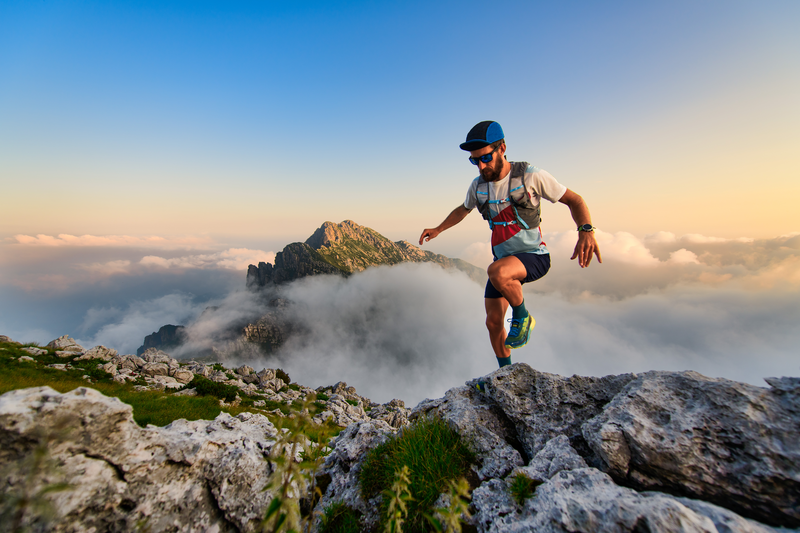
95% of researchers rate our articles as excellent or good
Learn more about the work of our research integrity team to safeguard the quality of each article we publish.
Find out more
BRIEF RESEARCH REPORT article
Front. Pharmacol. , 07 October 2020
Sec. Pharmacology of Ion Channels and Channelopathies
Volume 11 - 2020 | https://doi.org/10.3389/fphar.2020.581151
In recent years, transmembrane voltage has been found to modify agonist potencies at several G protein-coupled receptors (GPCRs). Whereas the voltage sensitivities of the Gαi/o-coupled dopamine D2-like receptors (D2R, D3R, D4R) have previously been investigated, the putative impact of transmembrane voltage on agonist potency at the mainly Gαs/olf-coupled dopamine D1-like receptors (D1R, D5R) has hitherto not been reported. Here, we assayed the potency of dopamine in activating G protein-coupled inward rectifier potassium (GIRK) channels co-expressed with D1R and D5R in Xenopus oocytes, at -80 mV and at 0 mV. Furthermore, GIRK response deactivation rates upon dopamine washout were measured to estimate dopamine dissociation rate (koff) constants. Depolarization from -80 to 0 mV was found to reduce dopamine potency by about 7-fold at both D1R and D5R. This potency reduction was accompanied by an increase in estimated dopamine koffs at both receptors. While the GIRK response elicited via D1R was insensitive to pertussis toxin (PTX), the response evoked via D5R was reduced by 64% (-80 mV) and 71% (0 mV) in the presence of PTX. Injection of oocytes with Gαs antisense oligonucleotide inhibited the D1R-mediated response by 62% (-80 mV) and 76% (0 mV) and abolished the D5R response when combined with PTX. Our results suggest that depolarization decreases dopamine affinity at D1R and D5R. The voltage-dependent affinities of dopamine at D1R and D5R may be relevant to the functions of these receptors in learning and memory.
Dopamine (DA) receptors are G protein-coupled receptors (GPCRs) subgrouped into the D1-like (D1R and D5R) and the D2-like (D2R, D3R, and D4R) receptor families. The D1-like receptors are mainly coupled to stimulatory Gαs/olf proteins and expressed in striatum, cortex, amygdala, and hippocampus (Huang et al., 2001; Sarinana et al., 2014) where they are involved in cognitive functions such as memory and attention (Sawaguchi and Goldman-Rakic, 1994; Carr et al., 2017).
D1R activation has been closely related to prefrontal cortex functioning by a U-shaped relationship; moderate D1R activation is required for optimal performance in learning and memory tasks (Goldman-Rakic et al., 2004; Arnsten et al., 2010). Recent data also implicate D5R in working memory and prefrontal cortex function (Carr et al., 2017). Accordingly, D1R agonists (many of which are also D5R agonists) and positive allosteric modulators are being investigated as putative therapeutics for the treatment of cognitive deficits, e.g., in Alzheimer’s disease, schizophrenia, and Parkinson’s disease (Lewis et al., 2015; Bruns et al., 2018; Luderman et al., 2018; Yang et al., 2018).
Agonist affinities and functional potencies at a number of GPCRs, including D2R, D4R, and muscarinic M2 receptors (M2R; (Ben-Chaim et al., 2003; Sahlholm et al., 2008a), have been shown to be regulated by the membrane potential. Interestingly, DA potency in D3R-mediated GIRK activation was not significantly different between -80 and 0 mV, suggesting that this receptor might be insensitive to voltage (Sahlholm et al., 2008a). However, the influence of the membrane potential on agonist potency at the D1-like family of receptors remains unexplored. Several previous experimental investigations of GPCR voltage-dependence have made use of two-electrode voltage-clamp in Xenopus oocytes heterologously expressing GPCRs and G protein-coupled inward-rectifying potassium (GIRK) channels. This has allowed for investigation of GPCR activity by measuring GIRK activation evoked by Gαi/o-coupled GPCRs (Ben-Chaim et al., 2003; Sahlholm et al., 2008a; Sahlholm et al., 2011; Sahlholm et al., 2012) and by Gαq-coupled GPCRs in the presence of a chimeric Gαq-i protein (Ohana et al., 2006).
