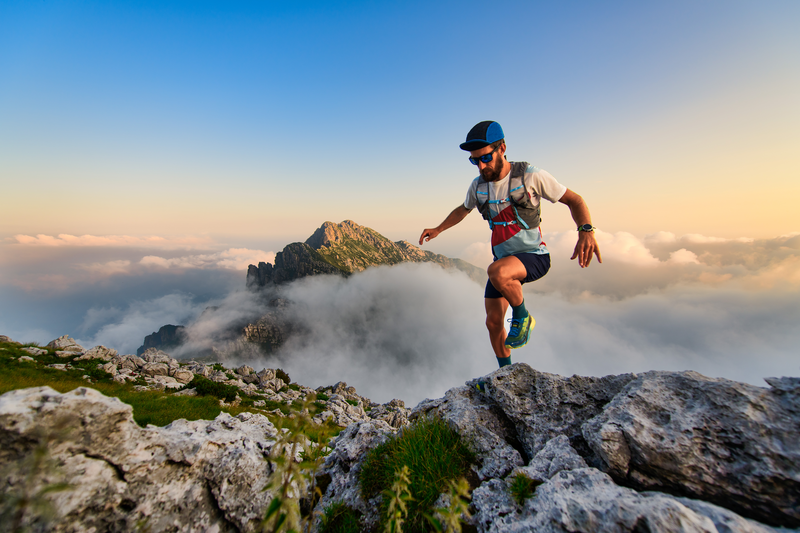
94% of researchers rate our articles as excellent or good
Learn more about the work of our research integrity team to safeguard the quality of each article we publish.
Find out more
ORIGINAL RESEARCH article
Front. Pharmacol. , 21 January 2021
Sec. Ethnopharmacology
Volume 11 - 2020 | https://doi.org/10.3389/fphar.2020.600219
Ilex kudingcha C.J. Tseng tea and insect tea, as traditional Chinese teas, are favored for their original craftsmanship, unique flavor, and biological functionality. In this study, ultra high-performance liquid chromatography-triple quadrupole mass spectrometry (UHPLC-QqQ-MS) was used to analyze the bioactive components of the extracts of Ilex kudingcha and insect tea, and D-galactose-induced aging mice were used to compare the in vivo anti-aging effects of Ilex kudingcha and insect tea extracts. The results were remarkable, UHPLC-QqQ-MS analysis showed that ITP contains 29 ingredients, while IKDCP contains 26 ingredients. However, due to the large differences in the content of the main chemical components in IKDCP and ITP, the effects are equally different. At the same time, the in vivo research results suggesting that the anti-aging effects of IKDCP and ITP (500 mg/kg) include the regulation of viscera indices of major organs; improvement in liver, skin, and spleen tissue morphology; decreased production of inflammatory cytokines; up regulation of SOD, CAT, GSH, GSH-PX, and T-AOC and down regulation of NO and MDA levels in serum and liver tissue; reductions in the concentration of pro-inflammatory factors, and increases in the concentration of anti-inflammatory factor. RT-qPCR and western blot assay also showed that IKDCP and ITP affect anti-aging by regulating the gene and protein expression of GSH-PX, GSH1, SOD1, SOD2, and CAT. The overall results indicate that ITP is more effective in treating oxidative damage in aging mice induced by D-galactose. Thus, ITP appears to be an effective functional drink owing to its rich nutritional components and anti-aging activities.
Aging is a comprehensive and complex physiological and pathological process of the decline of body molecules, cells, organs, and other functions, and is the result of multiple factors (Finkel, 2000). At present, the mechanism of aging is unclear. The most widely accepted theory is that of free radicals, which was first proposed by Harman in 1956 (Harman, 1956), who noted that free radicals are always generated in the body. Under normal circumstances, the body is located in a dynamic equilibrium that continuously generates and scavenges free radicals. With the increase in age, the amount of oxygen free radicals in the body accumulates, and the body is not in equilibrium, which will destroy the cell structure, and cause lipid peroxidation and cross-linking of protein and nucleic acid molecules. In addition, problems such as decreased biological enzymatic activity as well as DNA gene mutations or abnormal replication occur (Clement et al., 2019; Sánchez-Rodríguez et al., 2019). Those processes interfere with the normal metabolic activities of the human body, cause diseases, and accelerate human aging.
Based on the physiological characteristics of various experimental animals, establishing animal models to emulate clinical aging symptoms has become an important method for studying aging mechanisms and evaluating anti-aging drugs (Das et al., 2001). At present, although the etiology and mechanism of aging have not been clarified, a variety of aging animal models have been introduced in the study of anti-aging pathogenesis. Most of these models are designed according to modern aging theory, and can be roughly divided into ozone damage aging models, SAM mouse rapid aging models, thymic aging models, natural animal aging models, and D-galactose aging models (Song et al., 1999; Wei et al., 2005). In D-galactose-induced aging animal models, the pathological biochemical characteristics of mouse tissues and organs are similar to those of natural aging animal models. This model gets the advantages of easy modeling and good reproducibility. The principle of action is tantamount to inject animals with D-galactose for a continuous period of time to increase the galactose concentration in the animal body. D-Galactose generates glyoxal and hydrogen peroxide under the action of galactose oxidase, which increases reactive oxygen species and causes lipid peroxidation and an increase in superoxide anion free radicals. This causes further lipid peroxidation and an increase in superoxide anion free radicals, as well as behavioral abnormalities, biochemical changes, immune dysfunction, histopathological changes, abnormal gene expression and regulation, and decreased cell growth and reproductive ability in D-galactose aging model mice, which eventually leads to aging of the organism (Lin L. et al., 2018; Bo-Htay et al., 2018). Vitamin C is a natural antioxidant and the most common functional antioxidant. Many researchers have conducted research on the antioxidant effect of VC. Its antioxidant effect is remains relatively stable and obvious. The use of VC as a positive control for aging models has been widely verified and used (Yi et al., 2019; Forman et al., 2020; Li C. et al., 2019b).
Non-Camellia Tea was proposed by Xiao Peigen in 2011 (Ren et al., 2019). There are mainly four types, Jinyinhua tea, Juhua tea, small leaf kuding tea, and Vine tea. They are long-term drinking form folks all over the world, and non-camellia varieties for the prevention of chronic metabolic diseases. In addition, ‘Sweet tea’, the leaves of lithocarpus polystachyus rehd, is also a kind of Non-Camellia Tea, a new natural source of biologically active dihydrochalcone with various health benefits (Shang et al., 2020). Ilex kudingcha C.J. Tseng, as a non-camellia tea, is a traditional Chinese tea that is widely used, second only to black/green tea (Camellia sinensis). It is a folk traditional medicinal plant in southwest of China, rich in various effective ingredients, and has a long history of folk use. In recent years, it has become increasingly popular with consumers, and is known as “beauty tea”, “yishou tea”, “green gold” and other names (Yi et al., 2019). The chemical composition of Ilex kudingcha processed by holly leaves is relatively complex, mainly including triterpenes and glycosides, flavonoids, polyphenols, amino acids, volatile oils and polysaccharides and other effective substances. It has health care and medicinal effects of preventing and treating cardiovascular diseases, anti-oxidation, lowering blood fat, anti-virus and lowering blood sugar (Gan et al., 2018; Xie et al., 2018; Zhou et al., 2018).
Insect tea is a unique drink made from Ilex kudingcha that is digested by enzymes and microorganisms in larvae produced by insects attracted by the fermented of boiled rice water (Zhu et al. 2019). In the 16th century, insect tea, as a traditional drink of ethnic minorities in southwest China and one of China’s traditional export commodities, was included in Li Shizhen’s Compendium of Materia Medica -Materia Medica classics (Xu et al., 2013). Studies have shown that insect tea contains crude protein, amino acids, polyphenols, tannins, vitamin C, and the mineral elements K, Mg, Cu, Fe, P, and Zn and other chemical components, with a variety of beneficial health and pharmacological effects, such as anti-oxidation, anti-tumor, and lowering of blood pressure and blood lipids (Mao et al., 2019; Mao et al., 2020).
In recent years, there has been continuous research on Ilex kudingcha, but there is less research on insect tea made from Ilex kudingcha. Some studies have shown that the polyphenols in Ilex kudingcha play an important role in its health functions, such as anti-oxidant, anti-inflammatory, and anti-cancer (Song et al., 2016; Zhai et al., 2017). Studies have also shown that insect tea contains a variety of functional ingredients, including polyphenols, minerals, and amino acids, with approximately 10% polyphenols (Liao et al., 2016; Zhao et al., 2018). In this context, the aging model of mice induced by D-galactose was used to evaluate the protective effect of Ilex kudingcha polyphenol extract (IKDCP) and insect tea polyphenol extract (ITP) on aging, and determine their bioactive compounds.
