- 1Department of Pharmacology and Toxicology, College of Pharmacy, Prince Sattam Bin Abdulaziz University, Al-Kharj, Saudi Arabia
- 2Department of Pharmacognosy, School of Pharmacy, College of Health Sciences, Mekelle University, Mekelle, Ethiopia
- 3Department of Biological Sciences, National University of Medical Sciences, Rawalpindi, Pakistan
- 4Faculty of Natural and Health Science, Al Zaytoonh University of Science and Technology, Salfeet, Palestine
- 5Central Laboratory, Department of Pharmaceutical Chemistry, College of Pharmacy, King Saud University, Riyadh, Saudi Arabia
- 6Department of Pharmacology and Toxicology, College of Pharmacy, King Saud University, Riyadh, Saudi Arabia
The genus Thymus is traditionally used for the treatment of hyperactive airways complaints. The purpose of the current study is to investigate the potential tracheal relaxant effect and possible mechanism(s) of the essential oil of Thymus serrulatus (TS Oil) in isolated guinea pig tracheal tissues. The essential oil was obtained from the fresh erial parts of Thymus serrulatus, and its phyto-components were identified by GC-MS analysis. Guinea pig tracheal preparations were used for testing the tracheal relaxant effect of TS Oil with the determination of the mechanism(s) involved in this relaxation. GC-MS findings reveal that terpenes, fragrance constituents, saponins, and higher fatty acids are present in TS Oil. In isolated guinea pig trachea, TS Oil inhibited carbachol (CCh, 1 µM) and K+ (80 mM)-induced contractions in a pattern similar to that of dicyclomine. TS Oil, at 0.3 mg/ml, shifted parallel CCh-curves towards the right, followed by a non-parallel shift at higher concentration (1 mg/ml), thus suppressing maximum response in the same manner as produced by dicyclomine. Pretreatment of tissues with TS Oil (1 and 3 mg/ml) also produced a rightward shift of Ca++ concentration-response curves (CRCs) in the same manner as caused by verapamil. Further, TS Oil at low concentrations (0.3 and 1 mg/ml) shifted isoprenaline-induced inhibitory CRCs towards the left and increased cAMP levels in isolated tracheal homogenates similar to papaverine, a phosphodiesterase (PDE) inhibitor. In the antimicrobial assay performed by the agar well diffusion method, TS Oil was found most active against Candida albicans and Staphylococcus aureus where the zone of inhibition measured was 28 mm. Additionally, there was little difference between standard strains of gram-positive and gram-negative bacteria. However, methicillin-resistant S. aureus (MRSA) showed a small zone of inhibition as compared to standard strains (22 mm). From these results, it can be concluded that the essential oil of T. serrulatus has the potential to produce antimicrobial effects while causing tracheal relaxation mediated possibly by anticholinergic effects, Ca++ channel blockade, and PDE inhibition whereas additional mechanism(s) cannot be ruled out.
Introduction
Asthma is a condition of chronic obstruction of airways, inflammation, and mucus production, which results in shortness of breath and/or cough. It usually occurs in the evening and/or in the early morning as tracheal sensitivity to many stimulators increases at these times. Various immune cells contribute to the exacerbation of asthma including mast cells, eosinophils, and T-cells (Guo et al., 2018). Reports show that some selected species of bacteria and fungi such as Klebsiella pneumoniae, Pseudomonas eruginosa, Staphylococcus aureus, and Candida albicans and methicillin-resistant S. aureus (MRSA) are also involved in causing airways diseases such as laryngitis, pharyngitis, and tracheitis (Costa et al., 2015; Gileles-Hillel et al., 2015). However, certain infections are difficult to treat. Further, the therapeutic options available to address respiratory disorders have several limitations including cost and side effects. Thus, alternative remedies, such as aromatic plants, have been used to treat these disorders and possibly have bronchodilatory and antimicrobial effects (Costa et al., 2015; Cheesman et al., 2017).
Thymus serrulatus Hochst. ex Benth (Lamiaceae), a much-branched perennial subshrub that grows in the Afromontane and Afroalpine zones of Ethiopia and Eritrea (Damtie and Mekonnen, 2015). In Ethiopia, it is found in the northern highlands of Semien Shoa, Tigray, and Wollo areas (Melka et al., 2016, Damtie et al., 2018). It is known by the local communities as Tosign in Amhara and Tesni/Thasne in Tigray region of Ethiopia (Damtie et al., 2017).
T. serrulatus is an endemic medicinal plant traditionally used for various disease conditions including flue (Meresa et al., 2017) and cough (Melka et al., 2016). In various scientific studies the plant has been found active against various bacterial and fungal strains (Damtie and Mokonen, 2016), possess diuretic and antihyperlipidemic (Melka et al., 2016; Getachew, 2018). Also, the smooth muscle relaxant effect (vasodilatory) of the plant was observed in an ex vivo study by (Geleta et al., 2015).
In different part of the world, various species of thymus (T. linearis, T. vulgaris, and T. serphyllum, etc.) are known for their traditional us in the treatment of respiratory disorders such as bronchitis, asthma, pertussis, laryngitis, tonsillitis, and cough caused by common colds (Begrow et al., 2010; Alamgeer et al., 2018). The essential oil of various thyme species were reported to be active against airways disorders (Horváth and Ács, 2015). The essential oil obtained from T. serrulatus collected from different Ethiopian localities reported the presence of active ingredients such as thymol, carvacrol, p-cymene, γ-terpenene, and rosmarinic acid (Asfaw et al., 2000; Damtie et al., 2018). Further, terpenes, such as thymol and carvacrol, that have been found as major constituents in essential oil-containing plants have also been reported to possess bronchodilatory effects and reduce inflammation in airways disorders (Zhou et al., 2014). Further, the findings reported by (Ahmad et al., 2010) show that thymol and carvacrol have antibacterial and antifungal activities in addition to multiple other biological effects. Based on the above mentioned traditional and scientific evidences of various thymus species and their essential oils active against various airways disorders, we in this study aimed to chemically characterize, evaluate the possible tracheal relaxant effects of T. serrulatus in ex vivo model along with antimicrobial property of T. serrulatus essential oil.
Materials and Methods
Plant Material and Extraction
A sample of fresh erial parts of T. serrulatus, was collected during the flowering stage (October 2018) from the Amba Alaje mountain area located in Amba Alaje District (12° 59′ 54.4″N, 39° 32′ 52.3″E) South Tigray, Ethiopia. After it was collected, the plant material was identified according to the Flora of Tropical Africa (Daniel, 1900) and authenticated by botanist, Dr Getinet Masresha from the Department of Biology, University of Gondar and for further reference, the specimen has been deposited at the herbarium of the University with voucher number TH-001/2011.
After collection, the fresh aerial parts of T. serrulatus were cut into small pieces and subjected to hydro-distillation (water distillation) for 3 h by using a Clevenger-type apparatus. Hydro-distillation was performed 11 times until enough amount of sample was collected. The obtained essential oil was dried using anhydrous sodium sulfate and stored in tightly closed vials at 4°C until further testing and analysis (Asfaw et al., 2000). The calculated essential oil yield was expressed in percentage (% v/w), based on the weight of the fresh plant material. A stock solution of the obtained oil was prepared by mixing 300 uL of oil (≈300 mg) with 700 uL of distilled water containing 10% DMSO to obtain a stock concentration of 300 mg/ml. The formula, “density = mass/volume (d = m/v),” was used while calculating the mass of T. serrulatus oil where the density of oil was found equal to one. Further dilutions were prepared from this stock solution (300 mg/ml) where the final bath concentration of DMSO obtained was <1% in bronchodilatory and antimicrobial experiments.