Although GIRK channel opening is elicited by Gβγ, which can be associated with a range of Gα subunits, activation of native GIRK currents is mediated almost exclusively via Gαi/o- and not Gαs/olf-proteins. This specificity may be achieved through the higher rate of turnover of the G protein cycle of Gαi/o-proteins, liberating higher local concentrations of Gβγ upon receptor activation (Touhara and MacKinnon, 2018). However, based on previously published work with similarly Gs-coupled β-adrenergic receptors (Lim et al., 1995; Wellner-Kienitz et al., 2001; Hatcher-Solis et al., 2014), we speculated that high expression levels of D1R and D5R in Xenopus oocytes might allow their downstream Gαs/olf proteins to release sufficient amounts of Gβγ to activate GIRK channels, thus allowing us to investigate the putative voltage sensitivities of these receptors. Importantly, previous work has shown that neither G protein dissociation into Gα and Gβγ, nor GIRK activation by Gβγ, are intrinsically voltage dependent processes, thus making GIRK currents a suitable readout for studies of GPCR voltage sensitivity (Ben-Chaim et al., 2003). Here, we report the differential potencies of DA in D1R- and D5R-mediated GIRK activation at -80 and 0 mV.
Wildtype human D1R (from Dr. Marc Caron, Duke University, NC), D5R (from the cDNA Resource Center, Bloomsberg, PA; www.cdna.org) were in pcDNA3.1 while PTX-S1 cDNA (from Dr. Eitan Reuveny, Weizmann Institute of Science, Israel) was in pGEM-HE. GIRK1 (Kir3.1) and GIRK4 (Kir3.4) (GenScript, Piscataway, NJ) cDNA were in pXOOM (provided by Dr. Søren-Peter Olesen, University of Copenhagen, Denmark). Plasmids were linearized using the appropriate restriction enzymes (D1R, D5R; XhoI, PTX-S1; Nhe1 and GIRK1/GIRK4; NotI), followed by in vitro transcription using the T7 mMessage mMachine kit (Ambion, Austin, TX). cRNA concentration and purity were determined by spectrophotometry.
Oocytes from the African clawed toad, Xenopus laevis, were isolated surgically as described previously (Sahlholm et al., 2011). The surgical procedures have been approved by the Swedish National Board for Laboratory Animals and the Stockholm Ethical Committee. Following 24 h of incubation at 12°C, oocytes were injected with 4.5 ng D1R receptor cRNA or 25.5 ng D5R receptor cRNA and 50 pg of each GIRK1 and GIRK4 cRNA, using the Nanoject II (Drummond Scientific, Broomall, PA) and a volume of 50 nl per oocyte. When used, 3 ng PTX-S1 cRNA was injected, based on previous observations of complete inhibition of D2R-induced GIRK activation with this amount of cRNA (Agren et al., 2018a). In a subset of experiments, 10 pmol/oocyte (in a volume of 50 nl) of an antisense DNA oligonucleotide (sequence: GCTCATATTGGCGCAGGTGCAT) directed against X. laevis Gαs mRNA was injected 48 h before electrophysiology recordings. This treatment has previously been described to abolish Gs-dependent signaling in oocytes (de la Pena et al., 1995).
Following cRNA injection into oocytes and 7 days of incubation at 12°C, electrophysiological experiments were conducted using the parallel eight-channel, two-electrode voltage-clamp, OpusXpress 6000A (Molecular Devices, San Jose, CA). Continuous perfusion was maintained at 1.5 ml/min. Data were acquired at membrane potentials of -80 mV or 0 mV and sampled at 134 Hz using the OpusXpress 1.10.42 (Molecular Devices) software. To increase the inward rectifier potassium channel current at negative potentials, a high potassium extracellular buffer was used (in mM: 64 NaCl, 25 KCl, 0.8 MgCl2, 0.4 CaCl2, 15 HEPES, 1 ascorbic acid, adjusted to pH 7.4), yielding a K+ reversal potential of about -40 mV. In experiments with 1 mM KCl, the NaCl concentration was 88 mM. DA was purchased from Sigma-Aldrich (St. Louis, MO). Recordings were performed at room temperature (22 °C).
Electrophysiological data were analyzed in Clampfit 10.6 (Molecular Devices). Concentration-response curves were fitted using least squares nonlinear regression in GraphPad Prism 8 (GraphPad Software, San Diego, CA). For each oocyte and each holding voltage, the current response to each concentration of DA tested was normalized to the response to the maximally effective concentration of DA at the same voltage and in the same oocyte. The following equation was fitted to the normalized agonist data:
where Y is the normalized response, X the logarithm of DA concentration, and n the Hill slope.
