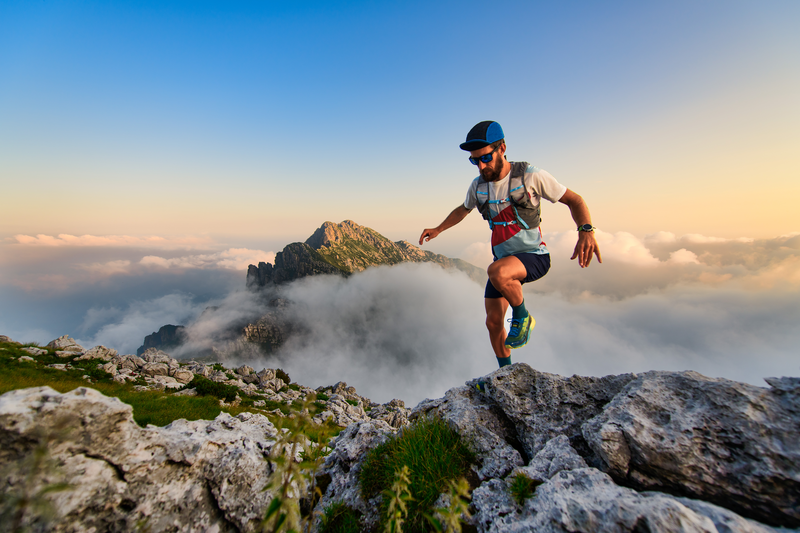
94% of researchers rate our articles as excellent or good
Learn more about the work of our research integrity team to safeguard the quality of each article we publish.
Find out more
ORIGINAL RESEARCH article
Front. Pharmacol. , 01 April 2021
Sec. Ethnopharmacology
Volume 12 - 2021 | https://doi.org/10.3389/fphar.2021.590602
This article is part of the Research Topic Integrative Pharmacology-based Research on Traditional Medicine: Methodologies, Medical and Pharmacological Applications View all 73 articles
Objectives: This study aimed to explore the mechanism of Xiong-Pi-Fang (XPF) in the treatment of coronary heart disease (CHD) with depression by an integrative strategy combining serum pharmacochemistry, network pharmacology analysis, and experimental validation.
Methods: An ultrahigh performance liquid chromatography-quadrupole-time-of-flight tandem mass spectrometry (UPLC-Q-TOF/MS) method was constructed to identify compounds in rat serum after oral administration of XPF, and a component-target network was established using Cytoscape, between the targets of XPF ingredients and CHD with depression. Furthermore, Gene Ontology and Kyoto Encyclopedia of Genes and Genomes pathway enrichment analyses were performed to deduce the mechanism of XPF in treating CHD with depression. Finally, in a chronic unpredictable mild stress (CUMS)-and isoproterenol (ISO)-induced rat model, TUNEL was used to detect the apoptosis index of the myocardium and hippocampus, ELISA and western blot were used to detect the predicted hub targets, namely AngII, 5-HT, cAMP, PKA, CREB, BDNF, Bcl-2, Bax, Cyt-c, and caspase-3.
Results: We identified 51 compounds in rat serum after oral administration of XPF, which mainly included phenolic acids, saponins, and flavonoids. Network pharmacology analysis revealed that XPF may regulate targets, such as ACE2, HTR1A, HTR2A, AKT1, PKIA, CREB1, BDNF, BCL2, BAX, CASP3, cAMP signaling pathway, and cell apoptosis process in the treatment of CHD with depression. ELISA analysis showed that XPF decreased Ang-II content in the circulation and central nervous system, inhibited 5-HT levels in peripheral circulation, and increased 5-HT content in the central nervous system and cAMP content in the myocardia and hippocampus. Meanwhile, western blot analysis indicated that XPF could upregulate the expression levels of PKA, CREB, and BDNF both in the myocardia and hippocampus. TUNEL staining indicated that the apoptosis index of myocardial and hippocampal cells increased in CUMS-and ISO-induced CHD in rats under depression, and XPF could increase the expression of Bcl-2, inhibit the expression of Bax, Cyt-c, and caspase-3, and rectify the injury of the hippocampus and myocardium, which exerted antidepressant and antimyocardial ischemia effects.
Conclusion: Our study proposed an integrated strategy, combining serum pharmacochemistry and network pharmacology to investigate the mechanisms of XPF in treating CHD with depression. The mechanism of XPF in treating CHD with depression may be related to the activation of the cAMP signaling pathway and the inhibition of the apoptosis.
Coronary heart disease (CHD) is a chronic and complex disease that poses a serious threat to human health (Mozaffarian et al., 2016; Blais et al., 2020). Traditional risk factors are mainly related to hypertension, hyperlipidemia, diabetes, etc (Li C et al., 2020). Moreover, depression is another independent risk factor for CHD, as it has been shown to diminish the quality of life of patients with CHD and also increase the incidence and mortality of major adverse cardiac events (Su et al., 2018; de Heer et al., 2020). Increasing evidence suggests that the incidence of CHD with depression is increasing, ranging from approximately 15–18%, moreover, approximately 31% of these patients develop major depressive disorder (MDD) (Carney and Freedland, 2017). However, there are no effective chemicals to improve the quality of life and survival rate of patients with comorbid CHD and depression (Lee et al., 2018; Magaard et al., 2018).
The ideal medication for CHD with depression would be integrated, improving myocardial blood supply and regulating nervous system function at the same time (Yeung et al., 2014; Chen M et al., 2018; Xue et al., 2019). Xiong-Pi-Fang (XPF), a classical traditional Chinese medicine (TCM) formula, consists of Radix Bupleuri (Bupleurum chinense DC. and Bupleurum scorzonerifolium Willd.) 15 g; Ligusticum wallichii (Conioselinum anthriscoides 'Chuanxiong' and Ligusticum striatum DC.) 12 g; Rhizoma Cyperi (Cyperus rotundus L.) 12 g; Sanders (Santalum album L.) 9 g; Fructus aurantii (Citrus × aurantium L. and Citrus trifoliata L.) 9 g; Dried tangerine peel (Citrus × aurantium L.) 15 g; Pinellia ternata (Pinellia ternata (Thunb.) Makino) 15 g; Herba Menthae (Mentha haplocalyx Briq.) 10 g; Perilla Stem (Perilla frutescens (L.) Britton) 15 g; Poria Cocos (Wolfiporia extensa (Peck) Ginns) 15 g; Rhizoma Atractylodis Macrocephalae (Atractylodes Macrocephala Koidz) 15 g; Radix Glycyrrhizae (Glycyrrhiza uralensis Fisch.) 9 g. It is widely used in clinical practices, showing satisfactory therapeutic effects in relieving angina pectoris, chest pain, and depression symptoms (Hou et al., 2017). However, it is difficult to fully understand the therapeutic mechanism of XPF for treating CHD with depression solely using traditional pharmacological methods. Hence, an integrated pharmacology-based strategy including computer high-throughput analytical techniques and biological experimental tools was established in this study, which provides a helpful method to reveal the potential bioactive ingredients and modern pharmacological mechanism of TCM (Park et al., 2018; Fang et al., 2019).
Integrative pharmacology, combined with conventional pharmacology, network pharmacology, bioinformatics, and other disciplines, is the systematic study of the overall interactions between drugs and humans at the molecular, cell, organ, and network levels (Hart and Xie, 2016; Zhang R. et al., 2018; Zhang R. et al., 2019). Network pharmacology, characterized by integration, systematization, and emphasis on drug interaction (Yuan et al., 2017), predicts the biological molecular mechanism of drug treatment of diseases from a holistic perspective by constructing an interaction network between active ingredients and disease targets. However, most TCM-related databases provide information on chemical ingredients extracted in vitro (Zhang W et al., 2019). Previous studies have shown that the drug ingredients absorbed into the blood circulation may be the main active constituents (Chen et al., 2014; Li et al., 2016). Therefore, it is critical to combine serum pharmacochemistry with a network pharmacology strategy to accurately establish the network.
In this study, an integrated pharmacology strategy employing serum pharmacochemistry, network pharmacological analysis, and experimental validation was conducted to illustrate the therapeutic mechanism of XPF in treating CHD with depression (Figure 1). Briefly, An ultrahigh performance liquid chromatography-quadrupole-time-of-flight tandem mass spectrometry (UPLC-Q-TOF/MS) method was established to determine the main active components of XPF in rat serum. Furthermore, a serum pharmacochemistry-based network was constructed and the component–target network between CHD comorbid depression-relevant genes and the targets of active components in XPF was established. Finally, we validated the predicted molecular mechanisms obtained from the network analysis of XPF in treating CHD with depression in a chronic unpredictable mild stress (CUMS)-and isoproterenol (ISO)-induced CHD in a rat model of depression.
