- 1Division of Gastroenterology, Massachusetts General Hospital and Harvard Medical School, Boston, MA, United States
- 2Institute of Molecular Pathobiochemistry, Experimental Gene Therapy and Clinical Chemistry (IFMPEGKC), University Hospital, RWTH Aachen, Aachen, Germany
Both acute and chronic hepatic inflammation likely result from an imbalance in the TH1/TH2 cell response and can lead to liver fibrosis and end-stage liver disease. More recently, a novel CD4+ T helper cell subset was described, characterized by the production of IL-17 and IL-22. These TH17 cells 50were predominantly implicated in host defense against infections and in autoimmune diseases. Interestingly, studies over the last 10 years revealed that the development of TH17 cells favors pro-inflammatory responses in almost all tissues and there is a reciprocal relationship between TH17 and TReg cells. The balance between TH17and TReg cells is critical for immune reactions, especially in injured liver tissue and the return to immune homeostasis. The pathogenic contribution of TH17 and TReg cells in autoimmunity, acute infection, and chronic liver injury is diverse and varies among disease etiologies. Understanding the mechanisms underlying TH17 cell development, recruitment, and maintenance, along with the suppression of TReg cells, will inform the development of new therapeutic strategies in liver diseases. Active manipulation of the balance between pathogenic and regulatory processes in the liver may assist in the restoration of homeostasis, especially in hepatic inflammation.
Introduction
CD4 T cells play a central role in mediating the host immune response to pathogens and in autoimmunity, cancer, and chronic inflammation. They maintain and enhance CD8 T cell responses, interact with B cells to induce antibody development, regulate the function of monocytes/macrophages, and orchestrate the immune response to pathogens. CD4+ T cells also modulate immune homeostasis by suppressing pro-inflammatory immune responses, build immunologic memory, and control autoimmunity (Zhu et al., 2010). These functions are achieved through the differentiation of naïve CD4+ T cells into subsets of effector, memory, and regulatory T cells.
T cell activation and differentiation rely on different stimuli, and differentiation is initiated by a cognate antigen presented by specialized antigen-presenting cells (APCs) or other immune cells. Fragmented antigens are presented on major histocompatibility complex 2 (MHC-2) molecules and recognized by the T cell receptor (TCR). Various co-stimulatory receptors and cytokines are essential for T cell activation and determine the direction of T cell differentiation.
Distinct subpopulations of CD4 T cells originating from a common precursor were first described in 1986: in mouse T cell clones, Mosmann and Coffman found that two types of T helper cells could be distinguished by their cytokine production, lymphokine activity, and transcription factor and surface marker expression (Mosmann et al., 1986). The authors defined type 1 T helper cells (TH1) by their secretion of interferon-γ (IFN-γ), interleukin-2 (IL-2) and tumor necrosis factor-α (TNF-α). Type 2 T helper cells (TH2) were characterized by the expression of IL-4, IL-5, and IL-13. Interestingly, the cytokines secreted by each mature T helper cell subset directly antagonize the development and differentiation of the corresponding opposite T helper cell subtype, thereby sustaining lineage-specific immune responses.
This profile has since been refined. Intensive studies of the complex cytokine milieu and transcription factor networks involved in the differentiation of CD4 T helper cell subsets originating from the same naïve CD4 T cell precursor identified many more T helper cell subsets. The potentially distinct T cell lineages include not only TH1, TH2, TH17, and peripheral regulatory T cells (pTReg) cells, but also TH9, TH22, regulatory type 1 (Tr1), and follicular helper T cells (TFH) (Saravia et al., 2019). CD4+ T cell lineages are now understood to be a plastic and flexible network. One CD4+ subset cannot differentiate from only one distinct precursor cell, but rather differentiates from different subsets depending on the environmental milieu (Figure 1).
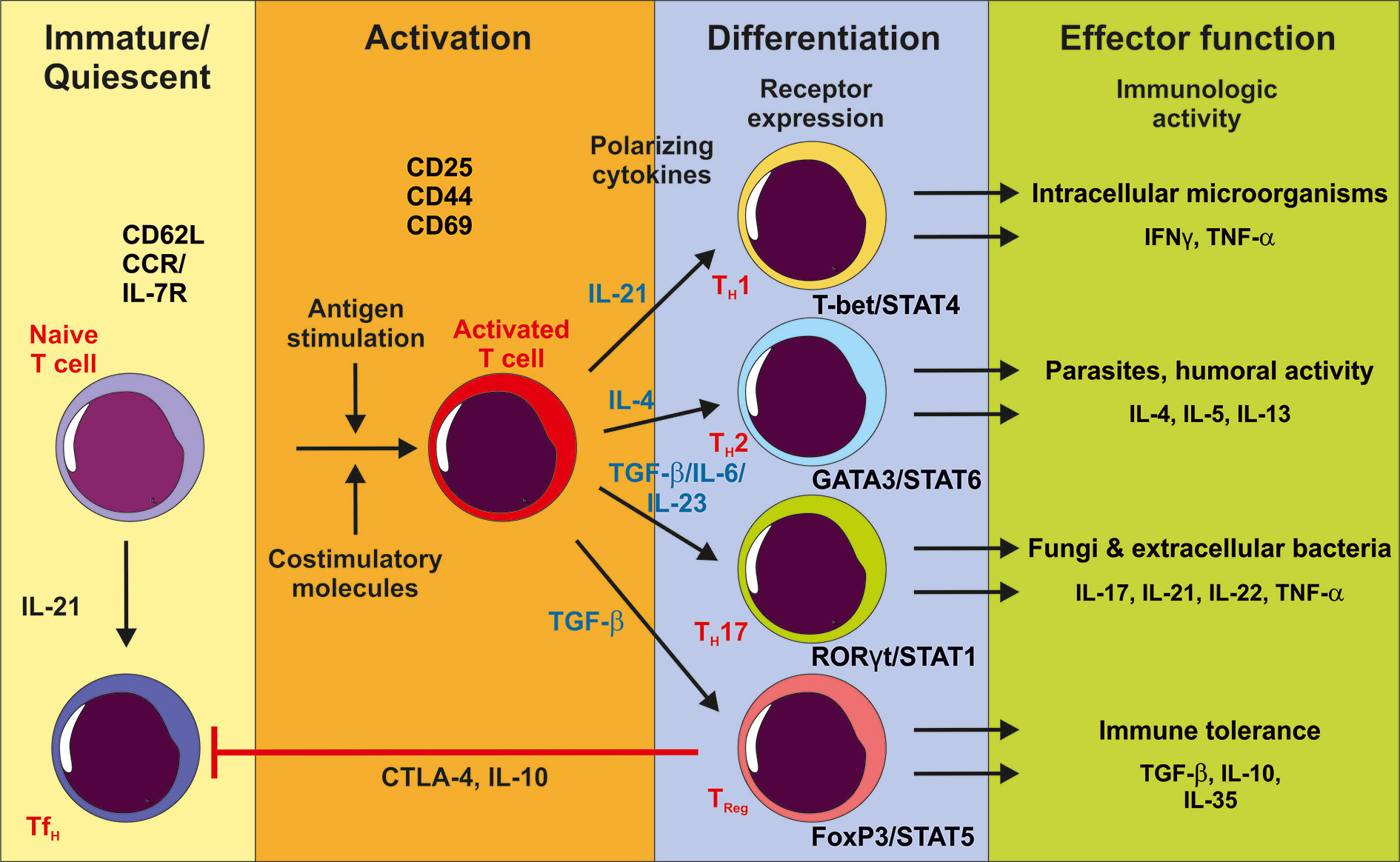
Figure 1 After activation of the T cell receptor by antigen stimulation and co-stimulation, immature CD4+ naïve T cells proliferate and can differentiate into different effector T cells depending on the cytokine milieu. IL-21 stimulation promotes the TH1 subpopulation accompanied with T-bet/STAT4 expression and effector function against intracellular pathogens. TH2 cell populations develop after IL-4 stimulation with GATA3/STAT6 upregulation and supporting anti-parasite immune response and humoral activity. TGF-β in the presence of pro-inflammatory cytokines promotes TH17 cell differentiation. TH17 cells support the immune response against fungi and extracellular bacteria, whereas TReg cells have an immune tolerant function by producing anti-inflammatory cytokines and inhibiting TFH function. Although these specific cytokines are important for CD4+ T cell subpopulation development, the differentiation is a plastic and flexible network.
In this review, we discuss developmental differences between TH17 and TReg cells and their roles in health and disease, with a focus on liver disease. The fragile balance between these two cell types was recently found to play a crucial role in maintaining immune homeostasis. A shift of this balance drives pro-inflammatory immune responses, especially in chronic inflammatory diseases, cancer and autoimmunity. Here, we highlight the intrahepatic effects of this balance in acute and chronic inflammation as well as in liver cancer and autoimmunity.
TH17 Cells in Health and Disease
A T helper cell subset of IL-17 producing TH17 cells defends against fungal and extracellular bacterial infection and are integral in tissue inflammation and autoimmune diseases (Tesmer et al., 2008). While it was first believed that these cells are an inflammatory subset within the TH1 lineage, it was later determined that TH17 cells are an independent T helper cell lineage (Aarvak et al., 1999).
TH17 Cell Development
The discovery of a new T helper cell subset with pro-inflammatory properties, referred to as TH17 cells because of their expression of IL-17A, revolutionized the understanding of the adaptive immune system in the early 2000s (Park et al., 2005). TH17 cell differentiation in secondary lymphoid organs depends on an inflammatory cytokine milieu consisting of IL-23, TGF-β, IL-6, IL-1β, and IL-21 to activate the expression of the lineage-specific transcription factor RORγt, fostering TH17 cell generation. The role of RORγt was described by Ivanov et al. in 2006, who found this transcription factor to be expressed by IL-17 producing T helper cells in the lamina propria; in RORγt-deficient mice, IL-17+ cells were absent (Ivanov et al., 2006).
TH17 cells expand in the periphery and at the tissue site of inflammation and secrete a distinct group of effector molecules such as IL-17A, IL-17F, IL-21, IL-22, and IL-6 and express IL-23 receptor (IL-23R) on their surface (Bettelli et al., 2006; Mangan et al., 2006). Human TH17 cells originate from a CD161+ (the human equivalent to NK1.1) precursor in the thymus and umbilical cord blood and express CCR4 and CCR6 but not CXCR3 (Cosmi et al., 2008; Maggi et al., 2010).
Autocrine- and paracrine-derived TGF-β plays an interesting role during TH17 cell polarization. During the induction of RORγt expression, TGF-β synergizes with IL-6. TGF-β and IL-6 typically have opposing effects, but in this setting these proteins amplify the maturation of TH17 cells. In an autocrine amplification loop, TGF-β is further able to synergize with IL-21, which is predominantly produced by TH17 cells. This synergy promotes and enhances TH17 cell differentiation and pro-inflammatory immune responses (Gutcher et al., 2011). High concentrations of TGF-β in the absence of pro-inflammatory cytokines can lead to the inhibition of RORγt expression.
TGF-β can also induce the surface expression of IL-23R on differentiating TH17 cells, along with IL-6/IL-21, making the cells responsive to the inflammatory cytokine IL-23. IL-23 is a member of the IL-12 cytokine family and is mainly produced by APCs. It enhances the activation of STAT3, which together with RORγt stabilizes TH17 cell function. Therefore, IL-23 is not only important for TH17 cell generation and thereby the activation and maintenance of inflammatory responses at the tissue site of inflammation, but also promotes persistent chronic inflammation by supporting the proliferation of TH17 cells within the activated memory T cell pool (Aggarwal et al., 2003; Zhou et al., 2007; McGeachy et al., 2009).
In 2007, McGeachy et al. and Korn et al. described “classical” and “alternative” modes of TH17 cell activation that were further supported by a study from Ghoreschi et al. in 2010 (Korn et al., 2007; McGeachy et al., 2007; Ghoreschi et al., 2010). The different modes are driven by the availability of IL-23 and TGF-β. “Classical” TH17 cells, which arise from naïve CD4 T cells in the presence of TGF-β and IL-6 and the relative lack of IL-23, act through nonpathogenic expression of IL-10. Absence of expression of the TGF-β RII prevents the formation of TH17 cells that mediate the development of experimental autoimmune encephalomyelitis (EAE) (Veldhoen et al., 2006). The idea of a nonpathogenic TH17 cell subtype is further underlined by the fact that in homeostasis, TH17 cells are present in the intestine without detrimental effects. “Alternative” TH17 cells mature in the presence of IL-23 and are the pathogenic TH17 subtype. Overall, the development of TH17 cells is driven by a complex equilibrium of cytokine milieu, which influences many fine-tuning processes and a spectrum of effector functions.
The secretion of cytokines from either terminally differentiated TH1 (IFN-γ) or TH2 (IL-4) cells antagonizes the expansion of other T helper cell subtypes to sustain a lineage-specific immune response during infection. The differentiation of TH17 cells is negatively regulated by IFN-γ and IL-4 via the inhibition of IL-23 and by TReg cells via retinoic acid and IL-2. Opposingly, IL-17 and IL-23 hamper the development of TH1 cells (Harrington et al., 2005; Nakae et al., 2007). Interestingly, another IFN-γ and IL-4 independent pathway controls the development of TH17 cells. This process is driven by an additional member of the IL-12 family, IL-27. Like IL-12 and IL-23, IL-27 is secreted by APCs and acts independently of IFN-γR, IL-6R, and T-Bet but requires STAT1. Batten et al. and Stumhofer et al. found that a lack of IL-27 signaling lead to an increase in TH17 cells in autoimmune encephalomyelitis and chronic encephalitis (Batten et al., 2006; Stumhofer et al., 2006).
Unlike TH1 and TH2 cells, TH17 cells have an unstable cytokine memory and convey a surprising capacity of late-stage plasticity in their polarization status to adapt to a changing microenvironment. Because TH17 cells express low levels of IL-12R, they influence a phenotype shift after IL-12 stimulation that downregulates IL-17 and makes the cells susceptible to polarizing into a TH1-, but not a TH2-, like phenotype (Lee et al., 2009). This plasticity and the synergy between TH1 and TH17 cells is important for host defense mechanisms, as shown in a mouse model of Mycobacterium tuberculosis infection in which an early TH17 immune response recruited TH1 cells to the site of inflammation and promoted the development of T cell memory (Khader et al., 2007). It can also be a driving mechanism in autoimmunity and cancer.
TH17 Cells in Host Defense and Autoimmunity
Until 1996, it was assumed that autoimmune diseases are the consequence of a dysregulation of TH1 responses. A study by Ferber et al. showed that loss of IFN-γ did not prevent the development of EAE but rather worsened disease progression (Ferber et al., 1996). Based on those findings, Oppmann and colleagues described IL-23 as new cytokine secreted by dendritic cells (DCs) that can induce the production of IFN-γ and IL-17 (Oppmann et al., 2000). Antibody-mediated blockade of IL-23 and generation of IL-23 deficient mice highlighted its involvement in the development of Crohn’s disease, psoriasis, EAE, and collagen-induced arthritis and the experimental animals either showed delayed and reduced disease severity or never developed autoimmune disease (Cua et al., 2003; Murphy et al., 2003; Mannon et al., 2004; Krueger et al., 2007). Clinical trials using monoclonal antibodies interfering with IL-12 and IL-23, such as ustekinumab, showed promising results in the improvement of psoriasis and psoriatic arthritis symptoms. In chronic ulcerative colitis patients, blockade of the interaction of IL-12 and IL-23 and their specific receptors on TH1 and TH17 cells also showed beneficial effects (Sands et al., 2019). Other antibodies, such as secukinumab, specifically targeting IL-17A were also highly effective in psoriasis patients (Sanford and McKeage, 2015).
Additionally, blockade of IL-17 or the loss of the regulatory mediators RORγt and IL-6 resulted in comparable outcomes via a lack of infiltration of TH17 cells into the tissue sites of inflammation, pointing to a crucial role for these cells in the development and progression of autoimmune diseases. Finally, experiments performing an adoptive transfer of TH17 cells clearly showed that these cells, but not TH1 cells, modulate autoimmune conditions in mice. This finding was supported in human patients with multiple sclerosis, rheumatoid arthritis, and psoriasis, in whom increased levels of IL-17 and IL-23 were observed (Ziolkowska et al., 2000; Cho et al., 2004; Vaknin-Dembinsky et al., 2006). Although an increasing body of evidence points to a dominant role of TH17 cells as inducers of autoimmunity, it is important to note that TH1 cells are also crucial in the development of autoimmunity. Each target tissue of inflammation actively participates in the formation of a site-specific cytokine milieu through its cellular composition.