The washout decay time constant, τoff, was obtained from single exponential fits (using Levenberg-Marquardt least-squares fitting in Clampfit 10.6) to the washout-induced current deactivation time course. The responses to 1 µM (D1R) and 100 nM (D5R) DA were used for analysis of response deactivation kinetics. The first 10 s following agonist washout were discarded, and the exponential function was fit to the data over ~70% of the response amplitude. koff was calculated from τoff using the following relation:
Data are represented as mean ± SEM. Concentration-response data were analyzed by comparing the fractional responses to DA at individual concentrations at -80 mV and at 0 mV using Student’s paired t-test or, if data were not normally distributed, Wilcoxon signed rank test. Normality was assessed using the Shapiro-Wilk test. Current amplitudes were compared using one-way ANOVA with Tukey’s multiple comparisons test or, if data were not normally distributed, Kruskal-Wallis test with Dunn’s multiple comparisons test. koff rates were compared using the Wilcoxon signed rank test. The significance threshold was p<0.05. Statistical analyses were performed in GraphPad Prism 8.
The effects of DA application on membrane currents were investigated in oocytes co-expressing D1R or D5R with GIRK1/4 channels. DA was found to elicit inward currents at -80 mV, whereas at 0 mV, outward currents were observed. No appreciable current response to DA could be recorded in oocytes expressing D1R or D5R in the absence of GIRK channels at either voltage (Supplementary Figure 1), nor did DA elicit any response in oocytes expressing GIRK channels without D1R or D5R (not shown).
DA potency at D1-like receptors was investigated by DA applications (40-s applications, each followed by 100-s washout) of increasing concentration to oocytes injected with D1R or D5R and GIRK1/4 cRNA. Submaximally effective concentrations were applied, followed by a maximally effective concentration (30 µM for D1R and 3 µM for D5R), at both -80 and 0 mV (Figures 1A, B). At D1R, the DA EC50 was 125 nM at -80 mV, increasing significantly to 906 nM at 0 mV (Figure 1C), a potency shift of about 7-fold. The DA EC50 at D5R was 6.1 nM at -80 mV, again increasing significantly to 44 nM at 0 mV (Figure 1D), resulting in a ~ 7-fold decrease in DA potency. The current–voltage relationships of the membrane currents, as assessed by 4-s ramps and normalized to the amplitude at -80 mV, were virtually superimposable in the absence and in the presence of DA (Supplementary Figure 2).
Figure 1 Voltage-sensitive potency and G protein-dependence of GIRK activation by D1-like receptors. (A) Representative traces of D1R-evoked GIRK currents upon application and washout of 100 nM, 1, and 30 µM DA at 0 (blue) and -80 mV (black). (B) Representative traces of D5R-evoked GIRK currents upon application and washout of 10 nM, 100 nM, and 3 µM DA at 0 (red) and -80 mV (black). (C) Concentration-response curves for D1R-evoked GIRK current at -80 mV and 0 mV. The pEC50 for DA was 6.902 ± 0.120 (125 nM; Hill slope 1.419 ± 0.348) and 6.043 ± 0.078 (906 nM; Hill slope 1.074 ± 0.165) at -80 mV and 0 mV, respectively (n = 3 - 5). *p < 0.05, **p < 0.01, ***p < 0.001, Student’s paired t-test. (D) Concentration-response curves for D5R-evoked GIRK current at -80 mV and 0 mV. The pEC50 for DA was 8.211 ± 0.065 (6 nM; Hill slope 1.301 ± 0.166) and 7.361 ± 0.078 (44 nM; Hill slope 0.959 ± 0.116) at -80 mV and 0 mV, respectively (n = 6). ***p < 0.001, Student’s paired t-test, §p < 0.05, Wilcoxon signed rank test. Curves were obtained by fitting a variable-slope sigmoidal function to the data using least squares nonlinear regression (see Methods). (E) Mean currents (± SEM) evoked by 10 µM DA in oocytes expressing D1R and GIRK1/4 with or without PTX-S1 or Gαs antisense oligonucleotide at 0 mV (top) and -80 mV (bottom). At -80 mV, mean currents were -1.12 ± 0.21 µA (D1R; n = 7), -1.06 ± 0.15 µA (+PTX-S1; n = 7), and -0.43 ± 0.13 µA (+Gαs antisense oligonucleotide; n = 6). At 0 mV, mean currents were 0.025 ± 0.007 µA (D1R; n = 7), 0.020 ± 0.003 µA (+PTX-S1; n = 7), and 0.006 ± 0.004 µA (+Gαs antisense oligonucleotide; n = 7). *; p<0.05, Tukey’s multiple comparisons test. (F) Mean currents (± SEM) evoked by 10 µM DA in oocytes expressing D5R and GIRK1/4 with or without PTX-S1, and Gαs antisense oligonucleotide at 0 mV (top) and -80 mV (bottom). At -80 mV, mean currents were -2.92 ± 0.35 µA (D5R; n = 7), -1.05 ± 0.16 µA (+PTX-S1; n = 6), and 0.012 ± 0.021 µA (+PTX-S1+Gαs antisense oligonucleotide; n = 3). Note that in the presence of antisense oligonucleotide, there was a small deflection in positive direction during DA application. At 0 mV, mean currents were 0.100 ± 0.018 µA (D5R; n = 7), 0.029 ± 0.005 µA (+PTX-S1; n = 6) and 0.006 ± 0.010 µA (+PTX-S1+Gαs antisense oligonucleotide; n = 4). ***p < 0.001, Tukey’s multiple comparisons test. §p < 0.05, §§p < 0.01, Kruskal-Wallis test with Dunn’s multiple comparisons test.
