- 1College of Public Health, Zhengzhou University, Zhengzhou, China
- 2Department of Radiation Oncology, Henan Provincial People’s Hospital, Zhengzhou University People’s Hospital, Zhengzhou, China
- 3School of Pharmaceutical Sciences, Zhengzhou University, Zhengzhou, China
- 4General ICU, Henan Key Laboratory of Critical Care Medicine, The First Affiliated Hospital of Zhengzhou University, Zhengzhou, China
- 5College of Agriculture and Natural Sciences, University of Cape Coast, Cape Coast, Ghana
- 6Department of Respiratory and Critical Care Medicine, Henan Provincial People’s Hospital & People’s Hospital of Zhengzhou University, Zhengzhou, China
Background: Carbapenemase-producing Klebsiella pneumoniae (CpKP) has been implicated as an increasing threat to public health. CpKP is a ubiquitous, opportunistic pathogen that causes both hospital and community acquired infections. This organism hydrolyzes carbapenems and other β-lactams and thus, leading to multiple resistance to these antibiotics. Despite the difficult to treat nature of infections caused by CpKP, little has been discussed on the mortality, clinical response and microbiological success rates associated with various antibiotic regimen against CpKP. This meta-analysis was designed to fill the paucity of information on the clinical impact of various antibiotic therapeutic regimens among patients infected with CpKP.
Materials and Methods: Literature in most English databases such as Medline through PubMed, Google Scholar, Web of Science, Cochrane Library and EMBASE, were searched for most studies published between the years 2015–2020. Data were analyzed using the R studio 2.15.2 statistical software program (metaphor and meta Package, Version 2) by random-effects (DerSimonian and Laird) model.
Results: Twenty-one (21) studies including 2841 patients who had been infected with CpKP were analysed. The overall mortality rate was 32.2% (95%CI = 26.23–38.87; I2 = 89%; p-value ≤ 0.01, Number of patients = 2716). Pooled clinical and microbiological success rates were 67.6% (95%CI = 58.35–75.64, I2 = 22%, p-value = 0.25, Number of patients = 171) and 74.9% (95%CI = 59.02–86.09, I2 = 53%, p-value = 0.05, Number of patients = 121), respectively. CpKP infected patients treated with combination therapy are less likely to die as compared to those treated with monotherapy (OR = 0.55, 95%CI = 0.35–0.87, p-value = 0.01, Number of patients = 1,475). No significant difference existed between the mortality rate among 60years and above patients vs below 60years (OR = 0.84, 95%CI = 0.28–2.57, p-value = 0.76, 6 studies, Number of patients = 1,688), and among patients treated with triple therapy vs. double therapy (OR = 0.50, 95%CI = 0.21–1.22, p-value = 0.13, 2 studies, Number of patients = 102). When compared with aminoglycoside-sparing therapies, aminoglycoside-containing therapies had positive significant outcomes on both mortality and microbiological success rates.
Conclusion: New effective therapies are urgently needed to help fight infections caused by this organism. The effective use of various therapeutic options and the strict implementation of infection control measures are of utmost importance in order to prevent infections caused by CpKP. Strict national or international implementation of infection control measures and treatment guidelines will help improve healthcare, and equip governments and communities to respond to and prevent the spread of infectious diseases caused by CpKP.
Introduction
Klebsiella pneumoniae is one of the Enterobacteriaceae that has increasingly become a threat to public health (Effah et al., 2020), and has been implicated in severe mortality and morbidity (Munoz-Price et al., 2013; World Health Organization, 2017). K. pneumoniae is a ubiquitous, opportunistic pathogen that causes both hospital and community acquired infections including pneumonia, urinary tract infection, bloodstream infections, cystitis, surgical wound infections, endocarditis, pyogenic liver abscesses and endogenous endophthalmitis (Podschun and Ullmann, 1998; Navon-Venezia et al., 2017). Based on several resistant mechanisms employed by this organism, there have been several strains that have been reported circulating worldwide. These mechanisms which include but not limited to vertical and horizontal transfer of resistant genes, efflux pump mechanisms and the dissemination and acquisition of resistant genes that are carried on plasmids and transposons have resulted in these hard to treat strains. The most notable strain of K. pneumoniae is the type that produces extended-spectrum β-lactamases (ESBLs). ESBL-K. pneumoniae (ESBL-KP) are now distributed worldwide and has been implicated in numerous outbreaks (Paterson and Yu, 1999; Tumbarello et al., 2006). According to Beigverdi et al. (2019), the pooled prevalence of ESBL-KP was 43.5% (95% CI 39.3–47.9%) among clinical K. pneumoniae isolates in Iran and the prevalence of each resistant mediated gene varies. Carbapenems are usually the antibiotics that are the first call or the first-line therapy that are employed during the treatment of severe infections caused by ESBL-producing K. pneumoniae (Pitout and Laupland, 2008). However, Yigit et al. (2001), Jacob et al. (2013) and other authors have reported on K. pneumoniae carbapenemase (KPC)-producing K. pneumoniae isolate. Carbapenemases are lactamases that hydrolyze carbapenems and other β-lactams and thus, provides resistance to these antibiotics. The Carbapenemase genes have since 2001 being spreading to all corners of the world. The KPC gene is the most common and the most prevalent of all the carbapenemases followed by the OXA genes (mainly blaOXA-48, blaOXA-162, and blaOXA-181), NDM genes, VIM genes, IMP genes, SME genes and others. The spread of these encoding plasmids poses a significant threat to public health as their acquisition leads to a multi-drug resistant (MDR) and extremely drug resistant (XDR) pathogen. In terms of epidemiological data, the prevalence of carbapenemase-producing Enterobacteriaceae (CPE) vary considerably from country to country. KPC and other carbapenemases including IPM, VIM, OXA-48 and NDM are known to be endemic in Israel, Japan, Greece, Turkey and India, respectively, and have been distributed across the globe (Cantón et al., 2012). KPC producers are also known to be endemic in some areas of Latin America, such as Colombia and Argentina (Levy Hara et al., 2013). There have been reported imports of KPC producers from endemic areas into Europe and this has led to a widespread of KPC producers to almost every part of Europe (Munoz-Price et al., 2013). European countries with the highest KPC-producing isolates are Italy and Greece, with rates ranging between 25 and 50% and >50% for invasive infections caused by CPE (European Antimicrobial Resistance Surveillance Network (EARS-Net), 2017). In Israel, KPC producers have been linked to numerous healthcare-associated reports and some community-acquired cases. The extent to which KPC producers are distributed in South East Asia is unknown, even though China may face some endemic situations. Reports on KPC-producing isolates in India are scanty, however, NDM and OXA-48-like carbapenemases are the most commonly reported. ST 258, a K. pneumoniae clone that produces KPC-2 or KPC-3, has been widely identified worldwide (Cuzon et al., 2010). Several outbreaks of different NDM producers have been documented in Pakistan, India and Sri Lanka (Poirel et al., 2011). In India, the prevalence of NDM is estimated at 5–15% (Day et al., 2013). Because of their close ties with India and Pakistan, the United Kingdom (UK), has recorded considerable spread of NDM producers (Johnson and Woodford, 2013). Since then, there have been a number of reports on NDM producers in Enterobacteriaceae from countries all over the globe, including countries in Africa, Asia, Europe, Australia, and the Americas (Berrazeg et al., 2014). OXA-48 producers have been extensively reported in Turkey, North Africa, the Middle East and India as the cause of healthcare-associated outbreaks (European Antimicrobial Resistance Surveillance Network (EARS-Net), 2017; Girlich et al., 2014). There is a growing prevalence of this carbapenemase in many countries of Europe, including France. As reported by the European Antimicrobial Resistance Surveillance Network (EARS-Net), 2017, carbapenem resistance was rampantly recorded in K. pneumoniae between the years 2014 and 2018, with some countries reporting resistance rates exceeding 10%.
Due to the difficult to treat nature of infections caused by carbapenem-producing K. pneumoniae (CpKP), there are limited treatment options that are currently available. Studies have reported that antibiotics such as colistin, gentamicin, tigecycline, and fosfomycin that are used for the treatment of infections caused by CpKP can lead to severe adverse effects such as nephrotoxicity (Akajagbor et al., 2013; Osorio et al., 2017) and hepatotoxicity (Lim et al., 2010; Florescu et al., 2012). According to (Tumbarello et al., 2012a; Daikos et al., 2014), it has been reported that during bacteremic infections, combination treatment regimens are recommended; however, it has been reported by Gutiérrez-Gutiérrez et al. (2017) that, combination therapies should be used when patients are in critical conditions. Also, in some retrospective studies on bloodstream infections caused by CpKP, it was realized that patients that were placed on antibiotic combination regimen were significantly more likely to survive than patients who were on monotherapeutic regimen (Zarkotou et al., 2011; Qureshi et al., 2012). Although a lot of studies have asserted that the use of more than one in vitro active drug may be superior to monotherapy, the rate of clinical failure still remains high (Tzouvelekis et al., 2014; Tumbarello et al., 2015a; Gutiérrez-Gutiérrez et al., 2017).
Despite the milestones achieved by various researchers in their quest to add to knowledge on the mortality, clinical response and microbiological response rates associated with various antibiotic regimen against carbapenemase-producing K. pneumoniae, there is still a paucity of information that needs to be filled. Most of the available review studies have focused on the mortality outcomes associated with these regimens (Tzouvelekis et al., 2014; Xu et al., 2017; Rodríguez-Baño et al., 2018) and these studies have not focused on CpKP but rather the broader carbapenem-resistant Enterobacteriaceae (CRE). To know the true effect of these therapeutic regimens, there is a need to evaluate the total clinical outcomes (mortality rates, clinical success rate and the microbiological success rates) associated with these regimens.
In this current study, we reviewed the clinical impact of various antibiotic therapeutic regimen against CpKP infected patients. First, we reviewed on various mono and combination therapies utilized in the treatment of infections caused by CpKP. Then, we finally performed a meta-analysis to evaluate the therapeutic outcomes of various antibiotic regimen on various clinical outcomes.
