- 1Department of Pharmacy, The Eighth Affiliated Hospital, Sun Yat-sen University, Shenzhen, China
- 2Department of Chemical and Biological Engineering, Hong Kong University of Science and Technology, Kowloon, China
- 3Department of Gastroenterology, The Eighth Affiliated Hospital, Sun Yat-sen University, Shenzhen, China
- 4Department of Cardiovascular, The Eighth Affiliated Hospital, Sun Yat-sen University, Shenzhen, China
Myocardial ischemia (MI) is one of the most common cardiovascular diseases with high incidence and mortality. Huang-Lian-Jie-Du-Tang (HLJDT) is a classic traditional Chinese prescription to clear “heat” and “poison”. In this study, we used a deliberate strategy integrating the methods of network pharmacology, pharmacodynamics, and metabonomics to investigate the molecular mechanism and potential targets of HLJDT in the treatment of MI. Firstly, by a network pharmacology approach, a global view of the potential compound-target-pathway network based on network pharmacology was constructed to provide a preliminary understanding of bioactive compounds and related targets of HLJDT for elucidating its molecular mechanisms in MI. Subsequently, in vivo efficacy of HLJDT was validated in a rat model. Meanwhile, the corresponding metabonomic profiles were used to explore differentially induced metabolic markers thus providing the metabolic mechanism of HLJDT in treating MI. The results demonstrated the myocardial protection effect of HLJDT on ischemia by a multicomponent-multitarget mode. This study highlights the reliability and effectiveness of a network pharmacology-based approach that identifies and validates the complex of natural compounds in HLJDT for illustrating the mechanism for the treatment of MI.
Introduction
Acute myocardial ischemia (AMI) refers to a pathological state in which blood perfusion is reduced due to coronary artery occlusion, insufficient blood flow, or oxygen supply. It is one of the most common cardiovascular diseases with a high incidence rate and high mortality rate (Turer and Hill, 2010; Davidson et al., 2019). Studies have shown that the pathogenesis of AMI is closely related to biological pathways including energy metabolism, oxidative stress (Kaul and Ito, 2004; Luqman et al., 2007), apoptosis (Garg et al., 2003), calcium homeostasis (Katopodis et al., 1997), angiogenesis (Lee et al., 2000), and inflammatory and immune responses (Rui et al., 2005; Arslan et al., 2011). In the theory of traditional Chinese medicine (TCM), AMI belongs to the category of “chest obstruction” and “cardialgia”, which can be treated by “invigorating the circulation of Qi and blood”, “dispersing stagnation”, and “dredging collaterals”. Various Chinese herbal medicines, such as Salvia miltiorrhiza, Rhodiolarosea, Forsythia suspensa, Sini Tang, and Tongxinluo, have been reported to improve myocardial ischemia and protect the heart (Liu et al., 2013; Guo et al., 2016), which indicates the advantage of TCM in myocardial protection. Nevertheless, as the multi-component, multi-target, and multi-channel synergistic characteristics of TCM, it is inappropriate to use the mode of “one drug-one target” to elaborate its mechanism of action. Moreover, it is difficult to carry out systematic research on the level of tissues, organs, cells, and molecules, which remain an obstacle for the modernization of TCM (Wang et al., 2012).
Network pharmacology is one of the emerging strategies based on multi-disciplinary technologies such as system biology, multi-directional pharmacology, computational biology, and network analysis, which systematically reveals the core molecular targets and pharmacodynamics methods of TCM (Hopkins, 2008; Li and Zhang, 2013). In this approach, the multi-level relationship between “drug-compound-target-pathway-disease” is established by using various technologies including Omics technology, high-throughput screening, network visualization, or network analysis. It helps us understand the molecular basis of disease, predicts the pharmacological mechanism, and finds herbal compounds of high efficiency and low toxicity.
Huang-Lian-Jie-Du-Tang (HLJDT) is a classic traditional Chinese prescription to clear “heat” and “poison”, which was first published in Gehong’s “elbow reserve emergency prescription”. It consists of the rhizoma of Coptis chinensis Franch (Huanglian 9 g), the radix of Scutellaria baicalensis Georgi (Huangqin 6 g), the cortex of Phellodendron chinense C.K. Schneid (Huangbo 6 g) and the fructus of Gardenia jasminoides J. Ellis (Zhizi, 9 g) (Shen et al., 2003). Modern pharmacology research shows that HLJDT has obvious anti-inflammatory, antibacterial, anti-endotoxin, and antipyretic effects (Li et al., 2012; Lv et al., 2017). Recent studies showed that HLJDT could significantly reduce cerebral ischemia injury, cholesterol, etc. (Sekiya et al., 2005; Wang et al., 2013) Studies showed that the effective components in HLJDT could mainly be divided into three categories: 1) alkaloids: mainly from the rhizoma of Coptis chinensis Franch and the cortex of Phellodendron chinense C.K. Schneid. Berberine and Phellodendron amurense are the focuses of current studies. 2) Flavonoids: mainly from the radix of Scutellaria baicalensis Georgi. The representative components are baicalein, scutellarin, and baicalin. 3) Iridoids: mainly from the fructus of Gardenia jasminoides J. Ellis and its representative component is gardenside (Han et al., 2007; Ma et al., 2009; Chen et al., 2010). Although there have been many studies on the efficacy and mechanism of HLJDT, most of them focus on a single component and the multi-component and multi-target mechanism is still unclear.
In this study, we used a deliberate strategy integrating the methods of network pharmacology, pharmacodynamics, and metabonomics to investigate the molecular mechanism and potential target of HLJDT for treatment of MI. Firstly, an MI-related “potential compounds-target-pathway” regulatory network was generated by network pharmacology to identify potential bioactive compounds and related targets of HLJDT. Subsequently, in vivo experimental verification of the efficacy was performed in a rat MI model by ligation of the coronary artery. The metabonomics approach was applied to explore differentially induced metabolic markers, thus providing the metabolic mechanism of HLJDT in treating MI. This study highlights the reliability and effectiveness of a network pharmacology-based approach that identifies and validates the complexity of natural compounds in HLJDT for illustrating the mechanism of action in the treatment of MI. The overall flowchart for elucidating the mechanism of HLJDT in the treatment of MI is illustrated in Figure 1.
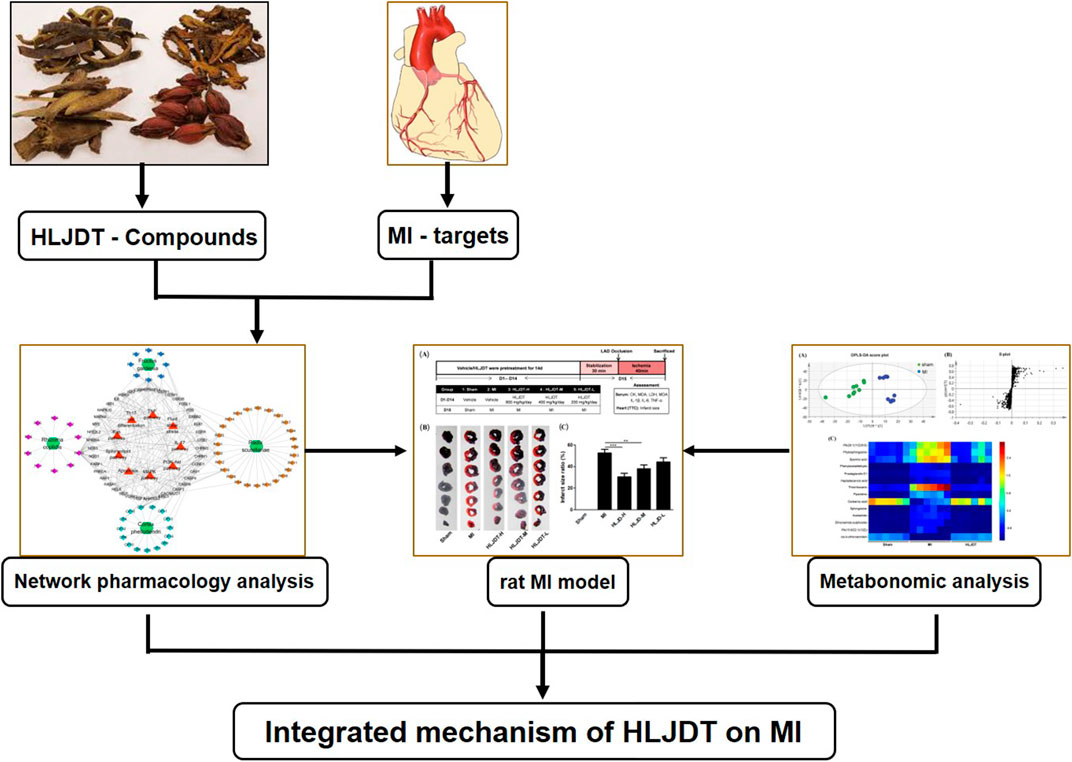
FIGURE 1. Integrated flowchart for elucidating the mechanism of HLJDT in the treatment of MI. Firstly, a global view of the potential compound–target–pathway network based on network pharmacology was constructed to provide a preliminary mechanisms prediction the of HLJDT for elucidating its molecular mechanisms in MI. Subsequently, in vivo efficacy of HLJDT was validated in a rat model. Meanwhile, the corresponding metabonomic profiles were used to explore differentially induced metabolic markers thus providing the metabolic mechanism of HLJDT in treating MI. These results will demonstrate the myocardial protection effect and illustrating the mechanism of HLJDT for the treatment of MI.