For extraction, 200 g of crushed Ilex kudingcha leaves (Hainan Coconut Island Agricultural E-commerce Co., Ltd., Hainan, China) and insect tea (Guizhou Zouzizhi Tea Development Co., Ltd, Guizhou, China) samples were weighed. Next, 1800 ml of distilled water was added at a ratio of 1:9 (v/v), and extraction was conducted at 90°C for 30 min. After a repeat of the extraction, the two extracts were combined, the filtrate was enclosed in a round-bottomed flask, and the liquid was dried by rotary evaporation to obtain IKDCP and ITP (Zhang J. et al., 2020; Zhu et al., 2019).
HPLC-grade methyl alcohol was purchased from Adamas Reagents, Ltd. (Shanghai, China). All the standards used for identification by UPLC-QqQ-MS were purchased from Shanghai Yuanye Biotechnology Co., Ltd. (Shanghai, China). Ultrapure water was available from Hangzhou Wahaha Group Co., Ltd. (Hangzhou, China).
The chemical composition of IKDCP and ITP was analyzed by ultra-high-pressure liquid chromatography (UPLC, Agilent, CA, USA), and triple quadruple mass spectrometry (G6460C-MS, Agilent, CA, USA) was conducted using a spectrophotometer equipped with an electrospray ionization (ESI) source. The ZORBAX Eclipse plus C18 column (100 × 2.1 mm id., 1.8 μm, Agilent, Waldbronn, Germany) was used to separate the compounds. The mobile phase consisted of a gradient of (A) 0.1% aqueous formic acid and (B) acetonitrile at a flow rate of 0.3 ml/min. The gradient elution was set as follows: 0–2 min, 20% B, 2–8 min, 20%–95% B, 8–13 min, 95% B, 13–15 min 20% B. Solutions of IKDCP and ITP were prepared by completely dissolving 100 mg of sample in 5 ml methanol. The sample injection volume was 5 μL, and the column temperature was 30°C. The ESI source was simultaneously operated in positive and negative ion mode, and the dynamic multiple reaction monitoring (MRM) data were acquired. The drying gas flow and temperature were 10 ml/min and 350°C, respectively (Qian et al., 2018; Rocchetti et al., 2018). All the compounds were identified by comparing the dynamic MRM information including retention time (RT), fragmentor voltages, collision energies (CE), and transitions with reference standards (Table 1). All analyses were performed three times in parallel, and the results are given in nanogram per Gram of dry weight.
Fifty 6-week-old SPF grade female Kunming mice (Chongqing Medical University, Chongqing, China. License number: SCXK (yu) 2017–0001) were fed for 1 week to adapt to the environment, and then were divided into five groups: normal group, model group, vitamin C group (VC), IKDCP group, and ITP group, with 10 mice in each group. The mice in each group were fed normal maintenance food and drinking water. Additionally, the mice in the vitamin C group, IKDCP group, and ITP group received vitamin C, IKDCP, and ITP by intragastric administration at the concentrations of 100, 500, and 500 mg/kg once a day, respectively. The normal group and the model group were given corresponding doses of distilled water. The modeling for each group began on the 15th day of administration. Except for the normal group (who received an injection of an equal volume of normal saline), the other groups were treated with D-galactose (Sinopharm Chemical Reagent Co., Ltd., China) at 120 mg/kg intraperitoneally daily for 6 weeks to induce the aging model. After 8 weeks, all mice were fasted for 18 h, euthanized and then were removed from the eyeballs for blood. The heart, liver, skin, spleen, and kidney were gathered for subsequent experiments. The heart, liver, spleen, and kidney tissue was weighed, and the organ index was calculated by the formula: organ index (%) = organ mass (g)/mouse body mass (g) × 100 (Wu et al., 2020; Li et al., 2019).
Mouse blood was centrifuged at 4,000 rpm and 4°C for 10 min, and the supernatant was harvested. The serum content of NO, T-AOC, SOD, CAT, GSH-PX, GSH, and MDA of mice was determined by kit instructions (Nanjing Jiancheng Bioengineering Institute, Nanjing, Jiangsu, China).
Depending on the kit instructions, the serum levels of cytokines IL-6, IL-12, TNF-α, and IL-1β were determined by enzyme-linked immunosorbent assay (ELISA, Abcam, Cambridge, MA, USA).
According to the kit instructions, 100 mg of liver tissue was removed, and 900 μL of physiological saline was added. The tissue was subsequently homogenized with a tissue homogenizer. The obtained tissue homogenate was centrifuged at 4,000 rpm and 4°C for 10 min, and the supernatant was harvested. The mouse liver tissue levels of NO, T-AOC, SOD, CAT, GSH-PX, GSH, and MDA were determined by kit instructions (Nanjing Jiancheng Bioengineering Institute, Nanjing, Jiangsu, China).
Samples of liver, skin, and spleen tissues with the size of 0.5 cm2 were collected and fixed in tissue fixative for 48 h. The liver, skin, and spleen tissues were dehydrated, made transparent, waxed, embedded, sectioned, baked, sliced, and then stained with H&E. The tissue morphological changes were observed under an optical microscope and analyzed by image acquisition (BX43; Olympus, Tokyo, Japan).
To extract the total RNA, 100 mg liver tissue, skin tissue, and spleen tissue of the mice were weighed, and 1 ml of TRIzol reagent (Invitrogen, Carlsbad, CA, USA) was separately added to the sample tissues, which were then pulverized using a tissue homogenizer. The concentration of the extracted total RNA was diluted to 1 μg/μL, and 1 μL of the diluted total RNA solution was submitted to reverse transcription using a kit to obtain the cDNA template. The reaction system was as follows: 1 μL of cDNA template was mixed with 10 μL of SYBR Green PCR Master Mix, 1 μL upstream and downstream primers, and 7 μL of enzyme-free water. The system was amplified under the following conditions: denatured at 95°C for 60 s, annealed at 60°C for 30 s, and extended at 72°C for 35 s, and cycled 40 times. Finally, the DNA was detected at 95°C for 30 s and 55°C for 35 s. The expression levels of the anti-oxidant genes SOD1, SOD2, CAT, GSH-PX, and GSH1 were determined by RT-qPCR. The sequences of the related primers are shown in Table 2. GAPDH was used as an internal reference (StepOnePlus Real-Time PCR System; Thermo Fisher Scientific, Waltham, MA, USA), and the levels of the relevant genes were calculated according to the 2−∆∆CT method (Li et al., 2019; Liao et al., 2016).
Samples of 100 mg liver tissue, skin tissue, and spleen tissue of the mice were homogenized with 1 ml of RIPA buffer (Thermo Fisher Scientific) and 10 μL of PMSF, and then centrifuged at 12,000 rpm and 4°C for 10 min. The intermediate protein layer solution was collected, and a BCA kit was used for protein quantification. It was determined that the protein loading amount was 50 μg, and the loading volume was 10 μL. The concentration of each sample was diluted to 6 μg/μL, and the diluted protein was mixed with sample buffer at the ratio of 4:1 and heated at 100°C for 10 min. Then, acrylamide, resolving buffer, stacking buffer, distilled water, 10% APS, and TEMED (Thermo Fisher Scientific) were mixed in proportion to make a SDS-PAGE separation gel and stacking gel, and the mixed solution was poured between the gel plates. The samples and the prestained protein ladder (Thermo Fisher Scientific) were separately loaded into the sample wells of the gel plate, and the protein-loaded SDS-PAGE gel was subjected to vertical gel electrophoresis until the prestained protein ladder was separated. A PVDF membrane (Thermo Fisher Scientific) was activated with methanol for 1 min and then transmembrane operation. After that, the PVDF membrane was blocked with TBS containing 0.1% Tween-20 (TBST) solution containing 5% skim milk for 1 h, transferred to 5% skim milk containing primary antibody, and incubated at 25°C for 2 h. The membrane and the secondary antibody were incubated at 25°C for 1 h. The PVDF membrane was washed 5 times with TBST, treated with Supersignal West Pico PLUS, and then placed in the iBright FL1000 (Thermo Fisher Scientific) imaging system for observation (Liao et al., 2016; Qian et al., 2018).
The serum and tissue assays for each mouse were performed in three parallel experiments, and the average value was calculated. The data are presented as the mean ± standard deviation (SD). SPSS statistical software (SPSS Inc, Chicago, Illinois, USA) was used for data analysis, one-way analysis of variance (ANOVA) for Post-Hoc two-two comparison was used to determine whether there were significant differences among groups of data at the level of p < 0.05.