To assess whether GIRK-activation was mediated via Gαi/o proteins, the catalytic subunit of pertussis toxin (PTX-S1), which inactivates Gαi/o proteins by ADP-ribosylation, was expressed in the oocytes (Vivaudou et al., 1997). In addition, oocytes were injected with an antisense oligonucleotide designed to knock down Gαs expression (de la Pena et al., 1995). Co-expression of PTX-S1 with D1R and GIRK1/4 did not significantly affect GIRK response amplitudes to 10 µM DA, neither at -80 mV nor at 0 mV. However, injection of the Gαs antisense oligonucleotide strongly and significantly suppressed the DA-evoked current response by 62% (-80 mV) and 76% (0 mV; Figure 1E). In contrast, in oocytes expressing D5R and GIRK1/4, the amplitudes of GIRK responses to 10 µM DA were significantly reduced by 64% and 71% compared to control at -80 and 0 mV, respectively, when PTX-S1 was introduced. With the further addition of the Gαs antisense oligonucleotide, the DA response was virtually abolished (Figure 1F).
The rate of GIRK response deactivation upon removal of agonist has been used to approximate the time course of agonist dissociation from its receptor (Bunemann et al., 2001; Benians et al., 2003) and changes in the rates of GIRK deactivation between hyperpolarized and depolarized potentials have previously been shown to reflect reciprocal increases or decreases in agonist dissociation rates and consequently, affinities (Ben-Chaim et al., 2003; Ohana et al., 2006; Ben Chaim et al., 2013). Exponential functions were fitted to the time courses of GIRK current deactivation upon DA washout (see Methods). The rate of response decay was observed to increase, both at D1R and at D5R, when the membrane was depolarized from -80 mV to 0 mV (Figure 2). As judged by the rate of current increase (at -80 mV) upon wash-in of buffer containing 25 mM KCl from a baseline reading in buffer containing 1 mM KCl, the rate of solution exchange was faster than the fastest rate of decrease of the GIRK response upon DA washout (Supplementary Figure 3).
Figure 2 Current deactivation rate upon DA washout. Following depolarization, the deactivation rate increased at the D1R (-80 mV; 0.070 ± 0.005 s-1, 0 mV; 0.097 ± 0.011 s-1; n = 12 for both potentials; p = 0.0049, Wilcoxon signed rank test) and D5R (-80 mV; 0.034 ± 0.004 s-1, 0 mV; 0.072 ± 0.009 s-1; n = 15 for both potentials; p < 0.001, Wilcoxon signed rank test) following depolarization. Horizontal bars indicate means ± SEM. **, p = 0.0049; ***, p < 0.001.
Finally, to visualize the change in DA potency when stepping from one voltage to another, we performed experiments in oocytes co-expressing D1R with GIRK1/4 channels where a 40-s prepulse to between -80 to 0 mV was followed by a -80 mV post-pulse, in the absence or presence of an intermediately- (300 nM) or a maximally (30 µM) effective concentration of DA. Subtracting the basal, agonist-independent current from the current evoked in the presence of agonist, a slow current increase at -80 mV was evident following depolarized prepulses in the presence of 300 nM, but not 30 µM DA (Supplementary Figure 4). This behavior of GIRK currents, which has been referred to as “relaxation”, has earlier been demonstrated to be a consequence of receptor voltage sensitivity and to reflect the increase in agonist binding to the receptor, at submaximally effective concentrations, upon hyperpolarization of the membrane (Moreno-Galindo et al., 2011; Sahlholm, 2011).
Previous investigations have found evidence for reduced agonist potencies and affinities at depolarized potentials in several Gαi/o-coupled GPCRs, including M2R, D2R, histamine H3 and H4, and the metabotropic glutamate 3 receptor (mGluR3) (Ben-Chaim et al., 2003; Ben-Chaim et al., 2006; Sahlholm et al., 2008b; Sahlholm et al., 2012), as well as the Gαs-coupled β-adrenergic receptors (Birk et al., 2015). Conversely, a number of Gαq-coupled muscarinic, metabotropic glutamate, and prostanoid receptors demonstrated increased agonist potencies at depolarized potentials (Ben-Chaim et al., 2003; Ohana et al., 2006; Kurz et al., 2020). The potency shifts of 7-fold between -80 and 0 mV observed here for D1R and D5R are among the more pronounced shifts reported so far.