Materials and Methods
Network Pharmacology Analysis
Predicting Potential Gene Targets of Chemical Composition in Huang-Lian-Jie-Du-Tang
The chemical compositions of HLJDT were collected from the Traditional Chinese Medicine Systems Pharmacology database (TCMSP, http://lsp.nwu.edu.cn/) (Ru et al., 2014), which is a unique database platform of Chinese herbal medicine system pharmacology for capturing the relationship among drugs, targets, and diseases. Those meeting the criteria of bioavailability (OB) ≥30% and drug-likeness (DL) ≥0.1 (Xu et al., 2012) were used for further analysis. The HPLC chromatogram of HLJDT obtained from the methanol extract of HLJDT is shown in Supplementary Figure S1. The potential gene targets of the major compounds in HLJDT were searched from the TCMSP and PubMed databases. Totally, 448 HLJDT-related targets were obtained (Supplementary Table S2).
MI-Associated Target Genes
The target gene number (PDB ID number) was converted into a gene name corresponding to the Genecards database through the UniProt database. The key words “myocardial ischemia” or “myocardial infarction” were input into the Genecards database (http://www.genecards.org, a database providing a detailed genome, proteome, transcription, genetic, and functional overview of all known and predicted human genes) and the Online Mendelian Inheritance in Man database (OMIM) to collect target genes related to MI. The common target genes were screened out as the target of HLJDT in the treatment of MI.
Pathway Enrichment and Construction of the C-T-P Network
In order to explain the potential role of the active components in HLJDT in gene function and signaling pathways, we used DAVID (database for Annotation, Visualization and Integrated Discovery) to analyze the KEGG pathways of the predicted targets. The results of KEGG enrichment analysis were visualized using the Omicshare online analysis platform. The components-targets-pathway (C-P-T) network of HLJDT for treating MI was constructed by utilizing the network-visualization software Cytoscapev3.6.1.
Experimental Validation of Huang-Lian-Jie-Du-Tang on MI in vivo
Animals
Male Sprague–Dawley (SD) rats (180–200 g) were purchased from the SLAC Laboratory Animal Co., Ltd. (Shanghai, China). The animals were housed in standard pathogen-free cages in a climate-controlled environment with a 12 h light/12 h dark cycle. Sterile food and water were naturally provided. All procedures were conducted in accordance with the guidelines for care and use of laboratory animals from the Second Military Medical University and government.
Animals Grouping and Drug Administration
The experimental procedure of the in vivo MI rat model is shown in Figure 2A. Fifty SD rats were randomly divided into five groups (10 rats per group): 1) a sham group (vehicle-treated + sham); 2) an MI group (vehicle-treated + MI); 3) a high-dose HLJDT group (800 mg/kg/day); 4) a middle-dose HLJDT group (400 mg/kg/day); and 5) a low-dose HLJDT group (200 mg/kg/day). The vehicle and HLJDT were pretreated by gavage once per day for 14 consecutive days before the establishment of the MI model.
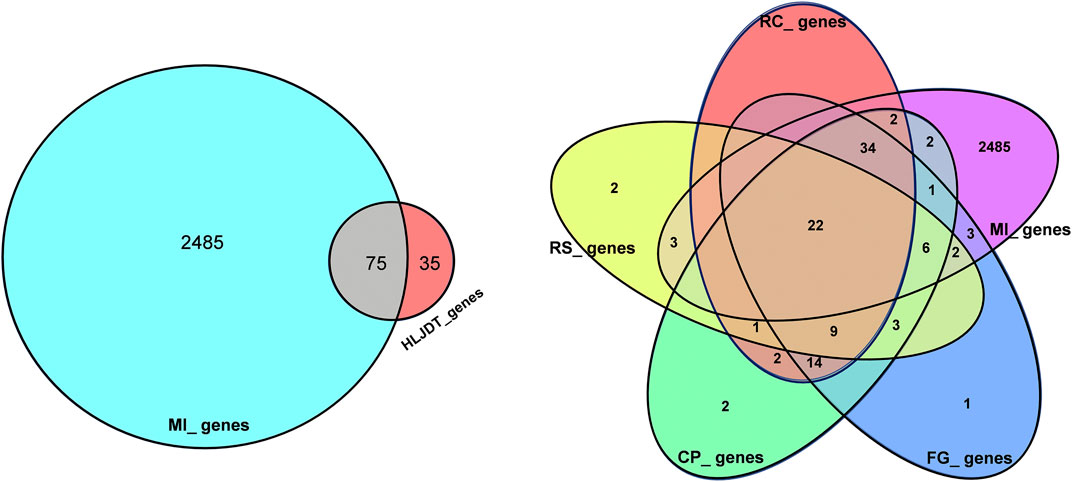
FIGURE 2. Overlaps between different gene sets. (A) Overlaps between MI diseases genes from HLJDT-related genes; (B) Overlaps between different gene sets from MI and 4 main herbs in HLJDT prescription. RC‐Rhizoma coptidis, RS-Radix scutellariae, CP‐Cortex phellodendri, FG-Fructus gardenia.
Establishment of the Rat MI Model
The 50 experimental rats were anesthetized with isoflurane inhalation and needle electrodes were inserted into the subcutaneous limbs. A catheter containing 0.1% heparin saline was inserted into the left ventricle through the left common carotid artery. Electrocardiograph (ECG) and left ventricular hemodynamic changes were recorded by a BL-410 biological function experiment system and the changes of the S-T segment were used to determine the success of modeling. Endotracheal intubation and artificial ventilators were used for mechanical ventilation. The MI model was established by ligating the left anterior descending coronary artery (LADCA) (Wang et al., 2002). After the LAD was exposed between the left auricle and the pulmonary conus, a small section of polyethylene tube was put through with a 4–0 black silk ligature to block LAD blood flow for 30 min. The sham group was handled using the same operation process without the ligation step. Five rats died throughout the experiment and the other 45 rats who survived were successfully modeled (the modeling rate was up to 90%), including 10 rats in the group (1), eight rats in group (2), 10 rats in group (3), eight rats in group (4), and nine rats in group (5).
Sample Collection and Detection
The experimental rats were sacrificed 30 min after modeling. Immediately, blood was collected from the abdominal aorta. The supernatant serum obtained was collected and stored at −20°C for analysis of the biochemical indexes including CK, MDA, SOD, LDH and IL-1β, IL-6, IL-17, and TNF-α. The remaining serum was stored at −80°C for metabonomic analysis. Simultaneously, the hearts were rapidly removed for 2,3,5-triphenyltetrazolium chloride (TTC) immersion to determine the myocardial infarct size.
Metabonomic Analysis
UPLC-Q-TOF/MS Measurement and Data Analysis
Metabonomic analysis was performed by the Agilent 1,290 Infinity Ⅱ UHPLC system coupled to an Agilent 6545 UHD and Accurate-Mass Q-TOF/MS. Mobile phase: A: aqueous solution. B: acetonitrile solution. Flow rate: 0.35 ml/min. Column temperature: 25°C. Injection volume: 2 μL. Gradient elution condition optimized: 0–2 min, 1% B; 2–7 min, 1–15% B; 7–9 min, 15–50% B; 9–13 min, 50–95% B; 13–15 min, 95% B. Post time was set as 5 min. Mass spectrometry was operated in both positive and negative ion modes. Differential metabolites were further identified by MS/MS with collision energy of 10 v, 20 v, and 40 v. Raw data were converted by the self-contained software of the Agilent system. Then the peak was identified by the XCMS program in the R software platform. The peel data were subjected to internal standard normalization and weight normalization and the flesh data were subjected to internal standard normalization. Visualization matrices containing sample name, m/z-RT pair, and peak area were obtained. For the peel simples, 1,428 features were obtained in the positive mode. After editing, the data matrices were imported into SIMCA-P 13.0 After preprocessing, the data were analyzed by Orthogonal Partial Least Square Discriminate Analysis (OPLS-DA).
