- 1Institute of Translational Medicine, The First Hospital, Jilin University, Changchun, China
- 2Department of Urology, The First Hospital of Jilin University, Changchun, China
- 3Department of Traditional Chinese Medicine, The First Hospital of Jilin University, Changchun, China
- 4Department of Respiratory Medicine, The First Hospital of Jilin University, Changchun, China
Cisplatin (CDDP) is a widely used drug for cancer treatment that exhibits major side effects in normal tissues, such as nephrotoxicity in kidneys. The Nrf2 signaling pathway, a regulator of mitochondrial dysfunction, oxidative stress and inflammation, is a potential therapeutic target in CDDP-induced nephrotoxicity. We explored the underlying mechanisms in wild-type (WT) and Nrf2−/− mice on CDDP-induced renal dysfunction in vivo. We found that Nrf2 deficiency aggravated CDDP-induced nephrotoxicity, and Daph treatment significantly ameliorated the renal injury characterized by biochemical markers in WT mice and reduced the CDDP-induced cell damage. In terms of the mechanism, Daph upregulated the SIRT1 and SIRT6 expression in vivo and in vitro. Furthermore, Daph inhibited the expression level of NOX4, whereas it activated Nrf2 translocation and antioxidant enzymes HO-1 and NQO1, and alleviated oxidative stress and mitochondrial dysfunction. Moreover, Daph suppressed CDDP-induced NF-κB and MAPK inflammation pathways, as well as p53 and cleaved caspase-3 apoptosis pathways. Notably, the protective effects of Daph in WT mice were completely abrogated in Nrf2−/− mice. Moreover, Daph enhanced, rather than attenuated, the tumoricidal effect of CDDP.
Introduction
Cisplatin (CDDP), an inorganic platinum compound, is a common and effective chemotherapeutic drug for the treatment of different types of solid tumors in the head and neck, lung, bladder, ovaries and testicles (Wang and Lippard 2005; Pabla and Dong 2008). However, the serious side effects of CDDP, especially nephrotoxicity, limit its clinical applications. Although extensive hydration can reduce renal injury, more than a quarter of patients develop renal problems, leading to renal dysfunction and acute renal failure (Kuriakose and Kurup 2008). Therefore, it is necessary to identify effective therapeutic methods or agents to reduce the nephrotoxicity induced by CDDP.
In addition to DNA damage, a positive feedback loop among oxidative stress, inflammation, and apoptosis leads to tubular toxicity and vascular injury, which is a major cause of CDDP-induced renal injury (Jamieson and Lippard 1999; Zhang et al., 2008; Pabla et al., 2009). CDDP nephrotoxicity is characterized by the activation of proinflammatory cytokines and chemokines, and the inhibition of mitogen-activated protein kinases, such as extracellular signal-regulated kinase (ERK), p38, and c-jun NH(2)-terminal kinase (JNK), and nuclear factor-kappaB (NF-κB) alleviated CDDP-induced kidney injury via inhibiting the inflammatory response (Potocnjak et al., 2016; Yu et al., 2018). Moreover, tubular cell apoptosis is a characteristic feature of CDDP nephrotoxicity, and inhibition of CDDP-induced the upregulation levels of Bax and p53 and downregulation level of Bcl-2 protects CDDP-induced kidney injury by inhibiting apoptosis (Taira and Yoshida 2012; Wang et al., 2018). Oxidative stress is defined as the production of free radicals and reactive metabolites (referred as oxidants or reactive oxygen species, ROS) and protective mechanisms can be initiated (called antioxidants) to eliminate the imbalance between them. ROS imbalance is directly associated with inflammation, mitochondrial dysfunction and cell apoptosis (Reuter et al., 2010; Wu et al., 2018). Nuclear factor (erythroid-derived 2)-like 2/antioxidant response element (Nrf2/ARE) pathway is the major pathway that responds to ROS by activating phase II detoxification enzymes at the transcriptional level, and the activation of this pathway is one of the main defense mechanisms against oxidative stress. Previous studies have shown that Nrf2-deficiency enhances susceptibility to CDDP-induced nephrotoxicity, and Nrf2 may play a key role to prevent renal injury (Liu et al., 2009; Aleksunes et al., 2010).
Sirtuins are an evolutionarily conserved family of NAD+-dependent deacetylases with a variety of cellular functions associated with mitochondrial energy homeostasis and antioxidant activity. Seven sirtuins (SIRT1-7) are expressed in different subcellular regions of mammalians and play a vital role in variety of diseases, including metabolic syndrome, diabetes and cancer. In the kidney, the most widely studied sirtuin is SIRT1, which is a nuclear sirtuin and regulates nucleosome histone acetylation and activity of several transcriptional factors, exerting cytoprotective effects by inhibiting inflammation, apoptosis and fibrosis (Vaquero et al., 2004). Based on these activities, SIRT1 deficits of aged mice result in increased susceptibility to CDDP-induced acute kidney injury (Guan et al., 2017). For other nuclear sirtuins, SIRT6, which exert antioxidant activity, has been shown to recruit sites for DNA double-strand breaks, promoting its repair through ADP-ribosylation (Lerrer et al., 2016). Furthermore, SIRT6 attenuated CDDP-induced acute kidney injury by inhibiting ERK1/2 expression (Li et al., 2018). Recently, studies have indicated that SIRT1 might exert antioxidant activity, while SIRT6 could have alleviated DNA damage. Therefore, the activity of SIRT1 and SIRT6 were increased to regulate the Nrf2 antioxidant pathway (Mao et al., 2011; Meng et al., 2015).
Although young transgenic Nrf2 knockout mice did not show crucial total phenotypic perturbations in the absence of chemical or pathological stress, it has been shown that Nrf2 deficiency leads to the development of lupus-like autoimmune nephritis in aged female mice (Yoh et al., 2001). Nrf2 knockout mice are also more susceptible to exposure to hyperglycemia and streptozotocin-induced diabetic nephropathy (Yoh et al., 2008; Jiang et al., 2010). In view of these findings, and in light of the pharmacological induction of Nrf2, it has been shown to reduce the pathological burden of chronic kidney disease (Pergola et al., 2011), renal fibrosis (Oh et al., 2012), diabetic nephropathy (de Haan 2011) and focal segmental glomerulosclerosis (Tsai et al., 2011) in animal models. Therefore, targeting Nrf2 as a therapeutic strategy will be of great significance to human renal diseases (Ruiz et al., 2013).
As a major Nrf2 activator, natural products exert antioxidant activity by modulating the Nrf2/ARE pathway. Daph is a natural coumarin derivative isolated from the genus Daphne, clinically used to treat rheumatoid arthritis and coagulopathy (Liang et al., 2010). Our previous studies indicated that Daph activated Nrf2 antioxidant pathways and ameliorated tertbutylhydroperoxide (t-BHP)-induced mitochondrial dysfunction and cell death (Lv et al., 2017). Furthermore, we also found that Daph had protective effects against lipopolysaccharide/d-galactosamine-induced acute liver failure via inhibition of NLRP3, MAPK and NF-κB (Lv et al., 2018). However, the molecular mechanisms of Daph against CDDP-induced nephrotoxicity have not previously been illuminated. Therefore, the aim of this study was to investigate the protective effects, molecular mechanisms and potential targets of Daph on CDDP-induced nephrotoxicity.
Materials and Methods
Reagents and Antibodies
Daph (purity > 98%) was obtained from the Chengdu Pufei De Biotech Co., Ltd. Primary antibodies directed against SIRT1 andSIRT6 were obtained from Santa Cruz. CDDP and the inhibitors of SIRT1 and SIRT6 were supplied by Medchem express. Antibodies against NOX4, Nrf2, NQO1, HO-1,β-actin, GAPDH, Lamin B, p-JNK, JNK, p-ERK, ERK, p-p38, p38, p65, HMGB1, p53, Caspase-3, BAX, BCL-2were obtained from Abcam or Cell Signaling. Hoechst 33342 was purchased from Invitrogen. The horseradish peroxidase (HRP)-conjugated anti-rabbit and anti-mouse IgG were purchased from Proteintech. Additionally, BUN, CRE, GSH, MDA, MPO and SOD test kits were purchased from Nanjing Jiancheng Bioengineering Institute.
Animals
C57BL/6 WT mice (Liaoning Changsheng Technology Industrial, Co., Ltd. Certificate SCXK2015-0001) and Nrf2 knockout mice (The Jackson Laboratory, Bar Harbor, ME, United States) were housed in a 12-h dark/12-h light circle and allowed free access to standard diet and water unless specifically indicated. All animal studies were reviewed and approved by the Animal Ethical Committee of Jilin University.
Cisplatin-Induced AKI
Five group mice were randomly divided: the control, CDDP only (10 or 20 mg/kg) and CDDP (20 mg/kg) + Daph (40 mg/kg) or NAC (50 mg/kg), Daph (40 mg/kg). The mice were fasted and Daph or NAC was i.p. treated for three consecutive days. One hour after the first Daph or NAC treatment, CDDP or saline was administrated. After 72 h, the blood and kidney tissues were collected to evaluate biochemical analysis, pathological changes and related mechanism.
Histological Assessment and Terminal Deoxynucleotidyltransferase-Mediated dUTP Nick End-Labeling Assay
Fresh kidney tissues were fixed in Neutral-buffered formalin and paraffin-embedded sections were stained with HE and TUNEL, images were collected and analyzed using a microscope and fluorescence microscope. The pathological index was scored based on the percentage of tubules with necrosis, detachment, dilation, cell swelling, or cast formation.
Biochemical Analysis
The blood and kidney tissues were collected to evaluate biochemical analysis. Serum BUN, serum creatinine, GSH, SOD, MDA and MPO levels were assessed using an assay kit according to the manufacturer’s instructions.
Cell Culture and CCK-8 Analysis
Human renal proximal tubule cells (HK-2) supplied by the China Cell Line Bank and cultured in 1,640 medium with 10% FBS, 100 U/mL of penicillin, 100 U/mL of streptomycin and 3 mM glutamine at 37°C in a 5% CO2 atmosphere. HK-2 cells seeded onto 96-well plates for cell viability. One hour before treated with or without CDDP (25 μM), cells were treated with Daph (2.5, 5 or 10 μg/ml). After 18 h, cell viability was detected by Cell Counting Kit-8.
Hoechst 33342/PI Staining
HK-2 cells were seeded onto 12-well plates. After treatments, Hoechst 33342 and PI were added for 15 min at 37°C. After washing with warm PBS twice, the cells were immediately detected by a fluorescence microscope.
Quantification of Apoptotic and Necrosis Cells
The quantification of apoptotic and necrosis cells was measured by flow cytometry. After treatments, the cells were washed with PBS, Annexin Ⅴ and propidium iodide were added. The percentage of apoptosis and necrosis were detected by flow cytometry.