Chemicals and Animals
Chemicals used in this study include atropine sulfate, carbachol (CCh), dicyclomine, isoprenaline, verapamil, and papaverine were procured from Sigma (St. Louis, MO, United States). The cAMP enzyme immunoassay kit used to estimate cAMP was procured from Sigma-Aldrich Co., United States. It is worth noting that all other chemicals used were of analytical grade.
Guinea-pigs (either sex, weighing 510–560 g) were housed at the animal house located at College of Pharmacy, PSAU, Saudi Arabia in a controlled environment maintained at 25 ± 2°C. The animals were provided with free access to water and commercial standard diet. All experiments were done as per the guidelines of the Institute of Laboratory Animal Resources, Commission on Life Sciences, National Research Council (National Research Council, 1996). The study was approved by Bio-Ethical Research Committee (BERC), Prince Sattam Bin Abdulaziz University (Approval number BERC-004–12–19).
GC-MS Analysis
The extracted oil of T. serrulatus was analyzed by the Perkin Elmer gas chromatograph (Clarus 600) coupled with a Perkin Elmer (Clarus 600 T) mass selective detector. Subsequently, 1 µL of the aliquots of the extracts were injected into Elite 5-MS capillary column (30m × 250 µm I. D, 0.25 µm film thickness; Perkin Elmer, Shelton, CT, United States) in the splitless mode of 1:20. The column temperature was kept at 40°C during sample injection and was raised to 150°C for 2 min having rate of 10°C/min and further increased to 300°C held for 2 min. The injector temperature was at 280°C, inlet line temperature at 220°C, and source temperature was 220°C. The total run time was 32 min. Helium was used as the carrier gas at a flow rate of 1.0 ml per min. Detection was carried out by using MS detection in electron-ionization mode using a single quadrupole detector and full-scan monitoring mode (m/z 40–600).
Each individual phyto-constituent was recognized on the basis of its retention time and mass spectrum included in the National Institute of Standards and Technology (NIST, 2005) and Wiley 2006. Out of 90 identified compounds, most of the compounds were identified from the matrix, and some came from the column itself. The compounds that occupied a negligible percentage of the total areas were discarded. Finally, about 50 compounds with match factor 60% were added in the method.
Ex-vivo Studies on Isolated Guinea-Pig Trachea
The trachea was isolated from guinea pig sacrificed by cervical dislocation and exsanguinated. Approximately, 2–3 cartilaginous rings, constituting one tracheal tissue, were opened by a longitudinal cut opposite to the smooth muscle layer. The opened tissue was then mounted in a 20 ml organ tube containing Kreb’s solution (37°C) and bubbled with carbogen. A tension equal to 1 g was constantly maintained in each of the tracheal tissues during the experiment. During the equilibration of tissue for 1 h, the Krebs solution in the bath was replaced every 15 min, while CCh (1 µM) and K+ (80 mM) were repeatedly used to stabilize the tissue until constant contractions of each agonist were achieved. After stabilization, the relaxant effect of TS Oil was determined by adding it cumulatively every 10 min to get the concentration-dependent effects. Tracheal contraction and relaxation were recorded by using isometric transducers attached to emkaBath with IOX software (France).
To investigate the possible effect of the plant oil to exhibit inhibitory action on Ca++ channels, the tracheal tissues were depolarized with high K+ (80 mM). K+ > 30 mM is known to depolarize the tissue by opening of voltage-gated Ca++ channels and/or other transporters and channels such as; Ca-Na exchanger and TRP channels and thus, causing smooth muscle contractions (Wray, 2010). Meanwhile, the substance inhibiting high K+-induced contraction might in some way leads to a reduction in the Ca++ influx through these pathways and might be considered as a Ca++ channel blockers (Hussain et al., 2008). In the next step, to endorse the Ca++ channel blockade (CCB) effect of TS Oil, the tracheal tissues stabilized in normal Kreb’s solution were immersed in Ca++-free Kreb’s solution containing EDTA (0.1 mM) where the tissues were incubated for 30 min to remove Ca++ from the tissue. Thereafter, the Ca++-free Kreb’s solution was replaced with K+-rich and Ca++-free Kreb’s solution. After incubation of tracheal tissue for an hour in K+-rich and Ca++-free Kreb’s solution, Ca++ induced contractions were recorded to obtained control CRCs. When the Ca++-CRCs were found to be superimposable, the tracheal tissues were incubated with TS Oil for 1 h. The CRCs of Ca++ were repeated in the presence of increasing concentrations (1 and 3 mg/ml) of the TS Oil and the results were compared using verapamil (0.03 and 0.1 µM) as standard (positive control).
To test whether the tracheal relaxant effect of the TS Oil also involves any additional mechanism(s), such as anticholinergic like effects, the CRCs of CCh were constructed by adding CCh in cumulative manner to the bath and the tissues were washed with fresh Krebs solution after achieving CCh maximum peaks. The CCh CRCs were repeated in the presence of pre-incubated tissues with increasing concentrations (0.3 and 1 mg/ml) of TS Oil for 1 h (Shah and Gilani, 2010).
Usually, plant materials that produce tracheal relaxation also show inhibitory actions on the PDE-enzyme, hence the method described by (Gilani et al., 2005) was followed to assess the presence of possible PDE inhibitory effects in our plant essential oil. Briefly, inhibitory CRCs of isoprenaline were constructed against CCh-induced contractions in the absence (control) and in the presence of TS Oil.
The cAMP Estimation
Phosphodiesterase’s decreases the levels of active cAMP and cGMP by metabolizing them to inactive forms AMP and GMP. Therefore, one of the mechanisms for an agent that causes increase in the levels of tissue cAMP and GMP is considered PDE inhibition. Hence, to test TS Oil for the possible PDE inhibitory action, cAMP contents of the trachea were measured by enzyme immunoassay using the direct cAMP enzyme immunoassay kit (Sigma-Aldrich, Saint Lewis, United States). For this purpose, two sets of tracheal tissues, one set was contracted with CCh alone (control) and second set was contracted with CCh followed by inhibition with increasing concentrations of TS Oil (treated). Both types of the tracheal sets were immediately removed from the organ bath and were frozen in liquid nitrogen. After grinding the tissues into fine powder, homogenization was done immediately in 0.1 M HCl (10 volumes) followed by centrifugation (600 g, 10 min) at 22°C. The supernatant was extracted from centrifuged samples and diluted with 0.1 M HCl and stored at −80°C till the time of determination of cAMP. The tissue concentration of cAMP content was expressed as pmol/mg protein.
In-vitro Antimicrobial Activity
Microbial Strains
TS Oil was tested for the possible antimicrobial effect against both, the reference strains from the American Type Culture Collection (ATCC) and National Collection of Type Culture (NCTC) and clinical strains. These strains have role in respiratory diseases and include Klebsiella pneumoniae, Pseudomonas aeruginosa, Staphylococcus aureus, and Candida albicans while the clinical pathogens include K. pneumoniae and methicillin-resistant S. aureus (MRSA). All the tested pathogen strains were routinely grown aerobically at 37°C.