Qualitative Analysis of Metabolites and Screening of Differential Metabolites
The metabolites were qualitatively analyzed by searching the self-built standard substance database according to the results of the secondary spectrum and then the METLIN, HMDB, HMDB-SERUM, KEGG, CHEBI, LIPID, and other public databases were searched to provide alternative substances. In our study, the Variable Importance in Projection (VIP, threshold >1) of the first principal component of the OPLS-DA model and the p value (threshold <0.05) of Student’s t-test were used to find differentially expressed metabolites.
Statistical Analysis
Data were analyzed by one-way analysis of variance (ANOVA) followed by a Dunnett's test or a Kruskal-Wallis ANOVA on Ranks followed by a Dunn’s test for multiple comparisons and expressed as the means ± SEM. *p < 0.05 was considered a significant difference.
Results
Prediction of the Pharmacological Mechanism of Huang-Lian-Jie-Du-Tang on MI by a Network Pharmacology Approach
Herbal Compounds in Huang-Lian-Jie-Du-Tang
Using the TCMSP database, 429 compounds were retrieved, where 48 were in Rhizoma coptidis, 143 were in Radix scutellariae, 140 were in Cortex phellodendri, and 98 were in Fructus gardenia. With the criteria of OB larger than 30% and DL larger than 0.18, 106 chemical ingredients were screened out, where 14 were in Rhizoma coptidis, 36 were in Radix scutellariae, 37 were in Cortex phellodendri, and 15 were in Fructus gardenia. After taking out the duplicated parts, 84 chemical constituents were accepted, and their structures were retrieved from the PubChem database (Supplementary Table S1). Finally, 59 chemical ingredients were screened out for further target prediction analysis (Table 1).
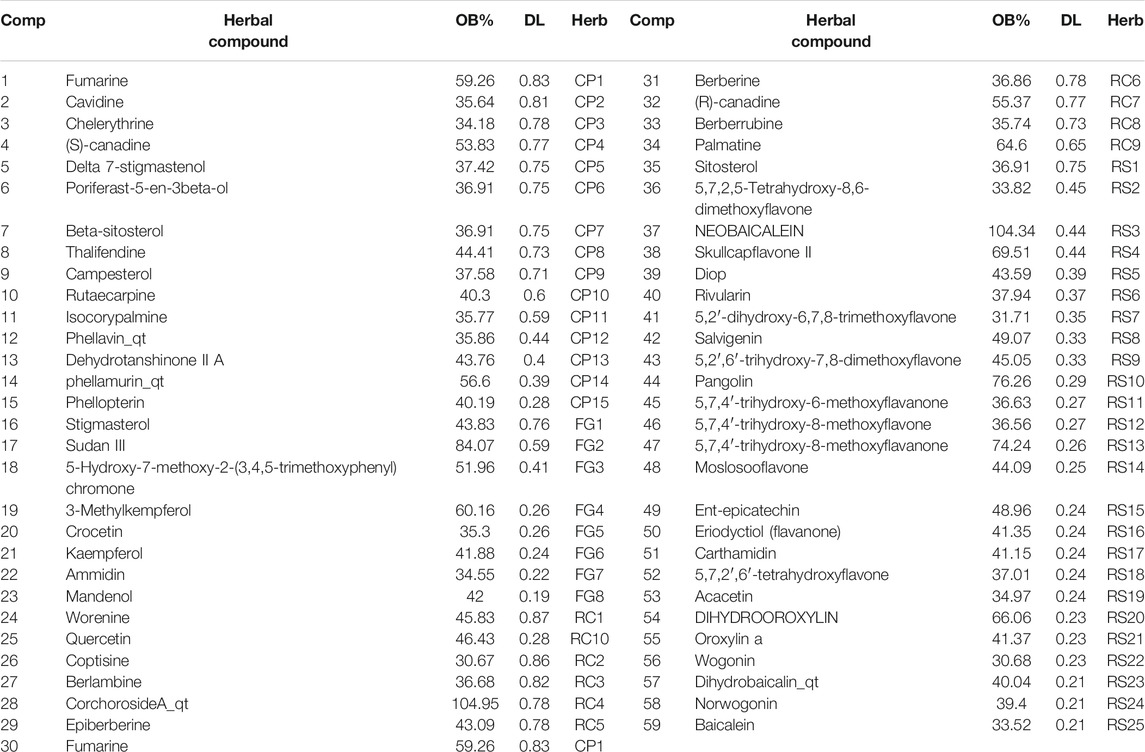
TABLE 1. Compounds in HLJDT with oral bioavailability (OB) larger than 30% and drug-likeness (DL) larger than 0.18.
Identification of Huang-Lian-Jie-Du-Tang-Related Targets
Overall, 2,138 known distinct MI-related targets were eventually collected from the GenesCard database (Supplementary Table S3) and 375 were collected from the OMIM database (Supplementary Table S4), which included almost all the target genes related to MI that have been identified or are being studied. The shared potential target genes of HLJDT with MI-related target genes that correspond to disease progression and treatment were considered potential targets of HLJDT. Among the 2485 MI-related target genes, 75 genes were closely related to HLJDT (Table 2). Figure 3 shows the number of shared overlap targets by HLJDT and MI-related targets.
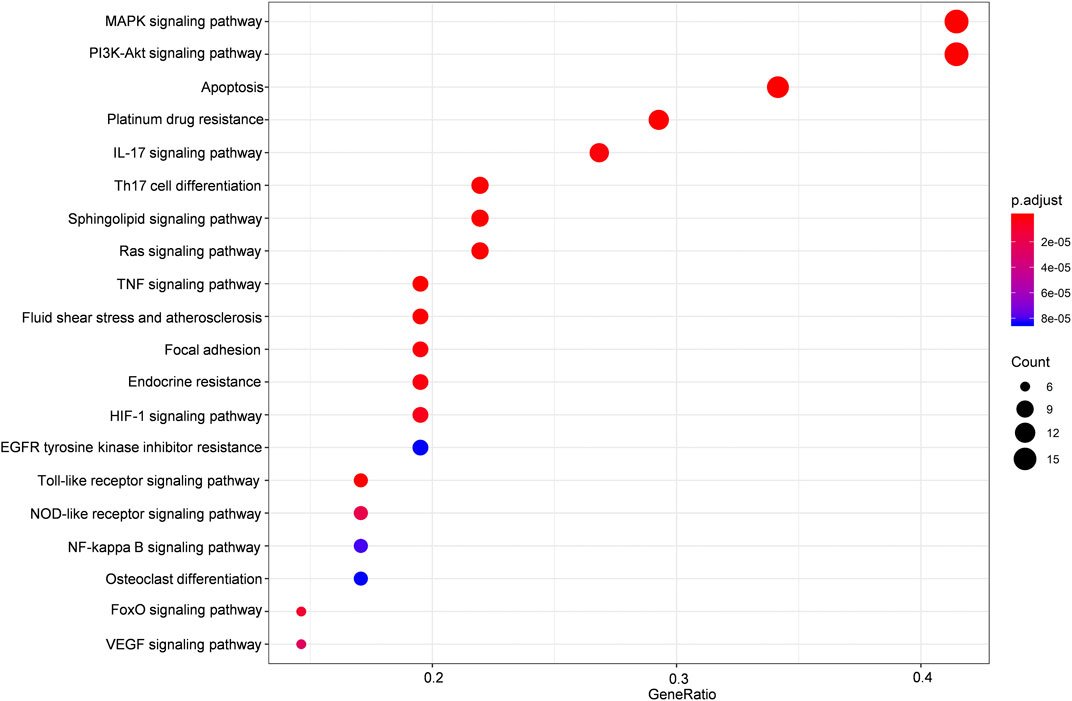
FIGURE 3. KEGG enrichment analysis of the predicted targets of HLJDT for MI treatment. Dot plot showing the top 20 KEGG pathways: the size of the dots corresponds to the number of genes annotated in the entry, and the color of the dots corresponds to the corrected P‐value.
Enrichment Analysis and Construction of a Regulatory Network
The potential target genes of HLJDT obtained above were then imported for KEGG pathway enrichment to explore potential signaling pathways for HLJDT in treating MI. The top 20 potential signaling pathways are listed in Figure 4. Among them, nine pathways including the MAPK signaling pathway (hsa04010), the PI3K-Akt signaling pathway (hsa04151), apoptosis (hsa04210), the IL-17 signaling pathway (hsa04657), Th17 cell differentiation (hsa04659), the sphingolipid signaling pathway (hsa04071), the Ras signaling pathway (hsa04014), the TNF signaling pathway (hsa04668), and fluid shear stress (hsa05418) were mainly found to be involved in apoptosis, inflammation, immunity, or oxidative stress biological processes related to MI.