Current and Emerging Treatment Strategies Against CpKP
Monotherapy
The most active aminoglycoside against CpKP is gentamicin (Castanheira et al., 2010; Souli et al., 2010; Livermore et al., 2011). There are limited data on the use of aminoglycosides as monotherapy, however, aminoglycoside monotherapy appears to be the most effective in the treatment of urinary tract infections (UTIs) caused by CpKP (Hirsch and Tam, 2010; Satlin et al., 2011; Tzouvelekis et al., 2014). When compared to the use of polymyxin B or tigecycline in the treatment of CpKP bacteriuria, treatment with an in vitro active aminoglycoside resulted in a much greater rate of microbiological clearance (Satlin et al., 2011). The use of targeted gentamicin among CpKP-induced sepsis patients was associated with reduced mortality (20.7 versus 61.9%, p = 0.02) until Day 30 (Gonzalez-Padilla et al., 2015). Also, oral gentamicin treatment was effective in the eradication of CpKP from the gastrointestinal reservoir which means that gentamycin could serve as additional tool in the combat against the nosocomial spread and severe infections caused by this difficult-to-treat organism (Zuckerman et al., 2011).
In a study by Lee et al. (2009), blood isolates from one patient infected with CpKP and treated with polymyxin B monotherapy showed a significantly increased polymyxin B MIC in just 5 days (0.75 g/ml to 1,024 g/ml). A meta-analysis of 15 studies involving 55 different patients concluded that colistin monotherapy had lower clinical success than colistin combination therapy (with tigecycline or gentamicin) for the treatment of infections caused by CpKP (14.3% [1 of 7] vs. 72.7% [8 of 11]) (Hirsch and Tam, 2010).
In addition, a study involving a small number of patients infected with CpKP concluded that 71.4 percent (5 of 7) had a favourable clinical outcome with tigecycline treatment (Hirsch and Tam, 2010). In two separate cohort studies, high mortality rates were reported to be associated with the use of tigecycline monotherapy in the treatment of bloodstream infections caused by CpKP (Tumbarello et al., 2012b; Daikos et al., 2014). One study assessed the therapeutic outcomes of tigecycline on 164 non-duplicate clinical strains of CpKP isolated from hospital-acquired pneumonia (HAP). The study found that doubling the tigecycline dose resulted in a higher cumulative fraction of response, which is an indication of better clinical efficacy (90.2 percent vs. 71.2 percent) (Trecarichi et al., 2016). Balandin Moreno et al. (2014) discovered no statistical significant differences in mortality rates between high-dose tigecycline and standard-dose tigecycline.
Other potential monotherapies with promising outcomes include: cefiderocol, a new siderophore cephalosporin, which has shown significant in vitro and in vivo activity against CpKP (Saisho et al., 2018). Cefiderocol has a unique antibacterial mechanism in which its catechol side chain binds to ferric acid, and the complex is actively carried into bacteria via bacterial iron transporters (Ito et al., 2016). Cefiderocol is also very effective at inhibiting carbapenemase hydrolysis (Wright et al., 2017). Cefiderocol has shown considerable in vitro activity against carbapenem-resistant Enterobacteriaceae (CRE) isolates, recording a cefiderocol MICs of less than 4 mg/L in 97.0 percent (991/1,022) of isolates (Hackel et al., 2018). Eravacycline is another unique synthetic tetracycline analogue which overcomes the basic mechanism of tetracycline resistance and possesses in vitro action against CpKP. However, its clinical evidence on the treatment of CpKP is sparse. Apramycin is also a monotherapy with promising action against CpKP. Apramycin is an aminoglycoside that has been traditionally used in veterinary medicine.
Combination Regimens
Treatment with a dual-carbapenem may be an effective option for CpKP infections (Ceccarelli et al., 2013; Giamarellou et al., 2013). Based on experimental data, it has been known that the affinity of KPC enzyme for ertapenem is higher than other carbapenems; therefore, when administered together, KPC preferentially inactivates ertapenem, thereby preventing degradation and subsequently enhancing the potency of the other carbapenems (Bulik and Nicolau, 2011; Wiskirchen et al., 2013). According to case reports, ertapenem in combination with doripenem or meropenem has been used successfully to treat CpKP infections (bacteremia, ventilator-associated pneumonia, and UTI). Dual-carbapenem therapy is a promising option that may be most effective when used concomitantly with a third drug (Zavascki et al., 2013). To assess the mortality rate associated with carbapenem-containing-combination therapy for CpKp bacteremia, Daikos et al. (2014) performed a large cohort study and reported an increased mortality rate from 19.4% (6 of 31, MIC ≤8 μg/ml) to 35.5% (11 of 31, MIC >8 μg/ml). In a meta-analysis of 20 clinical studies, it was realized that therapies that contain carbapenem recorded lower mortality rates compared to the non-carbapenem-containing regimens (18.8 vs. 30.7%) (Tzouvelekis et al., 2014). In addition, a retrospective study from a 10-bed intensive care unit found that carbapenem-sparing-combination therapy was effective in 24 of 26 (92 percent) patients with CpKP infections (Sbrana et al., 2013). A non-controlled case series study found that combining two carbapenems (ertapenem plus prolonged infusion of meropenem or doripenem) can be effective in treating CpKP infections, with clinical success rates ranging from 39 to 77.8 percent (Cprek and Gallagher, 2016; Souli et al., 2017).
A new β-lactam/β-lactamase inhibitor (BL/BLI), ceftazidime/avibactam, has been approved for the treatment of complicated intra-abdominal infection, complicated urinary tract infection, hospital-acquired and ventilator-associated pneumonia caused by CpKP (Kaye and Pogue, 2015). Avibactam, unlike most β-lactamase inhibitors, is not a β-lactam. However, this novel drug is a unique synthetic non-β-lactam (diazabicyclooctane)/β-lactamase inhibitor that inhibits a wide range of β-lactamases, including Ambler Class A (GEM, SHV, CTX-M, and KPC), Class C (AmpC), and some Class D (OXA-48) β-lactamases (de Jonge et al., 2016). However, Class B metallo- β-lactamases (MBLs) (IMP, VIM, VEB, and NDM) are not inhibited by avibactam (Syue et al., 2016; Wong and van Duin, 2017). Because of this great inhibitory potential, avibactam, when added to ceftazidime, restores the potent activity of ceftazidime against CpKP and other CRE.
Furthermore, to achieve a more efficient synergistic effect in the treatment of infections caused by CpKP, meropenem/vaborbactam (MER-VAB) can be used alone or in combination with non-active in vitro drugs (Qureshi et al., 2012; Guimarães et al., 2019; Tumbarello et al., 2019). Because Ambler class A and C carbapenemases are responsible for conferring resistance to meropenem by most bacteria, vaborbactam, which is a novel boron-containing serine lactamase inhibitor, has been proposed as a suitable option for restoring the efficacy of meropenem against organisms possessing these enzymes (Castanheira et al., 2017). However, it has no in vitro activity against Class B MBLs producers (NDM or VIM) or Class D OXA-48 β-lactamases (Castanheira et al., 2016; Nelson et al., 2017). The development of resistance to MER-VAB by CpKp is rarely documented. However, when it happens, it may be as a result of mutation in ompK35 and ompK36 or, mutation of kvrA which leads to downregulation of the ompK35/36 porin. Furthermore, when compared to CAZ/AVI, MER-VAB is less affected by KPC-2 mutations which confers resistance to CAZ/AVI. Base on this, it can be argued that MER-VAB offers the best therapeutic option available for CpKP.
Imipenem/cilastatin and relebactam therapy is one of the potent therapies against CpKP, and is made up of a combination of an approved carbapenem and a novel BLI. The chemical structure of relebactam is similar to that of avibactam (Watkins et al., 2013). The structure is made up of a diazabicyclooctane core, which under in vitro conditions, covalently and reversibly binds to Class A and C β-lactamases. Relebactam also possesses similar inhibitory mechanism as avibactam (Blizzard et al., 2014). Just like avibactam, relebactam cannot inhibit Class D OXA-48 (Petty et al., 2018; Zhanel et al., 2018).
Cefepime/zidebactam is one promising therapy which is made up of a combination of cefepime and the novel BLI, zidebactam. Unlike the other BLI, Zidebactam possess dual inhibitory mechanisms; it has high-affinity to penicillin binding protein 2 (PBP2) and also inhibit Ambler class A and C carbapenemase enzymes. When compared to the use of cefepime alone, the combination of zidebactam with cefepime has been shown to be a more effective therapy against CpKp (Livermore et al., 2017; Thomson et al., 2019). Other promising and emerging BL/BLI combination therapy against CpKP are Cefepime/taniborbactam, Imipenem/relebactam, Meropenem/nacubactam and Meropenem/QPX7728 combination.
Materials and Methods
This study followed the required guidelines and reporting procedures for Systematic Reviews and Meta-analysis (PRISMA) (Moher et al., 2009).
Search Strategy
The literature search was conducted in most English databases such as Medline through PubMed, Web of Science, Cochrane Library, EMBASE, and Google scholar. The search was carried out by three independent individuals. The keywords employed in the search were Klebsiella pneumoniae*, carbapenem-producing*, antibiotic regimens*, clinical outcomes*, mortality rate*, clinical success*, microbiological success*, carbapenem resistance*, VIM-producing*, IMP-producing*, NDM-producing*, Metallo beta lactamase*, OXA*, KPC*, CRE* and the search was restricted to studies published between the years 2015–2020.
Inclusion and Exclusion Strategy
For a study to meet the inclusion criteria of this study, it should have reported the outcomes among CpKP infected patients following antibiotic regimes. Prospective and retrospective randomized-controlled trials (RCTs), cohort and case control studies as well as case series and case reports were included. Studies were originally screened for eligibility in this review based on their titles and abstracts. Eligible studies were chosen in three steps: first, on the basis of the title, then on the basis of the abstract, and finally, on the basis of the full-text publication. Studies were deemed suitable for this work if clinical outcomes such as clinical response, microbiological response and mortality had been reported; if the phenotypic detection of the carbapenemase genes was done by methods such as Etest, or doubledisk synergy test based on the guidelines provided by the Clinical and Laboratory Standards Institute, 2015; and if molecular methods such as PCR were used in the confirmation and detection of the carbapenemase gene. Studies that were excluded from this study were, conference proceedings, editorials, duplicate publications, animal and in vitro studies.
Data Extraction Methods
For this meta-analysis, the following data were extracted from the various studies; the name of the first author, the type of Study, the year of publication, the country where the study was conducted, the study period, sample size, study characteristics (such as the site of infection, age of patients, comorbidities, etc), the susceptibility breakpoints standards used and clinical outcomes (such as mortality, clinical success, microbiological success).
Study Quality Assessment
Three reviewers assessed the quality of the studies base on the quality assessment scale. Any disagreements among the reviewers were resolved through dialogue. For this meta-analysis, the validity of the non-randomized studies was assessed with the Newcastle-Ottawa Scale (NOS) for non-randomized studies (Wells, 2004); for a study to be included, a score of 5 or more was needed.