Antimicrobial Assay
The screening activity of TS Oil was performed by the agar well diffusion method. A suspension of tested microbes equivalent to 0.5 McFarland was cultivated in Mueller-Hinton agar (MHA, Scharlau). TS Oil (50 µL) and 1% DMSO as control were added in each well, and the plates were incubated at 37°C for 24 h and zones of inhibitions were measured.
Determination of Minimum Inhibitory Concentration (MIC)
MIC assays of TS Oil were performed by the broth dilution method by following standard guidelines as detailed in (Clinical and Laboratory Standards Institute (CLSI), 2016). Nine dilutions of TS Oil, ranging from 12.5to 150 μg/ml, were prepared. Selected strain of each pathogen (10 µL) was inoculated into each individual concentration of TS Oil. For testing sterility, Mueller-Hinton broth (MHB, Scharlau) was tested alone as control whereas vehicle (DMSO) effect was tested on untreated bacteria inoculated on MHB alone and with 1% DMSO as growth control. After 24 h incubation at 37 °C, the MIC of the TS Oil was calculated based on the lowest concentration of antimicrobial agent that inhibited visible growth. Minimal microbicidal concentration (MMC) in 24 h incubation was evaluated by the sub-culture of 10 µL from the broth dilution in Mueller-Hinton agar, Scharlau. All assays were run in duplicate to authenticate and statistically analyze the data.
Statistical Analysis
Values are represented as mean ± standard error of the mean (SEM) and the median effective concentrations (EC50) with 95% confidence intervals (CI). The statistical parameters applied were Student’s t-test or Repeated Measures ANOVA followed by Bonferroni’s post-test for comparisons of CRCs with their respective controls. Differences were considered statistically significant at P < 0.05. CRCs were analyzed by non-linear regression using the GraphPad program (Graph Pad, San Diego, CA, United States).
Results
Essential Oil Yield (%)
Hydro-distillation of the erial part of the T. serrulatus gave 0.09% (v/w, on a fresh weight basis) pale yellow essential oil with a characteristic odor.
GC-MS Analysis
Mainly flavoring components like terpenes, sesquiterpenes, and higher fatty acids were found to present in TS Oil; however, it tested negative for other classes (Figure 1). The details of all compounds and their retention time (RT) are shown in Table 1.
Ex-vivo Effects on Guinea-Pig Trachea
Effect on CCh and High K+-Mediated Contractions
In the in-vitro experiments shown in Figure 2A, TS Oil inhibited CCh (1 µM) and K+ (80 mM)-induced contractions. A higher potency was observed against CCh with EC50 measurement of 0.86 mg/ml (0.64–1.24, n = 4) as compared to high K+ where the obtained EC50 value was 5.24 mg/ml (4.22–6.34, n = 5). A positive control drug, dicyclomine, behaved in a pattern similar to TS Oil against CCh and K+ (80 mM) with respective EC50 values of 0.48 µM (0.38–0.52, n = 4) and 5.16 µM (4.86–6.62, n = 4) (Figure 2B). On the other hand, unlike the plant oil and dicyclomine, verapamil was found more potent against K+-induced contractions with an EC50 value of 0.44 µM (0.32–0.58, n = 3) as compared to CCh-mediated contractions with EC50 value of 5.27 µM (4.55–6.42, n = 3) as seen in Figure 2C. Results of atropine, as shown in Figure 2D, show only selective relaxation against CCh with EC50 measurement of 0.06 µM (0.04–0.07, n = 3), while zero efficacy was observed against high K+.
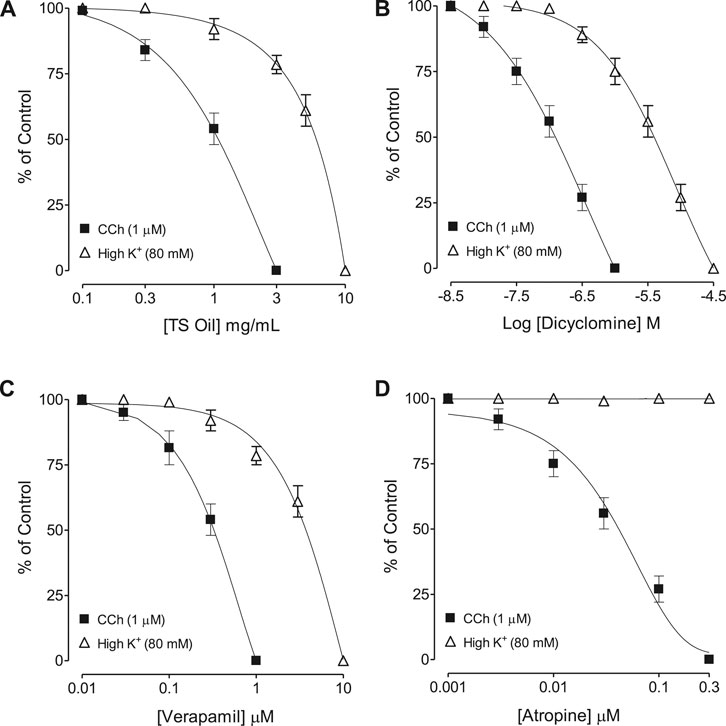
FIGURE 2. Concentration-response curves showing comparison of (A)Thymus serrulatus essential oil (TS Oil), (B) dicylomine, (C) verapamil, and (D) atropine for the inhibitory effect against carbachol (CCh) and high K + -induced contractions in isolated guinea-pig tracheal preparations. Values shown are mean ± SEM of 3–5 individual experiments conducted on the isolated tracheal tissue preparations from 3 to 5 different guinea-pigs.
Effect on CCh-Curves
In the anticholinergic, subtype antimuscarinic assay, in the absence of TS Oil, CRCs of CCh achieved maximum contraction (100%) with EC50 values of 0.21 µM (0.16–0.27; n = 5) while TS Oil pre-incubation at 0.3 mg/ml produced a parallel shift in CCh-peaks toward right without affecting the maximal response (100%) with EC50 values of 2.27 µM (1.78–2.90; n = 5), whereas the pre-incubation of trachea with next higher concentration of TS Oil (1.0 mg/ml) reduced the efficacy of CCh peaks to 73% with obtained EC50 values of 15.18 µM (11.40–21.92; n = 5) as shown in Figure 3A. Similar to TS Oil, dicyclomine (0.03 and 0.1 µM) pre-incubation, exhibited a similar pattern of shift in CCh peaks (Figure 3B), while the pre-incubation of verapamil at both concentrations (0.1 and 0.3 µM) produced a non-parallel rightward shift with the suppression CCh peaks (Figure 3C). Meanwhile, atropine (0.03 and 0.1 µM) pre-incubation, caused a parallel shift CCh CRCs towards right without the suppression of CCh peaks at both tested concentrations (Figure 3D).
