- 1Key Laboratory of Chinese Materia Medica (Ministry of Education), Heilongjiang University of Chinese Medicine, Harbin, China
- 2Department of Laboratory Diagnostics, The First Affiliated Hospital, Heilongjiang University of Chinese Medicine, Harbin, China
- 3Department of General Surgery, The Second Affiliated Hospital of Harbin Medical University, Harbin, China
- 4Department of Rheumatology, The First Affiliated Hospital, Heilongjiang University of Chinese Medicine, Harbin, China
- 5Department of Natural Medicinal Chemistry, College of Pharmacy, Guangdong Pharmaceutical University, Guangzhou, China
Systemic lupus erythematosus (SLE) is a multisystem autoimmune inflammatory condition that affects multiple organs and provokes extensive and severe clinical manifestations. Lupus nephritis (LN) is one of the main clinical manifestations of SLE. It refers to the deposition of immune complexes in the glomeruli, which cause kidney inflammation. Although LN seriously affects prognosis and represents a key factor of disability and death in SLE patients, its mechanism remains unclear. The NACHT, leucine-rich repeat (LRR), and pyrin (PYD) domains-containing protein 3 (NLRP3) inflammasome regulates IL-1β and IL-18 secretion and gasdermin D-mediated pyroptosis and plays a key role in innate immunity. There is increasing evidence that aberrant activation of the NLRP3 inflammasome and downstream inflammatory pathways play an important part in the pathogenesis of multiple autoimmune diseases, including LN. This review summarizes research progress on the elucidation of NLRP3 activation, regulation, and recent clinical trials and experimental studies implicating the NLRP3 inflammasome in the pathophysiology of LN. Current treatments fail to provide durable remission and provoke several sides effects, mainly due to their broad immunosuppressive effects. Therefore, the identification of a safe and effective therapeutic approach for LN is of great significance. Phytochemicals are found in many herbs, fruits, and vegetables and are secondary metabolites of plants. Evidence suggests that phytochemicals have broad biological activities and have good prospects in a variety of diseases, including LN. Therefore, this review reports on current research evaluating phytochemicals for targeting NLRP3 inflammasome pathways in LN therapy.
Introduction
Systemic lupus erythematosus (SLE) is a prototypic multisystemic autoimmune condition with unclear etiology, attributed to loss of immune tolerance towards autoantigens and production of antinuclear autoantibodies (Bentham et al., 2015). Nuclear autoantigens/antibody complexes are deposited in multiple organs, resulting in tissue damage and severe clinical manifestations, including malar rash, arthralgia, fever, renal failure, and cardiovascular diseases (Tanaka et al., 2018; Durcan et al., 2019). Lupus nephritis (LN) is a main clinical manifestation of SLE; it negatively affects the quality of life of SLE patients and their long-term prognosis (Davidson, 2016; Fanouriakis et al., 2020). Approximately 50% of SLE patients develop renal disease at some stage (Almaani et al., 2017). Hence, it is necessary to further elucidate LN-related pathogenic mechanisms to develop practical therapeutic approaches.
Inflammasome refers to multiprotein immune complexes assembled by pattern recognition receptors (PRRs) in the cytoplasm. Its activation mediates inflammatory responses to cellular damage and pathogenic microbial infections (Franchi et al., 2012; Strowig et al., 2012). As an important component of the innate immunity, inflammasomes operate as a central pathogenic mechanism in various diseases (Duncan and Canna, 2018; Yang et al., 2019; Spel and Martinon, 2020). Several studies have reported that the NACHT, leucine-rich repeat (LRR), and pyrin (PYD) domains-containing protein 3 (NLRP3) inflammasome is involved in the occurrence and development of LN (Zhao et al., 2015; Zhang et al., 2018a; Lin Q. et al., 2019). This study summarizes current knowledge on the role of the NLRP3 inflammasome in the pathogenesis of LN.
Recently, phytochemicals have attracted much attention due to their cost, efficacy, and safety (Zhang et al., 2018b). We also present a review of several phytochemicals that have been shown to interfere with NLRP3 inflammasome-related signaling pathways in the context of LN.
Overview of the NLRP3 Inflammasome
Inflammasomes mediate caspase-1 activation and induce the maturation and release of the proinflammatory cytokines IL-1 and IL-18, initiating a cascade of inflammatory responses. They also trigger caspase-1-dependent pyroptosis and induce cell death under pathologic inflammatory and stress conditions. Among different inflammasomes, the NLRP3 inflammasome has been the most extensively studied and elucidated (Hoffman et al., 2001). Different studies showed that activation of the NLRP3 inflammasome is closely related to multiple autoinflammatory diseases (Baroja-Mazo et al., 2014; Lu et al., 2017; Louvrier et al., 2020). Therefore, its role in LN pathogenesis has attracted more attention (Zhao et al., 2013a; Lech et al., 2015).
The NLRP3 inflammasome is a multiprotein oligomeric complex consisting of NLRP3, adapter apoptosis-associated speck-like (ASC) protein, and procaspase-1 (Martinon et al., 2009; Guo et al., 2015). NLRP3 inflammasome activation usually involves a priming step and an activation step (Latz et al., 2013). At priming, the engagement of PRRs, such as toll-like receptors (TLR) or cytokine receptors, activates the transcription factor NF-κB, which upregulates the expression of NLRP3 and pro-IL-1β and pro-IL-18 cytokine precursors (Mishra et al., 2013; Kelley et al., 2019).
At the activation stage, the NLRP3 inflammasome is activated through the recognition of various pathogen-associated molecular patterns (PAMPs) or damage-associated molecular patterns (DAMPs) molecules (Lamkanfi and Dixit, 2014; Swanson et al., 2019), including bacterial U1-snRNP, ATP, and dsDNA (Mariathasan et al., 2006; Shin et al., 2012; Muñoz-Planillo et al., 2013; Shin et al., 2013). These stimuli activate the NLRP3 inflammasome through several mechanisms that are not fully clarified. Current research suggests the potential involvement of K+ efflux, mitochondrial dysfunction, lysosomal rupture, or generation of reactive oxygen species (ROS) (Katsnelson et al., 2016; Yu and Lee, 2016; Han et al., 2018). In addition, NEK7 also interacts with NLRP3 and modulates the activation of NLRP3 inflammasomes (He et al., 2016; Chen et al., 2019).
Upon activation, the NLRP3 binds the stimulatory ligands and the NACHT domains promote self-mediated oligomerization of several NLRP3. NLRP3 combines with ASC through homotypic N-terminal PYD-PYD interactions. Assembled ASC recruits procaspase-1 through its C-terminal CARD-CARD interactions to form the NLRP3 inflammasome. Subsequently, the functional inflammasome initiates self-catalysis of procaspase-1 to caspase-1, followed by self-enzymatic hydrolysis of caspase-1, which generates the large p20 and small p10 subunits (Elliott and Sutterwala, 2015; Sharif et al., 2019). The p20 subunit converts pro-IL-1β and pro-IL-18 into IL-1β and IL-18, respectively, and promotes the secretion of these cytokines, which have a broad spectrum of inflammatory activities (Spalinger et al., 2017; Mende et al., 2018; Abbate et al., 2020). Simultaneously, activated caspase-1 cleaves gasdermin D (GSDMD) protein, leading to the formation of plasma membrane pores, triggering gasdermin D-mediated pyroptosis (Miao et al., 2010; He et al., 2015).
Studies have identified a noncanonical inflammasome representing another crucial mechanism of inflammasome activation (Kayagaki et al., 2011). Noncanonical inflammasomes are generally activated by Gram-negative bacteria-derived molecules, including intracellular lipopolysaccharides and toxins. These inflammasomes mediate the activation of intracellular receptors caspase-11 in mice and caspase-4 and caspase-5 in humans, which are oligomerized after binding LPS and activate pyroptosis, together with inflammatory responses (Shi et al., 2014; Kayagaki et al., 2015; Lagrange et al., 2018). In addition, there is evidence that caspase-8 participates in the activation of NLRP3 inflammasome in human monocytes through an alternative NLRP3 inflammasome pathway (Xiang et al., 2020). Under LPS stimulation, the NLRP3 inflammasome activation is triggered by the TLR4-TRIF-RIPK1-FADD-CASP8 cascade signaling pathway, which then activates caspase-1 and secretes IL-1β (Gaidt et al., 2016; Zito et al., 2020) (Figure 1).