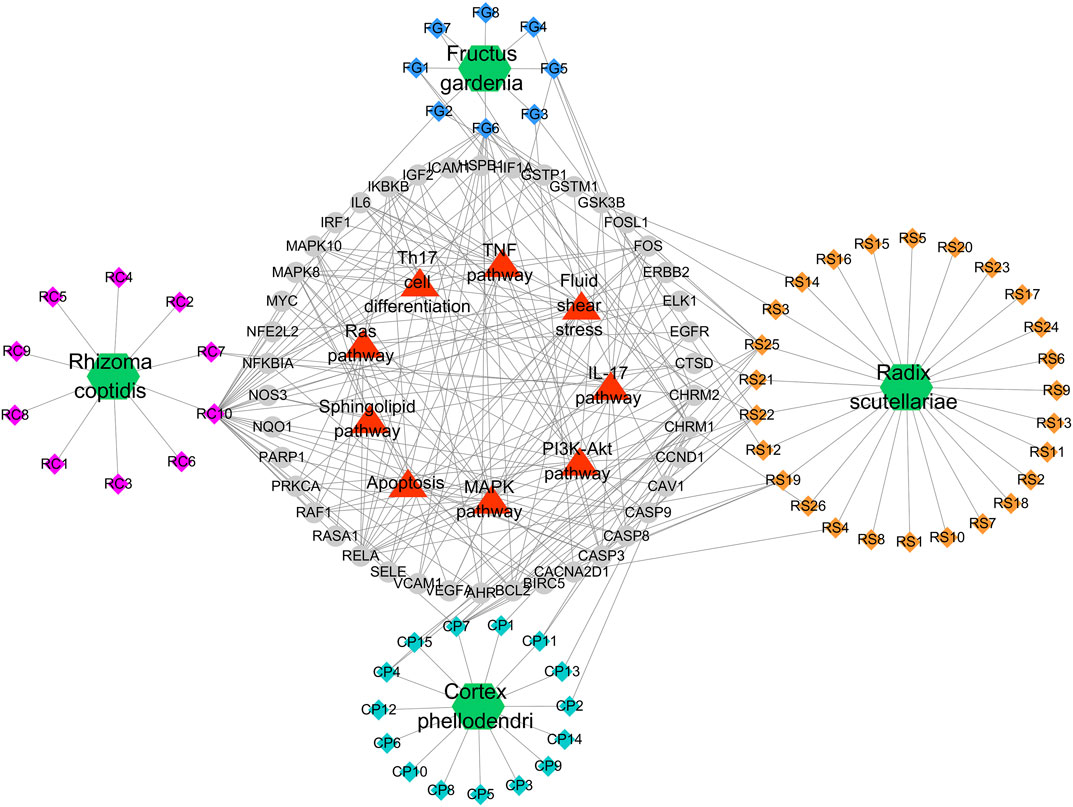
FIGURE 4. Potential Compound-Targets-Pathway (pC-T-P) network of HLJDT in the treatment of MI. There were 4 kinds of herbs, 59 compounds, 42 predicted targets and 9 signaling pathways on the network. Green hexagon represents 4 kinds of herbs of in HLJDT, every color represents the single medicine. Diamond represents compounds, each color represents one herb in HLJDT; Gray circle represents potential targets, while the red triangle represents the predicted signaling pathways. RC, Rhizoma coptidis; RS, Radix scutellariae; CP, Cortex phellodendri; FG, Fructus gardenia.
A global view of the potential compound–target–pathway (C-T-D) network including 114 nodes (4 herbs, 59 compounds, 42 predicted targets, and nine signaling pathways) and 270 edges was constructed (Figure 5) to further clarify the specific mechanism of HLJDT. This network presented the complex relationship among the active components of HLJDT, the target genes, and the related predicted pathways. The top components with the highest number of connections to target nodes were quercetin (RC10) in the rhizoma of Coptis chinensis, kaempferol (FG6) in the fructus of Gardenia jasminoides J. Ellis; oroxylin (RS21), wogonin (RS22), and baicalein (RS25) in the radix of Scutellaria baicalensis Georgi, and β-sitosterol (CP7) in the cortex of Phellodendron chinense C.K. Schneid. Meanwhile, some of the target genes were involved in diverse pathways, for example, MAPK8 participated in diverse pathways, including apoptosis, the PI3K-Akt pathway, the TNF pathway, and the MAPK pathway, while IL-17 was involved in the IL-17 signaling pathway, Th17 cell differentiation, and apoptosis, etc. These results indicated that HLJDT probably acted on MI in a multi-target, multi-pathway, and overall integrative mode.
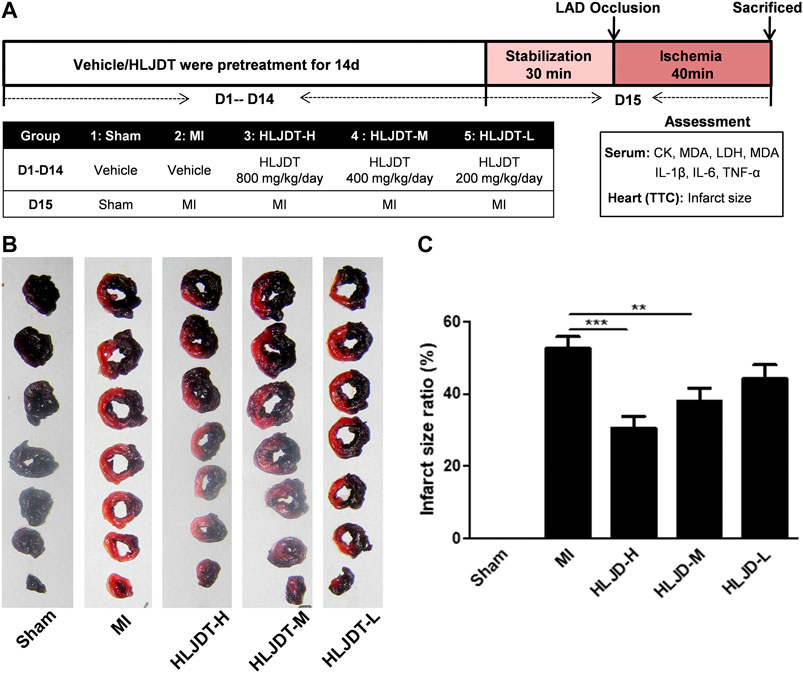
FIGURE 5. HLJDT reduced infarct size after MI. (A)The experimental procedures of in vivo MI rat model; (B) Representative photographs of TTC stained heart slices obtained after MI; (C) Graphic representation of myocardial infarct size. (B) Bar graphic representation of statistical analysis myocardial infarct size (n < 6). All data expressed as mean ± SEM, n=6/group. **p<0.01, ***p<0.001 vs. MI group
In vivo Validation of the Efficacy of Huang-Lian-Jie-Du-Tang
The rat MI model was established to evaluate the potential myocardial protection of HLJDT. Representative photographs of TTC-stained heart slices and the corresponding quantitative statistical analysis of myocardial infarct size obtained after MI are shown in Figures 2B,C, respectively. The myocardial infarction area of the MI group was significantly increased after ischemia-induced injury compared with the sham group. As expected, the average areas of infarct were significantly reduced after pretreatment with HLJDT in a dose dependent manner.
Meanwhile, the MI group exhibited elevated levels of cardiac markers such as creatine kinase (CK), CM-KB, aspartate aminotransferase (AST), lactate dehydrogenase (LDH), anti-oxidant enzymes superoxide dismutase (SOD), inflammation, and immune related factors: interleukin-6 (IL-6), IL-1β, IL-17, and TNF-α, while the MI group exhibited reduced levels of malondialdehyde (MDA). Oral administration of HLJDT could significantly improve the above related levels (Figures 6A–H). The results showed that HLJDT exerted a potential preventive effect against MI.
