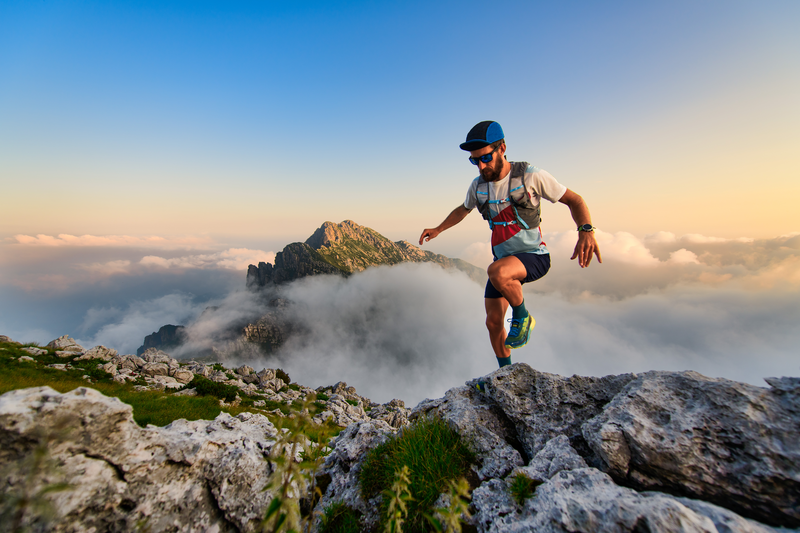
94% of researchers rate our articles as excellent or good
Learn more about the work of our research integrity team to safeguard the quality of each article we publish.
Find out more
SYSTEMATIC REVIEW article
Front. Pharmacol. , 21 January 2021
Sec. Neuropharmacology
Volume 11 - 2020 | https://doi.org/10.3389/fphar.2020.618184
This article is part of the Research Topic Cannabidiol Treatment in Neurotherapeutic Interventions, Volume I View all 13 articles
Background: Accumulating evidence suggests that the non-intoxicating cannabinoid compound cannabidiol (CBD) may have antipsychotic and anxiolytic properties, and thus may be a promising new agent in the treatment of psychotic and anxiety disorders. However, the neurobiological substrates underlying the potential therapeutic effects of CBD are still unclear. The aim of this systematic review is to provide a detailed and up-to-date systematic literature overview of neuroimaging studies that investigated the acute impact of CBD on human brain function.
Methods: Papers published until May 2020 were included from PubMed following a comprehensive search strategy and pre-determined set of criteria for article selection. We included studies that examined the effects of CBD on brain function of healthy volunteers and individuals diagnosed with a psychiatric disorder, comprising both the effects of CBD alone as well as in direct comparison to those induced by ∆9-tetrahydrocannabinol (THC), the main psychoactive component of Cannabis.
Results: One-ninety four studies were identified, of which 17 met inclusion criteria. All studies investigated the acute effects of CBD on brain function during resting state or in the context of cognitive tasks. In healthy volunteers, acute CBD enhanced fronto-striatal resting state connectivity, both compared to placebo and THC. Furthermore, CBD modulated brain activity and had opposite effects when compared to THC following task-specific patterns during various cognitive paradigms, such as emotional processing (fronto-temporal), verbal memory (fronto-striatal), response inhibition (fronto-limbic-striatal), and auditory/visual processing (temporo-occipital). In individuals at clinical high risk for psychosis and patients with established psychosis, acute CBD showed intermediate brain activity compared to placebo and healthy controls during cognitive task performance. CBD modulated resting limbic activity in subjects with anxiety and metabolite levels in patients with autism spectrum disorders.
Conclusion: Neuroimaging studies have shown that acute CBD induces significant alterations in brain activity and connectivity patterns during resting state and performance of cognitive tasks in both healthy volunteers and patients with a psychiatric disorder. This included modulation of functional networks relevant for psychiatric disorders, possibly reflecting CBD’s therapeutic effects. Future studies should consider replication of findings and enlarge the inclusion of psychiatric patients, combining longer-term CBD treatment with neuroimaging assessments.
Recently, there has been a growing interest in cannabidiol (CBD) as a therapeutic substance, due to its putative antipsychotic, anxiolytic and anti-craving effects (Iseger and Bossong, 2015; Rohleder et al., 2016; Batalla et al., 2019). CBD is one of the more than 100 cannabinoids that can be derived from the cannabis plant and is, unlike the main psychoactive compound delta-9-tetrahydrocannabinol (THC), devoid of intoxicating effects (Freeman et al., 2019). Since most conventional treatments in psychiatry, such as antipsychotics and antidepressants, are associated with limited response rates and adverse events that often limit tolerability and adherence (Blessing et al., 2015; Samara et al., 2019), there is an urgent need for developing novel pharmaceutical treatments (Leucht et al., 2013; Blessing et al., 2015; Lally and MacCabe, 2015). In this regard, CBD has been proposed as novel therapeutic compound in several psychiatric disorders, such as psychosis (Iseger and Bossong, 2015; Batalla et al., 2019), anxiety disorders (Blessing et al., 2015), substance use disorders (Chye et al., 2019; Freeman et al., 2020) and autism spectrum disorders (Poleg et al., 2019; Fusar-Poli et al., 2020).
CBD effects are most likely related to the endocannabinoid system (Rohleder et al., 2016), although its precise mechanism of action is not yet fully understood. Animal studies have shown that CBD has no significant affinity with the cannabinoid receptors CB1 and CB2 (Bisogno et al., 2001; Jones et al., 2010), but may act as an antagonist of both in presence of CB1 agonists (Thomas et al., 2007). It has been hypothesized that the antagonistic effects of CBD might be through negative allosteric modulation of the CB1 receptor (Laprairie et al., 2015; Rohleder et al., 2016). Other suggested molecular targets include different types of receptors, such as serotonin type 1A (5HT1A), peroxisome proliferator-activated receptor gamma (PPARgamma), vanilloid receptor 1 (TRPV1), GPR55, and GPR18 (Pertwee, 2008; Gururajan and Malone, 2016). In addition, CBD has been shown to increase plasma levels of the endogenous cannabinoid anandamide, which was related to its antipsychotic effects (Leweke et al., 2012). Hence, CBD may exert a protective effect on disturbances of the endocannabinoid system, as observed in several psychiatric disorders (Leweke et al., 2007; Morgan et al., 2013; Minichino et al., 2019).
Neuroimaging techniques provide a highly useful insight into the human neural processes involved in the behavioral effects of cannabinoids. An increasing number of neuroimaging studies have been performed to examine the human neural mechanisms underlying the effects of CBD. Although some of these studies have been included in excellent reviews that describe the impact of cannabis on human brain function in a broader context (Martín-Santos et al., 2010; Bhattacharyya et al., 2012a; Batalla et al., 2014; Weinstein et al., 2016), the aim of the current review is to provide a systematic and up-to-date overview of neuroimaging studies that investigated the effects of CBD on human brain function. This includes studies that examined the impact of CBD on brain function of healthy volunteers, comprising both the acute effects of CBD alone as well as in direct comparison to those induced by THC, and studies that investigated the neural substrates of acute CBD effects in patients with a psychiatric disorder.
This review was conducted in accordance with the Preferred Reporting Items for Systematic Reviews and Meta-Analyses (PRISMA) statement (Moher et al., 2009). PubMed was searched for neuroimaging studies investigating the impact of CBD on human brain function published until May 2020. See for the exact Pubmed search syntax the Supplementary Methods. References were screened for additional relevant studies.