Definitions and Outcomes
The definitions of outcomes that were used in this meta-analysis were those retrieved from individual studies. Crude mortality was defined as death by any cause evaluated at end of patient follow-up. Clinical success was defined as resolution of clinical signs and symptoms of the infections without relapse at the end of the antibiotic treatment. The microbiological success was defined as a culture negative for CpKP from subsequent specimen cultures. Different studies had different experimental durations. Generally, Clinical and microbiological outcome among studies was evaluated on D14 and D28 (some studies at the end of D30 or D90). Microbiological success was defined as a culture negative at D14 and/or D28. Follow-up also vary among studies and in some studies this was possible until discharge from the hospital or death. Mortality was evaluated at the end of the follow-up period (i.e. either D14, D28, D30 or D90).
Statistical Analysis
For the overall microbiological response, clinical success and mortality estimation, the Freeman-Tukey double arcsine transformed proportions and the random-effects (DerSimonian-Laird) model (Borenstein et al., 2009) were used. Following antibiotic therapies, the treatment outcomes were compared and the results were presented as odds ratios [OR]. Subgroup and sensitivity analyses were performed according to the various treatment regimens. Q test was used to evaluate between-study heterogeneity. The reliability of pooled assessments was evaluated via the leave-1-out sensitivity analyzes, and a study was considered relevant when the pooled result without that study, was beyond the 95% confidence interval [CI] of the overall pooled estimate. The degree of heterogeneity was quantified by I2 with the significance level set at 0.05. A high I2 value (>50%) indicated heterogeneity. If heterogeneity was not present (I2 < 50%), a fixed-effect model was applied for analysis; otherwise, a random effect model was adopted. The visualization approach of the funnel plots was utilized in the assessment of publication bias whilst the Egger’s test was used to quantify this bias. Statistical analyses were performed using the R studio 2.15.2 statistical software program (metaphor and meta Package, Version 2) by random-effects (DerSimonian and Laird) model.
Results
Systematic Literature Review and Study Characteristics
After an extensive literature search, 1,156 studies were initially retrieved. After record evaluation and assessment for duplicates, 367 references were excluded. Six hundred and forty-two (642) studies were further eliminated after the study titles and abstracts were evaluated. The Full-text assessment was carried out on 147 studies, of which 126 studies were further eliminated. The primary reasons for rejection were that the articles were conference proceedings (38), editorials (18), animal studies (31) and in vitro studies (39). Twenty-one (21) studies met the inclusion criteria set for this meta-analysis. The Prisma flow chat (Figure 1) gives a detailed screening and selection procedure of this study.
Table 1 describes and summarizes the basic characteristics of the studies included in this meta-analysis. These studies were made up of 2841 patients who had been infected by CpKP. The average age reported in these studies ranges from 46 to 69years. Comorbidities associated with these patients were urinary tract infections, bloodstream infections, pneumonia, diabetes, cancer, chronic renal failure and cardiovascular disease among others. Also, the bloodstream, lungs, urinary tract, skin and soft tissues were the common sites of infection. Based on the Newcastle-Ottawa scale for nonrandomized trials scores of the included studies, studies were rated as having good quality (Table 2).
Quantitative Synthesis
Overall Outcomes
The overall mortality rate from 15 studies involving 2716 patients that had received different antibiotic regimes was 32.2% (95%CI = 26.23–38.87; I2 = 89%; p-value ≤ 0.01) (Figure 2). Pooled clinical success was estimated in 9 studies involving 171 CpKP infected patients treated with various antibiotic regimes (Figure 3). The overall clinical success rate was 67.6% (95%CI = 58.35–75.64, I2 = 22%, p-value = 0.25) as compared to the microbiological success rate of 74.9% (95%CI = 59.02–86.09, I2 = 53%, p-value = 0.05) which was realized from 7 studies involving 121 CpKP infected patients that have been treated with different antibiotic regimen (Figure 4). From Figure 5, the resistant rate of CpKP isolates varied across the different types of antibiotics. The resistant rates were Colistin (47.6%), Gentamycin (63.1%), Tigecycline (25.7%), Fosfomycin (37.9%), Ertapenem (99.7%) and Meropenem (99.7%).
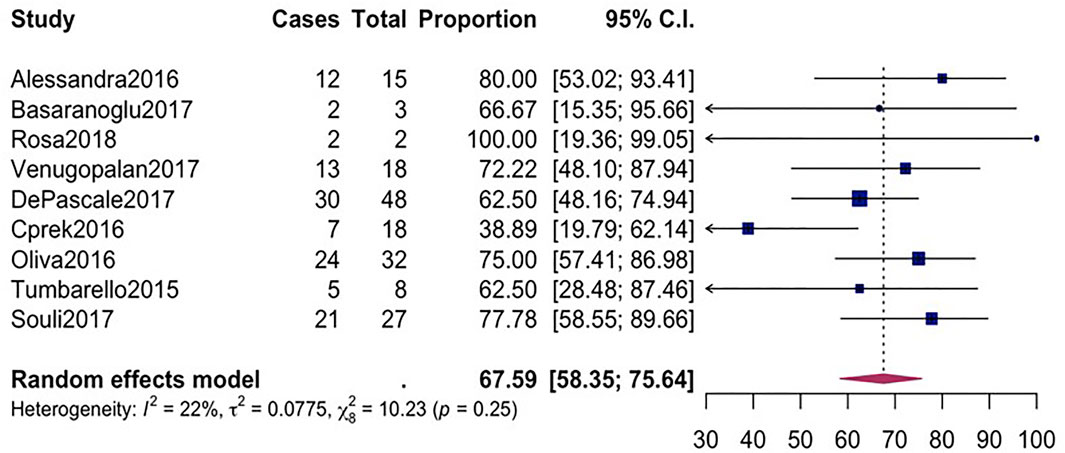
FIGURE 3. Forest plot for pooled clinical success among CpKP infected patients after antibiotic regimes.
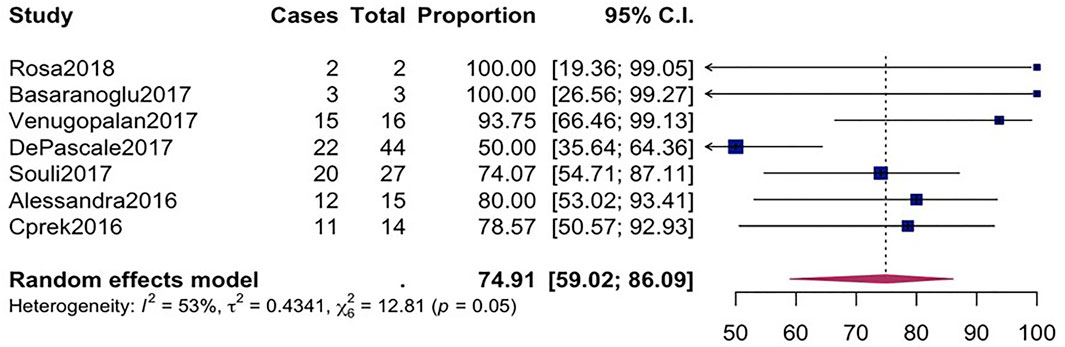
FIGURE 4. Forest plot for pooled microbiological success among CpKP infected patients after antibiotic.
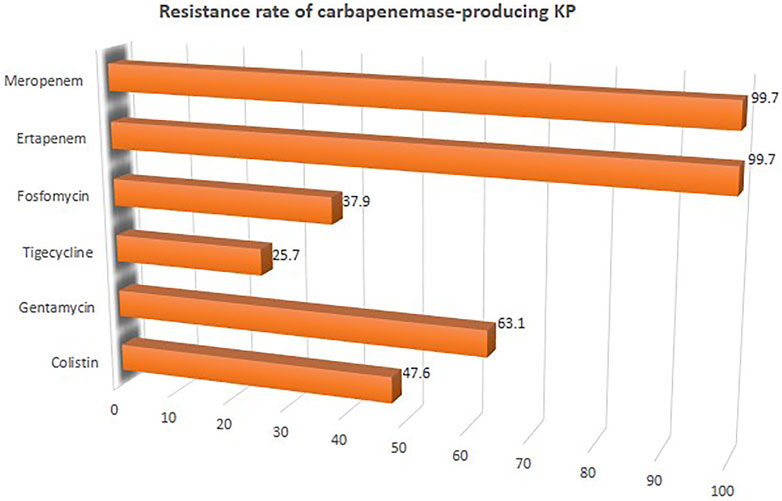
FIGURE 5. Resistant rate of CpKP to commonly used antibiotics in the treatment of CpKP infections. Colistin (n = 178), Gentamycin (n = 236), Tigecycline (n = 96), Fosfomycin (n = 89), Ertapenem (n = 373), Meropenem (n = 373).
Subgroup Analysis on Mortality Outcomes
Subgroup analysis on mortality was performed to ascertain the mortality rate of CpKP-infected patients. The following subgroup analyses were performed on patients: 1) Treated with combination therapy vs monotherapy 2) Among patients who are 60 years and beyond vs. less than 60 years 3) Who received triple combination therapy vs. double combination therapy 4) Among multicenter studies vs single center studies 5) Among studies published between 2015–2016 vs. studies published in 2017 and beyond 6) Among studies conducted in Europe vs others and 7) Among prospective studies vs retrospective studies (Table 3). Also various mortality outcomes associated with specific antibiotic class were analyzed. There is a significant difference between the mortality rate among CpKP patients treated with monotherapy and those treated with combination therapy. Patients treated with combination therapy had reduced death rate (OR = 0.55, 95%CI = 0.35–0.87, p-value = 0.01, 7 studies, Number of patients = 1,475). No significant difference existed between the mortality rate among the following subgroups: 60years and above patients vs below 60years (OR = 0.84, 95%CI = 0.28–2.57, p-value = 0.76, 6 studies, Number of patients = 1,688), triple therapy vs double therapy (OR = 0.50, 95%CI = 0.21–1.22, p-value = 0.13, 2 studies, Number of patients = 102), multicenter vs single center studies (OR = 1.01, 95%CI = 0.47–2.16, p-value = 0.98, 7 studies, Number of patients = 2404), studies published in 2017 and beyond vs studies published below 2017 (OR = 0.70, 95%CI = 0.33–1.47, p-value = 0.35, 8 studies, Number of patients = 2406) and prospective vs retrospective studies (OR = 0.71, 95%CI = 0.55–1.08, p-value = 0.13, 5 studies, Number of patients = 1706). Comparing studies conducted in Europe to those conducted elsewhere, it was realized that those in Europe had lower mortality as compared to those conducted elsewhere (OR = 0.50, 95%CI = 0.34–0.74, p-value ≤ 0.01, 7 studies, Number of patients = 1899). Comparing the mortality rates among the various antimicrobial class revealed that, apart from carbapenem vs aminoglycosides (OR = 1.37, 95%CI = 0.74–2.98, p-value = 0.02, 7 studies, Number of patients = 1,310) there were no significant difference between the mortality rates of CpKP patients treated with polymyxin vs tigecycline (OR = 0.89, 95%CI = 0.33–2.21, p-value = 0.67, 6 studies, Number of patients = 1,167), carbapenem vs. polymyxin (OR = 0.67, 95%CI = 0.46–1.66, p-value = 0.25, 4 studies, Number of patients = 860), and carbapenem vs tigecycline (OR = 0.98, 95%CI = 0.28–2.87, p-value = 0.78, 4 studies, Number of patients = 951) (Table 3). Comparing various combination therapies as seen in Table 3, it can be deduced that aminoglycosides-containing regimen significantly decreased the mortality rates among patients infected with CpKP as compared with patients treated with aminoglycosides-sparing regimen (OR = 0.86, 95%CI = 0.35–1.13, p-value = 0.04, 5 studies, Number of patients = 913).