In order to better understand the anti-oxidant effects of Ilex kudingcha and insect tea, the content of each polyphenol in IKDCP and ITP fragments was studied. We compared dynamic MRM information for 33 polyphenols (Figure 1). We found 29 and 26 polyphenol compounds in ITP and IKDCP. Cryptochlorogenic acid and rutin were abundant in IKDCP but could not be found in ITP. However, epicatechin, epigallocatechin gallate, epicatechin gallate, isovitexin, and hesperetin were detected in ITP, but not in IKDCP. Other polyphenols were detected in IKDCP and ITP, and the content of each substance was different (Table 3). In particular, kaempferol, narirutin, protocatechuic acid, caffeic acid, phloretin, taxifolin, isochlorogenic acid A, isochlorogenic acid B, and phloridzin were significantly higher in ITP than in IKDCP. In addition, chlorogenic acid, cryptochlorogenic acid, p-coumaric acid, isoquercitrin, and hesperidin accounted for 61.5% of the total content in IKDCP.
FIGURE 1. Extracted dynamic MRM chromatograms with retention times of standards of phenolic acids and flavonoids (A), polyphenols in Insect tea (B) and polyphenols in Ilex kuding tea(C).
The mice in the normal group exhibited the highest cardiac index, liver index, spleen index, and kidney index, while the lowest indices were observed for those in the model group (Table 4). After the administration of IKDCP, ITP, and vitamin C, the organ indices of all aging mice increased. There was no significant difference in the cardiac index between the IKDCP group and the ITP group, but there were significant differences in the other organ indexes (p < 0.05). The indices of the ITP group were significantly higher than those of the IKDCP group, and were comparable to the indices of the normal group. It can be concluded that IKDCP and ITP can alleviate the reduction of heart, liver, spleen, and kidney indexes to a certain extent, delay the degradation of mouse organs, and exert an antagonistic effect on organ atrophy.
Figure 2 indicates that the content of T-AOC, SOD, CAT, GSH-PX, and GSH in the serum of healthy mice (normal group) was the highest, while NO and MDA levels were lowest. The mice in the Aging (model group) exhibited a contrary trend. Compared with the model group, the content of T-AOC, SOD, CAT, GSH-PX, and GSH in the VC, ITP, and IKDCP groups was significantly increased (p < 0.05). In addition, the activity of T-AOC, SOD, CAT, GSH-PX, and GSH in the VC group was comparable with that in the IKDCP group, and the ITP group exhibited a higher content than the VC group with significant differences (p < 0.05). However, the content of NO and MDA was the lowest in the ITP group compared with the VC and IKDCP groups. Thus, the effect of ITP was stronger than that of VC and IKDCP.
FIGURE 2. The content of NO, T-AOC, SOD, CAT, GSH-PX, GSH and MDA in serum of mice (N = 10). a–eMean values with different letters in the bar are significantly different (p < 0.05) according to Duncan’s multiple-range test. VC: mice treated with vitamin C (100 mg/kg); IKDCP: mice treated with polyphenol of Ilex kudingcha (500 mg/kg); ITP: mice treated with polyphenol insect tea (500 mg/kg).
The serum cytokine detection assay showed that the serum levels of IL-1β, IL-6, and TNF-α in normal mice were lowest and that of IL-10 was the highest (Table 5), while these indices in the model group exhibited a contrary tendency. Vitamin C, IKDCP, and ITP alleviated the effects of aging in mice. The serum levels of cytokines IL-1β, IL-6, and TNF-α in aging mice (model group) were significantly higher than those in mice of the normal group, and IL-10 in the model group was significantly lower than that in normal mice without non-intervention (p < 0.05). The greatest decrease in IL-1β, IL-6, and TNF-α cytokines was attributed to the action of ITP, and its effect was significantly stronger than vitamin C and IKDCP.
As shown in Table 6, the content of SOD, CAT, GSH-PX, and GSH was highest and MDA was lowest in the liver tissues of normal mice, while the aging mice (model group) exhibited the opposite trend. After treatment with vitamin C, IKDCP, and ITP, compared with the model group, the SOD, CAT, GSH-PX, and GSH content in each group significantly increased (p < 0.05), and the MDA content significantly decreased (p < 0.05). The strongest effect was ascribed to ITP, with indicators that were similar to those of the normal group.
Normal mouse liver cells exhibited regular morphology, uniform size and staining, ordered hepatocyte cords, clear boundaries, and radial distribution centered on the central vein (Figure 3A). In the aging model group, the liver cells were unevenly arranged, the shape of the central vein was irregular, the arrangement of hepatocytes was disordered, the cell structure was destroyed, the boundaries were blurred, and the phenomenon of chromatin condensation was evident. However, the arrangement of mouse liver cell cords in mice treated with vitamin C, IKDCP, and ITP was basically orderly, and the morphology was slightly changed, which can alleviate cell necrosis and liver tissue incompleteness caused by D-galactose treatment in mice. The morphology of the liver tissue in the ITP treatment group resembled that of the normal group.
FIGURE 3. H&E pathological observation of liver tissue (A), skin tissue (B) and spleen tissue (C) in mice. Magnification ×100. VC: mice treated with vitamin C (100 mg/kg); IKDCP: mice treated with polyphenol of Ilex kudingcha (500 mg/kg); ITP: mice treated with polyphenol of insect tea (500 mg/kg).
A pathological section of skin tissue as viewed under an optical microscope with the aging model mice induced by D-galactose is shown in Figure 3B. The normal mouse epidermal structure was clear and complete, with abundant collagen fibers in the dermal layer, clear boundaries between the dermal layer and the epidermal layer, and a normal number of fat vacuoles. Compared to normal mice, the aging mice exhibited less collagen fibers, increased fat vacuoles, dermal layer invasion, and blurred boundaries between dermis and epidermis. Report to the model group, there were fewer fat vacuoles in the vitamin C, IKDCP, and ITP groups, and the osmotic phenomenon was effectively alleviated. The epidermal and dermal boundaries were more discernible. The results showed that IKDCP and ITP can alleviate the skin tissue lesions of aging model mice induced by D-galactose, with a stronger effect by ITP resulting in levels that were closer to normal as compared to IKDCP.
The spleen structure of normal mice was clear and intact, the junction of the cortex and pulp was clear, and the cells were regularly arranged (Figure 3C). The damaged spleen structure disappeared, the shape was irregular, the red pulp myeloid sinus expanded and was filled with a large number of red blood cells, white pulp lymphocytes decreased, red pulp myelin became narrow, and the cells were sparsely arranged. After treatment with vitamin C, IKDCP, and ITP, the spleen morphology and structure were significantly improved, the boundaries of red pulp and white pulp were clearer, germinal centers were more obvious, and cells were uniformly arranged. The results showed that vitamin C, IKDCP, and ITP alleviate the spleen tissue lesions of aging model mice induced by D-galactose. The strongest effect was observed for ITP treatment, which resulted in a spleen that was similar in appearance to a normal spleen.
The mRNA expression of GHS-PX, GSH1, SOD1, SOD2, and CAT was strongest in the liver, skin, and spleen tissues of normal mice (Figure 4 A, B, C respectively). However, the aging mice (model group) exhibited the opposite expression tendency. After vitamin C, IKDCP, and ITP processing, the expression of GHS-PX, GSH1, SOD1, SOD2, and CAT in liver, skin, and spleen tissues significantly increased (p < 0.05). Vitamin C, IKDCP, and ITP significantly inhibited the down regulation of related anti-oxidant genes. The effect of ITP was stronger, and the expression of GHS-PX, GSH1, SOD1, SOD2, and CAT in liver, skin, and spleen tissues of aging mice was similar to that of normal mice.
FIGURE 4. The GSH-PX, GSH1, SOD1, SOD2 and CAT mRNA expression in liver (A) skin (B) spleen (C) tissue of mice. a–eMean values with different letters in the bar are significantly different (p < 0.05) according to Duncan’s multiple-range test. VC: mice treated with vitamin C (100 mg/kg); IKDCP: mice treated with polyphenol of Ilex kudingcha (500 mg/kg); ITP: mice treated with polyphenol of insect tea (500 mg/kg).
Figures 5 indicate that the protein expression of CAT, SOD1, and SOD2 in liver (1), skin (2), and spleen 3) tissue of the model group was significantly (p < 0.05) lower than that of other groups, while the expression of the normal group mice were significantly stronger. Vitamin C, IKDCP, and ITP significantly up regulated CAT, SOD1, and SOD2 expression in the liver, skin, and spleen tissue of aging mice. ITP was more effective than IKDCP or vitamin C. The effect of ITP resulted in the expression of CAT, SOD1 and SOD2 in each aging mouse tissue that was similar to that of normal mice.