Role of NLRP3 in LN
LN results from the interactions between genetics, epigenetics, sex hormones, environment, and other factors (Cunningham et al., 2019; Rahbar Saadat et al., 2019; Wardowska et al., 2019). The core pathogenesis of LN is a loss of self-tolerance, leading to accumulation or deposition of autoantibodies and immune complexes in the kidney, activating the complement system and causing chronic inflammation (Wada et al., 2019). Renal lesions in LN patients are characterized by glomerulonephritis, vascular injury, and tubular atrophy, which eventually may progress to renal fibrosis or even renal failure (Leatherwood et al., 2019).
Recent findings showed that NLRP3 and associated inflammatory cytokines, including IL-1β and IL-18, are elevated in the blood and nephritis biopsies from LN patients (da Cruz et al., 2020; Huang et al., 2020). Moreover, to systematically explore the mechanisms of NLRP3 on LN, different mouse models have been analyzed. In several models, NLRP3 and related components are increased in LN mice compared with controls (Honarpisheh et al., 2016; Bonomini et al., 2019). In MRL/lpr mice, a spontaneous lupus model, the expression of NLRP3, ASC, and active caspase l-p20 subunit protein was upregulated in the kidney, compared with control mice. Furthermore, the IL-1β level was upregulated in renal homogenates. Blocking upstream P2X7 receptor inhibited NLRP3 inflammasome assembly and reduced proteinuria (Zhao et al., 2013b). Intraperitoneal injection of pristane stimulates the body to produce autoantibodies, which has become a classic model for SLE (Freitas et al., 2017). In Nlrp3-R258W mutant mice, more severe renal pathological changes occurred when intraperitoneal injection with pristane and specific abrogation of Nlrp3-R258W expression in myeloid cells conferred a therapeutic benefit to lupus Nlrp3-R258W mutant (Lu et al., 2017).
Since the publication of histological classification criteria for LN, much attention has been focused on glomerulopathy in patients with LN and on the mechanisms of glomerular lesions. Subsequent revisions of previous knowledge have also been based on the pathological characteristics of glomerular damage (Azzouz et al., 2019; Dörner and Furie, 2019; Zhou et al., 2019). Endothelial cells, basement membrane, and podocytes form a glomerular filtration barrier, which plays a key role in maintaining the structure and function of the kidney (Nawata et al., 2018). Currently, research on NLRP3 in the context of LN mainly focuses on podocytes. The NLRP3 inflammasome activated in glomerular podocytes results in severe proteinuria in mouse lupus models and in patients with LN (Fu et al., 2017; Fu et al., 2019). Studies support a role for the NLRP3 inflammasome in promoting podocyte injury and proteinuria during LN. The level of activated caspase-1 in podocytes from LN NZM2328 mice with severe proteinuria is elevated, as well as in urine and tissue biopsies from patients with active LN. MCC950, an NLRP3 inhibitor, significantly inhibited caspase-1 in NZM2328 mouse podocytes by preventing NLRP3 inflammasome activation, ameliorated proteinuria, and reduced renal tissue damage. In vitro, sera from LN NZM2328 mice activated the NLRP3 inflammasome and increased the IL-1β level in podocytes by inducing ROS (Fu et al., 2017).
Evidence of RIP3 and necrotic pathway activity was found in podocytes from class IV LN patients and in the kidney of lupus-prone mice. GSK872, an inhibitor of RIP3, reduced anti-dsDNA antibody titer and the size and weight of the spleen, as well as RIP3 activation in podocytes. The upregulation of NLRP3, caspase-1 p20, and IL-1β levels induced by serum IgG from LN diseased NZM2328 mice could be inhibited by GSK872 (Guo et al., 2019).
As pointed out above, several studies have demonstrated that NLRP3 inflammasome components are involved in the pathogenesis of LN. Therefore, modulating NLRP3 inflammasome signals may represent a significant and promising target for LN management (Fu et al., 2019; Yang et al., 2020) (Table 1).
Phytochemicals Targeting the NLRP3 Inflammasome in LN
Common treatments for LN predominantly involve corticosteroids, antimalarial drugs, immunosuppressive agents, and biologics, which, although effective, are commonly associated with immunogenicity (Singh et al., 2016; Fava and Petri, 2019). Currently, available drugs do not meet the clinical demands for LN patients and are limited by suboptimal efficacy and severe side effects (Dall’Era et al., 2019; Murphy and Isenberg, 2019). There is increasing concern that drugs targeting the NLRP3 pathway may be appropriate for LN therapy (Lin T.-J. et al., 2019; Wu et al., 2020). Phytochemicals are secondary metabolites of plants with various bioactivity and are found in many herbs, fruits, and vegetables. Evidence suggests that phytochemicals have broad biological activities, including antioxidant, antiviral, and anti-inflammatory effects, and hold good prospects to treat autoimmune diseases and improve lipid metabolism among other diseases (Du et al., 2019; Guo et al., 2019; Hu et al., 2019a; McKee et al., 2020). To date, several phytochemicals have been shown to affect LN progression by inhibiting the NLRP3 inflammasome (Figure 2).
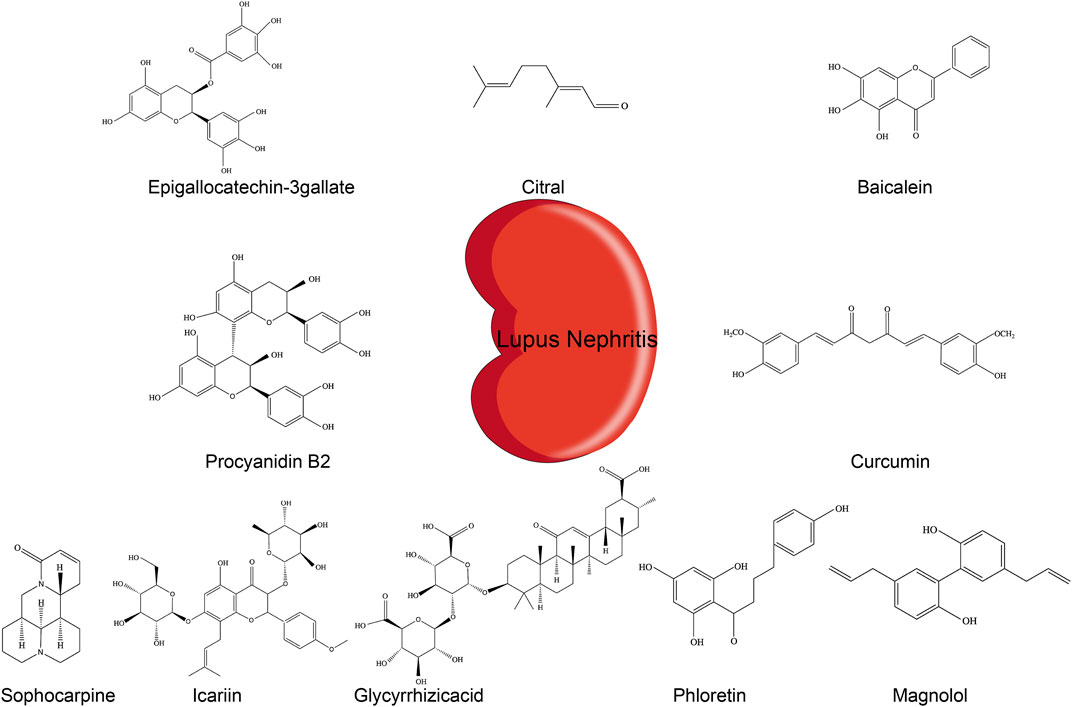
FIGURE 2. Chemical structures of different phytochemicals blocking NLRP3 inflammasome pathways in LN.
Epigallocatechin-3-Gallate (EGCG)
EGCG is a bioactive polyphenol contained in green tea. EGCG has anti-inflammatory and antioxidant activities and represents a promising drug for the treatment of inflammatory diseases (Mi et al., 2017; Byun et al., 2018). EGCG reduced the ROS level in serum and urine of lupus-prone NZB/W F1 mice. This effect occurred likely through inhibition of the production of renal NAD(P)H oxidase, mediated by the nuclear factor E2-related factor 2 (Nrf2). This pathway could be the cause of the downregulation of NLRP3 mRNA and protein and subsequent attenuation of IL-1β and IL-18 secretion, resulting in lower proteinuria and improved renal functions (Tsai et al., 2011).
Citral
Citral is a principal compound present in lemongrass and citrus fruits. It owes its therapeutic effects to its antioxidant (Li C.-C. et al., 2018) and antitumor activities Nigjeh et al., 2019). In a model of accelerated and severe LN (ASLN) in NZBxW F1 mice induced by lipopolysaccharide (LPS), citral treatment inhibited NLRP3 inflammasome signaling and IL-1β secretion by reducing ROS and COX-2. It also improved Nrf2 activation, ameliorated albuminuria secretion and renal function, and reduced the glomerulonephritis activity score and fibrinoid necrosis in the glomerulus. Consistent with these findings, citral inhibited caspase-1 activation and IL-1 secretion in ATP-induced macrophages in vitro (Ka et al., 2015).
