- Department of Internal Medicine, Xiang’an Hospital of Xiamen University, Xiamen, China
Uric acid (UA) is the end product of purine nucleotide metabolism in the human body. Hyperuricemia is an abnormally high level of UA in the blood and may result in arthritis and gout. The prevalence of hyperuricemia has been increasing globally. Epidemiological studies have shown that UA levels are positively correlated with cardiovascular diseases, including hypertension, atherosclerosis, atrial fibrillation (AF), and heart failure (HF). Hyperuricemia promotes the occurrence and development of cardiovascular diseases by regulating molecular signals, such as inflammatory response, oxidative stress, insulin resistance/diabetes, endoplasmic reticulum stress, and endothelial dysfunction. Despite extensive research, the underlying molecular mechanisms are still unclear. Allopurinol, a xanthine oxidase (XO) inhibitor, has been shown to improve cardiovascular outcomes in patients with HF, coronary heart disease (CHD), type 2 diabetes (T2D), and left ventricular hypertrophy (LVH). Whether febuxostat, another XO inhibitor, can improve cardiovascular outcomes as well as allopurinol remains controversial. Furthermore, it is also not clear whether UA-lowering treatment (ULT) can benefit patients with asymptomatic hyperuricemia. In this review, we focus on the latest cellular and molecular findings of cardiovascular disease associated with hyperuricemia and clinical data about the efficacy of ULT in patients with cardiovascular disease.
Introduction
Uric acid (UA) is the end product of purine metabolism in higher animals, such as humans and great apes. Under physiological conditions, UA synthesis and excretion are balanced in the body. Once this balance is disturbed, it leads to hyperuricemia. Normally, male UA levels greater than 7 mg/dL or female UA levels greater than 6 mg/dL are considered to be hyperuricemia (Hao et al., 2019). However, Virdis et al. confirmed that the threshold of UA level increased total mortality (4.7 mg/dL) and cardiovascular mortality (5.6 mg/dL) risk, which was significantly lower than clinical diagnostic criteria (Virdis et al., 2020). With increasingly unhealthy lifestyles, the incidence of hyperuricemia is increasing, and it has become the “fourth highest” after hypertension, hyperglycemia, and hyperlipidemia. It is estimated that the total number of patients with hyperuricemia was 170 million in China (Hao et al., 2019) and 32.5 million in the United States (Singh et al., 2019). Large-scale clinical studies on the relationship between serum uric acid (sUA) and cardiovascular diseases started from the Framingham Heart Study in the 1980s, the results of which indicate that UA does not have a causal role in the development of coronary heart disease (CHD), death from cardiovascular disease or all causes (Culleton et al., 1999). Recent epidemiological studies show that hyperuricemia may be involved in hypertension, diabetes, atherosclerosis, chronic kidney disease, and atrial fibrillation (AF) as well as the occurrence of cardiovascular events (Kuwabara et al., 2017a; Kuwabara et al., 2017b; Kuwabara et al., 2017c; Kuwabara et al., 2018a; Kuwabara et al., 2018b; Maruhashi et al., 2018). Experimental studies show that hyperuricemia promotes the occurrence and development of cardiovascular diseases by regulating molecular signals, such as inflammatory response (Xiao et al., 2015; Johnson et al., 2018; Lu et al., 2019), oxidative stress (Li et al., 2018), insulin resistance (Zhi et al., 2016), endothelial dysfunction (Maruhashi et al., 2018), and endoplasmic reticulum stress (Li P. et al., 2016; Yan et al., 2018).
An increasing number of clinical studies show that allopurinol can improve cardiovascular outcomes in patients with heart failure (HF), CHD, type 2 diabetes (T2D), and left ventricular hypertrophy (LVH) (Doehner et al., 2002; Farquharson et al., 2002; George et al., 2006; Noman et al., 2010; Rekhraj et al., 2013; Szwejkowski et al., 2013). Compared to allopurinol, whether febuxostat improves cardiovascular outcomes remains controversial (White et al., 2018; Zhang et al., 2018; Chen et al., 2019). Furthermore, whether UA-lowering treatment (ULT) is beneficial to patients with asymptomatic hyperuricemia is uncertain because these patients are often associated with a variety of risk factors (such as old age, chronic kidney disease, cardiovascular disease, obesity, metabolic syndrome, alcohol, or smoking habits, etc.) but without identified diseases. In addition, UA is an effective antioxidant in cardiovascular and neurodegenerative diseases (Huang et al., 2017), making the relationship between UA and cardiovascular disease more complicated.
This review focuses on the underlying mechanisms of cardiovascular disease associated with hyperuricemia and the efficacy of ULT in patients with cardiovascular disease.
UA and its Related Molecular Mechanism
UA and Oxidative Stress
UA is the final product of dietary purines. Existing evidence suggests that UA has a dual-face role in some cardiovascular and cerebrovascular diseases. On the one hand, UA has antioxidant activity and can scavenge reactive oxygen species (ROS). As one of the main endogenous antioxidants in the human body, UA contributes up to 60% of the plasma antioxidant capacity, which can protect cells from oxidative stress (Ames et al., 1981; Sautin and Johnson, 2008). The molecular mechanism of the antioxidation of UA are as follows: 1. It reacts directly with hydroxyl radicals, peroxynitrite, nitric oxide, and hydrogen peroxide, etc., forming stable intermediates (Gersch et al., 2009); 2. it cooperates with superoxide dismutase to scavenge oxygen radicals (Waring et al., 2001); 3. it chelates with metal ions (Davies et al., 1986); and 4. it inhibits the peroxynitrite-induced protein nitrification, protein, and lipid peroxidation (Whiteman et al., 2002). On the other hand, UA can promote oxidation activity in cells, which may be related to oxygen free radicals produced by ROS. The pro-oxidation mechanisms of UA include 1. reducing the production of nitric oxide in arterial endothelial cells and inhibiting vasodilation (Papezikova et al., 2013); 2. inhibiting the synthesis of adiponectin in adipocytes; 3. damaging the tricarboxylic acid cycle and fatty acid β oxidation; 4. activating the renin-angiotensin system, stimulating the proliferation of vascular smooth muscle cells and the production of angiotensin II (Ang II) (Corry et al., 2008); and 5. generating a chronic inflammatory reaction (Inaba et al., 2013). The dual effects of UA on anti- and pro-oxidants may be closely related to the xanthine oxidase (XO) activity in circulation. Several studies have associated the involvement of XO activity, a source of UA and ROS, to pro-oxidative and pro-inflammatory effects during pathological conditions (Boban et al., 2014; Kushiyama et al., 2016; Klisic et al., 2018).
In recent years, a lot of progress has been made on the relationship between UA and oxidative stress and its molecular mechanism. The physiological concentration of UA decreased oxidative stress–induced malondialdehyde and protein carbonyl contents, promoted superoxide dismutase (SOD) activity, and inhibited the formation of ROS in chicken embryo cardiomyocytes (Sun et al., 2017). The underlying mechanism was the NF-E2-related factor 2 (Nrf2) signal pathway (Sun et al., 2017). In contrast, treated with a high concentration of UA (1200 µM), the Nrf2 signaling pathway was inhibited, malondialdehyde and protein carbonyl contents were increased, and SOD activity was decreased (Figure 1A) (Sun et al., 2017). Our research team found that high UA inhibited the viability of H9c2 cardiomyocytes and increased the production of ROS (Li et al., 2018). Pretreatment with ROS scavenger (N-acetyl-L-cysteine) and extracellular signal-regulated kinase (ERK) inhibitor (PD98059) reversed the decrease of cell viability induced by high UA (Li et al., 2018). Further studies show that high UA-induced ROS may be closely related to ERK/p38 activation and phosphatidylinositol 3-kinase (PI3K)/Akt inhibition (Figure 1A) (Li et al., 2018). In addition, UA has a neuroprotective effect on dopaminergic neurons in Parkinson's disease mice, which may be related to Nrf2-ARE signal activation, reduction of oxidative damage, and neuroinflammation (Huang et al., 2017).
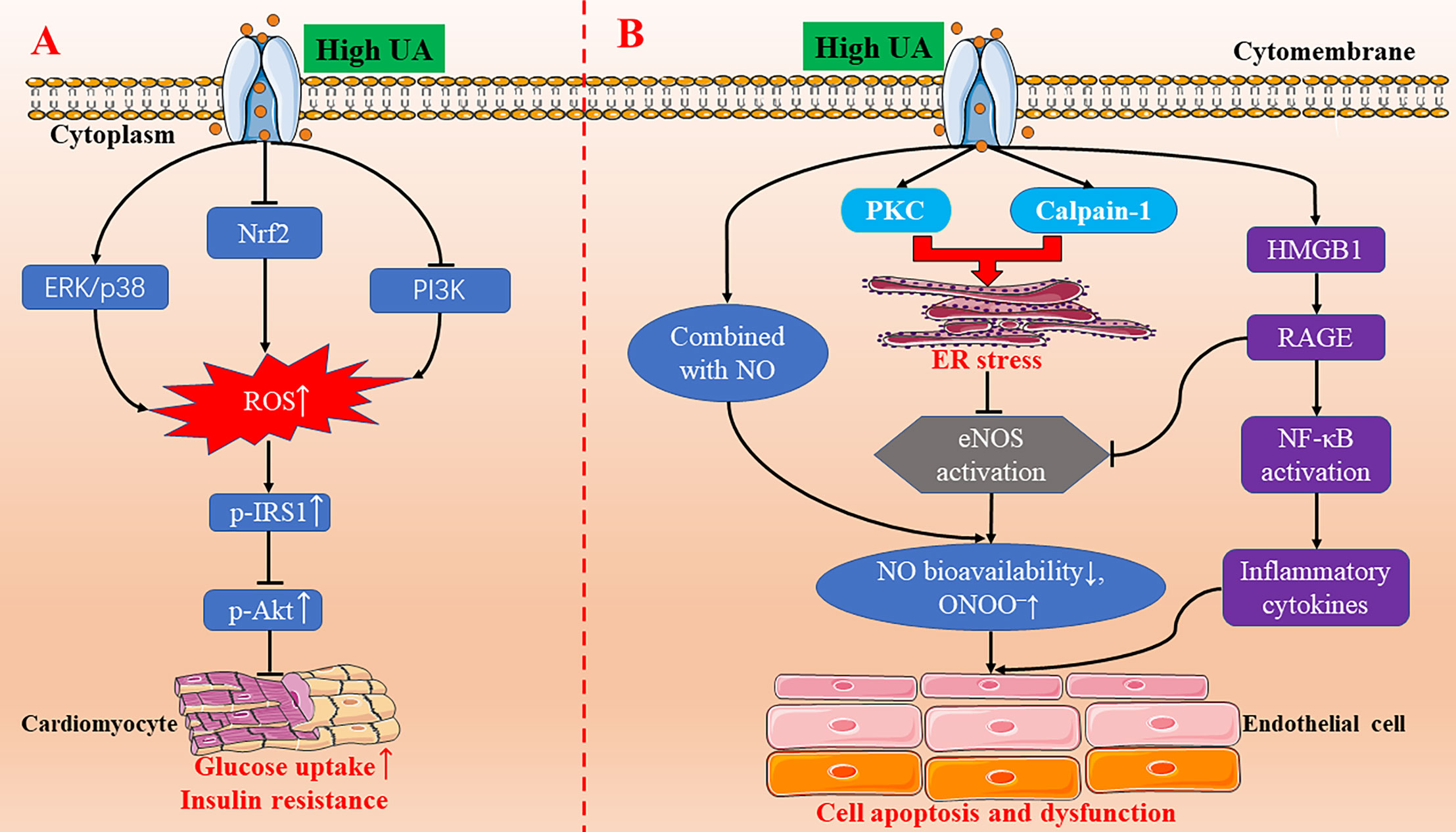
Figure 1 The molecular mechanisms of high UA-inducing oxidative stress, insulin resistance, endoplasmic reticulum stress, and endothelial dysfunction. (A) High UA activating the ERK/p38 signal pathway and inhibiting the Nrf2 and PI3K/Akt signal pathway, leading to an increase in the production of ROS. Increased ROS triggers IRS1/Akt phosphorylation to induce insulin resistance in cardiomyocytes. (B) High UA induces endothelial cell apoptosis and endothelial dysfunction through endoplasmic reticulum stress and the HMGB1/RAGE pathway. eNOS, endothelial nitric oxide synthase; ER, endoplasmic reticulum; ERK, extracellular signal-regulated kinase; HMGB1, high mobility group box chromosomal protein 1; NF-κB, nuclear factor κB; NO, nitric oxide; Nrf2, NF-E2-related factor 2; p-AKT, phospho-Akt; PI3K, phosphatidylinositol 3-kinase; p-IRS1, phospho-insulin receptor substrate 1; PKC, protein kinase C; RAGE, receptor for advanced glycation end products; ROS, reactive oxygen species; UA, uric acid.