The gut is an especially interesting organ to investigate the plasticity of TH17 cells. In homeostasis, the intestine is the primary site of TH17 cell differentiation, and the gut microbiota heavily influences its regulation. The TCR of intestinal TH17 cells has a distinct specificity for antigens coming from segmented filamentous bacteria, suggesting that these bacteria are critical for the induction of gut-resident TH17 maturation (Huber et al., 2012). The function of these cells under non-pathogenic conditions is to protect against microbial invasion and maintain intestinal barrier function as well as to maintain other barrier sites of the body such as the lung epithelium or the skin. Non-pathogenic characteristics of these cells can specifically be found in the small intestine, where they limit inflammation in response to bacterial or parasitic infections via the secretion of IL-10. The fragile and complexly controlled phenotype of TH17 cells can easily shift to an activated state in which cells attain pathogenicity and induce tissue inflammation that often leads to autoimmunity in the intestine and in other distant organs (Wu et al., 2010). In Peyer’s Patches, TH17 cells transition into a phenotype similar to TFH cells producing IL-21 and Bcl-6 that can induce the production of IgA antibodies by germinal center B cells (Hirota et al., 2013). Upon bacterial infection in the colon, TH17 cells can transform into cells producing IL-17 and IFN-γ simultaneously in an IL-23 dependent manner and further develop into a TH1-like cell type. Transferring this cell type can lead to a TH17/TH1 cell-induced transfer-colitis (Morrison et al., 2013; Harbour et al., 2015).
In humans, T cells expressing IL-17 and IFN-γ were found in peripheral blood and the gut lamina propria of patients with inflammatory bowel disease (Globig et al., 2014). TH17/TH1 cells are present at the organ site of inflammatory responses in different models of autoimmune diseases, such as in the colon in chronic colitis or as revealed by single-cell RNA-sequencing analysis in experimental autoimmune encephalitis (EAE), which serves as model for human multiple sclerosis (Neurath et al., 2002; Gaublomme et al., 2015). Hirota and colleagues demonstrated that these cells derive from TH17 rather than from TH1 cells (Hirota et al., 2011). This was further supported by a study from Bettelli et al. who showed that animals deficient for the transcription factor T-bet were protected against the development of EAE (Bettelli et al., 2004).
These findings suggest that T cells expressing IL-17 and IFN-γ, also known as double producers, are highly pathogenic and predominantly involved in autoimmune diseases and tissue inflammation. However, it is not fully understood whether the production of IFN-γ by TH17 cells serves to limit TH17 cell-induced inflammation or rather promotes inflammation.
Regulatory T Cells (TReg) in Health and Disease
TReg Development
Regulatory T cells (TReg) balance host defense against foreign pathogens, foster immune tolerance, and orchestrate immune homeostasis. The two main TReg subsets are natural TReg cells (nTReg), which provide central tolerance against self-antigens, and peripheral TReg cells (pTReg), which develop extrathymically from conventional T cells and recognize non-self-antigens (Sakaguchi, 2004). T cells maintain peripheral tolerance by regulating inflammatory responses against the microbiota, commensals, and pathogens (Hadis et al., 2011; Lathrop et al., 2011) (Figure 2).
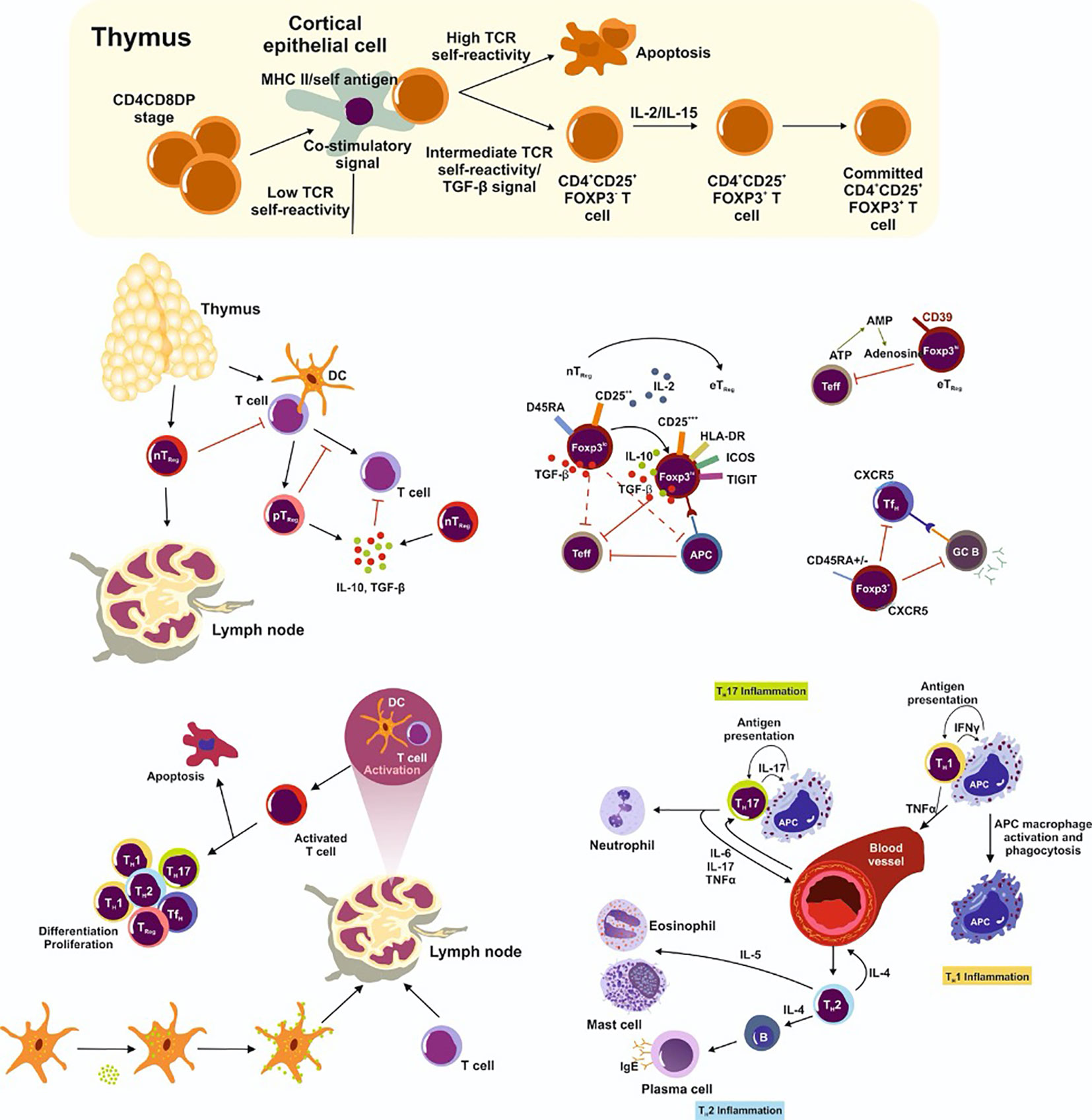
Figure 2 Natural TReg cells (nTReg) maturate in the thymus. Their development requires the interplay of TCR-dependent recognition of self-antigen, a specific cytokine milieu (including TGF-β, IL-2, IL-7, IL-15) and the presence of co-stimulatory factors. They then infiltrate into the periphery. In the lymph node, T cells encounter a specific antigen from antigen presenting cells and become activated. Depending on the strength of the TCR binding and the presence or absence of co-stimulatory factors, cells either differentiate and proliferate into effector cells or undergo apoptosis. Peripheral TReg (pTReg) cells develop predominately in the periphery from naïve CD4+ T cells. However, nTReg and pTReg cells share the lineage markers CD25 and FoxP3 which are highly expressed in effector TReg cells (eTReg). eTReg cells suppress antigen presenting cells (APC) and effector T cells (Teff) and are thereby very efficient in inhibiting the pro-inflammatory immune response. Furthermore, TReg cells express CD39 on the cell surface to convert extracellular ATP (eATP) to adenosine and prevent a pro-inflammatory immune response. Follicular helper T (TFH) cells suppress the germinal center B cell reaction. Importantly, TRegs also inhibit Teff in the circulation. TH17 cells secret pro-inflammatory cytokines and attract neutrophils to the inflammation site, while TH1 cells promote macrophage development and function and TH2 cells lead to plasma, eosinophil, and mast cell differentiation and generate a pro-inflammatory immune response.
Natural TReg Development
nTRegs develop during the neonatal period during thymocyte maturation (Liston et al., 2008). Stable nTReg development requires a complex network of antigen presenting cells (APCs) and 1) TCR-dependent recognition of self-antigens, 2) cytokine stimulation (IL-2, IL-15, and IL-7), and 3) other costimulatory signals (Bayer et al., 2005). The TCR repertoire of nTReg cells varies from the effector T cell repertoire with only minor overlap (Wong et al., 2007). The recognition affinity of self-antigens by nTReg cells is crucial for nTReg development and must fall between positive and negative selection (Maloy and Powrie, 2001). In addition to affinity to the MHC self-antigen complex, the expression level on the APC is important for optimal nTReg maturation. Cytokine stimulation by IL-2, IL-15, and IL-7 promote nTReg development, function, and homeostasis (Vang et al., 2008). Costimulatory signals through CD28 stimulation are also essential to maintain nTReg survival and to avoid defective nTReg development (Tai et al., 2005). Thus, costimulatory signals function as expansion, rather than as selective, signals.
During thymic differentiation of nTReg cells, immature single-positive CD4+ T cells express the IL-2 receptor α chain (CD25) (Setoguchi et al., 2005). CD25 has high IL-2 affinity, is continuously expressed on TReg cells, and benefits TReg responsiveness in comparison to effector cells when IL-2 concentrations are low. CD25 deficiency is related to defective TReg development, function, and an imbalanced immune system (Goudy et al., 2013). After differentiation, nTReg cells stabilize their lineage specific transcription factor forkhead box protein 3 (FOXP3) and gain suppressive functions (Fontenot et al., 2017). They further exhibit a specific CpG hypomethylation at three conserved non-coding DNA sequences (CNS) at the FOXP3 promotor, influencing the overall transcriptional activity of the cell (Toker et al., 2013). These epigenetic modifications are obligatory for TReg lineage stability, because they influence the activity of central signaling pathways like NF-κB, NFAT, STAT5, mTOR, and the binding of transcription factors to the FOXP3 promotor (Zheng et al., 2010). Further, TReg-specific demethylation regions (TSDRs) contribute to a specific demethylation signature abundant in TReg function-defining genes (e.g., CTLA-4, IL-2RA, IKzf4) which regulate the overall transcriptional activity, development, and function of TReg cells.
Although FOXP3 is interrelated with TReg function, its expression is not exclusive for TReg cells as it can be transiently upregulated in activated T cells and likewise several TReg specific genes are FOXP3 independent. However, FOXP3 is necessary but not sufficient to induce TReg cells (Hill et al., 2007).
Peripheral TReg Development
In contrast to nTReg cells, pTReg cells develop in the peripheral tissue from naïve CD4+ T cells across the lifespan of an individual. pTReg cells also have a slightly different TCR repertoire and prevent an overwhelming immune response from occurring in response to microbiota, commensals, and pathogens (Hadis et al., 2011; Lathrop et al., 2011). pTReg and nTReg cells share the expression of the lineage defining molecules CD25 and FOXP3. Although, the frequency of pTReg cells is low, their percentage can be enriched in different tissues under inflammatory conditions (Curotto de Lafaille et al., 2008). Like nTReg cells, the promotion of pTReg differentiation is driven by TCR signaling, TGF-β, and IL-2 and costimulatory signals (Chen et al., 2003). The importance of TGF-β signaling was demonstrated in mice, when TGF-β deficiency prevented pTReg differentiation and FOXP3 stabilization (Marie et al., 2005) suggesting that specific demethylation at the FOXP3 promotor is important for pTReg differentiation, function, and lineage stability (Takimoto et al., 2010).
pTReg development can differ by tissue. The process is highly induced in the intestine because of the special immune demands at this site. Primarily, mucosal DCs support pTReg development by producing TGF-β and retinoic acid (Coombes et al., 2007). The latter supports FOXP3 stabilization by CNS1 (Mucida et al., 2009). In addition, pTReg differentiation is enhanced by metabolites produced by the microbiota in the intestine, along with chromatin structure and FOXP3 stabilization facilitated by short chain fatty acids (Arpaia et al., 2013). Fascinatingly, a high percentage of the pTReg population in the intestine co-expresses RORγt and FOXP3 while preserving the overall epigenetic and genetic signature and function of TReg cells (Yang et al., 2016).
The peripheral FOXP3+ cell population is very heterogenous and can be divided into different subpopulations according to FOXP3, CD25, and CD45RA expression profiles reflecting their activation, cytokine expression, and manifestation of epigenetic changes. CD45RA+FOXP3lowCD25low are defined as resting or naïve TReg cells. CD45RA-FOXP3highCD25high are described as effector TReg cells (eTReg), and CD45RA-FOXP3lowCD25low cells are non TReg cells and represent activated conventional T cells. The CD45RA+FOXP3lowCD25low subpopulation is further characterized by the expression of naïve T cell markers, the majority of which express CD31, a thymic emigrant marker, and the TSDR is widely conserved. The CD45RA-FOXP3highCD25high subpopulation, in contrast, exhibits a highly suppressive and proliferative capacity and is profoundly demethylated (Miyara et al., 2009).
The Role of TReg Cells in Immune Homeostasis and Inflammation
TReg cells balance the immune response in homeostasis and inflammation. These cells orchestrate the immune response of effector T cells (Teff) and initiate anti-inflammatory mechanisms.
Basic Mechanism of TReg Function
TReg cells influence the immune response by producing anti-inflammatory cytokines such as IL-10 and TGF-β. IL-10 has a potent immunosuppressive function by inhibiting the production of pro-inflammatory chemokines and cytokines and establishing immune balance in response to a pathogen, autoimmune disease, and allergy (O’Garra et al., 2004). IL-10 directly inhibits co-stimulation via CD28 and ICOS and indirectly by the downregulation of co-stimulatory molecules on APCs (Taylor et al., 2007). In addition, IL-10 produced by TReg cells orchestrates antibody production in allergies from IgE toward IgG4. IgG4 and IL-10 production is upregulated in a course of allergen-specific immunotherapies and inhibits IgE-mediated anaphylaxis (Epp et al., 2018).
Interestingly, TRegs producing TGF-β may not be required for complete TReg function and influence overall TReg differentiation (Piccirillo, 2008). Numerous studies revealed the importance of TGF-β mediated TReg function and the upregulation of TGF-β to amend their suppressive function. TGF-β represses the cytolytic function of effector CD8+ cells by downregulating cytolytic genes (e.g., Fas ligand, perforin, granzyme A, B and IFN-γ) in autoimmune diseases and cancer (Thomas and Massague, 2005). In addition, TGF-β produced by TReg cells inhibits natural killer cell function and contributes to the overall anti-inflammatory effects of TGF-β (Cortez et al., 2017). Furthermore, TReg-mediated TGF-β can suppress naïve T cell activation and differentiation and can function as a self-regulating stimulus to maintain TReg development (Tran, 2012). Although the role of TGF-β in direct suppressive TReg function remains controversial, this cytokine seems important but not obligatory.
Another anti-inflammatory cytokine that complements the inhibitory repertoire of TReg cells is IL-35. IL-35 plays a suppressive role in autoimmune diseases, allergies, and cancer models. In addition to TReg cells, IL-35 is secreted by BReg cells and CD8+ cells. Thus, this cytokine prevents effector T cell expansion, cytokine production, and TH17 differentiation (Collison et al., 2007; Niedbala et al., 2007). IL-35 also supports TReg and BReg expansion and activation by influencing the immune response in autoimmunity (Dambuza et al., 2017). In sum, cytokines fundamentally contribute to suppressive TReg function.
TReg cells express various inhibitory receptors on the cell surface. One of the most important and well-studied inhibitory receptors is the cytotoxic T lymphocyte antigen-4 (CTLA-4), which is functionally and structurally related to CD28 and can bind B7 with a 50–100-fold higher affinity. CTLA-4 is upregulated in activated and exhausted T cells but continuously expressed on TReg cells and supports their inhibitory function. By binding to B7, CTLA-4 inhibits T cell activation, proliferation, and cytokine production including IL-2 (Krummel and Allison, 1996). CTLA-4 further leads to the removal of costimulatory receptors on APCs (Sansom, 2015). A defect in CTLA-4 function, for example by non-sense mutation in the gene encoding CTLA-4, leads to defective TReg function and is accompanied by complex autoimmune disorder and immunodeficiency in humans. Interestingly, patients had a higher TReg abundancy but decreased CTLA-4 expression on the TReg cell surface. Patients with the inherited heterozygous loss of function mutation develop systemic autoimmune disorders like type 1 diabetes, autoimmune thyroid disease, systemic lupus erythematosus, and inflammatory bowel disease (Kuehn et al., 2014; Schubert et al., 2014).
TReg cells also express the inhibitory receptors programmed cell death protein 1 (PD-1) and lymphocyte-activation gene function 3 (LAG-3). PD-1 and FOXP3 work collaboratively to maintain immune tolerance, with PD-1 important to maintaining the activation balance between effector T cells and TReg cells (Zhang B. et al., 2016). In a course of anti-PD-1 therapy in cancer, PD-1+ TReg cells were amplified and mediated cancer growth (Kamada et al., 2019). LAG-3 contributes to immunosuppressive TReg function in a tumor environment and promotes maternal tolerance during pregnancy (Camisaschi et al., 2010; Zhang and Sun, 2020). Hence, LAG-3 inhibits DC maturation and function. Thus, inhibitory receptors play a fundamental role in TReg function but remain poorly understood.