Sertraline hydrochloride (Pfizer Inc., United States), metoprolol (Asilkan, United Kingdom), Isoproterenol (Sigma Aldrich Co., United States) were purchased from the Shanghai Yuanye Biotechnology Co. Ltd (Shanghai, China). UPLC-Q-TOF/MS grade acetonitrile and HPLC grade acetonitrile, methanol, formic acid, were provided by Fisher Scientific International Inc (Fair Lawn, NJ). The ELISA assay kits for Ang-Ⅱ (142191202), 5-HT (106191202), cAMP (202004) were purchased from Tianjin Zihan Biotechnology Co., Ltd. (Tianjin, China). TUNEL assay kit (7E310H9) was purchased from Vazyme Biotech Co., Ltd. (Nanjing, China), Rabbit antihuman monoclonal antibodies PKA (5842), CREB (6188), BDNF (3189), Bcl2 (26,593-1-12p), Bax (50,599–2-1 g), Cytochrome c (10993-1-Ap), and mouse antihuman monoclonal antibodies caspase-3 (66,470-E1g) and β-actin were provided by Proteintech Group, Inc. (Wuhan, China).
Male Sprague-Dawley rats (weighing 200 ± 20 g) were purchased from the Experimental Animal Center of the Academy of Military Medical Sciences (Beijing, China), and kept in an environmentally controlled room (temperature 22 ± 2°C, humidity 50 ± 10%) with food and water available. This study was carried out in accordance with the principles of the Basel Declaration and the guidelines of the National Institutes of Health. All animal experiments were approved by the Ethics Committee of Tianjin University of Traditional Chinese Medicine (Tianjin, China).
XPF was prepared by the pharmacy of the Second Affiliated Hospital of Tianjin University of Traditional Chinese Medicine (Tianjin, China). Twelve rats were randomly divided into a control group (n = 6, 0.9% saline IG), and According to the calculation method of equivalent dose coefficient of experimental animals in Methodology of Pharmacological Experiment (Xu et al., 2018), the XPF group (n = 6, 32.7 g/kg XPF IG), equivalent to twice the clinical effective dose, for three days (Xu et al., 1997). All rats fasted for 12 h before the experiment and then the serum samples were collected at 120 min after oral administration, via the postorbital venous plexus. The serum samples were centrifuged at 12,000 rpm for 10 min at 4°C. Then, 300 μL of serum was added to three times the amount of acetonitrile, vortexed for 2 min, followed by ultrasonic extraction for 10 min, and centrifugation (12,000 rpm, 10 min). and The supernatant was collected and placed in a N2 blower to blow dry at 40°C, 50% acetonitrile (50 μL) was added to the residue, vortexed for 3 min, ultrasound for 10 min, centrifugation (12,000 rpm, 10 min), and the supernatant was collected for UPLC-Q-TOF/MS analysis.
UPLC-Q-TOF/MS analysis was analyzed on a Waters ACQUITYTM UPLC BEH C18 (100 mm × 2.1 mm, 1.7 μm) system, maintained at 30 °C. The flow rate was set at 0.45 ml/min, and the injection volume was 5 µL. The mobile phase was consisted of water (A) and methanol (B) both containing 0.1% (v/v) formic acid for astragaloside (0–5 min, 2% B; 5–8 min, 2%–20% B, 8–11 min, 20% B, 11–14 min, 20%–48% B, 14–20 min, 48%–70% B, 20–23 min, 70%–90% B, 23–26 min, 90%–100% B, 26–30 min, 100% B). The mass spectrometer analysis was performed with reaction monitoring both in positive and negative ion modes.
All targets of active components of XPF determined by serum pharmacochemistry analysis were collected from TCM-related databases, including TCMSP (http://tcmspw.com/tcmsp.php) (Ru et al., 2014), DrugBank (https://www.drugbank.ca/) (Wishart et al., 2018), Swiss Target Prediction (http://www.swisstargetprediction.ch/) (Gfeller et al., 2014), and Similarity Ensemble Approach (http://sea.bkslab.org/) (Wang et al., 2016). Known therapeutic targets related to CHD and depression were obtained from the DrugBank database (Wishart et al., 2018), Online Mendelian Inheritance in Man (OMIM) (http://www.omim.org) (Amberger and Hamosh, 2017), DisGeNet database (http://www.disgenet.org/web/DisGeNET/menu/home) (Li Y et al., 2020), and Therapeutic Target database (TTD) (https://db.idrblab.org/ttd/) (Li et al., 2018). The common potential target of XPF in the treatment of CHD with depression was obtained by comparing the component target with the disease target analysis. Then, the component–target network between the targets of active components in XPF and CHD with depression was established using Cytoscape (Version 3.8.0) (Otasek et al., 2019).
A network analyzer was used to calculate the topological analysis of the nodes of the component–target network. Nodes with degrees higher than the average number 4) were identified as the core targets, and were brought into the STRING database (https://string-db.org/) (Szklarczyk et al., 2017) to obtain the interacting proteins. Then, the PPI network was constructed using Cytoscape for visual analysis and, according to topological features (degree centrality), the major hub target was extracted. Further, the core targets were brought into the DAVID database (https://david.ncifcrf.gov) (Dennis et al., 2003), and Kyoto Encyclopedia of Genes and Genomes (KEGG) signaling pathway enrichment analysis and Gene ontology (GO) were performed to obtain the representative biological processes and pathways (cutoff at p < 0.05) of XPF in treating CHD with depression.
Sixty-four male Sprague-Dawley rats (weighing 200 ± 20 g) were randomly divided into a normal control group (n = 8) and a depressive-like behavioral group (n = 56). Experimental rats (n = 56) were exposed to chronic unpredictable mild stress (CUMS) to induce depression. The regimen consisted of immobilization for 4 h; cage tilting at 45° for 12 h; continuous illumination for 24 h; clip the distal 1 cm rat tails with tongs for 3 min; deprivation of water for 24 h; noise stimulation for 30 min; damp animal bedding for 24 h; foot shock for 3 min; removal of animal bedding for 24 h; high-speed agitation for 10 min; deprivation of food 24 h; and forced cold swim stress for 6 min at 4°C. Each animal received two stressors randomly per day for a total of 28 days. The normal control group (n = 8) remained undisturbed. Subsequently, the CUMS-induced rats were injected with ISO (8 mg/kg) for five days to form the CHD model (Aa et al., 2019; Hu et al., 2020). The rat model for CHD with depression induced by CUMS and ISO was evaluated by measuring rat body weight change, open field test, sucrose preference test, and ST-segment of the electrocardiogram. Then, all CHD with depression rats were randomly divided into a model group (n = 15, 0.9% saline, IG), Ser + Met group (n = 8, sertraline 10 mg/kg + metoprolol 5 mg/kg, IG), Ser group (n = 8, sertraline 10 mg/kg, IG), Met group (n = 8, metoprolol 10 mg/kg, IG), high-dose XPF (XPF-H) group (n = 8, 32.7 g/kg, IG), equivalent to CH 3.13 g/kg, CX 2.5 g/kg, XF 2.5 g/kg, TX 1.88 g/kg, ZQ 3.13 g/kg, CP 3.13 g/kg, BX 3.13 g/kg, BH 2.08 g/kg, FL 3.13 g/kg, BZ 3.13 g/kg, ZS 3.13 g/kg, GC 3.13 g/kg, medium-dose XPF (XPF-M) group (n = 8, 16.35 g/kg, IG), equivalent to CH 1.65 g/kg, CX 1.25 g/kg, XF 1.25 g/kg, TX 0.94 g/kg, ZQ 1.65 g/kg, CP 1.65 g/kg, BX 1.65 g/kg, BH 1.04 g/kg, FL 1.65 g/kg, BZ 1.65 g/kg, ZS 1.65 g/kg, GC 0.94 g/kg, and low-dose XPF (XPF-L) group (n = 8, 8.175 g/kg, IG), equivalent to CH 0.78 g/kg, CX 0.62 g/kg, XF 0.63 g/kg, TX 0.47 g/kg, ZQ 0.78 g/kg, CP 0.78 g/kg, BX 0.78 g/kg, BH 0.52 g/kg, FL 0.78 g/kg, BZ 0.78 g/kg, ZS 0.78 g/kg, GC 0.47 g/kg. The control group received 0.9% saline via oral administration. The drugs were administered daily for three weeks, while the animals were exposed to CUMS, except for the control group. At 24 h after the last treatment, all rats were sacrificed, and the brain, hippocampus, and heart tissues were rapidly extracted from each rat, stored at −80°C until analysis. One part of the brain and heart tissues were placed into a flask containing 4% paraformaldehyde.
TUNEL staining was performed according to the manufacturer’s instructions, to determine hippocampus and myocardial apoptosis. The apoptotic cells showed red fluorescence and the nucleus showed blue fluorescence, six high-power fields were selected from each sample. All cells and positive stained cells were counted, the percentage of positively stained cells was apoptotic index (AI) (AI = number of apoptotic cells/total number of nucleated cells).
The level of 5-HT, Ang-Ⅱ, cAMP in hippocampus and myocardial were quantified by ELISA assay kit, according to the manufacturer's instruction. After color development, the absorbance was measured at 450 nm with fluorescence reader (THERMO USA).