In short, previous studies have confirmed that UA has both antioxidant and pro-oxidant effects in vivo. This dual effect of UA has also been confirmed in cardiomyocytes. The latest experimental studies demonstrate that the antioxidation of UA may be involved in regulating the Nrf2-ARE, ERK/p38, and PI3K/Akt signaling pathways.
UA and Inflammatory Response
UA has been a focus of research not only for its role in oxidative stress, but also for its relation to various inflammatory diseases. Atherosclerosis is a chronic immuno-inflammatory cardiovascular disease. A large body of evidence suggests that elevated UA levels are strongly associated with the occurrence and development of atherosclerosis. High intracellular UA concentrations promote the expression of inflammatory markers, such as nuclear factor κB (NF-κB), growth factors, vasoconstrictive substances (Ang II, thromboxane, and endothelin-1), and chemokines via activating mitogen-activated protein kinases (MAPK) (Figure 2) (Xiao et al., 2015; Johnson et al., 2018). Additionally, hyperuricemia promoted macrophage M1/M2 polarization, which could be reversed by ULT (Jia et al., 2015). In the development of obesity and cardiorenal disease, UA tends to enhance the pro-inflammatory response of M1 and inhibit the anti-inflammatory response of M2 (Aroor et al., 2013). M1 macrophages secrete inflammatory cytokines, leading to insulin resistance and cardiac dysfunction (Aroor et al., 2013). On the contrary, M2 macrophages secrete interleukin-10 (IL-10), which inhibits cardiomyocyte hypertrophy and myocardial fibrosis (Figure 2) (Bene et al., 2014). Furthermore, in a randomized, open, parallel-controlled study involving 176 patients with T2D and asymptomatic hyperuricemia, allopurinol effectively lowered sUA, improved insulin resistance, reduced serum high-sensitivity C-reactive protein (hs-CRP) level, decreased carotid intima-media thickness, and ameliorated the exacerbation of atherosclerosis (Liu et al., 2015).
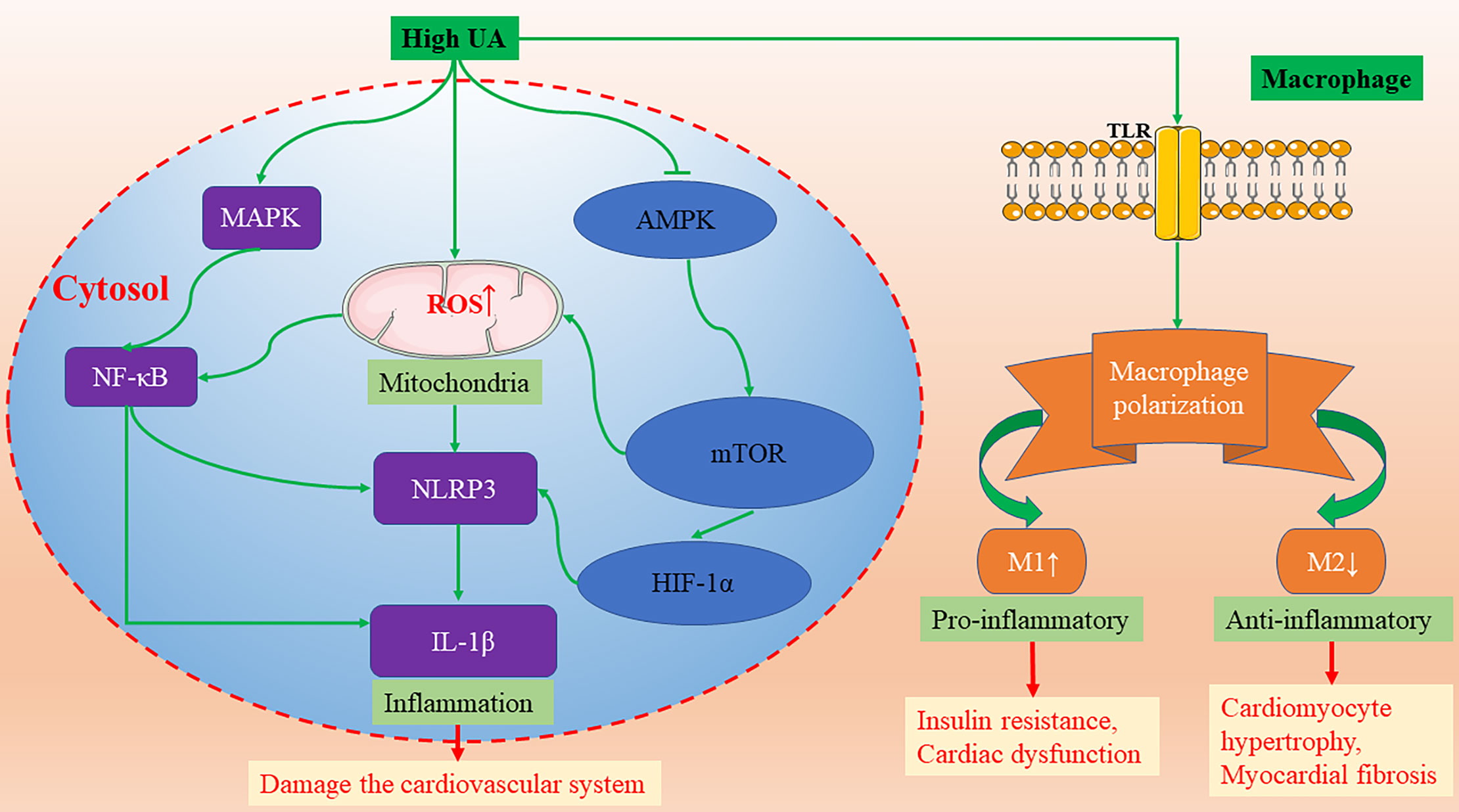
Figure 2 The molecular mechanisms of high UA activating NLRP3-inflammasomes and promoting macrophage M1/M2 polarization. AMPK, AMP-activated protein kinase; HIF-1α, hypoxia-inducible factor-1α; IL-1β, interleukin-1β; MAPK, mitogen activated protein kinases; mTOR, mammalian target of rapamycin; NF-κB, nuclear factor κB; NLRP3, nod-like receptor protein 3; TLR, Toll-like receptors; UA, uric acid.
The activation of inflammasomes plays a critical role in a variety of chronic inflammatory diseases. Recently, many studies have found that sUA can effectively activate inflammasomes in the cardiovascular system. The continuous activation of inflammasomes aggravates the inflammatory response and damages the cardiovascular system. Studies by Wang et al. report that UA can activate nod-like receptor protein 3 (NLRP3) inflammasomes and damage mitochondria, resulting in cellular damage of H9c2 cells (Wang et al., 2018). In addition, several studies also show that UA activates the NLRP3 inflammasome and induces interleukin-1β (IL-1β) release in a variety of cells, including monocytes, macrophages, vascular smooth muscle cells, and endothelial cells (Martinon et al., 2006; Matias et al., 2015; Alberts et al., 2019; Kim et al., 2019; Li et al., 2019; Yin et al., 2019). The molecular mechanism of UA-induced NLRP3/IL-1 β activation is through NF-κB activation and mitochondrial ROS (mROS) (Figure 2). Consistent with the latest research published by Kimura et al., UA promotes the secretion of IL-1β mediated by NLRP3 inflammasomes via regulating the AMP-activated protein kinase (AMPK)-mammalian target of rapamycin (mTOR) mROS and hypoxia-inducible factor-1α (HIF-1α) pathway in human peripheral blood mononuclear cells (Figure 2) (Kimura et al., 2020). In mice treated with uricase gene transfer and XO inhibitor, however, the decrease of UA level promoted the activation of AMPK, and the formation of atherosclerotic plaque was inhibited (Kimura et al., 2020).
In conclusion, UA has been shown to promote inflammation in a variety of cells. Hyperuricemia can promote the development of atherosclerosis by regulating inflammatory signal pathways, such as NLRP3-inflammasomes, macrophage M1/M2 polarization, and hs-CRP, and ULT can significantly reverse the formation of atherosclerotic plaques. Inhibition of UA-induced NLRP3 inflammasome activation may be a new therapeutic target for atherosclerosis.
UA and Insulin Resistance/Diabetes
Insulin resistance is closely related to diabetes, obesity, hyperlipidemia, hypertension, hyperuricemia, and other metabolic disorders. Current studies confirm that oxidative stress and inflammation may be the pathophysiological basis of insulin resistance. Hyperuricemia can promote oxidative stress in many cell lines. The rise of ROS level can induce insulin resistance. Oxidative stress may be the cause of insulin resistance–related cardiovascular complications because overgenerated ROS and insulin resistance may lead to cardiac dysfunction (Ritchie, 2009). Our team's research shows that high UA can increase ROS production and inhibit insulin-induced glucose uptake in H9c2 and primary cardiomyocytes, and N-acetyl-L-cysteine pretreatment can reverse the inhibitory effect of high UA on glucose uptake (Zhi et al., 2016). The molecular mechanism may be that high UA increases the phosphorylation of insulin receptor substrate 1 (IRS1) and inhibits the phosphorylation of Akt, which was blocked by N-acetyl-L-cysteine (Figure 1A) (Zhi et al., 2016). Hence, high UA can induce insulin resistance in cardiomyocytes in vitro and in vivo.