In addition to cytokines and inhibitory receptors, TReg cells use metabolic disruption to influence the immune response. IL-2 is one of the most important cytokines for T cell expansion and is mandatory for TReg function and differentiation (Davidson et al., 2007). TReg cells express the high-affinity IL-2 receptor CD25 on their surface and could have a metabolic advantage in comparison to effector T cells, especially in a milieu where the IL-2 concentration is low. Accordingly, cytokine deprivation by TReg cells induced apoptosis in effector T cells (Pandiyan et al., 2007). In contrast, IL-2 consumption was not required for TReg suppression (Oberle et al., 2007). Nevertheless, modern low-dose IL-2 therapies in various diseases could demonstrate a preferential TReg expansion and thereby have a positive effect on patient outcome (Hartemann et al., 2013; Matsuoka et al., 2013; He et al., 2016).
TReg cells use the membrane-bound ectonucleotidases CD73 and CD39 to generate adenosine from extracellular ATP to influence the immune response. Extracellular ATP usually promotes inflammation, whereas adenosine leads to anti-inflammatory effects. CD39 is abundant on TReg cells, whereas CD73 is intracellularly enriched in human TReg cells and upregulated after TReg activation (Schuler et al., 2014). TReg cells are sensitive to extracellular ATP, and the upregulation of CD39 is accompanied by remission of inflammatory bowel disease (Gibson et al., 2015). Thus, CD39 signaling is primarily a mechanism to suppress TH17 function and development. Adenosine leads to CTLA-4 and PD-1 upregulation in TReg cells and promotes TReg suppression of DC function (Ring et al., 2015).
The first indication that cytolytic mechanisms play a role in TReg function came from studies of granzyme B. In particular, granzyme B is upregulated in activated TReg cells and mediates suppression of B cell function, and granzyme B deficiency reduces TReg suppression. Granzyme B-expressing TReg cells are enriched in human colorectal cancer and potent suppressors of effector T cells. Further, TReg cells protect themselves from granzyme B-mediated killing by upregulating serine protease inhibitor 6 (Azzi et al., 2013; Sun et al., 2020).
TReg Function in Autoimmunity
Defects in molecules important for TReg function can lead to autoimmune diseases, underlying the importance of TReg effector proteins in pathophysiology. For example, the inherited IPEX syndrome (X-linked autoimmune syndrome immunodysregulation polyendocrinopathy enteropathy X-linked) is caused by different loss of function mutations in the FOXP3 gene. These defects can further lead to the development of autoimmune diseases like diabetes type 1, autoimmune colitis, or hepatitis (Le Bras and Geha, 2006). IL-2RA mutations cause a phenotype similar to IPEX syndrome and CD25 deficiency can increase the vulnerability to viral infection (Goudy et al., 2013). In addition to these monogenetic TReg diseases, TReg dysfunction appears in other immune deficient syndromes. Autoimmune diseases like type 1 diabetes, multiple sclerosis, systemic lupus erythematosus, myasthenia gravis, and rheumatoid arthritis are associated with altered TReg quantity or quality (Wakabayashi et al., 2006; Lapierre et al., 2013).
The direction of TReg alteration is controversial. Different studies show either an increase or decrease in TReg cell numbers based on the stage of the disease and the heterogeneous use of TReg-defining molecules. In addition, as explained above, TReg defining molecules can be upregulated after general T cell activation in humans (Pillai et al., 2007). Nevertheless, TReg cells are an important target for therapy of autoimmune diseases and remission can partly reverse the defective TReg function (Hartemann et al., 2013; Humrich et al., 2015; Rosenzwajg et al., 2015; He et al., 2016). Treatment with tocilizumab in patients with rheumatoid arthritis, for example, increased TReg frequency and restored TReg function (Kikuchi et al., 2015). Another therapeutic approach is the adoptive transfer of in vitro-expanded TReg cells. This strategy was beneficial in mouse models and is now being tested in humans (Morgan et al., 2005; Mathew et al., 2018).
Tumor tissue is especially challenging for the immune system. TReg cells impair the immune response against tumor antigens by effector T cells that evolve from potential self-reactive cells. In general, a TReg-enriched tumor tissue with decreased abundancy of CD8+ cells is associated with poor prognosis, metastasis, and reduced survival (Mougiakakos et al., 2010). Tumor-infiltrating TReg cells are primarily effector TReg cells (CD45RA-FOXP3highCD25high), which are active and proliferative. Furthermore, these cells differ in the expression of activation markers, TReg markers, and inhibitory receptors on their cell surface. Tumor-infiltrating TReg cells also express several chemokine receptors such as CCR4 and CCR8 (De Simone et al., 2016). In the tumor, TReg cells interact with immune cells, cancer cells, and fibroblasts to influence tumor immunity. Tumor-associated fibroblasts promote tumor progression and positively regulate TReg function and frequency (Kato et al., 2018). Furthermore, TReg cells and cancer cells bidirectionally support their growth and function. Immunosuppressive TReg function enables tumor progression, while cancer cells secrete TGF-β, IDO, and COX-2 to promote TReg trafficking and differentiation (Costa et al., 2018). Adenosine is produced by cancer cells and TReg cells and functions as a mediator, encouraging cancer cell growth, angiogenesis, and metastasis (Chimote et al., 2018). In addition to cancer cell effects, TReg cells have a tremendous impact on tumor-infiltrating immune cells. They reciprocally promote nonclassical monocytes, BReg differentiation, and MDSCs trafficking to create an immunosuppressive cell composition. Furthermore, TReg cells inhibit proinflammatory immune cells such as NK cells and cytotoxic lymphocytes to prevent an effective anti-tumor immune response (Chang et al., 2016; Sarhan et al., 2018). Modern immunotherapies use anti-CTLA-4 and anti-PD-1, which mainly target the TReg immune response (Ha et al., 2019).
An important cell subpopulation that balances the immune response in the course of pathogen infection and vaccination are follicular regulatory T cells (TFR). TFR cells affect the humoral immune response by influencing B cell maturation in the germinal center (GC). During this process, B cells undergo somatic hypermutation to establish a high affinity and effective humoral immune response. This development is supported by TFH cells and is tightly regulated by TFR to prevent autoimmunity and an imbalanced immune response (Wollenberg et al., 2011). TFR influence TFH frequency and function and in addition B cells directly by inhibiting their activation. Interestingly, TFR express a distinct TCR repertoire for foreign antigens and potential self-antigens. TFR resemble TFH cells in CXCR5, ICOS, and BCL-6 expression but TFR also express CD28, FOXP3, and Blimp1 (Chung et al., 2011). TFR cells differentiate from CD25+FOXP3+ cells and function as an effector TReg subpopulation (Linterman et al., 2011). CTLA-4 is the most important mode of TFR-mediated immune cell regulation, but PD-1 also contributes to full TFR function (Sage et al., 2014). TFR and TFH influence one another reciprocally, and the interaction can be influenced by the microenvironment. In a recently published study, different adjuvants balanced TFR frequency and function during vaccination (Bartsch et al., 2020). The TFR function was affected by IL-6 signaling and the altered TFR cell frequency influenced antibody glycosylation and the overall humoral immune response (Bartsch et al., 2020).
In addition to the GC reaction, TFR can be detected in the blood even at low frequency. Circulating TFR cells have a memory-like phenotype and fine tune the secondary response to an antigen by influencing reactivation of DCs to GC and cytokine production, antibody class-switching, and B cell activation (Sage et al., 2014). TFR dysfunction can be associated with autoimmune diseases, graft versus host reactions, and allergies (He et al., 2013). Overall, TReg cells play an important role in orchestrating the immune response in health and disease.
The TH17/TReg Cell Balance in the Liver
The liver is an immunogenetic organ exposed to a variety of antigens and pathogens from the digestive tract and is essential to building an effective immune response. Interestingly, and in contrast to the blood, the CD4/CD8 ratio is reversed in the liver (Doherty and O’Farrelly, 2000). The liver is naturally enriched with innate immune cells, namely, macrophages (Kupffer cells), natural killer (NK) cells, and NK T cells. Especially during fibrogenesis, infiltrating monocytes are important for continuous inflammation and the activation of extracellular matrix producing hepatic stellate cells (HSCs). The adaptive immune system also plays a critical role in these processes. The intrahepatic TReg frequency can differ from 1% to 5% among all intrahepatic lymphocytes (Oo et al., 2012). The expression of IL-17RA was observed on parenchymal and non-parenchymal cells including hepatocytes, HSCs, Kupffer cells, and endothelial cells, all of which exacerbate inflammatory reactions upon injury. In two different mouse models of hepatic fibrosis (bile duct ligation and carbon tetrachloride), the deletion of IL-17A, IL-23, and IL-17RA inhibited HSC activation and fibrosis development. This finding implies a direct functional link between IL-17A mediated stimulation of HSCs by activation of STAT3-dependent signaling (Meng et al., 2012). Further, isolation experiments in primary liver-resident cells revealed IL-17 production and IL-17 signal transmission by almost all liver-resident cell types. Kupffer cells especially express high levels of IL-17 and show significant upregulation of IL-17 and IL-1β upon stimulation. mRNA expression of IL-17RA and IL-17RC could additionally be found in hepatocytes, Kupffer cells, HSCs, and liver endothelial cells. Despite IL-17RA expression, hepatocytes and liver endothelial cells do not express IL-17 themselves (Zenewicz et al., 2007). Another study using a cell transplantation model pointed to a direct interaction between HSCs and TReg cells. Jiang et al. found that upon transplantation, HSCs in allogeneic recipients convey the selective expansion of CD4+CD25+FoxP3+ cells in an IL-2-dependent fashion to protect parenchymal cells from rejection (Jiang et al., 2008).
In contrast, CD4+ and CD8+ T cells, NK T cells, γδT cells, neutrophils, and macrophages do express IL-17 in the liver. The equilibrium of TH17 and TReg cells is regulated not only by differentiation but also at the epigenetic level. Interestingly, recent studies show that in the presence of IL-1β, IL-2, IL-21, and IL-23, IL-17 producing cells can also develop from TReg cells due to a differentiation switch that removes their suppressive function (Koenen et al., 2008; Deknuydt et al., 2009).
TReg and TH17 cells are also often significantly increased in chronic inflammatory liver diseases and are important to balance the persisting pro-inflammatory immune response. Interestingly, a reciprocal relationship between TH17 and TReg cells exists in their differentiation as in their effector function (Figure 3).
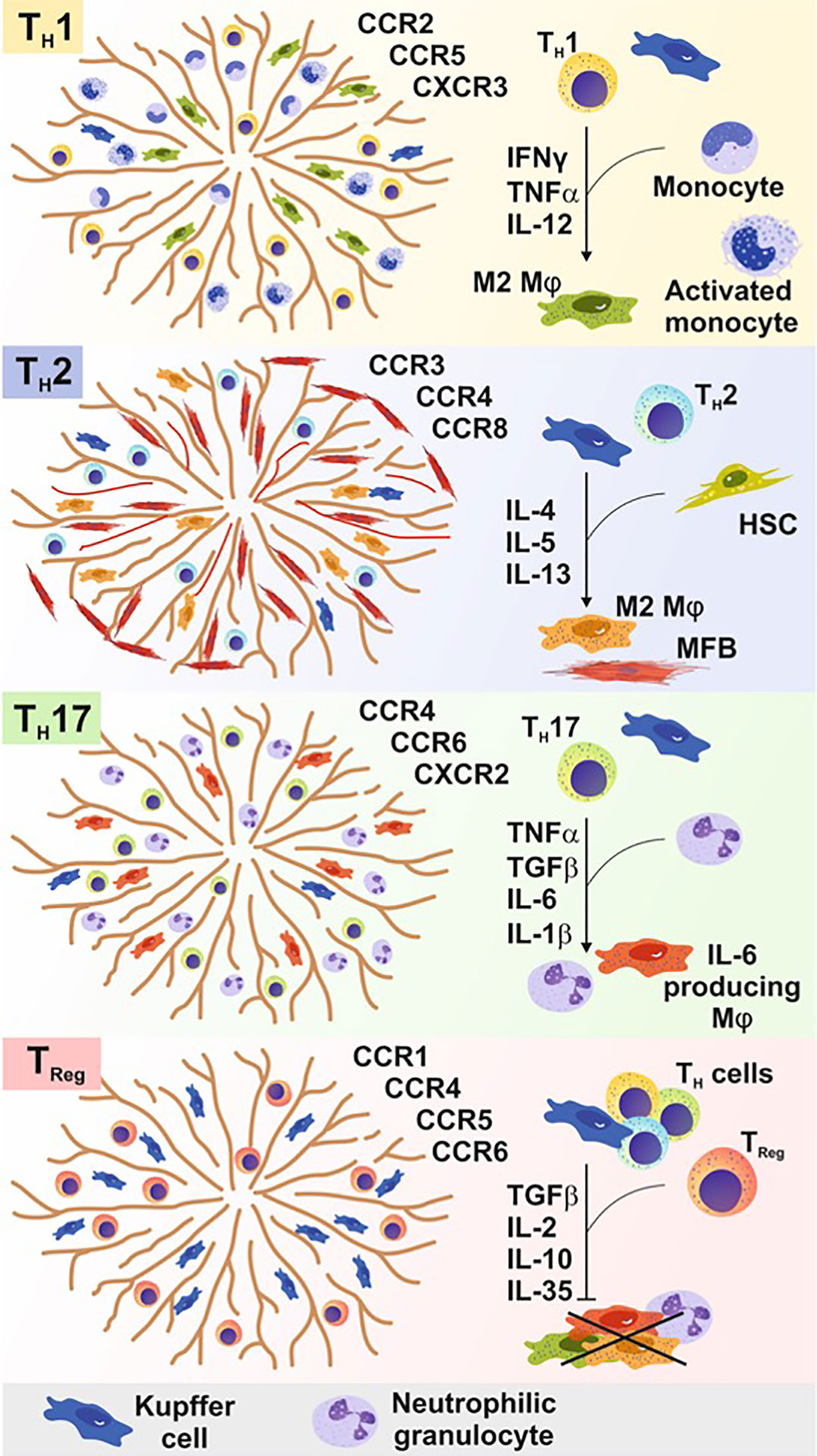
Figure 3 In acute and chronic liver diseases, T cells are crucial to either initiate, maintain, or terminate pro-inflammatory immune responses. Different T cell subpopulations develop, expand, and function based on a distinct cytokine milieu influenced by the presence of different types of immune cells. Different CD4+ cells recognize certain chemokine receptors within the liver and are thereby attracted by different cell types and stimuli to infiltrate the liver tissue. The differentiation of these subsets requires a specific cytokine milieu generated by non-parenchymal cells (e.g. monocytes) or parenchymal cells [e.g., hepatic stellate cells (HSCs)]. TH1 cell cells promote alternatively activated macrophage development (M2), whereas TH2 cells additionally promote liver myofibroblast (MFB) activation. TH17 cells leads to the recruitment of neutrophils, granulocytes, and macrophages to the site of inflammation to induce and sustain a pro-inflammatory immune response. Regulatory T (TReg) cells and follicular T (TFH) cells prevent the pro-inflammatory immune response of several parenchymal and non-parenchymal cells within the liver tissue in course of an inflammatory immune response.
TReg and TH17 cells can co-express the lineage defining transcription factors RORγt and FOXP3. Environmental conditions such as the tissue-specific cytokine milieu at the site of infection can influence this expression and influence the balance by fostering either TReg or TH17 cell development by simultaneous inhibition of the other cell type. TGF-β, for example, is required for the differentiation of both subsets; the absence or presence of proinflammatory cytokines defines whether a TH17 or TReg cell develops (Yang et al., 2008; Hammerich et al., 2011). In contrast, TH17 cells express IL-10 receptor α, which can convey a TReg-induced decrease in TH17 cells in an IL-10-dependent manner (Huber et al., 2011). Recent studies point to a delicate balance between TH17 and TReg cells crucial to maintaining tissue homeostasis. In addition to IL-6, other factors such as retinoic acid, rapamycin, or cytokines (e.g., IL-2 and IL-27) influence this balance significantly. TReg cells are assigned a decisive role in hepatic immunity. Results obtained in mouse models of acute and chronic liver disease also point to a major involvement of TH17 and TReg cells in a variety of human inflammatory liver diseases. For example, in a cancer milieu, glucose consumption by tumor cells preferentially promotes TReg differentiation and decreases TH17 cell development thereby supporting the immune escape strategy of tumor cells. Another important ubiquitous environmental condition during chronic and acute infection is hypoxia. Hypoxia can induce the hypoxia inducible factor 1α (HIF-1α) as an adaptive mechanism of the cells to low oxygen concentration. HIF-1α stabilization supports RORγt and IL-17 production while targeting FOXP3 to proteasomal degradation. Manipulation of the balance between pathogenic and regulatory processes in the liver are believed to allow the focused restoration of homeostasis especially during hepatic inflammation.
The detailed analysis of TH17 cells in human liver remains difficult because the cell frequency is low and cells can only be analyzed after their in vitro activation with phorbol 12-myristate 13-acetate (PMA) and ionomycin. This in vitro activation is interesting but must be critically considered because the extent to which it reflects the in vivo situation and cell status upon isolation is unknown.