FIGURE 5. The SOD1, SOD2 and CAT protein expression relative multiple of model (A), protein band (B) in liver (1), skin (2), and spleen (3) tissue of mice. a–eMean values with different letters in the bar are significantly different (p < 0.05) according to Duncan’s multiple-range test. VC: mice treated with vitamin C (100 mg/kg); IKDCP: mice treated with polyphenol of Ilex kudingcha (500 mg/kg); ITP: mice treated with polyphenol of insect tea (500 mg/kg).
Ilex kudingcha is traditionally used as an herbal medicine in China and Southwest Asia. Studies have shown that Ilex kudingcha is rich in polyphenols such as coffee base quinic acid (CQA) and its derivatives (Zhu et al., 2009). Ilex kudingcha has been shown to have many beneficial functions, including anti-oxidant, anti-obesity, anti-diabetic, anti-inflammatory, cardiovascular, hepatoprotective, and neurological functions (Liu et al., 2009; Thuong et al., 2009; Song et al., 2013). Insect tea is a traditional tea drink that is different from ordinary tea, and is consumed for good health and medicinal purposes. Insect tea contains 17 mineral elements, including K, Mg, Ca, Na, Fe, Mn, and Zn are essential trace elements used by the human body. The amounts of Fe, Zn, Ca, and Mg are higher than those found in some well-known teas, such as Longjing, Biluochun and Tieguanyin (Xu et al., 2013; Mao et al., 2020). In addition, insect tea is rich in crude protein, crude fiber, fat, tea polyphenols, caffeine, sugar, vitamins, and amino acids. It enhances detoxification, strengthens the stomach and digestion, and exerts free radical scavenging, anti-oxidant, and anti-inflammatory effects (Zhao et al., 2018; Zhu et al., 2019; Zhang et al., 2020). In this study, We made statistics on the previous research reports (Lin C. S. et al., 2018; Svenja et al., 2020; Zhang J. et al., 2020; Zhang W. et al., 2020; Zhu et al., 2019), obtained the active substances that may be contained in Ilex kudingcha and Insect tea, and established a database of biologically active ingredients. 33 bioactive components were detected in ITP and IKDCP by UHPLC-QqQ-MS, with the majority being chlorogenic acid and its isomers, as well as isoquercitrin, kaempferol, dihydroquercetin, naringin.
Chlorogenic acid is a phenolic acid with an ortho-hydroxyl group on an aromatic residue and is derived from the esterification reaction of trans-cinnamic acid (including caffeic acid, ferulic acid, and p-coumaric acid) with quinic acid. There are several subclasses of chlorogenic acids, including neochlorogenic acid, cryptochlorogenic acid, isochlorogenic acid A, isochlorogenic acid B, and isochlorogenic acid C. Chlorogenic acid is a secondary metabolite of polyphenols widely present in tea. It has strong antioxidant property, can chelate metal ions and scavenge free radicals, hypochlorous acid, peroxynitrite anions and nitric oxide, and the activity of scavenging free radicals is 10–30 times that of vitamin C (Kono et al., 1997; Lu et al., 2000). In addition, both clinical and basic researches have proved that chlorogenic acid is beneficial to health (Naveed et al., 2018; Kumar et al., 2020). As one of the most abundant and functional polyphenols in human diets and drinks, the intake of chlorogenic acid is negatively related to the risk of metabolic syndrome and chronic diseases (Lu et al., 2020), it has pharmacological effects such as strong antioxidant effects, lowering blood pressure and blood lipids, protecting liver and gallbladder, antibacterial, anti-inflammatory, and anti-tumor effect (Xia et al., 2019; Li et al., 2019). The results of the current study show that chlorogenic acid, cryptochlorogenic acid were present in higher quantities and as the main anti-oxidant components in IKDCP, which matched the finding of a previous report (Wu, et al., 2020). However, the Caffeic acid, isochlorogenic acids A, isochlorogenic acids B, and isochlorogenic acids C were prominent in ITP. It further shows that ITP and IKDCP have strong antioxidant capacity.
There are obvious differences in other biologically active components in ITP and IKDCP. Isoquercitrin is a flavonoid compound obtained by the hydrolysis of rutin. It is widely present in mulberry leaves, apocynum, white bamboo leaves, and solanum. It is one of the important active ingredients of IKDCP with anti-depressant, anti-inflammatory, anti-oxidant, sedative, and anti-cancer effects (Li et al., 2019). Kaempferol is a natural flavonoid with a variety of biological activities, including anti-oxidant and anti-inflammatory effects (Cheng et al., 2018), and its content in ITP is much higher than in IKDCP. Dihydroquercetin (taxifolin), widely present in Pinaceae plants, is a dihydroflavonoid compound. Because it is a polyhydroxy compound, especially 5,7-OH and 3,4-meta-dihydroxy, it confers resistance to oxidation that is stronger than that of general bioflavonoids. As a raw powerful anti-oxidant, it has a variety of biological activities in the human body, including anti-oxidation, scavenging free radicals, anti-virus, anti-inflammatory, vasodilation, and antibacterial effects (Yang et al., 2019). Its content in ITP is obviously higher than that in IKDCP, further indicating that the potential antioxidant activity of ITP must be preferable to that of IKDCP. Naringin (naringenin-7-O-rutinoside) is mainly present in citrus peel. Modern therapeutic research has proven that naringin has anti-oxidant, anti-inflammatory, and anti-allergic effects (Funaguchi et al., 2007), it was detected in higher levels in ITP. In summary, although the total content of IKDCP bioactive substances is relatively high, the composition types of IKDCP are reversely small. On the contrary, the total content of ITP is relatively low compared with IKDCP, but it contains a variety of components, and the content of each component is surprisingly high compared that with IKDCP. Therefore, we conclude that the presence of these active substances in ITP may be the main reason why their multiple pharmacological effects.
Continuously injecting D-galactose into animals for a long time will increase the galactose concentration in the animal body, and a large amount of galactitol is then generated by the action of aldose reductase. When galactitol cannot be eliminated in time, it will build up in the body. The normal osmotic pressure of the cell is destroyed, resulting in disorder of the cell's metabolic function and the accumulation of free radicals, which induces the body’s oxidative stress response, and eventually leads to the aging of the organism (Cui et al., 2006; Wei et al., 2005).
The organ index data for mice is one of the important basic indicators of biomedical research. Changes in organ quality can directly reflect the aging of the body. The liver and kidney are metabolic organs in mice, and a decline in their mass directly affects the animal’s metabolic capacity. The spleen is closely linked to the cellular immune response in animals and plays an important role in the immune mechanism. A decline in the quality of the spleen indicates that the organs have atrophied, with a concomitant reduction in their immune function. The organ index can directly reflect the structural changes in the organs, indirectly explaining the function of the organs. Therefore, observing the changes in the mouse organ index has principal reference value for determining whether a mouse is aging (Hsu et al., 2005; Isanga et al., 2008). Studies have demonstrated that when the body ages, many organs and their functions will be degraded, and the heart, liver, spleen, and kidney indexes will decline to some extent (He et al., 2020; Zhang et al., 2020). Parallel research results appeared in this study. The visceral indices of aging model mice were significantly lower than those of the normal group and the vitamin C, IKDCP, and ITP treatment groups, and a more obvious alleviation of mouse organ aging caused by D-galactose was observed after ITP treatment.
In molecular biology, it is believed that with the increase in age, the activity of anti-oxidant enzymes in the body continues to decline, resulting in the inability of oxygen free radicals in the body to be sufficiently removed and the generation of peroxides, which causes the aging and death of cells and accelerates the body's senescence. SOD, MDA, T-AOC, GSH, NO, and GSH-PX levels are common markers of in vivo enzymes and non-enzyme systems that reflect the level of oxidative stress in the body. Studies have shown that when the body ages, the MDA content increases and the SOD activity decrease. Therefore, the body’s SOD activity and MDA content can be used as important indicators to measure body aging (Tian et al., 2019).
SOD is a particularly important enzyme in the body’s anti-oxidant system. During the aging process of the body, excess superoxide anion radicals are generated. The lifespan of the superoxide anion radicals is relatively long, resulting in induction of the production of hydroxyl radicals and the like, which then cause harm to cells, tissues, and the body. SOD activity in the body can reflect the ability of endogenous anti-oxidant enzymes to protect the body. In previous studies, the changes in anti-oxidant enzyme activity and lipid peroxidation during the aging process of the body were measured, and it was confirmed that the decrease in SOD activity is closely related to the body's aging (Noblanc et al., 2020). CAT is a very important anti-oxidant enzyme in the body that is essential for the regulation of superoxide anion free radicals and hydrogen peroxide levels in the body. SOD catalyzes the reaction of superoxide anion free radicals, which results in the generation of hydrogen peroxide, and continuous accumulation of hydrogen peroxide has an inhibitory effect on SOD. CAT can convert the generated hydrogen peroxide into a non-toxic substance, thereby regulating the balance of hydrogen peroxide and superoxide anion free radicals in the body. SOD and CAT to coordinate with each other and work together to protect the body (Li et al., 2019).