Titles and abstracts were screened blind for eligibility by two authors (AB and JB). Discrepancies were discussed with a third author (MB). Inclusion criteria were: 1) use of neuroimaging techniques, and 2) administration of CBD to human subjects. Reviews and case reports were excluded.
Data extraction included: study information (e.g., title, authors, study design); sample characteristics (mean age, sex, handedness); cannabinoid dose and administration route; time interval between administration and imaging; imaging modality; cognitive task performed during imaging; and degree of sample overlap.
The search strategy yielded 194 studies, of which 15 studies met inclusion criteria. Two studies were found by additional references, resulting in a total of 17 included studies (Figure 1). In total, the current review comprised 115 healthy subjects, 33 individuals at clinical high risk (CHR) for psychosis, 13 patients with a psychotic disorder, 10 patients with anxiety disorder and 17 patients with an autism spectrum disorder.
In healthy subjects, 12 studies reported the acute effects of CBD compared to placebo (Crippa et al., 2004; Borgwardt et al., 2008; Bhattacharyya et al., 2009; Fusar-Poli et al., 2009; Fusar-Poli et al., 2010) or compared to THC (Bhattacharyya et al., 2010; Winton-Brown et al., 2011; Bhattacharyya et al., 2012b; Bhattacharyya et al., 2015; Freeman et al., 2018; Grimm et al., 2018; Wall et al., 2019). In individuals with a psychiatric disorder, five studies assessed the acute effects of CBD compared to placebo (Crippa et al., 2011; Bhattacharyya et al., 2018; Pretzsch et al., 2019; Wilson et al., 2019; O’Neill et al., 2020).
A cluster of eight studies performed different cognitive tasks (i.e., go-no go, verbal learning, emotional processing, visual and auditory processing) using the same sample of healthy subjects (Borgwardt et al., 2008; Bhattacharyya et al., 2009; Fusar-Poli et al., 2009; Bhattacharyya et al., 2010; Fusar-Poli et al., 2010; Winton-Brown et al., 2011; Bhattacharyya et al., 2012b; Bhattacharyya et al., 2015). In addition, the studies of Freeman et al. (2018) and Wall et al. (2019) used an overlapping sample of healthy participants, and those of Bhattacharyya et al. (2018) and Wilson et al. (2019) a similar cohort of CHR individuals. See Tables 1–3 for study characteristics and results of studies included in the current systematic review.
Nine double-blind placebo-controlled studies investigated the acute effects of CBD on brain function of healthy volunteers. One of these studies used Single Photon Emission Computed Tomography (SPECT) measuring regional cerebral blood flow, whereas eight studies applied functional Magnetic Resonance Imaging (fMRI), either at rest or during the performance of a cognitive task (Table 1).
Two studies investigated the acute effects of CBD during resting state (Crippa et al., 2004; Grimm et al., 2018). Crippa et al. (2004) measured cerebral blood flow using 99mTc-ethyl cysteinate dimer (99mTc-ECD) SPECT imaging in 10 healthy male volunteers using a cross-over design (Crippa et al., 2004). Administration of an oral dose of 400 mg CBD enhanced blood flow compared to placebo in an area consisting of the left parahippocampal and fusiform gyrus. Conversely, CBD attenuated blood flow in the left posterior cingulate cortex and in a cluster comprising the left amygdala, hippocampus, uncus and hypothalamus (Crippa et al., 2004). In another resting state study using fMRI to measure connectivity, 16 healthy male volunteers were given placebo, 10 mg oral THC and 600 mg oral CBD using a cross-over study design (Grimm et al., 2018). The striatum was set as a seed region and a whole brain analysis was performed. CBD increased connectivity between the right putamen and three clusters, situated mainly in the right prefrontal cortex (Grimm et al., 2018).
Seven studies performed different cognitive tasks (i.e., go-no go, verbal learning, emotional processing, visual and auditory processing) using the same sample of 15 healthy volunteers (Borgwardt et al., 2008; Bhattacharyya et al., 2009; Fusar-Poli et al., 2009; Fusar-Poli et al., 2010; Winton-Brown et al., 2011; Bhattacharyya et al., 2012b; Bhattacharyya et al., 2015). The authors explored the acute effects of 600 mg CBD, 10 mg THC and placebo on brain activity measured by fMRI using a double-blind cross-over design. During the sessions, ratings of anxiety (STAI), intoxication (AIS), psychotic symptoms (PANNS) and subjective feelings (VAMS) were obtained. The acute effects of CBD in direct comparison to those induced by THC administration are described in the next section.
A go-no go task was used to investigate brain activity during response inhibition and detection of salient stimuli (Borgwardt et al., 2008; Bhattacharyya et al., 2012b). Under conditions of response inhibition, CBD attenuated brain activity compared to placebo in the left posterior insula, left superior temporal gyrus and left transverse temporal gyrus (Borgwardt et al., 2008). During the presentation of a salient relative to a non-salient stimuli CBD attenuated activity in the left medial prefrontal cortex (Bhattacharyya et al., 2012b). In the same salient relative to non-salient stimuli comparison, Bhattacharyya et al. (2015) conducted connectivity analyses with the inferior frontal gyrus, dorsal striatum and posterior hippocampus set as seed regions. Compared to placebo, a CBD decreased connectivity was found between the following areas: the right inferior frontal gyrus and right insula, left cerebellum, left lingual gyrus and left thalamus; the left dorsal striatum and left anterior cingulate and left medial frontal gyrus; the left posterior hippocampus and right parahippocampus, left posterior cingulate gyrus and caudate tail. Conversely, CBD increased connectivity between the following areas: the left dorsal striatum and left body of the caudate nucleus and left inferior frontal gyrus; left posterior hippocampus and left parahippocampus (Bhattacharyya et al., 2015).
The verbal learning task consisted of an encoding block, where participants had to evaluate whether pairs of words fitted well together, and a recall block, during which participants matched the presented with the previously associated word (Bhattacharyya et al., 2009). CBD modulated activation during encoding conditions in the insula, midtemporal gyrus, lingual gyrus, precuneus and precentral gyrus. During recall, CBD modulated activation in the hippocampus. However, none of these findings were statistically significant (Bhattacharyya et al., 2009).
The emotional processing task consisted of a series of faces, including neutral, intermediate and extremely fearful faces (Fusar-Poli et al., 2009). Relative to placebo, administration of CBD did not alter brain activity during the presentation of neutral faces. During the presentation of intermediately fearful faces, CBD attenuated activity bilaterally in the posterior lobe of the cerebellum. During the processing of intensely fearful faces, CBD attenuated activity in the left medial temporal region (amygdala and anterior parahippocampal gyrus), the anterior and posterior cingulate gyri and the right posterior lobe of the cerebellum (Fusar-Poli et al., 2009). In addition, CBD decreased the number of skin conductance response fluctuations, a physiological measure of emotional response. Moreover, this decrease in skin conductance response covaried with the attenuation of activity in both the left amygdala and the anterior cingulate (Fusar-Poli et al., 2009). Based on these results Fusar-Poli et al. (2010) investigated the connectivity between these two regions in the same sample. Compared to placebo, administration of CBD disrupted connectivity between the left anterior cingulate cortex and the left amygdala while viewing fearful faces (Fusar-Poli et al., 2010).
While listening to neutral words, brain activity was increased during CBD relative to placebo in the bilateral temporal cortex, insula, parahippocampal gyrus and hippocampus (Winton-Brown et al., 2011). Conversely, CBD attenuated activity in the left superior temporal gyrus, insula, posterior middle temporal gyrus and supramarginal gyrus. During visual stimulation, CBD increased activity in the right occipital lobe, lingual gyrus, cerebellum and cuneus (Winton-Brown et al., 2011).