Compared to the D2-like DA receptors previously investigated (Sahlholm et al., 2008a), D5R and D1R present the strongest reductions in DA potency (~7-fold) between -80 and 0 mV, whereas D2R showed a somewhat smaller potency reduction of about 4-fold. D4R and D3R were the least sensitive, with ~2-fold and 1.1-fold (non-significant) lower DA potency at 0 compared to -80 mV (Sahlholm et al., 2008a). DA concentrations in the striatum vary between the low nanomolar range at extrasynaptic sites (corresponding to tonic DA signaling), to high micromolar concentrations in the immediate vicinity of active DA terminals (phasic DA signaling) (Marcott et al., 2014; Bamford et al., 2018). Assuming the in vivo EC50s of D1R and D5R to be similar to those observed here, one would expect the voltage sensitivity of D1R to be more relevant during phasic DA signaling, when DA concentrations transiently reach sufficiently high concentrations to activate this receptor. Conversely, the influence of voltage over D5R signaling might be more pronounced under tonic DA signaling conditions, which would be expected to produce a sub-saturating D5R response. Given the involvement of both D1R and D5R in learning and memory, it is tempting to speculate that their sensitivity to voltage may enable these receptors to function as a form of coincidence detectors, reporting on both the membrane potential and the presence of DA. For example, strong depolarization of the postsynaptic neuron may spatially restrict the signaling of D1-like receptors by allowing for efficient activation only of those receptors which are located sufficiently close to active DA terminals.
Overexpression of Gs-coupled receptors has been reported to support activation of GIRK channels, although much less efficiently than Gi/o-coupled receptors, in several expression systems including Xenopus oocytes (Lim et al., 1995; Wellner-Kienitz et al., 2001; Hatcher-Solis et al., 2014). Thus, we believe that the PTX-insensitive D1R-mediated GIRK activation observed here is likely to be elicited via Gs signaling, although this phenomenon may be a consequence of receptor overexpression and unlikely to take place in native tissue. Indeed, this conclusion is strengthened by the observation that injection of an antisense oligonucleotide directed towards X. laevis mRNA encoding Gαs strongly reduced the current response to DA in oocytes co-expressing D1R and GIRK channels.
Interestingly, in contrast to the D1R-evoked responses, a major component of the GIRK currents elicited upon D5R stimulation was PTX-sensitive, suggesting that D5R is able to activate Gi/o proteins in addition to Gs/olf. While D5R-mediated Gq signaling has been observed in some heterologous systems (So et al., 2009), Gi/o-coupling of D5R has, to the best of our knowledge, not been reported previously. In Xenopus oocytes, Gq-mediated calcium signaling would typically elicit a characteristic, rapidly desensitizing response mediated through endogenous calcium-activated chloride channels (Hansen and Bräuner-Osborne, 2009); however, we did not observe any such responses upon D5R activation.
Contrary to the present observations, D1R has previously been reported to couple to PTX-sensitive Go proteins in addition to Gs, while D5R was found to couple to the PTX-insensitive inhibitory G protein, Gz (Sidhu et al., 1998), which efficiently activates GIRK (Vorobiov et al., 2000). However, available evidence suggests that there is no detectable endogenous Gz expression in Xenopus oocytes (Vorobiov et al., 2000; Kalinowski et al., 2003), making it unlikely that Gz activation underlies the PTX-insensitive component of D5R-mediated GIRK activation observed here. Instead, it appears more likely that this component is Gs-mediated. Again, this assumption is strengthened by the abolition of the DA-induced current response by injection of Gαs antisense oligonucleotide into oocytes co-expressing D5R and GIRK with PTX-S1.
The depolarization-induced decrease in acetylcholine potency at the M2R has been related to a corresponding increase in acetylcholine koff at the M2R, and a consequent increase in GIRK response deactivation rate at depolarized potentials (Ben-Chaim et al., 2003; Ben Chaim et al., 2013; Agren et al., 2018b; López-Serrano et al., 2020). Similar findings have been reported for the mGluR3 and histamine H3 receptors (Ohana et al., 2006; Sahlholm et al., 2012). Likewise, in the present study, we found the rates of GIRK current deactivation upon DA washout to be increased at 0 mV compared to -80 mV. Presumably, these changes in deactivation rates reflect faster DA koffs at D1R and D5R at 0 mV.
Finally, it should be emphasized that the oocyte expression system does not represent a native environment for D1R and D5R, which interact with a host of other proteins in neurons and other tissues. However, this heterologous system provides a well-defined background with no detectable expression of endogenous DA receptors which lends itself well to stable voltage-clamp recordings and detection of even relatively small agonist-induced responses with a high signal-to-noise ratio. The DA EC50s for D1R- and D5R-induced GIRK activation at 0 mV in the present study agree fairly well with the reported values for high-affinity DA binding in isolated membranes in the literature, recapitulating the higher affinity of D5R (24 nM; Weinshank et al., 1991) compared to D1R (324 nM; Marcellino et al., 2012), suggesting that the DA binding characteristics of these receptors in oocytes are similar to those of D1R and D5R expressed in mammalian cells.
The present results reveal that DA potency at D1R and D5R is decreased upon depolarization from -80 to 0 mV. Our interpretation is that DA koff rate constants are affected by membrane voltage and contribute to a decrease in DA affinity upon depolarization. This dependence of D1R and D5R-mediated responses upon transmembrane voltage could allow these receptors, which have been implicated in learning and memory, to function as a sort of “coincidence detectors”, responding robustly to low concentrations of DA only at hyperpolarized potentials.
