., Batalha, V. L., Marques-Morgado, I., Coelho, J. E., Pereira, P., et al. (2018). Age-related shift in LTD is dependent on neuronal adenosine A2A receptors interplay with mGluR5 and NMDA receptors. Mol. Psychiatry 25 (8), 1876–1900. doi:10.1038/s41380-018-0110-9
Vincent, I. S., and Okusa, M. D. (2015). Adenosine 2A receptors in acute kidney injury. Acta Physiol. 214 (3), 303–310. doi:10.1111/apha.12508
Vollmar, B., and Menger, M. D. (2011). Intestinal ischemia/reperfusion: microcirculatory pathology and functional consequences. Langenbecks Arch. Surg. 396 (1), 13–29. doi:10.1007/s00423-010-0727-x
von Boyen, G., and Steinkamp, M. (2010). The role of enteric glia in gut inflammation. Neuron Glia Biol. 6 (4), 231–236. doi:10.1017/s1740925x11000068
Warren, C. A., Calabrese, G. M., Li, Y., Pawlowski, S.W., Figler, R. A., Rieger, J., et al. (2012). Effects of adenosine A2A receptor activation and alanyl-glutamine in Clostridium difficile toxin-induced ileitis in rabbits and cecitis in mice. BMC Infect. Dis. 12, 13. doi:10.1186/1471-2334-12-13
Welihinda, A. A., Kaur, M., Greene, K., Zhai, Y., and Amento, E. P. (2016). The adenosine metabolite inosine is a functional agonist of the adenosine A2A receptor with a unique signaling bias. Cell. Signal. 28 (6), 552–560. doi:10.1016/j.cellsig.2016.02.010
Xiao, W.-D., Chen, W., Sun, L.-H., Wang, W.-S., Zhou, S.-W., and Yang, H. (2011). The protective effect of enteric glial cells on intestinal epithelial barrier function is enhanced by inhibiting inducible nitric oxide synthase activity under lipopolysaccharide stimulation. Mol. Cell. Neurosci. 46 (2), 527–534. doi:10.1016/j.mcn.2010.12.007
Xiao, W., Wang, W., Chen, W., Sun, L., Li, X., Zhang, C., et al. (2014). GDNF is involved in the barrier-inducing effect of enteric glial cells on intestinal epithelial cells under acute ischemia reperfusion stimulation. Mol. Neurobiol. 50 (2), 274–289. doi:10.1007/s12035-014-8730-9
Yu, H., Yang, Z., Pan, S., Yang, Y., Tian, J., Wang, L., et al. (2015). Hypoxic preconditioning promotes the translocation of protein kinase C ε binding with caveolin-3 at cell membrane not mitochondrial in rat heart. Cell Cycle 14 (22), 3557–3565. doi:10.1080/15384101.2015.1084446
Keywords: A2AR, intestinal epithelial barrier, mGluR5, enteric glial cells, hypoxia
Citation: Sun L, Li X, Guan H, Chen S, Fan X, Zhou C, Yang H and Xiao W (2021) A Novel Role of A2AR in the Maintenance of Intestinal Barrier Function of Enteric Glia from Hypoxia-Induced Injury by Combining with mGluR5. Front. Pharmacol. 12:633403. doi: 10.3389/fphar.2021.633403
Received: 10 December 2020; Accepted: 25 March 2021;
Published: 10 May 2021.
Edited by:
Thomas Brzozowski, Jagiellonian University Medical College, PolandReviewed by:
Carmen Diniz, University of Porto, PortugalGeetha Samak, DVS College of Arts and Science, India
Copyright © 2021 Sun, Li, Guan, Chen, Fan, Zhou, Yang and Xiao. This is an open-access article distributed under the terms of the Creative Commons Attribution License (CC BY). The use, distribution or reproduction in other forums is permitted, provided the original author(s) and the copyright owner(s) are credited and that the original publication in this journal is cited, in accordance with accepted academic practice. No use, distribution or reproduction is permitted which does not comply with these terms.
*Correspondence: Weidong Xiao, d2VpZG9uZy54aWFvQDEyNi5jb20=; Hua Yang, aHdieWFuZ0AxMjYuY29t
†These authors have contributed equally to this work.