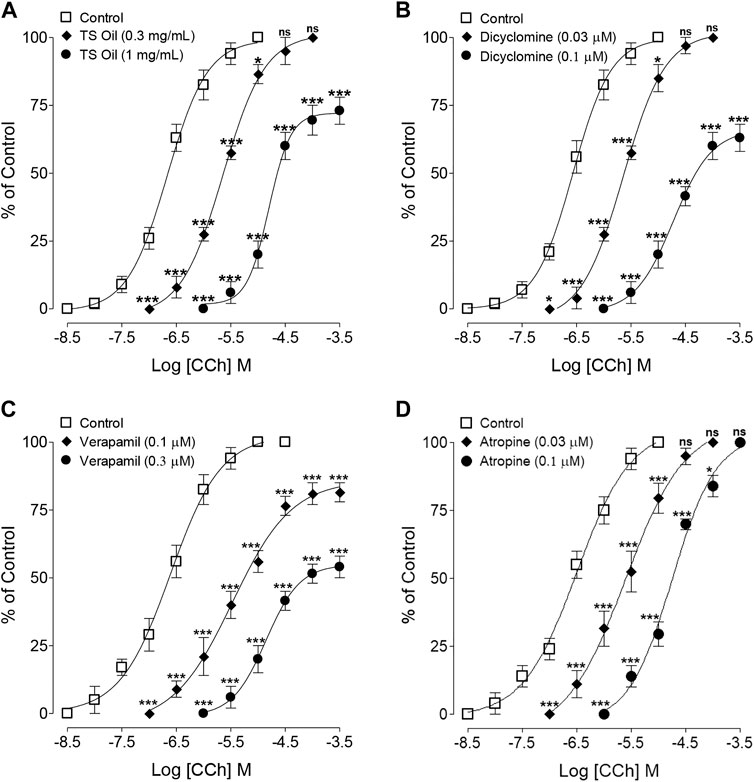
FIGURE 3. Concentration-response curves of carbachol (CCh) in the absence (control) and presence of increasing concentrations of (A)Thymus serrulatus essential oil (TS Oil), (B) dicyclomine, (C) verapamil, and (D) atropine in isolated guinea-pig tracheal preparations. Values shown are mean ± SEM of 3–4 individual experiments conducted on the isolated tracheal tissue preparations from 3 to 4 different guinea-pigs. *p < 0.05, **p < 0.01, and ***p < 0.001 shows comparison of the mean of CCh-mediated contractions in the pretreated tissues with TS Oil (A), dicyclomine (B), verapamil (C) and atropine (D) with the respective mean of CCh-mediated contractions in control (untreated) tracheal tissues (Repeated Measures ANOVA, followed by Bonferroni post-test).
Effect on Ca++-Curves
Following the preliminary results shown in Figure 2A showing effect of TS Oil against K+ (80 mM), the CCB like activity of TS Oil was further confirmed when the oil at both concentrations (1.0–3.0 mg/ml) produced a shift in the Ca++ CRCs toward right in a similar pattern of verapamil (Figures 4A,B). In the absence of TS Oil, CRCs of Ca++ achieved maximum contraction (100%) with EC50 values of 8.65 mM (6.47–11.56; n = 6) while TS Oil pre-incubation at 1 and 3 mg/ml produced a non-parallel rightward shift in with suppression of the maximal response to Ca++ (75%; 1 mg/ml) and (45%; 3 mg/ml) with EC50 values of 41.85 mM (26.20–66.84; n = 6) and 80.77 mM (37.39–174.50; n = 6), respectively, (Figures 4A,B).
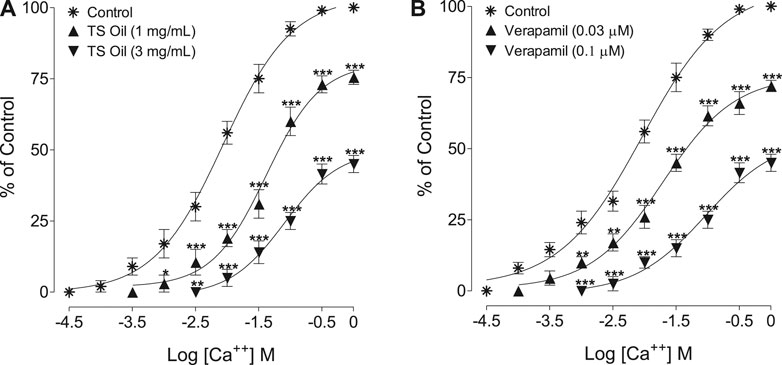
FIGURE 4. Concentration-response curves of Ca++ in the absence (control) and presence of the increasing concentrations of (A)Thymus serrulatus essential oil (TS Oil) and (B) verapamil in isolated guinea-pig tracheal preparations. The values shown are mean ± SEM of 3–4 individual experiments conducted on the isolated tracheal tissue preparations from 3 to 4 different guinea-pigs. *p < 0.05, **p < 0.01, and ***p < 0.001 shows comparison of the mean of Ca++-mediated contractions in the pretreated tissues with TS Oil. (A) and verapamil, (B) with the respective mean of Ca++-mediated contractions in control (untreated) tracheal tissues (Repeated Measures ANOVA, followed by Bonferroni post-test).
Effect on Isoprenaline-Curves
TS Oil pretreated tracheal tissues was concentration-dependent (0.3–1.0 mg/ml) and displaced the isoprenaline-induced inhibitory CRCs toward left similar to papaverine (1.0–3.0 μM) that was used as a control drug (Figures 5A,B). In the absence of TS Oil, 100% inhibition of CCh-mediated contractions was achieved by isoprenaline with EC50 values of 0.08 µM (0.05–0.12; n = 6) while TS Oil pre-incubation at 0.3 and1 did not affect the efficacy (100%) of the inhibitory response of isoprenaline against CCh, however significant reduction in the EC50 values [0.01 µM (0.010–0.017; n = 5) and 0.003 µM (0.002–0.004; n = 5), respectively, ] were observed (Figures 5A,B).
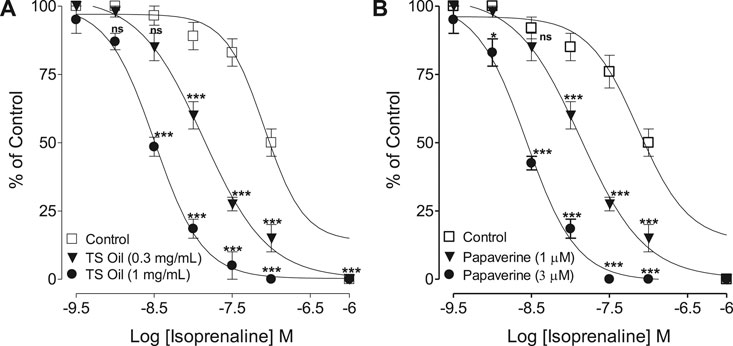
FIGURE 5. Inhibitory concentration-response curves of isoprenaline against carbachol (CCh)-induced contractions in the absence (control) and presence of different concentrations of (A)Thymus serrulatus essential oil (TS Oil) and (B) papaverine in isolated guinea-pig tracheal preparations. Values shown are mean ± SEM 3–4 individual experiments conducted on the isolated tracheal tissue preparations from 3 to 4 different guinea-pigs. nsp > 0.05, *p < 0.05, and ***p < 0.001 shows comparison of the mean of CCh-mediated inhibition by isoprenaline in the pretreated tissues with TS Oil (A) and verapamil, (B) with the respective mean of CCh-mediated inhibition by isoprenaline in control (untreated) tracheal tissues (Repeated Measures ANOVA, followed by Bonferroni post-test).