Baicalein
Baicalein is a flavonoid compound derived from the rhizome of the plant Scutellaria baicalensis Georgi. It has a broad spectrum of activities, including anticancer (Wu R. et al., 2018) and antioxidation (Woo et al., 2005). In a model of LN induced by pristane injections in BALB/C mice, baicalein treatment downregulated ROS production and enhanced Nrf2 activation. This effect was accompanied and prevented by NLRP3 inflammasome activation. Baicalein reduced albuminuria and improved renal function. Consistent with this effect, in vitro baicalein upregulated Nrf2 signaling and inhibited the NLRP3 inflammasome in LPS-primed myeloid-derived suppressor cells (MDSCs) (Li et al., 2019).
Sophocarpine
Sophocarpine (SPC) is a natural quinolizidine alkaloid compound mainly found in the traditional Chinese herb Sophorae flavescentis. Numerous studies suggest that it exhibits various effects, including anti-inflammatory (Zou et al., 2019) and antitumoral (Zhang et al., 2016). Li et al. (2018) demonstrated that weekly gavage of MRL/lpr female mice suffering from LN with 100 mg/kg sophocarpine reduced the level of NLRP3 protein, ASC, caspase-1, and IL-1β in renal tissue, possibly through inhibition of the NF-κB activator IKKs. Treatment reduced serum and renal IL-1β, IL-6, and TNF-α. It also diminished proteinuria, reduced immune complex deposition in kidney, and significantly improved kidney function (Li et al., 2018a).
Icariin
Icariin is a flavonol glucoside extracted from the herb Epimedium. Increasing evidence suggests that icariin possesses various pharmacological properties, such as antioxidant (Wu B. et al., 2018) and anti-inflammatory properties (El-Shitany and Eid, 2019).
In lupus-prone mice, treatment with 10 mg/kg/day of icariin for eight weeks reduced serum anti-dsDNA titer, decreased renal deposition of immune complexes, improved renal function, and alleviated the pathology. Moreover, icariin decreased IL-1β and TNF-α production in MRL/lpr mice, likely by inhibiting the NF-κB signaling pathway and the activation of NLRP3 and caspase-1 in kidney (Su B. et al., 2018).
Glycyrrhizic Acid (GA)
GA is a natural extract of Glycyrrhiza uralensis. Clinical and experimental studies showed that it has many effects, including antioxidative (Umar et al., 2019) and immunoregulatory (Wu et al., 2016). In MRL/lpr mice, GA reduced serum uric acid and creatinine levels, thereby preventing severe renal injury. It exerted a protective activity by virtue of downregulating the NF-κB signaling pathway and reducing NLRP3 inflammasome activation. Assessment of proteins from the NF-κB and NLRP3 pathways by Western blot showed that GA inhibited the phosphorylation of NF-κB and IκBα and the activation of NLRP3, ASC, and caspase-1 in renal tissues and decreased serum and kidney IL-1β, IL-6, and TNF-α levels in treated MRL/lpr mice (Wang Y. Y.et al., 2017).
Phloretin
Phloretin is a natural phenolic compound extracted from fruits. Recent studies revealed numerous significant activities of phloretin, including antioxidative, antiallergic (Huang et al., 2017a), and anti-inflammatory properties (Wu et al., 2019).
In MRL/lpr mice, phloretin attenuates renal injury and inhibits immune complex deposition (Hu and Yu, 2019b). Phloretin treatment decreased NLRP3, caspase-1, IL-1β, and TNF-α protein levels in renal tissue, as well as the levels of serum IL-1β and TNF-α. To further explore the regulation of NLRP3 by phloretin, Hu and colleagues assessed the NF-κB signaling pathway in vitro. Phloretin inhibited the cytoplasmic expression of p-IκB and p65 translocation to the nucleus and prevented NLRP3 inflammasome activation through inhibition of the NF-κB pathway.
Magnolol (MG)
MG is a hydroxylated biphenyl compound extracted from Magnolia officinalis. Previous studies suggested that it has a variety of activities; it is used in treatment of melanoma (Emran et al., 2019) and anti-inflammatory (Liu et al., 2019).
In MRL/lpr mice, MG exhibited protective effects on glomerular and vascular lesions, decreased TNF-α in serum and renal tissues, and inhibited NLRP3 activation and IL-1β secretion through increased phosphorylation of IκB and level of IKK-α and NF-κB-p65 in kidney tissues (Huang et al., 2017b).
Curcumin
Curcumin is a polyphenol extensively used in clinical treatment for cancer, bowel inflammation, and osteoarthritis (Marquardt et al., 2015; Bannuru et al., 2018; Burge et al., 2019). Curcumin can decrease proteinuria in female lupus-prone NZB/W F1 mice (Lee et al., 2013) and reduce PBMCs proliferation in patients with LN (Wang M. et al., 2017). In MRL/lpr mice, curcumin treatment downregulated serum anti-dsDNA antibody level, ameliorated proteinuria, reduced renal inflammation, and decreased caspase-1 p20 and renal IL-1β. In agreement with these findings, curcumin inhibited dsDNA-induced NLRP3 inflammasome activation in podocytes in vitro by reducing NLRP3 and caspase-1 p20 expression (Zhao et al., 2019).
Procyanidin B2 (PCB2)
PCB2 is a bioactive phenolic compound isolated from apples, cocoa, and grapes. Previous research found that it holds diverse properties, such as modulation of the gut microbiota and anti-inflammatory and antioxidant effects (Su H. et al., 2018; Jiang et al., 2018; Endo et al., 2020). Recent evidence demonstrated that PCB2 directly represses the NLRP3 gene (He et al., 2018).
In MRL/lpr mice, treatment with PCB2 ameliorated LN renal lesions and decreased IL-18 and IL-1β levels in serum and renal tissues. PCB2 diminished anti-dsDNA antibody level and downregulated immune complex deposition in kidney. Moreover, He and colleagues also showed in MRL/lpr mice that silencing the NLRP3 gene reduced the production of IL-18 and IL-1β. A similar effect was found in PCB2 treated mice (He et al., 2018).
Summary
ROS constitute a class of oxygen-containing compounds involved in cell metabolism (Reczek and Chandel, 2015; Lin et al., 2019; White et al., 2019). Under exogenous stimuli, such as silica and asbestos, ROS induces thioredoxin (TXN) dissociation from the thioredoxin-interacting protein (TXNIP). TXN binds and activates the NLRP3 inflammasome (Ding et al., 2018; Li et al., 2018b). Moreover, complexes deposited in the kidney activate the complement system and induce inflammatory cells that release proinflammatory factors and produce a large amount of ROS, inflammasome, thereby activating the NLRP3 inflammatory pathway and participate in the LN pathogenesis (Zhang et al., 2018). EGCG, citral, and baicalein downregulate NLRP3 levels by decreasing ROS, thereby reducing IL-1β and IL-18 secretion.
When externally stimulated, TLR signaling activates the transcription factor nuclear factor κB (NF-κB), upregulates NLRP3 expression, and promotes the production of the proinflammatory cytokines pro-IL-1β and pro-IL-18 in LN (Yi et al., 2017; Zhang et al., 2018). Sophocarpine, icariin, GA, phloretin, and magnolol could improve renal function by inhibiting the NF-kB pathway and reducing the expression of NLRP3. In addition, curcumin and PCB2 also could inhibit the expression of NLRP3 and have the potential to treat LN, according to the evaluation of anti-dsDNA antibody level and biochemical indexes (Table 2).
Conclusion and Future Perspectives
SLE is an autoimmune disease involving multiple system damage. LN is an important renal complication of SLE, its clinical manifestations are complex and diverse, and the course of the disease is protracted and difficult to heal. If not treated in time, LN seriously affects the quality of life and survival rate of the patients. So far, the etiology and pathogenesis of LN remain unclear. As indicated above, activation of the NLRP3 inflammasome can promote the occurrence and development of the pathological processes leading to LN by causing inflammatory responses. With extensive research on inflammasomes, our understanding of its impact and mechanism of action on LN has become deeper and broader. Treatments targeting the NLRP3 inflammasome have attracted increasing attention (Li et al., 2020). Current studies on NLRP3 inflammasome in LN mainly focus on the canonical NLRP3 inflammasome pathway and lacks the detection of noncanonical NLRP3 inflammasome-related indicators, such as caspase-11, caspase-4, and caspase-5. Compared with the inactive LN subgroup and healthy controls, the serum level of caspase-8 increased significantly in active LN (Petrackova et al., 2017). It is worth further verification whether caspase-8 is involved in the pathogenesis of LN through an alternative NLRP3 inflammasome pathway. The research on the noncanonical NLRP3 inflammasome and alternative NLRP3 inflammasome pathway is still in infancy, but whether they are involved in LN and what role they play in it deserve our attention and exploration.