Hippocampus, hearts tissue proteins were mechanically homogenized in lysis buffer, centrifuged at 12,000 rpm for 10 min at 4 °C, collected the supernatant. BCA protein assay was used to determine protein concentrations. Equal concentrations of protein were resolved on 10% SDS-PAGE gels, and were transferred onto PVDF membranes. These membranes PVDF were soaked in TBST buffer with 5% non-fat skim milk for 2 h, and then These membranes PVDF were incubated in the primary antibodies (PKA, CREB, BDNF, Bcl-2, Bax, Cyt-c, caspase-3, and β-actin) overnight at 4°C. Subsequently, the membrane was washed for 5 times with TBST, followed by secondary antibodies incubated with horseradish peroxidase for 2 h at room temperature. After rewashing with TBST, the membranes were scanned on X-ray film by chemiluminescence reaction, used ImageJ software to analysis the band intensity.
Data were expressed as mean ± SD, Differences between groups were analyzed using a one-way analysis of variance (ANOVA), followed by Dunnett’s t test. All data were analyzed statistically using GraphPad Prism 8.0 (GraphPad Software, Inc., La Jolla, CA, USA), p-value < 0.05 was considered significant.
Based on the established UPLC-Q-TOF/MS method, 51 serum prototypes of the drugs were analyzed and identified (Table 1). These compounds can be roughly divided into three categories: phenolic acids, saponins, and flavonoids. Figure 2 shows the base peak chromatogram (BPC) of each typical sample in the mode of positive and negative ions, and peaks 1–51 are the original components entering the blood. These 51 compounds of XPF detected in the serum were determined to be the main active components and further selected to predict the targets and pathways using network analysis.
FIGURE 2. BPI chromatograms of XPF in serum sample in positive (A) and negative. (B) Ion modes determined by UPLC-Q-TOF/MS.
In this work, 820 targets for the 51 (Nine of them had no therapeutic targets) components were explored by using TCMSP, Swiss Target Prediction, Drugbank, and Swiss Target Prediction as shown in Supplementary Table S1, and 1817 candidate targets of CHD and depression were obtained from Drugbank, OMIM, and DisGeNet databases as shown in Supplementary Table S2. Taking the intersection of component targets and candidate targets associated with CHD and depression, 168 consensus targets were generated as potential targets for XPF in treating CHD and depression, which were used to construct a component–target network using Cytoscape. As shown in Figure 3A, the network comprised 42 components, 168 targets, and two diseases, and included 212 nodes and 845 edges.
FIGURE 3. The networks associating with XPF in the treatment of CHD with depression. (A) The component-target network consisted of 42 components, 168 targets and 2 diseases including 212 nodes and 845 edges. (B) The PPI network of hub targets obtained from STRING database and constructed by Cytoscape.
Among the 168 consensus targets, 75 major targets with degrees higher than the average number 4) were considered as the major targets (Table 2). Ten components with degrees higher than 20 were identified as the main active components (Kaempferol and quercetin were not included because they are widely distributed and have many targets, but their pharmacological effects are weak) (Table 3). The 75 major targets were brought into the STRING database to obtain protein-protein interaction (PPI) predictions. The PPI network was constructed using Cytoscape, and hide disconnected nodes in the network (5 nodes). A network analyzer was used to calculate topological features (degree) of the 70 major targets (Figure 3B). Among these, 10 target genes (ACE2, HTR1A, HTR2A, AKT1, PKIA, CREB1, BDNF, BCL2, BAX, and CASP3), with higher degree were recognized as the major hub targets of XPF in treating CHD with depression. KEGG pathway enrichment and GO analyses of the 75 major targets were performed using the DAVID database. GO analysis showed 184 enriched processes (Supplementary Table S3), including 126 biological processes, 39 molecular functions, and 19 cellular components. The top 30 according to p-values are shown in Figure 4A. KEGG pathway enrichment showed 117 signaling pathways, as shown in Supplementary Table S4, and the top 20 pathways according to p-values are shown in Figure 4B.
FIGURE 4. GO and KEGG analysis of the candidate targets. (A) Biological process enrichment of candidate targets. (B) KEGG pathwayenrichment of candidate targets.
The open field test can reflect the spatial exploration behavior of rats (Chen et al., 2019), and sucrose preference test could be the objective indicator of hedonic behavior (Gross and Pinhasov, 2016b). All rats were evaluated by open field test, sucrose preference test before and after the CUMS and ISO-induced process, then all CHD with depression rats were randomly divided into 7 groups. After three weeks of administration, open field test and sucrose preference test were performed again of all rats. As shown in Table 4, after CUMS and ISO-induced, compared with the control group the open-field test and sucrose preference scores were significantly reduced in the model group and each administration groups (p < 0.005). It was shown that the depression model was successfully established. After treatment for 21 days, compared with the model group, XPF administration significantly improve the open-field test and sucrose preference scores (p< 0.005).
TABLE 4. The comparison of the open field test scores and sucrose preference of rats in different groups.
Apoptosis can cause myocardial and hippocampal injury and dysfunction, which are the major mechanisms of CHD and depression. As shown in Figure 5, the myocardial and hippocampus of the CUMS and ISO-induced rats were injured. The effects of XPF on myocardial apoptosis are shown in Figure 5A. And the effects of XPF on hippocampal apoptosis are shown in Figure 5B. Compared with the control group, the myocardial and TUNEL-positive cells were significantly increased in the model group, as shown in Figure 5C (p < 0.005). The myocardial apoptotic index of the XPF-H and XPF-M groups was significantly lower than that of the model group (p < 0.01). Sertraline 10 mg/kg + metoprolol 5 mg/kg and metoprolol 10 mg/kg also significantly inhibited the apoptosis in the myocardia (Figure 5C) (p < 0.005). Sertraline (10 mg/kg) and low-dose XPF treatment did not alter myocardial apoptosis.
FIGURE 5. Anti-apoptosis effect of XPF on CUMS and ISO-induced rats (×400). (A) Representative images of myocardial apoptosis stained by TUNEL. (B) Representative images of hippocampal apoptosis stained by TUNEL. (C) Rate of myocardial apoptosis in each group. (D) Rate of hippocampal apoptosis in each group. Data wer presented as means ± SD (n = 3). ***p < 0.05 compared with the control group, ##p < 0.01, ##p < 0.005 compared with the model group.
There were significant differences in the total apoptotic index in the hippocampus after XPF treatment for 21 days. In the model group, the apoptotic index significantly increased compared with the control group, as shown in Figure 5D. Furthermore, XPF administration significantly decreased the apoptotic index in the hippocampus. Additionally, sertraline and metoprolol also significantly inhibited apoptosis in the hippocampus (p < 0.005).
The results indicated that the brain and myocardial tissues of the rats were injured after CUMS and ISO-induced damage. It was shown that the CHD with depression model was successfully established, while the treatment of XPF can inhibit apoptosis of myocardial and brain tissue simultaneously.
According to key proteins with a higher degree in the PPI network and KEGG pathway enrichment analysis, it is suggested that the mechanism of XPF in the treatment of CHD with depression may be through the regulation of ACE2, HTR1A, HTR2A, AKT1, PKIA, CREB1, BDNF, BCL2, BAX, CASP3, cAMP signaling pathway, and the cell apoptosis process. As the second intracellular messenger, cAMP regulates the expression of downstream proteins and plays an important role in cell growth and apoptosis (Zhou et al., 2012). Bcl-2 and Bax, as targets downstream of CREB and BDNF, regulate apoptosis through the mitochondrial pathway (Mi et al., 2015; Tang et al., 2016). Recent studies have shown that the cAMP-PKA-CREB-BDNF signaling pathway is closely related to depression (Wang et al., 2017; Ye et al., 2017). Meanwhile, the related proteins in the cAMP-PKA-CREB-BDNF signaling pathway can also improve myocardial microcirculation, improve the ability of myocardial cells to resist ischemia and hypoxia, and effectively prevent the development and deterioration of CHD (Huang et al., 2016). To verify whether XPF works in treating CHD with depression by intervening in the cAMP-PKA-CREB-BDNF signaling pathway and cell apoptosis process, the expression of 5-HT, Ang-II, cAMP, PKA, CREB, BDNF, Bcl-2, Bax, Cyt-c, and caspase-3 in the myocardial and hippocampal tissues were detected.
As shown in Figures 6A–C, it was noted that compared with the control group, the levels of Ang-II and 5-HT were significantly increased in the myocardial tissues in the model group. Compared with the control group, the expression of cAMP in the myocardial tissues was markedly lower in the model group (p < 0.005). After treatment for 21 days, high-dose XPF decreased the levels of Ang-II and 5-HT in the myocardium (p < 0.01). The treatments with middle-dose XPF solely decreased the levels of Ang-II (p < 0.05), whereas low-dose XPF had no effect on the levels of Ang-II and 5-HT in the myocardium. The levels of cAMP were significantly increased, in a dose-dependent manner, in the myocardium of the rats in the XPF-H, XPF-M, and XPF-L groups (p < 0.005).