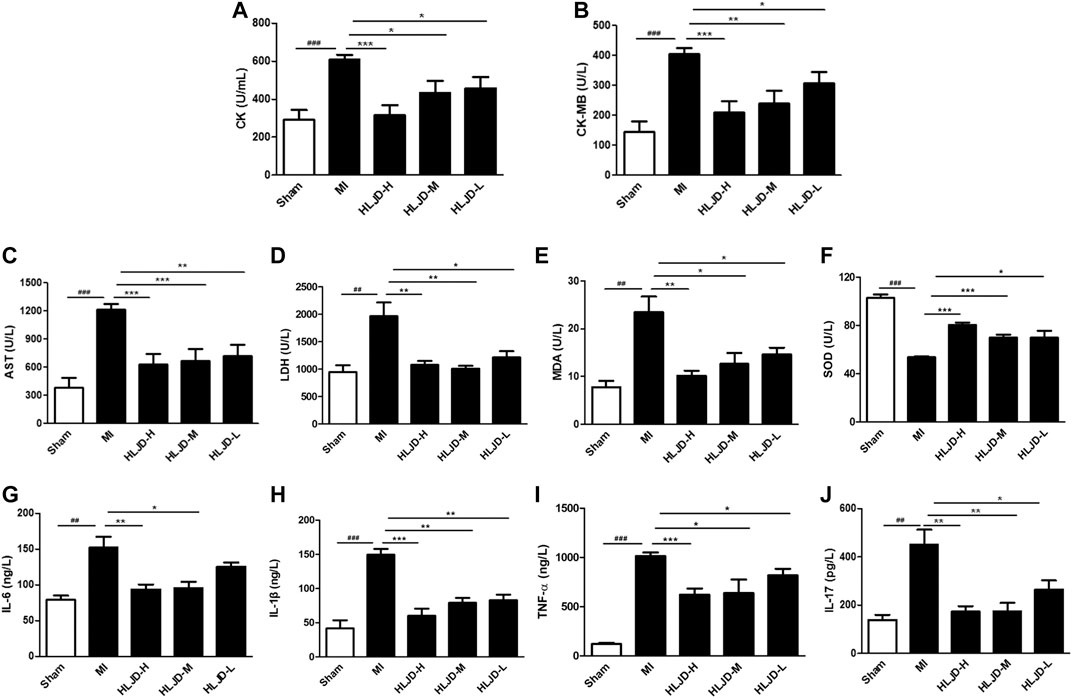
FIGURE 6. The effect of HLJDT on the release of serum biochemical indicators. (A) Creatine kinase (CK); (B) CK-MB; (C) aspartate aminotransferase (AST); (D) lactate dehydrogenase (LDH); (E) malondialdehyde (MDA); (F) superoxide dismutase (SOD); (G) IL-6; (H) IL-1β; (I) TNF-α; and (J) IL-17 (n = 6). Results were presented as mean ± SEM. ##p < 0.01, ###p < 0.001 vs. Sham group; **p < 0.01, ***p < 0.001 vs. MI group.
Metabonomic Analysis
Identification of Potential Metabolites
To identify the potential metabolic markers of HLJDT, the total ion chromatograms (TICs) of serum were extracted from the sham and MI groups. The OPLS score plot proved that the sham group were significantly different from the MI group (R2X = 0.168, Figure 7A). Moreover, the relative S-plot (Figure 7B) reflected the influences of variables on inter-group difference. Those points far from the origin contribute to inter-group difference significantly and correspond to larger VIP values. The S-plot showed the distribution of biomarkers with significant difference. The endogenous metabolites were identified by comparing them with standard references and mass assignments in online databases (Xu et al., 2015). Potential biomarkers were determined by the condition of fold changes larger than 1.5, p values less than 0.05, and VIP values larger than 2.0. They were PA (20:1 (11Z)/0:0), phytosphingosine, spermic acid 2, phenylacetaldehyde, prostaglandin E1, heptadecanoic acid, thromboxane, piperidine, carbamic acid, sphingosine, acetamide, ethionamide sulphoxide, PA (15:0/22:1 (13Z)), and cis-3-Chloroallyl aldehyde. The distribution of 14 metabolites were visually displayed by heatmap (Figure 7C). The corresponding detailed information including chemical name, m/z, formula, and differences are listed in Table 3. These metabonomic results demonstrate the efficacy of HLJDT.
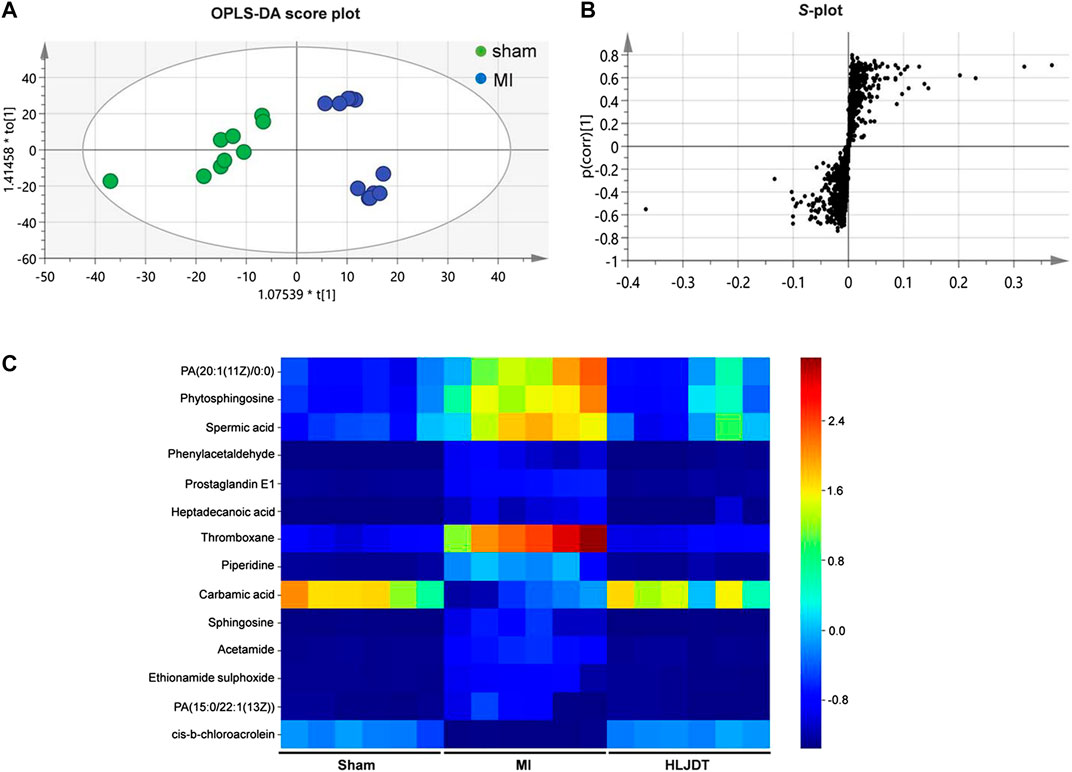
FIGURE 7. Metabolic data analysis of serum by UPLC-Q-TOF/MS (A) OPLS-DA score plot of serum samples from sham and MI model rats in positive mode (B) S-plot from OPLS-DA of serum samples from sham and MI model rats in positive mode (C) Comparison of normalized mass spectrometric peak intensities for potential biomarkers in different groups. Potential biomarkers were determined by the condition of fold changes larger than 1.5, p values less than 0.05, and VIP values larger than 2.0. They were PA (20:1 (11Z)/0:0), phytosphingosine, spermic acid 2, phenylacetaldehyde, prostaglandin E1, heptadecanoic acid, thromboxane, piperidine, carbamic acid, sphingosine, acetamide, ethionamide sulphoxide, PA (15:0/22:1 (13Z)), and cis-3-Chloroallyl aldehyde.
Pathway Analysis
The metabolic pathways closely related to the 14 difference markers were further analyzed by MetaboAnalyst 4.0. As shown in Figure 8, the top three pathways including phenylalanine metabolism, sphingolipid metabolism, and arachidonic acid metabolism played an important role in regulating MI.
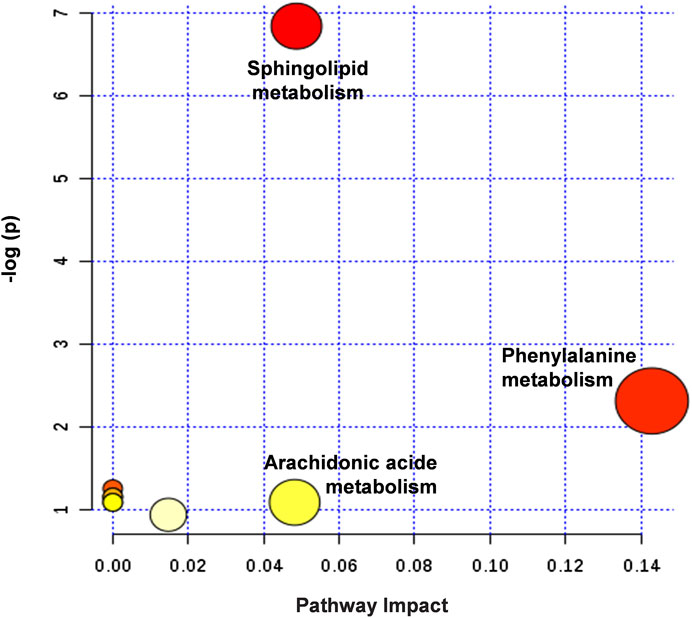
FIGURE 8. Summary of potential metabonomic pathways in MI injury treated by HLJDT. Among these metabolic pathways, phenylalanine metabolism, sphingolipid metabolism, and arachidonic acid metabolism were filtered out, which were considered as the most significant metabolic pathways.