Clinical studies have shown a link between hyperuricemia and diabetes (Kodama et al., 2009; van der Schaft et al., 2017); however, it is controversial whether hyperuricemia plays a causal role in diabetes. Recently, Lu et al. confirmed that there is no causal relationship between hyperuricemia and diabetes (Lu et al., 2020). High UA accelerates but does not cause diabetes because UA itself is insufficient to induce diabetes although it can damage glucose tolerance, leading to insulin resistance (Lu et al., 2020). Although the mechanism of high UA–induced myocardial insulin resistance has not been fully elucidated, it might be a novel potential mechanism of hyperuricemic-related cardiovascular disease.
UA and Endoplasmic Reticulum Stress
Cardiomyocyte apoptosis is one of the pathogeneses of myocardial anatomical reconstruction. Oxidative stress and endoplasmic reticulum stress are the key factors promoting apoptosis, which are involved in the pathogenesis of many diseases, including cardiovascular diseases. UA triggers oxidative stress and endoplasmic reticulum stress to signal the network to induce endothelial dysfunction via activating the protein kinase C (PKC) pathway in human umbilical vein endothelial cells (HUVECs) (Li P. et al., 2016). The latest research also demonstrates that UA can induce cardiomyocyte apoptosis in vitro and in vivo, and its molecular mechanism might be through the activation of calpain-1 and the endoplasmic reticulum stress signaling pathway (Figure 1B) (Yan et al., 2018). So far, the studies on UA-induced endoplasmic reticulum stress is scarce, but it is certain that there will be more research to clarify the relationship between the two in the future.
UA and Endothelial Dysfunction
Endothelial cells secrete a variety of vasoactive substances, including vasodilators (nitric oxide, prostaglandin I2, endothelium-derived hyperpolarizing factor, etc.) and vasoconstrictors (endothelin-1, thrombin A2, Ang II, etc.) (Vane et al., 1990; Maruhashi et al., 2018). Vasodilator nitric oxide plays a key role in the development of atherosclerosis. The increase of UA level in cells directly combined with nitric oxide, which resulted in the decrease of nitric oxide bioavailability and the increase of peroxynitrite (ONOO–) (Maruhashi et al., 2018). ONOO– is a strong oxidant, which can cause DNA damage, cell death, and lipid peroxidation (Maruhashi et al., 2018). In addition, Li et al. found that high UA-induced HUVEC apoptosis and endothelial dysfunction is through PKC-dependent endothelial nitric oxide synthase (eNOS) phosphorylation and endoplasmic reticulum stress, reducing eNOS activity and nitric oxide production (Li P. et al., 2016). Furthermore, a recent study also indicates that high UA inhibits eNOS expression and nitric oxide production in HUVECs, increases high mobility group box chromosomal protein 1 (HMGB1)/receptor for advanced glycation end products (RAGE) expression, activated NF-κB, and increased the inflammatory cytokine levels (Cai et al., 2017). All these results provide new insight into the mechanisms of UA-induced endothelial dysfunction (Figure 1B).
Endothelial dysfunction plays a critical role in the development and progression of atherosclerosis, leading to serious cardiovascular events. Experimental studies show that high UA aggravates the inflammation response and oxidative stress and, thus, leads to endothelial dysfunction (Xiao et al., 2015; Johnson et al., 2018; Li et al., 2018; Lu et al., 2019). Additionally, hyperuricemia-induced endothelial dysfunction is also confirmed in many clinical studies (Mercuro et al., 2004; Kato et al., 2005; Ho et al., 2010; Tomiyama et al., 2011; Sincer et al., 2014). All these experiments and clinical studies show that UA is not only a biomarker of cardiovascular risk, but also a causal risk factor of endothelial dysfunction (Katsiki and Mikhailidis, 2015). Notably, Borgi et al. found that ULT was not associated with endothelial dysfunction improvement in nonhypertensive, overweight/obese individuals (Borgi et al., 2017). Therefore, the hypothesis of the causal relationship between UA and endothelial dysfunction needs further study.
UA and Cardiovascular Disease
UA and Hypertension
Elevated sUA is strongly associated with hypertension, but the mechanism is not clear. Hyperinsulinemia induced by insulin resistance enhances renal sodium reabsorption, which may lead to hypertension (Forman et al., 2009; Battelli et al., 2018). Endothelial dysfunction caused by oxidative stress also plays a key role in the development of hypertension, kidney disease, and cardiovascular disease (Mortada, 2017). Studies have shown that UA significantly increased the production of ROS and Ang II in human endothelial cells (Yu et al., 2010).
In recent years, numerous studies have demonstrated the link between sUA and hypertension. In a prospective randomized study of 5748 healthy adolescents, a 10-year follow-up showed that elevated sUA was closely associated with hypertension and metabolic syndrome (Sun et al., 2015) Recently, a retrospective cohort study of 3584 prehypertensive patients also showed that increased sUA was a strong risk marker for developing hypertension from prehypertension (Kuwabara et al., 2018b). In another large-scale meta-analysis of 55,607 subjects in 18 prospective cohort studies, it was found that, for every 1 mg/dl increase in sUA, the incidence of hypertension increased by 13% (Grayson et al., 2011). This result is consistent with the result recently published by Bjornstad et al. that higher baseline sUA independently increased the risk of incident hypertension (hazard ratio 1.19, per 1 mg/dL increase in sUA) (Bjornstad et al., 2019). Arterial stiffness and inflammation may be involved in the risk of development of hypertension associated with hyperuricemia (Tomiyama et al., 2018), but this result is inconsistent with Cicero et al., who show that sUA is significantly correlated to hypertension and carotid intima–media thickness but not to aortic stiffness (Cicero et al., 2014). Interestingly, recently published experimental studies do not seem to be consistent with the above epidemiological and clinical studies. Chen et al. show that UA level is not positively correlated with blood pressure (BP) in people with early Parkinson's disease (Chen et al., 2018). In addition, the increase of UA level was also not related to the change of BP, cardiac morphology, and heart dysfunction in a Uricase/Uox knockout male mouse model (Chen et al., 2018).
Likewise, this close relationship between UA and hypertension also exists in pregnant women. The level of sUA in pregnant women is higher than that in normal, nonpregnant women, and the incidence of hypertensive disorders of pregnancy is significantly increased in pregnant women with hyperuricemia (Sakr et al., 2020). The mechanisms of increased UA level during pregnancy may be related to the decrease of estrogen concentration, the increase of high purine food intake, and the increase of XO activity. Increased UA level strongly correlated to the severity of preeclampsia and higher risk of gout in later life (Wang I. K. et al., 2016; Khaliq et al., 2018).
In conclusion, sUA is closely correlated with BP, but a consistent effect has not been observed in ananimal model and human studies. Fortunately, the recent published results by Johnson et al. give us an answer. After treatment with pegloticase, sUA remained at a low level in hyperuricemia patients, and its systolic and diastolic BP decreased significantly, which was independent of changes in renal function (Johnson et al., 2019). These findings give us reasons to believe that there may be a causal link between hyperuricemia and hypertension (Figure 3A).
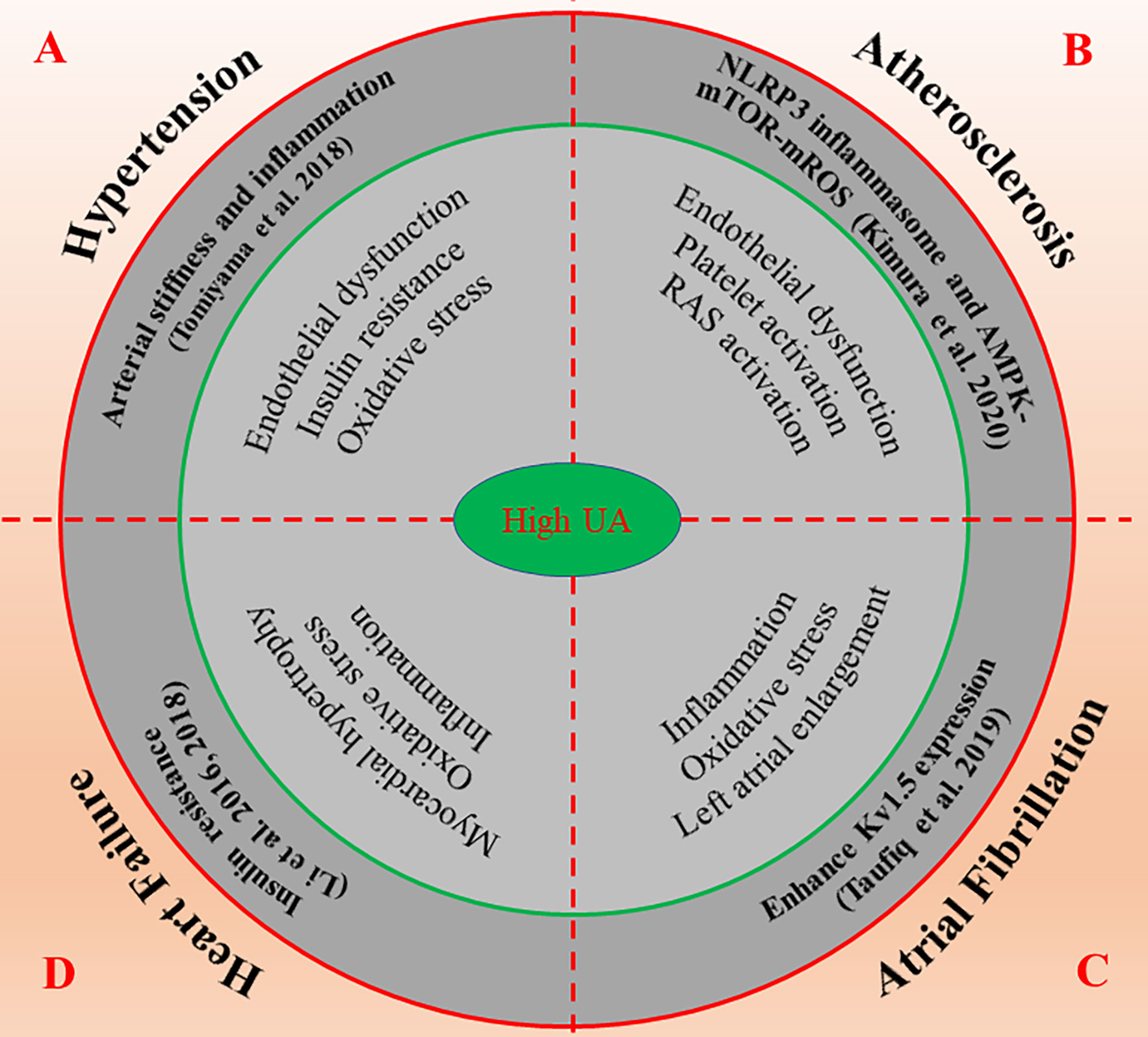
Figure 3 The possible molecular mechanisms of high UA promote the occurrence and development of cardiovascular diseases. High UA regulates numerous molecular signals such as inflammation, oxidative stress, insulin resistance, and endothelial dysfunction, thus affects the progression and prognosis of cardiovascular diseases including hypertension (A), atherosclerosis (B), atrial fibrillation (C) and heart failure (D). AMPK, AMP-activated protein kinase; mROS, mitochondrial ROS; mTOR, mammalian target of rapamycin; NLRP3, nod-like receptor protein 3; RAS, renin-angiotensin system; UA, uric acid.