In summary, CBD enhanced fronto-striatal connectivity and decreased limbic activity during resting state, and modulated brain activity showing task-specific patterns during different cognitive paradigms. For example, CBD increased activation relative to placebo in the parahippocampus during auditory processing, and reduced activation in this region during the processing of fearful faces. In addition, CBD decreased connectivity between fronto-limbic regions (i.e., anterior cingulate cortex and amygdala) during the processing of fearful faces and enhanced fronto-limbic-striatal connectivity (i.e., inferior frontal gyrus, dorsal striatum and posterior hippocampus) during salience processing.
Seven fMRI studies investigated the acute effects of CBD in direct comparison to those induced by THC, during resting state or a cognitive task. Some studies analysed regions in the brain where CBD and THC showed opposite activity relative to placebo, whereas others directly compared both substances (Table 2).
Grimm et al. (2018) conducted a resting state connectivity analysis on 16 healthy volunteers, where the striatum and frontal regions were set as regions of interest. While CBD enhanced frontal-striatal connectivity, THC did not alter this connectivity significantly, possibly due to low THC plasma concentrations during scanning. Direct comparison between the two substances showed that CBD increased connectivity relative to THC between the right putamen and frontal pole and paracingulate gyrus (Grimm et al., 2018).
In a double-blind, pseudo-randomized, within-subject study, Wall et al. (2019) investigated the effects on the resting-state functional connectivity of two strains of inhaled cannabis, containing THC (8 mg) without or with CBD (10 mg), and placebo in 17 occasional cannabis users. Connectivity analyses were performed to investigate the default mode network (defined as positive connectivity with the posterior cingulate cortex), executive control network (defined as negative connectivity with the posterior cingulate cortex) and salience (defined as positive connectivity with the anterior insula). Both strains of cannabis showed a significant reduction in mean connectivity in the default mode network relative to placebo. In the salience network, cannabis containing both THC and CBD caused a significant increase in connectivity compared to cannabis without CBD, but both strains did not differ significantly from placebo. No significant effects were found within the executive control network (Wall et al., 2019). Significant correlations between the subjective measures of feeling the drug’s effect and brain effects were only found after cannabis without CBD was administered. These correlations involved the posterior cingulate cortex region and the frontal pole region (Wall et al., 2019).
Freeman et al. (2018) investigated the acute effects of inhaled cannabis with and without CBD, while they listened to classical music and scrambled sound, using the same sample of occasional cannabis users as described by Wall et al. (2019). Both types of cannabis increased ratings of wanting to listen to music and enhanced sound perception. Inhalation of cannabis without CBD relative to placebo resulted in a dampened response to music bilaterally in the auditory cortex, right hippocampus, right ventral striatum and right amygdala. Cannabis with CBD did not significantly modulate activity relative to placebo or cannabis without CBD. Across all sessions, activation in the right ventral striatum was correlated with pleasure ratings and response to music. Moreover, this region showed an increased functional connectivity with the bilateral auditory cortex during music relative to scrambled sound. Cannabis with CBD had a greater impact on the functional connectivity between these two regions relative to cannabis without CBD (Freeman et al., 2018).
The other series of four studies performed different cognitive tasks (i.e., go-no go, verbal learning, emotional processing, visual and auditory processing) in a double-blind cross-over design of 600 mg CBD, 10 mg THC and placebo, using the same sample of 15 healthy volunteers as described by Borgwardt et al. (2008).
During an emotional processing task, CBD and THC had opposite effects relative to placebo in the left amygdala, fusiform, and lingual gyri, the lateral prefrontal cortex and the cerebellum (Bhattacharyya et al., 2010). The increased activity in the left amygdala following THC administration covaried with the level of anxiety assessed by the STAI, while the attenuated activity after CBD in the amygdala correlated to its anxiolytic effect measured by the VAMS. Opposite effects on skin conductance response fluctuations were also found following the administration of THC compared to CBD (Bhattacharyya et al., 2010).
During the recall phase of a verbal memory task, CBD enhanced and THC reduced brain activity in the striatum (Bhattacharyya et al., 2010). The reduction in the striatum activity after THC administration correlated with the severity of psychotic symptoms. Furthermore, during the recall phase opposite effects were found in a cluster consisting of the anterior cingulate and medial prefrontal cortex and in the lateral prefrontal cortex (Bhattacharyya et al., 2010).
During response inhibition, CBD increased and THC reduced activity in the left insula, left caudate and bilateral parahippocampal gyrus (Bhattacharyya et al., 2010). During a go-no go task, CBD attenuated and THC increased activity in the right superior, middle, inferior and orbifrontal gyri compared to placebo (Bhattacharyya et al., 2012b). Conversely, in left caudate, putamen, parahippocampal gyrus, thalamus and lingual gyrus, activation was attenuated by THC but augmented by CBD (Bhattacharyya et al., 2012b). Bhattacharyya et al. (2015) conducted a connectivity analyses on the same data with the inferior frontal gyrus, dorsal striatum and posterior hippocampus set as seed regions. CBD and THC modulated functional connectivity between these seeds and clusters in the rest of the brain in opposite direction (Bhattacharyya et al., 2015).
During processing of speech, CBD and THC showed opposite effects relative to placebo in the bilateral temporal cortex, whereas opposite effects were found in the bilateral occipital cortex while viewing a visual checkerboard (Bhattacharyya et al., 2010). A direct comparison of CBD and THC effects revealed significantly reduced activity after THC in the right superior and middle temporal gyrus during processing of speech. During visual processing, THC increased activity relative to CBD in the bilateral lingual and middle occipital gyrus, but reduced activity in several other occipital regions. Mixed effects were reported in the cerebellum (Winton-Brown et al., 2011).
In summary, CBD and THC showed dissonant effects during resting state and during several cognitive tasks. During resting state, CBD enhanced connectivity between fronto-striatal regions compared to THC, and cannabis with both THC and CBD increased connectivity within the salience network compared to cannabis without CBD. THC and CBD showed task-specific opposite effects during emotional processing (fronto-temporal), verbal memory (fronto-striatal), response inhibition (fronto-limbic-striatal), and auditory/visual processing (temporo-occipital).
Five neuroimaging studies reported the acute effects of CBD on brain function in patients with a psychiatric disorder. Three of these studies used fMRI: two in a similar cohort of individuals at clinical high for psychosis and one in a group of patients with established psychosis. One study used SPECT to investigate cerebral blood flow in patients with social anxiety disorder and one study examined metabolite concentrations using proton Magnetic Resonance Spectroscopy (1H-MRS) in patients with autism spectrum disorder (Table 3).