Measurement of Reactive Oxygen Species Generation
HK2 cells were seeded onto 96-well plates. Cells were treated with or without different dosages of Daph (2.5, 5 or 10 μg/ml) for 18 h, CDDP was administrated for 10 min, then DCFH-DA were incubated for 40 min at 37°C. After washing with warm PBS twice, the cells were immediately detected by a microplate reader and a fluorescence microscope.
Western Blotting Analysis
Kidney tissues and HK2 cells were lyzed in RIPA lysis buffer with protease and phosphatase inhibitors for 30 min. An NE-PER Nuclear and Cytoplasmic Extraction Reagent Kit was employed to obtain nuclear and cytoplasmic protein. To obtain the mitochondria protein, a Mitochondria Isolation Kit was employed. A BCA protein assay kit was employed to determined protein concentrations. Protein samples were analyzed by SDS-poly-acrylamide gel and transferred onto PVDF membrane. Then, the membranes blocked with 5% nonfat milk, and incubated with primary antibodies overnight. The membranes were washed for three times and incubated with secondary antibodies for 1 h, followed by detected with ECL. The data was analyzed by ImageJ software.
Statistical Analysis
All data are expressed as means ± SD. These results were employed one-way analysis of variance (ANOVA) and unpaired Student’s t test. Value of p < 0.05 was considered significant.
Results
Nrf2 Deficiency Aggravates Cisplatin-Induced AKI
A dose of 20 mg/kg CDDP successfully induced AKI in the WT and Nrf2−/− mice (Figure 1A). The kidney function was evaluated by the bodyweight, kidney index, BUN and serum creatinine (72 h of CDDP). The lost bodyweight, and the increase of kidney index, BUN and creatinine were higher in Nrf2−/− KI mice than those in WT mice (Figures 1B–E). In the group of CDDP, macroscopic kidneys exhibited whitening, which confirmed that Nrf2−/− mice were susceptible to toxin insult (Figure 1F). Consistently, the histological assessment of the kidneys indicated a significantly worse tubular injury in the Nrf2−/− mice than it in the WT mice, including severe dilation of the proximal tubules, cast formation, and massive detachment and necrosis of the tubular epithelium (Figures 1G,H).
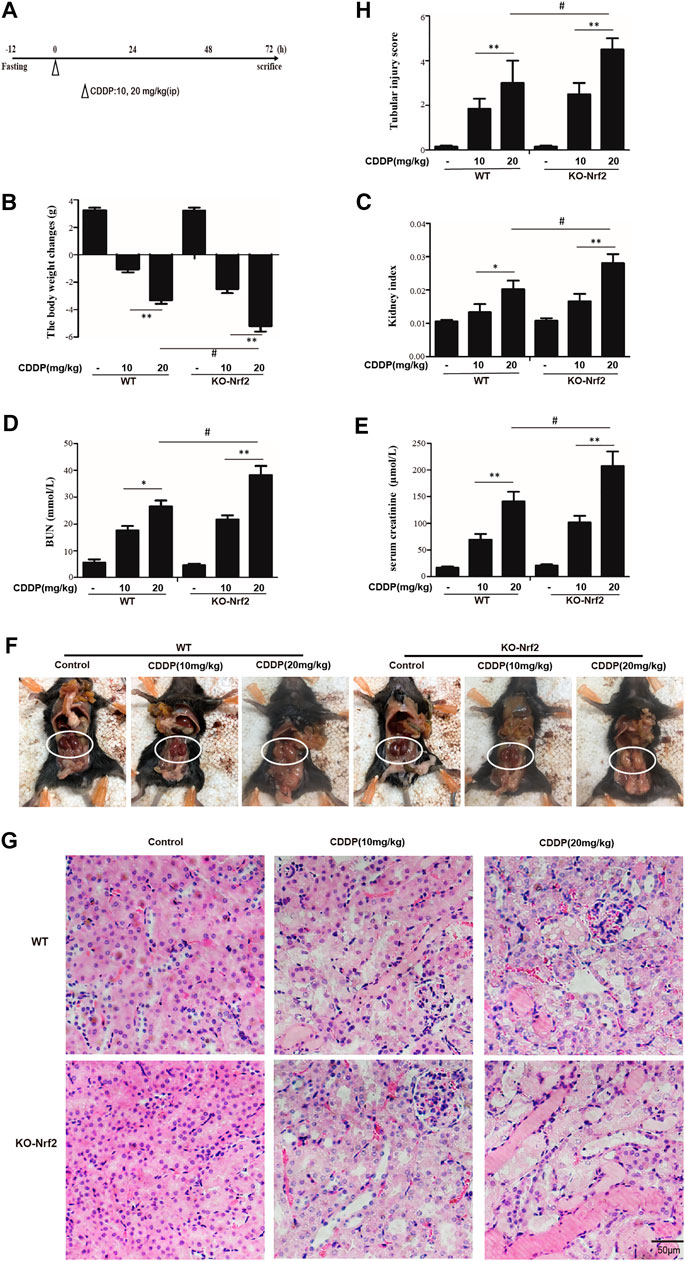
FIGURE 1. Nrf2−/− mice are more susceptible to CDDP-induced AKI. (A) An acute kidney injury model was induced 3 days after i.p. injection of CDDP (10, 20 mg/kg). WT mice and Nrf2 knockout (KO) mice treated with CDDP (10, 20 mg/kg) are shown. (B) Body weight and (C) Kidney/body weight ratio. (D,E) BUN and serum creatinine were measured. (F) macroscopic kidney and (G) hematoxylin and eosin (H&E). (H) Tubular injury scores for kidney damage. *p < 0.05, **p < 0.01.
Daph Effectively Ameliorates Cisplatin-Induced AKI in Wild-Type Mice
Our previous studies found that Daph, as the main Nrf2 activator, exert antioxidant activity by regulating the Nrf2/ARE pathway (Lv et al., 2017; Lv et al., 2018). We utilized it to investigate its protective effects on CDDP-induced nephrotoxicity. After fasting for 12 h, the mice received a single dose of CDDP alone (20 mg/kg) to induce AKI or in combination with the ip administration of Daph for three times (Figure 2B). As indicated in Figures 2C–I, the administration of Daph significantly reversed the CDDP-induced loss of body weight and the high levels of kidney index, BUN and serum creatinine and tubular necrosis.
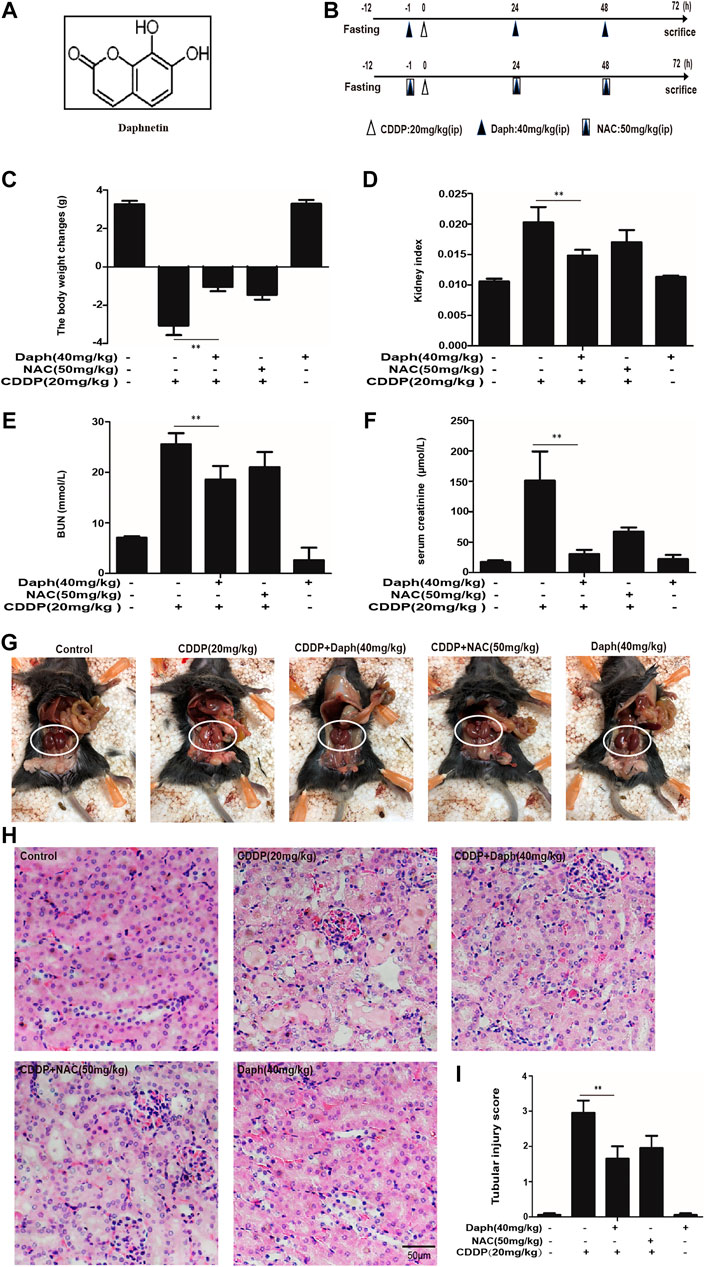
FIGURE 2. Daph protects against CDDP-induced AKI in WT mice. (A) The molecular structure of Daph. (B) Schematic schedule for CDDP alone and combined with Daph or NAC treatments in vivo. Mice were treated with Daph (40 mg/kg) or NAC (50 mg/kg) once a day for three consecutive days. CDDP (20 mg/kg) was administered once at 1 h after the first Daph or NAC injection. Mice were sacrificed at 72 h after the CDDP administration. (C) Body weight and (D) Kidney/body weight ratio. (E, F) BUN and serum creatinine were measured (G) macroscopic kidney and (H) hematoxylin and eosin (H&E). (I) Tubular injury scores for kidney damage. Data are presented as the mean ± SEM, n = 5 for all test groups. *p < 0.05, **p < 0.01.
Daph Protects Wild-Type Mice From Oxidative and Inflammatory Damage Induced by Cisplatin
As oxidative damage is crucial in CDDP-induced AKI, we investigated whether Daph pretreatment could reduce kidney damage by inhibiting oxidative stress. We detected SOD, GSH, MPO and MDA levels related to oxidation. As indicated in Figures 3A–D, Daph treatment significantly increased SOD and GSH levels, decreased MPO and MDA levels. Subsequently, we examined changes in the expression levels of Daph on oxidative protein. As presented in Figure 3E, Daph treatment remarkably suppressed the expression levels of NOX4, and increasing the expression levels of SIRT1, SIRT6, Nrf2, HO-1 and NQO1 compared with the CDDP only group. These data show that the protective effects of Daph on CDDP-induced kidney injury by enhancing SIRT1, SIRT6 and Nrf2 and its regulated antioxidant enzymes.