FIGURE 6. Experimental validation of key targets Ang-II, 5-HT, cAMP in rat myocardium and hippocampus induced by CUMS and ISO. (A) The levels of Ang-II in the myocardial were determined by ELISA. (B) The levels of 5-HT in the myocardial were determined by ELISA. (C) The levels of cAMP in the myocardial were determined by ELISA. (D) The levels of Ang-II in the hippocampus were determined by ELISA. (E) The levels of 5-HT in the hippocampus were determined by ELISA. (F) The levels of cAMP in the hippocampus were determined by ELISA. Data were presented as means ± SD (n = 3). ***p < 0.005 compared with the control group, ##p < 0.05, ##p < 0.01, ###p < 0.005.
As shown in Figures 6D–F, it was noted that, compared with the control group, the levels of Ang-II were significantly increased in the hippocampus of the model group. Conversely, the expression of 5-HT and cAMP in the hippocampus was markedly lower in the model group. After treatment for 21 days, the treatment with high-dose XPF and middle-dose XPF significantly decreased the levels of Ang-II (p < 0.005) and increased the levels of 5-HT and cAMP in the hippocampus (p < 0.05). Similarly, treatment with metoprolol markedly decreased the levels of Ang-II, and treatment with sertraline increased the levels of 5-HT and cAMP in the hippocampus (p < 0.05). However, low-dose XPF had no effect on the levels of Ang-II, 5-HT, and cAMP in the hippocampus.
As presented in Figures 7A–C, the results of the WB analysis indicated that the expression of PKA, CREB, and BDNF in the myocardium in the model group was significantly lower than that of the control group (p < 0.005). XPF could upregulate the expression of PKA, CREB, and BDNF compared with the model group (p < 0.05).
FIGURE 7. Experimental validation of key targets PKA, CREB, BDNF in rat myocardium and hippocampus induced by CUMS and ISO. (A) Quantitative analysis of PKA expression in the myocardial. (B) Quantitative analysis of CREB expression in the myocardial. (C) Quantitative analysis of BDNF expression in the hippocampal. (D) Quantitative analysis of PKA expression in the hippocampal. (E) Quantitative analysis of CREB expression in the hippocampal. (F) Quantitative analysis of CREB expression in the hippocampal. Data were presented as means ± SD (n = 3). ***p < 0.005 compared with the control group, ##p < 0.01, ##p < 0.005.
As shown in Figures 7D–F, the PKA, CREB, and BDNF protein levels of the model group were significantly lower than those of the control group. High-dose XPF and middle-dose XPF was able to upregulate the expression of PKA, CREB, and BDNF compared with the model group (p < 0.005). However, low-dose XPF had no effect on the levels of CREB and BDNF in the hippocampus.
As shown in Figure 8 and Table 5, compared with the control group, the levels of Bax, Cyt-c, and caspase-3 were significantly increased in the model group, although Bcl-2 levels significantly decreased. Treatment with XPF significantly increased the levels of Bcl-2 and decreased the levels of Bax, Cyt-c, and caspase-3 (p < 0.005), in a dose dependent manner.
FIGURE 8. Experimental validation of key targets Bcl-2, BAX, Cyt-c, caspase-3 in rat myocardium induced by CUMS and ISO. (A) Quantitative analysis of Bcl-2 expression in the myocardial. (B) Quantitative analysis of BAX expression in the myocardial. (C) Western blot analysis of Bcl-2 and BAX protein. (D) Quantitative analysis of Cyt-c expression in the myocardial. (E) Quantitative analysis of caspase-3 expression in the myocardial. (F) Western blot analysis of Cyt-c and caspase-3 protein. Data were presented as means ± SD (n = 3). ***p < 0.005 compared with the control group, ###p < 0.005 compared with the model group.
TABLE 5. Comparison of gray values of Bcl-2, Bax, Cyt-C and Caspase-3 in myocardium of rats in different groups.
As shown in Figure 9 and Table 6, the results of the WB analysis indicated that the expression of Bax, Cyt-c, and caspase-3 was significantly increased in the model group, while Bcl-2 levels were significantly decreased. After treatment for 21 days, high-dose XPF and middle-dose XPF significantly decreased the levels of Bax, Cyt-c, and caspase-3 (p < 0.005). High-dose, and middle-dose XPF also increased the levels of Bcl-2 (p < 0.05), in a dose-dependent manner. In contrast, low-dose XPF only inhibited the expression of Bax and Cyt-c (p < 0.005), but had no effect on the expression of Bcl-2 and caspase-3.
FIGURE 9. Experimental validation of key targets Bcl-2, BAX, Cyt-c, caspase-3 in rat hippocampus induced by CUMS and ISO. (A) Quantitative analysis of Bcl-2 expression in the hippocampal. (B) Quantitative analysis of BAX expression in the hippocampal. (C) Western blot analysis of Bcl-2 and BAX protein. (D) Quantitative analysis of Cyt-c expression in the hippocampal. (E) Quantitative analysis of caspase-3 expression in the hippocampal. (F) Western blot analysis of Cyt-c and caspase-3 protein. Data were presented as means ± SD (n = 3). ***p < 0.005 compared with the control group, ###p < 0.005 compared with the model group.
TABLE 6. Comparison of gray values of Bcl-2, Bax, Cyt-C and Caspase-3 in hippocampus of rats in different groups.
XPF, characterized by multiple components and targets, has been shown to have a relatively satisfactory therapeutic effect in treating CHD with depression. XPF can improve myocardial blood supply and mental state of patients at the same time, which reflects the overall concept of traditional Chinese medicine (Hou et al., 2017). Through this study, we found that XPF could protect the myocardium and hippocampus in CUMS and ISO-induced CHD in depression rats, it shows that XPF has the advantages and characteristics of treating the heart and brain at the same time. However, it is difficult to accurately identify and understand the active compounds and mechanisms of XPF in treating CHD with depression solely by using conventional pharmacological methods. Therefore, new research approaches to reveal the interaction between components of TCM and biological system networks are urgently required. Here, an integrated strategy was established by combining serum pharmacochemistry and network pharmacology to comprehensively and systematically investigate the components and mechanisms of XPF in treating CHD with depression, and validated in experiments.
In this study, 51 compounds were detected in rat serum after oral administration of XPF using UPLC-Q-TOF/MS technology. The identified constituents, mainly phenolic acids, saponins, and flavonoids, were determined to be the main active components of XPF. Among them, phenolic acids, such as rosmarinic acid and eugenol, can inhibit vascular smooth muscle cell proliferation and have a cardioprotective effect (Liu Y et al., 2018). Rosmarinic acid can treat neurodegenerative diseases via anti-neuroinflammatory activity (Wei et al., 2018). Furthermore, saponins, such as saikosaponin A, are the major bioactive component extracted from Radix Bupleuri (Chen et al., 2018; Liu R et al., 2018) and have anti-inflammatory and antioxidant pharmacological activities, as well as a good therapeutic effect on many diseases of the central nervous system and cardiovascular system (Guo et al., 2020). Flavonoids, such as hesperidin and hesperetin, can inhibit inflammatory reactions and oxidative stress, and protect nerve cells and vascular endothelium (Hwang et al., 2012; Van Rymenant et al., 2017).
Moreover, the component–target network between the targets of absorbed components in the serum of XPF and CHD with depression was built using Cytoscape, followed by topological parameters and PPI analysis. As a result, 8 components (eugenol, emodin, isorhamnetin, nobiletin, isoliquiritigenin, rosmarinic acid, 4-Hydroxy-3-butylphthalide, and acacetin), with higher degree centrality were determined to be the active ingredients of XPF, and 10 hub targets, including (ACE2, HTR1A, HTR2A, AKT1, PKIA, CREB1, BDNF, BCL2, BAX, CASP3, cAMP) were determined as hub targets of XPF in treating CHD with depression. GO analysis and KEGG pathway enrichment analysis showed that XPF may regulate the cAMP signaling and apoptosis pathways by interfering with the expression of Ang-II, 5-HT, cAMP, PKA, CREB, BDNF, Bcl-2, Bax, Cyt-c, and caspase-3.
In this study, CUMS and ISO-induced CHD in a rat model of depression was used to explore the mechanism of XPF in treating CHD with depression. These rats showed a large number of apoptotic cells in myocardial and hippocampal tissues compared with the control group, which indicated that the CHD with depression model was successfully established. Sertraline and metoprolol were used as positive control drugs to treat CHD in rats with depression. Sertraline can increase the content of 5-hydroxytryptamine (5-HT) in the brain and exert antidepressant-like effects (Sun et al., 2020). Metoprolol is a beta-blocker commonly used in the clinic (Podlesnikar et al., 2019; Qin et al., 2020). Studies have shown that metoprolol could reduce the oxygen consumption of the myocardium and reduce the risk of cardiovascular events (Assimon et al., 2018). However, co-administration of sertraline and metoprolol increases the blood concentration of metoprolol. Therefore, we adjusted the dose of metoprolol in the sertraline and metoprolol groups in this study (Bahar et al., 2018).