At present, treatment options for LN are limited. The main treatments consist of corticosteroids, antimalarials, and immunosuppressants, but these induce adverse reactions, such as immunosuppression and increased infection susceptibility. Therefore, it is extremely valuable to develop more effective treatments involving drugs with safety. This article summarizes the research progress made in recent years related to the therapeutic effects of phytochemicals on LN, acting through the NLRP3 inflammasome (Figure 2). The research of phytochemicals on NLRP3 in LN is limited to NLRP3 itself and the inflammatory factors IL-1β and pro-IL-18. In the context of lupus, there may be more specific targets upstream or downstream of NRLP3. This could help identify the role of phytochemicals on these specific molecules in other types of pathology and be tested in the context of lupus. A better understanding of the activation mechanisms of the NLRP3 inflammasome in LN will provide new ideas and approaches for the treatment of LN by phytochemicals. In addition, the clinical treatment of LN requires long-term medication, and the safety evaluation of the therapeutic dose and duration of phytochemicals needs to be further verified. This is a promising area, but there are many gaps that we need to fill in. There are significant differences in the incidence and severity of LN between different regions and races globally, and women are higher than men (Almaani et al., 2017). As summarized in this article, most of the studies on NLRP3 inflammasome in LN reported so far have been conducted in mouse models, and studies on LN patients with different genetic backgrounds will further determine the role of NLRP3 inflammasome.
Author Contributions
DW collected the literature and wrote the manuscript. LA translated and revised the article. YS and BY offered opinions for the tables and figures. SC checked the chemical structures. HK and QW organized thoughts and revised the review. All authors read and approved the final version of the manuscript.
Conflict of Interest
The authors declare that the research was conducted in the absence of any commercial or financial relationships that could be construed as a potential conflict of interest.
Publisher’s Note
All claims expressed in this article are solely those of the authors and do not necessarily represent those of their affiliated organizations, or those of the publisher, the editors and the reviewers. Any product that may be evaluated in this article, or claim that may be made by its manufacturer, is not guaranteed or endorsed by the publisher.
References
Abbate, A., Toldo, S., Marchetti, C., Kron, J., Van Tassell, B. W., and Dinarello, C. A. (2020). Interleukin-1 and the Inflammasome as Therapeutic Targets in Cardiovascular Disease. Circ. Res. 126, 1260–1280. doi:10.1161/CIRCRESAHA.120.315937
Almaani, S., Meara, A., and Rovin, B. H. (2017). Update on Lupus Nephritis. Clin. J. Am. Soc. Nephrol. 12, 825–835. doi:10.2215/CJN.05780616
Azzouz, D., Omarbekova, A., Heguy, A., Schwudke, D., Gisch, N., Rovin, B. H., et al. (2019). Lupus Nephritis Is Linked to Disease-Activity Associated Expansions and Immunity to a Gut Commensal. Ann. Rheum. Dis. 78, 947–956. doi:10.1136/annrheumdis-2018-214856
Bannuru, R. R., Osani, M. C., Al-Eid, F., and Wang, C. (2018). Efficacy of Curcumin and Boswellia for Knee Osteoarthritis: Systematic Review and Meta-Analysis. Semin. Arthritis Rheum. 48, 416–429. doi:10.1016/j.semarthrit.2018.03.001
Baroja-Mazo, A., Martín-Sánchez, F., Gomez, A. I., Martínez, C. M., Amores-Iniesta, J., Compan, V., et al. (2014). The NLRP3 Inflammasome Is Released as a Particulate Danger Signal that Amplifies the Inflammatory Response. Nat. Immunol. 15, 738–748. doi:10.1038/ni.2919
Bentham, J., Morris, D. L., Cunninghame Graham, D. S., Pinder, C. L., Tombleson, P., Behrens, T. W., et al. (2015). Genetic Association Analyses Implicate Aberrant Regulation of Innate and Adaptive Immunity Genes in the Pathogenesis of Systemic Lupus Erythematosus. Nat. Genet. 47, 1457–1464. doi:10.1038/ng.3434
Bonomini, F., Dos Santos, M., Veronese, F. V., and Rezzani, R. (2019). NLRP3 Inflammasome Modulation by Melatonin Supplementation in Chronic Pristane-Induced Lupus Nephritis. Int. J. Mol. Sci. 20, 3466. doi:10.3390/ijms20143466
Burge, K., Gunasekaran, A., Eckert, J., and Chaaban, H. (2019). Curcumin and Intestinal Inflammatory Diseases: Molecular Mechanisms of protection. Int. J. Mol. Sci. 20, 1912. doi:10.3390/ijms20081912
Byun, E.-B., Kim, W. S., Sung, N.-Y., and Byun, E.-H. (2018). Epigallocatechin-3-gallate Regulates Anti-inflammatory Action through 67-kDa Laminin Receptor-Mediated Tollip Signaling Induction in Lipopolysaccharide-Stimulated Human Intestinal Epithelial Cells. Cell. Physiol. Biochem. 46, 2072–2081. doi:10.1159/000489447
Chen, X., Liu, G., Yuan, Y., Wu, G., Wang, S., and Yuan, L. (2019). NEK7 Interacts with NLRP3 to Modulate the Pyroptosis in Inflammatory Bowel Disease via NF-Κb Signaling. Cell Death Dis 10, 906. doi:10.1038/s41419-019-2157-1
Cunningham, M. A., Richard, M. L., Wirth, J. R., Scott, J. L., Eudaly, J., Ruiz, P., et al. (2019). Novel Mechanism for Estrogen Receptor Alpha Modulation of Murine Lupus. J. Autoimmun. 97, 59–69. doi:10.1016/j.jaut.2018.10.011
da Cruz, H. L. A., Cavalcanti, C. A. J., de Azêvedo Silva, J., de Lima, C. A. D., Fragoso, T. S., Barbosa, A. D., et al. (2020). Differential Expression of the Inflammasome Complex Genes in Systemic Lupus Erythematosus. Immunogenetics 72, 217–224. doi:10.1007/s00251-020-01158-6
Dall'Era, M., Bruce, I. N., Gordon, C., Manzi, S., McCaffrey, J., and Lipsky, P. E. (2019). Current Challenges in the Development of New Treatments for Lupus. Ann. Rheum. Dis. 78, 729–735. doi:10.1136/annrheumdis-2018-214530
Davidson, A. (2016). What Is Damaging the Kidney in Lupus Nephritis? Nat. Rev. Rheumatol. 12, 143–153. doi:10.1038/nrrheum.2015.159
Ding, T., Wang, S., Zhang, X., Zai, W., Fan, J., Chen, W., et al. (2018). Kidney protection Effects of Dihydroquercetin on Diabetic Nephropathy through Suppressing ROS and NLRP3 Inflammasome. Phytomedicine 41, 45–53. doi:10.1016/j.phymed.2018.01.026