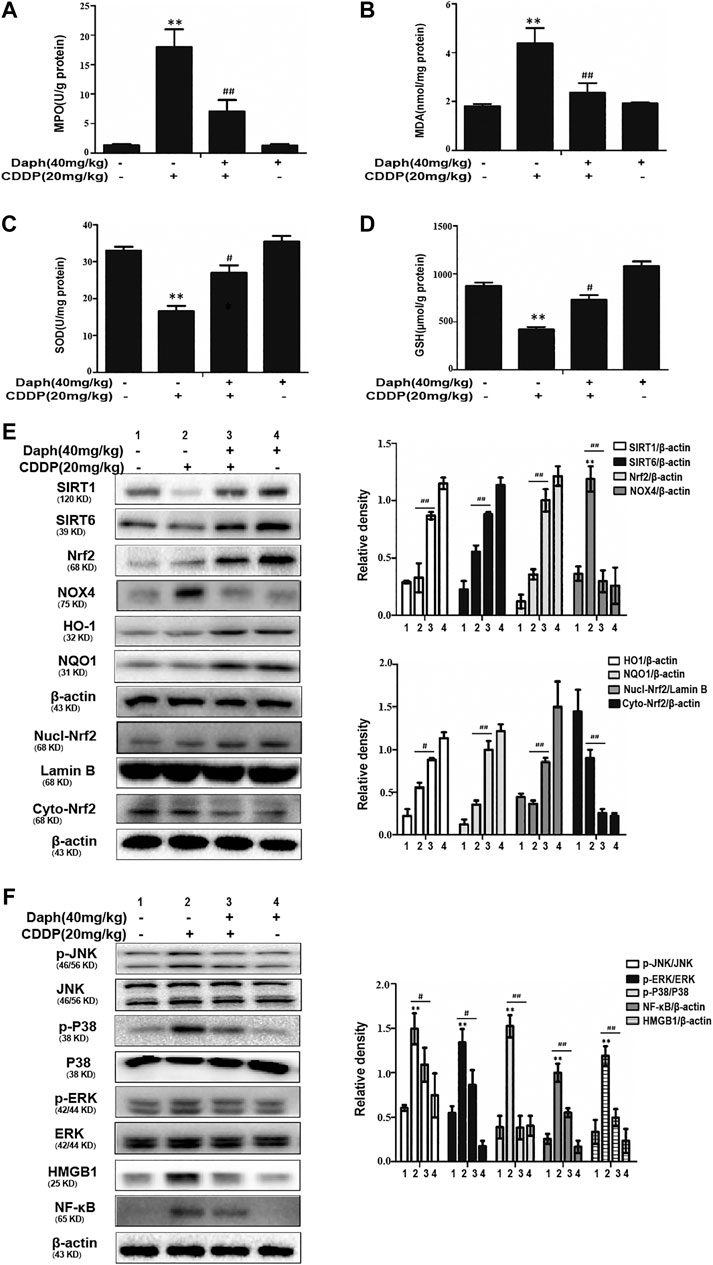
FIGURE 3. Daph inhibits oxidative stress and inflammation in WT mice. (A–D) MPO, MDA, SOD and GSH levels in AKI mice. (E) Western blots analysis showing the expression levels of SIRT1, SIRT6, total Nrf2, nuclear Nrf2, cytoplasmic Nrf2, HO-1, NQO1 and NOX4 in the kidneys from WT mice. (F) Western blots analysis showing the expression levels of p-JNK, JNK, p-p38, p38, (p)-ERK1/2, ERK1/2, HMGB1 and NF-κB in the kidneys from WT mice. Data are presented as the mean ± SEM, n = 5 for all test groups. *p < 0.05 and **p < 0.01 vs. the control group; #p < 0.05 and ##p < 0.01 vs. the CDDP group.
As indicated in Figure 3F, CDDP obviously enhancing the expression levels of NF-κB and HMGB1 and phosphorylation of JNK, ERK, and P38. But, treatment with Daph obviously suppress this phenomenon. In conclusion, increasing evidence demonstrates that the Nrf2-mediated signaling pathway is crucial for the suppression of oxidative stress and inflammatory responses in CDDP- induced nephrotoxicity.
Daph Alleviates Cisplatin-Induced Tubular Cell Apoptosis and Mitochondrial Dysfunction
In order to explore the underlying mechanism of Daph to improve CDDP-induced AKI, we examined the cell apoptosis in the mice after CDDP injection. Western blot and terminal deoxynucleotidyltransferase-mediated dUTP nick end-labeling (TUNEL) staining were used to identify the apoptotic cells in the kidney tissue. Daph inhibits the expression levels of p53, Bax and cleaved caspase-3, and Bcl-2 was significantly increased (Figure 4A). These results indicate that Daph protects from CDDP-induced apoptosis via p53 signaling inhibition. CDDP over capacity induced JNK activation (phosphorylation) in the cytoplasm and translocation of activated P-JNK to mitochondria. We subsequently observed Bax translocated to mitochondria after CDDP, and Daph almost entirely restrained this phenomenon (Figure 4B). As a result, Daph also completely abolished the release of the AIF and cytochrome c in the mitochondrial intermembrane (Figure 4B). The TUNEL assay (Figure 4C) showed that the corresponding DNA fragmentation was caused by the release of mitochondrial endonucleases. Consistent with blocking release of mitochondrial intermembrane protein, DNA fragmentation could also be reversed by Daph, as shown in the elimination of TUNEL positive cells.
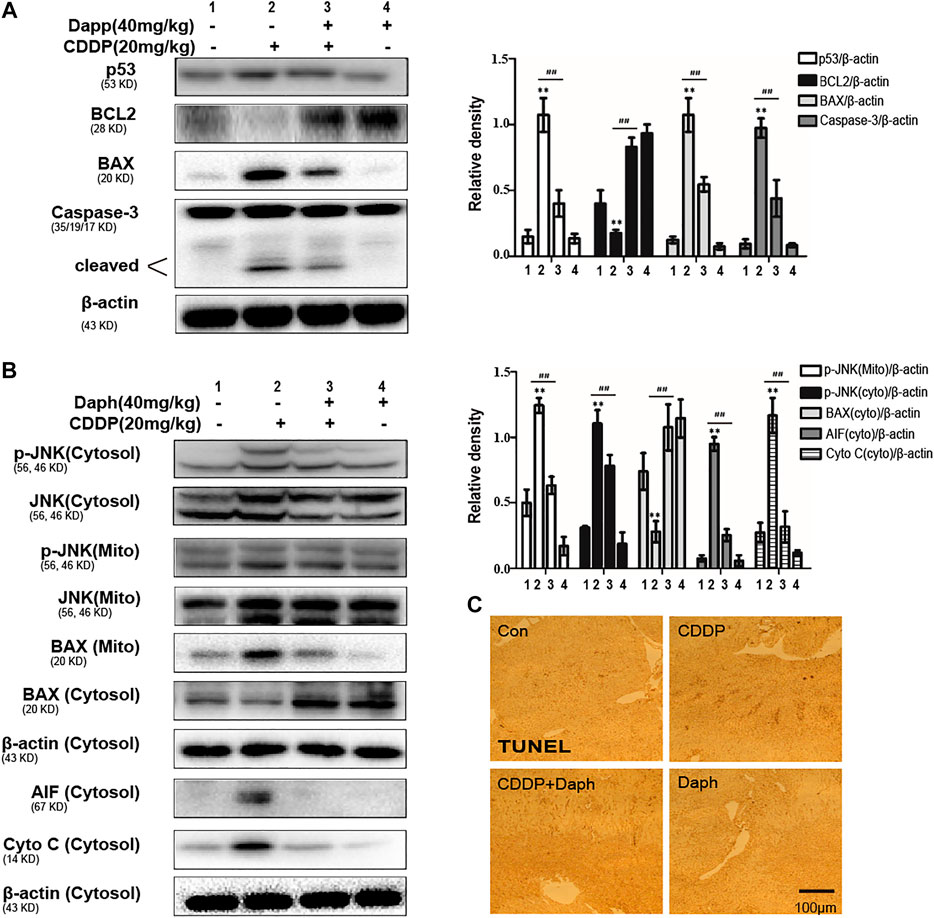
FIGURE 4. Daph ameliorated apoptosis and mitochondrial dysfunction in CDDP-induced AKI in WT mice. (A) Effects of Daph on CDDP-induced p53, Bcl2, Bax and cleaved caspase-3 in the kidneys from WT mice. (B) Effects of Daph on CDDP-induced phosphorylated JNK, Bax, AIF and cytochrome c in mitochondrial and cytosolic. (C) Representative images of the TUNEL assay of kidney. *p < 0.05 and **p < 0.01 vs. the control group; #p < 0.05 and ##p < 0.01 vs. the CDDP group.
The Effect of Daph is Dependent on Nrf2
To verify whether the protective effect of Daph on CDDP-induced AKI targets Nrf2, WT mice and Nrf2−/− mice were employed. We tested the bodyweight, kidney index, BUN, serum creatinine and histological examinations to assess renal function. The data demonstrated that Daph effectively improve CDDP-induced AKI in WT mice, which was abrogated in Nrf2−/− mice (Figures 5A–F). Importantly, Daph also increased the levels of anti-oxidative, anti-inflammation and anti-apoptosis in WT mice, but in Nrf2−/− mice was significantly inhibited and even exacerbated (Figure 6). The results show that Daph dependent on the upregulation of Nrf2 to protect against CDDP-induced AKI.