UA and CHD
Atherosclerosis is the most common cardiovascular disease with the highest morbidity and mortality. The possible mechanisms of UA induced CHD may include the following (Wu et al., 2012): 1. Vascular endothelial damage of large and micro vessels: urate is easy to deposit in the vascular wall and stimulate the proliferation of vascular smooth muscle cells; high UA can activate the renin-angiotensin system, inhibit nitric oxide production, and induce endothelial cell dysfunction (Corry et al., 2008; Sautin and Johnson, 2008; Papezikova et al., 2013). 2. UA can cause platelet activation, adhesion, and aggregation. 3. Hyperuricemia participates in the production of many inflammatory mediators (such as interleukin, C-reactive protein, etc.) (Inaba et al., 2013). 4. Hyperuricemia can increase the production of oxygen free radicals, which can cause low-density lipoprotein peroxidation, damage endothelial cells, promote vascular smooth muscle and intimal hyperplasia, etc. (Whiteman et al., 2002). 5. High UA can directly cause low-density lipoprotein oxidation (Wu et al., 2012).
A prospective cohort study of 457,915 subjects without cardiovascular disease found that UA concentrations >7.0 mg/dl significantly increased the risk of CHD in the general population (mainly adult women) (Braga et al., 2016). Another study suggested that sUA > 8 mg/dl was independently associated with three vessel diseases: coronary artery, HF, and LV enlargement in Chinese patients with early-onset CHD (Dai et al., 2015). In a meta-analysis of 12,677 subjects, elevated sUA level was found to be significantly associated with poor prognosis (all-cause mortality, cardiovascular mortality, and hospitalization for HF) in patients with LV dysfunction, HF, or both after myocardial infarction (MI) (von Lueder et al., 2015). sUA can be used as a low-cost and widely used biomarker to evaluate the clinical risk stratification of patients with MI. According to a systematic review of 29 prospective cohort studies involving 958,410 subjects, for every 1 mg/dl increase in sUA level, the mortality rate of CHD was 1.02 in men and 2.44 in women (Li M. et al., 2016). This result is consistent with the result published by Wang et al. that increased sUA levels are closely related to the risk of cardiovascular and all-cause mortality in people with suspected or definite CHD (Wang R. et al., 2016). For every 1 mg/dl increase in sUA, cardiovascular and all-cause mortality increased by 12% and 20%, respectively (Wang R. et al., 2016).
Although many clinical studies confirm that elevated sUA levels are strongly and independently associated with the occurrence and development of coronary atherosclerosis, the severity of coronary artery lesions and cardiovascular and all-cause mortality, the molecular mechanism of UA promoting atherosclerosis is still unclear. Plaque morphology, endothelial dysfunction, oxidative stress, and inflammation play a critical role in the development and progression of atherosclerosis, leading to serious cardiovascular events (Prasad et al., 2017). Optical coherence tomography showed a significant increase in plaque rupture in patients with sUA > 8.0 mg/dl (Kobayashi et al., 2018). Recently, Kimura et al. demonstrated that UA promotes the secretion of IL-1β mediated by NLRP3 inflammasomes via regulating the AMPK-mTOR mROS and HIF-1α pathway in human peripheral blood mononuclear cells (Kimura et al., 2020). In mice treated with uricase gene transfer and XO inhibitor, however, the decrease of UA level promoted the activation of AMPK and inhibited the formation of atherosclerotic plaque (Kimura et al., 2020). In addition, the coronary artery calcium (CAC) score can reflect total atherosclerotic burden, which is another effective index to predict future CVD events. Jun et al. evaluated the correlation between CAC and sUA in 9297 subjects by multidetector CT (Jun et al., 2018). The results show that sUA level is an independent predictor for development of moderate CAC in generally healthy adults (Jun et al., 2018).
In brief, hyperuricemia is a potential risk factor for CHD. The elevated sUA may lead to endothelial dysfunction through inflammation and oxidative stress and the formation of unstable lipid plaque in the coronary artery, which eventually leads to the occurrence of atherosclerosis. Therefore, lowering the level of UA is crucial in the prevention and treatment of CHD (Figure 3B).
UA and AF
AF is the most common heart rhythm disorder, and its major risk factors include advanced age, hypertension, obesity, diabetes, HF, valvular heart disease, and MI. In recent years, numerous clinical studies have confirmed that hyperuricemia is associated with AF after adjusting for various cardiovascular risk factors, and the correlation is stronger in women than in men (Liang et al., 2016; Zhang et al., 2016; Chen et al., 2017; Kuwabara et al., 2017c; Mantovani et al., 2018; Pak et al., 2018; Black-Maier and Daubert, 2019; Lin et al., 2019). The bases for sex-related differences in high UA and AF or CHD remains unknown. High UA has been associated with endothelial dysfunction in postmenopausal women, suggesting that high UA could be an independent risk factor for cardiovascular disease, including AF and CHD, particularly among postmenopausal women (Maruhashi et al., 2013; Lin et al., 2019).
In addition, sUA was also an independent risk factor of LA thrombus in patients with nonvalvular AF or with mitral stenosis in sinus rhythm (Tang et al., 2014; Ozturk et al., 2015). UA level is negatively correlated with the systolic function of the lower left atrial appendage (LAA) (Celik et al., 2015). It can provide prognostic information for long-term thromboembolic events in patients with AF (Celik et al., 2015). There are also studies suggesting that hyperuricemia is associated with an increased risk of AF recurrence after catheter ablation (Canpolat et al., 2014). In a recent meta-analysis involving 1298 patients with AF, however, elevated sUA was not associated with the risk of AF recurrence after catheter ablation (Zhao et al., 2016).
The mechanisms of high UA-promoted AF and thrombosis are not clear. A possible explanation for this association is that high UA induces oxidative stress and inflammation. Moreover, hyperuricemia was independently associated with increased left atrial diameter (Tamariz et al., 2011; Black-Maier and Daubert, 2019). Left atrial enlargement is closely related to the occurrence of AF and thrombosis. Furthermore, UA increases Kv1.5 protein expression by activating ERK and oxidative stress in mouse atrial myocytes (HL-1 cells) so as to enhance the ultra-rapid delayed-rectifier K+ channel currents and shorten the action potential duration (Maharani et al., 2015). Additionally, the molecular mechanism of the UA-induced enhancement of Kv1.5 expression also may be attributed to enhanced phosphorylation of Akt and heat shock factor 1 (HSF1), which leads to increased expressions of heat shock protein 70 (Hsp70) (Taufiq et al., 2019).
Although the relationship between hyperuricemia and AF has been widely recognized, the mechanism of occurrence/maintenance of AF caused by hyperuricemia has not been fully elucidated. The available evidence suggests that the UA-induced enhancement of Kv1.5 expression may be a novel mechanism. However, whether intervening can reduce the risk of AF remains unclear. It is clear that prospective intervention studies are needed in the future to demonstrate whether reducing the level of sUA is important for the prevention of AF (Figure 3C).
UA and HF
HF is the end stage of most cardiovascular diseases, which is closely related to hypertension, MI, AF, and valvular heart disease. UA may play an important role in HF. A large number of studies (Vaduganathan et al., 2014; Palazzuoli et al., 2017; Pavlusova et al., 2019) and meta-analyses (Huang et al., 2014) have evaluated the relationship between sUA and the risk and adverse outcome of HF. The results indicate that the increase of sUA may be an important risk factor for the incidence and prognosis of HF (Huang et al., 2014; Vaduganathan et al., 2014; Palazzuoli et al., 2017; Pavlusova et al., 2019).
However, the mechanism of hyperuricemia-induced HF and its prognosis is not clear. Inflammation and oxidative stress play key roles in the development and progression of HF. XO is the key enzyme responsible for conversion of purine bases to UA and represents the major source of ROS production in circulation (Boban et al., 2014; Klisic et al., 2018). Therefore, the overactivation of XO may be important for the increase of mortality and hospitalization of patients with high UA and HF. Jia et al. found that the western diet resulted in increased sUA, cardiomyocyte hypertrophy, myocardial oxidative stress, myocardial fibrosis, and diastolic dysfunction (Jia et al., 2015). Further studies found that it may be related to the western diet enhancing the activation of S6 kinase-1 growth pathway and transforming growth factor-β1/Smad2/3 signal pathway and macrophage polarization (Jia et al., 2015). The latest research found that Vericiguat, a stimulator of soluble guanylate cyclase, treatment for 12 weeks, significantly decreases in hs-CRP and sUA in HF patients with reduced ejection fraction (Kramer et al., 2020). In addition, our research team also found that high UA stimulates ROS production, inhibits insulin-induced glucose uptake, and thus, leads to myocardial insulin resistance in H9c2 and primary cultured cardiomyocytes (Zhi et al., 2016; Li et al., 2018). Insulin resistance can inhibit the uptake of myocardial glucose, impairing lipid metabolism, leading to myocardial energy metabolism disorder, and thus, affecting the diastolic and contractile function of myocardium. Therefore, high UA-induced myocardial insulin resistance may be an important pathological mechanism of HF. Further study on the molecular mechanism of high UA-induced myocardial insulin resistance may be a novel target for intervention of hyperuricemic-related cardiovascular diseases.
Taken together, the increase of sUA is closely related to the occurrence and prognosis of HF. Inflammation and oxidative stress play key roles in the development and progression of HF. We suggest for the first time that high UA-induced insulin resistance may be an important pathological mechanism of HF. The relationship between high UA-induced myocardial insulin resistance and HF and whether ULT can improve the clinical outcome of patients with HF need to be proved in future studies (Figure 3D).
Urate-lowering Treatments (ULT)
Currently, there are two main classes of ULT drugs commonly used in clinical practice: those inhibiting UA synthesis (XO inhibitors, such as allopurinol, febuxostat, etc.) and increasing UA excretion (e.g., benzbromarone, probenecid, etc.). Current studies confirm that ULT has a good effect in young hypertensive patients, but the effect on CHD, AF, and HF has not achieved satisfactory clinical results. In terms of drug selection, previous studies have suggested that febuxostat is more effective and safer than allopurinol. Recent studies have shown that ULT made no difference in the occurrence of primary end-point events (composite of cardiovascular death, nonfatal MI, nonfatal stroke, or unstable angina with urgent revascularization). However, cardiovascular death appeared to be higher in the febuxostat group compared to the allopurinol group (White et al., 2018). Therefore, febuxostat is neither recommended as the first-line ULT in the latest gout management guidelines nor for the treatment of asymptomatic hyperuricemia. Numerous experimental and clinical studies have confirmed that allopurinol can reduce the incidence of all-cause death, MI, and congestive HF in patients with hyperuricemia (Struthers et al., 2002; MacIsaac et al., 2016; Singh et al., 2017; Nidorf and Jelinek, 2018; Singh and Cleveland, 2018). For drugs promoting the excretion of UA, benzbromarone is superior to probenecid in efficacy and safety. Urate transporter 1 and organic-anion transporter 4 inhibitors, new drugs promoting the excretion of UA, are in the clinical trial stage.