Dörner, T., and Furie, R. (2019). Novel Paradigms in Systemic Lupus Erythematosus. The Lancet 393, 2344–2358. doi:10.1016/S0140-6736(19)30546-X
Du, H., Zhang, X., Zeng, Y., Huang, X., Chen, H., Wang, S., et al. (2019). A Novel Phytochemical, DIM, Inhibits Proliferation, Migration, Invasion and TNF-α Induced Inflammatory Cytokine Production of Synovial Fibroblasts from Rheumatoid Arthritis Patients by Targeting MAPK and AKT/mTOR Signal Pathway. Front. Immunol. 10, 1620. doi:10.3389/fimmu.2019.01620
Duncan, J. A., and Canna, S. W. (2018). The NLRC4 Inflammasome. Immunol. Rev. 281, 115–123. doi:10.1111/imr.12607
Durcan, L., O'Dwyer, T., and Petri, M. (2019). Management Strategies and Future Directions for Systemic Lupus Erythematosus in Adults. The Lancet 393, 2332–2343. doi:10.1016/S0140-6736(19)30237-5
El-Shitany, N. A., and Eid, B. G. (2019). Icariin Modulates Carrageenan-Induced Acute Inflammation through HO-1/Nrf2 and NF-kB Signaling Pathways. Biomed. Pharmacother. 120, 109567. doi:10.1016/j.biopha.2019.109567
Elliott, E. I., and Sutterwala, F. S. (2015). Initiation and Perpetuation of NLRP3 Inflammasome Activation and Assembly. Immunol. Rev. 265, 35–52. doi:10.1111/imr.12286
Emran, A. A., Chinna Chowdary, B. R., Ahmed, F., Hammerlindl, H., Huefner, A., Haass, N. K., et al. (2019). Magnolol Induces Cell Death through PI3K/Akt‐mediated Epigenetic Modifications Boosting Treatment of BRAF‐ and NRAS ‐mutant Melanoma. Cancer Med. 8, 1186–1196. doi:10.1002/cam4.1978
Endo, K., Matsui, R., Sugiyama, M., Asami, T., Inaba, C., Kobayashi, S., et al. (2020). Procyanidin B2 Gallate Regulates TNF-α Production from T Cells through Inhibiting Glycolytic Activity via mTOR-HIF-1 Pathway. Biochem. Pharmacol. 177, 113952. doi:10.1016/j.bcp.2020.113952
Fanouriakis, A., Kostopoulou, M., Cheema, K., Anders, H.-J., Aringer, M., Bajema, I., et al. (2020). 2019 Update of the Joint European League against Rheumatism and European Renal Association-European Dialysis and Transplant Association (EULAR/ERA-EDTA) Recommendations for the Management of Lupus Nephritis. Ann. Rheum. Dis. 79, 713–723. doi:10.1136/annrheumdis-2020-216924
Fava, A., and Petri, M. (2019). Systemic Lupus Erythematosus: Diagnosis and Clinical Management. J. Autoimmun. 96, 1–13. doi:10.1016/j.jaut.2018.11.001
Franchi, L., Muñoz-Planillo, R., and Núñez, G. (2012). Sensing and Reacting to Microbes through the Inflammasomes. Nat. Immunol. 13, 325–332. doi:10.1038/ni.2231
Freitas, E. C., de Oliveira, M. S., and Monticielo, O. A. (2017). Pristane-induced Lupus: Considerations on This Experimental Model. Clin. Rheumatol. 36, 2403–2414. doi:10.1007/s10067-017-3811-6
Fu, R., Guo, C., Wang, S., Huang, Y., Jin, O., Hu, H., et al. (2017). Podocyte Activation of NLRP3 Inflammasomes Contributes to the Development of Proteinuria in Lupus Nephritis. Arthritis Rheumatol. 69, 1636–1646. doi:10.1002/art.40155
Fu, R., Xia, Y., Li, M., Mao, R., Guo, C., Zhou, M., et al. (2019). Pim‐1 as a Therapeutic Target in Lupus Nephritis. Arthritis Rheumatol. 71, 1308–1318. doi:10.1002/art.40863
Gaidt, M. M., Ebert, T. S., Chauhan, D., Schmidt, T., Schmid-Burgk, J. L., Rapino, F., et al. (2016). Human Monocytes Engage an Alternative Inflammasome PathwayPathogenesis of Lupus Nephritis: RIP3 Dependent Necroptosis and NLRP3 Inflammasome Activation. Immunityj. Autoimmun. 44, 833–846. doi:10.1016/j.immuni.2016.01.012
Guo, H., Callaway, J. B., and Ting, J. P.-Y. (2015). Inflammasomes: Mechanism of Action, Role in Disease, and Therapeutics. Nat. Med. 21, 677–687. doi:10.1038/nm.3893
Guo, X.-X., Zeng, Z., Qian, Y.-Z., Qiu, J., Wang, K., Wang, Y., et al. (2019). Wheat Flour, Enriched with γ-Oryzanol, Phytosterol, and Ferulic Acid, Alleviates Lipid and Glucose Metabolism in High-Fat-Fructose-Fed Rats. Nutrients 11, 1697. doi:10.3390/nu11071697
Han, Y., Xu, X., Tang, C., Gao, P., Chen, X., Xiong, X., et al. (2018). Reactive Oxygen Species Promote Tubular Injury in Diabetic Nephropathy: The Role of the Mitochondrial Ros-Txnip-Nlrp3 Biological axis. Redox Biol. 16, 32–46. doi:10.1016/j.redox.2018.02.013
He, J., Sun, M., and Tian, S. (2018). Procyanidin B2 Prevents Lupus Nephritis Development in Mice by Inhibiting NLRP3 Inflammasome Activation. Innate Immun. 24, 307–315. doi:10.1177/1753425918780985
He, W.-t., Wan, H., Hu, L., Chen, P., Wang, X., Huang, Z., et al. (2015). Gasdermin D Is an Executor of Pyroptosis and Required for Interleukin-1β Secretion. Cell Res 25, 1285–1298. doi:10.1038/cr.2015.139
He, Y., Zeng, M. Y., Yang, D., Motro, B., and Núñez, G. (2016). NEK7 Is an Essential Mediator of NLRP3 Activation Downstream of Potassium Efflux. Nature 530, 354–357. doi:10.1038/nature16959
Hoffman, H. M., Mueller, J. L., Broide, D. H., Wanderer, A. A., and Kolodner, R. D. (2001). Mutation of a New Gene Encoding a Putative Pyrin-like Protein Causes Familial Cold Autoinflammatory Syndrome and Muckle-Wells Syndrome. Nat. Genet. 29, 301–305. doi:10.1038/ng756
Honarpisheh, M., Desai, J., Marschner, J. A., Weidenbusch, M., Lech, M., Vielhauer, V., et al. (2016). Regulated Necrosis-Related Molecule mRNA Expression in Humans and Mice and in Murine Acute Tissue Injury and Systemic Autoimmunity Leading to Progressive Organ Damage, and Progressive Fibrosis. Biosci. Rep. 36. doi:10.1042/BSR20160336
Hu, W. H., Chan, G. K., Duan, R., Wang, H. Y., Kong, X. P., Dong, T. T., et al. (2019a). Synergy of Ginkgetin and Resveratrol in Suppressing VEGF-Induced Angiogenesis: A Therapy in Treating Colorectal Cancer. Cancers 11, 1828. doi:10.3390/cancers11121828
Hu, Y. Y., and Yu, P. C. (2019b). Protective Effect of Phloretin on Lupus Nephritis in MRL/lpr Mice via NF-Κb. Zhong Guo Mian Yi Xue Za Zhi 35. doi:10.3969/j.issn.1000-484X.2019.06.016
Huang, F., Zhang, R.-Y., and Song, L. (2017b). Beneficial Effect of Magnolol on Lupus Nephritis in MRL/lpr Mice by Attenuating the NLRP3 Inflammasome and NF-Κb Signaling Pathway: A Mechanistic Analysis. Mol. Med. Rep. 16, 4817–4822. doi:10.3892/mmr.2017.7154
Huang, T., Yin, H., Ning, W., Wang, X., Chen, C., Lin, W., et al. (2020). Expression of Inflammasomes NLRP1, NLRP3 and AIM2 in Different Pathologic Classification of Lupus Nephritis. Clin. Exp. Rheumatol. 38, 680–690.