Bhattacharyya et al. (2018) conducted an fMRI double-blind randomized trial on 33 medication-naïve CHR subjects and 19 healthy controls, using the same verbal learning task as described in previous studies (Bhattacharyya et al., 2009; Bhattacharyya et al., 2010). Patients were administered 600 mg CBD or placebo, while healthy controls were not given any drug. During encoding conditions, the group of patients who received placebo (indicative of the at-risk state) showed altered brain activity compared to the healthy control group in clusters involving the frontal gyrus, the insula, claustrum, dorsal striatum, pre- and postcentral gyrus, parietal gyrus, cerebellum, lingual gyrus, subcallosal gyrus, cingulate cortex, precuneus and cuneus. During recall conditions, the group of patients who received placebo showed altered brain activity relative to the healthy control group in clusters comprising the frontal gyrus, insula, cuneus, fusiform, lingual gyrus, posterior cingulate, cerebellum, occipital gyrus, fusiform gyrus, parahippocampal gyrus, midbrain, cerebellum, thalamus and temporal gyrus. A linear comparison across the three groups (patients receiving CBD, patients receiving placebo, and control subjects receiving no drug) revealed several clusters in which CBD showed intermediate activation compared to the placebo and healthy control group. For instance, during encoding, the CBD group showed intermediate activation in clusters encompassing the frontal gyrus, insula, striatum, precentral gyrus, cerebellum, fusiform gyrus, cingulate cortex, subcallosal gyrus and occipital gyrus. During recall, the CBD group showed intermediate activation (relative to the placebo and control group) in clusters comprising the frontal gyrus, insula, striatum, precentral gyrus, cerebellum, fusiform gyrus, cingulate cortex, occipital gyrus, parahippocampal gyrus, midbrain, thalamus and temporal gyrus (Bhattacharyya et al., 2018).
Wilson et al. (2019) conducted a monetary incentive delay task in the same 32 CHR medication-naïve subjects and 19 healthy controls reported by Bhattacharyya et al. (2018). This tasked was used to investigate motivational salience conditions by comparing brain activation during reward and loss relative to neutral anticipation. The group of patients who received placebo showed greater brain activity compared to the healthy control group in clusters encompassing the frontal opercula, insula, parietal operculum, frontal gyri, and temporal gyri. A linear comparison between the three groups revealed intermediate activation in the CBD group (compared to the placebo and control group) in three clusters: the left insula and parietal operculum, left frontal operculum, and left superior frontal gyrus (Wilson et al., 2019).
One fMRI study explored the effects of CBD on patients with established psychosis (O’Neill et al., 2020), where 15 patients on antipsychotic treatment were given 600 mg CBD or placebo in a double-blind, randomized, within-subject design. In this study, 19 healthy participants were scanned but were not given any drugs. During the scanning procedure all participants performed a verbal learning task, the same used in previously described studies (Bhattacharyya et al., 2009; Bhattacharyya et al., 2010; Bhattacharyya et al., 2018). The medial temporal lobe, prefrontal cortex and striatum/pallidum were selected as regions of interest and activation patterns as well as a connectivity analysis were performed (O’Neill et al., 2020). Patients after CBD administration showed a trend level towards a greater decrease in median total PANSS score compared to those receiving placebo. Healthy controls scored better on both encoding and recall of the task compared to patients (after CBD or placebo). Patients under placebo showed increased activation compared to controls in the right inferior frontal gyrus and left inferior and middle frontal gyrus during encoding, while having both increasing and attenuating effects in two different clusters in the left middle frontal gyrus. A linear comparison between the three groups showed that patients under CBD treatment had intermediate activation in several clusters located bilaterally in the inferior frontal gyrus, and the left middle frontal gyrus. The two clusters in the right inferior frontal gyrus were similar to clusters found in the placebo vs. control analysis (O’Neill et al., 2020). During recall, patients under placebo showed increased activation relative to healthy controls in the right middle and inferior frontal gyri and right hippocampus, but decreased activation in the left hippocampus. Similar clusters were found in all of these areas such that CBD had intermediate activation relative to the placebo and control group. Patients under placebo condition displayed increased connectivity between the hippocampus and the right caudate head and left caudate body during recall conditions. CBD had intermediate functional connectivity relative to the other two groups in connections between the hippocampus and right caudate head, left caudate body and left putamen (O’Neill et al., 2020).
Crippa et al. (2011) investigated the acute effect of an oral dose of 400 mg CBD in 10 medication-naïve patients with social anxiety disorder, while using 99mTc-ECD SPECT imaging to measure cerebral blood flow in a within-subject design. Compared to placebo, CBD decreased subjective anxiety and blood flow in a cluster consisting of the left parahippocampal gyrus and hippocampus, but enhanced blood flow in the right posterior cingulate gyrus (Crippa et al., 2011).
Pretsch et al. (2019) investigated the acute effects of 600 mg CBD on 17 patients with autism spectrum disorder and 17 healthy controls. Magnetic resonance spectroscopy was used to measure glutamate and glutamine (Glx) and inhibitory γ-aminobutyric acid and macromolecules (GABA+) levels in two voxels placed in the basal ganglia and dorsomedial prefrontal cortex. Both groups received placebo and CBD. The effect of CBD on Glx levels showed the same pattern in both patients and controls: CBD increased Glx levels relative to baseline in the basal ganglia and decreased Glx levels in the prefrontal cortex. However, the effects of CBD on GABA + levels showed an opposite pattern between groups: GABA + levels in both the basal ganglia and prefrontal cortex increased in the control group after CBD administration but decreased in the patients with autism (Pretzsch et al., 2019).
In summary, acute brain effects after CBD administration were different in patients with a psychiatric disorder compared to healthy controls. In subjects at CHR for psychosis, CBD administration showed intermediate activity in brain areas involved in memory and reward processing compared to placebo and healthy controls. An intermediate activity was also reported in patients with psychosis after CBD administration during a memory task. CBD also modified limbic activity in subjects with social anxiety, and showed similar (glutamate) and opposite (GABA) patterns of metabolite levels in patients with autism compared to healthy controls.
The current review provides a systematic literature overview of studies that investigated the acute effects of CBD on the human brain of healthy volunteers and individuals diagnosed with a psychiatric disorder. Overall, studies in healthy subjects showed that CBD modulated brain activity and had opposite effects when compared to THC in resting state and during several cognitive paradigms (i.e., salience, emotional, memory, response inhibition, auditory/visual processing), following task-specific activation patterns. Acute CBD administration also modulated brain activity in patients with psychiatric disorders by 1) showing intermediate activity compared to placebo and healthy controls in individuals at CHR and with established psychosis, 2) engaging with resting limbic activity in subjects with anxiety disorders, and 3) exhibiting similar (glutamate) and opposite (GABA) metabolite levels in patients with autism compared to healthy controls.
The acute administration of CBD in healthy volunteers modulated networks relevant for psychiatric disorders during resting state and several cognitive tasks, such as fronto-striatal and fronto-limbic circuitry. Fronto-striatal connectivity was enhanced after CBD administration during resting state (Grimm et al., 2018) and activity increased during salience processing (Bhattacharyya et al., 2015). Interestingly, lower functional connectivity in fronto-striatal circuitry has been reported in psychosis, and has been associated with more severe positive symptoms (Fornito et al., 2013). In addition, CBD decreased fronto-limbic activity during resting state (Crippa et al., 2004) and emotional processing (Fusar-Poli et al., 2010). Functional fMRI studies have shown activation of limbic areas in anxiety disorders (e.g., during panic attacks or panic anticipation) (Pfleiderer et al., 2007; Dresler et al., 2013). Based on a mechanistic account of these networks, these findings suggest that CBD might prove useful as treatment by restoring imbalanced networks in these and probably other neurological (Nenert et al., 2020) and psychiatric conditions, such as substance use disorders (Freeman et al., 2020). Regarding the last, converging preclinical and clinical evidence have shown promising effects of CBD on reducing craving, negative affect and motivation for substance use (Chye et al., 2019; Hurd et al., 2019; Freeman et al., 2020; Spanagel, 2020), phenomena associated with fronto-striatal and limbic network disbalances (Koob and Volkow, 2016; Volkow and Boyle, 2018).