Effect on cAMP Levels
In the cAMP estimation assay of TS Oil, the cAMP levels measured in the untreated tracheal tissues (vehicle control) were 89.65 ± 0.745 pmol of cAMP/mg protein of tissue homogenate while in pre-incubated tissues with 0.3 and 1 mg/ml of TS Oil, the cAMP levels measured were 97.02 ± 1.459 (p < 0.05) and 105.42 ± 2.390 (p < 0.01) pmol/mg protein, respectively, (Figure 6A). The positive control (papaverine) pre-incubation with 1 and 3 µM increased the cAMP levels to 97.9 3 ± 1.365 pmol/mg protein (p < 0.05) and 104.43 ± 2.078 (p < 0.01) pmol/mg protein, respectively, (Figure 6B).
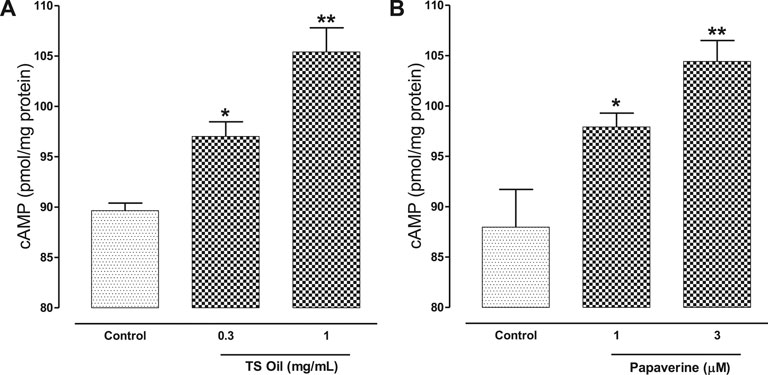
FIGURE 6. Effect of Thymus serrulatus essential oil (TS Oil) in carbachol (CCh)-induced contractions in the absence (control) and presence of different concentrations of (A)Thymus serrulatus essential oil (TS Oil) and (B) papaverine on the cyclic nucleotide content of guinea-pig trachea. Values shown are mean ± SEM of 3–4 individual experiments conducted on the isolated tracheal tissue preparations from 3 to 4 different guinea-pigs. *P < 0.05 and **P < 0.01, considered as statistically significant versus their respective controls (unpaired t-test).
In-vitro Antimicrobial Effects
Antibiotic Susceptibility Pattern of Clinical Pathogens
The antibiotic susceptibility pattern was depicted in Table 2 where K. pneumoniae was found resistant to Ampicillin and Amoxicillin/clavulanate only while, MRSA showed resistance to Imipenem, Cefoxitin, Cefotaxime, Ampicillin, Penciling, Oxacillin, and Amoxicillin/clavulanate.
Screening Activity and MIC Determination
The TS Oil was found most active against C. albicans and S. aureus where the zone of inhibition was measured at 28 mm as compared to MRSA, which showed a zone of inhibition of 22 mm. Additionally, there was little difference (2–3 mm) between standard strains gram-positive and gram-negative bacteria. The vehicle (<1% DMSO) was found to be inert as no zone of inhibition was observed in the wells of the cultivated plates (data not shown).
The MIC and MMC of TS Oil against the tested microbes was depicted in Table 3. C. albicans showed greater sensitivity to TS Oil (MIC 75 μg/ml) as compared to gram-positive and gram-negative bacteria. Meanwhile, MRSA (MIC 125 μg/ml) was found more resistant as compared to standard S. aureus (MIC 90 μg/ml) and other tested microbes. Moreover, no difference (p > 0.05) was observed in the activity of TS Oil against clinical and standard strains of K. pneumoniae (MIC 95 μg/ml).
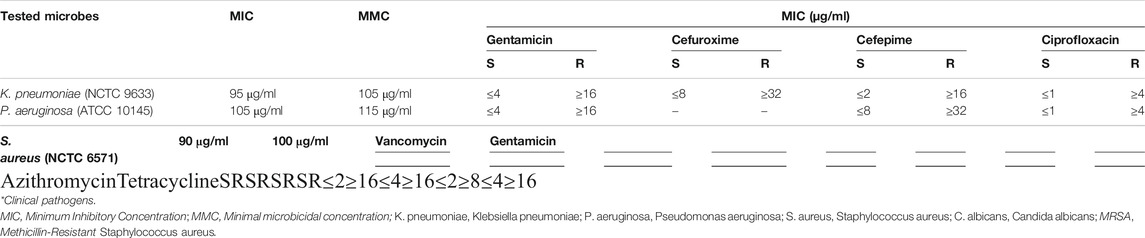
TABLE 3. Minimum Inhibitory Concentration (MIC) and Minimal Microbial Concentration (MMC) of Thymus serrulatus essential oil (TS Oil) vs. tested microbes and MIC of some antibiotics as per Clinical Laboratory Standard Institute (CLSI).
Discussion
This study attempted to investigate the possible therapeutic uses of T. serrulatus essential oil in hyperactive airways disorders and its effectiveness against related microbial infections (Meresa et al., 2017). For this purpose, T. serrulatus essential oil extracted by hydro-distillation was tested on isolated guinea-pig tracheal tissues mounted in organ bath with physiological buffer and carbogen. The antimicrobial assay was conducted on selected microbial strains, and GC-MS analysis was done to characterize the active ingredients present in the essential oil. In the preliminary ex-vivo experiments, TS Oil was found active and inhibit the contractions induced by selected tracheal spasmogens such as carbachol (1 µM) and high K+ (80 mM) (Imam et al., 2020; Rehman et al., 2020). Experiments were further extended to know the mechanism(s) involved in the observed tracheal relaxation. In this context, it has been reported earlier that medicinal plants usually produced bronchodilatory effects by anticholinergic, Ca++ channel inhibition and/or phosphodiesterase inhibition (Ansari et al., 2020). To elucidate the possible involvement of these mechanism(s), the pattern of the inhibitory CRCs of TS Oil in guinea-pig isolated tracheal tissues against CCh and high K+ (80 mM) were critically analysed. It was clearly evident in the results that TS Oil selectively show more potency to inhibit carbachol as compared to high K+-induced contractions, hence we hypothesized that the tracheal relaxant effects of TS Oil are targeted at muscarinic receptors and Ca++ channels. Dicyclomine, an antagonist of muscarinic receptors as well as an inhibitor of Ca++ ion influx (Downie et al., 1977), exhibited a similar pattern of inhibition with lower EC50 values against CCh when compared with high K+. On the other hand, verapamil, a pure Ca++ channel inhibitor (Fleckenstein, 1977), was found more potent against the K+-induced contractions compared to CCh whereas, atropine, a muscarinic receptor antagonist (Delmendo et al., 1989), showed efficacy against CCh-induced contractions only, as expected. The observed dual effect of TS Oil, as an anticholinergic and CCB like, was reconfirmed by standard protocols suggesting the construction of the CCh and Ca++ CRCs, respectively, in the absence and presence of pre-incubated concentrations of TS Oil in isolated trachea. In the antimuscarinic assay, the pre-incubation of the tracheal tissue with lower concentration of TS Oil resulted in a parallel shift of CCh-curves towards right without the suppression of its maximum effect, which is a distinctive feature of a competitive antagonist, such as atropine (Arunlakhshana and Schild, 1959), whereas the next higher concentration of TS Oil produced a non-parallel displacement in CCh-curves to the right with the suppression of the maximum effect. This suppression of the maximum response suggests the presence of non-specific inhibition, similar to that observed previously and in the current study with verapamil, a Ca++ channel blocker (Gilani et al., 2008). When tested, dicyclomine also caused shifting of CCh-curves toward the right in a pattern similar to that of TS Oil. However, the treatment of tracheal tissues with verapamil at both, lower and higher concentrations caused a non-parallel shift of CCh-curves toward right with suppression of the maximum response. To prove our second hypothesis of possible involvement of TS Oil with Ca++ channels, the pre-treated tracheal tissues with TS Oil produced a rightward shift of the Ca++ curves with suppression of maximum response in a pattern similar to verapamil, which may indicate the Ca++ inhibitory like effects of TS Oil. Although TS Oil inhibited high K+-mediated contractions and also shifted and inhibited the Ca++ CRCs which indicates CCB like activity, however the production of second messengers, such as cAMP and cGMP, which, in turn, activate PKA and PKG respectively, leading to the blocking of these channels which need to be further ascertained by using a direct voltage-dependent Ca++ channel activator, such as dihydropyridine Bay K8644 (Allen et al., 1985).