The raw data supporting the conclusions of this article will be made available by the authors, without undue reservation.
The animal study was reviewed and approved by Swedish National Board for Laboratory Animals, Stockholm Ethical Committee (Stockholms djurförsöksetiska nämnd).
RÅ and KS designed the experiments, RÅ conducted the experiments, RÅ and KS analyzed data, RÅ drafted the first version of the manuscript. KS supervised the project. All authors contributed to the article and approved the submitted version.
This study was supported by grants from Stiftelsen Lars Hiertas Minne, Åhlénstiftelsen, and Magnus Bergvalls stiftelse (to KS). KS is currently a fellow at the Wallenberg Center for Molecular Medicine at Umeå University.
The authors declare that the research was conducted in the absence of any commercial or financial relationships that could be construed as a potential conflict of interest.
The authors wish to thank prof. Paolo Frumento, Università di Pisa, Italy, for helpful consultations.
The Supplementary Material for this article can be found online at: https://www.frontiersin.org/articles/10.3389/fphar.2020.581151/full#supplementary-material
Agren, R., Arhem, P., Nilsson, J., Sahlholm, K. (2018a). The Beta-Arrestin-Biased Dopamine D2 Receptor Ligand, UNC9994, Is a Partial Agonist at G-Protein-Mediated Potassium Channel Activation. Int. J. Neuropsychopharmacol. 21, 1102–1108. doi: 10.1093/ijnp/pyy059
Agren, R., Sahlholm, K., Nilsson, J., Arhem, P. (2018b). Point mutation of a conserved aspartate, D69, in the muscarinic M2 receptor does not modify voltage-sensitive agonist potency. Biochem. Biophys. Res. Commun. 496, 101–104. doi: 10.1016/j.bbrc.2018.01.005
Arnsten, A. F. T., Vijayraghavan, S., Wang, M., Gamo, N. J., Paspalas, C. D. (2010). “Dopamine’s influence on prefrontal cortical cognition: actions and circuits in behaving primates,” in Dopamine handbook (New York: Oxford University Press).
Bamford, N. S., Wightman, R. M., Sulzer, D. (2018). Dopamine’s Effects on Corticostriatal Synapses during Reward-Based Behaviors. Neuron 97, 494–510. doi: 10.1016/j.neuron.2018.01.006
Ben-Chaim, Y., Chanda, B., Dascal, N., Bezanilla, F., Parnas, I., Parnas, H. (2006). Movement of ‘gating charge’ is coupled to ligand binding in a G-protein-coupled receptor. Nature 444, 106–109. doi: 10.1038/nature05259
Ben-Chaim, Y., Tour, O., Dascal, N., Parnas, I., Parnas, H. (2003). The M2 muscarinic G-protein-coupled receptor is voltage-sensitive. J. Biol. Chem. 278, 22482–22491. doi: 10.1074/jbc.M301146200
Ben Chaim, Y., Bochnik, S., Parnas, I., Parnas, H. (2013). Voltage affects the dissociation rate constant of the m2 muscarinic receptor. PLoS One 8, e74354. doi: 10.1371/journal.pone.0074354
Benians, A., Leaney, J. L., Tinker, A. (2003). Agonist unbinding from receptor dictates the nature of deactivation kinetics of G protein-gated K+ channels. Proc. Natl. Acad. Sci. 100, 6239. doi: 10.1073/pnas.1037595100
Birk, A., Rinne, A., Bünemann, M. (2015). Membrane Potential Controls the Efficacy of Catecholamine-induced β1-Adrenoceptor Activity. J. Biol. Chem. 290, 27311–27320. doi: 10.1074/jbc.M115.665000
Bruns, R. F., Mitchell, S. N., Wafford, K. A., Harper, A. J., Shanks, E. A., Carter, G., et al. (2018). Preclinical profile of a dopamine D1 potentiator suggests therapeutic utility in neurological and psychiatric disorders. Neuropharmacology 128, 351–365. doi: 10.1016/j.neuropharm.2017.10.032
Bunemann, M., Bucheler, M. M., Philipp, M., Lohse, M. J., Hein, L. (2001). Activation and deactivation kinetics of alpha 2A- and alpha 2C-adrenergic receptor-activated G protein-activated inwardly rectifying K+ channel currents. J. Biol. Chem. 276, 47512–47517. doi: 10.1074/jbc.M108652200
Carr, G. V., Maltese, F., Sibley, D. R., Weinberger, D. R., Papaleo, F. (2017). The Dopamine D5 Receptor Is Involved in Working Memory. Front. Pharmacol. 8, 666. doi: 10.3389/fphar.2017.00666
de la Pena, P., Del Camino, D., Pardo, L. A., Dominguez, P., Barros, F. (1995). Gs couples thyrotropin-releasing hormone receptors expressed in Xenopus oocytes to phospholipase C. J. Biol. Chem. 270, 3554–3559. doi: 10.1074/jbc.270.8.3554