Along these lines, CBD also showed opposite effects compared to THC during resting state and several cognitive paradigms in healthy volunteers. It is known that THC has pro-psychotic and anxiogenic properties, particularly evident with high potency cannabis strains (rich in THC) and at high doses (Campeny et al., 2020; Van der Steur et al., 2020). Opposite neurophysiological effects were reported on prefrontal, striatal and limbic areas, which are relevant neural substrates of psychosis and anxiety, and during several cognitive processes, such as salience, verbal memory, response inhibition, emotional processing and auditory/visual processing. Importantly, striatum activity correlated with severity of psychotic symptoms after THC (Bhattacharyya et al., 2010; Bhattacharyya et al., 2012b), and divergent amygdala activity correlated with severity of anxiety after CBD and THC (Bhattacharyya et al., 2010). These opposite brain effects may therefore underlie the neural basis for the antipsychotic and anxiolytic properties of CBD, and suggest that CBD might be able to counterbalance THC induced effects (Colizzi and Bhattacharyya, 2017). However, CBD concentrations needed to offset the effects of THC in healthy individuals are still unclear, as CBD might also have different effects when administered at different doses (Solowij et al., 2019).
Acute CBD administration also affected brain networks of subjects diagnosed with a psychiatric disorder. In individuals at CHR for psychosis, CBD showed intermediate activity compared to patients receiving placebo and healthy subjects in regions involved in reward and salience processing (Bhattacharyya et al., 2018; Wilson et al., 2019). A similar intermediate activity was reported in subjects with established psychosis during a memory task (O’Neill et al., 2020). These findings are consistent with the enhanced activity observed in fronto-striatal regions in healthy subjects after CBD (Bhattacharyya et al., 2015; Grimm et al., 2018). Altogether, these findings suggest that CBD could contribute to normalise disbalanced fronto-striatal activity in patients at CHR or with established psychosis. In addition, Crippa and colleagues showed that CBD reduced cerebral blood flow in (para) limbic areas (i.e., hippocampus, parahippocampal and inferior temporal gyrus) in subjects with social anxiety (Crippa et al., 2011). This is congruent with decreased fronto-limbic activity in healthy individuals reported after CBD (Crippa et al., 2004; Fusar-Poli et al., 2010), and suggest that the anxiolytic effects of CBD may be related to the capacity of this compound to modify brain activity in (para) limbic areas (Crippa et al., 2011). Finally, a spectroscopy study in autism spectrum disorder and healthy controls showed similar glutamate (i.e., increased in basal ganglia, and decreased in prefrontal cortex in both groups) and opposite GABA (i.e., decreased levels in patients and increased in controls in both basal ganglia and prefrontal cortex) effects after CBD administration (Pretzsch et al., 2019). This study adds to preclinical evidence that CBD may modulate the activity of other neurotransmitters, even after a single dose (Crippa et al., 2018). This has implications for the homeostasis of other neurotransmitter systems, such as glutamate, GABA and dopamine. However, the underlying molecular mechanisms explaining the relationship between CBD and other neurotransmitters needs further study.
One of these molecular mechanisms may involve the ability of CBD to directly inhibit the reuptake of anandamide. This endocannabinoid has shown anti-inflammatory activity (Pisanti et al., 2017), and its increase after CBD has been related to antipsychotic effects (Leweke et al., 2012; Rohleder et al., 2016). Because endocannabinoids act as retrograde messengers, it has been hypothesized that increased endocannabinoid concentrations after CBD may attenuate presynaptic release of GABA and glutamate, as well as stabilise dopamine neurotransmission (Gururajan and Malone, 2016). In addition, most of the reported effects after CBD administration occurred in brain areas rich in CB1 receptors (Burns et al., 2007). Chronic cannabis use is associated with reductions in endogenous cannabinoids and down-regulation of CB1 receptors (Hirvonen et al., 2012; Morgan et al., 2013), while CBD antagonistic effects could be related to modulation of cannabinoid receptors by binding to a distinct allosteric site (Laprairie et al., 2015). Given that CBD may attenuate THC effects, it has also been speculated that CBD may be able to prevent down-regulation of CB1 receptors on the long-term, and thus decrease the risk of developing psychosis and/or substance use disorders (Wall et al., 2019). Other possible mechanisms of action of CBD involve its agonist activity towards 5HT1A receptors (Soares and Campos, 2017), partial agonist activity on dopamine D2 receptors (Seeman, 2016), and the activation of vanilloid receptor 1, a non-selective calcium channel, facilitating glutamate pre-synaptic release (Campos et al., 2012).
This review must be read with a series of limitations taken into account. First, included papers often employed different methodologies (e.g., imaging method, route of administration, applied doses), although we used strict inclusion and exclusion criteria for article selection to avoid excessive heterogeneity between studies. For example, whereas most studies administered CBD and THC as individual cannabinoid compounds in separate sessions (Borgwardt et al., 2008; Bhattacharyya et al., 2012b; Grimm et al., 2018), some studies examined the impact of CBD on brain function by comparing effects of cannabis containing THC only to cannabis with both THC and CBD (Freeman et al., 2018; Wall et al., 2019). Regarding differences in applied cognitive paradigms, it is important to note that the described effects on brain activity might depend on the nature of the task used or stimuli presented, as different tasks might provoke distinct brain activity patterns. For instance, whereas memory paradigms may heavily rely on recruitment of temporal and prefrontal areas (Bhattacharyya et al., 2009; Bhattacharyya et al., 2018; O’Neill et al., 2020), emotional and salience processing mainly involve limbic activation (Fusar-Poli et al., 2009; Bhattacharyya et al., 2012b). One final methodological aspect that should be taken into account is that the clinical and brain effects of CBD might be different depending on age and illness progression (Di Marzo et al., 2015; Batalla et al., 2019; Colizzi et al., 2020), or be influenced by the concomitant use of medication (e.g., antipsychotics) or drugs of abuse. However, the within-subject design of the studies where concomitant use of medication or cannabis was allowed probably mitigated these confounding effects (Table 3). Second, most of the included studies used overlapping samples of mainly male healthy subjects or patients with psychiatric disorders to explore the effects of CBD. Although the studies reviewed herein offer a consistent picture indicating that CBD has modulatory effects over neural networks relevant for psychosis, anxiety and addiction, this highlights the need for replication of findings in independent and larger cohorts also including female subjects.
Suggestions can be made for future research on the impact of CBD on brain function. First, because all studies included in the current review examined the acute effects of CBD administration, future research should focus on longer-term CBD treatment of patients with a psychiatric disorder in combination with neuroimaging assessments, in order to elucidate neural substrates underlying the therapeutic effects of CBD. In this respect, two excellent examples of studies nearing completion are 1) 3 weeks CBD treatment of individuals at CHR for psychosis (Institute of Psychiatry, King’s College London), and 2) 4-week add-on CBD treatment of early-onset patients with a psychotic disorder (University Medical Center Utrecht, Netherlands), both in combination with baseline and follow-up functional MRI and 1H-MRS techniques. Second, because the clinical response to CBD has been shown to differ between patients (Batalla et al., 2019), future studies could also apply neuroimaging techniques to contribute to identification of those patients that may particularly benefit from CBD treatment.
In conclusion, neuroimaging studies have shown that CBD modulates brain activity and connectivity in neural systems relevant for psychosis and anxiety, possibly reflecting CBD’s therapeutic effects. Future studies should consider replication of findings and enlarge the inclusion of psychiatric patients, combining longer-term CBD treatment with neuroimaging assessments.
The original contributions presented in the study are included in the article/Supplementary Material, further inquiries can be directed to the corresponding author.
AB and JB performed the systematic search and drafted the manuscript, and MB screened potentially eligible publications and critically revised the manuscript for important intellectual content. AB, JB, AP, and MB provided critical revisions for important intellectual content and significantly contributed to the manuscript. All authors have read and agreed to the published version of the manuscript.