Autoimmune Diseases
One example of specific immune change in the liver is autoimmune hepatitis (AIH). Although the cause of AIH is not fully understood, T cell mediated liver tissue destruction is involved and AIH could be associated with genetic and environmental alterations. AIH leads to chronic liver inflammation, circulating autoantibodies, and elevated liver enzymes (Tait et al., 1989). Zhao et al. showed that patients with AIH have increased serum levels of IL-17 and IL-23 together with an increased frequency of TH17 cells in the liver compared to controls. Furthermore, the frequency and function of TReg cells in the blood was decreased (Ferri et al., 2010). By analyzing the T cell composition in the liver, it was demonstrated that the total TReg number was not altered in AIH patients. In contrast, these patients displayed higher hepatic expression of the TH17-related cytokines IL-17, IL-23, IL-6, and RORγt. In vitro experiments showed that IL-17 induces IL-6 via MAPK signaling in hepatocytes, which in turn stimulates TH17 cell differentiation and infiltration in a positive feedback loop (Zhao et al., 2011). These results are supported by a retrospective study of 100 AIH patients. In addition to elevated serum levels of IL-17, IL-6, IL-21, and TNF-α, an increased frequency of TH17 cells was observed. Pro-inflammatory cytokines were positively correlated with liver injury, whereas IL-10 was negatively regulated with autoantibodies (An, 2019). Likewise, TReg cells from AIH patients had decreased CD39 expression and functionally failed to prevent TH17 accumulation mediated by extracellular ATP (Grant et al., 2014). Remission in AIH patients was associated with restored T cell balance, and the infusion of ex vivo expanded TReg cells was beneficial in a murine model (Lapierre et al., 2013). In sum, the balance of TReg and TH17 composition at the site of inflammation and TReg function is critical in AIH pathomechanisms.
Primary biliary cirrhosis (PBC) is a chronic cholestatic liver disease characterized by the loss of immune self-tolerance leading to the chronic injury of biliary epithelial cells. Ninety percent of affected patients are women older than 40 years. The importance of the TH17/TReg balance in disease progression of primary biliary cirrhosis (PBC) is evident when considering that a knockout for CD25 (IL-2Rα) in mice serves as an animal model for this disease. Mice spontaneously develop autoantibodies caused by a loss of function of TReg cells and acquire biliary duct damage similar to that observed in PBC patients (Wakabayashi et al., 2006). Deficiency of functional TReg cells leads to elevated TH17 cell numbers in the liver and elevated IL-17 levels in these mice compared to wildtype controls. A possible explanation might be the missing repressive function of IL-2 during TH17 cell differentiation. In line with the results obtained in mice, patients suffering from liver fibrosis due to PBC show a higher frequency of TH17 cells in blood than healthy control patients. Liver biopsy samples of PBC patients point to a dislocation of these cells around the portal tracts (Shi et al., 2015). Patients with cirrhosis secondary to PBC displayed an even higher infiltration of TH17 cells into liver tissue (Tan et al., 2013). However, the exact mechanisms that cause an induction of TH17 cells in livers of IL-2RA knockout animals remain elusive.
Primary sclerosing cholangitis (PSC) is another chronic-inflammatory liver disease with an unknown pathogenesis. Similar to PBC, PSC can lead to liver fibrosis and obliteration of intra-and extrahepatic bile ducts. PSC is often associated with chronic ulcerative colitis, and there is no effective treatment (Hirschfield et al., 2013). Patients with also have a decreased peripheral TReg frequency with epigenetic changes. Furthermore, a decrease in TReg numbers was associated with an IL-2RA gene polymorphism and lead to reduced TReg function (Sebode et al., 2014), (Figure 4).
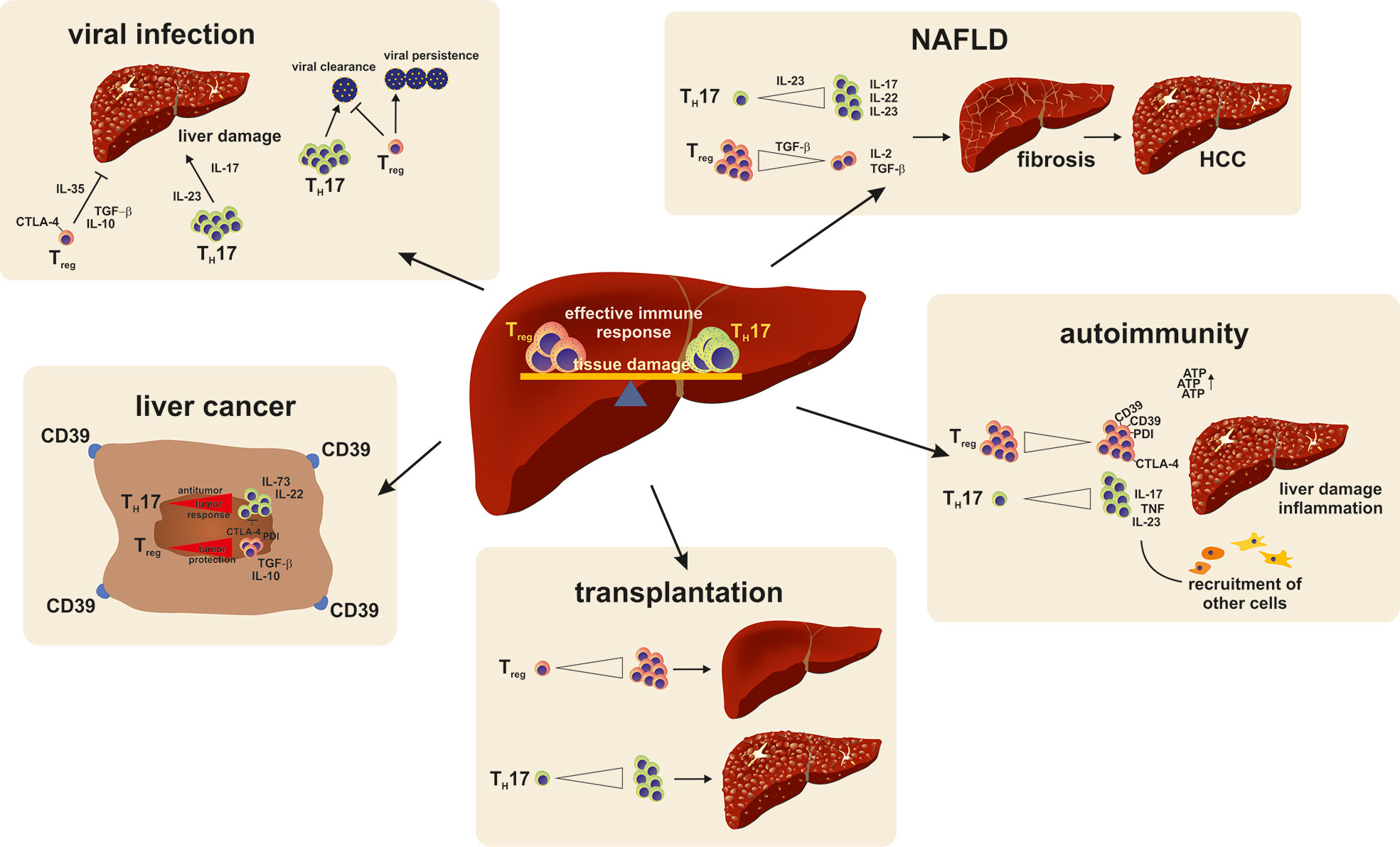
Figure 4 A reciprocal relationship exists between TH17 and TReg cells. Their balance is found to be important in the persistence or recovery from liver injury. A shift of the balance to a more dominant TH17 cell response favors pro-inflammatory reactions and persisting damage. In viral infections, TH17 are especially important for viral clearance but at the same time cause liver damage. TReg cell on the other hand prevent liver damage but also trigger viral persistence by strengthening the anti-inflammatory immune response. An imbalance of the TH17/TReg milieu to a dominant TH17 cell response favors disease development and progression in NAFLD. In autoimmune diseases a TReg cell responses have beneficial effects in regards to self-tolerance, restore immune homeostasis and are even proposed as a treatment option in acute and chronic transplant rejection reactions. In a tumor environment TReg cells support tumor cells from being targeted by the immune system and hence promote tumor growth and metastasis.
Acute Liver Injury
Mouse models of acute liver injury were used to further investigate the role of TH17 cells in the liver. In the concanavalin A- (ConA-) model of acute T cell induced hepatitis, IL-1- deficient animals were challenged and knockout mice developed less severe injury with higher TReg numbers compared to wildtype mice (Nagata et al., 2008). However, these results are controversial; another study found in the same model that IL-17-deficient mice seemed to develop a comparable level of liver injury after ConA-treatment (Zenewicz et al., 2007). In another interesting mouse model of drug-induced liver injury (halothane injection intraperitoneally), mice had increased serum levels of IL-17. After the administration of an IL-17 neutralizing antibody serum, liver enzymes AST and ALT had significantly decreased levels, with a downregulation of inflammatory cytokines such as TNF-α. These beneficial effects could be directly reversed by the application of recombinant IL-17 (Kobayashi et al., 2009).
Viral Infection
The TReg/TH17 balance is essential for an effective immune response and at the same time preventing excessive liver injury during viral infection. Thus, the liver is affected by several viruses, and some of them lead to persistent infection and can cause liver cirrhosis, organ failure, and cancer. During acute hepatitis A virus (HAV) infection, serum IL-17 levels are correlated with liver injury, and liver resident and circulating TReg frequencies are negatively linked to elevation of ALT and AST (Choi et al., 2015).
The major problem in persistent viral infection is a failure of the T cell response involving T cell exhaustion due to persistent antigen presentation. TReg cells also play a role in the immunopathology of persistent viral infection. It was demonstrated that liver sinusoidal endothelial cells (LSEC) are potent enough to promote TReg differentiation by the continuous induction of FOXP3 in conventional T cells, although all liver cells were able to induce TReg differentiation. TReg stabilization did not require inflammation but did require TGF-β, which is abundant on the LSEC cell membrane. Experimentally, LSEC-induced TReg cells expressed FOXP3 and had efficient inhibitory functions on effector T cells in vitro and in vivo (Carambia et al., 2014). Antigen presentation on LSECs and thereby an early TReg development in a course of sub-infectious viral infection of hepatotropic viruses, e.g., chronic hepatitis C virus (HCV), can support ineffective virus clearance and chronic infection (Park et al., 2013). In contrast, virus-specific TH17 cells were correlated with liver injury and inflammation, but TH17 quantity could not be linked to effective viral clearance. Furthermore, IL-23 and IL-17 levels in HCV-infected patients were elevated, and IL-23 therapy was reported to modulate the antiviral response by preferentially promoting TH17 immune cells (Meng et al., 2016). In a course of HCV infection, TReg cells were mainly found in necro-inflammatory liver areas to inhibit the effector CD8 T cell response, which is the main cause of liver damage in HCV and hepatitis B virus (HBV) infection. Isolated TReg cells from HCV-infected patients suppressed virus-specific CD8+ cells, whereas the depletion of TReg cells increased their proliferation. Furthermore, TCR analysis demonstrated that the effective TReg population during chronic HCV infection is heterogeneous and consists of nTReg and pTReg cells (Losikoff et al., 2012). In comparing the TReg cells of patients who spontaneously resolved the infection and patients with persistent infection, core virus-specific TReg cells were primarily found in patients with chronic HCV infection. They inhibit the virus-specific T cell response by producing IL-10 and IL-35 (Langhans et al., 2010). Furthermore, several studies investigated a positive correlation between TReg quantity and function with chronic HCV progression (Cabrera et al., 2004; Ebinuma et al., 2008). TH17 cells on the other hand are enriched in livers of patients with chronic HCV infection mainly driven by Tim-3, which leads to a differential regulation of IL-12 and IL-23 (Wang et al., 2013).
The TReg/TH17 balance also plays an important role in chronic HBV infection. TReg cells inhibit the antiviral response of effector T cells (Yan et al., 2014). The frequency of circulating TReg cells is differently described in chronic HBV infection, but there are some indications that the TReg frequency is increased in severe chronic HBV infection. Furthermore, TReg frequencies could be positively correlated with viral load, HBeAg (Hepatitis B envelope Antigen), and HBsAg (Hepatitis B surface Antigen) (Manigold and Racanelli, 2007; TrehanPati et al., 2011). Patients with a predominant TH17 response have high plasma viral loads. Especially in HBV infection and HBV-induced cirrhosis, IL-17+ cells increase with cirrhosis stage and the HBcAg (Hepatitis B core Antigen) mediates TH17 cell responses by an IL-17R-induced activation of monocytes/macrophages. This effect leads to the production of elevated levels of pro-inflammatory cytokines such as IL-6, TNF-α, IL-12, and IL-23 (Sun et al., 2012). Further, the elevation of TReg and TFR cells in chronically HBV infected patients associated with elevated IL-10 and TGF-β levels in comparison to healthy individuals (Liu et al., 2020). TReg differentiation was thereby promoted by TGF-β production of hepatic stellate cells and activation of Notch signaling during chronic inflammation. In a course of antiviral response, TH17 cells increased in quantity accompanied with an increase in viral load (Ichikawa et al., 2011; Yan et al., 2014). In addition, in course of antiviral therapy, PD-1 expression decreased on TH17 cells and other effector T cells, indicating an improved T cell exhaustion; however, PD-1 was not present on TReg cells (Wei et al., 2013). The TH17/TReg balance is critical in the development of liver cirrhosis in chronically-infected patients. An imbalance in TH17/TReg cells was thereby an independent predictive factor for decompensated liver cirrhosis (Lan et al., 2019). In addition, Yang et al. demonstrated that IL-35 is responsible to balance the TReg and TH17 balance in acute and chronic HBV infection by preferentially increasing virus-specific TReg cells and the prevention of TH17 cell differentiation (Yang et al., 2019). In conclusion, TReg and TH17 cells contribute to the immune response during viral liver infection and the optimal balance is important for an effective antiviral immune response and prevention of complications (Figure 4).
Alcoholic and Nonalcoholic Steatohepatitis (ASH and NASH)
Patients with alcoholic steatohepatitis (ASH) show a direct correlation between the severity of inflammation and the amount of liver damage. The degradation of ethanol mediated by cytochrome P450 2E1 (CYP2E1) is associated with various inflammatory responses within the liver. The immune response leads to the production of reactive oxygen species (ROS) and TNF-α and the infiltration of immune cells. T cells infiltrating into the liver secrete high levels of pro-inflammatory cytokines, thereby attracting neutrophils to the tissue site of inflammation. Neutrophil recruitment could be closely linked to the prominence of TH17 cells. This in turn leads to increased IL-17 serum levels in ASH patients, which could be directly linked to progressive liver damage. Further, the number of TReg cells decreases in the blood of these patients (Lemmers et al., 2009).
Nonalcoholic steatohepatitis (NASH) is related to metabolic syndrome. This condition has become the most common cause of chronic liver disease and will likely be the main cause for liver transplantations within the next decade (Younossi et al., 2019). Patients with non-alcoholic fatty liver disease (NAFLD) have a high risk to progress from simple steatosis to more advanced disease stages such as NASH, cirrhosis, and hepatocellular cancer (HCC). The exact mechanisms of the pathogenesis of NASH are poorly understood. However, the role of the activation of the adaptive immune system via a TH17-mediated immune responses is becoming evident.
Targeting the balance between TH17 and TReg cells is a relatively new approach and currently topic of many research studies. The lack of available human tissue samples makes it difficult to investigate this as a potential new treatment option. Nevertheless, recent studies in mice and few data from humans indicate a decisive role of CD4+ T cells in the progression from NAFLD to NASH up to HCC development (Hammerich et al., 2011; Rau et al., 2016). A key mechanism within this process is a strong infiltration of neutrophils together with an increased IL-6 signaling and TH17 accumulation (Hubscher, 2006). This further leads to a depolarization of the intrahepatic CD4+ cell response to a more TH17 cell-driven reaction; at the same time, TReg cell activity is suppressed (Gomes et al., 2016; Rau et al., 2016). Although the total TReg number of circulating and intrahepatic TReg cells is not altered, the overall TH17/TReg balance is shifted to a more dominant pro-inflammatory immune response.
The majority of data on TH17 cells in NASH is limited to mice. Different mouse models for diet-induced nonalcoholic steatohepatitis, such as the methionine-choline deficient-diet (MCD-diet) and the widely used high fat diet (HFD) model, can lead to steatohepatitis and subsequent fibrosis. Disease development and progression in these models is accompanied with an increase in TReg cell numbers at early disease stages in which only steatosis is present, and shifts significantly to a more dominant TH17 cell-driven response at later time points when steatohepatitis and beginning fibrosis are present. In the liver fibrosis CCL4 mouse model, increased IL-17 levels led to an elevated collagen1α1 expression in HSCs triggered by STAT3 signaling (Meng et al., 2012). Gomes et al. showed in 2016 that the excess of nutrients leads to the expression of the factor unconventional prefoldin RPB5 interactor (URI) in liver of mice treated with different steatohepatitis-inducing diets. URI promoted HCC development via a shift of the CD4+ T cell composition during NASH and NASH-HCC development. The overexpression of human URI in mouse hepatocytes led to spontaneous development of steatohepatitis, which could be strengthened by feeding steatohepatitis-inducing diets (CD-HFD) or MCD. Disease was accompanied by increased IL-17 and TH17 cells in blood and liver. Mice with a heterozygous, hepatocyte-specific deficiency for URI were protected from the development of steatohepatitis together with decreased numbers of TH17 cells. Inhibiting the differentiation of TH17 cells through the blockade of RORγt lead to an improved in lipid metabolism, insulin resistance, and HCC development. The application of recombinant IL-17 in wildtype mice induced steatohepatitis, the infiltration of neutrophils to white adipose tissue, and led to an increased number of TH17 cells.