In the current study, aging induced by D-galactose caused oxidative stress response in the body. The excess production of superoxide anion radicals and hydroxyl radical pairs reduced the body's anti-oxidant enzyme activity. IKDCP and ITP can inhibit this phenomenon, increase anti-oxidant enzyme activity, and restore normal serum and liver tissue levels of SOD and CAT, thereby delaying the body’s aging. Due to the richness and high content of polyphenols in ITP, plus the minerals, amino acids, and other active substances in the insect tea, a synergistic effect may occur that amplifies the body’s ability to scavenge free radicals, resulting in increased effectiveness for ITP in its action to regulate D-galactose-induced aging as compared to IKDCP.
NO is a highly reactive free radical in the body that can relax vascular smooth muscle, inhibit platelet aggregation, and mediate cytotoxic effects and immune regulation. Aberrant production of NO is closely related to the occurrence and development of certain diseases. With an increase in the aging of the body, the concentration of NO continuously increases (Livak et al., 2001). The lipid peroxidation metabolite MDA can damage biological macromolecules such as proteins and nucleic acids, causing the body to age and develop various diseases. The level of MDA indirectly reflects the degree of free radical attack on the body cells, and it is another important indicator of the degree of oxidative damage in the body (Si et al., 2019). Reducing the MDA content in tissues and serum are of great significance to alleviate oxidative damage. T-AOC is a reliable indicator of all the enzymes and non-enzymes in the serum. It can remove active oxygen, maintain the dynamic balance of active oxygen in the internal environment, and balance the state of dynamic redox in the body (Wang C. et al., 2019).
GSH-PX is an important anti-oxidant enzyme that is widely present in the body and catalyzes the decomposition of hydrogen peroxide and peroxides. Its active center is selenocysteine (Wang et al., 2016). D-Galactose induces the body to produce excess reactive oxygen species, attacks lipid substances in cells, and generates lipid peroxides, while GSH-PX can specifically catalyze the reduction reaction of reduced glutathione to hydrogen peroxide and peroxide, remove harmful peroxide metabolites in the cell, promote the decomposition of hydrogen peroxide, and block the lipid peroxidation chain reaction, thereby protecting the integrity of the cell membrane structure and function. The amount of GSH-PX in the serum reflects the body’s oxidative stress and its anti-oxidant capacity (Giardino et al., 2002).
It has been reported that probiotics and tea polyphenols can increase the levels of MDA and NO in serum, kidney, and liver tissues and decrease the activities of SOD, T-AOC, and GSH-PX caused by D-galactose in aging mice (Li et al., 2020). The same results were achieved in this study. After intragastric administration of IKDCP, ITP, and vitamin C, the levels of MDA and NO in serum and liver tissue decreased, and the activities of SOD, T-AOC, GSH, and GSH-PX increased. The parameters of the ITP group basically returned to normal levels, indicating that ITP possessed strong in vivo anti-oxidant and health-promoting capabilities. By eliminating free radicals, ITP reduces the loss of anti-oxidant enzymes and increases the body’s anti-oxidant capacity, thus preventing oxidative damage and achieving anti-aging effects.
Oxidative stress is related to the occurrence of various diseases. Free radicals in the body can promote the production and metabolism of arachidonic acid, increase the production of inflammatory intermediates, and promote the production of plasma chemokines that will aggravate inflammation. IL-1β and TNF-α are produced by macrophages and monocytes. They are the most important cytokines in the early stage of inflammation, and are also known as alert cytokines. They can increase cell permeability and cause inflammatory cells to aggregate, with increasing cell infiltration (Bhatia et al., 2004). IL-6 is a pleiotropic cytokine, mainly formed by the differentiation of activated T cells and monocyte macrophages, and it has a certain effect in regulating the body’s aging, inflammation, and disease (Maccioni et al., 2009). IL-10, which is mainly produced by Th2 cells, inhibits the release of immune-regulating cytokines by Thl cells, and inhibits the release of inflammatory mediators, cytokines, and oxygen free radicals. When induced by D-galactose, mice generate glycosylation terminal irreversible polymers called AGEs in vivo. AGEs induce the expression of transcription factors NF-κB, thereby further upregulating the production of multiple cytokines (Song et al., 1999; Maczurek et al., 2008). With the continuous aging of the mouse body, a large number of ROS are activated in the body, which can induce the expression of TNF-α, IL-1β, and IL-6 (Woo et al., 2014). The serum pro-inflammatory factors TNF-α, IL-1β, and IL-6 increased, while the anti-inflammatory factor IL-10 decreased (Zeng et al., 2020).
The results of the current study show that administering VC, IKDCP, and ITP to aging mice induced by D-galactose can effectively alleviate the oxidative stress pathway, hinder the large increase in inflammatory factors, and lead to normal serum levels of IL-1β, IL-6, IL-10, and TNF-α. Both IKDCP and ITP reduced the aggregation of inflammatory cells and inhibited the inflammatory response, but a stronger effect was obtained by ITP rather than IKDCP, and ITP is more effectual in regulating D-galactose-induced inflammation.
The process of aging is extremely complex and involves the decreasing function of multiple organs, such as the liver, spleen, and skin tissues. The liver is a major organ that detoxifies potentially harmful chemicals and regulates the environmental stability of the body (Lebel et al., 2011). We observed obvious changes in sinusoidal endothelial cells in aging mice, as well as irregular hepatic cell morphology, untidy arrangement, and eosinophilia and microfocal necrosis that appeared and was accompanied by inflammatory cell infiltration. This is in agreement with the liver tissue of aging mice as reported by Anantharaju (Anantharaju et al., 2019).
The skin is the largest organ in the body, and skin changes are one of the main characteristics that accompany aging. With the aging of the body, the skin undergoes degenerative changes, collagen fibers in the dermal tissue are reduced, the skin is thinner, the water content is reduced, and skin shrinkage occurs (Wang Y. et al., 2019). The spleen is the most important secondary lymphoid organ in the body. It contains nearly one-fourth of all lymphocytes necessary for innate and adaptive immune responses, and it is the center of cellular and humoral immunity (El-naseery et al., 2020). The spleen structure of the oxidatively damaged mice disappeared, the shape was irregular, the red pulp myelinous sinus expanded and was filled with a large number of red blood cells, the white pulp lymphocytes were reduced, the red pulp myelin was narrowed, and the cells were sparsely arranged. Our data show that the liver tissue, skin, and spleen of aging mice induced by D-galactose lose normal structures, but after vitamin C, IKDCP, and ITP treatment, the oxidative damage to the liver, skin, and spleen tissue was alleviated, liver cells were neatly arranged, a small amount of cell necrosis and inflammatory cell infiltration appeared, skin shrinkage appeared to be relieved, and the dermal layer became thick. In the spleen, the boundary between the white pulp region and the red pulp region was rich. The atrophy of the white pulp region is scarce, and the cells at that location are densely arranged. The overall effect from ITP is more optimal as compared to IKDCP and vitamin C.
Studies have shown that aging is related to free radicals generated in organisms, which can react with lipids, proteins, and nucleic acids to produce lipid peroxides, which denature proteins, inactivate enzymes, and break DNA chains. This series of events subsequently causes biofilm damage to the system and allows the disease to develop. Anti-aging related genes and proteins in tissues, such as Cu/Zn-SOD, Mn-SOD, CAT, GSH1, and GSH-PX, can be used as genetic indicators for detecting oxidative damage to liver, skin, and spleen caused by D-galactose induction (Li et al., 2019; Jin et al., 2020). Previous studies have shown that D-galactose-induced oxidative damage can regulate and restore the free radical enzymes SOD and GSH-PX in the body (Li et al., 2019). Therefore, by measuring the expression levels of these important anti-oxidant-related genes and proteins, the anti-oxidant effects of IKDCP and ITP were determined, and their anti-oxidant capacity was compared. Compared with the model group, IKDCP and ITP upregulated the expression of Cu/Zn-SOD, Mn-SOD, CAT, GSH1, and increased GSH-PX mRNA and protein, indicating that the free radicals produced by oxidative metabolism in the liver, skin, and spleen tissue of aging mice induced by D-galactose can be eliminated. An anti-oxidant effect was observed after ITP treatment, which upregulated the expression of anti-oxidant-related genes and proteins so that they were similar to that of normal organisms, which is consistent with the results of Guo et al. (2020) and Yi et al. (2019).