Goldman-Rakic, P. S., Castner, S. A., Svensson, T. H., Siever, L. J., Williams, G. V. (2004). Targeting the dopamine D1 receptor in schizophrenia: insights for cognitive dysfunction. Psychopharmacol. (Berl.) 174, 3–16. doi: 10.1007/s00213-004-1793-y
Hansen, K. B., Bräuner-Osborne, H. (2009). Xenopus oocyte electrophysiology in GPCR drug discovery. Methods Mol. Biol. 552, 343–357. doi: 10.1007/978-1-60327-317-6_25
Hatcher-Solis, C., Fribourg, M., Spyridaki, K., Younkin, J., Ellaithy, A., Xiang, G., et al. (2014). G protein-coupled receptor signaling to Kir channels in Xenopus oocytes. Curr. Pharm. Biotechnol. 15, 987–995. doi: 10.2174/1389201015666141031111916
Huang, X., Lawler, C. P., Lewis, M. M., Nichols, D. E., Mailman, R. B. (2001). D1 dopamine receptors. Int. Rev. Neurobiol. 48, 65–139. doi: 10.1016/S0074-7742(01)48014-7
Kalinowski, R. R., Jaffe, L. A., Foltz, K. R., Giusti, A. F. (2003). A receptor linked to a Gi-family G-protein functions in initiating oocyte maturation in starfish but not frogs. Dev. Biol. 253, 139–149. doi: 10.1006/dbio.2002.0860
Kurz, M., Krett, A.-L., Bünemann, M. (2020). Voltage Dependence of Prostanoid Receptors. Mol. Pharmacol. 97, 267. doi: 10.1124/mol.119.118372
Lewis, M. A., Hunihan, L., Watson, J., Gentles, R. G., Hu, S., Huang, Y., et al. (2015). Discovery of D1 Dopamine Receptor Positive Allosteric Modulators: Characterization of Pharmacology and Identification of Residues that Regulate Species Selectivity. J. Pharmacol. Exp. Ther. 354, 340–349. doi: 10.1124/jpet.115.224071
Lim, N. F., Dascal, N., Labarca, C., Davidson, N., Lester, H. A. (1995). A G protein-gated K channel is activated via beta 2-adrenergic receptors and G beta gamma subunits in Xenopus oocytes. J. Gen. Physiol. 105, 421–439. doi: 10.1085/jgp.105.3.421
López-Serrano, A. L., De Jesús-Pérez, J. J., Zamora-Cárdenas, R., Ferrer, T., Rodríguez-Menchaca, A. A., Tristani-Firouzi, M., et al. (2020). Voltage-induced structural modifications on M2 muscarinic receptor and their functional implications when interacting with the superagonist iperoxo. Biochem. Pharmacol. 177, 113961. doi: 10.1016/j.bcp.2020.113961
Luderman, K. D., Conroy, J. L., Free, R. B., Southall, N., Ferrer, M., Sanchez-Soto, M., et al. (2018). Identification of Positive Allosteric Modulators of the D1 Dopamine Receptor That Act at Diverse Binding Sites. Mol. Pharmacol. 94, 1197–1209. doi: 10.1124/mol.118.113175
Marcellino, D., Kehr, J., Agnati, L. F., Fuxe, K. (2012). Increased affinity of dopamine for D(2) -like versus D(1) -like receptors. Relevance for volume transmission in interpreting PET findings. Synapse 66, 196–203. doi: 10.1002/syn.21501
Marcott, P. F., Mamaligas, A. A., Ford, C. P. (2014). Phasic dopamine release drives rapid activation of striatal D2-receptors. Neuron 84, 164–176. doi: 10.1016/j.neuron.2014.08.058
Moreno-Galindo, E. G., Sanchez-Chapula, J. A., Sachse, F. B., Rodriguez-Paredes, J. A., Tristani-Firouzi, M., Navarro-Polanco, R. A. (2011). Relaxation gating of the acetylcholine-activated inward rectifier K+ current is mediated by intrinsic voltage sensitivity of the muscarinic receptor. J. Physiol. 589, 1755–1767. doi: 10.1113/jphysiol.2010.204115
Ohana, L., Barchad, O., Parnas, I., Parnas, H. (2006). The metabotropic glutamate G-protein-coupled receptors mGluR3 and mGluR1a are voltage-sensitive. J. Biol. Chem. 281, 24204–24215. doi: 10.1074/jbc.M513447200
Sahlholm, K. (2011). The role of RGS protein in agonist-dependent relaxation of GIRK currents in Xenopus oocytes. Biochem. Biophys. Res. Commun. 415, 509–514. doi: 10.1016/j.bbrc.2011.10.106
Sahlholm, K., Barchad-Avitzur, O., Marcellino, D., Gomez-Soler, M., Fuxe, K., Ciruela, F., et al. (2011). Agonist-specific voltage sensitivity at the dopamine D2S receptor–molecular determinants and relevance to therapeutic ligands. Neuropharmacology 61, 937–949. doi: 10.1016/j.neuropharm.2011.06.022
Sahlholm, K., Marcellino, D., Nilsson, J., Fuxe, K., Arhem, P. (2008a). Differential voltage-sensitivity of D2-like dopamine receptors. Biochem. Biophys. Res. Commun. 374, 496–501. doi: 10.1016/j.bbrc.2008.07.052
Sahlholm, K., Nilsson, J., Marcellino, D., Fuxe, K., Arhem, P. (2008b). Voltage-dependence of the human dopamine D2 receptor. Synapse 62, 476–480. doi: 10.1002/syn.20509