Interestingly, data from human NASH patients correlated with the expression of URI with high IL-17 levels and hepatic steatosis (Gomes et al., 2016). Another study points to overall diminished CD4+ T cell numbers in NASH, and that this reduction is an essential factor in the progression from NASH to HCC development (Ma et al., 2016). The observed changes in the T helper cell profile during NASH development can be caused either by a depolarization of CD4+ T cells to a TH17 cell phenotype, or by a relative shift of the CD4+ T cell composition in the liver due to depletion of other T helper cell subsets, or driven by an altered infiltration of distinct CD4+ T cell subsets.
A study from Rau et al. in 2016 showed that the progression from NAFLD to NASH is directly correlated with an increased frequency in TH17 cells in blood and liver of NAFLD and NASH patients together with an altered TH17/TReg balance depicted by an increased TH17/TReg cell ratio in both compartments (Rau et al., 2016). In visceral adipose tissue and subcutaneous adipose tissue of morbid obese patients, an increase in the mRNA expression of IL-17 was found compared to normal weight patients. The same patients also showed increased numbers of TH17 cells in both adipose tissues and peripheral blood mononuclear cells (PBMCs), while TReg cell numbers were decreased due to impaired survival of these cells (McLaughlin et al., 2014). A recent study published in April 2020 further points to the direct relationship between liver and adipose tissue in regulating the TH17/TReg balance. Van Herck and colleagues demonstrated that mice fed a high-fat high-fructose diet displayed an increase in TH17 cells in both compartments, with a simultaneous decrease in TReg cells. After removing the steatohepatitis-inducing diet, the disruption in the TH17/TReg balance persisted. The administration of an IL-17 neutralizing antibody subsequently decreased the pro-inflammatory immune response in the liver (Van Herck et al., 2020). NASH is further closely related to the development of HCC. The involvement of TH17 cells in tumor formation and patient survival was recently described as influencing the prevention of apoptosis in tumor cells induced by TH17 cells due to IL-17 promoting angiogenesis and an IL-23 driven tumor growth (Langowski et al., 2006; Zhang et al., 2009). Understanding the role of TH17/TReg balance and targeting it therapeutically is an interesting approach for the treatment of NASH (Figure 4).
Liver Fibrosis and Hepatocellular Carcinoma
Chronic liver disease changes and impairs organ structure and function. Liver disease leads to tissue replacement and scaring subsequently leading to liver fibroses and cirrhosis. Several studies describe the importance of TH17 and TReg cells in this process. Although the exact role of TReg cells in liver fibrogenesis is not fully understood, TReg cells support liver fibrosis by influencing metalloproteinases activation (Zhang X. et al., 2016). TH17 cells further signal on non-lymphoid cells such as endothelial cells, fibroblasts, and keratinocytes inducing the production of inflammatory cytokines such as IL-6, GM-CSF, IL-1, TGF-β, TNF-α, and MCP-1 to attract immune cells thereby promoting pro-inflammatory immune responses. IL-17 receptor signaling further activates the expression of antimicrobial peptides and matrix metalloproteinases, whereas the latter are important for the degradation of scar tissue during infection (Bettelli et al., 2008). In patients with more severe liver cirrhosis, an increased frequency of circulating TReg cells, but a decreased TReg/TH17 ratio was positively correlated with disease progression (Li et al., 2012). More particularly, the abundant expression of TGF-β and IL-6 in the liver, which both favor the differentiation of TH17 cells, points to a major contribution of these cells during this process. Liver cirrhosis is one of the major risk factors for the development of HCC. Thus, 80%–90% of HCC develop in the course of chronic inflammation (Refolo et al., 2020). HCC is associated with a poor prognosis, is hard to detect, is aggressive, and has limited therapeutic options. TReg and TH17 cells were increased in tumor tissue in comparison to the surrounding liver tissue. Not only high intra-tumoral TReg frequencies and a decreased TH17 quantity but also CD39 expressed by tumor cells and TReg cells facilitated HCC growth, metastasis, and poor prognosis by mainly affecting TH17 function and differentiation (Bettini et al., 2012). TReg cell depletion, however, negatively influenced HCC growth (Cany et al., 2011). Interestingly, high IL-17 and IL-17R expression in the tumor tissue and elevated circulating TH17 cells are also associated with poor survival and early HCC recurrence. Sorafenib is currently used in HCC treatment and targets varies kinases expressed in TReg cells. This therapy negatively affects the TReg frequency, which can be correlated to overall improved survival (Voron et al., 2014). To improve the overall survival of HCC patients, new therapeutic approaches are essential. A defect in T cell function is described and immunotherapies are potential effective treatment strategies. One possibility could be checkpoint inhibition. PD-1 upregulation for example can be detected in circulating TReg cells in HCC patients, supporting the overall immune dysregulation. Therefore, anti PD-1 is a promising strategy. This therapy reverses the TReg mediated inhibition of TH17 cells and other effector T cells (Langhans et al., 2010) (Figure 4).
Liver Transplantation
Liver transplantation is in many cases the only possibility to cure end-stage liver disease. The short-term outcome has significantly improved, but chronic organ rejection and the side effects of immunosuppressive therapy remain a concern. The optimal immunosuppressive treatment to prevent organ rejection and toxicity and at the same time avoid opportunistic infections must be tightly balanced and will vary between individuals. The state of optimal immunotherapy, called operational tolerance, is difficult to achieve, and most patients require a life-long therapy with numerous side effects. Thus, new therapeutic approaches following liver transplantation are urgently needed.
TReg cells play a leading role in averting the cause of graft-versus-host disease (GvHD) which leads to organ rejection. TReg/TH17 balance plays a fundamental role in rejection pathogenesis (Wang et al., 2019), and TH17 cells were found to be elevated during acute and chronic organ rejection. In addition, the pro-inflammatory cytokine milieu during organ rejection can induce RORγt and IL-17 expression in TReg cells. Thus, TReg cells seem to contribute to organ rejection. Indeed, an early decrease in TReg frequency is a risk factor for suspected acute and biopsy proven acute rejection (Han et al., 2020). In addition, CD39 expression in the transplanted liver tissue influences the TReg and TH17 immune response and thereby organ rejection and GvHD (Yoshida et al., 2015). Adoptive TReg transfer was protective in mice, and currently several clinical trials are testing the efficiency and safety in humans (Romano et al., 2017). Another study tested the TReg therapy in patients 6–12 months after liver transplantation and demonstrated an increase in circulating TReg cells and reduced anti-donor T cell response (Sanchez-Fueyo et al., 2020). Although the long-term effects are not evaluated, TReg therapy could be a promising therapeutic approach. In contrast to the beneficial effects, in transplant patients with HCV infection, early high levels of TReg cells and TH1 cells after liver transplantation are associated with severe recurrent HCV infection (Ghazal et al., 2019).
In addition to T cell-mediated organ rejection, danger associated molecular patterns (DAMPs) play an important role in triggering sterile inflammation in the liver after organ transplantation. Sterile inflammation could be detected in different solid organs after implantation, and sterile inflammation influences the transplant tolerance and chronic rejection. A recently published study described the correlation between elevated DAMPs and acute postoperative multi-organ dysfunction (Nagakawa et al., 2020). In sum, several studies implicate the contribution of sterile inflammation in acute and chronic organ rejection and are reviewed elsewhere (Braza et al., 2016) (Figure 4).
Conclusion
TH17 and TReg cells are essential in orchestrating the intrahepatic immune response in health and disease. Under homeostatic conditions their balance must be tightly regulated to have an effective immune response and to prevent tissue damage. In the course of several diseases, their balance is shifted. Especially in the liver, the TH17/TReg response is tremendously important. An overwhelming TH17 response, for example in NASH, can promote the inflammatory state of disease and is associated with disease progression. On the other hand, TReg cells prevent the anti-tumoral immune response in HCC and promote metastasis and cancer growth. Furthermore, both cell subsets can be beneficial in different liver diseases settings. Thus, TH17 cells are necessary for effective pathogen clearance in the liver and TReg cells are important to coordinate the immune response in autoimmunity and after liver transplantation. Most of the findings on the role of TH17 and TReg cells were generated in mice. The importance of investigating their influence in humans is highlighted in several disease settings. Many studies demonstrate the necessity of investigating the function and quantity of these cells in liver tissue specifically, as circulating cells may not reflect intrahepatic conditions. In addition to altered function and quantities in liver diseases, TH17/TReg cell balance could be a therapeutic target in disease settings.
Future studies should investigate the function of TH17 and TReg cells in liver tissue and review their balance. Furthermore, different signals that might influence TH17/TReg cells, especially in the liver, must be analyzed to fully understand the liver-specific pathomechanism and to inform a liver-specific therapy.
Author Contributions
Conceptualization: HD and LB. Writing—original draft preparation: HD, LB, and RW. Visualization: SW. funding acquisition: HD, LB, and RW. All authors contributed to the article and approved the submitted version.
Funding
The research was funded by the German Research Foundation (RW: SFB/TRR57, HD: DR 1161/1-1 LB: BA 7175/1-1).
Conflict of Interest
The authors declare that the research was conducted in the absence of any commercial or financial relationships that could be construed as a potential conflict of interest.
References
Aarvak, T., Chabaud, M., Miossec, P., Natvig, J. B. (1999). IL-17 is produced by some proinflammatory Th1/Th0 cells but not by Th2 cells. J. Immunol. 162 (3), 1246–1251.
Aggarwal, S., Ghilardi, N., Xie, M. H., de Sauvage, F. J., Gurney, A. L. (2003). Interleukin-23 promotes a distinct CD4 T cell activation state characterized by the production of interleukin-17. J. Biol. Chem. 278 (3), 1910–1914. doi: 10.1074/jbc.M207577200
An, J. (2019). Expression and significance of Th17 cells and related factors in patients with autoimmune hepatitis. Comb. Chem. High Throughput Screen 22 (4), 232–237. doi: 10.2174/1386207322666190402160455
Arpaia, N., Campbell, C., Fan, X., Dikiy, S., van der Veeken, J., deRoos, P., et al. (2013). Metabolites produced by commensal bacteria promote peripheral regulatory T-cell generation. Nature 504 (7480), 451–455. doi: 10.1038/nature12726
Azzi, J., Skartsis, N., Mounayar, M., Magee, C. N., Batal, I., Ting, C., et al. (2013). Serine protease inhibitor 6 plays a critical role in protecting murine granzyme B-producing regulatory T cells. J. Immunol. 191 (5), 2319–2327. doi: 10.4049/jimmunol.1300851
Bartsch, Y. C., Eschweiler, S., Leliavski, A., Lunding, H. B., Wagt, S., Petry, J., et al. (2020). IgG Fc sialylation is regulated during the germinal center reaction upon immunization with different adjuvants. J. Allergy Clin. Immunol. 146 (3), 652–666.e11. doi: 10.1016/j.jaci.2020.04.059
Batten, M., Li, J., Yi, S., Kljavin, N. M., Danilenko, D. M., Lucas, S., et al. (2006). Interleukin 27 limits autoimmune encephalomyelitis by suppressing the development of interleukin 17-producing T cells. Nat. Immunol. 7 (9), 929–936. doi: 10.1038/ni1375
Bayer, A. L., Yu, A., Adeegbe, D., Malek, T. R. (2005). Essential role for interleukin-2 for CD4(+)CD25(+) T regulatory cell development during the neonatal period. J. Exp. Med. 201 (5), 769–777. doi: 10.1084/jem.20041179
Bettelli, E., Sullivan, B., Szabo, S. J., Sobel, R. A., Glimcher, L. H., Kuchroo, V. K. (2004). Loss of T-bet, but not STAT1, prevents the development of experimental autoimmune encephalomyelitis. J. Exp. Med. 200 (1), 79–87. doi: 10.1084/jem.20031819
Bettelli, E., Carrier, Y., Gao, W., Korn, T., Strom, T. B., Oukka, M., et al. (2006). Reciprocal developmental pathways for the generation of pathogenic effector TH17 and regulatory T cells. Nature 441 (7090), 235–238. doi: 10.1038/nature04753
Bettelli, E., Korn, T., Oukka, M., Kuchroo, V. K. (2008). Induction and effector functions of T(H)17 cells. Nature 453 (7198), 1051–1057. doi: 10.1038/nature07036
Bettini, M., Castellaw, A. H., Lennon, G. P., Burton, A. R., Vignali, D. A. (2012). Prevention of autoimmune diabetes by ectopic pancreatic beta-cell expression of interleukin-35. Diabetes 61 (6), 1519–1526. doi: 10.2337/db11-0784
Braza, F., Brouard, S., Chadban, S., Goldstein, D. R. (2016). Role of TLRs and DAMPs in allograft inflammation and transplant outcomes. Nat. Rev. Nephrol. 12 (5), 281–290. doi: 10.1038/nrneph.2016.41
Cabrera, R., Tu, Z., Xu, Y., Firpi, R. J., Rosen, H. R., Liu, C., et al. (2004). An immunomodulatory role for CD4(+)CD25(+) regulatory T lymphocytes in hepatitis C virus infection. Hepatology 40 (5), 1062–1071. doi: 10.1002/hep.20454
Camisaschi, C., Casati, C., Rini, F., Perego, M., De Filippo, A., Triebel, F., et al. (2010). LAG-3 expression defines a subset of CD4(+)CD25(high)Foxp3(+) regulatory T cells that are expanded at tumor sites. J. Immunol. 184 (11), 6545–6551. doi: 10.4049/jimmunol.0903879
Cany, J., Tran, L., Gauttier, V., Judor, J. P., Vassaux, G., Ferry, N., et al. (2011). Immunotherapy of hepatocellular carcinoma: is there a place for regulatory T-lymphocyte depletion? Immunotherapy 3 (4 Suppl), 32–34. doi: 10.2217/imt.11.29
Carambia, A., Freund, B., Schwinge, D., Heine, M., Laschtowitz, A., Huber, S., et al. (2014). TGF-beta-dependent induction of CD4(+)CD25(+)Foxp3(+) Tregs by liver sinusoidal endothelial cells. J. Hepatol. 61 (3), 594–599. doi: 10.1016/j.jhep.2014.04.027
Chang, A. L., Miska, J., Wainwright, D. A., Dey, M., Rivetta, C. V., Yu, D., et al. (2016). CCL2 Produced by the glioma microenvironment is essential for the recruitment of regulatory T cells and myeloid-derived suppressor cells. Cancer Res. 76 (19), 5671–5682. doi: 10.1158/0008-5472.CAN-16-0144
Chen, W., Jin, W., Hardegen, N., Lei, K. J., Li, L., Marinos, N., et al. (2003). Conversion of peripheral CD4+CD25- naive T cells to CD4+CD25+ regulatory T cells by TGF-beta induction of transcription factor Foxp3. J. Exp. Med. 198 (12), 1875–1886. doi: 10.1084/jem.20030152
Chimote, A. A., Balajthy, A., Arnold, M. J., Newton, H. S., Hajdu, P., Qualtieri, J., et al. (2018). A defect in KCa3.1 channel activity limits the ability of CD8(+) T cells from cancer patients to infiltrate an adenosine-rich microenvironment. Sci. Signal 11 (527), eaaq1616. doi: 10.1126/scisignal.aaq1616
Cho, M. L., Yoon, C. H., Hwang, S. Y., Park, M. K., Min, S. Y., Lee, S. H., et al. (2004). Effector function of type II collagen-stimulated T cells from rheumatoid arthritis patients: cross-talk between T cells and synovial fibroblasts. Arthritis Rheum. 50 (3), 776–784. doi: 10.1002/art.20106
Choi, Y. S., Lee, J., Lee, H. W., Chang, D. Y., Sung, P. S., Jung, M. K., et al. (2015). Liver injury in acute hepatitis A is associated with decreased frequency of regulatory T cells caused by Fas-mediated apoptosis. Gut 64 (8), 1303–1313. doi: 10.1136/gutjnl-2013-306213
Chung, Y., Tanaka, S., Chu, F., Nurieva, R., II, Martinez, G. J., Rawal, S., et al. (2011). Follicular regulatory T cells expressing Foxp3 and Bcl-6 suppress germinal center reactions. Nat. Med. 17 (8), 983–988. doi: 10.1038/nm.2426
Collison, L. W., Workman, C. J., Kuo, T. T., Boyd, K., Wang, Y., Vignali, K. M., et al. (2007). The inhibitory cytokine IL-35 contributes to regulatory T-cell function. Nature 450 (7169), 566–569. doi: 10.1038/nature06306
Coombes, J. L., Siddiqui, K. R., Arancibia-Carcamo, C. V., Hall, J., Sun, C. M., Belkaid, Y., et al. (2007). A functionally specialized population of mucosal CD103+ DCs induces Foxp3+ regulatory T cells via a TGF-beta and retinoic acid-dependent mechanism. J. Exp. Med. 204 (8), 1757–1764. doi: 10.1084/jem.20070590
Cortez, V. S., Ulland, T. K., Cervantes-Barragan, L., Bando, J. K., Robinette, M. L., Wang, Q., et al. (2017). SMAD4 impedes the conversion of NK cells into ILC1-like cells by curtailing non-canonical TGF-beta signaling. Nat. Immunol. 18 (9), 995–1003. doi: 10.1038/ni.3809
Cosmi, L., De Palma, R., Santarlasci, V., Maggi, L., Capone, M., Frosali, F., et al. (2008). Human interleukin 17-producing cells originate from a CD161+CD4+ T cell precursor. J. Exp. Med. 205 (8), 1903–1916. doi: 10.1084/jem.20080397
Costa, A., Kieffer, Y., Scholer-Dahirel, A., Pelon, F., Bourachot, B., Cardon, M., et al. (2018). Fibroblast heterogeneity and immunosuppressive environment in human breast cancer. Cancer Cell 33 (3), 463–479 e410. doi: 10.1016/j.ccell.2018.01.011
Cua, D. J., Sherlock, J., Chen, Y., Murphy, C. A., Joyce, B., Seymour, B., et al. (2003). Interleukin-23 rather than interleukin-12 is the critical cytokine for autoimmune inflammation of the brain. Nature 421 (6924), 744–748. doi: 10.1038/nature01355
Curotto de Lafaille, M. A., Kutchukhidze, N., Shen, S., Ding, Y., Yee, H., Lafaille, J. J. (2008). Adaptive Foxp3+ regulatory T cell-dependent and -independent control of allergic inflammation. Immunity 29 (1), 114–126. doi: 10.1016/j.immuni.2008.05.010
Dambuza, I. M., He, C., Choi, J. K., Yu, C. R., Wang, R., Mattapallil, M. J., et al. (2017). IL-12p35 induces expansion of IL-10 and IL-35-expressing regulatory B cells and ameliorates autoimmune disease. Nat. Commun. 8 (1), 719. doi: 10.1038/s41467-017-00838-4
Davidson, T. S., DiPaolo, R. J., Andersson, J., Shevach, E. M. (2007). Cutting Edge: IL-2 is essential for TGF-beta-mediated induction of Foxp3+ T regulatory cells. J. Immunol. 178 (7), 4022–4026. doi: 10.4049/jimmunol.178.7.4022
De Simone, M., Arrigoni, A., Rossetti, G., Gruarin, P., Ranzani, V., Politano, C., et al. (2016). Transcriptional landscape of human tissue lymphocytes unveils uniqueness of tumor-infiltrating T regulatory cells. Immunity 45 (5), 1135–1147. doi: 10.1016/j.immuni.2016.10.021
Deknuydt, F., Bioley, G., Valmori, D., Ayyoub, M. (2009). IL-1beta and IL-2 convert human Treg into T(H)17 cells. Clin. Immunol. 131 (2), 298–307. doi: 10.1016/j.clim.2008.12.008
Doherty, D. G., O’Farrelly, C. (2000). Innate and adaptive lymphoid cells in the human liver. Immunol. Rev. 174, 5–20. doi: 10.1034/j.1600-0528.2002.017416.x
Ebinuma, H., Nakamoto, N., Li, Y., Price, D. A., Gostick, E., Levine, B. L., et al. (2008). Identification and in vitro expansion of functional antigen-specific CD25+ FoxP3+ regulatory T cells in hepatitis C virus infection. J. Virol. 82 (10), 5043–5053. doi: 10.1128/JVI.01548-07
Epp, A., Hobusch, J., Bartsch, Y. C., Petry, J., Lilienthal, G. M., Koeleman, C. A. M., et al. (2018). Sialylation of IgG antibodies inhibits IgG-mediated allergic reactions. J. Allergy Clin. Immunol. 141 (1), 399–402 e398. doi: 10.1016/j.jaci.2017.06.021
Ferber, I. A., Brocke, S., Taylor-Edwards, C., Ridgway, W., Dinisco, C., Steinman, L., et al. (1996). Mice with a disrupted IFN-gamma gene are susceptible to the induction of experimental autoimmune encephalomyelitis (EAE). J. Immunol. 156 (1), 5–7.