Under these experimental conditions, XPF could decrease Ang-II content in the circulation and central system, inhibit 5-HT level in peripheral circulation, and increase 5-HT content in the central nervous system. Meanwhile, XPF could upregulate the expression levels of cAMP, PKA, CREB, and BDNF both in the myocardium and hippocampus, which matched the predicted results of the network pharmacological analysis. Interestingly, sertraline regulated the cAMP, PKA, CREB, and BDNF levels in the hippocampus, but had a limited effect on the myocardium. Metoprolol can regulate the cAMP, PKA, CREB, and BDNF expression in myocardial, but it has a limited effect on the central nervous system. XPF treatment not only activated the cAMP signal pathway, but also further inhibited apoptosis and protected the myocardium and hippocampus. The cAMP signaling pathway can increase the expression of Bcl-2 and inhibit the activation of caspase-3 by regulating the expression of CREB and BDNF proteins (Mo et al., 2020; Yang et al., 2020). In this study, XPF rectified the injury of the hippocampus and myocardium caused by CHD in depressed rats, significantly decreasing the hippocampal and myocardial levels of Bax, Cyt-c, caspase-3, and increased Bcl-2 expression, which suggests that XPF may inhibit hippocampal and myocardial apoptosis, improve heart function and nerve function, and exert antidepressant and anti-myocardial ischemia effects.
Chronic stressful life events can stimulate the renin-angiotensin-aldosterone system (RAAS). Evidence has shown that Ang-II levels are significantly higher in patients with CHD and depression. Ang-II is metabolized into Ang-(1–7), which directly interferes with the intestinal uptake of tryptophan and affects the metabolism of 5-HT (Klempin et al., 2018; W et al., 2020). The decrease of 5-HT levels in the central nervous system leads to depression or other mental disorders (Jiao et al., 2019; Wenxiu et al., 2020). 5-HT metabolic disorder inhibits the cAMP signaling pathway (Louiset et al., 2017). The cAMP signaling pathway was observed in both CHD and depression patients (Amare et al., 2017; Wang and Mao, 2019; Sandrini et al., 2020).
Activated cAMP and PKA can phosphorylate the Ca2+channel on the cell membrane, promote calcium flow in cardiomyocytes, promote excitation contraction coupling, enhance myocardial contractility, improve the anti-ischemic effects of cardiomyocytes, and produce protective effects on cardiomyocytes (Inserte et al., 2004; Zhang et al., 2010). At the same time, cAMP and PKA are also widely distributed in the nervous system, which can guide the regeneration of neurons, improve the plasticity of synapses, and play a role in protecting the nervous system (Zhang M et al., 2018). CREB is involved in a wide range of neural plasticity processes, including neuronal survival, neuroprotection, neurogenesis, synaptic plasticity, and regulation of emotional expression (Liu et al., 2016; Tang et al., 2020). BDNF is a classical downstream target gene of CREB, which can nourish nerves and promote the differentiation, increment, and regeneration of neurons (Wang et al., 2019). BDNF also has a modulatory role in cardiovascular function, is involved in angiogenesis maintenance of endothelial integrity (Kaess et al., 2015; Jin et al., 2018), repairing myocardial microcirculation, and maintaining myocardial cell function after myocardial injury (Lee et al., 2017). Low expression of CREB and BDNF causes Bax overexpression, Bcl-2 expression is inhibited, and the promotion of the release of apoptosis factors such as cytochrome c (Cyt-c) into the cytoplasm and increased caspase-3. Caspase-3, the final executive factor of apoptosis, leads to apoptosis (Xu et al., 2018). Bcl-2 can form a heterodimer with Bax to inhibit its function, Bcl-2 can also inhibit the activation of caspase-3, and inhibit apoptosis (Abu et al., 2018). Meng (Meng and Wang, 2013) have found that Baishile (BSL) capsule could significantly improve the CUMS rats with depression-like behavior; K252a could block the cAMP signaling pathway to antagonize the antidepressant effect of BSL capsule. Jiang (Jiang et al., 2017) have found that acupuncture could improve depressive-like behaviors via PKA/CREB signaling pathway, H89 could block the PKA/CREB signaling pathway to antagonize the antidepressant effect of acupuncture. Mao (Mao and Zhang, 2014) have found H89 could block the PKA/CREB signaling pathway to antagonize the myocardial protective effect of Danlou tablets. Therefore, enhanced expression of related proteins in the cAMP signaling pathway and inhibition of the cell apoptosis process contributes to the alleviation of angina pectoris, chest pain, and depression symptoms. Essentially, it promotes protection of the cardiovascular and nervous system against coronary heart disease with depression.
The 8 active components (eugenol, emodin, isorhamnetin, nobiletin, isoliquiritigenin, rosmarinic acid, 4-Hydroxy-3-butylphthalide, and acacetin) in XPF have been reported to activate the cAMP signaling pathway of CHD and depression. Eugenol can reverse oxidative stress, inhibit of caspase-3 activity, and it has an anti-myocardial ischemia effect. Emodin opposes CUMS-induced depressive-like behavior in rats by upregulating the levels of hippocampal BDNF (Choudhary et al., 2006; Liu et al., 2017). Isorhamnetin has a positive effect on H/R-induced injury by reducing caspase-3 and attenuating oxidative stress in H9c2 cardiomyocytes (Zhao et al., 2018). Nobiletin and acacetin exerted antidepressant-like effects by increasing the level of 5-HT in the hippocampus (Yi et al., 2011). Isoliquiritigenin has been shown to have neuroprotective effects by increasing the level of cAMP in peripheral nerves (Shindo et al., 1992). Rosmarinic acid can regulate the expression of BDNF in the hippocampus (Makhathini et al., 2018), and inhibit angiotensin-converting enzyme activity (Ferreira et al., 2018).
Increasing evidence has shown a reciprocal causation relationship between CHD and depression. Regulating psychopathy can significantly improve the quality of life of patients with CHD and reduce the incidence of acute cardiovascular events (Kuhlmann et al., 2019). Recently, TCM has attracted increasing attention in treating cardiovascular and psychological diseases because of its holistic approach and satisfactory clinical efficacy. Through this study, we found that XPF could protect the myocardium and hippocampus in CUMS and ISO-induced CHD in depression rats by regulating the cAMP signal cascade. Furthermore, XPF can inhibit cell apoptosis, improve heart function, reduce neuropathy, improve nerve function, and exert an antidepressant and antimyocardial ischemia effect. These results preliminarily show the material basis and mechanism of XPF in the treatment of CHD with depression.
In this study, we proposed an integrative systems pharmacology strategy to illustrate the mechanism of XPF in treating CHD with depression by combining serum pharmacochemistry, network analysis, and experimental validation. First, we identified 51 ingredients in rat serum after oral administration of XPF, which was used for the construction and analysis of the component–target network between the targets of absorbed components in serum and CHD with depression. As a result, 10 components with higher degree centrality were determined to be the active ingredients of XPF, and 10 hub genes, including ACE2, HTR1A, HTR2A, AKT1, PKIA, CREB1, BDNF, BCL2, BAX, CASP3, and cAMP were determined as targets of XPF in treating CHD with depression. Furthermore, the hub targets (AngⅡ, 5-HT, cAMP, PKA, CREB, BDNF, Bcl-2, Bax, Cyt-c, and caspase-3) predicted by network pharmacology analysis were validated. We confirmed the myocardial protective and neuroprotective effects of XPF in treating CHD with depression, which was associated with its activation of the cAMP signaling pathway and inhibition of myocardium and hippocampus apoptosis. In conclusion, these results indicate that the integrated pharmacology strategy provides an efficient approach for exploring the pharmacological mechanisms of TCM.
The raw data supporting the conclusions of this article will be made available by the authors, without undue reservation.
The animal study was reviewed and approved by and the guidelines of the National Institutes of Health. All animal experiments were approved by the Ethics Committee of Tianjin University of Traditional Chinese Medicine. Written informed consent was obtained from the owners for the participation of their animals in this study.
LZ is the first author and performed all the experiments and drafted the manuscript. YZ contributed toward study design and revised the manuscript. LP, FD, and JC helped the first author, prepared the materials of this paper. ZC, SZ, and MZ provided fund support. WD and XX contributed toward study design, experimental setup, results supervision, and manuscript correction.
This work was supported by National Natural Science Foundation of China (81774227); Natural Science Fund of Tianjin City (17JCZDJC34600); Technology program in key areas of Tianjin (20190101); Scientific research project of Tianjin Education Commission (2018KJ014).
The authors declare that the research was conducted in the absence of any commercial or financial relationships that could be construed as a potential conflict of
The Supplementary Material for this article can be found online at: https://www.frontiersin.org/articles/10.3389/fphar.2021.590602/full#supplementary-material.
The authors would like to acknowledge Editage Language Services for providing language assistance and for proofreading the manuscript.