Notably, sodium glucose cotransporter 2 inhibitors (SGLT-2; dapagliflozin, empagliflozin, canagliflozin, etc.) can effectively reduce sUA level by accelerating the excretion of UA (Wilcox et al., 2018; Zhao et al., 2018; Xin et al., 2019). Dapagliflozin, the earliest SGLT-2 inhibitor, reduced the level of UA in a dose-dependent manner. Compared to placebo, dapagliflozin 2.5/5/10 mg can decrease serum UA by 30.9/36.3/47.6 μmol/L, respectively (Bailey et al., 2010). The UA-lowering effect of SGLT-2 inhibitors can be attributed to glucose transporter-9 (GLUT-9) isoform 2. SGLT-2 inhibitors increased glucose concentration in renal tubules, activated GLUT-9 in proximal tubules, promoted glucose transport to cells, and excreted UA, and high glucose in collecting tubules inhibited the reabsorption of UA, both of which increased the excretion of UA. Recently, many clinical studies and meta-analyses confirmed that SGLT-2 inhibitors can reduce main cardiovascular adverse events, HF admission rate, and all-cause mortality in patients with hyperuricemia (Zinman et al., 2015; Neal et al., 2017; Wilcox et al., 2018; Zhao et al., 2018; McMurray et al., 2019; Wiviott et al., 2019; Xin et al., 2019). Therefore, SGLT-2 inhibitors are particularly suitable for reducing the risk of cardiovascular death in patients with T2D with hyperuricemia.
Conclusions and Perspectives
Collectively, the relationship between hyperuricemia and cardiovascular disease is becoming clearer, which is attributed to the study progress of UA. First, Tomiyama et al. found that hyperuricemia increased arterial stiffness and inflammation, which may be involved in the risk of development of hypertension. Second, UA induces NLRP3 inflammasome-dependent inflammation activation via the AMPK-mTOR-mROS and HIF-1α pathways. In contrast, decrease of UA level promotes the activation of AMPK and inhibits the formation of atherosclerotic plaque. Furthermore, UA significantly enhances the expression of Kv1.5 protein and enhances Akt and HSF1 phosphorylation, leading to increased Hsp70 expression. This result suggests that inhibition of the Akt-HSF1-Hsp70 pathway may be a novel therapeutic approach against AF in patients with hyperuricemia. Last but not least, high UA induces insulin resistance in cardiomyocytes through the ROS-IRS1/Akt phosphorylation pathway. High UA-induced insulin resistance may be an important pathological mechanism of HF.
A large number of studies have shown that UA levels are positively correlated with hypertension, CHD, AF, and HF (Table 1); however, there was no satisfactory clinical outcome in the ULT of these cardiovascular diseases. Most clinical studies show that febuxostat improves cardiovascular outcomes in patients with gout, but whether it is as effective as allopurinol remains controversial. Allopurinol may increase the risk of serious adverse reactions, so it has not been widely used in clinical practice. Fortunately, SGLT-2 inhibitors (dapagliflozin, etc.) have a good prospect in ULT, meanwhile reducing main cardiovascular adverse events, HF admission rate, and all-cause mortality in patients with T2D and hyperuricemia. However, all of these need to be further confirmed by more large-scale clinical randomized controlled trials.
Author Contributions
WY initiated this review, read lots of literature, and wrote the manuscript. J-DC revised our first draft and provided valuable comments. All authors contributed to the article and approved the submitted version.
Funding
This work was supported by grants from the National Natural Science Foundation of China (81570772 and 81471081), the Natural Science Foundation of Fujian Province (2020J01010) and the Xiang’an Hospital of Xiamen University (PM201809170002).
Conflict of Interest
The authors declare that the research was conducted in the absence of any commercial or financial relationships that could be construed as a potential conflict of interest.
References
Alberts, B. M., Barber, J. S., Sacre, S. M., Davies, K. A., Ghezzi, P., Mullen, L. M. (2019). Precipitation of Soluble Uric Acid Is Necessary for In Vitro Activation of the NLRP3 Inflammasome in Primary Human Monocytes. J. Rheumatol. 46 (9), 1141–1150. doi: 10.3899/jrheum.180855
Ames, B. N., Cathcart, R., Schwiers, E., Hochstein, P. (1981). Uric acid provides an antioxidant defense in humans against oxidant- and radical-caused aging and cancer: a hypothesis. Proc. Natl. Acad. Sci. U.S.A. 78 (11), 6858–6862. doi: 10.1073/pnas.78.11.6858
Aroor, A. R., Demarco, V. G., Jia, G., Sun, Z., Nistala, R., Meininger, G. A., et al. (2013). The role of tissue Renin-Angiotensin-aldosterone system in the development of endothelial dysfunction and arterial stiffness. Front. Endocrinol. (Lausanne) 4, 161. doi: 10.3389/fendo.2013.00161
Bailey, C. J., Gross, J. L., Pieters, A., Bastien, A., List, J. F. (2010). Effect of dapagliflozin in patients with type 2 diabetes who have inadequate glycaemic control with metformin: a randomised, double-blind, placebo-controlled trial. Lancet 375 (9733), 2223–2233. doi: 10.1016/S0140-6736(10)60407-2
Battelli, M. G., Bortolotti, M., Polito, L., Bolognesi, A. (2018). The role of xanthine oxidoreductase and uric acid in metabolic syndrome. Biochim. Biophys. Acta Mol. Basis Dis. 1864 (8), 2557–2565. doi: 10.1016/j.bbadis.2018.05.003
Bene, N. C., Alcaide, P., Wortis, H. H., Jaffe, I. Z. (2014). Mineralocorticoid receptors in immune cells: emerging role in cardiovascular disease. Steroids 91, 38–45. doi: 10.1016/j.steroids.2014.04.005
Bjornstad, P., Laffel, L., Lynch, J., El Ghormli, L., Weinstock, R. S., Tollefsen, S. E., et al. (2019). Elevated Serum Uric Acid Is Associated With Greater Risk for Hypertension and Diabetic Kidney Diseases in Obese Adolescents With Type 2 Diabetes: An Observational Analysis From the Treatment Options for Type 2 Diabetes in Adolescents and Youth (TODAY) Study. Diabetes Care 42 (6), 1120–1128. doi: 10.2337/dc18-2147
Black-Maier, E., Daubert, J. P. (2019). Editorial Commentary: Prevention and treatment of atrial fibrillation: Is hyperuricemia the next target? Trends Cardiovasc. Med. 29 (1), 48–49. doi: 10.1016/j.tcm.2018.07.006
Boban, M., Kocic, G., Radenkovic, S., Pavlovic, R., Cvetkovic, T., Deljanin-Ilic, M., et al. (2014). Circulating purine compounds, uric acid, and xanthine oxidase/dehydrogenase relationship in essential hypertension and end stage renal disease. Ren. Fail 36 (4), 613–618. doi: 10.3109/0886022X.2014.882240
Borgi, L., McMullan, C., Wohlhueter, A., Curhan, G. C., Fisher, N. D., Forman, J. P. (2017). Effect of Uric Acid-Lowering Agents on Endothelial Function: A Randomized, Double-Blind, Placebo-Controlled Trial. Hypertension 69 (2), 243–248. doi: 10.1161/HYPERTENSIONAHA.116.08488
Braga, F., Pasqualetti, S., Ferraro, S., Panteghini, M. (2016). Hyperuricemia as risk factor for coronary heart disease incidence and mortality in the general population: a systematic review and meta-analysis. Clin. Chem. Lab. Med. 54 (1), 7–15. doi: 10.1515/cclm-2015-0523
Cai, W., Duan, X. M., Liu, Y., Yu, J., Tang, Y. L., Liu, Z. L., et al. (2017). Uric Acid Induces Endothelial Dysfunction by Activating the HMGB1/RAGE Signaling Pathway. BioMed. Res. Int. 2017:4391920. doi: 10.1155/2017/4391920
Canpolat, U., Aytemir, K., Yorgun, H., Sahiner, L., Kaya, E. B., Cay, S., et al. (2014). Usefulness of serum uric acid level to predict atrial fibrillation recurrence after cryoballoon-based catheter ablation. Europace 16 (12), 1731–1737. doi: 10.1093/europace/euu198
Celik, M., Yalcinkaya, E., Yuksel, U. C., Gokoglan, Y., Bugan, B., Kabul, H. K., et al. (2015). Increased serum uric acid levels are correlated with decreased left atrial appendage peak flow velocity in patients with atrial fibrillation. Med. Princ. Pract. 24 (3), 263–268. doi: 10.1159/000373892
Chen, Y., Xia, Y., Han, X., Yang, Y., Yin, X., Qiu, J., et al. (2017). Association between serum uric acid and atrial fibrillation: a cross-sectional community-based study in China. BMJ Open 7 (12), e019037. doi: 10.1136/bmjopen-2017-019037
Chen, X., Umeh, C. C., Tainsh, R. E., Feng, D. D., Maguire, M., Zuo, F., et al. (2018). Dissociation between urate and blood pressure in mice and in people with early Parkinson’s disease. EBioMedicine 37, 259–268. doi: 10.1016/j.ebiom.2018.10.039
Chen, C. H., Chen, C. B., Chang, C. J., Lin, Y. J., Wang, C. W., Chi, C. C., et al. (2019). Hypersensitivity and Cardiovascular Risks Related to Allopurinol and Febuxostat Therapy in Asians: A Population-Based Cohort Study and Meta-Analysis. Clin. Pharmacol. Ther. 106 (2), 391–401. doi: 10.1002/cpt.1377
Cicero, A. F., Salvi, P., D’Addato, S., Rosticci, M., Borghi, C., Brisighella Heart Study, g (2014). Association between serum uric acid, hypertension, vascular stiffness and subclinical atherosclerosis: data from the Brisighella Heart Study. J. Hypertens. 32 (1), 57–64. doi: 10.1097/HJH.0b013e328365b916
Corry, D. B., Eslami, P., Yamamoto, K., Nyby, M. D., Makino, H., Tuck, M. L. (2008). Uric acid stimulates vascular smooth muscle cell proliferation and oxidative stress via the vascular renin-angiotensin system. J. Hypertens. 26 (2), 269–275. doi: 10.1097/HJH.0b013e3282f240bf