In conclusion, we evaluated there in vivo protective effects on D-galactose-induced aging mice, and their potential bioactive substances. The results showed that the anti-aging effects of IKDCP and ITP (500 mg/kg) include the regulation of viscera indices of major organs; improvement in liver, skin, and spleen tissue morphology; a decrease in inflammatory cytokine production; upregulation of SOD, CAT, GSH, GSH-PX, and T-AOC, and downregulation of NO and MDA levels in serum and liver tissue; reductions in the concentration of pro-inflammatory factors (IL-6, TNF-α, and IL-1β); and increases in the concentration of anti-inflammatory factor IL-10. RT-qPCR and western blot assay also showed that IKDCP and ITP affect anti-aging by regulating the gene and protein expression of GSH-PX, GSH1, SOD1, SOD2, and CAT. In addition, these biological activities of IKDCP and ITP may be conferred by their chemical constituents, including flavonoids and phenolic acids. The 33 compounds detected by UHPLC-QqQ-MS have previously been shown to have a variety of biological activities, including anti-aging, anti-inflammatory, and anti-cancer. However, due to the substantial differences in the content of the primary chemical components in IKDCP and ITP, the effects are also different. The overall results indicate that ITP is more effective for treating aging mice with oxidative damage that was induced by D-galactose.
Ilex kudingcha and insect tea are stored for posterity and publicly accessible in Chongqing Collaborative Innovation Center for Functional Food, Chongqing University of Education, China, and the Ilex kudingcha and insect tea can be obtained from the corresponding authors.
The original contributions presented in the study are included in the article/Supplementary Material, further inquiries can be directed to the corresponding author.
The protocol for these experiments was approved by the Ethics Committee of Chongqing Collaborative Innovation Center for Functional Food (201905030B), Chongqing, China.
JM performed the majority of the experiments and wrote the manuscript; FY finished revising and proofreading this paper; FT, XZ, YP, and XL contributed to the data analysis; XZ designed and supervised the study, and checked the final manuscript.
This research was funded by Chongqing University Innovation Research Group Project (CXQTP20033) and the Introduction of High-level Person-nel Research Start-up Fund of Chongqing University of Education (2013BSRC001), China.
The authors declare that the research was conducted in the absence of any commercial or financial relationships that could be construed as a potential conflict of interest.
IKDCP, Ilex kudingcha polyphenol; ITP, insect tea polyphenol; UHPLC-QqQ-MS, ultra high-performance liquid chromatography-triple quadrupole mass spectrometry; SOD, superoxide dismutase; CAT, catalase; GSH, glutathione; GSH-PX, glutathione peroxidase; T-AOC, total antioxidant capacity; NO, nitric oxide; MDA, mobile device assistant; SOD1, Cu/Zn superoxide dismutase; SOD2, Mn superoxide dismutase; GAPDH, glyceraldehyde-3-phosphate dehydrogenase; TNF-α, tumor necrosis factor-α; IL-1β, andinterleukin-1β; IL-6, interleukin-6; IL-10, interleukin-10; RT-qPCR, real-time quantitative polymerase chain reaction; ESI, electrospray ionization; MRM, multiple reaction monitoring; DPPH, 2, 2-diphenyl-1-picrylhydrazyl; VC, vitamin C; ABTS, 2, 2′-azino-bis (3-ethylbenzothiazoline-6-sulphonic acid); RIPA, radioimmunoprecipitation; PMSF, phenylmethylsulfonyl fluoride; TEMED, N,N,N,N-tetramethylethylenediamine; APS, ammonium persulfate; PVDF, polyvinylidene fluoride; TBST, Tris-buffered saline containing 0.1% Tween-20; AGEs, advanced glycation end-products; ROS, reactive oxygen species; NF-κB, nuclear factor κ-light-chain-enhancer of activated B cells.
Anantharaju, A., Feller, A., and Chedid, A. (2019). Aging liver. A review. Gerontology. 48, 343–353. doi:10.1159/000065506
Bhatia, M., and Moochhala, S. (2004). Role of inflammatory mediators in the pathophysiology of acute respiratory distress syndrome. J. Pathol. 202, 145–156. doi:10.1002/path.1491
Bo-Htay, C., Palee, S., Apaijai, N., Chattipakorn, S. C., and Chattipakorn, N. 2018). Effects of d-galactose-induced ageing on the heart and its potential interventions. J. Cell Mol. Med. 22, 1392–1410. doi:10.1111/jcmm.13472
Cheng, X., Yang, Y., Yang, H., Wang, Y., and Du, G. (2018). Kaempferol alleviates LPS-induced neuroinflammation and BBB dysfunction in mice via inhibiting HMGB1 release and down-regulating TLR4/MyD88 pathway. Int. Immunopharm. 56, 29–35. doi:10.1016/j.intimp.2018.01.002
Clement, M. V., and Luo, L. (2019). Organismal aging and oxidants beyond macromolecules damage. Proteomics. 20, e1800400, doi:10.1002/pmic.201800400
Cui, X., Zuo, P., Zhang, Q., Li, X., Hu, Y., Long, J., et al. (2006). Chronic systemic D-galactose exposure induces memory loss, neurodegeneration, and oxidative damage in mice: protective effects of R-alpha-lipoic acid. J. Neurosci. Res. 84, 647–654. doi:10.1002/jnr.20899
Das, N., Levine, R. L., Orr, W. C., and Sohal, R. S. (2001). Selectivity of protein oxidative damage during aging in drosophila melanogaster. Biochem. J. 360, 209–216. doi:10.1042/0264-6021:3600209
El-naseery, N. I., Mousa, H. S. E., Noreldin, A. E., El-Far, A. H., and Elewa, Y. H. A. (2020). Aging-associated immunosenescence via alterations in splenic immune cell populations in rat. Life Sci. 241, 117168. doi:10.1016/j.lfs.2019.117168
Finkel, T., and Holbrook, N. J. (2000). Oxidants, oxidative stress and the biology of ageing. Nature. 408, 239–247. doi:10.1038/35041687
Forman, K., Martínez, F., Cifuentes, M., Fernández, M., Bertinat, R., Torres, P., et al. (2020). Dehydroascorbic acid, the oxidized form of vitamin C, improves renal histology and function in old mice. J. Cell. Physiol. 235, 9773–9784. doi:10.1002/jcp.29791
Funaguchi, N., Ohno, Y., La, B. L., Asai, T., Yuhgetsu, H., Sawada, M., et al. (2007). Narirutin inhibits airway inflammation in an allergic mouse model. Clin. Exp. Pharmacol. Physiol. 34, 766–770. doi:10.1111/j.1440-1681.2007.04636.x
Gan, R. Y., Zhang, D., Wang, M., and Corke, H. (2018). Health benefits of bioactive compounds from the genus ilex, a source of traditional caffeinated beverages. Nutrients. 10 (11), 1682. doi:10.3390/nu10111682
Giardino, R., Giavaresi, G., Fini, M., Torricelli, P., and Guzzardella, G. A. (2002). The role of different chemical modifications of superoxide dismutase in preventing a prolonged muscular ischemia/reperfusion injury. Artif. Cells Blood Substit. Immobil. Biotechno. 30, 189–198. doi:10.1081/BIO-120004339
Guo, H., Kuang, Z., Zhang, J., Zhao, X., Pu, P., and Yan, J. (2020). The preventive effect of apocynum venetum polyphenols on D-galactose-induced oxidative stress in mice. Experimental and Therapeutic Medicine. 19, 557–568. doi:10.3892/etm.2019.8261
Harman, D. (1956). Aging: a theory based on free radical and radiation chemistry. J. Gerontol. 11, 298. doi:10.1093/geronj/11.3.298
He, C., Wu, Q., Hayashi, N., Nakano, F., Nakatsukasa, E., and Tsuduki, T. (2020). Carbohydrate-restricted diet alters the gut microbiota, promotes senescence and shortens the life span in senescence-accelerated prone mice. J. Nutr. Biochem. 78, 108326. doi:10.1016/j.jnutbio.2019.108326