Aa, N., Guo, J. H., Cao, B., Sun, R. B., Ma, X. H., Chu, Y., et al. (2019). Compound danshen dripping pills normalize a reprogrammed metabolism of myocardial ischemia rats to interpret its time-dependent efficacy in clinic trials: a metabolomic study. Metabolomics 15, 128. doi:10.1007/s11306-019-1577-3
Abu, Z. E., Hussein, M., and Ali, H. (2018). Ascorbic acid protects male rat brain from oral potassium dichromate-induced oxdative DNA damage and apoptotic changes: the expression patterns of caspase-3, P 53, Bax, and Bcl-2 genes. Environ. Sci. Pollut. Res. Int. 25, 13056–13066. doi:10.1007/s11356-018-1546-9
Amare, A. T., Schubert, K. O., Klingler-Hoffmann, M., Cohen-Woods, S., and Baune, B. T. (2017). The genetic overlap between mood disorders and cardiometabolic diseases: a systematic review of genome wide and candidate gene studies. Transl Psychiatry 7, e1007. doi:10.1038/tp.2016.261
Amberger, J. S., and Hamosh, A. (2017). Searching online mendelian inheritance in man (OMIM): a knowledgebase of human genes and genetic phenotypes. Curr. Protoc. Bioinformatics 58, 1–121. doi:10.1002/cpbi.2712.12
Assimon, M. M., Brookhart, M. A., Fine, J. P., Heiss, G., Layton, J. B., and Flythe, J. E. (2018). A comparative study of carvedilol versus metoprolol Initiation and 1-year mortality among individuals receiving maintenance hemodialysis. Am. J. Kidney Dis. 72, 337–348. doi:10.1053/j.ajkd.2018.02.350
Bahar, M. A., Kamp, J., Borgsteede, S. D., Hak, E., and Wilffert, B. (2018). The impact of CYP2D6 mediated drug-drug interaction: a systematic review on a combination of metoprolol and paroxetine/fluoxetine. Br. J. Clin. Pharmacol. 84, 2704–2715. doi:10.1111/bcp.13741
Blais, C., Rochette, L., Ouellet, S., and Huynh, T. (2020). Complex evolution of epidemiology of vascular diseases, including increased disease burden: from 2000 to 2015. Can. J. Cardiol. 36, 740–746. doi:10.1016/j.cjca.2019.10.021
Carney, R. M., and Freedland, K. E. (2017). Depression and coronary heart disease. Nat. Rev. Cardiol. 14, 145–155. doi:10.1038/nrcardio.2016.181
Chen, B., Li, J., Xie, Y., Ming, X., Li, G., Wang, J., et al. (2019). Cang-ai volatile oil improves depressive-like behaviors and regulates DA and 5-HT metabolism in the brains of CUMS-induced rats. J. Ethnopharmacol. 244, 112088. doi:10.1016/j.jep.2019.112088
Chen, M., Men, L., Ou, L., Li, T., Li, M., Zhong, X., et al. (2018). Effectiveness and safety of modified ʽHuoxue Shugan' formulas on coronary heart disease combined with depression: protocol for a systematic review. BMJ Open 8, e022868. doi:10.1136/bmjopen-2018-022868
Chen, X. Q., Chen, S. J., Liang, W. M., Wang, M., Li, C. F., Wang, S. S., et al. (2018). Saikosaponin A attenuates perimenopausal depression-like symptoms by chronic unpredictable mild stress. Neurosci. Lett. 662, 283–289. doi:10.1016/j.neulet.2017.09.046
Chen, X. Z., Cao, Z. Y., Liao, L. M., Liu, Z. Z., and Du, J. (2014). Application of serum pharmacology in evaluating the antitumor effect of Fuzheng Yiliu Decoction from Chinese medicine. Chin. J. Integr. Med. 20, 450–455. doi:10.1007/s11655-013-1544-1
Choudhary, R., Mishra, K. P., and Subramanyam, C. (2006). Interrelations between oxidative stress and calcineurin in the attenuation of cardiac apoptosis by eugenol. Mol. Cel. Biochem. 283, 115–122. doi:10.1007/s11010-006-2386-3
de Heer, E. W., Palacios, J. E., Adèr, H. J., van Marwijk, H. W. J., Tylee, A., and van der Feltz-Cornelis, C. M. (2020). Chest pain, depression and anxiety in coronary heart disease: consequence or cause? A prospective clinical study in primary care. J. Psychosom. Res. 129, 109891. doi:10.1016/j.jpsychores.2019.109891
Dennis, G., Sherman, B. T., Hosack, D. A., Yang, J., Gao, W., Lane, H. C., et al. (2003). DAVID: database for annotation, visualization, and integrated discovery. Genome Biol. 4, P3. doi:10.1186/gb-2003-4-5-p3
Fang, J., Cai, C., Chai, Y., Zhou, J., Huang, Y., Gao, L., et al. (2019). Quantitative and systems pharmacology 4. Network-based analysis of drug pleiotropy on coronary artery disease. Eur. J. Med. Chem. 161, 192–204. doi:10.1016/j.ejmech.2018.10.020
Ferreira, L. G., Evora, P. R. B., Capellini, V. K., Albuquerque, A. A., Carvalho, M. T. M., Gomes, R. A. D. S., et al. (2018). Effect of rosmarinic acid on the arterial blood pressure in normotensive and hypertensive rats: role of ACE. Phytomedicine 38, 158–165. doi:10.1016/j.phymed.2017.02.006
Gfeller, D., Grosdidier, A., Wirth, M., Daina, A., Michielin, O., and Zoete, V. (2014). SwissTargetPrediction: a web server for target prediction of bioactive small molecules. Nucleic Acids Res. 42, W32–W38. doi:10.1093/nar/gku293
Gross, M., and Pinhasov, A. (2016b). Chronic mild stress in submissive mice: marked polydipsia and social avoidance without hedonic deficit in the sucrose preference test. Behav. Brain Res. 298, 25–34. doi:10.1016/j.bbr.2015.10.049
Guo, J., Zhang, F., Gao, J., Guan, X., Liu, B., Wang, X., et al. (2020). Proteomics-based screening of the target proteins associated with antidepressant-like effect and mechanism of Saikosaponin A. J. Cel. Mol. Med. 24, 174–188. doi:10.1111/jcmm.14695
Hart, T., and Xie, L. (2016). Providing data science support for systems pharmacology and its implications to drug discovery. Expert Opin. Drug Discov. 11, 241–256. doi:10.1517/17460441.2016.1135126
Hou, S. S., Du, W. X., Li, X. X., Zhang, Y., et al. (2017). Professor Du Wuxun's experience in treating climacteric arrhythmia. J. Sichuan Traditional Chin. Med. 35, 133–134.
Hu, Y., Dong, X., Zhang, T., Ma, H., Yang, W., Wang, Y., et al. (2020). Kai-Xin-San suppresses matrix metalloproteinases and myocardial apoptosis in rats with myocardial infarction and depression. Mol. Med. Rep. 21, 508–516. doi:10.3892/mmr.2019.10807
Huang, H., Hong, Q., Tan, H. L., Xiao, C. R., and Gao, Y. (2016). Ferulic acid prevents LPS-induced up-regulation of PDE4B and stimulates the cAMP/CREB signaling pathway in PC12 cells. Acta Pharmacol. Sin. 37, 1543–1554. doi:10.1038/aps.2016.88
Hwang, S. L., Lin, J. A., Shih, P. H., Yeh, C. T., and Yen, G. C. (2012). Pro-cellular survival and neuroprotection of citrus flavonoid: the actions of hesperetin in PC12 cells. Food Funct. 3, 1082–1090. doi:10.1039/c2fo30100h
Inserte, J., Garcia-Dorado, D., Ruiz-Meana, M., Agulló, L., Pina, P., and Soler-Soler, J. (2004). Ischemic preconditioning attenuates calpain-mediated degradation of structural proteins through a protein kinase A-dependent mechanism. Cardiovasc. Res. 64, 105–114. doi:10.1016/j.cardiores.2004.06.001
Jiang, H., Zhang, X., Wang, Y., Zhang, H., Li, J., Yang, X., et al. (2017). Mechanisms underlying the antidepressant response of acupuncture via PKA/CREB signaling pathway. Neural Plast. 2017, 4135164. doi:10.1155/2017/4135164
Jiao, H., Yan, Z., Ma, Q., Li, X., Jiang, Y., Liu, Y., et al. (2019). Influence of Xiaoyaosan on depressive-like behaviors in chronic stress-depressed rats through regulating tryptophan metabolism in hippocampus. Neuropsychiatr. Dis. Treat. 15, 21–31. doi:10.2147/NDT.S185295
Jin, H., Chen, Y., Wang, B., Zhu, Y., Chen, L., Han, X., et al. (2018). Association between brain-derived neurotrophic factor and von Willebrand factor levels in patients with stable coronary artery disease. BMC Cardiovasc. Disord. 18, 23. doi:10.1186/s12872-018-0762-z
Kaess, B. M., Preis, S. R., Lieb, W., Beiser, A. S., Yang, Q., Chen, T. C., et al. (2015). Circulating brain-derived neurotrophic factor concentrations and the risk of cardiovascular disease in the community. J. Am. Heart Assoc. 4, e001544. doi:10.1161/JAHA.114.001544