Culleton, B. F., Larson, M. G., Kannel, W. B., Levy, D. (1999). Serum Uric Acid and Risk for Cardiovascular Disease and Death: The Framingham Heart Study. Ann. Intern. Med. 131 (1), 7–13. doi: 10.7326/0003-4819-131-1-199907060-00003
Dai, X. M., Wei, L., Ma, L. L., Chen, H. Y., Zhang, Z. J., Ji, Z. F., et al. (2015). Serum uric acid and its relationship with cardiovascular risk profile in Chinese patients with early-onset coronary artery disease. Clin. Rheumatol. 34 (9), 1605–1611. doi: 10.1007/s10067-015-2878-1
Davies, K. J., Sevanian, A., Muakkassah-Kelly, S. F., Hochstein, P. (1986). Uric acid-iron ion complexes. A new aspect of the antioxidant functions of uric acid. Biochem. J. 235 (3), 747–754. doi: 10.1042/bj2350747
Doehner, W., Schoene, N., Rauchhaus, M., Leyva-Leon, F., Pavitt, D. V., Reaveley, D. A., et al. (2002). Effects of xanthine oxidase inhibition with allopurinol on endothelial function and peripheral blood flow in hyperuricemic patients with chronic heart failure: results from 2 placebo-controlled studies. Circulation 105 (22), 2619–2624. doi: 10.1161/01.cir.0000017502.58595.ed
Farquharson, C. A., Butler, R., Hill, A., Belch, J. J., Struthers, A. D. (2002). Allopurinol improves endothelial dysfunction in chronic heart failure. Circulation 106 (2), 221–226. doi: 10.1161/01.cir.0000022140.61460.1d
Forman, J. P., Choi, H., Curhan, G. C. (2009). Uric acid and insulin sensitivity and risk of incident hypertension. Arch. Intern. Med. 169 (2), 155–162. doi: 10.1001/archinternmed.2008.521
George, J., Carr, E., Davies, J., Belch, J. J., Struthers, A. (2006). High-dose allopurinol improves endothelial function by profoundly reducing vascular oxidative stress and not by lowering uric acid. Circulation 114 (23), 2508–2516. doi: 10.1161/CIRCULATIONAHA.106.651117
Gersch, C., Palii, S. P., Imaram, W., Kim, K. M., Karumanchi, S. A., Angerhofer, A., et al. (2009). Reactions of peroxynitrite with uric acid: formation of reactive intermediates, alkylated products and triuret, and in vivo production of triuret under conditions of oxidative stress. Nucleosides Nucleotides Nucleic Acids 28 (2), 118–149. doi: 10.1080/15257770902736400
Grayson, P. C., Kim, S. Y., LaValley, M., Choi, H. K. (2011). Hyperuricemia and incident hypertension: a systematic review and meta-analysis. Arthritis Care Res. (Hoboken) 63 (1), 102–110. doi: 10.1002/acr.20344
Hao, Y., Li, H., Cao, Y., Chen, Y., Lei, M., Zhang, T., et al. (2019). Uricase and Horseradish Peroxidase Hybrid CaHPO(4) Nanoflower Integrated with Transcutaneous Patches for Treatment of Hyperuricemia. J. BioMed. Nanotechnol. 15 (5), 951–965. doi: 10.1166/jbn.2019.2752
Ho, W. J., Tsai, W. P., Yu, K. H., Tsay, P. K., Wang, C. L., Hsu, T. S., et al. (2010). Association between endothelial dysfunction and hyperuricaemia. Rheumatol. (Oxford) 49 (10), 1929–1934. doi: 10.1093/rheumatology/keq184
Huang, H., Huang, B., Li, Y., Huang, Y., Li, J., Yao, H., et al. (2014). Uric acid and risk of heart failure: a systematic review and meta-analysis. Eur. J. Heart Fail 16 (1), 15–24. doi: 10.1093/eurjhf/hft132
Huang, T. T., Hao, D. L., Wu, B. N., Mao, L. L., Zhang, J. (2017). Uric acid demonstrates neuroprotective effect on Parkinson’s disease mice through Nrf2-ARE signaling pathway. Biochem. Biophys. Res. Commun. 493 (4), 1443–1449. doi: 10.1016/j.bbrc.2017.10.004
Inaba, S., Sautin, Y., Garcia, G. E., Johnson, R. J. (2013). What can asymptomatic hyperuricaemia and systemic inflammation in the absence of gout tell us? Rheumatol. (Oxford) 52 (6), 963–965. doi: 10.1093/rheumatology/ket001
Jia, G., Habibi, J., Bostick, B. P., Ma, L., DeMarco, V. G., Aroor, A. R., et al. (2015). Uric acid promotes left ventricular diastolic dysfunction in mice fed a Western diet. Hypertension 65 (3), 531–539. doi: 10.1161/HYPERTENSIONAHA.114.04737
Johnson, R. J., Bakris, G. L., Borghi, C., Chonchol, M. B., Feldman, D., Lanaspa, M. A., et al. (2018). Hyperuricemia, Acute and Chronic Kidney Disease, Hypertension, and Cardiovascular Disease: Report of a Scientific Workshop Organized by the National Kidney Foundation. Am. J. Kidney Dis. 71 (6), 851–865. doi: 10.1053/j.ajkd.2017.12.009
Johnson, R. J., Choi, H. K., Yeo, A. E., Lipsky, P. E. (2019). Pegloticase Treatment Significantly Decreases Blood Pressure in Patients With Chronic Gout. Hypertension 74 (1), 95–101. doi: 10.1161/HYPERTENSIONAHA.119.12727
Jun, J. E., Lee, Y. B., Lee, S. E., Ahn, J. Y., Kim, G., Jin, S. M., et al. (2018). Elevated serum uric acid predicts the development of moderate coronary artery calcification independent of conventional cardiovascular risk factors. Atherosclerosis 272, 233–239. doi: 10.1016/j.atherosclerosis.2018.02.014
Kato, M., Hisatome, I., Tomikura, Y., Kotani, K., Kinugawa, T., Ogino, K., et al. (2005). Status of endothelial dependent vasodilation in patients with hyperuricemia. Am. J. Cardiol. 96 (11), 1576–1578. doi: 10.1016/j.amjcard.2005.07.068
Katsiki, N., Mikhailidis, D. P. (2015). Hyperuricaemia in cardiovascular diseases: a passive or an active player? Med. Princ. Pract. 24 (3), 269–270. doi: 10.1159/000381398
Khaliq, O. P., Konoshita, T., Moodley, J., Naicker, T. (2018). The Role of Uric Acid in Preeclampsia: Is Uric Acid a Causative Factor or a Sign of Preeclampsia? Curr. Hypertens. Rep. 20 (9), 80. doi: 10.1007/s11906-018-0878-7
Kim, S. K., Choe, J. Y., Park, K. Y. (2019). Anti-inflammatory effect of artemisinin on uric acid-induced NLRP3 inflammasome activation through blocking interaction between NLRP3 and NEK7. Biochem. Biophys. Res. Commun. 517 (2), 338–345. doi: 10.1016/j.bbrc.2019.07.087
Kimura, Y., Yanagida, T., Onda, A., Tsukui, D., Hosoyamada, M., Kono, H. (2020). Soluble Uric Acid Promotes Atherosclerosis via AMPK (AMP-Activated Protein Kinase)-Mediated Inflammation. Arterioscler. Thromb. Vasc. Biol. 40 (3), 570–582. doi: 10.1161/ATVBAHA.119.313224
Klisic, A., Kocic, G., Kavaric, N., Jovanovic, M., Stanisic, V., Ninic, A. (2018). Xanthine oxidase and uric acid as independent predictors of albuminuria in patients with diabetes mellitus type 2. Clin. Exp. Med. 18 (2), 283–290. doi: 10.1007/s10238-017-0483-0
Kobayashi, N., Asai, K., Tsurumi, M., Shibata, Y., Okazaki, H., Shirakabe, A., et al. (2018). Impact of Accumulated Serum Uric Acid on Coronary Culprit Lesion Morphology Determined by Optical Coherence Tomography and Cardiac Outcomes in Patients with Acute Coronary Syndrome. Cardiology 141 (4), 190–198. doi: 10.1159/000496053
Kodama, S., Saito, K., Yachi, Y., Asumi, M., Sugawara, A., Totsuka, K., et al. (2009). Association between serum uric acid and development of type 2 diabetes. Diabetes Care 32 (9), 1737–1742. doi: 10.2337/dc09-0288
Kramer, F., Voss, S., Roessig, L., Igl, B. W., Butler, J., Lam, C. S. P., et al. (2020). Evaluation of high-sensitivity C-reactive protein and uric acid in vericiguat-treated patients with heart failure with reduced ejection fraction. Eur. J. Heart Fail. 22 (9), 1675–1683. doi: 10.1002/ejhf.1787
Kushiyama, A., Nakatsu, Y., Matsunaga, Y., Yamamotoya, T., Mori, K., Ueda, K., et al. (2016). Role of Uric Acid Metabolism-Related Inflammation in the Pathogenesis of Metabolic Syndrome Components Such as Atherosclerosis and Nonalcoholic Steatohepatitis. Mediators Inflammation 2016, 1–15. doi: 10.1155/2016/8603164
Kuwabara, M., Kuwabara, R., Hisatome, I., Niwa, K., Roncal-Jimenez, C. A., Bjornstad, P., et al. (2017a). “Metabolically Healthy” Obesity and Hyperuricemia Increase Risk for Hypertension and Diabetes: 5-year Japanese Cohort Study. Obes. (Silver Spring) 25 (11), 1997–2008. doi: 10.1002/oby.22000
Kuwabara, M., Niwa, K., Hisatome, I., Nakagawa, T., Roncal-Jimenez, C. A., Andres-Hernando, A., et al. (2017b). Asymptomatic Hyperuricemia Without Comorbidities Predicts Cardiometabolic Diseases: Five-Year Japanese Cohort Study. Hypertension 69 (6), 1036–1044. doi: 10.1161/HYPERTENSIONAHA.116.08998
Kuwabara, M., Niwa, K., Nishihara, S., Nishi, Y., Takahashi, O., Kario, K., et al. (2017c). Hyperuricemia is an independent competing risk factor for atrial fibrillation. Int. J. Cardiol. 231, 137–142. doi: 10.1016/j.ijcard.2016.11.268
Kuwabara, M., Borghi, C., Cicero, A. F. G., Hisatome, I., Niwa, K., Ohno, M., et al. (2018a). Elevated serum uric acid increases risks for developing high LDL cholesterol and hypertriglyceridemia: A five-year cohort study in Japan. Int. J. Cardiol. 261, 183–188. doi: 10.1016/j.ijcard.2018.03.045
Kuwabara, M., Hisatome, I., Niwa, K., Hara, S., Roncal-Jimenez, C. A., Bjornstad, P., et al. (2018b). Uric Acid Is a Strong Risk Marker for Developing Hypertension From Prehypertension: A 5-Year Japanese Cohort Study. Hypertension 71 (1), 78–86. doi: 10.1161/HYPERTENSIONAHA.117.10370
Li, Z., Shen, Y., Chen, Y., Zhang, G., Cheng, J., Wang, W. (2018). High Uric Acid Inhibits Cardiomyocyte Viability Through the ERK/P38 Pathway via Oxidative Stress. Cell Physiol. Biochem. 45 (3), 1156–1164. doi: 10.1159/000487356
Li, H., Qian, F., Liu, H., Zhang, Z. (2019). Elevated Uric Acid Levels Promote Vascular Smooth Muscle Cells (VSMC) Proliferation via an Nod-Like Receptor Protein 3 (NLRP3)-Inflammasome-Dependent Mechanism. Med. Sci. Monit. 25, 8457–8464. doi: 10.12659/MSM.916667