Ferri, S., Longhi, M. S., De Molo, C., Lalanne, C., Muratori, P., Granito, A., et al. (2010). A multifaceted imbalance of T cells with regulatory function characterizes type 1 autoimmune hepatitis. Hepatology 52 (3), 999–1007. doi: 10.1002/hep.23792
Fontenot, J. D., Gavin, M. A., Rudensky, A. Y. (2017). Pillars article: Foxp3 programs the development and function of CD4+CD25+ regulatory T cells. Nat. Immunol. 2003. 4, 330–336. J. Immunol. 198 (3), 986–992. doi: 10.1038/ni904
Gaublomme, J. T., Yosef, N., Lee, Y., Gertner, R. S., Yang, L. V., Wu, C., et al. (2015). Single-cell genomics unveils critical regulators of Th17 cell pathogenicity. Cell 163 (6), 1400–1412. doi: 10.1016/j.cell.2015.11.009
Ghazal, K., Morales, O., Barjon, C., Dahlqvist, G., Aoudjehane, L., Ouaguia, L., et al. (2019). Early high levels of regulatory T cells and T helper 1 may predict the progression of recurrent hepatitis C after liver transplantation. Clin. Res. Hepatol. Gastroenterol. 43 (3), 273–281. doi: 10.1016/j.clinre.2018.10.005
Ghoreschi, K., Laurence, A., Yang, X. P., Tato, C. M., McGeachy, M. J., Konkel, J. E., et al. (2010). Generation of pathogenic T(H)17 cells in the absence of TGF-beta signalling. Nature 467 (7318), 967–971. doi: 10.1038/nature09447
Gibson, D. J., Elliott, L., McDermott, E., Tosetto, M., Keegan, D., Byrne, K., et al. (2015). Heightened expression of CD39 by regulatory T lymphocytes Is associated with therapeutic remission in inflammatory bowel disease. Inflammation Bowel. Dis. 21 (12), 2806–2814. doi: 10.1097/MIB.0000000000000566
Globig, A. M., Hennecke, N., Martin, B., Seidl, M., Ruf, G., Hasselblatt, P., et al. (2014). Comprehensive intestinal T helper cell profiling reveals specific accumulation of IFN-gamma+IL-17+coproducing CD4+ T cells in active inflammatory bowel disease. Inflammation Bowel. Dis. 20 (12), 2321–2329. doi: 10.1097/MIB.0000000000000210
Gomes, A. L., Teijeiro, A., Buren, S., Tummala, K. S., Yilmaz, M., Waisman, A., et al. (2016). Metabolic inflammation-associated IL-17A causes non-alcoholic steatohepatitis and hepatocellular carcinoma. Cancer Cell 30 (1), 161–175. doi: 10.1016/j.ccell.2016.05.020
Goudy, K., Aydin, D., Barzaghi, F., Gambineri, E., Vignoli, M., Ciullini Mannurita, S., et al. (2013). Human IL2RA null mutation mediates immunodeficiency with lymphoproliferation and autoimmunity. Clin. Immunol. 146 (3), 248–261. doi: 10.1016/j.clim.2013.01.004
Grant, C. R., Liberal, R., Holder, B. S., Cardone, J., Ma, Y., Robson, S. C., et al. (2014). Dysfunctional CD39(POS) regulatory T cells and aberrant control of T-helper type 17 cells in autoimmune hepatitis. Hepatology 59 (3), 1007–1015. doi: 10.1002/hep.26583
Gutcher, I., Donkor, M. K., Ma, Q., Rudensky, A. Y., Flavell, R. A., Li, M. O. (2011). Autocrine transforming growth factor-beta1 promotes in vivo Th17 cell differentiation. Immunity 34 (3), 396–408. doi: 10.1016/j.immuni.2011.03.005
Ha, D., Tanaka, A., Kibayashi, T., Tanemura, A., Sugiyama, D., Wing, J. B., et al. (2019). Differential control of human Treg and effector T cells in tumor immunity by Fc-engineered anti-CTLA-4 antibody. Proc. Natl. Acad. Sci. U.S.A. 116 (2), 609–618. doi: 10.1073/pnas.1812186116
Hadis, U., Wahl, B., Schulz, O., Hardtke-Wolenski, M., Schippers, A., Wagner, N., et al. (2011). Intestinal tolerance requires gut homing and expansion of FoxP3+ regulatory T cells in the lamina propria. Immunity 34 (2), 237–246. doi: 10.1016/j.immuni.2011.01.016
Hammerich, L., Heymann, F., Tacke, F. (2011). Role of IL-17 and Th17 cells in liver diseases. Clin. Dev. Immunol. 2011, 345803. doi: 10.1155/2011/345803
Han, J. W., Joo, D. J., Kim, J. H., Rha, M. S., Koh, J. Y., Park, H. J., et al. (2020). Early reduction of regulatory T cells is associated with acute rejection in liver transplantation under tacrolimus-based immunosuppression with basiliximab induction. Am. J. Transplant. 20 (8), 2058–2069. doi: 10.1111/ajt.15789
Harbour, S. N., Maynard, C. L., Zindl, C. L., Schoeb, T. R., Weaver, C. T. (2015). Th17 cells give rise to Th1 cells that are required for the pathogenesis of colitis. Proc. Natl. Acad. Sci. U.S.A. 112 (22), 7061–7066. doi: 10.1073/pnas.1415675112
Harrington, L. E., Hatton, R. D., Mangan, P. R., Turner, H., Murphy, T. L., Murphy, K. M., et al. (2005). Interleukin 17-producing CD4+ effector T cells develop via a lineage distinct from the T helper type 1 and 2 lineages. Nat. Immunol. 6 (11), 1123–1132. doi: 10.1038/ni1254
Hartemann, A., Bensimon, G., Payan, C. A., Jacqueminet, S., Bourron, O., Nicolas, N., et al. (2013). Low-dose interleukin 2 in patients with type 1 diabetes: a phase 1/2 randomised, double-blind, placebo-controlled trial. Lancet Diabetes Endocrinol. 1 (4), 295–305. doi: 10.1016/S2213-8587(13)70113-X
He, J. S., Meyer-Hermann, M., Xiangying, D., Zuan, L. Y., Jones, L. A., Ramakrishna, L., et al. (2013). The distinctive germinal center phase of IgE+ B lymphocytes limits their contribution to the classical memory response. J. Exp. Med. 210 (12), 2755–2771. doi: 10.1084/jem.20131539
He, J., Zhang, X., Wei, Y., Sun, X., Chen, Y., Deng, J., et al. (2016). Low-dose interleukin-2 treatment selectively modulates CD4(+) T cell subsets in patients with systemic lupus erythematosus. Nat. Med. 22 (9), 991–993. doi: 10.1038/nm.4148
Hill, J. A., Feuerer, M., Tash, K., Haxhinasto, S., Perez, J., Melamed, R., et al. (2007). Foxp3 transcription-factor-dependent and -independent regulation of the regulatory T cell transcriptional signature. Immunity 27 (5), 786–800. doi: 10.1016/j.immuni.2007.09.010
Hirota, K., Duarte, J. H., Veldhoen, M., Hornsby, E., Li, Y., Cua, D. J., et al. (2011). Fate mapping of IL-17-producing T cells in inflammatory responses. Nat. Immunol. 12 (3), 255–263. doi: 10.1038/ni.1993
Hirota, K., Turner, J. E., Villa, M., Duarte, J. H., Demengeot, J., Steinmetz, O. M., et al. (2013). Plasticity of Th17 cells in Peyer’s patches is responsible for the induction of T cell-dependent IgA responses. Nat. Immunol. 14 (4), 372–379. doi: 10.1038/ni.2552
Hirschfield, G. M., Karlsen, T. H., Lindor, K. D., Adams, D. H. (2013). Primary sclerosing cholangitis. Lancet 382 (9904), 1587–1599. doi: 10.1016/S0140-6736(13)60096-3
Huber, S., Gagliani, N., Esplugues, E., O’Connor, W., Jr., Huber, F. J., Chaudhry, A., et al. (2011). Th17 cells express interleukin-10 receptor and are controlled by Foxp3(-) and Foxp3+ regulatory CD4+ T cells in an interleukin-10-dependent manner. Immunity 34 (4), 554–565. doi: 10.1016/j.immuni.2011.01.020
Huber, S., Gagliani, N., Flavell, R. A. (2012). Life, death, and miracles: Th17 cells in the intestine. Eur. J. Immunol. 42 (9), 2238–2245. doi: 10.1002/eji.201242619
Hubscher, S. G. (2006). Histological assessment of non-alcoholic fatty liver disease. Histopathology 49 (5), 450–465. doi: 10.1111/j.1365-2559.2006.02416.x
Humrich, J. Y., von Spee-Mayer, C., Siegert, E., Alexander, T., Hiepe, F., Radbruch, A., et al. (2015). Rapid induction of clinical remission by low-dose interleukin-2 in a patient with refractory SLE. Ann. Rheum. Dis. 74 (4), 791–792. doi: 10.1136/annrheumdis-2014-206506
Ichikawa, S., Mucida, D., Tyznik, A. J., Kronenberg, M., Cheroutre, H. (2011). Hepatic stellate cells function as regulatory bystanders. J. Immunol. 186 (10), 5549–5555. doi: 10.4049/jimmunol.1003917
Ivanov, I. I., McKenzie, B. S., Zhou, L., Tadokoro, C. E., Lepelley, A., Lafaille, J. J., et al. (2006). The orphan nuclear receptor RORgammat directs the differentiation program of proinflammatory IL-17+ T helper cells. Cell 126 (6), 1121–1133. doi: 10.1016/j.cell.2006.07.035
Jiang, G., Yang, H. R., Wang, L., Wildey, G. M., Fung, J., Qian, S., et al. (2008). Hepatic stellate cells preferentially expand allogeneic CD4+ CD25+ FoxP3+ regulatory T cells in an IL-2-dependent manner. Transplantation 86 (11), 1492–1502. doi: 10.1097/TP.0b013e31818bfd13
Kamada, T., Togashi, Y., Tay, C., Ha, D., Sasaki, A., Nakamura, Y., et al. (2019). PD-1(+) regulatory T cells amplified by PD-1 blockade promote hyperprogression of cancer. Proc. Natl. Acad. Sci. U.S.A. 116 (20), 9999–10008. doi: 10.1073/pnas.1822001116
Kato, T., Noma, K., Ohara, T., Kashima, H., Katsura, Y., Sato, H., et al. (2018). Cancer-associated fibroblasts affect intratumoral CD8(+) and FoxP3(+) T cells via IL6 in the tumor microenvironment. Clin. Cancer Res. 24 (19), 4820–4833. doi: 10.1158/1078-0432.CCR-18-0205
Khader, S. A., Bell, G. K., Pearl, J. E., Fountain, J. J., Rangel-Moreno, J., Cilley, G. E., et al. (2007). IL-23 and IL-17 in the establishment of protective pulmonary CD4+ T cell responses after vaccination and during Mycobacterium tuberculosis challenge. Nat. Immunol. 8 (4), 369–377. doi: 10.1038/ni1449
Kikuchi, J., Hashizume, M., Kaneko, Y., Yoshimoto, K., Nishina, N., Takeuchi, T. (2015). Peripheral blood CD4(+)CD25(+)CD127(low) regulatory T cells are significantly increased by tocilizumab treatment in patients with rheumatoid arthritis: increase in regulatory T cells correlates with clinical response. Arthritis Res. Ther. 17, 10. doi: 10.1186/s13075-015-0526-4
Kobayashi, E., Kobayashi, M., Tsuneyama, K., Fukami, T., Nakajima, M., Yokoi, T. (2009). Halothane-induced liver injury is mediated by interleukin-17 in mice. Toxicol. Sci. 111 (2), 302–310. doi: 10.1093/toxsci/kfp165
Koenen, H. J., Smeets, R. L., Vink, P. M., van Rijssen, E., Boots, A. M., Joosten, I. (2008). Human CD25highFoxp3pos regulatory T cells differentiate into IL-17-producing cells. Blood 112 (6), 2340–2352. doi: 10.1182/blood-2008-01-133967
Korn, T., Bettelli, E., Gao, W., Awasthi, A., Jager, A., Strom, T. B., et al. (2007). IL-21 initiates an alternative pathway to induce proinflammatory T(H)17 cells. Nature 448 (7152), 484–487. doi: 10.1038/nature05970
Krueger, G. G., Langley, R. G., Leonardi, C., Yeilding, N., Guzzo, C., Wang, Y., et al. (2007). A human interleukin-12/23 monoclonal antibody for the treatment of psoriasis. N. Engl. J. Med. 356 (6), 580–592. doi: 10.1056/NEJMoa062382
Krummel, M. F., Allison, J. P. (1996). CTLA-4 engagement inhibits IL-2 accumulation and cell cycle progression upon activation of resting T cells. J. Exp. Med. 183 (6), 2533–2540. doi: 10.1084/jem.183.6.2533
Kuehn, H. S., Ouyang, W., Lo, B., Deenick, E. K., Niemela, J. E., Avery, D. T., et al. (2014). Immune dysregulation in human subjects with heterozygous germline mutations in CTLA4. Science 345 (6204), 1623–1627. doi: 10.1126/science.1255904
Lan, Y. T., Wang, Z. L., Tian, P., Gong, X. N., Fan, Y. C., Wang, K. (2019). Treg/Th17 imbalance and its clinical significance in patients with hepatitis B-associated liver cirrhosis. Diagn. Pathol. 14 (1), 114. doi: 10.1186/s13000-019-0891-4
Langhans, B., Braunschweiger, I., Arndt, S., Schulte, W., Satoguina, J., Layland, L. E., et al. (2010). Core-specific adaptive regulatory T-cells in different outcomes of hepatitis C. Clin. Sci. (Lond) 119 (2), 97–109. doi: 10.1042/CS20090661
Langowski, J. L., Zhang, X., Wu, L., Mattson, J. D., Chen, T., Smith, K., et al. (2006). IL-23 promotes tumour incidence and growth. Nature 442 (7101), 461–465. doi: 10.1038/nature04808
Lapierre, P., Beland, K., Yang, R., Alvarez, F. (2013). Adoptive transfer of ex vivo expanded regulatory T cells in an autoimmune hepatitis murine model restores peripheral tolerance. Hepatology 57 (1), 217–227. doi: 10.1002/hep.26023
Lathrop, S. K., Bloom, S. M., Rao, S. M., Nutsch, K., Lio, C. W., Santacruz, N., et al. (2011). Peripheral education of the immune system by colonic commensal microbiota. Nature 478 (7368), 250–254. doi: 10.1038/nature10434
Le Bras, S., Geha, R. S. (2006). IPEX and the role of Foxp3 in the development and function of human Tregs. J. Clin. Invest. 116 (6), 1473–1475. doi: 10.1172/JCI28880
Lee, Y. K., Turner, H., Maynard, C. L., Oliver, J. R., Chen, D., Elson, C. O., et al. (2009). Late developmental plasticity in the T helper 17 lineage. Immunity 30 (1), 92–107. doi: 10.1016/j.immuni.2008.11.005
Lemmers, A., Moreno, C., Gustot, T., Marechal, R., Degre, D., Demetter, P., et al. (2009). The interleukin-17 pathway is involved in human alcoholic liver disease. Hepatology 49 (2), 646–657. doi: 10.1002/hep.22680
Li, J., Qiu, S. J., She, W. M., Wang, F. P., Gao, H., Li, L., et al. (2012). Significance of the balance between regulatory T (Treg) and T helper 17 (Th17) cells during hepatitis B virus related liver fibrosis. PloS One 7 (6), e39307. doi: 10.1371/journal.pone.0039307
Linterman, M. A., Pierson, W., Lee, S. K., Kallies, A., Kawamoto, S., Rayner, T. F., et al. (2011). Foxp3+ follicular regulatory T cells control the germinal center response. Nat. Med. 17 (8), 975–982. doi: 10.1038/nm.2425