Klempin, F., Mosienko, V., Matthes, S., Villela, D. C., Todiras, M., Penninger, J. M., et al. (2018). Depletion of angiotensin-converting enzyme 2 reduces brain serotonin and impairs the running-induced neurogenic response. Cell Mol Life Sci 75, 3625–3634. doi:10.1007/s00018-018-2815-y
Kuhlmann, S. L., Arolt, V., Haverkamp, W., Martus, P., Ströhle, A., Waltenberger, J., et al. (2019). Prevalence, 12-month prognosis, and clinical management need of depression in coronary heart disease patients: a prospective cohort study. Psychother. Psychosom. 88, 300–311. doi:10.1159/000501502
Lee, H. W., Ahmad, M., Wang, H. W., and Leenen, F. H. (2017). Effects of exercise training on brain-derived neurotrophic factor in skeletal muscle and heart of rats post myocardial infarction. Exp. Physiol. 102, 314–328. doi:10.1113/EP086049
Lee, S. J., Koh, S., Kim, B. O., Kim, B., and Kim, C. (2018). Effect of type D personality on short-term cardiac rehabilitation in patients with coronary artery disease. Ann. Rehabil. Med. 42, 748–757. doi:10.5535/arm.2018.42.5.748
Li, F., Zhang, Y. B., Wei, X., Song, C. H., Qiao, M. Q., and Zhang, H. Y. (2016). Metabolic profiling of Shu-Yu capsule in rat serum based on metabolic fingerprinting analysis using HPLC-ESI-MSn. Mol. Med. Rep. 13, 4191–4204. doi:10.3892/mmr.2016.5082
Li, Y., Ge, F., and Wang, S. (2020). Four genes predict the survival of osteosarcoma patients based on TARGET database. J. Bioenerg. Biomembr. 52, 291–299. doi:10.1007/s10863-020-09836-6
Li, Y. H., Yu, C. Y., Li, X. X., Zhang, P., Tang, J., Yang, Q., et al. (2018). Therapeutic target database update 2018: enriched resource for facilitating bench-to-clinic research of targeted therapeutics. Nucleic Acids Res. 46, D1121–D1127. doi:10.1093/nar/gkx1076
Li, C., Ma, R., Zhang, X., Ma, J., Wang, X., He, J., et al. (2020). Risk of coronary heart disease in the rural population in Xinjiang: a nested case-control study in China. PLoS One 15, e0229598. doi:10.1371/journal.pone.0229598
Liu, R., Heiss, E. H., Waltenberger, B., Blažević, T., Schachner, D., Jiang, B., et al. (2018). Constituents of mediterranean spices counteracting vascular smooth muscle cell proliferation: Identification and characterization of rosmarinic acid methyl ester as a novel inhibitor. Mol. Nutr. Food Res. 62, e1700860. doi:10.1002/mnfr.201700860
Liu, Y., Gao, L., Zhao, X., Guo, S., Liu, Y., Li, R., et al. (2018). Saikosaponin a protects from pressure overload-induced cardiac fibrosis via inhibiting fibroblast activation or endothelial cell endMT. Int. J. Biol. Sci. 14, 1923–1934. doi:10.7150/ijbs.27022
Liu, Y. M., Hu, C. Y., Shen, J. D., Wu, S. H., Li, Y. C., and Yi, L. T. (2017). Elevation of synaptic protein is associated with the antidepressant-like effects of ferulic acid in a chronic model of depression. Physiol. Behav. 169, 184–188. doi:10.1016/j.physbeh.2016.12.003
Liu, Z., Qi, Y., Cheng, Z., Zhu, X., Fan, C., and Yu, S. Y. (2016). The effects of ginsenoside Rg1 on chronic stress induced depression-like behaviors, BDNF expression and the phosphorylation of PKA and CREB in rats. Neuroscience 322, 358–369. doi:10.1016/j.neuroscience.2016.02.050
Louiset, E., Duparc, C., Lenglet, S., Gomez-Sanchez, C. E., and Lefebvre, H. (2017). Role of cAMP/PKA pathway and T-type calcium channels in the mechanism of action of serotonin in human adrenocortical cells. Mol. Cel. Endocrinol. 441, 99–107. doi:10.1016/j.mce.2016.10.008
Magaard, J. L., Löwe, B., Brütt, A. L., and Kohlmann, S. (2018). Illness beliefs about depression among patients seeking depression care and patients seeking cardiac care: an exploratory analysis using a mixed method design. BMC Psychiatry 18, 366. doi:10.1186/s11888-018-1936-z
Makhathini, K. B., Mabandla, M. V., and Daniels, W. M. U. (2018). Rosmarinic acid reverses the deleterious effects of repetitive stress and tat protein. Behav. Brain Res. 353, 203–209. doi:10.1016/j.bbr.2018.07.010
Mao, Shuai., and Zhang, Minzhou. (2014). The effect of Danlou tablets and its components on ventricular remodeling after acute coronary syndrome. Guangzhou; Guangzhou University of Traditional Chinese Medicine.
Meng, P., and Wang, Y.. (2013). The Effect of Baishile capsule on hippocampus nerveregeneration of Chronic Unpredicted Mild Stressdepression model rats by signal pathway of cAMP-CREB-BDNF. Hunan Univ. traditional Chin. Med. 8, 35558–35572. doi:10.18632/oncotarget.16009
Mi, L., Chen, Y., Zheng, X., Li, Y., Zhang, Q., Mo, D., et al. (2015). MicroRNA-139-5p suppresses 3T3-L1 preadipocyte differentiation through notch and IRS1/PI3K/akt insulin signaling pathways. J. Cel. Biochem. 116, 1195–1204. doi:10.1002/jcb.25065
Mo, F., Tang, Y., Du, P., Shen, Z., Yang, J., Cai, M., et al. (2020). GPR39 protects against corticosterone-induced neuronal injury in hippocampal cells through the CREB-BDNF signaling pathway. J. Affect Disord. 272, 474–484. doi:10.1016/j.jad.2020.03.137
Mozaffarian, D., Mozaffarian, D., Benjamin, E. J., Go, A. S., Arnett, D. K., Blaha, M. J., et al. (2016). Executive summary: heart disease and stroke statistics--2016 update: a Report from the American Heart Association. Circulation 133, 447–454. doi:10.1161/CIR.0000000000000366
Otasek, D., Morris, J. H., Bouças, J., Pico, A. R., and Demchak, B. (2019). Cytoscape automation: empowering workflow-based network analysis. Genome Biol. 20, 185. doi:10.1186/s13059-019-1758-4
Park, S. Y., Park, J. H., Kim, H. S., Lee, C. Y., Lee, H. J., Kang, K. S., et al. (2018). Systems-level mechanisms of action of Panax ginseng: a network pharmacological approach. J. Ginseng Res. 42, 98–106. doi:10.1016/j.jgr.2017.09.001
Podlesnikar, T., Pizarro, G., Fernández-Jiménez, R., Montero-Cabezas, J. M., Sánchez-González, J., Bucciarelli-Ducci, C., et al. (2019). Effect of early metoprolol during ST-Segment elevation myocardial infarction on left ventricular strain: feature-tracking cardiovascular magnetic resonance substudy from the METOCARD-CNIC trial. JACC Cardiovasc. Imaging 12, 1188–1198. doi:10.1016/j.jcmg.2018.07.019
Qin, W., Zhang, L., Li, Z., Xiao, D., Zhang, Y., Yang, H., et al. (2020). Metoprolol protects against myocardial infarction by inhibiting miR-1 expression in rats. J. Pharm. Pharmacol. 72, 76–83. doi:10.1111/jphp.13192
Ru, J., Li, P., Wang, J., Zhou, W., Li, B., Huang, C., et al. (2014). TCMSP: a database of systems pharmacology for drug discovery from herbal medicines. J. Cheminform 6, 13. doi:10.1186/1758-2946-6-13
Sandrini, L., Castiglioni, L., Amadio, P., Werba, J. P., Eligini, S., Fiorelli, S., et al. (2020). Impact of BDNF Val66Met polymorphism on myocardial infarction: exploring the macrophage phenotype. Cells 9, 1084. doi:10.3390/cells9051084
Shindo, H., Tawata, M., Aida, K., and Onaya, T. (1992). The role of cyclic adenosine 3',5'-monophosphate and polyol metabolism in diabetic neuropathy. J. Clin. Endocrinol. Metab. 74, 393–398. doi:10.1210/jcem.74.2.1370506
Su, S. F., Chang, M. Y., and He, C. P. (2018). Social support, unstable angina, and stroke as predictors of depression in patients with coronary heart disease. J. Cardiovasc. Nurs. 33, 179–186. doi:10.1097/JCN.0000000000000419
Sun, W., Jin, Y., Wang, C., Zhao, S., Wang, X., Luo, M., et al. (2020). Stereoselective separation of isomeric sertraline with analytical countercurrent chromatography. J. Chromatogr. A. 1617, 460834. doi:10.1016/j.chroma.2019.460834
Szklarczyk, D., Morris, J. H., Cook, H., Kuhn, M., Wyder, S., Simonovic, M., et al. (2017). The STRING database in 2017: quality-controlled protein-protein association networks, made broadly accessible. Nucleic Acids Res. 45, D362–D368. doi:10.1093/nar/gkw937