Huang, W.-C., Fang, L.-W., and Liou, C.-J. (2017a). Phloretin Attenuates Allergic Airway Inflammation and Oxidative Stress in Asthmatic Mice. Front. Immunol. 8, 134. doi:10.3389/fimmu.2017.00134
Jiang, Y., Yang, W., and Gui, S. (2018). Procyanidin B2 Protects Rats from Paraquat-Induced Acute Lung Injury by Inhibiting NLRP3 Inflammasome Activation. Immunobiology 223, 555–561. doi:10.1016/j.imbio.2018.07.001
Ka, S.-M., Lin, J.-C., Lin, T.-J., Liu, F.-C., Chao, L. K., Ho, C.-L., et al. (2015). Citral Alleviates an Accelerated and Severe Lupus Nephritis Model by Inhibiting the Activation Signal of NLRP3 Inflammasome and Enhancing Nrf2 Activation. Arthritis Res. Ther. 17, 331. doi:10.1186/s13075-015-0844-6
Katsnelson, M. A., Lozada-Soto, K. M., Russo, H. M., Miller, B. A., and Dubyak, G. R. (2016). NLRP3 Inflammasome Signaling Is Activated by Low-Level Lysosome Disruption but Inhibited by Extensive Lysosome Disruption: Roles for K+ Efflux and Ca2+ Influx. Am. J. Physiology-Cell Physiol. 311, C83–C100. doi:10.1152/ajpcell.00298.2015
Kayagaki, N., Stowe, I. B., Lee, B. L., O’Rourke, K., Anderson, K., Warming, S., et al. (2015). Caspase-11 Cleaves Gasdermin D for Non-canonical Inflammasome Signalling. Nature 526, 666–671. doi:10.1038/nature15541
Kayagaki, N., Warming, S., Lamkanfi, M., Walle, L. V., Louie, S., Dong, J., et al. (2011). Non-canonical Inflammasome Activation Targets Caspase-11. Nature 479, 117–121. doi:10.1038/nature10558
Kelley, N., Jeltema, D., Duan, Y., and He, Y. (2019). The NLRP3 Inflammasome: An Overview of Mechanisms of Activation and Regulation. Int. J. Mol. Sci. 20, 3328. doi:10.3390/ijms20133328
Lagrange, B., Benaoudia, S., Wallet, P., Magnotti, F., Provost, A., Michal, F., et al. (2018). Human Caspase-4 Detects Tetra-Acylated LPS and Cytosolic Francisella and Functions Differently from Murine Caspase-11. Nat. Commun. 9, 242. doi:10.1038/s41467-017-02682-y
Lamkanfi, M., and Dixit, V. M. (2014). Mechanisms and Functions of Inflammasomes. Cell 157, 1013–1022. doi:10.1016/j.cell.2014.04.007
Latz, E., Xiao, T. S., and Stutz, A. (2013). Activation and Regulation of the Inflammasomes. Nat. Rev. Immunol. 13, 397–411. doi:10.1038/nri3452
Leatherwood, C., Speyer, C. B., Feldman, C. H., D'Silva, K., Gómez-Puerta, J. A., Hoover, P. J., et al. (2019). Clinical Characteristics and Renal Prognosis Associated with Interstitial Fibrosis and Tubular Atrophy (IFTA) and Vascular Injury in Lupus Nephritis Biopsies. Semin. Arthritis Rheum. 49, 396–404. doi:10.1016/j.semarthrit.2019.06.002
Lech, M., Lorenz, G., Kulkarni, O. P., Grosser, M. O. O., Stigrot, N., Darisipudi, M. N., et al. (2015). NLRP3 and ASC Suppress Lupus-like Autoimmunity by Driving the Immunosuppressive Effects of TGF-β Receptor Signalling. Ann. Rheum. Dis. 74, 2224–2235. doi:10.1136/annrheumdis-2014-205496
Lee, H., Kim, H., Lee, G., Chung, H.-S., and Bae, H. (2013). Curcumin Attenuates Lupus Nephritis upon Interaction with Regulatory T Cells in New Zealand Black/White Mice. Br. J. Nutr. 110, 69–76. doi:10.1017/S0007114512004734
Li, C.-C., Yu, H.-F., Chang, C.-H., Liu, Y.-T., and Yao, H.-T. (2018). Effects of Lemongrass Oil and Citral on Hepatic Drug-Metabolizing Enzymes, Oxidative Stress, and Acetaminophen Toxicity in Rats. J. Food Drug Anal. 26, 432–438. doi:10.1016/j.jfda.2017.01.008
Li, D., Shi, G., Wang, J., Zhang, D., Pan, Y., Dou, H., et al. (2019). Baicalein Ameliorates Pristane-Induced Lupus Nephritis via Activating Nrf2/HO-1 in Myeloid-Derived Suppressor Cells. Arthritis Res. Ther. 21, 105. doi:10.1186/s13075-019-1876-0
Li, X., Wang, M., Hong, H., Luo, C., Liu, Z., and Yang, R. (2018a). Sophocarpine Attenuates Murine Lupus Nephritis via Inhibiting NLRP3 Inflammasome and NF-Κb Activation. Immunol. Res. 66, 521–527. doi:10.1007/s12026-018-9012-9
Li, X., Zhang, X., Pan, Y., Shi, G., Ren, J., Fan, H., et al. (2018b). mTOR Regulates NLRP3 Inflammasome Activation via Reactive Oxygen Species in Murine Lupus. Acta Biochim. Biophys. Sin. 50, 888–896. doi:10.1093/abbs/gmy088
Li, Z., Guo, J., and Bi, L. (2020). Role of the NLRP3 Inflammasome in Autoimmune Diseases. Biomed. Pharmacother. 130, 110542. doi:10.1016/j.biopha.2020.1105429
Lin, Q., Li, S., Jiang, N., Shao, X., Zhang, M., Jin, H., et al. (2019). PINK1-parkin Pathway of Mitophagy Protects against Contrast-Induced Acute Kidney Injury via Decreasing Mitochondrial ROS and NLRP3 Inflammasome Activation. Redox Biol. 26, 101254. doi:10.1016/j.redox.2019.101254
Lin, T.-J., Wu, C.-Y., Tsai, P.-Y., Hsu, W.-H., Hua, K.-F., Chu, C.-L., et al. (2019). Accelerated and Severe Lupus Nephritis Benefits from M1, an Active Metabolite of Ginsenoside, by Regulating NLRP3 Inflammasome and T Cell Functions in Mice. Front. Immunol. 10, 1951. doi:10.3389/fimmu.2019.01951
Liu, X., Wang, Y., Wu, D., Li, S., Wang, C., Han, Z., et al. (2019). Magnolol Prevents Acute Alcoholic Liver Damage by Activating PI3K/Nrf2/PPARγ and Inhibiting NLRP3 Signaling Pathway. Front. Pharmacol. 10, 1459. doi:10.3389/fphar.2019.01459
Louvrier, C., Assrawi, E., El Khouri, E., Melki, I., Copin, B., Bourrat, E., et al. (2020). NLRP3-associated Autoinflammatory Diseases: Phenotypic and Molecular Characteristics of Germline versus Somatic Mutations. J. Allergy Clin. Immunol. 145, 1254–1261. doi:10.1016/j.jaci.2019.11.035
Lu, A., Li, H., Niu, J., Wu, S., Xue, G., Yao, X., et al. (2017). Hyperactivation of the NLRP3 Inflammasome in Myeloid Cells Leads to Severe Organ Damage in Experimental Lupus. J. Immunol. 198, 1119–1129. doi:10.4049/jimmunol.1600659
Mariathasan, S., Weiss, D. S., Newton, K., McBride, J., O'Rourke, K., Roose-Girma, M., et al. (2006). Cryopyrin Activates the Inflammasome in Response to Toxins and ATP. Nature 440, 228–232. doi:10.1038/nature04515
Marquardt, J. U., Gomez-Quiroz, L., Arreguin Camacho, L. O., Pinna, F., Lee, Y.-H., Kitade, M., et al. (2015). Curcumin Effectively Inhibits Oncogenic NF-Κb Signaling and Restrains Stemness Features in Liver Cancer. J. Hepatol. 63, 661–669. doi:10.1016/j.jhep.2015.04.018
Martinon, F., Mayor, A., and Tschopp, J. (2009). The Inflammasomes: Guardians of the Body. Annu. Rev. Immunol. 27, 229–265. doi:10.1146/annurev.immunol.021908.132715
McKee, D. L., Sternberg, A., Stange, U., Laufer, S., and Naujokat, C. (2020). Candidate Drugs against SARS-CoV-2 and COVID-19. Pharmacol. Res. 157, 104859. doi:10.1016/j.phrs.2020.104859
Mende, R., Vincent, F. B., Kandane-Rathnayake, R., Koelmeyer, R., Lin, E., Chang, J., et al. (2018). Analysis of Serum Interleukin (IL)-1β and IL-18 in Systemic Lupus Erythematosus. Front. Immunol. 9, 1250. doi:10.3389/fimmu.2018.01250