Subgroup Analysis on Microbiological Outcomes
Because heterogeneity existed among the analysis conducted on the overall or pooled microbiological success, we performed subgroup analysis to check the source of the heterogeneity (Table 4). There is a significant difference between the microbiological success rates among CpKP patients treated with monotherapy compared to those treated with combination therapy. Patients treated with combination therapy had more success rate (OR = 3.78, 95%CI = 0.95 = 6–17.9, p-value= <0.001, 4 studies, Number of patients = 121). No significant difference existed between the microbiological success rate among the following subgroups: 60years and above patients vs below 60years (OR = 2.89, 95%CI = 0.57–7.13, p-value = 0.45, 2 studies, Number of patients = 96), triple therapy vs. double therapy (OR = 1.29, 95%CI = 0.89–2.96, p-value = 0.24, 2 studies, Number of patients = 85), multicenter vs single center studies (OR = 2.67, 95%CI = 0.34–1.46, p-value = 0.48, 5 studies, Number of patients = 136), and prospective vs. retrospective studies (OR = 0.71, 95%CI = 0.55–1.08, p-value = 0.13, 2 studies, Number of patients = 89). Comparing studies conducted in Europe to those conducted elsewhere, it was realized that those in Europe had higher microbiological success rate as compared to those conducted elsewhere (OR = 1.99, 95%CI = 0.18–2.84, p-value = 0.03, 3 studies, Number of patients = 102). Similar to the mortality rate, the microbiological success rates were significantly different among patients treated with carbapenem vs aminoglycosides (OR = 2.07, 95%CI = 0.84–9.41, p-value = 0.06, 2 studies, Number of patients = 84) but non-significant among those treated with polymyxin vs tigecycline (OR = 3.45, 95%CI = 0.93–9.64, p-value = 0.84, 3 studies, Number of patients = 103) (Table 4). Combination therapies that contain aminoglycosides significantly increased the microbiological success rates among patients infected with CpKP as compared with CpKP patients who were treated with combination therapies which did not contain aminoglycosides (OR = 2.98, 95%CI = 0.98–9.13, p-value = 0.03, 3 studies, Number of patients = 109).
Publication Bias and Sensitivity Analysis
The publication bias of the eligible papers used in our study was determined using Begg’s funnel and Egger’s test. Based on the symmetrical funnel plot for the various outcomes (Figure 6), it is evident that our results were not influenced by selection bias. Furthermore, the Egger quantitative test revealed that there was no major bias among our eligible studies, since all the pooled outcomes had p-values greater than 0.05 (p = 0.8203 for pooled mortality; p = 0.5942 for clinical success; and p = 0.189 for microbiological success). Analysis of the sensitivity or reliability of pooled estimates was then evaluated via leave-1-out sensitivity analysis. The statistical significance of the findings did not change when a specific study was omitted, demonstrating the validity and reliability of our research findings.
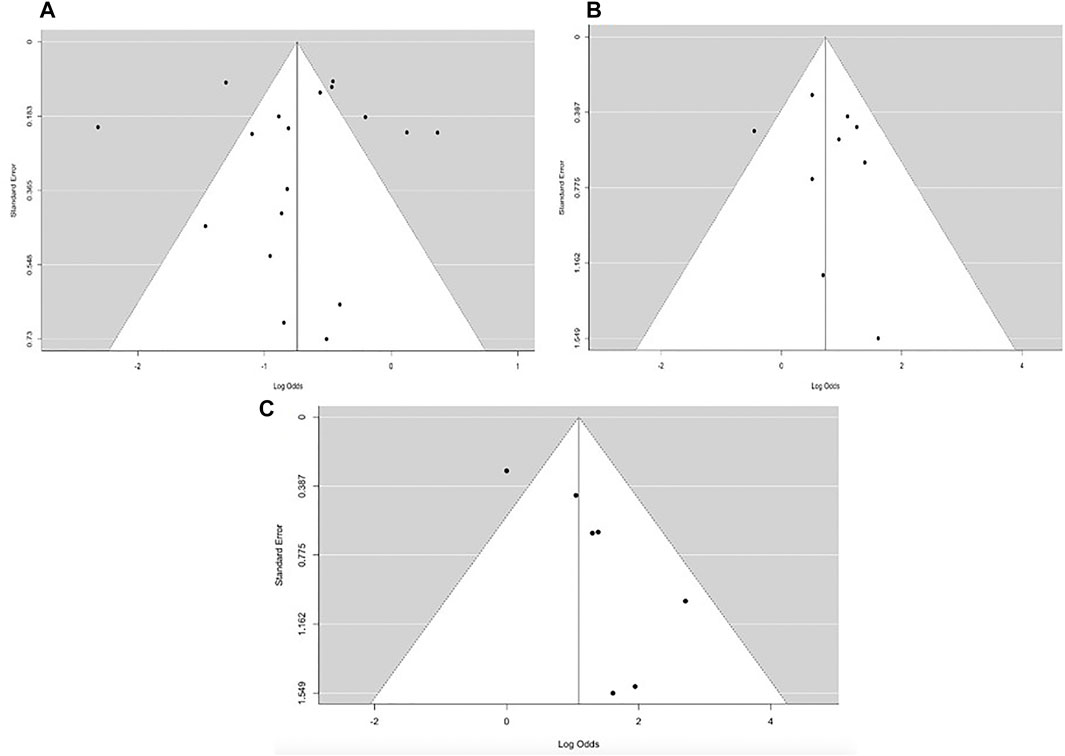
FIGURE 6. Funnel plot for overall (A) mortality rate (B) clinical success and (C) microbiological response.
Discussion
When clinicians and infection control agencies are faced with a sudden rise in infections that are caused by new antibiotic-resistant organisms such as CpKP, they turn to ask these two important questions: 1) whether there is an increase in the clinical and economic burden of the infection which is cause by this resistant organism relative to its susceptible counterpart. Knowledge on this will inform various decisions such as healthcare resource allocation, infection management and the control of these infections 2) The second question is whether treatment regimen data from clinical studies, are needed to maximize treatment-related outcomes. This particular question is very important when patients are infected with CpKP.
In this study, it was observed that tigecycline, fosfomycin and colistin recorded the highest susceptibility rate against CpKP as compared to the carbapenems which recorded almost 100% susceptibility. Bratu et al. (2005) reported that KPC-producing K. pneumoniae isolates are resistant to not only β-lactam antimicrobials but also aminoglycosides and fluoroquinolones. “The few remaining options are colistin, tigecycline, fosfomycin, and gentamicin, which usually harbor high MIC values, and share a suboptimal pharmacokinetic profile in terms of distribution at many infection sites (Gutiérrez-Gutiérrez et al., 2017; Poulakou et al., 2017).” Nonetheless, due to the risk of renal toxicity, the use of colistin and gentamycin has been limited. KPC-encoding blaKPC gene and other drug-resistant genes carried by K. pneumoniae may lead to a pronounced drug resistant (Villegas et al., 2007). Drug resistance has been noted to be associated with increased mortality because patients tend to receive inappropriate empiric therapies (Tumbarello et al., 2006; Cordery et al., 2008). The High crude or pooled mortality rate realized in this study shows that CpKP has stronger invasiveness.
There was a high clinical failure as compared to higher microbiological success in this meta-analysis. The reduced clinical success realized in this study, despite a high microbiological success, reemphasizes that other clinical factors, rather than just the antibiotics (either in combination or singly), may influence treatment results. The high overall 14 days through to 90 days mortality rate (32%) observed in this meta-analysis is a cause for concern. Although the mortality rate in this study is high, it is less when compared with the results of Xu et al. (2017), who reported a mortality rate of 42.14% among infections caused by carbapenem-resistant K. pneumoniae. Because most of the CpKP infected patients had other comorbidities, the results obtained from the pooled mortality rate may be overestimated. Also, there may be other factors which may have contributed to this high mortality. These are: the increase risk of renal and hepatic toxicity through the use of certain medications and the increasing use of antibiotics that belong to the Polymyxin family, may have contributed to patients’ mortality (Aguayo et al., 2016); increased virulence of carbapenemase-producing strains and the fact that the use of antibiotic regimes might, in turn, increase resistance to these same medications.
The lower mortality rate associated with combination therapy as compared to monotherapy is in line with previous findings (Tzouvelekis et al., 2014; Trecarichi et al., 2016). This may be as a result of the synergistic benefit that comes with the use of combination therapies during bacterial elimination and also the ability of combination therapies to provide a broad spectra of antibacterial activity especially during polymicrobial infections. The synergistic effects of combination therapies against CpKP have been confirmed in a lot of in vitro studies (Poirel et al., 2016; Oliva et al., 2017). In a study by Moher et al. (2009), the synergistic activity of combination therapies on CpKP was evaluated. During Severe infections caused by CpKP, it has been recommended to use combination treatment with more than one active agent (Tumbarello et al., 2012a; Qureshi et al., 2012; Tzouvelekis et al., 2012). In a study by Tumbarello et al. (2012a), it was reported that patients who received monotherapy had a mortality rate of 54% as compared to patients who received combination therapy with the best result been obtained from those who received triple combination therapy (Tumbarello et al., 2012b). Daikos et al. (2014) reported similar findings in a study of 205 patients with Bloodstream infections caused by KPC- or VIM-producing K. pneumoniae.