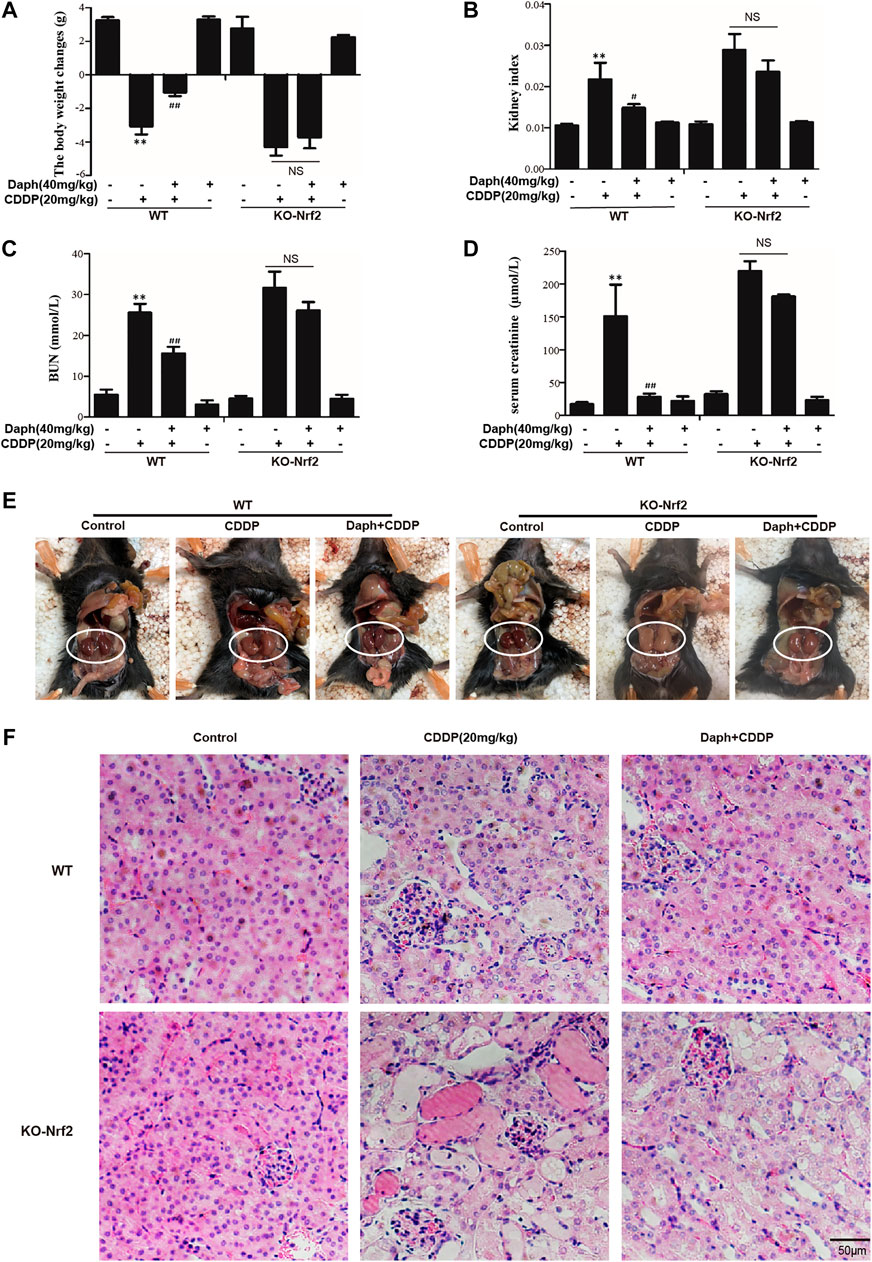
FIGURE 5. Nrf2 is regulated by Daph relieved CDDP-induced AKI in mice. Mice were treated with Daph (40 mg/kg) or NAC (50 mg/kg) once a day for three consecutive days. CDDP (20 mg/kg) was administered once at 1 h after the first Daph or NAC injection. Mice were sacrificed at 72 h after the CDDP administration. (A) Body weight and (B) Kidney/body weight ratio of WT and Nrf2−/− mice. (C,D) BUN and serum creatinine of WT and Nrf2−/− mice were measured. (E) macroscopic kidney and (F) hematoxylin and eosin (H&E). (G) Western blots analysis showing the expression levels of protein in the kidneys from of WT and Nrf2−/− mice. *p < 0.05 and **p < 0.01 vs. the CDDP group; ##p < 0.01 vs. the control group; NS, not significant.
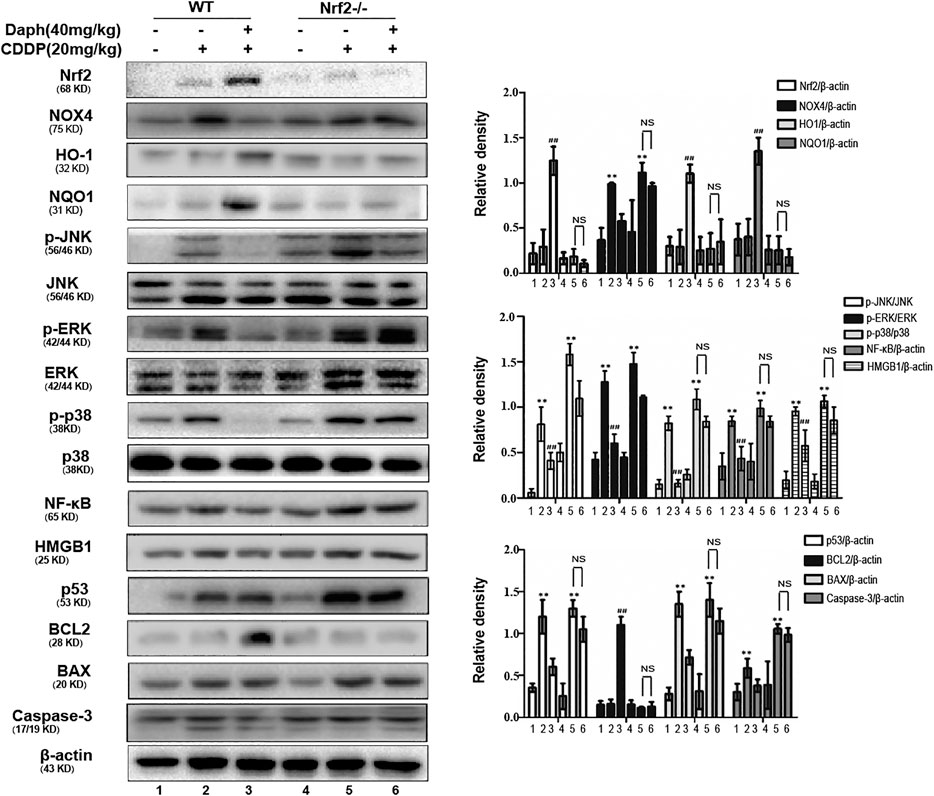
FIGURE 6. Nrf2 is regulated by Daph relieved CDDP-induced AKI in mice. Mice were treated with Daph (40 mg/kg) or NAC (50 mg/kg) once a day for three consecutive days. CDDP (20 mg/kg) was administered once at 1 h after the first Daph or NAC injection. Mice were sacrificed at 72 h after the CDDP administration. Western blots analysis showing the expression levels of protein in the kidneys from of WT and Nrf2−/− mice. *p < 0.05 and **p < 0.01 vs. the CDDP group; ##p < 0.01 vs. the control group; NS, not significant.
Daph Reduce Cisplatin-Induced Cell Death and Apoptosis in HK2 Cells
Then the protective effect of Daph was first examined within HK2. As shown in Figure 7A, the cell viability of HK2 cells treated with CDDP and Daph group increased compared to the CDDP group. And, CDDP-induced cytotoxicity was weakened in a dose-dependent manner. Furthermore, Daph, at 10 μg/ml, obviously reduced CDDP-induced PI positive cells and apoptosis cells (Figure 7B). Daph also alleviated CDDP-induced cell death in a concentration dependent manner too (Figure 7C). The results show that Daph could be effectively reduced CDDP-induced HK2 cell death.
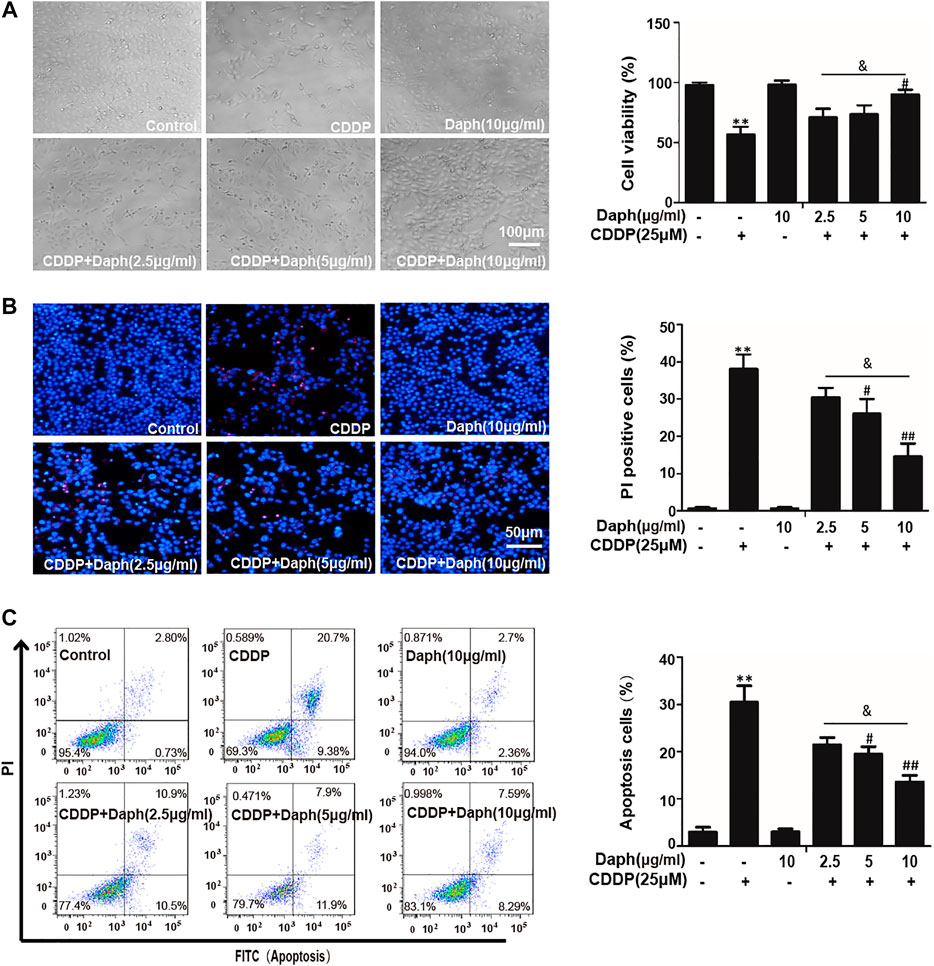
FIGURE 7. Daph attenuated CDDP-induced cell viability and apoptosis in HK2 cells. (A) Effects of Daph (2.5, 5 and 10 μg/ml, pretreatment for 1 h) on CDDP (25 μM, 18 h) induced cell death was analyzed by CCK8. (B) Hoechst/PI staining assay was used to detect the effects of Daph on CDDP induced apoptosis. (C) Flow cytometry was used to detect the effects of Daph on CDDP induced the percentages of apoptotic and necrotic cells. Data are presented as the mean ± SEM, and are representative of three independent experiments. *p < 0.05 and **p < 0.01 vs. the control group; #p < 0.05 and ##p < 0.01 vs. the CDDP group; & p < 0.05 vs. the Daph (10 μg/ml) plus CDDP group.
Daph Activated Antioxidative Pathway and Reduced Cisplatin-Induced Reactive Oxygen Species in HK2 Cells
The data in Figures 8A,B show that Daph significantly upregulated SIRT1, SIRT6, and Nrf2 and increased antioxidant enzyme expression in HK2 cells in a dose- and a time-dependent manner, respectively. Daph treatment significantly inhibited the production of ROS induced by CDDP (Figure 8C).