Mi, Y., Qi, G., Gao, Y., Li, R., Wang, Y., Li, X., et al. (2017). (‐)‐Epigallocatechin‐3‐gallate Ameliorates Insulin Resistance and Mitochondrial Dysfunction in HepG2 Cells: Involvement of Bmal1. Mol. Nutr. Food Res. 61, 1700440. doi:10.1002/mnfr.201700440
Miao, E. A., Leaf, I. A., Treuting, P. M., Mao, D. P., Dors, M., Sarkar, A., et al. (2010). Caspase-1-induced Pyroptosis Is an Innate Immune Effector Mechanism against Intracellular Bacteria. Nat. Immunol. 11, 1136–1142. doi:10.1038/ni.1960
Mishra, B. B., Rathinam, V. A. K., Martens, G. W., Martinot, A. J., Kornfeld, H., Fitzgerald, K. A., et al. (2013). Nitric Oxide Controls the Immunopathology of Tuberculosis by Inhibiting NLRP3 Inflammasome-dependent Processing of IL-1β. Nat. Immunol. 14, 52–60. doi:10.1038/ni.2474
Muñoz-Planillo, R., Kuffa, P., Martínez-Colón, G., Smith, B. L., Rajendiran, T. M., and Núñez, G. (2013). K+ Efflux Is the Common Trigger of NLRP3 Inflammasome Activation by Bacterial Toxins and Particulate Matter. Immunity 38, 1142–1153. doi:10.1016/j.immuni.2013.05.016
Murphy, G., and Isenberg, D. A. (2019). New Therapies for Systemic Lupus Erythematosus - Past Imperfect, Future Tense. Nat. Rev. Rheumatol. 15, 403–412. doi:10.1038/s41584-019-0235-5
Nawata, A., Hisano, S., Shimajiri, S., Wang, K.-Y., Tanaka, Y., and Nakayama, T. (2018). Podocyte and Endothelial Cell Injury lead to Nephrotic Syndrome in Proliferative Lupus Nephritis. Histopathology 72, 1084–1092. doi:10.1111/his.13454
Nigjeh, S. E., Yeap, S. K., Nordin, N., Rahman, H., and Rosli, R. (2019). In Vivo anti-tumor Effects of Citral on 4T1 Breast Cancer Cells via Induction of Apoptosis and Downregulation of Aldehyde Dehydrogenase Activity. Molecules 24, 3241. doi:10.3390/molecules24183241
Petrackova, A., Smrzova, A., Gajdos, P., Schubertova, M., Schneiderova, P., Kromer, P., et al. (2017). Serum Protein Pattern Associated with Organ Damage and Lupus Nephritis in Systemic Lupus Erythematosus Revealed by PEA Immunoassay. Clin. Proteomics 14, 32. doi:10.1086/s12014-017-9167-810.1186/s12014-017-9167-8
Rahbar Saadat, Y., Hejazian, M., Bastami, M., Hosseinian Khatibi, S. M., Ardalan, M., and Zununi Vahed, S. (2019). The Role of Microbiota in the Pathogenesis of Lupus: Dose it Impact Lupus Nephritis? Pharmacol. Res. 139, 191–198. doi:10.1016/j.phrs.2018.11.023
Reczek, C. R., and Chandel, N. S. (2015). ROS-dependent Signal Transduction. Curr. Opin. Cel Biol. 33, 8–13. doi:10.1016/j.ceb.2014.09.010
Sharif, H., Wang, L., Wang, W. L., Magupalli, V. G., Andreeva, L., Qiao, Q., et al. (2019). Structural Mechanism for NEK7-Licensed Activation of NLRP3 Inflammasome. Nature 570, 338–343. doi:10.1038/s41586-019-1295-z
Shi, J., Zhao, Y., Wang, Y., Gao, W., Ding, J., Li, P., et al. (2014). Inflammatory Caspases Are Innate Immune Receptors for Intracellular LPS. Nature 514, 187–192. doi:10.1038/nature13683
Shin, M. S., Kang, Y., Lee, N., Kim, S. H., Kang, K. S., Lazova, R., et al. (2012). U1-small Nuclear Ribonucleoprotein Activates the NLRP3 Inflammasome in Human Monocytes. J. Immunol. 188, 4769–4775. doi:10.4049/jimmunol.1103355
Shin, M. S., Kang, Y., Lee, N., Wahl, E. R., Kim, S. H., Kang, K. S., et al. (2013). Self Double-Stranded (Ds)DNA Induces IL-1β Production from Human Monocytes by Activating NLRP3 Inflammasome in the Presence of Anti-dsDNA Antibodies. J. Immunol. 190, 1407–1415. doi:10.4049/jimmunol.1201195
Singh, J. A., Hossain, A., Kotb, A., and Wells, G. (2016). Risk of Serious Infections with Immunosuppressive Drugs and Glucocorticoids for Lupus Nephritis: A Systematic Review and Network Meta-Analysis. BMC Med. 14, 137. doi:10.1186/s12916-016-0673-8
Spalinger, M. R., Lang, S., Gottier, C., Dai, X., Rawlings, D. J., Chan, A. C., et al. (2017). PTPN22 Regulates NLRP3-Mediated IL1B Secretion in an Autophagy-dependent Manner. Autophagy 13, 1590–1601. doi:10.1080/15548627.2017.1341453
Spel, L., and Martinon, F. (2020). Inflammasomes Contributing to Inflammation in Arthritis. Immunol. Rev. 294, 48–62. doi:10.1111/imr.12839
Strowig, T., Henao-Mejia, J., Elinav, E., and Flavell, R. (2012). Inflammasomes in Health and Disease. Nature 481, 278–286. doi:10.1038/nature10759
Su, B., Ye, H., You, X., Ni, H., Chen, X., and Li, L. (2018). Icariin Alleviates Murine Lupus Nephritis via Inhibiting NF-Κb Activation Pathway and NLRP3 Inflammasome. Life Sci. 208, 26–32. doi:10.1016/j.lfs.2018.07.009
Su, H., Li, Y., Hu, D., Xie, L., Ke, H., Zheng, X., et al. (2018). Procyanidin B2 Ameliorates Free Fatty Acids-Induced Hepatic Steatosis through Regulating TFEB-Mediated Lysosomal Pathway and Redox State. Free Radic. Biol. Med. 126, 269–286. doi:10.1016/j.freeradbiomed.2018.08.024
Swanson, K. V., Deng, M., and Ting, J. P.-Y. (2019). The NLRP3 Inflammasome: Molecular Activation and Regulation to Therapeutics. Nat. Rev. Immunol. 19, 477–489. doi:10.1038/s41577-019-0165-0
Tanaka, Y., Mizukami, A., Kobayashi, A., Ito, C., and Matsuki, T. (2018). Disease Severity and Economic burden in Japanese Patients with Systemic Lupus Erythematosus: A Retrospective, Observational Study. Int. J. Rheum. Dis. 21, 1609–1618. doi:10.1111/1756-185X.13363
Tsai, P.-Y., Ka, S.-M., Chang, J.-M., Chen, H.-C., Shui, H.-A., Li, C.-Y., et al. (2011). Epigallocatechin-3-gallate Prevents Lupus Nephritis Development in Mice via Enhancing the Nrf2 Antioxidant Pathway and Inhibiting NLRP3 Inflammasome Activation. Free Radic. Biol. Med. 51, 744–754. doi:10.1016/j.freeradbiomed.2011.05.016
Umar, S. A., Tanveer, M. A., Nazir, L. A., Divya, G., Vishwakarma, R. A., and Tasduq, S. A. (2019). Glycyrrhizic Acid Prevents Oxidative Stress Mediated DNA Damage Response through Modulation of Autophagy in Ultraviolet-B-Irradiated Human Primary Dermal Fibroblasts. Cel. Physiol. Biochem. 53, 242–257. doi:10.33594/000000133
Wada, Y., Gonzalez-Sanchez, H. M., Weinmann-Menke, J., Iwata, Y., Ajay, A. K., Meineck, M., et al. (2019). IL-34-Dependent Intrarenal and Systemic Mechanisms Promote Lupus Nephritis in MRL-Faslpr Mice. J. Am. Soc. Nephrol. 30, 244–259. doi:10.1681/ASN.2018090901
Wang, M., Zhou, G., Lv, J., Zeng, P., Guo, C., and Wang, Q. (2017). Curcumin Modulation of the Activation of PYK2 in Peripheral Blood Mononuclear Cells from Patients with Lupus Nephritis. Reumatalogia. 55, 269–275. doi:10.5114/reum.2017.72623
Wang, Y. Y., Li, H. T., Lu, Y., Jia, X. Y., Li, Y. L., Chen, S., et al. (2017). [Protective Effects of Glycyrrhizic Acid against Lupus Nephritis in MRL/lpr Mice]. Nan Fang Yi Ke Da Xue Xue Bao 37, 957–961. doi:10.3969/j.issn.1673-4254.2017.07.19
Wardowska, A., Komorniczak, M., Bułło-Piontecka, B., Dȩbska-Ślizień, M. A., and Pikuła, M. (2019). Transcriptomic and Epigenetic Alterations in Dendritic Cells Correspond with Chronic Kidney Disease in Lupus Nephritis. Front. Immunol. 10, 2026. doi:10.3389/fimmu.2019.02026