Integration of Network Pharmacology and Metabonomics
To integrate the above network pharmacology results and metabonomics data and explore the understanding of the underlying mechanisms from a systematic perspective, we focused on the correlation of the signaling pathway and metabolites through their common biological functions related to the pathophysiology of MI. A network with interactions among HLJDT compounds, targets, signaling pathways, metabolic pathways, and biological processes affected by HLJDT on MI is illustrated in Figure 9. The network suggested that the mechanism of HLJDT acting on MI was based on the attenuation of apoptosis, oxidative stress, and immune and inflammatory responses. This could lay the foundation for the biological connotation of the efficacy of the medicine and provide support for the next step of the “disease-syndrome-formula” of HLJDT.
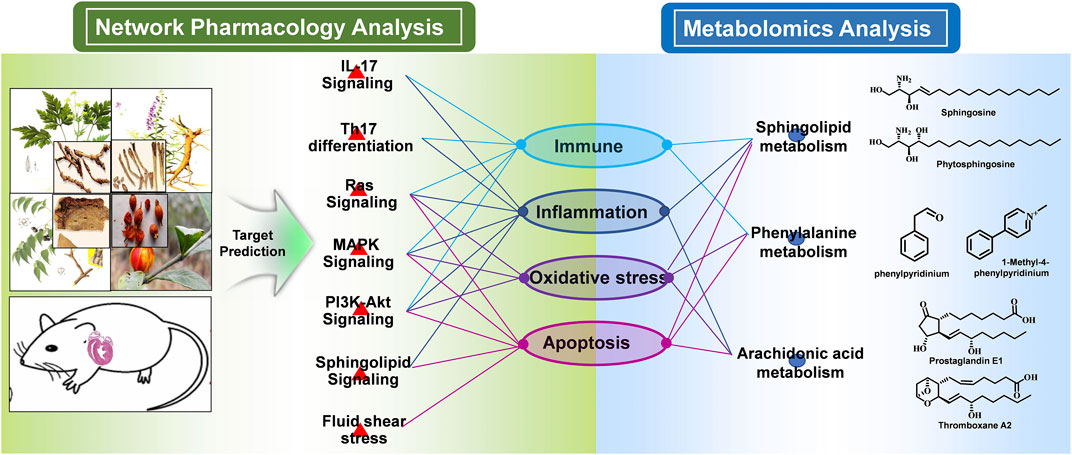
FIGURE 9. Integrated mechanism of network pharmacology and metabolomic analysis. The main signaling pathways, differentially regulated metabolites, and metabolic pathways were combined logically through the biological functions. From the pathway perspective, the HLJDT targets were significantly enriched in seven pathways including Th17 differentiation, IL-17, RAS, MAPK, Pi3k-Akt, sphingolipid, and fluid shear stress signaling pathways, which were critical in the regulation of the immune, inflammation, oxidative stress, and apoptosis process associated with MI. From the metabolomics perspective, the GO and KEGG pathway enrichment analysis of the differential metabolites revealed consistent results. In summary, network pharmacology analysis suggested that HLJDT could act as an immunomodulatory, anti-inflammatory, antioxidant stress, and anti-apoptotic agent to exert anti-MI effects.
Discussion
As TCM has the characteristics of a multi-component, multi-target, and multi-pathway synergistic action pattern, it is inappropriate to use the mode of “one drug-one target” to elaborate its mechanism. Network pharmacology is a new strategy for exploring the relationship between drugs and diseases from a global perspective by integrating system biology, multi-directional pharmacology, and computational biology. It is especially suitable for interpreting the complex relationship between drugs, targets, pathways, and diseases (Hopkins, 2008; Li and Zhang, 2013).
In our study, a global view of the potential compound–target–pathway network based on network pharmacology was constructed to investigate the molecular mechanism and potential targets of HLJDT in the treatment of MI. Active ingredients related to HLJDT were obtained from TCMSP (Ru et al., 2014). Protein targets directly affected by myocardial ischemia in HLJDT were screened through the Mendelian genetic database and biological pathway enrichment on the KEGG database (Li et al., 2012). Except for effective ingredients and target genes, the network revealed several meaningful signaling pathways including apoptosis, the PI3K-Akt signaling pathway, the TNF signaling pathway, the MAPK signaling pathway, the IL-17 signaling pathway, Th17 cell differentiation, and the sphingolipid pathway. Biological function enrichment and literature research inferred that these pathways are mainly related to the oxidative stress, apoptosis, inflammation, and immune response involved in MI progression (Whelan et al., 2010; Li et al., 2011; Li et al., 2012; Sinning et al., 2017; Yamamoto et al., 2017). These data provided a preliminary understanding and provoked our interest for further study.
After preliminary identification of the multi-dimensional regulatory network in the treatment of MI, the in vivo potency of HLJDT was validated in the MI rat model. HLJDT pretreatment significantly reduced the area of MI, and decreased the ameliorated myocardial injury biochemical markers CK, MDA, and LDH, anti-oxidant enzymes SOD, and inflammation and immune factors: IL-6, IL-1β, IL-17, and TNF-α. These results suggested that HLJDT exerted a potential preventive effect against MI.
Metabonomics is a discipline in which the metabolic changes of organisms are quantitatively detected at different times and in multiple directions under the conditions of pathophysiological stimulation and genetic factors change, and the mechanism of gene function regulation is explored by measuring the metabolic map of the whole organism (Jia et al., 2006). Metabonomics can be used to explore the correlation between metabolites and physiological and pathological changes through high-throughput analysis of metabolites in the body, and clarify the interaction of complex systems and responses to the outside body. Metabonomics mainly focus on the variety and quantity of endogenous small molecule metabolites (generally referred to MW < 1,000) under the action of internal and external factors (such as disease invasion, drug intervention, and environmental change, etc.) and their interrelations. Metabonomics studies the human body as one system, and has unique advantages in revealing the mechanism of complex diseases and drug metabolism mode, thus providing new ideas for the study of the complex system of TCM (Shyur and Yang, 2008).
Metabonomic analysis showed that there were 29 metabolites with significant differences between the sham group and MI group, where 14 biomarkers were regulated by HLJDT pretreatment, namely (PA (20:1 (11Z)/0:0), phytosphingosine, spermic acid 2, phenylacetaldehyde, prostaglandin E1, heptadecanoic acid, thromboxane, piperidine, carbamic acid, sphingosine, acetamide, ethionamide sulphoxide, PA (15:0/22:1 (13Z)), and cis-3-Chloroallyl aldehyde. These metabolites were mainly involved in three metabolic pathways including sphingolipid metabolism, phenylalanine metabolism, and arachidonic acid (AA) metabolism (Gross et al., 2005). The existing research shows that sphingolipid is a kind of complex compound in the skeleton and plays an important role in maintaining cell growth, signal transduction, and inflammation (Egom et al., 2012). An abnormal sphingolipid may cause atherosclerosis, cardiomyopathy, cancer, and other diseases (Jiang et al., 2011; Glaser and Fandrey, 2018). Ceramide is at the center of intracellular sphingolipid metabolism. It has the biological function to induce apoptosis, regulating cell differentiation, cellular immunity, and inflammatory response (Bai et al., 2007). The AA metabolic pathway plays an important role in the process of MI, as the main metabolites prostacyclin and thromboxane are closely related to the biological processes of vasodilation, anti-inflammatory, and anti-oxidation during the development of MI (Gross et al., 2005).