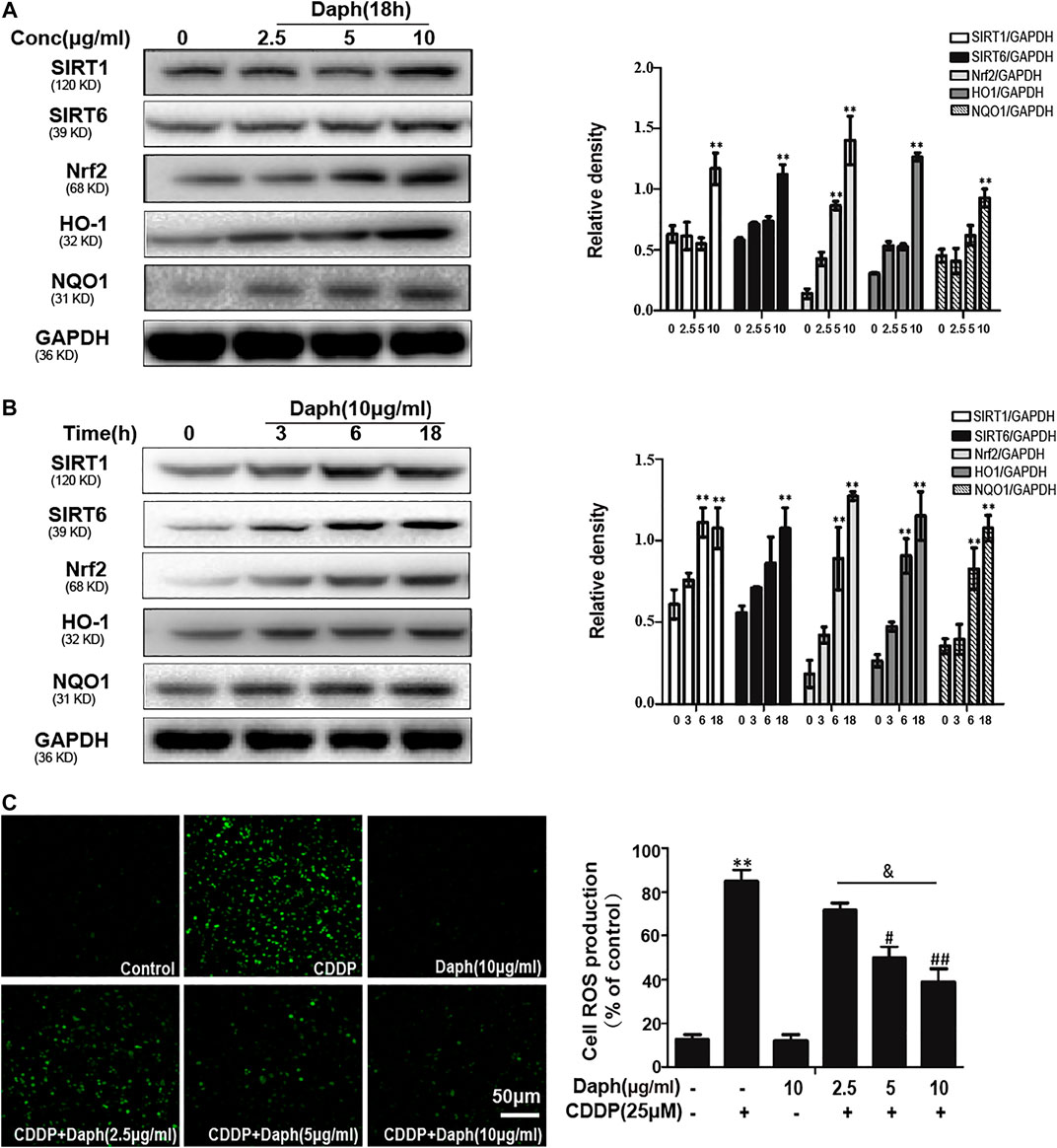
FIGURE 8. Daph activated antioxidant pathway and suppressed CDDP-induced ROS in HK2 cells. (A,B) Western blots analysis showing the expression levels of related protein in HK2 cells (C) HK2 cells were treated with Daph (2.5, 5 and 10 μg/ml) for 18 h, treated with CDDP (25 μM) for 10 min, DCFH-DA staining assay was used to detect ROS generation. Data are presented as the mean ± SEM, and are representative of three independent experiments. **p < 0.01 vs. the control group; #p < 0.05 and ##p < 0.01 vs. the CDDP group; & p < 0.05 vs. the Daph (10 μg/ml) plus CDDP group.
Daph Increases the Expression of SIRT1 and SIRT6 to Activate Nrf2 and Its Target Proteins to Reduce Cisplatin-Induced Cell Death
The expression level of Nrf2 was inhibited after 18 h of incubate with the inhibitors of SIRT1 and SIRT6, as indicated by Western blotting in HK-2 cells (Figures 9A–C). In addition, CCK-8 assay indicated that the protective effect of Daph on CDDP-induced cytotoxicity was blocked by SIRT1, SIRT6 and Nrf2 inhibitors (Figure 9E).
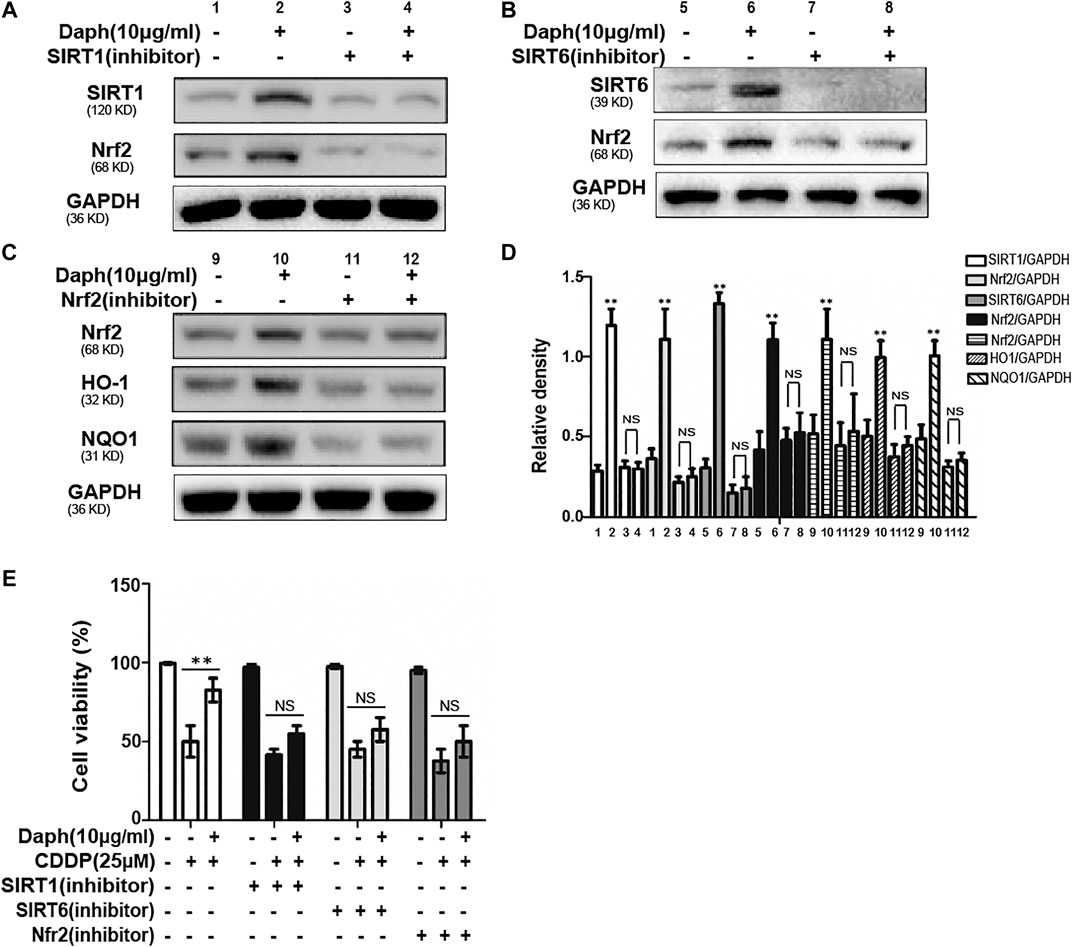
FIGURE 9. Daph regulates Nrf2 through SIRT1 and SIRT6 activation and attenuated CDDP-induced cytotoxicity in HK2 cells. (A–D) Western blots analysis showing the expression levels of SIRT1, SIRT6, Nrf2, HO1 and NQO1 after the treatment of SIRT1, SIRT6 and Nrf2 inhibitors in HK-2 cells. (E) Effects of Daph on CDDP-induced cell viability with SIRT1, SIRT6 and Nrf2 inhibitors. Data are presented as the mean ± SEM, and are representative of three independent experiments. **p < 0.01 vs. the control group; NS, not significant.
Daph Suppressed Cisplatin-Induced the Oxidative Stress, Inflammation and Apoptosis Pathway in HK2 Cells
As shown in Figure 10A, Daph enhances the expression levels of antioxidant proteins SIRT1, SIRT6, Nrf2 and its target genes HO-1 and NQO1, but excluding NOX4. Moreover, Daph could also reverse the activation/phosphorylation of CDDP-induced inflammation-related proteins HMGB1, NF-κB, JNK, p38 and ERK, and the expression levels of apoptosis-related proteins p53, cleaved caspase-3, Bax and Bcl2. to exert anti-inflammatory activity, as well as decreased CDDP-induced upregulation of cleaved caspase-3 and the Bax/Bcl2 ratio to inhibit apoptosis.