Liston, A., Nutsch, K. M., Farr, A. G., Lund, J. M., Rasmussen, J. P., Koni, P. A., et al. (2008). Differentiation of regulatory Foxp3+ T cells in the thymic cortex. Proc. Natl. Acad. Sci. U. S. A. 105 (33), 11903–11908. doi: 10.1073/pnas.0801506105
Liu, C., Xu, L., Xia, C., Long, Y., Liu, C., Lu, S., et al. (2020). Increased proportion of functional subpopulations in circulating regulatory T cells in patients with chronic hepatitis B. Hepatol. Res. 50 (4), 439–452. doi: 10.1111/hepr.13472
Losikoff, P. T., Self, A. A., Gregory, S. H. (2012). Dendritic cells, regulatory T cells and the pathogenesis of chronic hepatitis C. Virulence 3 (7), 610–620. doi: 10.4161/viru.21823
Ma, C., Kesarwala, A. H., Eggert, T., Medina-Echeverz, J., Kleiner, D. E., Jin, P., et al. (2016). NAFLD causes selective CD4(+) T lymphocyte loss and promotes hepatocarcinogenesis. Nature 531 (7593), 253–257. doi: 10.1038/nature16969
Maggi, L., Santarlasci, V., Capone, M., Peired, A., Frosali, F., Crome, S. Q., et al. (2010). CD161 is a marker of all human IL-17-producing T-cell subsets and is induced by RORC. Eur. J. Immunol. 40 (8), 2174–2181. doi: 10.1002/eji.200940257
Maloy, K. J., Powrie, F. (2001). Regulatory T cells in the control of immune pathology. Nat. Immunol. 2 (9), 816–822. doi: 10.1038/ni0901-816
Mangan, P. R., Harrington, L. E., O’Quinn, D. B., Helms, W. S., Bullard, D. C., Elson, C. O., et al. (2006). Transforming growth factor-beta induces development of the T(H)17 lineage. Nature 441 (7090), 231–234. doi: 10.1038/nature04754
Manigold, T., Racanelli, V. (2007). T-cell regulation by CD4 regulatory T cells during hepatitis B and C virus infections: facts and controversies. Lancet Infect. Dis. 7 (12), 804–813. doi: 10.1016/S1473-3099(07)70289-X
Mannon, P. J., Fuss, I. J., Mayer, L., Elson, C. O., Sandborn, W. J., Present, D., et al. (2004). Anti-interleukin-12 antibody for active Crohn’s disease. N. Engl. J. Med. 351 (20), 2069–2079. doi: 10.1056/NEJMoa033402
Marie, J. C., Letterio, J. J., Gavin, M., Rudensky, A. Y. (2005). TGF-beta1 maintains suppressor function and Foxp3 expression in CD4+CD25+ regulatory T cells. J. Exp. Med. 201 (7), 1061–1067. doi: 10.1084/jem.20042276
Mathew, J. M., V. J, H., LeFever, A., Konieczna, I., Stratton, C., He, J., et al. (2018). A phase I clinical trial with ex vivo expanded recipient regulatory T cells in living donor kidney transplants. Sci. Rep. 8 (1), 7428. doi: 10.1038/s41598-018-25574-7
Matsuoka, K., Koreth, J., Kim, H. T., Bascug, G., McDonough, S., Kawano, Y., et al. (2013). Low-dose interleukin-2 therapy restores regulatory T cell homeostasis in patients with chronic graft-versus-host disease. Sci. Transl. Med. 5 (179), 179ra143. doi: 10.1126/scitranslmed.3005265
McGeachy, M. J., Bak-Jensen, K. S., Chen, Y., Tato, C. M., Blumenschein, W., McClanahan, T., et al. (2007). TGF-beta and IL-6 drive the production of IL-17 and IL-10 by T cells and restrain T(H)-17 cell-mediated pathology. Nat. Immunol. 8 (12), 1390–1397. doi: 10.1038/ni1539
McGeachy, M. J., Chen, Y., Tato, C. M., Laurence, A., Joyce-Shaikh, B., Blumenschein, W. M., et al. (2009). The interleukin 23 receptor is essential for the terminal differentiation of interleukin 17-producing effector T helper cells in vivo. Nat. Immunol. 10 (3), 314–324. doi: 10.1038/ni.1698
McLaughlin, T., Liu, L. F., Lamendola, C., Shen, L., Morton, J., Rivas, H., et al. (2014). T-cell profile in adipose tissue is associated with insulin resistance and systemic inflammation in humans. Arterioscler. Thromb. Vasc. Biol. 34 (12), 2637–2643. doi: 10.1161/ATVBAHA.114.304636
Meng, F., Wang, K., Aoyama, T., Grivennikov, S. I., Paik, Y., Scholten, D., et al. (2012). Interleukin-17 signaling in inflammatory, Kupffer cells, and hepatic stellate cells exacerbates liver fibrosis in mice. Gastroenterology 143 (3), 765–776.e3. doi: 10.1053/j.gastro.2012.05.049
Meng, P., Zhao, S., Niu, X., Fu, N., Su, S., Wang, R., et al. (2016). Involvement of the interleukin-23/interleukin-17 axis in chronic hepatitis C virus infection and its treatment responses. Int. J. Mol. Sci. 17 (11). doi: 10.3390/ijms17071070
Miyara, M., Yoshioka, Y., Kitoh, A., Shima, T., Wing, K., Niwa, A., et al. (2009). Functional delineation and differentiation dynamics of human CD4+ T cells expressing the FoxP3 transcription factor. Immunity 30 (6), 899–911. doi: 10.1016/j.immuni.2009.03.019
Morgan, M. E., Flierman, R., van Duivenvoorde, L. M., Witteveen, H. J., van Ewijk, W., van Laar, J. M., et al. (2005). Effective treatment of collagen-induced arthritis by adoptive transfer of CD25+ regulatory T cells. Arthritis Rheum. 52 (7), 2212–2221. doi: 10.1002/art.21195
Morrison, P. J., Bending, D., Fouser, L. A., Wright, J. F., Stockinger, B., Cooke, A., et al. (2013). Th17-cell plasticity in Helicobacter hepaticus-induced intestinal inflammation. Mucosal. Immunol. 6 (6), 1143–1156. doi: 10.1038/mi.2013.11
Mosmann, T. R., Cherwinski, H., Bond, M. W., Giedlin, M. A., Coffman, R. L. (1986). Two types of murine helper T cell clone. I. Definition according to profiles of lymphokine activities and secreted proteins. J. Immunol. 136 (7), 2348–2357.
Mougiakakos, D., Johansson, C. C., Trocme, E., All-Ericsson, C., Economou, M. A., Larsson, O., et al. (2010). Intratumoral forkhead box P3-positive regulatory T cells predict poor survival in cyclooxygenase-2-positive uveal melanoma. Cancer 116 (9), 2224–2233. doi: 10.1002/cncr.24999
Mucida, D., Pino-Lagos, K., Kim, G., Nowak, E., Benson, M. J., Kronenberg, M., et al. (2009). Retinoic acid can directly promote TGF-beta-mediated Foxp3(+) Treg cell conversion of naive T cells. Immunity 30 (4), 471–472; author reply 472-473. doi: 10.1016/j.immuni.2009.03.008
Murphy, C. A., Langrish, C. L., Chen, Y., Blumenschein, W., McClanahan, T., Kastelein, R. A., et al. (2003). Divergent pro- and antiinflammatory roles for IL-23 and IL-12 in joint autoimmune inflammation. J. Exp. Med. 198 (12), 1951–1957. doi: 10.1084/jem.20030896
Nagakawa, K., Soyama, A., Hidaka, M., Adachi, T., Ono, S., Hara, T., et al. (2020). Elevated plasma levels of mitochondria-derived damage-associated molecular patterns during liver transplantation: predictors for postoperative multi-organ dysfunction syndrome. Tohoku J. Exp. Med. 250 (2), 87–93. doi: 10.1620/tjem.250.87
Nagata, T., McKinley, L., Peschon, J. J., Alcorn, J. F., Aujla, S. J., Kolls, J. K. (2008). Requirement of IL-17RA in Con A induced hepatitis and negative regulation of IL-17 production in mouse T cells. J. Immunol. 181 (11), 7473–7479. doi: 10.4049/jimmunol.181.11.7473
Nakae, S., Iwakura, Y., Suto, H., Galli, S. J. (2007). Phenotypic differences between Th1 and Th17 cells and negative regulation of Th1 cell differentiation by IL-17. J. Leukoc. Biol. 81 (5), 1258–1268. doi: 10.1189/jlb.1006610
Neurath, M. F., Weigmann, B., Finotto, S., Glickman, J., Nieuwenhuis, E., Iijima, H., et al. (2002). The transcription factor T-bet regulates mucosal T cell activation in experimental colitis and Crohn’s disease. J. Exp. Med. 195 (9), 1129–1143. doi: 10.1084/jem.20011956
Niedbala, W., Wei, X. Q., Cai, B., Hueber, A. J., Leung, B. P., McInnes, I. B., et al. (2007). IL-35 is a novel cytokine with therapeutic effects against collagen-induced arthritis through the expansion of regulatory T cells and suppression of Th17 cells. Eur. J. Immunol. 37 (11), 3021–3029. doi: 10.1002/eji.200737810
Oberle, N., Eberhardt, N., Falk, C. S., Krammer, P. H., Suri-Payer, E. (2007). Rapid suppression of cytokine transcription in human CD4+CD25 T cells by CD4+Foxp3+ regulatory T cells: independence of IL-2 consumption, TGF-beta, and various inhibitors of TCR signaling. J. Immunol. 179 (6), 3578–3587. doi: 10.4049/jimmunol.179.6.3578
Oo, Y. H., Banz, V., Kavanagh, D., Liaskou, E., Withers, D. R., Humphreys, E., et al. (2012). CXCR3-dependent recruitment and CCR6-mediated positioning of Th-17 cells in the inflamed liver. J. Hepatol. 57 (5), 1044–1051. doi: 10.1016/j.jhep.2012.07.008
Oppmann, B., Lesley, R., Blom, B., Timans, J. C., Xu, Y., Hunte, B., et al. (2000). Novel p19 protein engages IL-12p40 to form a cytokine, IL-23, with biological activities similar as well as distinct from IL-12. Immunity 13 (5), 715–725. doi: 10.1016/S1074-7613(00)00070-4
O’Garra, A., Vieira, P. L., Vieira, P., Goldfeld, A. E. (2004). IL-10-producing and naturally occurring CD4+ Tregs: limiting collateral damage. J. Clin. Invest. 114 (10), 1372–1378. doi: 10.1172/JCI23215
Pandiyan, P., Zheng, L., Ishihara, S., Reed, J., Lenardo, M. J. (2007). CD4+CD25+Foxp3+ regulatory T cells induce cytokine deprivation-mediated apoptosis of effector CD4+ T cells. Nat. Immunol. 8 (12), 1353–1362. doi: 10.1038/ni1536
Park, H., Li, Z., Yang, X. O., Chang, S. H., Nurieva, R., Wang, Y. H., et al. (2005). A distinct lineage of CD4 T cells regulates tissue inflammation by producing interleukin 17. Nat. Immunol. 6 (11), 1133–1141. doi: 10.1038/ni1261
Park, S. H., Veerapu, N. S., Shin, E. C., Biancotto, A., McCoy, J. P., Capone, S., et al. (2013). Subinfectious hepatitis C virus exposures suppress T cell responses against subsequent acute infection. Nat. Med. 19 (12), 1638–1642. doi: 10.1038/nm.3408
Piccirillo, C. A. (2008). Regulatory T cells in health and disease. Cytokine 43 (3), 395–401. doi: 10.1016/j.cyto.2008.07.469
Pillai, V., Ortega, S. B., Wang, C. K., Karandikar, N. J. (2007). Transient regulatory T-cells: a state attained by all activated human T-cells. Clin. Immunol. 123 (1), 18–29. doi: 10.1016/j.clim.2006.10.014
Rau, M., Schilling, A. K., Meertens, J., Hering, I., Weiss, J., Jurowich, C., et al. (2016). Progression from nonalcoholic fatty liver to nonalcoholic steatohepatitis is marked by a higher frequency of Th17 cells in the liver and an increased Th17/resting regulatory T cell ratio in peripheral blood and in the liver. J. Immunol. 196 (1), 97–105. doi: 10.4049/jimmunol.1501175
Refolo, M. G., Messa, C., Guerra, V., Carr, B. I., D’Alessandro, R. (2020). Inflammatory Mechanisms of HCC Development. Cancers (Basel) 12 (3), 641. doi: 10.3390/cancers12030641
Ring, S., Pushkarevskaya, A., Schild, H., Probst, H. C., Jendrossek, V., Wirsdorfer, F., et al. (2015). Regulatory T cell-derived adenosine induces dendritic cell migration through the Epac-Rap1 pathway. J. Immunol. 194 (8), 3735–3744. doi: 10.4049/jimmunol.1401434
Romano, M., Tung, S. L., Smyth, L. A., Lombardi, G. (2017). Treg therapy in transplantation: a general overview. Transpl. Int. 30 (8), 745–753. doi: 10.1111/tri.12909
Rosenzwajg, M., Churlaud, G., Mallone, R., Six, A., Derian, N., Chaara, W., et al. (2015). Low-dose interleukin-2 fosters a dose-dependent regulatory T cell tuned milieu in T1D patients. J. Autoimmun. 58, 48–58. doi: 10.1016/j.jaut.2015.01.001
Sage, P. T., Paterson, A. M., Lovitch, S. B., Sharpe, A. H. (2014). The coinhibitory receptor CTLA-4 controls B cell responses by modulating T follicular helper, T follicular regulatory, and T regulatory cells. Immunity 41 (6), 1026–1039. doi: 10.1016/j.immuni.2014.12.005
Sakaguchi, S. (2004). Naturally arising CD4+ regulatory t cells for immunologic self-tolerance and negative control of immune responses. Annu. Rev. Immunol. 22, 531–562. doi: 10.1146/annurev.immunol.21.120601.141122
Sanchez-Fueyo, A., Whitehouse, G., Grageda, N., Cramp, M. E., Lim, T. Y., Romano, M., et al. (2020). Applicability, safety, and biological activity of regulatory T cell therapy in liver transplantation. Am. J. Transplant. 20 (4), 1125–1136. doi: 10.1111/ajt.15700
Sands, B. E., Sandborn, W. J., Panaccione, R., O’Brien, C. D., Zhang, H., Johanns, J., et al. (2019). Ustekinumab as induction and maintenance therapy for ulcerative colitis. N. Engl. J. Med. 381 (13), 1201–1214. doi: 10.1056/NEJMoa1900750
Sanford, M., McKeage, K. (2015). Secukinumab: first global approval. Drugs 75 (3), 329–338. doi: 10.1007/s40265-015-0359-0
Sansom, D. M. (2015). IMMUNOLOGY. Moving CTLA-4 from the trash to recycling. Science 349 (6246), 377–378. doi: 10.1126/science.aac7888
Saravia, J., Chapman, N. M., Chi, H. (2019). Helper T cell differentiation. Cell Mol. Immunol. 16 (7), 634–643. doi: 10.1038/s41423-019-0220-6
Sarhan, D., Hippen, K. L., Lemire, A., Hying, S., Luo, X., Lenvik, T., et al. (2018). Adaptive NK cells resist regulatory T-cell suppression driven by IL37. Cancer Immunol. Res. 6 (7), 766–775. doi: 10.1158/2326-6066.CIR-17-0498
Schubert, D., Bode, C., Kenefeck, R., Hou, T. Z., Wing, J. B., Kennedy, A., et al. (2014). Autosomal dominant immune dysregulation syndrome in humans with CTLA4 mutations. Nat. Med. 20 (12), 1410–1416. doi: 10.1038/nm.3746