There was no significant difference in the mortality rates when patients received double and triple antibiotic combination therapies. This result suggests that before a combination therapy would be used, there must be a broader consultation and the decision must be guided by clinical projections (e.g. comorbidity of patient, pattern of antimicrobial susceptibility, site of infection, and disease severity). It is also important to consider any possible benefits derived from increasing the number of medications, along with the likelihood of increased adverse effects associated with these antibiotics (Tamma et al., 2012). In this meta-analysis, studies conducted in Europe had lower mortality as compared with studies conducted in other continents. This may be attributable to the advance medical care and the use of different treatment strategies such as the use of double and triple antibiotic regimes, the treatment of infections with tigecycline and polymyxins, and also the use of various adjunctive procedures (e.g., catheter removal, drainage, or debridement).
Because of the high rate of resistance, poor clinical outcomes, and the fact that new drugs to effectively treat these pathogens are several years away, antimicrobial stewardship programmes must be implemented immediately at both the national (country base) and regional levels of the world to ensure the best possible patient outcomes and to preserve antimicrobials for future use. Antimicrobial stewardship programmes, among other things, can optimize antimicrobial usage, enhance patient outcomes, minimize antimicrobial resistance and health-care-associated infections, and can also reduce health-care costs. In the management of major CpKP infections, clinicians should always consult a local infectious disease expert, and the treatment should always be based on antibiotic susceptibility test and the degree of the illness. In the management, consider empiric and antibiogram-directed combination therapy for patients who are critically unwell or have deep-seated infections, but one should be mindful of the toxicities of these drugs. Also, there is a need for new, efficient and rapid detection technologies for rapid identification and detection of resistance genes such as those of the carbapenemases. This will lead to rapid identification of carbapenemase producers, allowing adequate antibiotic stewardship. This will also help prevent the development of nosocomial outbreaks caused by CpKP. The rapid detection of carbapenemase producers may also have a significant impact in preventing their spread in the community, thereby reducing the use and misuse of antimicrobials.
The strengths of this meta-analysis are that, only one strain of a particular organism was used during our search and also because we employed a high sensitive search strategy. Sensitivity analysis by repeatedly eliminating one test at a time revealed that the findings of this study were consistent, thus symbolizing the reliability of the study. The reliability and generalizability of our results was more established because of the inclusion of studies from Europe, Asia, and the Americas. Some limitations of this study are that: subgroup analysis based on clinical success and gender were not conducted due to insufficient availability of primary studies data. Also, some included studies were of only moderate quality. Majority of studies used retrospective design and therefore may be prone to selection bias. It was not possible to provide a targeted account taking into consideration all confounders, which restricts the capacity of this meta-analysis to reliably establish a causal relationship.
Conclusion
In conclusion, there was high overall mortality and the mortality observed in CpKP-infected patients receiving combination therapy is lesser than that of those receiving monotherapy. Also, CpKP infected patients in Europe are less likely to die when compared with their counterparts in other continents. However, because most of the CpKP-infected patients had other comorbidities, the results obtained from the pooled mortality rate and the subgroup analysis may have been overestimated and one should be careful when drawing concrete conclusions from this. The choice of empirical treatment for patients with CpKP infections should be based on past treatment regimen and the antimicrobial resistant profiles of this organism in the hospital setting. Despite the advantages that combination therapies have over monotherapies, before a combination therapy would be used, there must be a broader consultation and the decision must be guided by clinical projections (e.g. comorbidity of patient, pattern of antimicrobial susceptibility, site of infection, and disease severity). New effective therapies are urgently needed to help fight infections caused by this organism. The effective use of various therapeutic options and the strict implementation of infection control measures (Poulou et al., 2012) are of utmost importance in order to prevent infections caused by CpKP. Strict national or international implementation of infection control measures and treatment guidelines will help improve healthcare, and equip governments and communities to respond to and prevent the spread of infectious diseases caused by CpKP.
Data Availability Statement
The raw data supporting the conclusion of this article will be made available by the authors, without undue reservation.
Author Contributions
CE contributed to the conceptualization of the work, data retrieval and analysis; ED, CA, SL, EN, TS, LM, JW, and ZX contributed to the data retrieval and analysis. YW and XZ contributed to the conceptualization, fund sourcing and supervision of the work. All authors read and approved the final manuscript.
Funding
This study was supported by the National Natural Science Foundation of China (No. 81573203, 81973099).
Conflict of Interest
The authors declare that the research was conducted in the absence of any commercial or financial relationships that could be construed as a potential conflict of interest.
Publisher’s Note
All claims expressed in this article are solely those of the authors and do not necessarily represent those of their affiliated organizations, or those of the publisher, the editors and the reviewers. Any product that may be evaluated in this article, or claim that may be made by its manufacturer, is not guaranteed or endorsed by the publisher.
References
Aguayo, A., Mella, S., Riedel, G., Bello, H., Domínguez, M., and González-Rocha, G. (2016). Colistín en la era post-antibiótica. Rev. Chil. Infectol. 33 (2), 166–176. doi:10.4067/s0716-10182016000200006
Akajagbor, D. S., Wilson, S. L., Shere-Wolfe, K. D., Dakum, P., Charurat, M. E., and Gilliam, B. L. (2013). Higher Incidence of Acute Kidney Injury with Intravenous Colistimethate Sodium Compared with Polymyxin B in Critically Ill Patients at a Tertiary Care Medical center. Clin. Infect. Dis. 57 (9), 1300–1303. doi:10.1093/cid/cit453
Alexandra, O., Scorzolini, L., Castaldi, D., Gizzi, F., De Angelis, M., Storto, M., et al. (2016). Double-Carbapenem Regimen, Alone or in Combination With Colistin, in the Treatment of Infections Caused by Carbapenem-Resistant Klebsiella Pneumoniae (CR-Kp). J. Infect. 74 (1), 103–106. doi:10.1016/j.jinf.2016.10.002
Balandin Moreno, B., Fernández Simón, I., Pintado García, V., Sánchez Romero, I., Isidoro Fernández, B., Romera Ortega, M. A., et al. (2014). Tigecycline Therapy for Infections Due to Carbapenemase-Producing Klebsiella pneumoniae in Critically Ill Patients. Scand. J. Infect. Dis. 46, 175–180. doi:10.3109/00365548.2013.861608
Beigverdi, R., Jabalameli, L., Jabalameli, F., and Emaneini, M. (2019). Prevalence of Extended-Spectrum β-lactamase-producing Klebsiella pneumoniae: First Systematic Review and Meta-Analysis from Iran. J. Glob. Antimicrob. Resist. 18, 12–21. doi:10.1016/j.jgar.2019.01.020
Berrazeg, M., Diene, S., Medjahed, L., Parola, P., Drissi, M., Raoult, D., et al. (2014). New Delhi Metallo-Beta-Lactamase Around the World: an eReview Using Google Maps. Euro Surveill. 19 (20), pii=20809. doi:10.2807/1560-7917.es2014.19.20.20809
Blizzard, T. A., Chen, H., Kim, S., Wu, J., Bodner, R., Gude, C., et al. (2014). Discovery of MK-7655, a β-lactamase Inhibitor for Combination with Primaxin®. Bioorg. Med. Chem. Lett. 24, 780–785. doi:10.1016/j.bmcl.2013.12.101
Borenstein, M., Hedges, L. V., Higgins, J. P., and Rothstein, H. R. (2009). Introduction to Meta-Analysis. John Wiley and Sons, 69–75. doi:10.1002/9780470743386
Bratu, S., Landman, D., Haag, R., Recco, R., Eramo, A., Alam, M., et al. (2005). Rapid Spread of Carbapenem-Resistant Klebsiella pneumoniae in New York City: a New Threat to Our Antibiotic Armamentarium. Arch. Intern. Med. 165 (12), 1430–1435. doi:10.1001/archinte.165.12.1430
Bulik, C. C., and Nicolau, D. P. (2011). Double-carbapenem Therapy for Carbapenemase-Producing Klebsiella pneumoniae. Antimicrob. Agents Chemother. 55, 3002–3004. doi:10.1128/AAC.01420-10
Cantón, R., Akóva, M., Carmeli, Y., Giske, C. G., Glupczynski, Y., Gniadkowski, M., et al. (2012). Rapid Evolution and Spread of Carbapenemases Among Enterobacteriaceae in Europe. Clin. Microbiol. Infect. 18, 413–431. doi:10.1111/j.1469-0691.2012.03821.x
Castanheira, M., Huband, M. D., Mendes, R. E., and Flamm, R. K. (2017). Meropenem-vaborbactam Tested against Contemporary Gram-Negative Isolates Collected Worldwide during 2014, Including Carbapenem-Resistant, KPC-Producing, Multidrug-Resistant, and Extensively Drug-Resistant Enterobacteriaceae. Antimicrob. Agents Chemother. 61, e00567–17. doi:10.1128/AAC.00567-17
Castanheira, M., Rhomberg, P. R., Flamm, R. K., and Jones, R. N. (2016). Effect of the β-Lactamase Inhibitor Vaborbactam Combined with Meropenem against Serine Carbapenemase-Producing Enterobacteriaceae. Antimicrob. Agents Chemother. 60, 5454–5458. doi:10.1128/AAC.00711-16
Castanheira, M., Sader, H. S., and Jones, R. N. (2010). Antimicrobial Susceptibility Patterns of KPC-Producing or CTX-M-Producing Enterobacteriaceae. Microb. Drug Resist. 16, 61–65. doi:10.1089/mdr.2009.0031
Ceccarelli, G., Falcone, M., Giordano, A., Mezzatesta, M. L., Caio, C., Stefani, S., et al. (2013). Successful Ertapenem-Doripenem Combination Treatment of Bacteremic Ventilator-Associated Pneumonia Due to Colistin-Resistant KPC-Producing Klebsiella pneumoniae. Antimicrob. Agents Chemother. 57, 2900–2901. doi:10.1128/AAC.00188-13
Chang, L. W., Buising, K. L., Jeremiah, C. J., Cronin, K., Poy Lorenzo, Y. S., Howden, B. P., et al. (2015). Managing a Nosocomial Outbreak of Carbapenem-Resistant Klebsiella pneumoniae: an Early Australian Hospital Experience. Intern. Med. J. 45 (10), 1037–1043. doi:10.1111/imj.12863
Clinical and Laboratory Standards Institute (2015). Performance Standards for Antimicrobial Susceptibility Testing; Twenty-Fifth Informational Supplement. CLSI Document M100-S25. Wayne, PA: CLSI.