Notably, in the main target genes and differentially regulated makers, the sphingolipid signaling pathway was found to be the only shared pathway. According to the literature, sphingolipids, the critical composition of the cell membrane, regulates cellular growth, differentiation, and aging (Mendelson et al., 2014). It includes sphingomyelin, cerebroside, and ganglioside. Among them, sphingomyelin plays a significant role in acute leukemia disease (Li et al., 2014). Some studies have indicated that sphingolipids were involved in inflammation, apoptosis, and cellular immunity response (Testai et al., 2014). Sphingosine 1-phosphate (S1P) is a biologically active sphingolipid and plays an important role in tumor genesis, the cardiovascular system, and the immune system through distinct signal transduction pathways (Mendelson et al., 2014). During the process of MI, S1P may play a significant role in the biological process of vascular endothelial protection, the inhibition of inflammation, and oxidative stress. Here we can reasonably speculate that during the early stage of MI, the inflammatory cells are stimulated by hypoxia to produce many kinds of proinflammatory factors. With the continuous activation and release of inflammatory factors, a positive feedback effect is formed, which leads to the loss of inflammatory response and serious myocardial damage (Knapp et al., 2012). HLJDT was proven to reduce the production of proinflammatory factors including IL-1β, TNF-α, IL-6, as well as IL-17, thus it is of great significance in alleviating ischemia.
Conclusion
The present study demonstrated the myocardial protection of HLJDT. An integrative approach of network pharmacology and metabonomic was performed to investigate the biological mechanisms of HLJDT for treating MI. By comprehensive analysis of the potential compound–target–pathway network and metabolic pathway enrichment, we speculated that the action mechanisms of HLJDT were attributed to the biological process of oxidative stress, apoptosis, and immune and inflammatory responses by regulating the sphingolipid pathway, PI3K-Akt pathway, and IL-17 signaling pathway. Meanwhile, the integrated network indicated that the top ingredients with the highest number of connections to target nodes were quercetin in the rhizoma of Coptis chinensis Franch, kaempferol in the fructus of Gardenia jasminoides J. Ellis, oroxylin, wogonin, and baicalein in the radix of Scutellaria baicalensis Georgi, and β-sitosterolin in the cortex of Phellodendron chinense C.K. Schneid. The results demonstrated the myocardial protection effect of HLJDT on ischemia with a multicomponent-multitarget mode. This study highlights the reliability and effectiveness of a network pharmacology-based approach that identifies and validates the complex of natural compounds in HLJDT for illustrating the mechanism of action in the treatment of MI.
Data Availability Statement
The raw data supporting the conclusion of this article will be made available by the authors, without undue reservation, to any qualified researcher.
Ethics Statement
The animal study was reviewed and approved by Second Military Medical University and government.
Author Contributions
Conceptualization: LL and HH. Data curation: WL and YZ. Formal analysis: WL, YZ, and CG. Funding acquisition: LL and WL. Investigation: LL, CG, and AZ. Methodology: LL, WD, WL, YW, and AZ. Project administration: HH and YL. Supervision: LL, HH, and YL. Validation: LL, WD, WL, and YW. Visualization: LL and YZ. Writing—original draft: LL. Writing—review & editing: LL.
Funding
This study was supported by the Outstanding Youth Foundation of the Eighth Affiliated Hospital, Sun Yat-sen University (No. 201700110), the Public Health Research Project of Futian District in Shenzhen (No. FTWS2017017), the Natural Science Foundation of Guangdong Province (No. 2018A030310318), and the Teacher Training Program for Young Teachers of Sun Yat-sen University (No. 0ykpy02).
Conflict of Interest
The authors declare that the research was conducted in the absence of any commercial or financial relationships that could be construed as a potential conflict of interest.
Acknowledgments
The authors sincerely acknowledge the support of Dr Dagui Chen for feeding the experimental rats and Dr Zhongxiao Zhang for metabonomics analysis during this work.
Supplementary Material
The Supplementary Material for this article can be found online at: https://www.frontiersin.org/articles/10.3389/fphar.2020.589175/full#supplementary-material.
References
Arslan, F., De Kleijn, D. P., and Pasterkamp, G. (2011). Innate immune signaling in cardiac ischemia. Nat. Rev. Cardiol. 8, 292–300. doi:10.1038/nrcardio.2011.38
Bai, Y., Wang, J., Shan, H., Lu, Y., Zhang, Y., Luo, X., et al. (2007). Sphingolipid metabolite ceramide causes metabolic perturbation contributing to HERG K+ channel dysfunction. Cell. Physiol. Biochem. 20, 429–440. doi:10.1159/000107527
Chen, Y., Zhang, H., Li, Y. X., Cai, L., Huang, J., Zhao, C., et al. 2010). Crocin and geniposide profiles and radical scavenging activity of gardenia fruits (Gardenia jasminoides Ellis) from different cultivars and at the various stages of maturation. Fitoterapia. 81, 269–273. doi:10.1016/j.fitote.2009.09.011
Davidson, S. M., Ferdinandy, P., Andreadou, I., Bøtker, H. E., Heusch, G., Ibáñez, B., et al. (2019). Multitarget strategies to reduce myocardial ischemia/reperfusion injury: JACC review topic of the week. J. Am. Coll. Cardiol. 73, 89–99. doi:10.1016/j.jacc.2018.09.086
Egom, E. E., Mamas, M. A., and Clark, A. L. (2012). The potential role of sphingolipid-mediated cell signaling in the interaction between hyperglycemia, acute myocardial infarction and heart failure. Expert Opin. Ther. Targets. 16, 791–800. doi:10.1517/14728222.2012.699043
Garg, S., Hofstra, L., Reutelingsperger, C., and Narula, J. (2003). Apoptosis as a therapeutic target in acutely ischemic myocardium. Curr. Opin. Cardiol. 18, 372–377. doi:10.1097/00001573-200309000-00009
Glaser, U. G., and Fandrey, J. (2018). Sphingolipids in inflammatory hypoxia. Biol. Chem. 399, 1169–1174. doi:10.1515/hsz-2018-0173
Gross, G. J., Falck, J. R., Gross, E. R., Isbell, M., Moore, J., and Nithipatikom, K. (2005). Cytochrome P450 and arachidonic acid metabolites: role in myocardial ischemia/reperfusion injury revisited. Cardiovasc. Res. 68, 18–25. doi:10.1016/j.cardiores.2005.06.007
Guo, S., Li, P., Fu, B., Chuo, W., Gao, K., Zhang, W., et al. (2016). Systems-biology dissection of mechanisms and chemical basis of herbal formula in treating chronic myocardial ischemia. Pharmacol. Res. 114, 196–208. doi:10.1016/j.phrs.2016.10.026
Han, J., Ye, M., Guo, H., Yang, M., Wang, B. R., and Guo, D. A. (2007). Analysis of multiple constituents in a Chinese herbal preparation Shuang-Huang-Lian oral liquid by HPLC-DAD-ESI-MSn. J. Pharmaceut. Biomed. Anal. 44, 430–438. doi:10.1016/j.jpba.2007.02.023
Hopkins, A. L. (2008). Network pharmacology: the next paradigm in drug discovery. Nat. Chem. Biol. 4, 682–690. doi:10.1038/nchembio.118
Jia, W., Liu, P., Jiang, J., Chen, M. J., Zhao, L. P., Zhou, M. M., et al. (2006). Application of metabonomics in complicated theory system research of traditional Chinese medicine. Zhongguo Zhongyao Zazhi. 31, 621–624.
Jiang, X. C., Goldberg, I. J., and Park, T. S. (2011). Sphingolipids and cardiovascular diseases: lipoprotein metabolism, atherosclerosis and cardiomyopathy. Adv. Exp. Med. Biol. 721, 19–39. doi:10.1007/978-1-4614-0650-1_2
Katopodis, J. N., Kolodny, L., Jy, W., Horstman, L. L., De Marchena, E. J., Tao, J. G., et al. (1997). Platelet microparticles and calcium homeostasis in acute coronary ischemias. Am. J. Hematol. 54, 95–101.