The authors declare that the research was conducted in the absence of any commercial or financial relationships that could be construed as a potential conflict of interest.
The Supplementary Material for this article can be found online at: https://www.frontiersin.org/articles/10.3389/fphar.2020.618184/full#supplementary-material.
AIS, intoxication scale; CB1, cannabinoid receptor 1; CB2, cannabinoid receptor 2; CBD, cannabidiol; CHR, clinical high risk; fMRI, functional magnetic resonance imaging; GABA, γ-aminobutyric acid; GPR18, G protein-coupled receptor 18; GPR55, G protein-coupled receptor 55; Glx, glutamate and glutamine; H-MRS, proton magnetic resonance spectroscopy; 5HT1A, serotonin type 1A receptor; PANNS, positive and negative syndrome scale; PPARgamma, peroxisome proliferator-activated receptor gamma; PRISMA, preferred reporting items for systematic reviews and meta-analyses; SPECT, single photon emission computed tomography; STAI, state-trait anxiety inventory; 99mTc-ECD, 99mTc-ethyl cysteinate dimer; THC, tetrahydrocannabinol; TRPV1, transient receptor potential vanilloid 1; VAMS, visual analog mood scales.
Batalla, A., Crippa, J. A., Busatto, G. F., Guimaraes, F. S., Zuardi, A. W., Valverde, O., et al. (2014). Neuroimaging studies of acute effects of THC and CBD in humans and animals: a systematic review. Curr. Pharmaceut. Des. 20, 2168–2185. doi:10.2174/13816128113199990432
Batalla, A., Janssen, H., Gangadin, S. S., and Bossong, M. G. (2019). The potential of cannabidiol as a treatment for psychosis and addiction: who benefits most? A systematic review. J. Clin. Med. 8, 1058. doi:10.3390/jcm8071058
Bhattacharyya, S., Atakan, Z., Martin-Santos, R., Crippa, J. A., and Mcguire, P. K. (2012a). Neural mechanisms for the cannabinoid modulation of cognition and affect in man: a critical review of neuroimaging studies. Curr. Pharmaceut. Des. 18, 5045–5054. doi:10.2174/138161212802884636
Bhattacharyya, S., Crippa, J. A., Allen, P., Martin-Santos, R., Borgwardt, S., Fusar-Poli, P., et al. (2012b). Induction of psychosis by Δ9-tetrahydrocannabinol reflects modulation of prefrontal and striatal function during attentional salience processing. Arch. Gen. Psychiatr. 69, 27–36. doi:10.1001/archgenpsychiatry.2011.161
Bhattacharyya, S., Falkenberg, I., Martin-Santos, R., Atakan, Z., Crippa, J. A., Giampietro, V., et al. (2015). Cannabinoid modulation of functional connectivity within regions processing attentional salience, Neuropsychopharmacology. 40, 1343–1352. doi:10.1038/npp.2014.258
Bhattacharyya, S., Fusar-Poli, P., Borgwardt, S., Martin-Santos, R., Nosarti, C., O’carroll, C., et al. (2009). Modulation of mediotemporal and ventrostriatal function in humans by Delta9-tetrahydrocannabinol: a neural basis for the effects of Cannabis sativa on learning and psychosis. Arch. Gen. Psychiatr. 66, 442–451. doi:10.1001/archgenpsychiatry.2009.17
Bhattacharyya, S., Morrison, P. D., Fusar-Poli, P., Martin-Santos, R., Borgwardt, S., Winton-Brown, T., et al. (2010). Opposite effects of delta-9-tetrahydrocannabinol and cannabidiol on human brain function and psychopathology, Neuropsychopharmacology. 35, 764–774. doi:10.1038/npp.2009.184
Bhattacharyya, S., Wilson, R., Appiah-Kusi, E., O’neill, A., Brammer, M., Perez, J., et al. (2018). Effect of cannabidiol on medial temporal, midbrain, and striatal dysfunction in people at clinical high risk of psychosis: a randomized clinical trial. JAMA Psychiatry. 75, 1107–1117. doi:10.1001/jamapsychiatry.2018.2309
Bisogno, T., Hanus, L., De Petrocellis, L., Tchilibon, S., Ponde, D. E., Brandi, I., et al. (2001). Molecular targets for cannabidiol and its synthetic analogues: effect on vanilloid VR1 receptors and on the cellular uptake and enzymatic hydrolysis of anandamide. Br. J. Pharmacol. 134, 845–852. doi:10.1038/sj.bjp.0704327
Blessing, E. M., Steenkamp, M. M., Manzanares, J., and Marmar, C. R. (2015). Cannabidiol as a potential treatment for anxiety disorders. Neurotherapeutics. 12, 825–836. doi:10.1007/s13311-015-0387-1
Borgwardt, S. J., Allen, P., Bhattacharyya, S., Fusar-Poli, P., Crippa, J. A., Seal, M. L., et al. (2008). Neural basis of Delta-9-tetrahydrocannabinol and cannabidiol: effects during response inhibition. Biol. Psychiatr. 64, 966–973. doi:10.1016/j.biopsych.2008.05.011
Burns, H. D., Van Laere, K., Sanabria-Bohórquez, S., Hamill, T. G., Bormans, G., Eng, W. S., et al. (2007). [18F]MK-9470, a positron emission tomography (PET) tracer for in vivo human PET brain imaging of the cannabinoid-1 receptor. Proc. Natl. Acad. Sci. U.S.A. 104, 9800–9805. doi:10.1073/pnas.0703472104
Campeny, E., Lopez-Pelayo, H., Nutt, D., Blithikioti, C., Oliveras, C., Nuno, L., et al. (2020). The blind men and the elephant: systematic review of systematic reviews of cannabis use related health harms. Eur. Neuropsychopharmacol. 33, 1–35.