Sahlholm, K., Nilsson, J., Marcellino, D., Fuxe, K., Arhem, P. (2012). Voltage sensitivities and deactivation kinetics of histamine H(3) and H(4) receptors. Biochim. Biophys. Acta 1818, 3081–3089. doi: 10.1016/j.bbamem.2012.07.027
Sarinana, J., Kitamura, T., Kunzler, P., Sultzman, L., Tonegawa, S. (2014). Differential roles of the dopamine 1-class receptors, D1R and D5R, in hippocampal dependent memory. Proc. Natl. Acad. Sci. U. S. A. 111, 8245–8250. doi: 10.1073/pnas.1407395111
Sawaguchi, T., Goldman-Rakic, P. S. (1994). The role of D1-dopamine receptor in working memory: local injections of dopamine antagonists into the prefrontal cortex of rhesus monkeys performing an oculomotor delayed-response task. J. Neurophysiol. 71, 515–528. doi: 10.1152/jn.1994.71.2.515
Sidhu, A., Kimura, K., Uh, M., White, B. H., Patel, S. (1998). Multiple coupling of human D5 dopamine receptors to guanine nucleotide binding proteins Gs and Gz. J. Neurochem. 70, 2459–2467. doi: 10.1046/j.1471-4159.1998.70062459.x
So, C. H., Verma, V., Alijaniaram, M., Cheng, R., Rashid, A. J., O’dowd, B. F., et al. (2009). Calcium signaling by dopamine D5 receptor and D5-D2 receptor hetero-oligomers occurs by a mechanism distinct from that for dopamine D1-D2 receptor hetero-oligomers. Mol. Pharmacol. 75, 843–854. doi: 10.1124/mol.108.051805
Touhara, K. K., MacKinnon, R. (2018). Molecular basis of signaling specificity between GIRK channels and GPCRs. eLife 7, e42908. doi: 10.7554/eLife.42908
Vivaudou, M., Chan, K. W., Sui, J. L., Jan, L. Y., Reuveny, E., Logothetis, D. E. (1997). Probing the G-protein regulation of GIRK1 and GIRK4, the two subunits of the KACh channel, using functional homomeric mutants. J. Biol. Chem. 272, 31553–31560. doi: 10.1074/jbc.272.50.31553
Vorobiov, D., Bera, A. K., Keren-Raifman, T., Barzilai, R., Dascal, N. (2000). Coupling of the muscarinic m2 receptor to G protein-activated K(+) channels via Galpha(z) and a receptor-Galpha(z) fusion protein. Fusion between the receptor and Galpha(z) eliminates catalytic (collision) coupling. J. Biol. Chem. 275, 4166–4170. doi: 10.1074/jbc.275.6.4166
Weinshank, R. L., Adham, N., Macchi, M., Olsen, M. A., Branchek, T. A., Hartig, P. R. (1991). Molecular cloning and characterization of a high affinity dopamine receptor (D1 beta) and its pseudogene. J. Biol. Chem. 266, 22427–22435.
Wellner-Kienitz, M. C., Bender, K., Pott, L. (2001). Overexpression of beta 1 and beta 2 adrenergic receptors in rat atrial myocytes. Differential coupling to G protein-gated inward rectifier K(+) channels via G(s) and G(i)/o. J. Biol. Chem. 276, 37347–37354. doi: 10.1074/jbc.M106234200
Keywords: dopamine D1 receptor (D1R), voltage sensitivity, G protein-coupled receptor, GIRK channels, binding kinetics, G protein selectivity, dopamine D5 receptor (D5R)
Citation: Ågren R and Sahlholm K (2020) Voltage-Dependent Dopamine Potency at D1-Like Dopamine Receptors. Front. Pharmacol. 11:581151. doi: 10.3389/fphar.2020.581151
Received: 08 July 2020; Accepted: 16 September 2020;
Published: 07 October 2020.
Edited by:
Murat Oz, Health Science Center Kuwait, KuwaitReviewed by:
Nathan Dascal, Tel Aviv University, IsraelCopyright © 2020 Ågren and Sahlholm. This is an open-access article distributed under the terms of the Creative Commons Attribution License (CC BY). The use, distribution or reproduction in other forums is permitted, provided the original author(s) and the copyright owner(s) are credited and that the original publication in this journal is cited, in accordance with accepted academic practice. No use, distribution or reproduction is permitted which does not comply with these terms.
*Correspondence: Kristoffer Sahlholm, a3Jpc3RvZmZlci5zYWhsaG9sbUB1bXUuc2U=
Disclaimer: All claims expressed in this article are solely those of the authors and do not necessarily represent those of their affiliated organizations, or those of the publisher, the editors and the reviewers. Any product that may be evaluated in this article or claim that may be made by its manufacturer is not guaranteed or endorsed by the publisher.
Research integrity at Frontiers
Learn more about the work of our research integrity team to safeguard the quality of each article we publish.