Cordery, R. J., Roberts, C. H., Cooper, S. J., Bellinghan, G., and Shetty, N. (2008). Evaluation of Risk Factors for the Acquisition of Bloodstream Infections with Extended-Spectrum Beta-Lactamase-Producing Escherichia coli and Klebsiella Species in the Intensive Care Unit; Antibiotic Management and Clinical Outcome. J. Hosp. Infect. 68 (2), 108–115. doi:10.1016/j.jhin.2007.10.011
Cprek, J. B., and Gallagher, J. C. (2016). Ertapenem-containing Double-Carbapenem Therapy for Treatment of Infections Caused by Carbapenem-Resistant Klebsiella pneumoniae. Antimicrob. Agents Chemother. 60 (1), 669–673. doi:10.1128/AAC.01569-15
Cuzon, G., Naas, T., Truong, H., Villegas, M. V., Wisell, K. T., Carmeli, Y., et al. (2010). Worldwide Diversity of Klebsiella pneumoniae that Produce Beta-Lactamase blaKPC-2 Gene. Emerg. Infect. Dis. 16, 1349–1356. doi:10.3201/eid1609.091389
Daikos, G. L., Tsaousi, S., Tzouvelekis, L. S., Anyfantis, I., Psichogiou, M., Argyropoulou, A., et al. (2014). Carbapenemase-producing Klebsiella pneumoniae Bloodstream Infections: Lowering Mortality by Antibiotic Combination Schemes and the Role of Carbapenems. Antimicrob. Agents Chemother. 58, 2322–2328. doi:10.1128/AAC.02166-13
Day, K. M., Salman, M., Kazi, B., Sidjabat, H. E., Silvey, A., Lanyon, C. V., et al. (2013). Prevalence of NDM-1 Carbapenemase in Patients with Diarrhoea in Pakistan and Evaluation of Two Chromogenic Culture media. J. Appl. Microbiol. 114, 1810–1816. doi:10.1111/jam.12171
de Jonge, B. L., Karlowsky, J. A., Kazmierczak, K. M., Biedenbach, D. J., Sahm, D. F., and Nichols, W. W. (2016). In Vitro susceptibility to Ceftazidime-Avibactam of Carbapenem-Nonsusceptible Enterobacteriaceae Isolates Collected during the INFORM Global Surveillance Study (2012 to 2014). Antimicrob. Agents Chemother. 60, 3163–3169. doi:10.1128/AAC.03042-15
De Oliveira, M. S., De Assis, D. B., Freire, M. P., Boas do Prado, G. V., Machado, A. S., Abdala, E., et al. (2015). Treatment of KPC-Producing Enterobacteriaceae: Suboptimal Efficacy of Polymyxins. Clin. Microbiol. Infect. 21 (2), 179. doi:10.1016/j.cmi.2014.07.010
De Pascale, G., Martucci, G., Montini, L., Panarello, G., Cutuli, S. L., Di Carlo, D., et al. (2017). Double Carbapenem as a rescue Strategy for the Treatment of Severe Carbapenemase-Producing Klebsiella pneumoniae Infections: a two-center, Matched Case-Control Study. Crit. Care 21 (1), 173. doi:10.1186/s13054-017-1769-z
Effah, C. Y., Sun, T., Liu, S., and Wu, Y. (2020). Klebsiella pneumoniae: an Increasing Threat to Public Health. Ann. Clin. Microbiol. Antimicrob. 19 (1), 1–9. doi:10.1186/s12941-019-0343-8
European Antimicrobial Resistance Surveillance Network (EARS-Net) (2017). Surveillance of Antimicrobial Resistance in Europe – Annual Report. Available at: https://ecdc.europa.eu/sites/portal/files/documents/AMR-surveillance-EARS-Net-2017.pdf (Accessed October, 2021).
Florescu, D. F., Qiu, F., McCartan, M. A., Mindru, C., Fey, P. D., and Kalil, A. C. (2012). What Is the Efficacy and Safety of Colistin for the Treatment of Ventilator-Associated Pneumonia? A Systematic Review and Meta-Regression. Clin. Infect. Dis. 54 (5), 670–680. doi:10.1093/cid/cir934
Freire, M. P., Abdala, E., Moura, M. L., de Paula, F. J., Spadão, F., Caiaffa-Filho, H. H., et al. (2015). Risk Factors and Outcome of Infections with Klebsiella pneumoniae Carbapenemase-Producing K. pneumoniae in Kidney Transplant Recipients. Infection 43 (3), 315–323. doi:10.1007/s15010-015-0743-4
Giamarellou, H., Galani, L., Baziaka, F., and Karaiskos, I. (2013). Effectiveness of a Double-Carbapenem Regimen for Infections in Humans Due to Carbapenemase-Producing Pandrug-Resistant Klebsiella pneumoniae. Antimicrob. Agents Chemother. 57, 2388–2390. doi:10.1128/AAC.02399-12
Giannella, M., Trecarichi, E. M., Giacobbe, D. R., De Rosa, F. G., Bassetti, M., Bartoloni, A., et al. (2018). Effect of Combination Therapy Containing a High-Dose Carbapenem on Mortality in Patients with Carbapenem-Resistant Klebsiella pneumoniae Bloodstream Infection. Int. J. Antimicrob. Agents 51 (2), 244–248. doi:10.1016/j.ijantimicag.2017.08.019
Girlich, D., Bouihat, N., Poirel, L., Benouda, A., and Nordmann, P. (2014). High Rate of Faecal Carriage of Extended-Spectrum β-lactamase and OXA-48 Carbapenemase-Producing Enterobacteriaceae at a university Hospital in Morocco. Clin. Microbiol. Infect. 20, 350–354. doi:10.1111/1469-0691.12325
Gonzalez-Padilla, M., Torre-Cisneros, J., Rivera-Espinar, F., Pontes-Moreno, A., López-Cerero, L., Pascual, A., et al. (2015). Gentamicin Therapy for Sepsis Due to Carbapenem-Resistant and Colistin-Resistant Klebsiella pneumoniae. J. Antimicrob. Chemother. 70 (3), 905–913. doi:10.1093/jac/dku432
Gregory, M. S., Rigatto, M. H., Falci, D. R., and Zavascki, A. P. (2019). Combination Therapy With Polymyxin B for Carbapenemase-Producing Klebsiella Pneumoniae Bloodstream Infection. Int. J. Antimicrob. Agents 53 (2), 152–157. doi:10.1016/j.ijantimicag.2018.10.010
Guimarães, T., Nouér, S. A., Martins, R. C. R., Perdigão Neto, L. V., Martins, W. M. B. S., Narciso Barbosa, A. C., et al. (2019). Ceftazidime-avibactam as Salvage Therapy for Infections Caused by Enterobacteriales Coresistant to Carbapenems and Polymyxins. Antimicrob. Agents Chemother. 63 (10), e00528–19. doi:10.1128/AAC.00528-19
Gutiérrez-Gutiérrez, B., Salamanca, E., de Cueto, M., Hsueh, P. R., Viale, P., Paño-Pardo, J. R., et al. (2017). Effect of Appropriate Combination Therapy on Mortality of Patients with Bloodstream Infections Due to Carbapenemase-Producing Enterobacteriaceae (INCREMENT): a Retrospective Cohort Study. Lancet Infect. Dis. 17 (7), 726–734. doi:10.1016/S1473-3099(17)30228-1
Hackel, M. A., Tsuji, M., Yamano, Y., Echols, R., Karlowsky, J. A., and Sahm, D. F. (2018). In Vitro activity of the Siderophore Cephalosporin, Cefiderocol, against Carbapenem-Nonsusceptible and Multidrug-Resistant Isolates of Gram-Negative Bacilli Collected Worldwide in 2014 to 2016. Antimicrob. Agents Chemother. 62, e01968–17. doi:10.1128/AAC.01968-17
Hirsch, E. B., and Tam, V. H. (2010). Detection and Treatment Options for Klebsiella pneumoniae Carbapenemases (KPCs): an Emerging Cause of Multidrug-Resistant Infection. J. Antimicrob. Chemother. 65, 1119–1125. doi:10.1093/jac/dkq108
Ito, A., Nishikawa, T., Matsumoto, S., Yoshizawa, H., Sato, T., Nakamura, R., et al. (2016). Siderophore Cephalosporin Cefiderocol Utilizes Ferric Iron Transporter Systems for Antibacterial Activity against Pseudomonas aeruginosa. Antimicrob. Agents Chemother. 60, 7396–7401. doi:10.1128/AAC.01405-16
Jacob, J. T., Klein, E., Laxminarayan, R., Beldavs, Z., Lynfield, R., Kallen, A. J., et al. (2013). Vital Signs: Carbapenem-Resistant Enterobacteriaceae. MMWR Morb Mortal Wkly Rep. 62 (9), 165–170.