Hsu, C., Lin, T., Chang, W., Wu, C., Lo, W., Wang, P., et al. (2005). Soyasaponin-I-modified invasive behavior of cancer by changing cell surface sialic acids. Gynecol. Oncol. 96, 415–422. doi:10.1016/j.ygyno.2004.10.010
Isanga, J., and Zhang, G. (2008). Soybean bioactive components and their implications to health—a review. Food Rev. Int. 24, 252–276. doi:10.1080/87559120801926351
Jin, X., He, Y., Liu, Z., Zhou, Y., Chen, X., Wang, G., et al. (2020). Lactic acid bacteria exhibit similar antioxidant capacities in Caenorhabditis elegans and Campylobacter jejuni infected mice. RSC Adv. 10, 3329–3342. doi:10.1039/C9RA06105C
Kono, Y., Kobayashi, K., Tagawa, S., Adachi, K., Ueda, A., Sawa, Y., et al. (1997). Antioxidant activity of polyphenolics in diets. Rate constants of reactions of chlorogenic acid and caffeic acid with reactive species of oxygen and nitrogen. Biochim. Biophys. Acta. 1335, 335–342. doi:10.1016/S0304-4165(96)00151-1
Kumar, R., Sharma, A., Iqbal, M. S., and Srivastava, J. K. (2020). Therapeutic promises of chlorogenic acid with special emphasis on its anti-obesity property. Curr. Mol. Pharmacol. 13, 7–16. doi:10.2174/1874467212666190716145210
Lebel, M., de Souza-Pinto, N. C., and Bohr, V. A. (2011). Metabolism, genomics, and DNA repair in the mouse aging liver. Curr Gerontol Geriatr Res., 2011, 859415–15. doi:10.1155/2011/859415
Li, J. J., Mo, L., and Song, J. L. (2019). Improvement effect of ficus vasculosa ethanol extract on d-galactose-induced mice aging. Natural Product Communications. 14, 1934578X1989667. doi:10.1177/1934578X19896676
Li, C., Huang, G., Tan, F., Zhou, X., Mu, J., and Zhao, X. (2019a). In vitro analysis of antioxidant, anticancer, and bioactive components of apocynum venetum tea extracts. J. Food Qual. 2019, 1–13. doi:10.1155/2019/2465341
Li, C., Tan, F., Yang, J., Yang, Y., Gou, Y., Li, S., et al. (2019b). Antioxidant effects of apocynum venetum tea extracts on d-galactose-induced aging model in mice. Antioxidants. 8, 381. doi:10.3390/antiox8090381
Li, X., Gao, J., Yu, Z., Jiang, W., Sun, W., Yu, C., et al. (2020). Regulatory effect of anwulignan on the immune function through its antioxidation and anti-apoptosis in d-galactose-induced aging mice. Clin. Interv. Aging. 15, 97–110. doi:10.2147/CIA.S237601
Liao, Y., Zou, X., Wang, C., and Zhao, X. (2016). Insect tea extract attenuates CCl4-induced hepatic damage through its antioxidant capacities in ICR mice. Food Sci Biotechnol. 25, 581–587. doi:10.1007/s10068-016-0081-z
Lin, C. S., , Xu, S. X., and Huang, Q. Y. (2018). Caffeoylquinic acid derivatives in daye kudingcha (thunb.) organs in differently developing stage by micellar electrokinetic chromatography. Bull. Bot. Res. 3, 453–459. doi:10.7525/j.issn.1673-5102.2018.03.018
Lin, L., , Yang, K., Zheng, L., Zhao, M., Sun, W., Zhu, Q., et al. (2018). Anti-aging effect of sea cucumber (Cucumaria frondosa) hydrolysate on fruit flies and d-galactose-induced aging mice. J. Funct. Foods. 47, 11–18. doi:10.1016/j.jff.2018.05.033
Liu, L., Sun, Y., Laura, T., Liang, X., Ye, H., and Zeng, X. (2009). Determination of polyphenolic content and antioxidant activity of kudingcha made from Ilex kudingcha C. J. Tseng. Food Chemistry. 112, 35–41. doi:10.1016/j.foodchem.2008.05.038
Livak, K. J., and Schmittgen, T. D. (2001). Analysis of relative gene expression data using real-time quantitative PCR and the 2(-delta delta C(T)) Method. Methods. 25, 402–408. doi:10.1006/meth.2001.1262
Lu, H. J., Tian, Z. M., Cui, Y. Y., Liu, Z. C., and Ma, X. Y. (2020). Chlorogenic acid: a comprehensive review of the dietary sources, processing effects, bioavailability, beneficial properties, mechanisms of action, and future directions. Compr. Rev. Food Sci. Food Saf. 19 (6), 3130–3158. doi:10.1111/1541-4337.12620
Lu, Y., and Foo, L. Y. (2000). Antioxidant and radical scavenging activities of polyphenols from apple pomace. Food Chem. 68, 81–85. doi:10.1016/S0308-8146(99)00167-3
Maccioni, R. B., Rojo, L. E., Fernández, J. A., and Kuljis, R. O. (2009). The role of neuroimmunomodulation in alzheimer's disease. Ann. N. Y. Acad. Sci. 1153, 240–246. doi:10.1111/j.1749-6632.2008.03972.x
Maczurek, A., Shanmugam, K., and Münch, G. (2008). Inflammation and the redox-sensitive AGE-RAGE pathway as a therapeutic target in alzheimer's disease. Ann. N. Y. Acad. Sci. 1126, 147–151. doi:10.1196/annals.1433.026
Mao, X., Kusstatscher, P., Li, H., Chen, X., Berg, G., Yang, M., et al. (2019). Microbiome-guided exploration of the microbial assemblage of the exotic beverage "insect tea" native to southwestern China. Front. Microbiol. 10, 3087. doi:10.3389/fmicb.2019.03087
Mao, X., Li, H., and Yang, M. (2019). The complete mitochondrial genome sequences of two insect-tea producers in pyralidae (lepidoptera) from south China: pyralis farinalis and orthopygia glaucinalis. Mitochondrial DNA B Resour. 4, 3850–3851. doi:10.1080/23802359.2019.1687030
Naveed, M., Hejazi, V., Abbas, M., Kamboh, A. A., Khan, G. J., Shumzaid, M., et al. (2018). Chlorogenic acid (CGA): a pharmacological review and call for further research. Biomed. Pharmacother. 97, 67–74. doi:10.1016/j.biopha.2017.10.064
Noblanc, A., Klaassen, A., and Robaire, B. (2020). The exacerbation of aging and oxidative stress in the epididymis of sod1 null mice, AntioxidantsBasel. 9 (2), 151. doi:10.3390/antiox9020151
Qian, Y., Zhang, J., Fu, X., Yi, R., Sun, P., Zou, M., et al. (2018). Preventive effect of raw liubao tea polyphenols on mouse gastric injuries induced by HCl/ethanol via anti-oxidative stress. Molecules. 23, 2848. doi:10.3390/molecules23112848
Ren, K., Han, B. Q., Lv, L. J., Zhang, G. J., Lei, L. J., Bai, X. R., et al. (2019). Non-camellia tea in China: traditional usage, phytochemistry, and pharmacology. Chinese herbal medicine. 2, 119–131. doi:10.1016/j.chmed.2019.04.001
Rocchetti, G., Chiodelli, G., Giuberti, G., Ghisoni, S., Baccolo, G., Blasi, F., et al. (2018). UHPLC-ESI-QTOF-MS profile of polyphenols in Goji berries (Lycium barbarum L.) and its dynamics during in vitro gastrointestinal digestion and fermentation. Journal of Functional Foods. 40, 564–572. doi:10.1016/j.jff.2017.11.042
Sánchez-Rodríguez, M. A., and Mendoza-Núñez, V. M. (2019). Oxidative stress indexes for diagnosis of health or disease in humans. Oxidative Medicine and Cellular Longevity. 2019 1–32. doi:10.1155/2019/4128152
Shang, A., Liu, H. Y., Luo, M., Shang, A., Xia, Y., Yang, X., et al. (2020). Sweet tea (Lithocarpus polystachyus rehd.) as a new natural source of bioactive dihydrochalcones with multiple health benefits. Crit. Rev. Food Sci. Nutr. 1–18. doi:10.1080/10408398.2020.1830363
Si, L., Lin, R., Jia, Y., Jian, W., Yu, Q., Wang, M., et al. (2019). Lactobacillus bulgaricus improves antioxidant capacity of black garlic in the prevention of gestational diabetes mellitus: a randomized control trial. Biosci. Rep. 39 (8), BSR20182254. doi:10.1042/BSR20182254
Song, C., Yu, Q., Li, X., Jin, S., Li, S., Zhang, Y., et al. (2016). The hypolipidemic effect of total saponins from kuding tea in high-fat diet-induced hyperlipidemic mice and its composition characterized by UPLC-QTOF-MS/MS. J. Food Sci. 81, H1313–H1319. doi:10.1111/1750-3841.13299
Song, J., Qian, Y., Li, G., and Zhao, X. (2013). Anti-inflammatory effects of kudingcha methanol extract (Ilex kudingcha C.J. Tseng) in dextran sulfate sodium-induced ulcerative colitis. Mol. Med. Rep. 8, 1256–1262. doi:10.3892/mmr.2013.1635