Li, M., Hu, X., Fan, Y., Li, K., Zhang, X., Hou, W., et al. (2016). Hyperuricemia and the risk for coronary heart disease morbidity and mortality a systematic review and dose-response meta-analysis. Sci. Rep. 6, 19520. doi: 10.1038/srep19520
Li, P., Zhang, L., Zhang, M., Zhou, C., Lin, N. (2016). Uric acid enhances PKC-dependent eNOS phosphorylation and mediates cellular ER stress: A mechanism for uric acid-induced endothelial dysfunction. Int. J. Mol. Med. 37 (4), 989–997. doi: 10.3892/ijmm.2016.2491
Liang, W. Y., Liu, W. W., Liu, M. L., Xiang, W., Feng, X. R., Huang, B., et al. (2016). Serum uric acid level and left ventricular hypertrophy in elderly male patients with nonvalvular atrial fibrillation. Nutr. Metab. Cardiovasc. Dis. 26 (7), 575–580. doi: 10.1016/j.numecd.2016.03.011
Lin, W. D., Deng, H., Guo, P., Liu, F. Z., Chen, R. Y., Fang, X. H., et al. (2019). High prevalence of hyperuricaemia and its impact on non-valvular atrial fibrillation: the cross-sectional Guangzhou (China) Heart Study. BMJ Open 9 (5), e028007. doi: 10.1136/bmjopen-2018-028007
Liu, P., Wang, H., Zhang, F., Chen, Y., Wang, D., Wang, Y. (2015). The Effects of Allopurinol on the Carotid Intima-media Thickness in Patients with Type 2 Diabetes and Asymptomatic Hyperuricemia: A Three-year Randomized Parallel-controlled Study. Intern. Med. 54 (17), 2129–2137. doi: 10.2169/internalmedicine.54.4310
Lu, J., Sun, M., Wu, X., Yuan, X., Liu, Z., Qu, X., et al. (2019). Urate-lowering therapy alleviates atherosclerosis inflammatory response factors and neointimal lesions in a mouse model of induced carotid atherosclerosis. FEBS J. 286 (7), 1346–1359. doi: 10.1111/febs.14768
Lu, J., He, Y., Cui, L., Xing, X., Liu, Z., Li, X., et al. (2020). Hyperuricemia Predisposes to the Onset of Diabetes via Promoting Pancreatic beta-Cell Death in Uricase-Deficient Male Mice. Diabetes 69 (6), 1149–1163. doi: 10.2337/db19-0704
MacIsaac, R. L., Salatzki, J., Higgins, P., Walters, M. R., Padmanabhan, S., Dominiczak, A. F., et al. (2016). Allopurinol and Cardiovascular Outcomes in Adults With Hypertension. Hypertension 67 (3), 535–540. doi: 10.1161/HYPERTENSIONAHA.115.06344
Maharani, N., Ting, Y. K., Cheng, J., Hasegawa, A., Kurata, Y., Li, P., et al. (2015). Molecular Mechanisms Underlying Urate-Induced Enhancement of Kv1.5 Channel Expression in HL-1 Atrial Myocytes. Circ. J. 79 (12), 2659–2668. doi: 10.1253/circj.CJ-15-0416
Mantovani, A., Rigolon, R., Civettini, A., Bolzan, B., Morani, G., Bonapace, S., et al. (2018). Hyperuricemia is associated with an increased prevalence of paroxysmal atrial fibrillation in patients with type 2 diabetes referred for clinically indicated 24-h Holter monitoring. J. Endocrinol. Invest. 41 (2), 223–231. doi: 10.1007/s40618-017-0729-4
Martinon, F., Petrilli, V., Mayor, A., Tardivel, A., Tschopp, J. (2006). Gout-associated uric acid crystals activate the NALP3 inflammasome. Nature 440 (7081), 237–241. doi: 10.1038/nature04516
Maruhashi, T., Nakashima, A., Soga, J., Fujimura, N., Idei, N., Mikami, S., et al. (2013). Hyperuricemia is independently associated with endothelial dysfunction in postmenopausal women but not in premenopausal women. BMJ Open 3 (11), e003659. doi: 10.1136/bmjopen-2013-003659
Maruhashi, T., Hisatome, I., Kihara, Y., Higashi, Y. (2018). Hyperuricemia and endothelial function: From molecular background to clinical perspectives. Atherosclerosis 278, 226–231. doi: 10.1016/j.atherosclerosis.2018.10.007
Matias, M. L., Romao, M., Weel, I. C., Ribeiro, V. R., Nunes, P. R., Borges, V. T., et al. (2015). Endogenous and Uric Acid-Induced Activation of NLRP3 Inflammasome in Pregnant Women with Preeclampsia. PloS One 10 (6), e0129095. doi: 10.1371/journal.pone.0129095
McMurray, J. J. V., Solomon, S. D., Inzucchi, S. E., Kober, L., Kosiborod, M. N., Martinez, F. A., et al. (2019). Dapagliflozin in Patients with Heart Failure and Reduced Ejection Fraction. N Engl. J. Med. 381 (21), 1995–2008. doi: 10.1056/NEJMoa1911303
Mercuro, G., Vitale, C., Cerquetani, E., Zoncu, S., Deidda, M., Fini, M., et al. (2004). Effect of hyperuricemia upon endothelial function in patients at increased cardiovascular risk. Am. J. Cardiol. 94 (7), 932–935. doi: 10.1016/j.amjcard.2004.06.032
Mortada, I. (2017). Hyperuricemia, Type 2 Diabetes Mellitus, and Hypertension: an Emerging Association. Curr. Hypertens. Rep. 19 (9), 69. doi: 10.1007/s11906-017-0770-x
Neal, B., Perkovic, V., Mahaffey, K. W., de Zeeuw, D., Fulcher, G., Erondu, N., et al. (2017). Canagliflozin and Cardiovascular and Renal Events in Type 2 Diabetes. N Engl. J. Med. 377 (7), 644–657. doi: 10.1056/NEJMoa1611925
Nidorf, M., Jelinek, M. (2018). Serendipity: How the search for meaning of serum uric acid might lead to the repurposing of an old drug in patients with cardiovascular disease. Eur. J. Prev. Cardiol. 25 (3), 231–232. doi: 10.1177/2047487317749039
Noman, A., Ang, D. S. C., Ogston, S., Lang, C. C., Struthers, A. D. (2010). Effect of High-Dose Allopurinol on Exercise in Patients With Chronic Stable Angina: A Randomised, Placebo Controlled Crossover Trial. Lancet 375 (9732), 2161–2167. doi: 10.1016/S0140-6736(10)60391-1
Ozturk, D., Celik, O., Akin, F., Akturk, F., Aslan, S., Ozyilmaz, S. O., et al. (2015). Usefulness of the uric acid and CHA(2)DS(2)-VASc score in prediction of left atrial thrombosis in patients with mitral stenosis and sinus rhythm. Cardiol. J. 22 (3), 336–342. doi: 10.5603/CJ.a2014.0059
Pak, S., Yatsynovich, Y., Valencia, D., Chen, T. (2018). Serum Uric Acid and Atrial Fibrillation: Meta-analysis. Crit. Pathw. Cardiol. 17 (3), 161–166. doi: 10.1097/HPC.0000000000000150
Palazzuoli, A., Ruocco, G., De Vivo, O., Nuti, R., McCullough, P. A. (2017). Prevalence of Hyperuricemia in Patients With Acute Heart Failure With Either Reduced or Preserved Ejection Fraction. Am. J. Cardiol. 120 (7), 1146–1150. doi: 10.1016/j.amjcard.2017.06.057
Papezikova, I., Pekarova, M., Kolarova, H., Klinke, A., Lau, D., Baldus, S., et al. (2013). Uric acid modulates vascular endothelial function through the down regulation of nitric oxide production. Free Radic. Res. 47 (2), 82–88. doi: 10.3109/10715762.2012.747677
Pavlusova, M., Jarkovsky, J., Benesova, K., Vitovec, J., Linhart, A., Widimsky, P., et al. (2019). Hyperuricemia treatment in acute heart failure patients does not improve their long-term prognosis: A propensity score matched analysis from the AHEAD registry. Clin. Cardiol. 42 (8), 720–727. doi: 10.1002/clc.23197
Prasad, M., Matteson, E. L., Herrmann, J., Gulati, R., Rihal, C. S., Lerman, L. O., et al. (2017). Uric Acid Is Associated With Inflammation, Coronary Microvascular Dysfunction, and Adverse Outcomes in Postmenopausal Women. Hypertension 69 (2), 236–242. doi: 10.1161/HYPERTENSIONAHA.116.08436
Rekhraj, S., Gandy, S. J., Szwejkowski, B. R., Nadir, M. A., Noman, A., Houston, J. G., et al. (2013). High-dose allopurinol reduces left ventricular mass in patients with ischemic heart disease. J. Am. Coll. Cardiol. 61 (9), 926–932. doi: 10.1016/j.jacc.2012.09.066
Ritchie, R. H. (2009). Evidence for a causal role of oxidative stress in the myocardial complications of insulin resistance. Heart Lung Circ. 18 (1), 11–18. doi: 10.1016/j.hlc.2008.11.003
Sakr, H. I., Khowailed, A. A., Al-Fakharany, R. S., Abdel-Fattah, D. S., Taha, A. A. (2020). Serum Uric Acid Level as a Predictive Biomarker of Gestational Hypertension Severity; A Prospective Observational Case-Control Study. Rev. Recent Clin. Trials 15 (3), 227–239. doi: 10.2174/1574887115666200709142119
Sautin, Y. Y., Johnson, R. J. (2008). Uric acid: the oxidant-antioxidant paradox. Nucleosides Nucleotides Nucleic Acids 27 (6), 608–619. doi: 10.1080/15257770802138558
Sincer, I., Kurtoglu, E., Caliskan, M., Akkaya, E., Vuruskan, E., Kucukosmanoglu, M., et al. (2014). Significant correlation between uric acid levels and flow-mediated dilatation in patients with masked hypertension. Clin. Exp. Hypertens. 36 (5), 315–320. doi: 10.3109/10641963.2013.827694
Singh, J. A., Cleveland, J. (2018). Allopurinol and the risk of incident peripheral arterial disease in the elderly: a US Medicare claims data study. Rheumatol. (Oxford) 57 (3), 451–461. doi: 10.1093/rheumatology/kex232
Singh, J. A., Ramachandaran, R., Yu, S., Curtis, J. R. (2017). Allopurinol use and the risk of acute cardiovascular events in patients with gout and diabetes. BMC Cardiovasc. Disord. 17 (1), 76. doi: 10.1186/s12872-017-0513-6
Singh, G., Lingala, B., Mithal, A. (2019). Gout and hyperuricaemia in the USA: prevalence and trends. Rheumatol. (Oxford) 58 (12), 2177–2180. doi: 10.1093/rheumatology/kez196
Struthers, A. D., Donnan, P. T., Lindsay, P., McNaughton, D., Broomhall, J., MacDonald, T. M. (2002). Effect of allopurinol on mortality and hospitalisations in chronic heart failure: a retrospective cohort study. Heart 87 (3), 229–234. doi: 10.1136/heart.87.3.229
Sun, H. L., Pei, D., Lue, K. H., Chen, Y. L. (2015). Uric Acid Levels Can Predict Metabolic Syndrome and Hypertension in Adolescents: A 10-Year Longitudinal Study. PloS One 10 (11), e0143786. doi: 10.1371/journal.pone.0143786
Sun, X., Jiao, H., Zhao, J., Wang, X., Lin, H. (2017). Unexpected effect of urate on hydrogen peroxide-induced oxidative damage in embryonic chicken cardiac cells. Free Radic. Res. 51 (7-8), 693–707. doi: 10.1080/10715762.2017.1362106