Johnson, A. P., and Woodford, N. (2013). Global Spread of Antibiotic Resistance: the Example of New Delhi Metallo-β-Lactamase (NDM)-mediated Carbapenem Resistance. J. Med. Microbiol. 62, 499–513. doi:10.1099/jmm.0.052555-0
Kaye, K. S., and Pogue, J. M. (2015). Infections Caused by Resistant Gram-Negative Bacteria: Epidemiology and Management. Pharmacotherapy 35, 949–962. doi:10.1002/phar.1636
Lee, J., Patel, G., Huprikar, S., Calfee, D. P., and Jenkins, S. G. (2009). Decreased Susceptibility to Polymyxin B during Treatment for Carbapenem-Resistant Klebsiella pneumoniae Infection. J. Clin. Microbiol. 47, 1611–1612. doi:10.1128/JCM.02466-08
Levy Hara, G., Gould, I., Endimiani, A., Pardo, P. R., Daikos, G., Hsueh, P. R., et al. (2013). Detection, Treatment, and Prevention of Carbapenemase-Producing Enterobacteriaceae: Recommendations from an International Working Group. J. Chemother. 25, 129–140. doi:10.1179/1973947812Y.0000000062
Liao, Y., Hu, G. H., Xu, Y. F., Che, J. P., Luo, M., Zhang, H. M., et al. (2017). Retrospective Analysis of Fosfomycin Combinational Therapy for Sepsis Caused by Carbapenem-Resistant Klebsiella pneumoniae. Exp. Ther. Med. 13 (3), 1003–1010. doi:10.3892/etm.2017.4046
Lim, L. M., Ly, N., Anderson, D., Yang, J. C., Macander, L., Jarkowski, A., et al. (2010). Resurgence of Colistin: a Review of Resistance, Toxicity, Pharmacodynamics, and Dosing. Pharmacotherapy 30 (12), 1279–1291. doi:10.1592/phco.30.12.1279
Livermore, D. M., Mushtaq, S., Warner, M., Vickers, A., and Woodford, N. (2017). In Vitro activity of Cefepime/zidebactam (WCK 5222) against Gram-Negative Bacteria. J. Antimicrob. Chemother. 72, 1373–1385. doi:10.1093/jac/dkw593
Livermore, D. M., Mushtaq, S., Warner, M., Zhang, J. C., Maharjan, S., Doumith, M., et al. (2011). Activity of Aminoglycosides, Including ACHN-490, against Carbapenem-Resistant Enterobacteriaceae Isolates. J. Antimicrob. Chemother. 66, 48–53. doi:10.1093/jac/dkq408
Machuca, I., Gutiérrez-Gutiérrez, B., Gracia-Ahufinger, I., Rivera Espinar, F., Cano, Á., Guzmán-Puche, J., et al. (2017). Mortality Associated with Bacteremia Due to Colistin-Resistant Klebsiella pneumoniae with High-Level Meropenem Resistance: Importance of Combination Therapy without Colistin and Carbapenems. Antimicrob. Agents Chemother. 61 (8), e00406. doi:10.1128/AAC.00406-17
Moher, D., Liberati, A., Tetzlaff, J., Altman, D. G., Altman, D., Antes, G., et al. (2009). Preferred Reporting Items for Systematic Reviews and Meta-Analyses: the PRISMA Statement. Plos Med. 6 (9), e1000097–896. doi:10.1371/journal.pmed.1000097
Munoz-Price, L. S., Poirel, L., Bonomo, R. A., Schwaber, M. J., Daikos, G. L., Cormican, M., et al. (2013). Clinical Epidemiology of the Global Expansion of Klebsiella pneumoniae Carbapenemases. Lancet Infect. Dis. 13, 785–796. doi:10.1016/S1473-3099(13)70190-7
Navon-Venezia, S., Kondratyeva, K., and Carattoli, A. (2017). Klebsiella pneumoniae: a Major Worldwide Source and Shuttle for Antibiotic Resistance. FEMS Microbiol. Rev. 41 (3), 252–275. doi:10.1093/femsre/fux013
Nelson, K., Hemarajata, P., Sun, D., Rubio-Aparicio, D., Tsivkovski, R., Yang, S., et al. (2017). Resistance to Ceftazidime-Avibactam Is Due to Transposition of KPC in a Porin-Deficient Strain of Klebsiella pneumoniae with Increased Efflux Activity. Antimicrob. Agents Chemother. 61, e00989–17. doi:10.1128/AAC.00989-17
Oliva, A., Gizzi, F., Mascellino, M. T., Cipolla, A., D'Abramo, A., D'Agostino, C., et al. (2016). Bactericidal and Synergistic Activity of Double-Carbapenem Regimen for Infections Caused by Carbapenemase-Producing Klebsiella pneumoniae. Clin. Microbiol. Infect. 22 (2), 147–153. doi:10.1016/j.cmi.2015.09.014
Oliva, A., Scorzolini, L., Cipolla, A., Mascellino, M. T., Cancelli, F., Castaldi, D., et al. (2017). In Vitro evaluation of Different Antimicrobial Combinations against Carbapenemase-Producing Klebsiella pneumoniae: the Activity of the Double-Carbapenem Regimen Is Related to Meropenem MIC Value. J. Antimicrob. Chemother. 72 (7), 1981–1984. doi:10.1093/jac/dkx084
Osorio, J., Barreto, J., Samboni, C. F., Cándelo, L. A., Álvarez, L. C., Benavidez, S., et al. (2017). Factores asociados a nefrotoxicidad por polimixina B en un hospital universitario de Neiva, Colombia. 2011-2015. Rev. Chil. Infectol. 34 (1), 7–13. doi:10.4067/s0716-10182017000100001
Papadimitriou-Olivgeris, M., Fligou, F., Bartzavali, C., Zotou, A., Spyropoulou, A., Koutsileou, K., et al. (2017). Carbapenemase-producing Klebsiella pneumoniae Bloodstream Infection in Critically Ill Patients: Risk Factors and Predictors of Mortality. Eur. J. Clin. Microbiol. Infect. Dis. 36 (7), 1125–1131. doi:10.1007/s10096-017-2899-6
Paterson, D. L., and Yu, V. L. (1999). Extended-spectrum Beta-Lactamases: a Call for Improved Detection and Control. Clin. Infect. Dis. 29 (6), 1419–1422. doi:10.1086/313559
Petty, L. A., Henig, O., Patel, T. S., Pogue, J. M., and Kaye, K. S. (2018). Overview of Meropenem-Vaborbactam and Newer Antimicrobial Agents for the Treatment of Carbapenem-Resistant Enterobacteriaceae. Infect. Drug Resist. 11, 1461–1472. doi:10.2147/IDR.S150447
Pitout, J. D., and Laupland, K. B. (2008). Extended-spectrum Beta-Lactamase-Producing Enterobacteriaceae: an Emerging Public-Health Concern. Lancet Infect. Dis. 8 (3), 159–166. doi:10.1016/S1473-3099(08)70041-0
Podschun, R., and Ullmann, U. (1998). Klebsiella Spp. As Nosocomial Pathogens: Epidemiology, Taxonomy, Typing Methods, and Pathogenicity Factors. Clin. Microbiol. Rev. 11 (4), 589–603. doi:10.1128/CMR.11.4.589
Poirel, L., Dortet, L., Bernabeu, S., and Nordmann, P. (2011). Genetic Features of blaNDM-1-Positive Enterobacteriaceae. Antimicrob. Agents Chemother. 55, 5403–5407. doi:10.1128/AAC.00585-11
Poirel, L., Kieffer, N., and Nordmann, P. (2016). In Vitro evaluation of Dual Carbapenem Combinations against Carbapenemase-Producing Enterobacteriaceae. J. Antimicrob. Chemother. 71 (1), 156–161. doi:10.1093/jac/dkv294
Poulakou, G., Matthaiou, D. K., Bassetti, M., Erdem, H., Dimopoulos, G., Curcio, D. J., et al. (2017). "Salvage Treatment" for Infections by Extensively- and Pan-Drug-Resistant Pathogens Is Common and Often Sub-optimal. Intensive Care Med. 43 (8), 1164–1166. doi:10.1007/s00134-017-4796-y
Poulou, A., Voulgari, E., Vrioni, G., Xidopoulos, G., Pliagkos, A., Chatzipantazi, V., et al. (2012). Imported Klebsiella pneumoniae Carbapenemase-Producing K. pneumoniae Clones in a Greek Hospital: Impact of Infection Control Measures for Restraining Their Dissemination. J. Clin. Microbiol. 50 (8), 2618–2623. doi:10.1128/JCM.00459-12
Qureshi, Z. A., Paterson, D. L., Potoski, B. A., Kilayko, M. C., Sandovsky, G., Sordillo, E., et al. (2012). Treatment Outcome of Bacteremia Due to KPC-Producing Klebsiella pneumoniae: Superiority of Combination Antimicrobial Regimens. Antimicrob. Agents Chemother. 56 (4), 2108–2113. doi:10.1128/AAC.06268-11
Rodríguez-Baño, J., Gutiérrez-Gutiérrez, B., Machuca, I., and Pascual, A. (2018). Treatment of Infections Caused by Extended-Spectrum-Beta-Lactamase-, AmpC-, and Carbapenemase-Producing Enterobacteriaceae. Clin. Microbiol. Rev. 31 (2), e00079–17. doi:10.1128/CMR.00079-17
Rosa, R., Rudin, S. D., Rojas, L. J., Hujer, A. M., Perez-Cardona, A., Perez, F., et al. (2018). “Double Carbapenem” and Oral Fosfomycin for the Treatment of Complicated Urinary Tract Infections Caused by blaNDM -Harboring Enterobacteriaceae in Kidney Transplantation.. Transpl. Infect Dis. 20 (1). doi:10.1111/tid.12795
Saisho, Y., Katsube, T., White, S., Fukase, H., and Shimada, J. (2018). Pharmacokinetics, Safety, and Tolerability of Cefiderocol, a Novel Siderophore Cephalosporin for Gram-Negative Bacteria, in Healthy Subjects. Antimicrob. Agents Chemother. 62, e02163–17. doi:10.1128/AAC.02163-17
Satlin, M. J., Kubin, C. J., Blumenthal, J. S., Cohen, A. B., Furuya, E. Y., Wilson, S. J., et al. (2011). Comparative Effectiveness of Aminoglycosides, Polymyxin B, and Tigecycline for Clearance of Carbapenem-Resistant Klebsiella pneumoniae from Urine. Antimicrob. Agents Chemother. 55, 5893–5899. doi:10.1128/AAC.00387-11
Sbrana, F., Malacarne, P., Viaggi, B., Costanzo, S., Leonetti, P., Leonildi, A., et al. (2013). Carbapenem-sparing Antibiotic Regimens for Infections Caused by Klebsiella pneumoniae Carbapenemase-Producing K. pneumoniae in Intensive Care Unit. Clin. Infect. Dis. 56, 697–700. doi:10.1093/cid/cis969
Shaw, E., Rombauts, A., Tubau, F., Padullés, A., Càmara, J., Lozano, T., et al. (2018). Clinical Outcomes after Combination Treatment with Ceftazidime/avibactam and Aztreonam for NDM-1/oxa-48/ctx-M-15-Producing Klebsiella pneumoniae Infection. J. Antimicrob. Chemother. 73 (4), 1104–1106. doi:10.1093/jac/dkx496