Song, X., Bao, M., Li, D., and Li, Y. M. 1999). Advanced glycation in d-galactose induced mouse aging model. Mech. Ageing Dev. 108, 239–251. doi:10.1016/s0047-6374(99)00022-6
Svenja, W., Kai, L., and Rimbach, G. (2020). Chemical composition, bioactivity and safety aspects of kuding tea-from beverage to herbal extract. Nutrients. 12. doi:10.3390/nu12092796
Thuong, P. T., Su, N. D., Ngoc, T. M., Hung, T. M., Dang, N. H., Thuan, N. D., et al. (2009). Antioxidant activity and principles of Vietnam bitter tea Ilex kudingcha. Food Chem. 113, 139–145. doi:10.1016/j.foodchem.2008.07.041R
Tian, Y., Wen, Z., Lei, L., Li, F., Zhao, J., Zhi, Q., et al. (2019). Coreopsis tinctoria flowers extract ameliorates D-galactose induced aging in mice via regulation of Sirt1-Nrf2 signaling pathway. Journal of Functional Foods. 60, 103464. doi:10.1016/j.jff.2019.103464
Wang, C., , Shen, Z., Yu, J., Yang, J., Meng, F., Jiang, X., et al. (2019). Protective effects of enzyme degradation extract from porphyra yezoensis against oxidative stress and brain injury in d-galactose-induced ageing mice. Br. J. Nutr. 123 (9), 975–986. doi:10.1017/S0007114519003088
Wang, Y., , Wang, L., Wen, X., Hao, D., Zhang, N., He, G., et al. (2019). NF-κB signaling in skin aging. Mech. Ageing Dev. 184, 111160. doi:10.1016/j.mad.2019.111160
Wang, Y., Zhou, J., Xia, X., Zhao, Y., and Shao, W. (2016). Probiotic potential of Lactobacillus paracasei FM-LP-4 isolated from Xinjiang camel milk yoghurt. Int. Dairy J. 62, 28–34. doi:10.1016/j.idairyj.2016.07.001
Wei, H., Li, L., Song, Q., Ai, H., Chu, J., and Li, W. (2005). Behavioural study of the D-galactose induced aging model in C57BL/6J mice. Behav. Brain Res. 157, 245–251. doi:10.1016/j.bbr.2004.07.003
Woo, J., Gu, W., Kim, K., Jang, S., Han, M. J., and Kim, D. (2014). Lactobacillus pentosus var. plantarum C29 ameliorates memory impairment and inflammaging in a d-galactose-induced accelerated aging mouse model. Anaerobe. 27, 22–26. doi:10.1016/j.anaerobe.2014.03.003
Wu, Y., Yang, J., Liu, X. J., Zhang, Y., Lei, A. l., Yi, R. K., et al. (2020). Preventive effect of small-leaved Kuding tea (Ligustrum robustum) on high-diet-induced obesity in C57BL/6J mice. Food Sci. Nutr. 8, 4512–4522. doi:10.1002/fsn3.1758
Xia, J., Zhao, B., Zan, J., Wang, P., and Chen, L. (2019). Simultaneous determination of phenolic acids and flavonoids in Artemisiae Argyi Folium by HPLC-MS/MS and discovery of antioxidant ingredients based on relevance analysis. J. Pharmaceut. Biomed. Anal. 175, 112734. doi:10.1016/j.jpba.2019.06.031
Xie, M., Chen, G., Wan, P., Dai, Z., Zeng, X., and Sun, Y. (2019). Effects of dicaffeoylquinic acids from ilex kudingcha on lipid metabolism and intestinal microbiota in high-fat-diet-fed mice. J. Agric. Food Chem. 67, 171–183. doi:10.1021/acs.jafc.8b05444
Xu, L., Pan, H., Lei, Q., Xiao, W., Peng, Y., and Xiao, P. (2013). Insect tea, a wonderful work in the Chinese tea culture. Food Res. Int. 53, 629–635. doi:10.1016/j.foodres.2013.01.005
Yang, C., Lin, Y., Liu, K., Peng, W., and Hsu, C. (2019). Hepatoprotective mechanisms of taxifolin on carbon tetrachloride-induced acute liver injury in mice. Nutrients. 11, 2655. doi:10.3390/nu11112655
Yi, R., Zhang, J., Sun, P., Qian, Y., and Zhao, X. (2019). Protective effects of kuding tea (ilex kudingcha c. j. tseng) polyphenols on UVB-induced skin aging in SKH1 hairless mice. Molecules. 24, 1016. doi:10.3390/molecules24061016
Zeng, L., Lin, L., Peng, Y., Yuan, D., Zhang, S., Gong, Z., et al. (2020). l-Theanine attenuates liver aging by inhibiting advanced glycation end products in d-galactose-induced rats and reversing an imbalance of oxidative stress and inflammation. Exp. Gerontol. 131, 110823. doi:10.1016/j.exger.2019.110823
Zhai, X., Ren, D., Luo, Y., Hu, Y., and Yang, X. (2017). Chemical characteristics of an ilex kuding tea polysaccharide and its protective effects against high fructose-induced liver injury and vascular endothelial dysfunction in mice. Food Funct. 8, 2536–2547. doi:10.1039/c7fo00490g
Zhang, J., Lu, D. Y., Yuan, Y., Chen, J. X., Yi, S., Chen, B. C., et al. (2020). Liubao Insect tea polyphenols prevent HCl/ethanol induced gastric damage through its antioxidant ability in mice. RSC Adv. 10, 4984–4995. doi:10.1039/c9ra09641h
Zhang, W., , Liu, X., and Piao, L. (2020). Chlorogenic acid-enriched extract of Ilex kudingcha C. J. Tseng tea inhibits neutrophil recruitment in injured zebrafish by promoting reverse migration via the focal adhesion pathway. J. Food Biochem. 44, doi:e13279. doi:10.1111/jfbc.13279
Zhang, Z., , He, S., Cao, X., Ye, Y., Yang, L., Wang, J., et al. (2020). Potential prebiotic activities of soybean peptides Maillard reaction products on modulating gut microbiota to alleviate aging-related disorders in D-galactose-induced ICR mice. Journal of Functional Foods. 65, 103729. doi:10.1016/j.jff.2019.103729
Zhao, X., Song, J., Yi, R., Li, G., Sun, P., Park, K., et al. (2018). Comparison of antioxidative effects of insect tea and its raw tea (kuding tea) polyphenols in kunming mice. Molecules. 23, 204. doi:10.3390/molecules23010204
Zhou, J., Yi, H., Zhao, Z., Shang, X., Zhu, M., Kuang, G., et al. (2018). Simultaneous qualitative and quantitative evaluation of Ilex kudingcha C. J. tseng by using UPLC and UHPLC-qTOF-MS/MS. J. Pharmaceut. Biomed. Anal. 155, 15–26. doi:10.1016/j.jpba.2018.02.037
Zhu, F., Cai, Y., Sun, M., Ke, J., Lu, D., and Corke, H. (2009). Comparison of major phenolic constituents and in vitro antioxidant activity of diverse Kudingcha genotypes from Ilex kudingcha, Ilex cornuta, and Ligustrum robustum. J. Agric. Food Chem. 57, 6082–6089. doi:10.1021/jf901020h
Keywords: ilex kudingcha, insect tea, polyphenols, aging, UHPLC-QqQ-MS
Citation: Mu J, Yang F, Tan F, Zhou X, Pan Y, Long X and Zhao X (2021) Determination of Polyphenols in Ilex kudingcha and Insect Tea (Leaves Altered by Animals) by Ultra-high-performance Liquid Chromatography-Triple Quadrupole Mass Spectrometry (UHPLC-QqQ-MS) and Comparison of Their Anti-Aging Effects. Front. Pharmacol. 11:600219. doi: 10.3389/fphar.2020.600219
Received: 29 August 2020; Accepted: 21 December 2020;
Published: 21 January 2021.
Edited by:
Karl Tsim, Hong Kong University of Science and Technology, Hong KongReviewed by:
Christian Zidorn, University of Kiel, GermanyCopyright © 2021 Mu, Yang, Tan, Zhou, Pan, Long and Zhao. This is an open-access article distributed under the terms of the Creative Commons Attribution License (CC BY). The use, distribution or reproduction in other forums is permitted, provided the original author(s) and the copyright owner(s) are credited and that the original publication in this journal is cited, in accordance with accepted academic practice. No use, distribution or reproduction is permitted which does not comply with these terms.
*Correspondence: Xin Zhao, emhhb3hpbkBjcXVlLmVkdS5jbg==
†These authors have contributed equally to this work
Disclaimer: All claims expressed in this article are solely those of the authors and do not necessarily represent those of their affiliated organizations, or those of the publisher, the editors and the reviewers. Any product that may be evaluated in this article or claim that may be made by its manufacturer is not guaranteed or endorsed by the publisher.
Research integrity at Frontiers
Learn more about the work of our research integrity team to safeguard the quality of each article we publish.