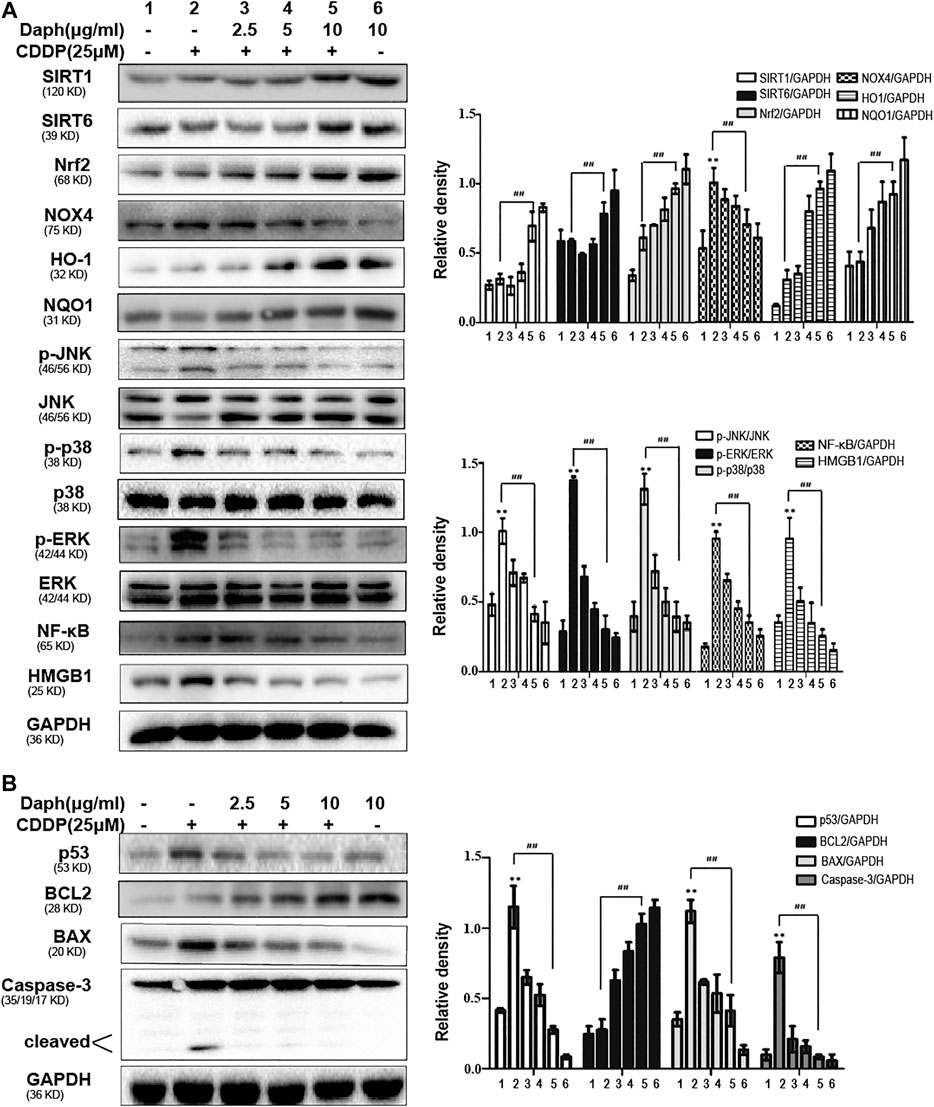
FIGURE 10. Daph inhibits CDDP-induced oxidative stress, inflammation and apoptosis in HK2 cells. (A,B) Western blots analysis showing the protein expression levels of oxidative stress, inflammation and apoptosis in HK2 cells. Data are presented as the mean ± SEM, and are representative of three independent experiments. **p < 0.01 vs. the control group; ##p < 0.01 vs. the CDDP group.
Daph Does Not Compromise the Anti-Cancer Activity of Cisplatin in Human Ovarian Carcinoma Cell Lines and Non-Small Cell Lung Cancer Cell Lines Cell Lines
The effect of Daph on the anti-cancer activity of CDDP was subsequently assessed in human ovarian carcinoma cell lines A2780, OVCAR-8 and SKOV3 and the NSCLC cell lines A549 and H1299. The results revealed that Daph did not display a notable influence on CDDP induced decrease of the cell viability in OVCAR-8, SKOV3, H1299 and A549 cells (Figure 11). Interestingly, it enhanced the anti-cancer activity of CDDP in A2780 (Figure 11). The result indicates that Daph does not compromise the anticancer activity of CDDP at least in human ovarian carcinoma cell lines and the NSCLC cell lines.
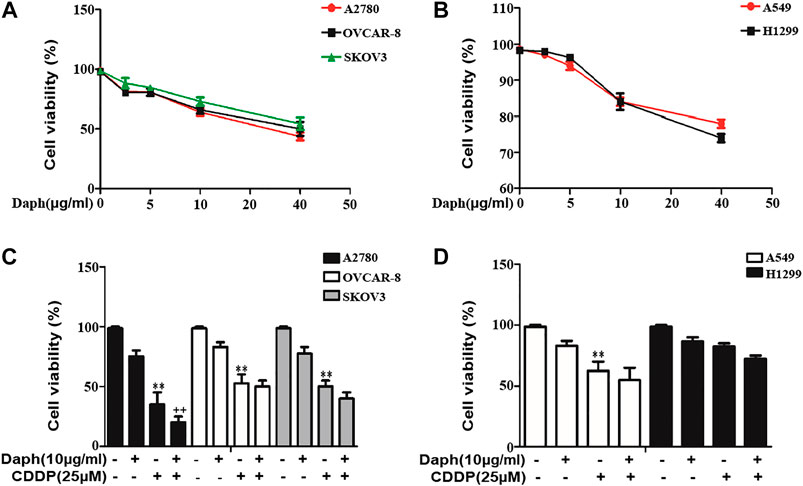
FIGURE 11. Daph did not compromise the anti-cancer activity of CDDP in human ovarian carcinoma cell lines and the NSCLC cell lines. (A,B) Effect of Daph on the cell viability of the human ovarian carcinoma cell line A2780, OVCAR-8 and SKOV3 and the NSCLC cell lines A549 and H1299 after treatment for 48 h (n = 4) (C,D) Effect of Daph (10 μg/ml, pretreatment for 1 h) on the anti-cancer activity of CDDP (48 h) in the human ovarian carcinoma cell lines A2780, OVCAR-8 and SKOV3 and the NSCLC cell lines A549 and H1299. Data are presented as the mean ± SEM, and are representative of three independent experiments. **p < 0.01 vs. the control group; ##p < 0.01 vs. the CDDP group.
Effects of Daph on Cisplatin’s Tumoricidal Activity
In the present study, we demonstrated that Daph effectively attenuated CDDP-induced AKI, suggesting that Daph is a potential therapeutic agent. However, Daph should not interfere with the tumoricidal effect of CDDP in order to be a potential therapeutic agent against side effects of CDDP. Therefore, we examined whether Daph could affect CDDP-induced tumoricidal activity by using a B16 melanoma tumor mouse model. C57BL/6 mice were subcutaneously injected with B16 mouse melanoma tumor cells and treated with CDDP and/or Daph. As shown in Figure 12, Daph did not attenuate the tumoricidal effect of CDDP, but rather enhanced it.
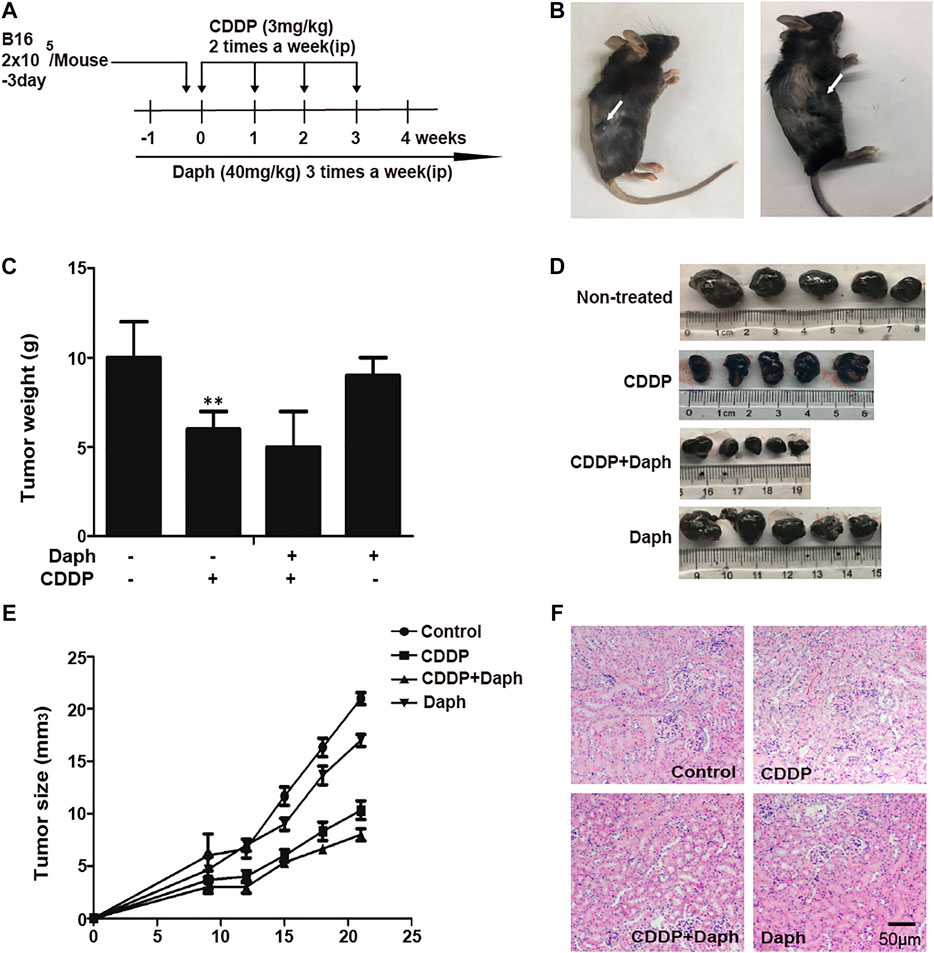
FIGURE 12. Daph did not compromise the tumoricidal activity of CDDP in mice. (A) Experimental study design and time chart for assessing the effect of Daph on the tumoricidal activity of CDDP in mice. B16 melanoma cells (2 × 105/mouse) were inoculated into subcutaneous femoral left region of mice 3 days before an initial injection of CDDP. CDDP was intraperitoneally injected two times per week at 3 mg/kg body weight, and Daph was administered three times per week at 40 mg/kg body weight from 1 to 4 weeks. Four weeks after the initial injection of CDDP, all mice in each group were assessed to evaluate various parameters. (B–E) The tumor volume was measured. Melanoma solid tumor was removed at 4 weeks, photographed, weighed and measured (F) Kidney specimens were stained with H&E. Data show the Mean ± SEM, and are representative of three independent experiments. **p < 0.01 vs. the control group.
Discussion
CDDP is widely used in the treatment of various types of cancer; however, its use may be limited by severe side effects, such as ototoxicity, neurotoxicity and particularly nephrotoxicity. The cytotoxic mechanisms of CDDP include oxidative stress by ROS, mitochondrial dysfunction, and the formation of DNA adducts (Oh et al., 2014). Thus, new ways of suppressing oxidative stress should be a potential method to treat CDDP-induced nephrotoxicity.