Tang, X. H., Zhang, G. F., Xu, N., Duan, G. F., Jia, M., Liu, R., et al. (2020). Extrasynaptic CaMKIIα is involved in the antidepressant effects of ketamine by downregulating GluN2B receptors in an LPS-induced depression model. J. Neuroinflammation 17, 181. doi:10.1186/s11974-020-01843-z
Tang, Z., Wei, J., Yu, Y., Zhang, J., Liu, L., Tang, W., et al. (2016). γ-Secretase inhibitor reverts the Notch signaling attenuation of osteogenic differentiation in aged bone marrow mesenchymal stem cells. Cell Biol. Int. 40, 439–447. doi:10.1002/cbin.10583
Van Rymenant, E., Grootaert, C., Beerens, K., Needs, P. W., Kroon, P. A., Kerimi, A., et al. (2017). Vasorelaxant activity of twenty-one physiologically relevant (poly)phenolic metabolites on isolated mouse arteries. Food Funct. 8, 4331–4335. doi:10.1039/c7fo01273j
Wenxiu, H., Zhijie, W., Ruili, D., Yujin, G., Hailiang, Z., Chunmei, G., et al. (2020). Angiotensin-Ⅱ and angiotensin-(1-7) imbalance affects comorbidity of depression and coronary heart disease. Peptides 131, 170353. doi:10.1016/j.peptides.2020.170353
Wang, C., Guo, J., and Guo, R. (2017). Effect of XingPiJieYu decoction on spatial learning and memory and cAMP-PKA-CREB-BDNF pathway in rat model of depression through chronic unpredictable stress. BMC Complement. Altern. Med. 17, 73. doi:10.1186/s11906-016-1543-9
Wang, J. Q., and Mao, L. (2019). The ERK pathway: molecular mechanisms and treatment of depression. Mol. Neurobiol. 56, 6197–6205. doi:10.1007/s11035-019-1524-3
Wang, N., Ma, J., Liu, J., Wang, J., Liu, C., Wang, H., et al. (2019). Histamine H3 receptor antagonist enhances neurogenesis and improves chronic cerebral hypoperfusion-induced cognitive impairments. Front. Pharmacol. 10, 1583. doi:10.3389/fphar.2019.01583
Wang, Z., Liang, L., Yin, Z., and Lin, J. (2016). Improving chemical similarity ensemble approach in target prediction. J. Cheminform 8, 20. doi:10.1186/s13321-016-0130-x
Wei, Y., Chen, J., Hu, Y., Lu, W., Zhang, X., Wang, R., et al. (2018). Rosmarinic acid mitigates lipopolysaccharide-induced neuroinflammatory responses through the inhibition of TLR4 and CD14 expression and NF-κB and NLRP3 inflammasome activation. Inflammation 41, 732–740. doi:10.1007/s10753-017-0728-9
Wishart, D. S., Feunang, Y. D., Guo, A. C., Lo, E. J., Marcu, A., Grant, J. R., et al. (2018). DrugBank 5.0: a major update to the DrugBank database for 2018. Nucleic Acids Res. 46, D1074–D1082. doi:10.1093/nar/gkx1037
Xu, F., Ren, L., Song, M., Shao, B., Han, Y., Cao, Z., et al. (2018). Fas- and mitochondria-mediated signaling pathway involved in osteoblast apoptosis induced by AlCl3. Biol. Trace Elem. Res. 184, 173–185. doi:10.1007/s11011-017-1176-y
Xu, S. Y., Dian, R. L., and Chen, X. (1997). Methodology of pharmacological experiment. Bejing: People's Medical Publishing House.
Xue, Y.-j., Xie, Y., Zhao, G.-l., Zhou, B.-d., Li, K., Li, S.-y., et al. (2019). Oral Chinese herbal medicine for depressive disorder in patients after percutaneous coronary intervention: a systematic review and meta-analysis. Chin. J. Integr. Med. 26, 617. doi:10.1007/s11655-019-2702-x
Yang, D., Wu, W., Gan, G., Wang, D., Gong, J., Fang, K., et al. (2020). (-)-Syringaresinol-4-O-β-D-glucopyranoside from Cortex Albizziae inhibits corticosterone-induced PC12 cell apoptosis and relieves the associated dysfunction. Food Chem. Toxicol. 141, 111394. doi:10.1016/j.fct.2020.111394
Ye, Y. L., Zhong, K., Liu, D. D., Xu, J., Pan, B. B., Li, X., et al. (2017). Huanglian-Jie-Du-Tang extract ameliorates depression-like behaviors through BDNF-TrkB-CREB pathway in rats with chronic unpredictable stress. Evid. Based Complement. Alternat Med. 2017, 7903918. doi:10.1155/2017/7903918
Yeung, W. F., Chung, K. F., Ng, K. Y., Yu, Y. M., Ziea, E. T., and Ng, B. F. (2014). A systematic review on the efficacy, safety and types of Chinese herbal medicine for depression. J. Psychiatr. Res. 57, 165–175. doi:10.1016/j.jpsychires.2014.05.016
Yi, L. T., Xu, H. L., Feng, J., Zhan, X., Zhou, L. P., and Cui, C. C. (2011). Involvement of monoaminergic systems in the antidepressant-like effect of nobiletin. Physiol. Behav. 102, 1–6. doi:10.1016/j.physbeh.2010.10.008
Yuan, H., Ma, Q., Cui, H., Liu, G., Zhao, X., Li, W., et al. (2017). How can synergism of traditional medicines benefit from network pharmacology?. Molecules 22. doi:10.3390/molecules22071135
Zhang, L., Xu, C. Q., Hong, Y., Zhang, J. L., Liu, Y., Zhao, M., et al. (2010). Propranolol regulates cardiac transient outward potassium channel in rat myocardium via cAMP/PKA after short-term but not after long-term ischemia. Naunyn Schmiedebergs Arch. Pharmacol. 382, 63–71. doi:10.1007/s00210-010-0520-y
Zhang, M., Liu, W., Zhou, Y., Li, Y., Qin, Y., and Xu, Y. (2018). Neurodevelopmental toxicity induced by maternal PM2.5 exposure and protective effects of quercetin and Vitamin C. Chemosphere 213, 182–196. doi:10.1016/j.chemosphere.2018.09.009
Zhang, R., Guo, L., Ji, Z., Li, X., Zhang, C., Ma, Z., et al. (2018). Radix scutellariae attenuates CUMS-Induced depressive-like behavior by promoting neurogenesis via cAMP/PKA pathway. Neurochem. Res. 43, 2111–2120. doi:10.1007/s11064-018-2635-3
Zhang, R., Zhu, X., Bai, H., and Ning, K. (2019). Network pharmacology databases for traditional Chinese medicine: review and assessment. Front. Pharmacol. 10, 123. doi:10.3389/fphar.2019.00123
Zhang, W., Huai, Y., Miao, Z., Qian, A., and Wang, Y. (2019). Systems pharmacology for investigation of the mechanisms of action of traditional Chinese medicine in drug discovery. Front. Pharmacol. 10, 743. doi:10.3389/fphar.2019.00743
Zhao, T. T., Yang, T. L., Gong, L., and Wu, P. (2018). Isorhamnetin protects against hypoxia/reoxygenation-induced injure by attenuating apoptosis and oxidative stress in H9c2 cardiomyocytes. Gene 666, 92–99. doi:10.1016/j.gene.2018.05.009
Keywords: integrative pharmacology, serum pharmacochemistry, network pharmacology analysis, Xiong-Pi-Fang, coronary heart disease with depression
Citation: Zhang L, Zhang Y, Zhu M, Pei L, Deng F, Chen J, Zhang S, Cong Z, Du W and Xiao X (2021) An Integrative Pharmacology-Based Strategy to Uncover the Mechanism of Xiong-Pi-Fang in Treating Coronary Heart Disease with Depression. Front. Pharmacol. 12:590602. doi: 10.3389/fphar.2021.590602
Received: 02 August 2020; Accepted: 11 February 2021;
Published: 01 April 2021.
Edited by:
Yanqiong Zhang, China Academy of Chinese Medical Sciences, ChinaReviewed by:
Nasra Ayuob, Mansoura University, EgyptCopyright © 2021 Zhang, Zhang, Zhu, Pei, Deng, Chen, Zhang, Cong, Du and Xiao. This is an open-access article distributed under the terms of the Creative Commons Attribution License (CC BY). The use, distribution or reproduction in other forums is permitted, provided the original author(s) and the copyright owner(s) are credited and that the original publication in this journal is cited, in accordance with accepted academic practice. No use, distribution or reproduction is permitted which does not comply with these terms.
*Correspondence: Wuxun Du, Y25kdXd1eEAxNjMuY29t; Xuefeng Xiao, a2FpMTIxOUAxNjMuY29t
†These authors have contributed equally to this work
Disclaimer: All claims expressed in this article are solely those of the authors and do not necessarily represent those of their affiliated organizations, or those of the publisher, the editors and the reviewers. Any product that may be evaluated in this article or claim that may be made by its manufacturer is not guaranteed or endorsed by the publisher.
Research integrity at Frontiers
Learn more about the work of our research integrity team to safeguard the quality of each article we publish.