Schuler, P. J., Saze, Z., Hong, C. S., Muller, L., Gillespie, D. G., Cheng, D., et al. (2014). Human CD4+ CD39+ regulatory T cells produce adenosine upon co-expression of surface CD73 or contact with CD73+ exosomes or CD73+ cells. Clin. Exp. Immunol. 177 (2), 531–543. doi: 10.1111/cei.12354
Sebode, M., Peiseler, M., Franke, B., Schwinge, D., Schoknecht, T., Wortmann, F., et al. (2014). Reduced FOXP3(+) regulatory T cells in patients with primary sclerosing cholangitis are associated with IL2RA gene polymorphisms. J. Hepatol. 60 (5), 1010–1016. doi: 10.1016/j.jhep.2013.12.027
Setoguchi, R., Hori, S., Takahashi, T., Sakaguchi, S. (2005). Homeostatic maintenance of natural Foxp3(+) CD25(+) CD4(+) regulatory T cells by interleukin (IL)-2 and induction of autoimmune disease by IL-2 neutralization. J. Exp. Med. 201 (5), 723–735. doi: 10.1084/jem.20041982
Shi, T., Zhang, T., Zhang, L., Yang, Y., Zhang, H., Zhang, F. (2015). The distribution and the fibrotic role of elevated inflammatory Th17 cells in patients with primary biliary cirrhosis. Med. (Baltimore) 94 (44), e1888. doi: 10.1097/MD.0000000000001888
Stumhofer, J. S., Laurence, A., Wilson, E. H., Huang, E., Tato, C. M., Johnson, L. M., et al. (2006). Interleukin 27 negatively regulates the development of interleukin 17-producing T helper cells during chronic inflammation of the central nervous system. Nat. Immunol. 7 (9), 937–945. doi: 10.1038/ni1376
Sun, H. Q., Zhang, J. Y., Zhang, H., Zou, Z. S., Wang, F. S., Jia, J. H. (2012). Increased Th17 cells contribute to disease progression in patients with HBV-associated liver cirrhosis. J. Viral. Hepat. 19 (6), 396–403. doi: 10.1111/j.1365-2893.2011.01561.x
Sun, B., Liu, M., Cui, M., Li, T. (2020). Granzyme B-expressing treg cells are enriched in colorectal cancer and present the potential to eliminate autologous T conventional cells. Immunol. Lett. 217, 7–14. doi: 10.1016/j.imlet.2019.10.007
Tai, X., Cowan, M., Feigenbaum, L., Singer, A. (2005). CD28 costimulation of developing thymocytes induces Foxp3 expression and regulatory T cell differentiation independently of interleukin 2. Nat. Immunol. 6 (2), 152–162. doi: 10.1038/ni1160
Tait, B., Mackay, I. R., Board, P., Coggan, M., Emery, P., Eckardt, G. (1989). HLA A1, B8, DR3 extended haplotypes in autoimmune chronic hepatitis. Gastroenterology 97 (2), 479–481. doi: 10.1016/0016-5085(89)90088-7
Takimoto, T., Wakabayashi, Y., Sekiya, T., Inoue, N., Morita, R., Ichiyama, K., et al. (2010). Smad2 and Smad3 are redundantly essential for the TGF-beta-mediated regulation of regulatory T plasticity and Th1 development. J. Immunol. 185 (2), 842–855. doi: 10.4049/jimmunol.0904100
Tan, Z., Qian, X., Jiang, R., Liu, Q., Wang, Y., Chen, C., et al. (2013). IL-17A plays a critical role in the pathogenesis of liver fibrosis through hepatic stellate cell activation. J. Immunol. 191 (4), 1835–1844. doi: 10.4049/jimmunol.1203013
Taylor, A., Akdis, M., Joss, A., Akkoc, T., Wenig, R., Colonna, M., et al. (2007). IL-10 inhibits CD28 and ICOS costimulations of T cells via src homology 2 domain-containing protein tyrosine phosphatase 1. J. Allergy Clin. Immunol. 120 (1), 76–83. doi: 10.1016/j.jaci.2007.04.004
Tesmer, L. A., Lundy, S. K., Sarkar, S., Fox, D. A. (2008). Th17 cells in human disease. Immunol. Rev. 223, 87–113. doi: 10.1111/j.1600-065X.2008.00628.x
Thomas, D. A., Massague, J. (2005). TGF-beta directly targets cytotoxic T cell functions during tumor evasion of immune surveillance. Cancer Cell 8 (5), 369–380. doi: 10.1016/j.ccr.2005.10.012
Toker, A., Engelbert, D., Garg, G., Polansky, J. K., Floess, S., Miyao, T., et al. (2013). Active demethylation of the Foxp3 locus leads to the generation of stable regulatory T cells within the thymus. J. Immunol. 190 (7), 3180–3188. doi: 10.4049/jimmunol.1203473
Tran, D. Q. (2012). TGF-beta: the sword, the wand, and the shield of FOXP3(+) regulatory T cells. J. Mol. Cell Biol. 4 (1), 29–37. doi: 10.1093/jmcb/mjr033
TrehanPati, N., Kotillil, S., Hissar, S. S., Shrivastava, S., Khanam, A., Sukriti, S., et al. (2011). Circulating Tregs correlate with viral load reduction in chronic HBV-treated patients with tenofovir disoproxil fumarate. J. Clin. Immunol. 31 (3), 509–520. doi: 10.1007/s10875-011-9509-7
Vaknin-Dembinsky, A., Balashov, K., Weiner, H. L. (2006). IL-23 is increased in dendritic cells in multiple sclerosis and down-regulation of IL-23 by antisense oligos increases dendritic cell IL-10 production. J. Immunol. 176 (12), 7768–7774. doi: 10.4049/jimmunol.176.12.7768
Van Herck, M. A., Vonghia, L., Kwanten, W. J., Jule, Y., Vanwolleghem, T., Ebo, D., et al. (2020). Diet reversal and immune modulation show key role for liver and adipose tissue T cells in murine non-alcoholic steatohepatitis. Cell Mol. Gastroenterol. Hepatol. 10 (3), 467–490. doi: 10.1016/j.jcmgh.2020.04.010
Vang, K. B., Yang, J., Mahmud, S. A., Burchill, M. A., Vegoe, A. L., Farrar, M. A. (2008). IL-2, -7, and -15, but not thymic stromal lymphopoeitin, redundantly govern CD4+Foxp3+ regulatory T cell development. J. Immunol. 181 (5), 3285–3290. doi: 10.4049/jimmunol.181.5.3285
Veldhoen, M., Hocking, R. J., Flavell, R. A., Stockinger, B. (2006). Signals mediated by transforming growth factor-beta initiate autoimmune encephalomyelitis, but chronic inflammation is needed to sustain disease. Nat. Immunol. 7 (11), 1151–1156. doi: 10.1038/ni1391
Voron, T., Marcheteau, E., Pernot, S., Colussi, O., Tartour, E., Taieb, J., et al. (2014). Control of the immune response by pro-angiogenic factors. Front. Oncol. 4, 70. doi: 10.3389/fonc.2014.00070
Wakabayashi, K., Lian, Z. X., Moritoki, Y., Lan, R. Y., Tsuneyama, K., Chuang, Y. H., et al. (2006). IL-2 receptor alpha(-/-) mice and the development of primary biliary cirrhosis. Hepatology 44 (5), 1240–1249. doi: 10.1002/hep.21385
Wang, J. M., Shi, L., Ma, C. J., Ji, X. J., Ying, R. S., Wu, X. Y., et al. (2013). Differential regulation of interleukin-12 (IL-12)/IL-23 by Tim-3 drives T(H)17 cell development during hepatitis C virus infection. J. Virol. 87 (8), 4372–4383. doi: 10.1128/JVI.03376-12
Wang, K., Song, Z. L., Wu, B., Zhou, C. L., Liu, W., Gao, W. (2019). The T-helper cells 17 instead of Tregs play the key role in acute rejection after pediatric liver transplantation. Pediatr. Transplant. 23 (3), e13363. doi: 10.1111/petr.13363
Wei, F., Zhong, S., Ma, Z., Kong, H., Medvec, A., Ahmed, R., et al. (2013). Strength of PD-1 signaling differentially affects T-cell effector functions. Proc. Natl. Acad. Sci. U.S.A. 110 (27), E2480–E2489. doi: 10.1073/pnas.1305394110
Wollenberg, I., Agua-Doce, A., Hernandez, A., Almeida, C., Oliveira, V. G., Faro, J., et al. (2011). Regulation of the germinal center reaction by Foxp3+ follicular regulatory T cells. J. Immunol. 187 (9), 4553–4560. doi: 10.4049/jimmunol.1101328
Wong, J., Obst, R., Correia-Neves, M., Losyev, G., Mathis, D., Benoist, C. (2007). Adaptation of TCR repertoires to self-peptides in regulatory and nonregulatory CD4+ T cells. J. Immunol. 178 (11), 7032–7041. doi: 10.4049/jimmunol.178.11.7032
Wu, H. J., Ivanov, I. I., Darce, J., Hattori, K., Shima, T., Umesaki, Y., et al. (2010). Gut-residing segmented filamentous bacteria drive autoimmune arthritis via T helper 17 cells. Immunity 32 (6), 815–827. doi: 10.1016/j.immuni.2010.06.001
Yan, Z., Zhou, J., Zhang, M., Fu, X., Wu, Y., Wang, Y. (2014). Telbivudine decreases proportion of peripheral blood CD4+CD25+CD127low T cells in parallel with inhibiting hepatitis B virus DNA. Mol. Med. Rep. 9 (5), 2024–2030. doi: 10.3892/mmr.2014.2042
Yang, X. O., Nurieva, R., Martinez, G. J., Kang, H. S., Chung, Y., Pappu, B. P., et al. (2008). Molecular antagonism and plasticity of regulatory and inflammatory T cell programs. Immunity 29 (1), 44–56. doi: 10.1016/j.immuni.2008.05.007
Yang, B. H., Hagemann, S., Mamareli, P., Lauer, U., Hoffmann, U., Beckstette, M., et al. (2016). Foxp3(+) T cells expressing RORgammat represent a stable regulatory T-cell effector lineage with enhanced suppressive capacity during intestinal inflammation. Mucosal. Immunol. 9 (2), 444–457. doi: 10.1038/mi.2015.74
Yang, L., Jia, S., Shao, X., Liu, S., Zhang, Q., Song, J., et al. (2019). Interleukin-35 modulates the balance between viral specific CD4(+)CD25(+)CD127(dim/-) regulatory T cells and T helper 17 cells in chronic hepatitis B virus infection. Virol. J. 16 (1), 48. doi: 10.1186/s12985-019-1158-0
Yoshida, O., Dou, L., Kimura, S., Yokota, S., Isse, K., Robson, S. C., et al. (2015). CD39 deficiency in murine liver allografts promotes inflammatory injury and immune-mediated rejection. Transpl. Immunol. 32 (2), 76–83. doi: 10.1016/j.trim.2015.01.003
Younossi, Z., Tacke, F., Arrese, M., Chander Sharma, B., Mostafa, I., Bugianesi, E., et al. (2019). Global Perspectives on Nonalcoholic Fatty Liver Disease and Nonalcoholic Steatohepatitis. Hepatology 69 (6), 2672–2682. doi: 10.1002/hep.30251
Zenewicz, L. A., Yancopoulos, G. D., Valenzuela, D. M., Murphy, A. J., Karow, M., Flavell, R. A. (2007). Interleukin-22 but not interleukin-17 provides protection to hepatocytes during acute liver inflammation. Immunity 27 (4), 647–659. doi: 10.1016/j.immuni.2007.07.023
Zhang, Y. H., Sun, H. X. (2020). Immune checkpoint molecules in pregnancy: Focus on regulatory T cells. Eur. J. Immunol. 50 (2), 160–169. doi: 10.1002/eji.201948382
Zhang, J. P., Yan, J., Xu, J., Pang, X. H., Chen, M. S., Li, L., et al. (2009). Increased intratumoral IL-17-producing cells correlate with poor survival in hepatocellular carcinoma patients. J. Hepatol. 50 (5), 980–989. doi: 10.1016/j.jhep.2008.12.033
Zhang, B., Chikuma, S., Hori, S., Fagarasan, S., Honjo, T. (2016). Nonoverlapping roles of PD-1 and FoxP3 in maintaining immune tolerance in a novel autoimmune pancreatitis mouse model. Proc. Natl. Acad. Sci. U.S.A. 113 (30), 8490–8495. doi: 10.1073/pnas.1608873113
Zhang, X., Feng, M., Liu, X., Bai, L., Kong, M., Chen, Y., et al. (2016). Persistence of cirrhosis is maintained by intrahepatic regulatory T cells that inhibit fibrosis resolution by regulating the balance of tissue inhibitors of metalloproteinases and matrix metalloproteinases. Transl. Res. 169, 67–79 e61-62. doi: 10.1016/j.trsl.2015.10.008
Zhao, L., Tang, Y., You, Z., Wang, Q., Liang, S., Han, X., et al. (2011). Interleukin-17 contributes to the pathogenesis of autoimmune hepatitis through inducing hepatic interleukin-6 expression. PloS One 6 (4), e18909. doi: 10.1371/journal.pone.0018909
Zheng, Y., Josefowicz, S., Chaudhry, A., Peng, X. P., Forbush, K., Rudensky, A. Y. (2010). Role of conserved non-coding DNA elements in the Foxp3 gene in regulatory T-cell fate. Nature 463 (7282), 808–812. doi: 10.1038/nature08750
Zhou, L., Ivanov, I. I., Spolski, R., Min, R., Shenderov, K., Egawa, T., et al. (2007). IL-6 programs T(H)-17 cell differentiation by promoting sequential engagement of the IL-21 and IL-23 pathways. Nat. Immunol. 8 (9), 967–974. doi: 10.1038/ni1488
Zhu, J., Yamane, H., Paul, W. E. (2010). Differentiation of effector CD4 T cell populations (*). Annu. Rev. Immunol. 28, 445–489. doi: 10.1146/annurev-immunol-030409-101212
Ziolkowska, M., Koc, A., Luszczykiewicz, G., Ksiezopolska-Pietrzak, K., Klimczak, E., Chwalinska-Sadowska, H., et al. (2000). High levels of IL-17 in rheumatoid arthritis patients: IL-15 triggers in vitro IL-17 production via cyclosporin A-sensitive mechanism. J. Immunol. 164 (5), 2832–2838. doi: 10.4049/jimmunol.164.5.2832
Keywords: TH17 cells, TReg cells, TH17/TReg balance, liver, autoimmune diseases, viral infection
Citation: Drescher HK, Bartsch LM, Weiskirchen S and Weiskirchen R (2020) Intrahepatic TH17/TReg Cells in Homeostasis and Disease—It’s All About the Balance. Front. Pharmacol. 11:588436. doi: 10.3389/fphar.2020.588436
Received: 28 July 2020; Accepted: 15 September 2020;
Published: 02 October 2020.
Edited by:
Stefano Fiorucci, University of Perugia, ItalyReviewed by:
Michele Biagioli, University of Perugia, ItalyJae-Sung Kim, Washington University in St. Louis, United States
Copyright © 2020 Drescher, Bartsch, Weiskirchen and Weiskirchen. This is an open-access article distributed under the terms of the Creative Commons Attribution License (CC BY). The use, distribution or reproduction in other forums is permitted, provided the original author(s) and the copyright owner(s) are credited and that the original publication in this journal is cited, in accordance with accepted academic practice. No use, distribution or reproduction is permitted which does not comply with these terms.
*Correspondence: Hannah K. Drescher, aGRyZXNjaGVyQG1naC5oYXJ2YXJkLmVkdQ==; Lea M. Bartsch, bGJhcnRzY2gxQG1naC5oYXJ2YXJkLmVkdQ==
†These authors have contributed equally to this work