Campos, A. C., Moreira, F. A., Gomes, F. V., Del Bel, E. A., and Guimarães, F. S. (2012). Multiple mechanisms involved in the large-spectrum therapeutic potential of cannabidiol in psychiatric disorders. Philos. Trans. R. Soc. Lond. B Biol. Sci. 367, 3364–3378. doi:10.1098/rstb.2011.0389
Chye, Y., Christensen, E., Solowij, N., and Yücel, M. (2019). The endocannabinoid system and cannabidiol’s promise for the treatment of substance use disorder. Front. Psychiatr. 10, 63. doi:10.3389/fpsyt.2019.00063
Colizzi, M., and Bhattacharyya, S. (2017). Does cannabis composition matter? Differential effects of delta-9-tetrahydrocannabinol and cannabidiol on human cognition. Curr. Addict. Rep. 4, 62–74. doi:10.1007/s40429-017-0142-2
Colizzi, M., Ruggeri, M., and Bhattacharyya, S. (2020). Unraveling the intoxicating and therapeutic effects of cannabis ingredients on psychosis and cognition. Front. Psychol. 11, 833. doi:10.3389/fpsyg.2020.00833
Crippa, J. A., Derenusson, G. N., Ferrari, T. B., Wichert-Ana, L., Duran, F. L., Martin-Santos, R., et al. (2011). Neural basis of anxiolytic effects of cannabidiol (CBD) in generalized social anxiety disorder: a preliminary report. J. Psychopharmacol. 25, 121–130. doi:10.1177/0269881110379283
Crippa, J. A., Guimaraes, F. S., Campos, A. C., and Zuardi, A. W. (2018). Translational investigation of the therapeutic potential of cannabidiol (CBD): toward a new age. Front. Immunol. 9, 2009. doi:10.3389/fimmu.2018.02009
Crippa, J. A., Zuardi, A. W., Garrido, G. E., Wichert-Ana, L., Guarnieri, R., Ferrari, L., et al. (2004). Effects of cannabidiol (CBD) on regional cerebral blood flow, Neuropsychopharmacology. 29, 417–426. doi:10.1038/sj.npp.1300340
Di Marzo, V., Stella, N., and Zimmer, A. (2015). Endocannabinoid signalling and the deteriorating brain. Nat. Rev. Neurosci. 16, 30–42. doi:10.1038/nrn3876
Dresler, T., Guhn, A., Tupak, S. V., Ehlis, A. C., Herrmann, M. J., Fallgatter, A. J., et al. (2013). Revise the revised? New dimensions of the neuroanatomical hypothesis of panic disorder. J. Neural. Transm. 120, 3–29. doi:10.1007/s00702-012-0811-1
Fornito, A., Harrison, B. J., Goodby, E., Dean, A., Ooi, C., Nathan, P. J., et al. (2013). Functional dysconnectivity of corticostriatal circuitry as a risk phenotype for psychosis. JAMA Psychiatry. 70, 1143–1151. doi:10.1001/jamapsychiatry.2013.1976
Freeman, T. P., Hindocha, C., Baio, G., Shaban, N. D. C., Thomas, E. M., Astbury, D., et al. (2020). Cannabidiol for the treatment of cannabis use disorder: a phase 2a, double-blind, placebo-controlled, randomised, adaptive Bayesian trial. Lancet Psychiatry. 7, 865–874. doi:10.1016/S2215-0366(20)30290-X
Freeman, T. P., Hindocha, C., Green, S. F., and Bloomfield, M. A. P. (2019). Medicinal use of cannabis based products and cannabinoids. BMJ. 365, l1141. doi:10.1136/bmj.l1141
Freeman, T. P., Pope, R. A., Wall, M. B., Bisby, J. A., Luijten, M., Hindocha, C., et al. (2018). Cannabis dampens the effects of music in brain regions sensitive to reward and emotion. Int. J. Neuropsychopharmacol. 21, 21–32. doi:10.1093/ijnp/pyx082
Fusar-Poli, L., Cavone, V., Tinacci, S., Concas, I., Petralia, A., Signorelli, M. S., et al. (2020). Cannabinoids for people with ASD: a systematic review of published and ongoing studies. Brain Sci. 10, 572. doi:10.3390/brainsci10090572
Fusar-Poli, P., Allen, P., Bhattacharyya, S., Crippa, J. A., Mechelli, A., Borgwardt, S., et al. (2010). Modulation of effective connectivity during emotional processing by Delta 9-tetrahydrocannabinol and cannabidiol. Int. J. Neuropsychopharmacol. 13, 421–432. doi:10.1017/S1461145709990617
Fusar-Poli, P., Crippa, J. A., Bhattacharyya, S., Borgwardt, S. J., Allen, P., Martin-Santos, R., et al. (2009). Distinct effects of {delta}9-tetrahydrocannabinol and cannabidiol on neural activation during emotional processing. Arch. Gen. Psychiatry. 66, 95–105. doi:10.1001/archgenpsychiatry.2008.519
Grimm, O., Löffler, M., Kamping, S., Hartmann, A., Rohleder, C., Leweke, M., et al. (2018). Probing the endocannabinoid system in healthy volunteers: cannabidiol alters fronto-striatal resting-state connectivity. Eur. Neuropsychopharmacol. 28, 841–849. doi:10.1016/j.euroneuro.2018.04.004
Gururajan, A., and Malone, D. T. (2016). Does cannabidiol have a role in the treatment of schizophrenia? Schizophr. Res. 176, 281–290. doi:10.1016/j.schres.2016.06.022
Hirvonen, J., Goodwin, R. S., Li, C. T., Terry, G. E., Zoghbi, S. S., Morse, C., et al. (2012). Reversible and regionally selective downregulation of brain cannabinoid CB1 receptors in chronic daily cannabis smokers. Mol. Psychiatry. 17, 642–649. doi:10.1038/mp.2011.82
Hurd, Y. L., Spriggs, S., Alishayev, J., Winkel, G., Gurgov, K., Kudrich, C., et al. (2019). Cannabidiol for the reduction of cue-induced craving and anxiety in drug-abstinent individuals with heroin use disorder: a double-blind randomized placebo-controlled trial. Am. J. Psychiatry. 176, 911–922. doi:10.1176/appi.ajp.2019.18101191
Iseger, T. A., and Bossong, M. G. (2015). A systematic review of the antipsychotic properties of cannabidiol in humans. Schizophr. Res. 162, 153–161. doi:10.1016/j.schres.2015.01.033
Jones, N. A., Hill, A. J., Smith, I., Bevan, S. A., Williams, C. M., Whalley, B. J., et al. (2010). Cannabidiol displays antiepileptiform and antiseizure properties in vitro and in vivo. J. Pharmacol. Exp. Therapeut. 332, 569–577. doi:10.1124/jpet.109.159145
Koob, G. F., and Volkow, N. D. (2016). Neurobiology of addiction: a neurocircuitry analysis. Lancet Psychiatry. 3, 760–773. doi:10.1016/S2215-0366(16)00104-8
Lally, J., and Maccabe, J. H. (2015). Antipsychotic medication in schizophrenia: a review. Br. Med. Bull. 114, 169–179. doi:10.1093/bmb/ldv017
Laprairie, R. B., Bagher, A. M., Kelly, M. E., and Denovan-Wright, E. M. (2015). Cannabidiol is a negative allosteric modulator of the cannabinoid CB1 receptor. Br. J. Pharmacol. 172, 4790–4805. doi:10.1111/bph.13250
Leucht, S., Cipriani, A., Spineli, L., Mavridis, D., Orey, D., Richter, F., et al. (2013). Comparative efficacy and tolerability of 15 antipsychotic drugs in schizophrenia: a multiple-treatments meta-analysis. Lancet. 382, 951–962. doi:10.1016/S0140-6736(13)60733-3
Leweke, F. M., Giuffrida, A., Koethe, D., Schreiber, D., Nolden, B. M., Kranaster, L., et al. (2007). Anandamide levels in cerebrospinal fluid of first-episode schizophrenic patients: impact of cannabis use. Schizophr. Res. 94, 29–36. doi:10.1016/j.schres.2007.04.025
Leweke, F. M., Piomelli, D., Pahlisch, F., Muhl, D., Gerth, C. W., Hoyer, C., et al. (2012). Cannabidiol enhances anandamide signaling and alleviates psychotic symptoms of schizophrenia. Transl. Psychiatry. 2, e94. doi:10.1038/tp.2012.15
Martín-Santos, R., Fagundo, A. B., Crippa, J. A., Atakan, Z., Bhattacharyya, S., Allen, P., et al. (2010). Neuroimaging in cannabis use: a systematic review of the literature. Psychol. Med. 40, 383–398. doi:10.1017/S0033291709990729