The Nrf2/ARE pathway plays a vital role in upregulating cytoprotective genes and enzymes in response to oxidative stress. The present study investigated the mechanisms that underlie the heightened sensitivity of Nrf2 knockout mice to CDDP nephrotoxicity. We compared mouse models of CDDP-induced nephrotoxicity in Nrf2-knockout and WT mice. The results indicated that the renal function, histology and macroscopic kidneys appeared whitening were all significantly worse in the Nrf2 knockout mice (Figure 1). In the past several decades, the abundant availability, structural diversity and nontoxic nature of herbal molecules have made them potential sources of antioxidants to counteract oxidative stress by modulating the Nrf2/ARE pathway (Zhang et al., 2018). Our previous studies screened numerous activators of Nrf2, in which we found that Daph, as the main Nrf2 activator, natural products counteract oxidative stress by regulating the Nrf2/ARE pathway. We found that Daph-mediated Nrf2 antioxidant signaling pathways ameliorated tertbutylhydroperoxide (t-BHP)-induced mitochondrial dysfunction and cell death (Lv et al., 2017). Furthermore, we also found that Daph had protective effects against lipopolysaccharide/d-galactosamine-induced acute liver failure via inhibition of NLRP3, MAPK and NF-κB (Lv et al., 2018). The result was shown that Daph alleviate CDDP-induced nephrotoxicity in HK-2 cells, as shown in the cell viability, ROS and apoptosis. This study suggested that Daph could inhibit CDDP-induced nephrotoxicity.
The pathogenesis of AKI involves complex pathway crosstalk associated with oxidative stress, inflammation and apoptosis. It has been suggested that oxidative stress, a redox state imbalance, impaired energy metabolism, cell apoptosis and inflammation are likely associated with CDDP-induced acute kidney injury (Miller et al., 2010). Oxidative stress can activate various transcription factors, including the inflammatory signaling pathway NF-κB and the apoptotic pathway p53. Activation of these transcription factors can lead to the expression levels of more than 500 different genes, including inflammatory cytokines, chemokines, growth factors and cell cycle regulatory molecules (Reuter et al., 2010). Therefore, new ways to inhibit oxidative stress should be potential methods for treating CDDP-induced nephrotoxicity. In this study, CDDP-induced oxidative stress was characterized by high levels of MDA and MPO and low levels of SOD and GSH, which was reversed by Daph (Figures 3A–D). And, Daph clearly suppressed the CDDP-induced ROS generation in HK-2 cells (Figure 8C). Thus, Daph may counteract CDDP-induced nephrotoxicity via suppressing oxidative stress. In addition, Nrf2, a critical redox sensor, is one of the master regulators of antioxidant responses by activating the antioxidant genes such as HO-1 and NQO1, which are known to counteract ROS (Gorrini et al., 2013). Our results suggested that Daph upregulated the expression levels of Nrf2, HO-1 and NQO1and downregulated the ROS-generating the expression level of NOX4 in vivo and in vitro (Figures 3E, 10A). It has been reported that SIRT1 and SIRT6 play an effective antioxidant role by enhancing the transcriptional activity of Nrf2 (Zhang et al., 2017). Our research shows that Nrf2 activation and cytoprotection by Daph in CDDP-induced HK-2 cells were abolished by SIRT1 and SIRT6 inhibitors (Figure 9). In summary, these results suggested that the renal protection of Daph might be due to its antioxidative properties, which could be achieved by increasing SIRT1 and SIRT6 levels and further activating the Nrf2 pathway.
Recently it has been reported that CDDP accumulates in mitochondria and causes mitochondrial dysfunction (dos Santos et al., 2012). Mitochondrial fragmentation has been demonstrated as an early event in the apoptosis of various mammalian cells including the early renal cell injury that ultimately results in proximal tubular cells death (Linkermann et al., 2014; Martinou and Youle 2011). The manipulation of mitochondrial function may provide a novel therapeutic option to minimize CDDP nephrotoxicity (Choi et al., 2015). In general, CDDP is considered to be a cytotoxic drug, which results in apoptosis via the mitochondrial death pathway (Yan et al., 2016). As a member of the mitogen-activated protein kinase family, JNK is a signaling pathway in response to extracellular stimuli, including CDDP, and CDDP-induced cell death is associated with its phosphorylation and translocation to mitochondria (Yan et al., 2016). Our results suggested that Daph treatment significantly inhibited phosphorylation of JNK and translocation to mitochondria induced by CDDP. JNK has also been shown to modify the activity of numerous proteins located in the mitochondria or the nucleus, for example Bax, it can translocate to mitochondria, as well as speed up mitochondria re lease AIF and cyto-c, thereby promoting mitochondrial dysfunction. In our study, CDDP induced Bax mitochondrial translocation and cyto-c and AIF released from mitochondria, which was reversed by Daph. In addition, other mechanisms of CDDP-induced cell apoptosis and death are due to the downregulation of Bcl-2 and the upregulation of cleaved caspase-3 and p53 (Rjiba-Touati et al., 2012). As shown in Figure 4, Daph reduced CDDP-induced apoptosis via the upregulation of Bcl-2 and the downregulation of p53 and cleaved caspase-3 expression. These results demonstrated that the protective effects of Daph involve the restoration of mitochondrial function and the suppression of apoptosis. In CDDP-induced nephrotoxicity, activation of the NF-κB pathway releases pro-inflammatory cytokines, which can further stimulate the activation of NF-κB and MAPK signaling (Wang et al., 2015). In this study, Daph significantly down-regulated the expression levels of NF-κB, HMGB1, JNK, ERK and p38 in vivo and in vitro (Figures 3F, 10A).
In order to study the role of Daph in CDDP nephrotoxicity, we used SIRT1, SIRT6 and Nrf2 inhibitors in vitro. As shown in Figure 9, SIRT1 or SIRT6 inhibitor suppressed Daph-induced Nrf2 activation and CDDP-induced cytotoxicity, indicating that Daph activates Nrf2 through the SIRT1/SIRT6 pathway and exerts a protective effect on CDDP-induced cytotoxicity. To further elucidate whether the antioxidative activity of Daph in CDDP-induced acute kidney injury is directly related to Nrf2 activation, Nrf2-knockout mice were used to study the presence of potential functions. This research indicates that Daph protects from CDDP-induced kidney damage in WT mice via SIRT1/SIRT6-Nrf2 pathway, while Nrf2-knockout mice were abrogated.
The results shown that CDDP-induced AKI was effective ameliorated, indicating that Daph is a potential therapeutic drug. However, the tumoricidal effect of CDDP should not be interfered with by Daph, which is a potential therapeutic for the side effects of CDDP. Therefore, we examined whether Daph could affect CDDP-induced tumoricidal activity using a human ovarian carcinoma cell lines and non-small cell lung cancer cell lines (NSCLC) and melanoma mouse models. As shown in Figures 11 and 12, Daph did not attenuate the tumoricidal effect of CDDP in the human ovarian carcinoma cell lines and non-small cell lung cancer cell lines, but rather enhanced it. B16 mouse melanoma tumor cells were injected subcutaneously into C57BL/6 mice and treated with CDDP and/or Daph. Daph not only attenuated CDDP-induced kidney damage, but also increased anticancer effects on CDDP in a syngeneic tumor model. Based on current research, Daph may be an original and promising therapeutic agent for the treatment of CDDP-induced nephrotoxicity and/or as an adjuvant for the treatment of CDDP for cancer.
In conclusion, the absence of Nrf2 exacerbates CDDP-induced nephrotoxicity in mice, and Nrf2 pharmacological activation may be a novel therapeutic strategy to inhibit kidney damage. We have identified Daph as a potential agent to attenuate renal injury by suppressing oxidative stress, inflammation and apoptosis via SIRT1/SIRT6-Nrf2 activation. Moreover, Daph enhances the chemotherapeutic effects of CDDP in syngeneic tumor models while protecting kidneys from nephrotoxicity.
Data Availability Statement
All datasets presented in this study are included in the article/Supplementary Material.
Ethics Statement
All animal studies were reviewed and approved by the Animal Ethical Committee of Jilin University.
Author Contributions
XF, a PhD student, performed all of the experiments and data analysis, first draft of the manuscript. wrote the paper and performed the experiments. WW and JH contributed to the experimental design, research and drafting of the manuscript. XC and LP contributed to the experimental design, research and was lead author on drafting the manuscript. All authors read and approved the final manuscript.
Conflict of Interest
The authors declare that the research was conducted in the absence of any commercial or financial relationships that could be construed as a potential conflict of interest.
Acknowledgments
This work was supported by the National Natural Science Foundation of China (Grant No. 81970576) and the Natural Science Foundation of Jilin (No. 20200201378JC and No. JLSCZD2019-065).