According to (Gilani et al., 2005), medicinal plants that exhibit tracheal relaxant effects usually show PDE inhibition and this effect usually co-exists with Ca++ channel blocking activity. Phosphodiesterase enzymes belong to a superfamily that has a role in catalyzing the breakdown of intracellular second messenger molecules, including cAMP and cGMP; therefore, the inhibition of PDE will indirectly increase the intracellular levels of cAMP (Beshay et al., 2001). Maintaining a high level of cAMP in airways produces a bronchodilatory effect by causing the smooth muscles of tracheal tissues to relax. To explore the possible involvement of PDE-inhibition as one of the additional mechanism(s) in the tracheal relaxation, TS Oil was tested in indirect (Isoprenaline CRCs) and direct assay (cAMP estimation). In the indirect assay, isoprenaline inhibitory CRCs were plotted against CCh-induced contractions in the absence and presence of TS Oil increasing concentrations. Interestingly, the pre-incubation of TS Oil causes a concentration-dependent potentiating effect on the isoprenaline-mediated inhibitory CRCs which is considered one of the possible indications of the tissue PDE inhibition as PDE inhibitors are known to potentiate the effect of isoprenaline (Lorenz and Wells, 1983). A similar pattern of a leftward shift in the isoprenaline-mediated inhibitory CRCs was observed with papaverine, a known PDE inhibitor (Lipworth, 2005). Furthermore, in the cAMP quantitative assay, TS Oil pre-incubated tissues showed an increase in the levels of cAMP in dose-dependent manner, similar to papaverine. This further strengthens the possible involvement of PDE inhibition as one of the added mechanism(s) in the tracheal relaxation of TS Oil. Notably, cAMP concentration is also increased by the beta-2 receptor stimulation present in the airways that finally causes activation of adenylate cyclase (AC) and thus causing smooth muscles relaxation (Martin et al., 1994). The possible stimulant effect of TS Oil on beta-2 receptors might be excluded as the tracheal relaxant response was not effected in the pre-incubated tissues with propranolol, a beta receptor blocker (data not shown), however TS Oil effect on AC activation further need to be investigated. No change in the inhibitory effect was observed in the several reported studies show that anticholinergics and PDE inhibitors play an important role in the management of asthma (Barnes, 2006), but their use is limited because they cause cardiac stimulation, a serious adverse event (Nicholas, 2006; Nawarth, 1981). It’s worth mentioning that PDE enzyme type-4 (PDE-4) is selectively involved in causing the smooth muscles relaxation of the airways, therefore we recommend to establish the inhibitory role of TS Oil on PDE-4. Ca++ channel blockers have also been reported for their usefulness in bronchoconstriction (Twiss et al., 2002) with cardio-suppressant effect (Billman, 1992). The presence of CCB-constituent(s) in the TS Oil with antimuscarinic and PDE inhibitory like activities is possibly meant by nature to compensate the tachycardia which is generally observed when anticholinergics or PDE inhibitors if administered alone. This type of combination of mechanism(s) supports the concept of the use of natural remedies due to their synergistic and/or side-effect neutralizing components (Gilani et al., 2005). Further, such combinations in a single plant reduce the cost of therapy and offer advantages in evidence-based studies (Ernst, 2005).
Studies show the involvement of multiple microbes, such as bacteria and fungi, causing airways diseases (Gileles-Hillel et al., 2015; Cheesman et al., 2017). The infections caused by antibiotic-resistant pathogens such as MRSA are complicated and challenging to treat. It has been reported that aromatic plant essential oils exhibit an antimicrobial effect when used alone (Costa et al., 2015) or in combination with antibiotics to combat multidrug-resistant bacteria (Cheesman et al., 2017). Therefore, TS Oil was also tested for possible antimicrobial potential to sort out added role of the TS Oil in addressing infectious-related airways disorders. Interestingly, TS Oil showed antimicrobial effects against MRSA, S. aureus, and C. albicans, which might further demonstrate its therapeutic effects on microbial infections of respiratory system. Further studies are recommended to know the mechanism of the observed antimicrobial effect of TS Oil. GS-analysis of the TS Oil revealed the presence of various monoterpene hydrocarbons. These include the chemotypes of Thymus species; thymol (34.3%) and carvacrol (19.5%) that are identified as major phyto-constituents. Minor phyto-constituents like monoterpenes such as pinene, cymene, and caryophyllene oxide have also been identified. Thymol and carvacrol have been reported to possess bronchodilatory, antihistaminic, and sympathomimetic properties (Boskabady and Jandaghi, 2003). Besides antibacterial and antifungal activities (Ahmad et al., 2010), thymol and carvacrol also reduce the levels of IgE, IL-4, IL-5, and IL-13, as well as the number of inflammatory cells in the airways (Zhou et al., 2014). Hence, the observed combination of activities (tracheal relaxation and antimicrobial) in the essential oil of T. serrulatus might be co-related to its major phytochemical constituents—thymol and carvacrol. However, the involvement of more phytochemicals and unexplored mechanism(s) cannot be ruled out.
The conventional bronchodilators used in asthma include β2 agonist, anticholinergics and PDE inhibitors are well known for their cardiac stimulation which is a serious side effect (Undem, 2006). However, it is possible that the presence of combination of Ca++ channel blockers with anticholinergics and PDE inhibitory like agents, as determined in the essential oil of T. serrulatus, are likely to offset the cardiac stimulation usually associated with these bronchodilators, particularly when given orally.
In conclusion, the above findings show that the hydrodistilled essential oil of Thymus serrulatus possess a unique combination of tracheal relaxant mechanisms that include anticholinergic, Ca++ antagonist and PDE inhibitory-like with added antimicrobial effects against strains involved in respiratory infections. However, further detailed studies are recommended to investigate and precisely identify the molecules that cause the observed activities.
Data Availability Statement
The original contributions presented in the study are included in the article/Supplementary Material, further inquiries can be directed to the corresponding authors.
Ethics Statement
The animal study was reviewed and approved by Bio-Ethical Research Committee (BERC), Prince Sattam Bin Abdulaziz University (Approval number BERC-004–12–19).
Author Contributions
Conceptualization, NR and MA; methodology, formal analysis and investigation, NR, MA, TH, AK, KA, SA, and FI; software, NR, MA, and SA; resources, NR, MA, AK, KA, and FI; writing—original draft preparation, NR and MA, writing—review and editing, AK, KA, SA, and FI; supervision, NR; funding acquisition, MA. All authors have read and agreed to the published version of the manuscript.