Szwejkowski, B. R., Gandy, S. J., Rekhraj, S., Houston, J. G., Lang, C. C., Morris, A. D., et al. (2013). Allopurinol reduces left ventricular mass in patients with type 2 diabetes and left ventricular hypertrophy. J. Am. Coll. Cardiol. 62 (24), 2284–2293. doi: 10.1016/j.jacc.2013.07.074
Tamariz, L., Agarwal, S., Soliman, E. Z., Chamberlain, A. M., Prineas, R., Folsom, A. R., et al. (2011). Association of serum uric acid with incident atrial fibrillation (from the Atherosclerosis Risk in Communities [ARIC] study). Am. J. Cardiol. 108 (9), 1272–1276. doi: 10.1016/j.amjcard.2011.06.043
Tang, R. B., Dong, J. Z., Yan, X. L., Du, X., Kang, J. P., Wu, J. H., et al. (2014). Serum uric acid and risk of left atrial thrombus in patients with nonvalvular atrial fibrillation. Can. J. Cardiol. 30 (11), 1415–1421. doi: 10.1016/j.cjca.2014.06.009
Taufiq, F., Maharani, N., Li, P., Kurata, Y., Ikeda, N., Kuwabara, M., et al. (2019). Uric Acid-Induced Enhancements of Kv1.5 Protein Expression and Channel Activity via the Akt-HSF1-Hsp70 Pathway in HL-1 Atrial Myocytes. Circ. J. 83 (4), 718–726. doi: 10.1253/circj.CJ-18-1088
Tomiyama, H., Higashi, Y., Takase, B., Node, K., Sata, M., Inoue, T., et al. (2011). Relationships among hyperuricemia, metabolic syndrome, and endothelial function. Am. J. Hypertens. 24 (7), 770–774. doi: 10.1038/ajh.2011.55
Tomiyama, H., Shiina, K., Vlachopoulos, C., Iwasaki, Y., Matsumoto, C., Kimura, K., et al. (2018). Involvement of Arterial Stiffness and Inflammation in Hyperuricemia-Related Development of Hypertension. Hypertension 72 (3), 739–745. doi: 10.1161/HYPERTENSIONAHA.118.11390
Vaduganathan, M., Greene, S. J., Ambrosy, A. P., Mentz, R. J., Subacius, H. P., Chioncel, O., et al. (2014). Relation of serum uric acid levels and outcomes among patients hospitalized for worsening heart failure with reduced ejection fraction (from the efficacy of vasopressin antagonism in heart failure outcome study with tolvaptan trial). Am. J. Cardiol. 114 (11), 1713–1721. doi: 10.1016/j.amjcard.2014.09.008
van der Schaft, N., Brahimaj, A., Wen, K. X., Franco, O. H., Dehghan, A. (2017). The association between serum uric acid and the incidence of prediabetes and type 2 diabetes mellitus: The Rotterdam Study. PloS One 12 (6), e0179482. doi: 10.1371/journal.pone.0179482
Vane, J. R., Anggard, E. E., Botting, R. M. (1990). Regulatory functions of the vascular endothelium. N Engl. J. Med. 323, 27–36. doi: 10.1056/NEJM199007053230106
Virdis, A., Masi, S., Casiglia, E., Tikhonoff, V., Cicero, A. F. G., Ungar, A., et al. (2020). Identification of the Uric Acid Thresholds Predicting an Increased Total and Cardiovascular Mortality Over 20 Years. Hypertension 75 (2), 302–308. doi: 10.1161/hypertensionaha.119.13643
von Lueder, T. G., Girerd, N., Atar, D., Agewall, S., Lamiral, Z., Kanbay, M., et al. (2015). Serum uric acid is associated with mortality and heart failure hospitalizations in patients with complicated myocardial infarction: findings from the High-Risk Myocardial Infarction Database Initiative. Eur. J. Heart Fail 17 (11), 1144–1151. doi: 10.1002/ejhf.419
Wang, G. L., Yuan, H. M., Wang, Z. F., Zong, B. B., Ye, Y., Zhang, J., et al. (2018). Soluble Uric Acid Activates NLRP3 Inflammasome in Myocardial Cells Through Down-regulating UCP2. Sichuan Da Xue Xue Bao Yi Xue Ban (Article Chinese) 49 (4), 512–517.
Wang, I. K., Chen, J. H., Muo, C. H., Chou, C. Y., Liu, Y. L., Lin, S. Y., et al. (2016). Subsequent risk of gout for women with hypertensive disorders in pregnancy: a retrospective cohort study. J. Hypertens. 34 (5), 914–919. doi: 10.1097/HJH.0000000000000888
Wang, R., Song, Y., Yan, Y., Ding, Z. (2016). Elevated serum uric acid and risk of cardiovascular or all-cause mortality in people with suspected or definite coronary artery disease: A meta-analysis. Atherosclerosis 254, 193–199. doi: 10.1016/j.atherosclerosis.2016.10.006
Waring, W. S., Webb, D. J., Maxwell, S. R. (2001). Systemic uric acid administration increases serum antioxidant capacity in healthy volunteers. J. Cardiovasc. Pharmacol. 38 (3), 365–371. doi: 10.1097/00005344-200109000-00005
White, W. B., Saag, K. G., Becker, M. A., Borer, J. S., Gorelick, P. B., Whelton, A., et al. (2018). Cardiovascular Safety of Febuxostat or Allopurinol in Patients with Gout. N Engl. J. Med. 378 (13), 1200–1210. doi: 10.1056/NEJMoa1710895
Whiteman, M., Ketsawatsakul, U., Halliwell, B. (2002). A reassessment of the peroxynitrite scavenging activity of uric acid. Ann. N Y Acad. Sci. 962, 242–259. doi: 10.1111/j.1749-6632.2002.tb04072.x
Wilcox, C. S., Shen, W., Boulton, D. W., Leslie, B. R., Griffen, S. C. (2018). Interaction Between the Sodium-Glucose-Linked Transporter 2 Inhibitor Dapagliflozin and the Loop Diuretic Bumetanide in Normal Human Subjects. J. Am. Heart Assoc. 7 (4), e007046. doi: 10.1161/JAHA.117.007046
Wiviott, S. D., Raz, I., Bonaca, M. P., Mosenzon, O., Kato, E. T., Cahn, A., et al. (2019). Dapagliflozin and Cardiovascular Outcomes in Type 2 Diabetes. N Engl. J. Med. 380 (4), 347–357. doi: 10.1056/NEJMoa1812389
Wu, G. J., Han, Y. L., Wang, X. Z. (2012). Research Progress on the relationship between hyperuricemia and coronary heart disease. Chin. J. Cardiovasc. Rehablil. Med. 21 (2), 216–217.
Xiao, J., Zhang, X. L., Fu, C., Han, R., Chen, W., Lu, Y., et al. (2015). Soluble uric acid increases NALP3 inflammasome and interleukin-1beta expression in human primary renal proximal tubule epithelial cells through the Toll-like receptor 4-mediated pathway. Int. J. Mol. Med. 35 (5), 1347–1354. doi: 10.3892/ijmm.2015.2148
Xin, Y., Guo, Y., Li, Y., Ma, Y., Li, L., Jiang, H. (2019). Effects of sodium glucose cotransporter-2 inhibitors on serum uric acid in type 2 diabetes mellitus: A systematic review with an indirect comparison meta-analysis. Saudi J. Biol. Sci. 26 (2), 421–426. doi: 10.1016/j.sjbs.2018.11.013
Yan, M., Chen, K., He, L., Li, S., Huang, D., Li, J. (2018). Uric Acid Induces Cardiomyocyte Apoptosis via Activation of Calpain-1 and Endoplasmic Reticulum Stress. Cell Physiol. Biochem. 45 (5), 2122–2135. doi: 10.1159/000488048
Yin, W., Zhou, Q. L., OuYang, S. X., Chen, Y., Gong, Y. T., Liang, Y. M. (2019). Uric acid regulates NLRP3/IL-1beta signaling pathway and further induces vascular endothelial cells injury in early CKD through ROS activation and K(+) efflux. BMC Nephrol. 20 (1), 319. doi: 10.1186/s12882-019-1506-8
Yu, M.-A., Sánchez-Lozada, L. G., Johnson, R. J., Kang, D.-H. (2010). Oxidative stress with an activation of the renin–angiotensin system in human vascular endothelial cells as a novel mechanism of uric acid-induced endothelial dysfunction. J. Hypertension. 28(6), 1234–1242. doi: 10.1097/HJH.0b013e328337da1d
Zhang, C. H., Huang, D. S., Shen, D., Zhang, L. W., Ma, Y. J., Wang, Y. M., et al. (2016). Association Between Serum Uric Acid Levels and Atrial Fibrillation Risk. Cell Physiol. Biochem. 38 (4), 1589–1595. doi: 10.1159/000443099
Zhang, M., Solomon, D. H., Desai, R. J., Kang, E. H., Liu, J., Neogi, T., et al. (2018). Assessment of Cardiovascular Risk in Older Patients With Gout Initiating Febuxostat Versus Allopurinol: Population-Based Cohort Study. Circulation 138 (11), 1116–1126. doi: 10.1161/CIRCULATIONAHA.118.033992
Zhao, J., Liu, T., Korantzopoulos, P., Letsas, K. P., Zhang, E., Yang, Y., et al. (2016). Association between serum uric acid and atrial fibrillation recurrence following catheter ablation: A meta-analysis. Int. J. Cardiol. 204, 103–105. doi: 10.1016/j.ijcard.2015.11.167
Zhao, Y., Xu, L., Tian, D., Xia, P., Zheng, H., Wang, L., et al. (2018). Effects of sodium-glucose co-transporter 2 (SGLT2) inhibitors on serum uric acid level: A meta-analysis of randomized controlled trials. Diabetes Obes. Metab. 20 (2), 458–462. doi: 10.1111/dom.13101
Zhi, L., Yuzhang, Z., Tianliang, H., Hisatome, I., Yamamoto, T., Jidong, C. (2016). High Uric Acid Induces Insulin Resistance in Cardiomyocytes In Vitro and In Vivo. PloS One 11 (2), e0147737. doi: 10.1371/journal.pone.0147737
Keywords: uric acid, cardiovascular disease, molecular mechanism, therapeutics, clinical prospect
Citation: Yu W and Cheng J-D (2020) Uric Acid and Cardiovascular Disease: An Update From Molecular Mechanism to Clinical Perspective. Front. Pharmacol. 11:582680. doi: 10.3389/fphar.2020.582680
Received: 13 July 2020; Accepted: 16 September 2020;
Published: 16 November 2020.
Edited by:
Yusuf Tutar, University of Health Sciences, TurkeyReviewed by:
Claudio Borghi, University of Bologna, ItalyCarlos Alonso Escudero, University of the Bío Bío, Chile
Copyright © 2020 Yu and Cheng. This is an open-access article distributed under the terms of the Creative Commons Attribution License (CC BY). The use, distribution or reproduction in other forums is permitted, provided the original author(s) and the copyright owner(s) are credited and that the original publication in this journal is cited, in accordance with accepted academic practice. No use, distribution or reproduction is permitted which does not comply with these terms.
*Correspondence: Wei Yu, eXV3ZWkxMDYxMDA0OEAxMjYuY29t; Ji-Dong Cheng, amlkb25nY2hlbmczNkBob3RtYWlsLmNvbQ==