Kaul, S., and Ito, H. (2004). Microvasculature in acute myocardial ischemia: part II: evolving concepts in pathophysiology, diagnosis, and treatment. Circulation. 109, 310–315. doi:10.1161/01.CIR.0000111583.89777.F9
Knapp, M., Zendzian-Piotrowska, M., Błachnio-Zabielska, A., Zabielski, P., Kurek, K., and Górski, J. (2012). Myocardial infarction differentially alters sphingolipid levels in plasma, erythrocytes and platelets of the rat. Basic Res. Cardiol. 107, 294. doi:10.1007/s00395-012-0294-0
Lee, S. H., Wolf, P. L., Escudero, R., Deutsch, R., Jamieson, S. W., and Thistlethwaite, P. A. (2000). Early expression of angiogenesis factors in acute myocardial ischemia and infarction. N. Engl. J. Med. 342, 626–633. doi:10.1056/NEJM200003023420904
Li, S., and Zhang, B. (2013). Traditional Chinese medicine network pharmacology: theory, methodology and application. Chin. J. Nat. Med. 11, 110–120. doi:10.1016/S1875-5364(13)60037-0
Li, Y., Si, R., Feng, Y., Chen, H. H., Zou, L., Wang, E., et al. (2011). Myocardial ischemia activates an injurious innate immune signaling via cardiac heat shock protein 60 and toll-like receptor 4. J. Biol. Chem. 286, 31308–31319. doi:10.1074/jbc.M111.246124
Li, J., Zhang, H., and Zhang, C. (2012). Role of inflammation in the regulation of coronary blood flow in ischemia and reperfusion: mechanisms and therapeutic implications. J. Mol. Cell. Cardiol. 52, 865–872. doi:10.1016/j.yjmcc.2011.08.027
Li, L., Zeng, H., Shan, L., Yuan, X., Li, Y., Liu, R., et al. (2012). The different inhibitory effects of Huang-Lian-Jie-Du-Tang on cyclooxygenase 2 and 5-lipoxygenase. J. Ethnopharmacol. 143, 732–739. doi:10.1016/j.jep.2012.07.037
Li, X. Z., Liu, Z. P., Li, J., and Fang, H. S. (2012). Recent progress and application of KEGG database in the research of bioinformatics. Pharmaceut. Biotechnol. 19, 535–539.
Li, W., Tang, Y., Guo, J., Shang, E., Qian, Y., Wang, L., et al. (2014). Comparative metabolomics analysis on hematopoietic functions of herb pair Gui-Xiong by ultra-high-performance liquid chromatography coupled to quadrupole time-of-flight mass spectrometry and pattern recognition approach. J. Chromatogr., A. 1346, 49–56. doi:10.1016/j.chroma.2014.04.042
Liu, Q., Li, J., Wang, J., Li, J., Janicki, J. S., and Fan, D. (2013). Effects and mechanisms of Chinese herbal medicine in ameliorating myocardial ischemia-reperfusion injury. Evid Based Complement Alternat Med. 2013, 925625. doi:10.1155/2013/925625
Luqman, N., Sung, R. J., Wang, C. L., and Kuo, C. T. (2007). Myocardial ischemia and ventricular fibrillation: pathophysiology and clinical implications. Int. J. Cardiol. 119, 283–290. doi:10.1016/j.ijcard.2006.09.016
Lv, Y., Wang, J., Xu, D., Liao, S., Li, P., Zhang, Q., et al. (2017). Comparative study of single/combination use of Huang-Lian-Jie-Du decoction and berberine on their protection on sepsis induced acute liver injury by NMR metabolic profiling. J. Pharmaceut. Biomed. Anal. 145, 794–804. doi:10.1016/j.jpba.2017.07.062
Ma, C. H., Li, Z. X., Wang, L. X., Tang, Y. H., Xiao, H. B., and Huang, C. G. (2009). Identification of major alkaloids in rat urine by HPLC/DAD/ESI-MS/MS method following oral administration of Cortex Phellodendri decoction. Helv. Chim. Acta. 92, 379–398. doi:10.1002/hlca.200800315
Mendelson, K., Evans, T., and Hla, T. (2014). Sphingosine 1-phosphate signalling. Development. 141, 5–9. doi:10.1242/dev.094805
Ru, J., Li, P., Wang, J., Zhou, W., Li, B., Huang, C., et al. (2014). TCMSP: a database of systems pharmacology for drug discovery from herbal medicines. J. Cheminf. 6, 13. doi:10.1186/1758-2946-6-13
Rui, T., Feng, Q., Lei, M., Peng, T., Zhang, J., Xu, M., et al. (2005). Erythropoietin prevents the acute myocardial inflammatory response induced by ischemia/reperfusion via induction of AP-1. Cardiovasc. Res. 65, 719–727. doi:10.1016/j.cardiores.2004.11.019
Sekiya, N., Kainuma, M., Hikiami, H., Nakagawa, T., Kouta, K., Shibahara, N., et al. (2005). Oren-gedoku-to and keishi-bukuryo-gan-ryo inhibit the progression of atherosclerosis in diet-induced hypercholesterolemic rabbits. Biol. Pharm. Bull. 28, 294–298. doi:10.1248/bpb.28.294
Shen, J., Cao, X. F., and Liu, J. F. (2003). Studies on HPLC fingerprint of several kinds of huanglianjiedu decoction. Chinese Traditional Patent Medicine. 25, 433. doi:10.3969/j.issn.1001-1528.2003.06.001
Shyur, L. F., and Yang, N. S. (2008). Metabolomics for phytomedicine research and drug development. Curr. Opin. Chem. Biol. 12, 66–71. doi:10.1016/j.cbpa.2008.01.032
Sinning, C., Westermann, D., and Clemmensen, P. (2017). Oxidative stress in ischemia and reperfusion: current concepts, novel ideas and future perspectives. Biomarkers Med. 11, 11031–11040. doi:10.2217/bmm-2017-0110
Testai, F. D., Kilkus, J. P., Berdyshev, E., Gorshkova, I., Natarajan, V., and Dawson, G. (2014). Multiple sphingolipid abnormalities following cerebral microendothelial hypoxia. J. Neurochem. 131, 530–540. doi:10.1111/jnc.12836
Turer, A. T., and Hill, J. A. (2010). Pathogenesis of myocardial ischemia-reperfusion injury and rationale for therapy. Am. J. Cardiol. 106, 360–368. doi:10.1016/j.amjcard.2010.03.032
Wang, J., Liu, X., Ren, B., Rupp, H., Takeda, N., and Dhalla, N. S. (2002). Modification of myosin gene expression by imidapril in failing heart due to myocardial infarction. J. Mol. Cell. Cardiol. 34, 847–857. doi:10.1006/jmcc.2002.2023
Wang, Y., Fan, X., Qu, H., Gao, X., and Cheng, Y. (2012). Strategies and techniques for multi-component drug design from medicinal herbs and traditional Chinese medicine. Curr. Top. Med. Chem. 12, 1356–1362. doi:10.2174/156802612801319034
Wang, P. R., Wang, J. S., Zhang, C., Song, X. F., Tian, N., and Kong, L. Y. (2013). Huang-Lian-Jie-Du-Decoction induced protective autophagy against the injury of cerebral ischemia/reperfusion via MAPK-mTOR signaling pathway. J. Ethnopharmacol. 149, 270–280. doi:10.1016/j.jep.2013.06.035
Whelan, R. S., Kaplinskiy, V., and Kitsis, R. N. (2010). Cell death in the pathogenesis of heart disease: mechanisms and significance. Annu. Rev. Physiol. 72, 19–44. doi:10.1146/annurev.physiol.010908.163111
Xu, X., Zhang, W., Huang, C., Li, Y., Yu, H., Wang, Y., et al. (2012). A novel chemometric method for the prediction of human oral bioavailability. Int. J. Mol. Sci. 13, 6964–6982. doi:10.3390/ijms13066964
Xu, W., Chen, D., Wang, N., Zhang, T., Zhou, R., Huan, T., et al. (2015). Development of high-performance chemical isotope labeling LC-MS for profiling the human fecal metabolome. Anal. Chem. 87, 829–836. doi:10.1021/ac503619q
Keywords: HLJDT, myocardial ischemia, network pharmacology, metabonomics, multitarget
Citation: Li L, Dai W, Li W, Zhang Y, Wu Y, Guan C, Zhang A, Huang H and Li Y (2021) Integrated Network Pharmacology and Metabonomics to Reveal the Myocardial Protection Effect of Huang-Lian-Jie-Du-Tang on Myocardial Ischemia. Front. Pharmacol. 11:589175. doi: 10.3389/fphar.2020.589175
Received: 30 July 2020; Accepted: 02 December 2020;
Published: 04 February 2021.
Edited by:
Francesco Rossi, University of Campania Luigi Vanvitelli, ItalyReviewed by:
Shuping Wang, School of Medicine, Shanghai Jiao Tong University, ChinaYuanyan Liu, Beijing University of Chinese Medicine, China
Hui Hui Zhao, School of Traditional Chinese Medicine, Beijing University of Chinese Medicine, China
Copyright © 2021 Li, Dai, Li, Zhang, Wu, Guan, Zhang, Huang and Li. This is an open-access article distributed under the terms of the Creative Commons Attribution License (CC BY). The use, distribution or reproduction in other forums is permitted, provided the original author(s) and the copyright owner(s) are credited and that the original publication in this journal is cited, in accordance with accepted academic practice. No use, distribution or reproduction is permitted which does not comply with these terms.
*Correspondence: Hui Huang, huanghui765@163.com; Yuzhen Li, Yuzhen628@126.com