Conflict of Interest
The authors declare that the research was conducted in the absence of any commercial or financial relationships that could be construed as a potential conflict of interest.
Acknowledgments
The authors are thankful to the Department of Pharmacology and Toxicology, College of Pharmacy, Prince Sattam Bin Abdulaziz University, Al Kharj, Saudi Arabia for providing necessary facilities to carry out this research.
References
Ahmad, A., Khan, A., Akhtar, F., Yousuf, S., Xess, I., Khan, L. A., et al. (2010). Fungicidal activity of thymol and carvacrol by disrupting ergosterol biosynthesis and membrane integrity against Candida. Eur. J. Clin. Microbiol. Infect. Dis. 30, 41–50. doi:10.1007/s10096-010-1050-8
Alamgeer, Y. W., Asif, H., Sharif, A., Riaz, H., Bukhari, I. A., and Assiri, A. M. (2018). Traditional medicinal plants used for respiratory disorders in Pakistan: a review of the ethno-medicinal and pharmacological evidence. Chin. Med. 13 (1), 1–29. doi:10.1186/s13020-018-0204-y
Allen, S. L., Foster, R. W., Small, R. C., and Towart, R. (1985). The effects of the dihydropyridine Bay K 8644 in Guinea-pig isolated trachealis. Br. J. Pharmacol. 86 (1), 171–180. doi:10.1111/j.1476-5381.1985.tb09447.x
Ansari, M. N., Rehman, N. U., Karim, A., Bahta, T., Abujheisha, K. Y., Ahamad, S. R., et al. (2020). Evaluation of bronchodialatory and antimicrobial activities of Otostegia fruticosa: a multi-mechanistic approach. Saudi Pharm. J. 28, 281–289. doi:10.1016/j.jsps.2020.01.007
Arunlakhshana, O., and Schild, H. O. (1959). Some quantitative uses of drug antagonists. Br. J. Pharmacol. 14, 48–58. doi:10.1111/j.1476-5381.1959.tb00928.x
Asfaw, N., Storesund, H. J., Skattebøl, L., Tønnesen, F., and Aasen, A. J. (2000). Volatile oil constituents of two Thymus species from Ethiopia. Flavour Fragr. J. 15, 123–125. doi:10.1002/(SICI)1099-1026(200003/04)15:2<123::AID-FFJ879>3.0.CO;2-8
Barnes, P. J. (2006). Drugs for asthma. Br. J. Pharmacol. 147, S297–S303. doi:10.1038/sj.bjp.0706437
Begrow, F., Engelbertz, J., Feistel, B., Lehnfeld, R., Bauer, K., and Verspohl, E. (2010). Impact of thymol in thyme extracts on their antispasmodic action and ciliary clearance. Planta Med. 76 (4), 311–318. doi:10.1055/s-0029-1186179
Beshay, E., Croze, F., and Prud’homme, G. J. (2001). The phosphodiesterase inhibitors pentoxifylline and rolipram suppress macrophage activation and nitric oxide production in vitro and in vivo. Clin. Immunol. 98, 272–279. doi:10.1006/clim.2000.4964
Billman, G. E. (1992). “The antiarrythmic effects of the calcium antagonists,” in Calcium antagonists in clinical medicine. Editor M. Epstein (Philadelphia, United States: Hanley and Belfus Inc.), 183–212.
Boskabady, M. H., and Jandaghi, P. (2003). Relaxant effects of carvacrol on Guinea pig tracheal chains and its possible mechanisms. Pharmazie 58 (9), 661–663.
Cheesman, M. J., Ilanko, A., Blonk, B., and Cock, I. E. (2017). Developing new antimicrobial therapies: are synergistic combinations of plant extracts/compounds with conventional antibiotics the solution? Pharmacogn. Rev. 11 (22), 57–72. doi:10.4103/phrev.phrev_21_17
Clinical and Laboratory Standards Institute (CLSI) (2016). Performance standards for antimicrobial susceptibility testing; twenty fourth informational supplement M100-S24. 13th Edn. Wayne, PA, United States: Approved Standard, 332.
Costa, D. C., Costa, H. S., Albuquerque, T. G., Ramos, F., Castilho, M. C., and Sanches-Silva, A. (2015). Advances in phenolic compounds analysis of aromatic plants and their potential applications. Trends Food Sci. Technol. 45, 336–354. doi:10.1016/j.tifs.2015.06.009
Damtie, D., Braunberger, C., Conrad, J., Mekonnen, Y., and Beifuss, U. (2018). Composition and hepatoprotective activity of essential oils from Ethiopian thyme species (Thymus serrulatus and Thymus schimperi). J. Essent. Oil Res. 31, 120–128. doi:10.1080/10412905.2018.1512907
Damtie, D., Mekonnen, Y., and Eyado, A. (2017). Acute oral toxicity study of Thymus serrulatus and Thymus schimperi from Ethiopia. Ethiop. J. Sci. Technol. 10 (3), 181–192. doi:10.4314/ejst.v10i3.3
Damtie, D., and Mekonnen, Y. (2015). Thymus species in Ethiopia: distribution, medicinal value, economic benefit, current status, and threatening factors. Ethiop. J. Sci. Technol. 8 (2), 81–92. doi:10.4314/ejst.v8i2.3
Daniel, O. (1900). Flora of tropical Africa. Editor W.T. Thiselton-Dyer (London, United Kingdom: L. Reeve and Co.), 5, 454.
Delmendo, R. E., Michel, A. D., and Whiting, R. L. (1989). Affinity of muscarinic receptor antagonists for three putative muscarinic receptor binding sites. Br. J. Pharmacol. 96, 457–464. doi:10.1111/j.1476-5381.1989.tb11838.x
Downie, J. W., Twiddy, D. A., and Awad, S. A. (1977). Antimuscarinic and noncompetitive antagonist properties of dicyclomine hydrochloride in isolated human and rabbit bladder muscle. J. Pharmacol. Exp. Ther. 201, 662–668.
Ernst, E. (2005). The efficacy of herbal medicine-an overview. Fundam. Clin. Pharmacol. 19, 405–409. doi:10.1111/j.1472-8206.2005.00335.x
Fleckenstein, A. (1977). Specific Pharmacology of calcium in myocardium, cardiac pacemakers, and vascular smooth muscle. Annu. Rev. Pharmacol. Toxicol. 17, 149–166. doi:10.1146/annurev.pa.17.040177.001053
Geleta, B., Eyasu, M., Kebamo, S., Debella, A., Makonnen, E., and Abebe, A. (2015). In vitro vasodilatory effect of aqueous leaf extract of Thymus serrulatus on thoracic aorta of Guinea pigs. Asian Pac. J. Trop. Biomed. 5 (1), 15–18. doi:10.1016/S2221-1691(15)30164-7
Getachew, H. (2018). Evaluation of antihypertensive, hypotensive and antihyperlipidemic activities of aqueous crude extract of Thymus serrulatus leaves in rats. Available at: http://etd.aau.edu.et/handle/123456789/14300?show=full (Accessed January 15, 2021).