Minichino, A., Senior, M., Brondino, N., Zhang, S. H., Godwlewska, B. R., Burnet, P. W. J., et al. (2019). Measuring disturbance of the endocannabinoid system in psychosis: a systematic review and meta-analysis. JAMA Psychiatry. 76, 914–923. doi:10.1001/jamapsychiatry.2019.0970
Moher, D., Liberati, A., Tetzlaff, J., and Altman, D. G. (2009). Reprint--preferred reporting items for systematic reviews and meta-analyses: the PRISMA statement. Phys. Ther. 89, 873–880. doi:10.1093/ptj/89.9.873
Morgan, C. J., Page, E., Schaefer, C., Chatten, K., Manocha, A., Gulati, S., et al. (2013). Cerebrospinal fluid anandamide levels, cannabis use and psychotic-like symptoms. Br. J. Psychiatry. 202, 381–382. doi:10.1192/bjp.bp.112.121178
Nenert, R., Allendorfer, J. B., Bebin, E. M., Gaston, T. E., Grayson, L. E., Houston, J. T., et al. (2020). Cannabidiol normalizes resting-state functional connectivity in treatment-resistant epilepsy. Epilepsy Behav. 112, 107297. doi:10.1016/j.yebeh.2020.107297
O’neill, A., Wilson, R., Blest-Hopley, G., Annibale, L., Colizzi, M., Brammer, M., et al. (2020). Normalization of mediotemporal and prefrontal activity, and mediotemporal-striatal connectivity, may underlie antipsychotic effects of cannabidiol in psychosis. Psychol. Med. 2020, 1–11. doi:10.1002/central/CN-02086740/full
Pertwee, R. G. (2008). Ligands that target cannabinoid receptors in the brain: from THC to anandamide and beyond. Addiction Biol. 13, 147–159. doi:10.1111/j.1369-1600.2008.00108.x
Pfleiderer, B., Zinkirciran, S., Arolt, V., Heindel, W., Deckert, J., and Domschke, K. (2007). fMRI amygdala activation during a spontaneous panic attack in a patient with panic disorder. World J. Biol. Psychiatr. 8, 269–272. doi:10.1080/15622970701216673
Pisanti, S., Malfitano, A. M., Ciaglia, E., Lamberti, A., Ranieri, R., Cuomo, G., et al. (2017). Cannabidiol: state of the art and new challenges for therapeutic applications. Pharmacol. Ther. 175, 133–150. doi:10.1016/j.pharmthera.2017.02.041
Poleg, S., Golubchik, P., Offen, D., and Weizman, A. (2019). Cannabidiol as a suggested candidate for treatment of autism spectrum disorder. Prog. Neuropsychopharmacol. Biol. Psychiatry. 89, 90–96. doi:10.1016/j.pnpbp.2018.08.030
Pretzsch, C. M., Freyberg, J., Voinescu, B., Lythgoe, D., Horder, J., Mendez, M. A., et al. (2019). Effects of cannabidiol on brain excitation and inhibition systems; a randomised placebo-controlled single dose trial during magnetic resonance spectroscopy in adults with and without autism spectrum disorder. Neuropsychopharmacology. 44, 1398–1405. doi:10.1038/s41386-019-0333-8
Rohleder, C., Müller, J. K., Lange, B., and Leweke, F. M. (2016). Cannabidiol as a potential new type of an antipsychotic. A critical review of the evidence. Front. Pharmacol. 7, 422. doi:10.3389/fphar.2016.00422
Samara, M. T., Nikolakopoulou, A., Salanti, G., and Leucht, S. (2019). How many patients with schizophrenia do not respond to antipsychotic drugs in the short term? An analysis based on individual patient data from randomized controlled trials. Schizophr. Bull. 45, 639–646. doi:10.1093/schbul/sby095
Seeman, P. (2016). Cannabidiol is a partial agonist at dopamine D2High receptors, predicting its antipsychotic clinical dose. Transl. Psychiatry. 6, e920. doi:10.1038/tp.2016.195
Soares, V. P., and Campos, A. C. (2017). Evidences for the anti-panic actions of cannabidiol. Curr. Neuropharmacol. 15, 291–299. doi:10.2174/1570159x14666160509123955
Solowij, N., Broyd, S., Greenwood, L. M., Van Hell, H., Martelozzo, D., Rueb, K., et al. (2019). A randomised controlled trial of vaporised Delta(9)-tetrahydrocannabinol and cannabidiol alone and in combination in frequent and infrequent cannabis users: acute intoxication effects. Eur. Arch. Psychiatry Clin. Neurosci. 269, 17–35. doi:10.1007/s00406-019-00978-2
Spanagel, R. (2020). Cannabinoids and the endocannabinoid system in reward processing and addiction: from mechanisms to interventions. Dialogues Clin. Neurosci. 22, 241–250. doi:10.31887/DCNS.2020.22.3/rspanagel
Thomas, A., Baillie, G. L., Phillips, A. M., Razdan, R. K., Ross, R. A., and Pertwee, R. G. (2007). Cannabidiol displays unexpectedly high potency as an antagonist of CB1 and CB2 receptor agonists in vitro. Br. J. Pharmacol. 150, 613–623. doi:10.1038/sj.bjp.0707133
Van der Steur, S. J., Batalla, A., and Bossong, M. G. (2020). Factors moderating the association between cannabis use and psychosis risk: a systematic review. Brain Sci. 10, 97. doi:10.3390/brainsci10020097
Volkow, N. D., and Boyle, M. (2018). Neuroscience of addiction: relevance to prevention and treatment. Am. J. Psychiatr. 175, 729–740. doi:10.1176/appi.ajp.2018.17101174
Wall, M. B., Pope, R., Freeman, T. P., Kowalczyk, O. S., Demetriou, L., Mokrysz, C., et al. (2019). Dissociable effects of cannabis with and without cannabidiol on the human brain’s resting-state functional connectivity. J. Psychopharmacol. 33, 822–830. doi:10.1177/0269881119841568
Weinstein, A., Livny, A., and Weizman, A. (2016). Brain imaging studies on the cognitive, pharmacological and neurobiological effects of cannabis in humans: evidence from studies of adult users. Curr. Pharmaceut. Des. 22, 6366–6379. doi:10.2174/1381612822666160822151323
Wilson, R., Bossong, M. G., Appiah-Kusi, E., Petros, N., Brammer, M., Perez, J., et al. (2019). Cannabidiol attenuates insular dysfunction during motivational salience processing in subjects at clinical high risk for psychosis. Transl. Psychiatry. 9, 203. doi:10.1038/s41398-019-0534-2
Keywords: cannabidiol, delta9-tetrahydrocannabinol, Cannabis (marijuana), neuroimaging, functional MRI
Citation: Batalla A, Bos J, Postma A and Bossong MG (2021) The Impact of Cannabidiol on Human Brain Function: A Systematic Review. Front. Pharmacol. 11:618184. doi: 10.3389/fphar.2020.618184
Received: 16 October 2020; Accepted: 17 December 2020;
Published: 21 January 2021.
Edited by:
Francisco Navarrete Rueda, Miguel Hernández University of Elche, SpainReviewed by:
Olga Valverde, Pompeu Fabra University, SpainCopyright © 2021 Batalla, Bos, Postma and Bossong. This is an open-access article distributed under the terms of the Creative Commons Attribution License (CC BY). The use, distribution or reproduction in other forums is permitted, provided the original author(s) and the copyright owner(s) are credited and that the original publication in this journal is cited, in accordance with accepted academic practice. No use, distribution or reproduction is permitted which does not comply with these terms.
*Correspondence: Matthijs G. Bossong, bS5ib3Nzb25nQHVtY3V0cmVjaHQubmw=
Disclaimer: All claims expressed in this article are solely those of the authors and do not necessarily represent those of their affiliated organizations, or those of the publisher, the editors and the reviewers. Any product that may be evaluated in this article or claim that may be made by its manufacturer is not guaranteed or endorsed by the publisher.
Research integrity at Frontiers
Learn more about the work of our research integrity team to safeguard the quality of each article we publish.