Souli, M., Galani, I., Antoniadou, A., Papadomichelakis, E., Poulakou, G., Panagea, T., et al. (2010). An Outbreak of Infection Due to Beta-Lactamase Klebsiella pneumoniae Carbapenemase 2-producing K. pneumoniae in a Greek University Hospital: Molecular Characterization, Epidemiology, and Outcomes. Clin. Infect. Dis. 50, 364–373. doi:10.1086/649865
Souli, M., Karaiskos, I., Masgala, A., Galani, L., Barmpouti, E., and Giamarellou, H. (2017). Double-carbapenem Combination as Salvage Therapy for Untreatable Infections by KPC-2-Producing Klebsiella pneumoniae. Eur. J. Clin. Microbiol. Infect. Dis. 36, 1305–1315. doi:10.1007/s10096-017-2936-5
Syue, L. S., Chen, Y. H., Ko, W. C., and Hsueh, P. R. (2016). New Drugs for the Treatment of Complicated Intra-abdominal Infections in the Era of Increasing Antimicrobial Resistance. Int. J. Antimicrob. Agents 47, 250–258. doi:10.1016/j.ijantimicag.2015.12.021
Tamma, P. D., Cosgrove, S. E., and Maragakis, L. L. (2012). Combination Therapy for Treatment of Infections with Gram-Negative Bacteria. Clin. Microbiol. Rev. 25 (3), 450–470. doi:10.1128/CMR.05041-11
Tanır Basaranoglu, S., Ozsurekci, Y., Aykac, K., Arıkan, K. O., Buyukcam, A., Cengiz, A. B., et al. (2017). Add-on Therapy with Ertapenem in Infections with Multidrug Resistant Gram-Negative Bacteria: Pediatric Experience. Case Rep. Infect. Dis. 2017, 8096420. doi:10.1155/2017/8096420
Thomson, K., AbdelGhani, S., Snyder, J., and Thomson, G. (2019). Activity of Cefepime-Zidebactam against Multidrug-Resistant (MDR) Gram-Negative Pathogens. Antibiotics 8, 32. doi:10.3390/antibiotics8010032
Trecarichi, E. M., Pagano, L., Martino, B., Candoni, A., Di Blasi, R., Nadali, G., et al. (2016). Bloodstream Infections Caused by Klebsiella pneumoniae in Onco-Hematological Patients: Clinical Impact of Carbapenem Resistance in a Multicentre Prospective Survey. Am. J. Hematol. 91 (11), 1076–1081. doi:10.1002/ajh.24489
Tumbarello, M., Spanu, T., Sanguinetti, M., Citton, R., Montuori, E., Leone, F., et al. (2006). Bloodstream Infections Caused by Extended-Spectrum-Beta-Lactamase-Producing Klebsiella pneumoniae: Risk Factors, Molecular Epidemiology, and Clinical Outcome. Antimicrob. Agents Chemother. 50 (2), 498–504. doi:10.1128/AAC.50.2.498-504.2006
Tumbarello, M., Trecarichi, E. M., Corona, A., De Rosa, F. G., Bassetti, M., Mussini, C., et al. (2019). Efficacy of Ceftazidime-Avibactam Salvage Therapy in Patients with Infections Caused by Klebsiella pneumoniae Carbapenemase-Producing K. pneumoniae. Clin. Infect. Dis. 68 (3), 355–364. doi:10.1093/cid/ciy492
Tumbarello, M., Trecarichi, E. M., De Rosa, F. G., Giannella, M., Giacobbe, D. R., Bassetti, M., et al. (2015a). Infections Caused by KPC-Producing Klebsiella pneumoniae: Differences in Therapy and Mortality in a Multicentre Study. J. Antimicrob. Chemother. 70 (7), 2133–2143. doi:10.1093/jac/dkv086
Tumbarello, M., Viale, P., Bassetti, M., De Rosa, F. G., Spanu, T., and Viscoli, C. (2015b). Infections Caused by KPC-Producing Klebsiella pneumoniae: Differences in Therapy and Mortality in a Multicentre Study-Aauthors' Response. J. Antimicrob. Chemother. 70 (10), 2922. doi:10.1093/jac/dkv200
Tumbarello, M., Viale, P., Viscoli, C., Trecarichi, E. M., Tumietto, F., Marchese, A., et al. (2012a). Predictors of Mortality in Bloodstream Infections Caused by Klebsiella pneumoniae Carbapenemase-Producing K. pneumoniae: Importance of Combination Therapy. Clin. Infect. Dis. 55, 943–950. doi:10.1093/cid/cis588
Tumbarello, M., Viale, P., Viscoli, C., Trecarichi, E. M., Tumietto, F., Marchese, A., et al. (2012b). Predictors of Mortality in Bloodstream Infections Caused by Klebsiella pneumoniae Carbapenemase-Producing K. pneumoniae: Importance of Combination Therapy. Clin. Infect. Dis. 55 (7), 943–950. doi:10.1093/cid/cis588
Tzouvelekis, L. S., Markogiannakis, A., Piperaki, E., Souli, M., and Daikos, G. L. (2014). Treating Infections Caused by Carbapenemase-Producing Enterobacteriaceae. Clin. Microbiol. Infect. 20, 862–872. doi:10.1111/1469-0691.12697
Tzouvelekis, L. S., Markogiannakis, A., Psichogiou, M., Tassios, P. T., and Daikos, G. L. (2012). Carbapenemases in Klebsiella pneumoniae and Other Enterobacteriaceae: an Evolving Crisis of Global Dimensions. Clin. Microbiol. Rev. 25 (4), 682–707. doi:10.1128/CMR.05035-11
Venugopalan, V., Nogid, B., Le, T. N., Rahman, S. M., and Bias, T. E. (2017). Double Carbapenem Therapy (DCT) for Bacteremia Due to Carbapenem-Resistant Klebsiella pneumoniae (CRKP): from Test Tube to Clinical Practice. Infect. Dis. (Lond) 49 (11-12), 867–870. doi:10.1080/23744235.2017.1350880
Villegas, M. V., Lolans, K., Correa, A., Kattan, J. N., Lopez, J. A., and Quinn, J. P. (2007). First Identification of Pseudomonas aeruginosa Isolates Producing a KPC-type Carbapenem-Hydrolyzing Beta-Lactamase. Antimicrob. Agents Chemother. 51 (4), 1553–1555. doi:10.1128/AAC.01405-06
Watkins, R. R., Papp-Wallace, K. M., Drawz, S. M., and Bonomo, R. A. (2013). Novel β-lactamase Inhibitors: a Therapeutic hope against the Scourge of Multidrug Resistance. Front. Microbiol. 4, 392. doi:10.3389/fmicb.2013.00392
Wells, G. A. S. B. (2004). The Newcastle-Ottawa Scale (NOS) for Assessing the Quality of Nonrandomised Studies in Meta-Analysis.
Wiskirchen, D. E., Crandon, J. L., and Nicolau, D. P. (2013). Impact of Various Conditions on the Efficacy of Dual Carbapenem Therapy against KPC-Producing Klebsiella pneumoniae. Int. J. Antimicrob. Agents 41, 582–585. doi:10.1016/j.ijantimicag.2013.02.015
Wong, D., and van Duin, D. (2017). Novel Beta-Lactamase Inhibitors: Unlocking Their Potential in Therapy. Drugs 77, 615–628. doi:10.1007/s40265-017-0725-1
World Health Organization (2017). Global Priority List of Antibiotic-Resistant Bacteria to Guide Research, Discovery, and Development of New Antibiotics. Geneva: WHO.
Wright, H., Bonomo, R. A., and Paterson, D. L. (2017). New Agents for the Treatment of Infections with Gram-Negative Bacteria: Restoring the Miracle or False Dawn? Clin. Microbiol. Infect. 23, 704–712. doi:10.1016/j.cmi.2017.09.001
Xu, L., Sun, X., and Ma, X. (2017). Systematic Review and Meta-Analysis of Mortality of Patients Infected with Carbapenem-Resistant Klebsiella pneumoniae. Ann. Clin. Microbiol. Antimicrob. 16 (1), 18–12. doi:10.1186/s12941-017-0191-3
Yigit, H., Queenan, A. M., Anderson, G. J., Domenech-Sanchez, A., Biddle, J. W., Steward, C. D., et al. (2001). Novel Carbapenem-Hydrolyzing Beta-Lactamase, KPC-1, from a Carbapenem-Resistant Strain of Klebsiella pneumoniae. Antimicrob. Agents Chemother. 45 (4), 1151–1161. doi:10.1128/AAC.45.4.1151-1161.2001
Zarkotou, O., Pournaras, S., Tselioti, P., Dragoumanos, V., Pitiriga, V., Ranellou, K., et al. (2011). Predictors of Mortality in Patients with Bloodstream Infections Caused by KPC-Producing Klebsiella pneumoniae and Impact of Appropriate Antimicrobial Treatment. Clin. Microbiol. Infect. 17 (12), 1798–1803. doi:10.1111/j.1469-0691.2011.03514.x
Zavascki, A. P., Bulitta, J. B., and Landersdorfer, C. B. (2013). Combination Therapy for Carbapenem-Resistant Gram-Negative Bacteria. Expert Rev. Anti Infect. Ther. 11, 1333–1353. doi:10.1586/14787210.2013.845523
Zhanel, G. G., Lawrence, C. K., Adam, H., Schweizer, F., Zelenitsky, S., Zhanel, M., et al. (2018). Imipenem-Relebactam and Meropenem-Vaborbactam: Two Novel Carbapenem-β-Lactamase Inhibitor Combinations. Drugs 78, 65–98. doi:10.1007/s40265-017-0851-9
Zuckerman, T., Benyamini, N., Sprecher, H., Fineman, R., Finkelstein, R., Rowe, J. M., et al. (2011). SCT in Patients with Carbapenem Resistant Klebsiella pneumoniae: a Single center Experience with Oral Gentamicin for the Eradication of Carrier State. Bone Marrow Transpl. 46 (9), 1226–1230. doi:10.1038/bmt.2010.279
Keywords: Klebsiella pneumoniae, carbapenemase-producing, clinical outcomes, meta-analysis, antibiotic regimen
Citation: Effah CY, Drokow EK, Agboyibor C, Liu S, Nuamah E, Sun T, Miao L, Wang J, Xu Z, Wu Y and Zhang X (2021) Evaluation of the Therapeutic Outcomes of Antibiotic Regimen Against Carbapenemase-Producing Klebsiella pneumoniae: A Systematic Review and Meta-Analysis. Front. Pharmacol. 12:597907. doi: 10.3389/fphar.2021.597907
Received: 24 August 2020; Accepted: 19 October 2021;
Published: 04 November 2021.
Edited by:
Joseph O Fadare, Ekiti State University, NigeriaReviewed by:
Brian Godman, University of Strathclyde, United KingdomMarc Henri De Longueville, UCB Pharma, Belgium
Copyright © 2021 Effah, Drokow, Agboyibor, Liu, Nuamah, Sun, Miao, Wang, Xu, Wu and Zhang. This is an open-access article distributed under the terms of the Creative Commons Attribution License (CC BY). The use, distribution or reproduction in other forums is permitted, provided the original author(s) and the copyright owner(s) are credited and that the original publication in this journal is cited, in accordance with accepted academic practice. No use, distribution or reproduction is permitted which does not comply with these terms.
*Correspondence: Yongjun Wu, wuyongjun135@126.com; Xiaoju Zhang, 15837101166@163.com