White, S. M., Avantaggiati, M. L., Nemazanyy, I., Di Poto, C., Yang, Y., Pende, M., et al. (2019). YAP/TAZ Inhibition Induces Metabolic and Signaling Rewiring Resulting in Targetable Vulnerabilities in NF2-Deficient Tumor Cells. Develop. Cel 49, 425–443. doi:10.1016/j.devcel.2019.04.014
Woo, A., Cheng, C., and Waye, M. (2005). Baicalein Protects Rat Cardiomyocytes from Hypoxia/reoxygenation Damage via a Prooxidant Mechanism. Cardiovasc. Res. 65, 244–253. doi:10.1016/j.cardiores.2004.09.027
Wu, B., Feng, J.-Y., Yu, L.-M., Wang, Y.-C., Chen, Y.-Q., Wei, Y., et al. (2018). Icariin Protects Cardiomyocytes against Ischaemia/reperfusion Injury by Attenuating Sirtuin 1-dependent Mitochondrial Oxidative Damage. Br. J. Pharmacol. 175, 4137–4153. doi:10.1111/bph.14457
Wu, C.-Y., Hua, K.-F., Chu, C.-L., Yang, S.-R., Arbiser, J. L., Yang, S.-S., et al. (2020). Tris DBA Ameliorates Accelerated and Severe Lupus Nephritis in Mice by Activating Regulatory T Cells and Autophagy and Inhibiting the NLRP3 Inflammasome. J. Immunol. 204, 1448–1461. doi:10.4049/jimmunol.1801610
Wu, M., Li, P., An, Y., Ren, J., Yan, D., Cui, J., et al. (2019). Phloretin Ameliorates Dextran Sulfate Sodium-Induced Ulcerative Colitis in Mice by Regulating the Gut Microbiota. Pharmacol. Res. 150, 104489. doi:10.1016/j.phrs.2019.104489
Wu, Q., Tang, Y., Hu, X., Wang, Q., Lei, W., Zhou, L., et al. (2016). Regulation of Th1/Th2 Balance through OX40/OX40L Signalling by Glycyrrhizic Acid in a Murine Model of Asthma. Respirology 21, 102–111. doi:10.1111/resp.12655
Wu, R., Murali, R., Kabe, Y., French, S. W., Chiang, Y. M., Liu, S., et al. (2018). Baicalein Targets GTPase‐Mediated Autophagy to Eliminate Liver Tumor-Initiating Stem Cell-like Cells Resistant to mTORC1 Inhibition. Hepatology 68, 1726–1740. doi:10.1002/hep.30071
Xiang, H., Zhu, F., Xu, Z., and Xiong, J. (2020). Role of Inflammasomes in Kidney Diseases via Both Canonical and Non-canonical Pathways. Front. Cel. Dev. Biol. 8, 106. doi:10.3389/fcell.2020.00106
Yang, B.-Y., Cheng, Y.-G., Liu, Y., Liu, Y., Tan, J.-Y., Guan, W., et al. (2019). Datura Metel L. Ameliorates Imiquimod-Induced Psoriasis-like Dermatitis and Inhibits Inflammatory Cytokines Production through TLR7/8-MyD88-NF-Κb-NLRP3 Inflammasome Pathway. Molecules 24, 2157. doi:10.3390/molecules24112157
Yang, S.-R., Hua, K.-F., Chu, L. J., Hwu, Y.-K., Yang, S.-M., Wu, C.-Y., et al. (2020). Xenon Blunts NF-Κb/nlrp3 Inflammasome Activation and Improves Acute Onset of Accelerated and Severe Lupus Nephritis in Mice. Kidney Int. 98, 378–390. doi:10.1016/j.kint.2020.02.033
Yi, H., Peng, R., Zhang, L.-y., Sun, Y., Peng, H.-m., Liu, H.-d., et al. (2017). LincRNA-Gm4419 Knockdown Ameliorates NF-Κb/nlrp3 Inflammasome-Mediated Inflammation in Diabetic Nephropathy. Cel Death Dis 8, e2583. doi:10.1038/cddis.2016.451
Yu, J.-W., and Lee, M.-S. (2016). Mitochondria and the NLRP3 Inflammasome: Physiological and Pathological Relevance. Arch. Pharm. Res. 39, 1503–1518. doi:10.1007/s12272-016-0827-4
Zhang, D., Xu, J., Ren, J., Ding, L., Shi, G., Li, D., et al. (2018). Myeloid-derived Suppressor Cells Induce Podocyte Injury through Increasing Reactive Oxygen Species in Lupus Nephritis. Front. Immunol. 9, 1443. doi:10.3389/fimmu.2018.01443
Zhang, H., Liu, J., Li, G., Wei, J., Chen, H., Zhang, C., et al. (2018a). Fresh Red Raspberry Phytochemicals Suppress the Growth of Hepatocellular Carcinoma Cells by PTEN/AKT Pathway. Int. J. Biochem. Cel Biol. 104, 55–65. doi:10.1016/j.biocel.2018.09.003
Zhang, H., Liu, L., and Li, L. (2018b). Lentivirus-mediated Knockdown of FcγRI (CD64) Attenuated Lupus Nephritis via Inhibition of NF-Κb Regulating NLRP3 Inflammasome Activation in MRL/lpr Mice. J. Pharmacol. Sci. 137, 342–349. doi:10.1016/j.jphs.2018.05.012
Zhang, P.-P., Wang, P.-Q., Qiao, C.-P., Zhang, Q., Zhang, J.-P., Chen, F., et al. (2016). Differentiation Therapy of Hepatocellular Carcinoma by Inhibiting the Activity of AKT/GSK-3β/β-catenin axis and TGF-β Induced EMT with Sophocarpine. Cancer Lett. 376, 95–103. doi:10.1016/j.canlet.2016.01.011
Zhao, J., Wang, H., Dai, C., Wang, H., Zhang, H., Huang, Y., et al. (2013a). P2X7Blockade Attenuates Murine Lupus Nephritis by Inhibiting Activation of the NLRP3/ASC/Caspase 1 Pathway. Arthritis Rheum. 65, 3176–3185. doi:10.1002/art.38174
Zhao, J., Wang, H., Huang, Y., Zhang, H., Wang, S., Gaskin, F., et al. (2015). Lupus Nephritis: Glycogen Synthase Kinase 3β Promotion of Renal Damage through Activation of the NLRP3 Inflammasome in Lupus-Prone Mice. Arthritis Rheumatol. 67, 1036–1044. doi:10.1002/art.38993
Zhao, J., Wang, J., Zhou, M., Li, M., Li, M., and Tan, H. (2019). Curcumin Attenuates Murine Lupus via Inhibiting NLRP3 Inflammasome. Int. Immunopharmacology 69, 213–216. doi:10.1016/j.intimp.2019.01.046
Zhao, J., Zhang, H., Huang, Y., Wang, H., Wang, S., Zhao, C., et al. (2013b). Bay11-7082 Attenuates Murine Lupus Nephritis via Inhibiting NLRP3 Inflammasome and NF-Κb Activation. Int. Immunopharmacology 17, 116–122. doi:10.1016/j.intimp.2013.05.027
Zhou, X.-J., Klionsky, D. J., and Zhang, H. (2019). Podocytes and Autophagy: A Potential Therapeutic Target in Lupus Nephritis. Autophagy 15, 908–912. doi:10.1080/15548627.2019.1580512
Zito, G., Buscetta, M., Cimino, M., Dino, P., Bucchieri, F., and Cipollina, C. (2020). Cellular Models and Assays to Study NLRP3 Inflammasome Biology. Int. J. Mol. Sci. 21, 4294. doi:10.3390/ijms21124294f
Keywords: NLRP3 inflammasome, lupus nephritis, phytochemicals, podocyte, treatment
Citation: Wu D, Ai L, Sun Y, Yang B, Chen S, Wang Q and Kuang H (2021) Role of NLRP3 Inflammasome in Lupus Nephritis and Therapeutic Targeting by Phytochemicals. Front. Pharmacol. 12:621300. doi: 10.3389/fphar.2021.621300
Received: 26 October 2020; Accepted: 14 July 2021;
Published: 20 August 2021.
Edited by:
Massimo Bertinaria, University of Turin, ItalyReviewed by:
Diego Angosto Bazarra, Instituto Murciano de investigaciones Biosanitarias (IMIB), SpainVanessa D’Antongiovanni, University of Pisa, Italy
Copyright © 2021 Wu, Ai, Sun, Yang, Chen, Wang and Kuang. This is an open-access article distributed under the terms of the Creative Commons Attribution License (CC BY). The use, distribution or reproduction in other forums is permitted, provided the original author(s) and the copyright owner(s) are credited and that the original publication in this journal is cited, in accordance with accepted academic practice. No use, distribution or reproduction is permitted which does not comply with these terms.
*Correspondence: Qiuhong Wang, cWh3YW5naGViQDE2My5jb20=; Haixue Kuang, aHhrdWFuZ0B5YWhvby5jb20=