Gilani, A. H., Khan, A.-U., Subhan, F., and Khan, M. (2005). Antispasmodic and bronchodilator activities of St John’s wort are putatively mediated through dual inhibition of calcium influx and phosphodiesterase. Fundam. Clin. Pharmacol. 19, 695–705. doi:10.1111/j.1472-8206.2005.00378.x
Gilani, A. H., Khan, A.-U., Ali, T., and Ajmal, S. (2008). Mechanisms underlying the antispasmodic and bronchodilatory properties of Terminalia bellerica fruit. J. Ethnopharmacol. 116, 528–538. doi:10.1016/j.jep.2008.01.006
Gileles-Hillel, A., Shoseyov, D., Polacheck, I., Korem, M., Kerem, E., and Cohen-Cymberknoh, M. (2015). Association of chronic Candida albicans respiratory infection with a more severe lung disease in patients with cystic fibrosis. Pediatr. Pulmonol. 50 (11), 1082–1089. doi:10.1002/ppul.23302
Guo, Z.-Y., Zhang, Z.-Y., Xiao, J.-Q., Qin, J.-H., and Zhao, W. (2018). Antibacterial effects of leaf extract of Nandina domestica and the underlined mechanism. Evid. Based Complement. Altern. Med. 2018, 1–9. doi:10.1155/2018/8298151
Horváth, G., and Ács, K. (2015). Essential oils in the treatment of respiratory tract diseases highlighting their role in bacterial infections and their anti-inflammatory action: a review. Flavour Fragr. J. 30 (5), 331–341. doi:10.1002/ffj.3252
Hussain, A. I., Anwar, F., Hussain Sherazi, S. T., and Przybylski, R. (2008). Chemical composition, antioxidant and antimicrobial activities of basil (Ocimum basilicum) essential oils depends on seasonal variations. Food Chem. 108, 986–995. doi:10.1016/j.foodchem.2007.12.010
Imam, F., Rehman, N. U., Ansari, M. N., Qamar, W., Afzal, M., and Alharbi, K. S. (2020). Effect of Roflumilast in airways disorders via dual inhibition of phosphodiesterase and Ca2+-channel. Saudi Pharm. J. 28, 698–702. doi:10.1016/j.jsps.2020.04.011
Khan, A., Rehman, N.-U., Gilani, A.-H., Ahmed, Z., Al-Massara, S., El-Gamal, A., et al. (2018). Possible mechanism(s) underlying the antidiarrheal, antispasmodic and bronchodilatory activities of the pericarp of Albizia lebbeck. Int. J. Pharmacol. 15, 56–65. doi:10.3923/ijp.2019.56.65
Lipworth, B. J. (2005). Phosphodiesterase-4 inhibitors for asthma and chronic obstructive pulmonary disease. Lancet 365, 167–175. doi:10.1016/S0140-6736(05)17708-3
Lorenz, K. L., and Wells, J. N. (1983). Potentiation of the effects of sodium nitroprusside and of isoproterenol by selective phosphodiesterase inhibitors. Mol. Pharmacol. 23, 424–430. doi:10.1111/j.1476-5381.tb13641.x
Martin, C. A. E., Naline, E., Bakdach, H., and Advenier, C. (1994). β3-adrenoceptor agonists, BRL 37344 and SR 58611A, do not induce relaxation of human, sheep and Guinea-pig airway smooth muscle in vitro. Eur. Respir. J. 7, 1610–1615. doi:10.1183/09031936.94.07091610
Melka, A. E., Makonnen, E., Debella, A., Fekadu, N., and Geleta, B. (2016). Diuretic activity of the aqueous crude extract and solvent fractions of the leaves of Thymus serrulatus in mice. J. Exp. Pharmacol. 8, 61–67. doi:10.2147/JEP.S121133
Meresa, B. A., Fekadu, N., Degu, S., Tadele, A., and Geleta, B. (2017). An ethno botanical review on medicinal plants used for the management of hypertension. J. Clin. Exp. Pharmacol. 07, 1–16. doi:10.4172/2161-1459.1000228
National Research Council (1996). Guide for the care and use of laboratory animals. Washington, DC: National Academy Press, 1–7.
Nawarth, H. (1981). Action potential, membrane currents and force of contraction in cat ventricular heart muscle treated with papaverine. J. Pharmacol. Exp. Ther. 218, 544–549.
Nicholas, J. G. (2006). Anticholinergic agents in asthma and COPD. Eur. J. Pharmacol. 533, 36–39. doi:10.1016/j.ejphar.2005.12.072
Rehman, N. U., Ansari, M. N., and Samad, A. (2020). In silico, ex vivo and in vivo studies of roflumilast as a potential antidiarrheal and antispasmodic agent: inhibition of the PDE-4 enzyme and voltage-gated Ca++ ion channels. Molecules 25, 1008. doi:10.3390/molecules25041008
Shah, A. J., and Gilani, A.-H. (2010). Bronchodilatory effect of Acorus calamus (Linn.) is mediated through multiple pathways. J. Ethnopharmacol. 131, 471–477. doi:10.1016/j.jep.2010.07.024
Wray, S. (2010). “Chapter 127–calcium signaling in smooth muscle,” in Handbook of cell signaling. 2nd Edn, Editors R. A Bradshaw, and E. A Dennis (Cambridge, MA: Academic Press), 1009–1025.
Twiss, M. A., Harman, E., Chesrown, S., and Handeles, L. (2002). Efficacy of calcium channel blockers as maintenance therapy for asthma. Br. J. Clin. Pharmacol. 53, 243–249. doi:10.1046/j.0306-5251.2001.01560.x
Undem, B. J. (2006). “Pharmacotherapy of asthma,” in Goodman and Gilman’s the pharmacological bases of therapeutics. 11th Edn, Editors L. L. Brunton, J. S. Lazo, and K. L. Parker (New York, NY: McGraw-Hil), 717–736.
Keywords: antimicrobial, asthma, bronchodilatation, Ca++ channel blocker, phosphodiesterase inhibitor, Thymus serrulatus
Citation: Rehman NU, Ansari MN, Haile T, Karim A, Abujheisha KY, Ahamad SR and Imam F (2021) Possible Tracheal Relaxant and Antimicrobial Effects of the Essential Oil of Ethiopian Thyme Species (Thymus serrulatus Hochst. ex Benth.): A Multiple Mechanistic Approach. Front. Pharmacol. 12:615228. doi: 10.3389/fphar.2021.615228
Received: 08 October 2020; Accepted: 22 February 2021;
Published: 05 April 2021.
Edited by:
Lin Lin, Jiangsu University, ChinaReviewed by:
Nidal Jaradat, An-Najah National University, PalestineJavad Sharifi-Rad, Shahid Beheshti University of Medical Sciences, Iran
Luiz Henrique Cesar Vasconcelos, Federal University of Paraíba, Brazil
Copyright © 2021 Rehman, Ansari, Haile, Karim, Abujheisha, Ahamad and Imam This is an open-access article distributed under the terms of the Creative Commons Attribution License (CC BY). The use, distribution or reproduction in other forums is permitted, provided the original author(s) and the copyright owner(s) are credited and that the original publication in this journal is cited, in accordance with accepted academic practice. No use, distribution or reproduction is permitted which does not comply with these terms.
*Correspondence: Najeeb Ur Rehman, bl9yZWhtYW41QGhvdG1haWwuY29t; Mohd Nazam Ansari, bmF6YW0uYW5zYXJpQGdtYWlsLmNvbQ==