References
Aleksunes, L. M., Goedken, M. J., Rockwell, C. E., Thomale, J., Manautou, J. E., and Klaassen, C. D. (2010). Transcriptional regulation of renal cytoprotective genes by Nrf2 and its potential use as a therapeutic target to mitigate cisplatin-induced nephrotoxicity. J. Pharmacol. Exp. Therapeut. 335, 2–12. doi:10.1124/jpet.110.170084
Choi, Y. M., Kim, H. K., Shim, W., Anwar, M. A., Kwon, J. W., Kwon, H. K., et al. (2015). Mechanism of cisplatin-induced cytotoxicity is correlated to impaired metabolism due to mitochondrial ROS generation. PloS One. 10, e0135083. doi:10.1371/journal.pone.0135083
de Haan, J. B. (2011). Nrf2 activators as attractive therapeutics for diabetic nephropathy. Diabetes. 60, 2683–2684. doi:10.2337/db11-1072
dos Santos, N. A., Carvalho Rodrigues, M. A., Martins, N. M., and dos Santos, A. C. (2012). Cisplatin-induced nephrotoxicity and targets of nephroprotection: an update. Arch. Toxicol. 86, 1233–1250. doi:10.1007/s00204-012-0821-7
Gorrini, C., Harris, I. S., and Mak, T. W. (2013). Modulation of oxidative stress as an anticancer strategy. Nat. Rev. Drug Discov. 12, 931–947. doi:10.1038/nrd4002
Guan, Y., Wang, S. R., Huang, X. Z., Xie, Q. H., Xu, Y. Y., Shang, D., et al. (2017). Nicotinamide mononucleotide, an NAD(+) precursor, rescues age-associated susceptibility to AKI in a sirtuin 1-dependent manner. J. Am. Soc. Nephrol. 28, 2337–2352. doi:10.1681/ASN.2016040385
Jamieson, E. R., and Lippard, S. J. (1999). Structure, recognition, and processing of cisplatin-DNA adducts. Chem. Rev. 99, 2467–2498. doi:10.1021/cr980421n
Jiang, T., Huang, Z., Lin, Y., Zhang, Z., Fang, D., and Zhang, D. D. (2010). The protective role of Nrf2 in streptozotocin-induced diabetic nephropathy. Diabetes. 59, 850–860. doi:10.2337/db09-1342
Kuriakose, G. C., and Kurup, M. G. (2008). Evaluation of renoprotective effect of Aphanizomenon flos-aquae on cisplatin-induced renal dysfunction in rats. Ren. Fail. 30, 717–725. doi:10.1080/08860220802134730
Lerrer, B., Gertler, A. A., and Cohen, H. Y. (2016). The complex role of SIRT6 in carcinogenesis Carcinogenesis 37, 108–118. doi:10.1093/carcin/bgv167
Li, Z., Xu, K., Zhang, N., Amador, G., Wang, Y., Zhao, S., et al. (2018). Overexpressed SIRT6 attenuates cisplatin-induced acute kidney injury by inhibiting ERK1/2 signaling. Kidney Int. 93, 881–892. doi:10.1016/j.kint.2017.10.021
Liang, S. C., Ge, G. B., Liu, H. X., Zhang, Y. Y., Wang, L. M., Zhang, J. W., et al. (2010). Identification and characterization of human UDP-glucuronosyltransferases responsible for the in vitro glucuronidation of daphnetin. Drug Metab. Dispos. 38, 973–980. doi:10.1124/dmd.109.030734
Linkermann, A., Chen, G., Dong, G., Kunzendorf, U., Krautwald, S., and Dong, Z. (2014). Regulated cell death in AKI. J. Am. Soc. Nephrol. 25, 2689–2701. doi:10.1681/ASN.2014030262
Liu, M., Grigoryev, D. N., Crow, M. T., Haas, M., Yamamoto, M., Reddy, S. P., et al. (2009). Transcription factor Nrf2 is protective during ischemic and nephrotoxic acute kidney injury in mice. Kidney Int. 76, 277–285. doi:10.1038/ki.2009.157
Lv, H., Liu, Q., Zhou, J., Tan, G., Deng, X., and Ci, X. (2017). Daphnetin-mediated Nrf2 antioxidant signaling pathways ameliorate tert-butyl hydroperoxide (t-BHP)-induced mitochondrial dysfunction and cell death. Free Radic. Biol. Med. 106, 38–52. doi:10.1016/j.freeradbiomed.2017.02.016
Lv, H., Fan, X., Wang, L., Feng, H., and Ci, X. (2018). Daphnetin alleviates lipopolysaccharide/d-galactosamine-induced acute liver failure via the inhibition of NLRP3, MAPK and NF-kappaB, and the induction of autophagy. Int. J. Biol. Macromol. 119, 240–248. doi:10.1016/j.ijbiomac.2018.07.101
Mao, Z., Hine, C., Tian, X., Van Meter, M., Au, M., Vaidya, A., et al. (2011). SIRT6 promotes DNA repair under stress by activating PARP1. Science 332, 1443–1446. doi:10.1126/science.1202723
Martinou, J. C., and Youle, R. J. (2011). Mitochondria in apoptosis: Bcl-2 family members and mitochondrial dynamics. Dev. Cell 21, 92–101. doi:10.1016/j.devcel.2011.06.017
Meng, Z., Li, J., Zhao, H., Liu, H., Zhang, G., Wang, L., et al. (2015). Resveratrol relieves ischemia-induced oxidative stress in the hippocampus by activating SIRT1. Exp. Ther. Med. 10, 525–530. doi:10.3892/etm.2015.2555
Miller, R. P., Tadagavadi, R. K., Ramesh, G., and Reeves, W. B. (2010). Mechanisms of cisplatin nephrotoxicity Toxins 2, 2490–2518. doi:10.3390/toxins2112490
Oh, C. J., Kim, J. Y., Choi, Y. K., Kim, H. J., Jeong, J. Y., Bae, K. H., et al. (2012). Dimethylfumarate attenuates renal fibrosis via NF-E2-related factor 2-mediated inhibition of transforming growth factor-beta/Smad signaling. PLoS One. 7, e45870. doi:10.1371/journal.pone.0045870
Oh, G. S., Kim, H. J., Choi, J. H., Shen, A., Choe, S. K., Karna, A., et al. (2014). Pharmacological activation of NQO1 increases NAD(+) levels and attenuates cisplatin-mediated acute kidney injury in mice. Kidney Int. 85, 547–560. doi:10.1038/ki.2013.330
Pabla, N., and Dong, Z. (2008). Cisplatin nephrotoxicity: mechanisms and renoprotective strategies. Kidney Int. 73, 994–1007. | doi:10.1038/sj.ki.5002786
Pabla, N., Murphy, R. F., Liu, K., and Dong, Z. (2009). The copper transporter Ctr1 contributes to cisplatin uptake by renal tubular cells during cisplatin nephrotoxicity. Am. J. Physiol. Ren. Physiol. 296, F505–F511. doi:10.1152/ajprenal.90545.2008
Pergola, P. E., Raskin, P., Toto, R. D., Meyer, C. J., Huff, J. W., Grossman, E. B., et al. (2011). Bardoxolone methyl and kidney function in CKD with type 2 diabetes. N. Engl. J. Med. 365, 327–336. doi:10.1056/NEJMoa1105351
Potocnjak, I., Skoda, M., Pernjak-Pugel, E., Persic, M. P., and Domitrovic, R. (2016). Oral administration of oleuropein attenuates cisplatin-induced acute renal injury in mice through inhibition of ERK signaling. Mol. Nutr. Food Res. 60, 530–541. doi:10.1002/mnfr.201500409
Reuter, S., Gupta, S. C., Chaturvedi, M. M., and Aggarwal, B. B. (2010). Oxidative stress, inflammation, and cancer: how are they linked? Free Radic. Biol. Med. 49, 1603–1616. doi:10.1016/j.freeradbiomed.2010.09.006
Rjiba-Touati, K., Ayed-Boussema, I., Bouaziz, C., Belarbia, A., Azzabi, A., Achour, A., et al. (2012). Protective effect of erythropoietin against cisplatin-induced nephrotoxicity in rats: antigenotoxic and antiapoptotic effect. Drug Chem. Toxicol. 35, 89–95. doi:10.3109/01480545.2011.589440
Ruiz, S., Pergola, P. E., Zager, R. A., and Vaziri, N. D. (2013). Targeting the transcription factor Nrf2 to ameliorate oxidative stress and inflammation in chronic kidney disease. Kidney Int. 83, 1029–1041. doi:10.1038/ki.2012.439
Taira, N., and Yoshida, K. (2012). Post-translational modifications of p53 tumor suppressor: determinants of its functional targets. Histol. Histopathol. 27, 437–443. doi:10.14670/HH-27.437
Tsai, P. Y., Ka, S. M., Chao, T. K., Chang, J. M., Lin, S. H., Li, C. Y., et al. (2011). Antroquinonol reduces oxidative stress by enhancing the Nrf2 signaling pathway and inhibits inflammation and sclerosis in focal segmental glomerulosclerosis mice. Free Radic. Biol. Med. 50, 1503–1516. doi:10.1016/j.freeradbiomed.2011.02.029
Vaquero, A., Scher, M., Lee, D., Erdjument-Bromage, H., Tempst, P., and Reinberg, D. (2004). Human SirT1 interacts with histone H1 and promotes formation of facultative heterochromatin. Mol. Cell 16, 93–105. doi:10.1016/j.molcel.2004.08.031
Wang, D., and Lippard, S. J. (2005). Cellular processing of platinum anticancer drugs. Nat. Rev. Drug Discov. 4, 307–320. doi:10.1038/nrd1691
Wang, W., Zhou, P. H., Xu, C. G., Zhou, X. J., Hu, W., and Zhang, J. (2015). Baicalein attenuates renal fibrosis by inhibiting inflammation via down-regulating NF-kappaB and MAPK signal pathways. J. Mol. Histol. 46, 283–290. doi:10.1007/s10735-015-9621-8
Wang, Z., Li, Y. F., Han, X. Y., Sun, Y. S., Zhang, L. X., Liu, W., et al. (2018). Kidney protection effect of ginsenoside Re and its underlying mechanisms on cisplatin-induced kidney injury. Cell. Physiol. Biochem. 48, 2219–2229. doi:10.1159/000492562
Wu, M. Y., Yiang, G. T., Lai, T. T., and Li, C. J. (2018). The oxidative stress and mitochondrial dysfunction during the pathogenesis of diabetic retinopathy. Oxidat. Med. Cell. Longev. 2018, 3420187. doi:10.1155/2018/3420187
Yan, D., An, G., and Kuo, M. T. (2016). C-Jun N-terminal kinase signalling pathway in response to cisplatin. J. Cell Mol. Med. 20, 2013–2019. doi:10.1111/jcmm.12908
Yoh, K., Hirayama, A., Ishizaki, K., Yamada, A., Takeuchi, M., Yamagishi, S., et al. (2008). Hyperglycemia induces oxidative and nitrosative stress and increases renal functional impairment in Nrf2-deficient mice. Gene Cell. 13, 1159–1170. doi:10.1111/j.1365-2443.2008.01234.x
Yoh, K., Itoh, K., Enomoto, A., Hirayama, A., Yamaguchi, N., Kobayashi, M., et al. (2001). Nrf2-deficient female mice develop lupus-like autoimmune nephritis. Kidney Int. 60, 1343–1353. doi:10.1046/j.1523-1755.2001.00939.x
Yu, X., Meng, X., Xu, M., Zhang, X., Zhang, Y., Ding, G., et al. (2018). Celastrol ameliorates cisplatin nephrotoxicity by inhibiting NF-kappaB and improving mitochondrial function. EBioMedicine 36, 266–280. doi:10.1016/j.ebiom.2018.09.031
Zhang, B., Ramesh, G., Uematsu, S., Akira, S., and Reeves, W. B. (2008). TLR4 signaling mediates inflammation and tissue injury in nephrotoxicity. J. Am. Soc. Nephrol. 19, 923–932. doi:10.1681/ASN.2007090982
Zhang, W., Wei, R., Zhang, L., Tan, Y., and Qian, C. (2017). Sirtuin 6 protects the brain from cerebral ischemia/reperfusion injury through NRF2 activation. Neuroscience 366, 95–104. doi:10.1016/j.neuroscience.2017.09.035
Keywords: cisplatin, nephrotoxicity, Nrf2, oxidative stress, SIRT1, SIRT6
Citation: Fan X, Wei W, Huang J, Peng L and Ci X (2020) Daphnetin Attenuated Cisplatin-Induced Acute Nephrotoxicity With Enhancing Antitumor Activity of Cisplatin by Upregulating SIRT1/SIRT6-Nrf2 Pathway. Front. Pharmacol. 11:579178. doi: 10.3389/fphar.2020.579178
Received: 01 July 2020; Accepted: 19 October 2020;
Published: 08 December 2020.
Edited by:
Norberto Perico, Mario Negri Pharmacological Research Institute (IRCCS), ItalyReviewed by:
Patrick C. Baer, University Hospital Frankfurt, GermanyRobert Louis Safirstein, Yale University, United States
Copyright © 2020 Fan, Wei, Huang, Peng and Ci. This is an open-access article distributed under the terms of the Creative Commons Attribution License (CC BY). The use, distribution or reproduction in other forums is permitted, provided the original author(s) and the copyright owner(s) are credited and that the original publication in this journal is cited, in accordance with accepted academic practice. No use, distribution or reproduction is permitted which does not comply with these terms.
*Correspondence: Xinxin Ci, Y2l4aW54aW5Aamx1LmVkdS5jbg==