- 1The School of Chinese Medicine for Post-Baccalaureate, I-Shou University, Kaohsiung, Taiwan
- 2Amulette Chinese Medicine Clinic, Tainan City, Taiwan
- 3Department of Biological Science and Technology, I-Shou University, Kaohsiung, Taiwan
- 4School of Chemistry and Materials Science, Nanjing University of Information Science and Technology, Nanjing, China
- 5Department of Science and Engineering of Oxide Materials and Nanomaterials, Polytechnic University of Bucharest, Bucharest, Romania
- 6Department of Microbiology and Immunology, University of Bucharest, Bucharest, Romania
- 7Taiwan Instrument Research Institute, National Applied Research Laboratories, Taipei, Taiwan
- 8Pharmacy Department of E-Da Hospital, Kaohsiung City, Taiwan
Cordyceps militaris (C. militaris) is a fungus with a long history of widespread use in folk medicine, and its biological and medicinal functions are well studied. A crucial pharmacological effect of C. militaris is immunomodulation. In this review, we catalog the immunomodulatory effects of different extracts of C. militaris, namely total extracts, polysaccharides and cordycepin. Total extracts obtained using water or 50% ethyl alcohol and polysaccharides from C. militaris were discovered to tend to promote type 1 immunity, whereas total extracts obtained using 70–80% ethyl alcohol and cordycepin from C. militaris were more likely to promote type 2 immunity. This article is the first to classify the immunomodulatory effects of different extracts of C. militaris. In addition, we discovered a relationship between different segments or extracts and differing types of immunity. This review can provide the readers a comprehensive understanding on the immunomodulatory effects of the precious folk medicine and guidance on its use for both health people and those with an immunodeficiency.
Introduction
Cordyceps militaris (C. militaris), belonging to the family Clavicipitaceae, is a fungus with a long history of widespread use in folk medicine (Figure 1) (Zheng et al., 2011; Wang et al., 2018; Yoon et al., 2018; Qin et al., 2019; Zhang et al., 2019; Zhang et al., 2019; Xiang et al., 2020). Although C. militaris is found in East Asia, C. militaris is difficult to find in the wild due to its rarity. Thus, many investigators have been devoted to focused on the artificial cultivation of C. militaris using cutting-edge technology (Cui, 2015). Many biological and pharmacological functions of this fungus have been identified, such as its antioxidative, antihyperglycemic, antitumor, and immunomodulatory effects (Won and Park, 2005; Dong et al., 2013; Shen et al., 2017; Meng et al., 2018; Yoon et al., 2018; Qin et al., 2019; Sun et al., 2019; Zhang et al., 2019; Guo et al., 2020; Khan and Tania, 2020; Zhang et al., 2020a; Zhang et al., 2020c). In addition, our group has demonstrated that cultivate C. militaris can induce apoptosis and autophagy in human glioblastoma cells (Lin et al., 2011; Yang et al., 2012). Numerous review articles have focused on the biological activities of C. militaris, some of which are summarized in Table 1 and Figure 2 (Das et al., 2010; Lin and Li, 2011; Yue et al., 2013; Liu et al., 2016a; Liu et al., 2016b). In addition, some of the active compounds identified in this fungus have been tested in preclinical trials and clinical trials (Sun et al., 2014; Kang et al., 2015; Sun et al., 2019; He et al., 2020).
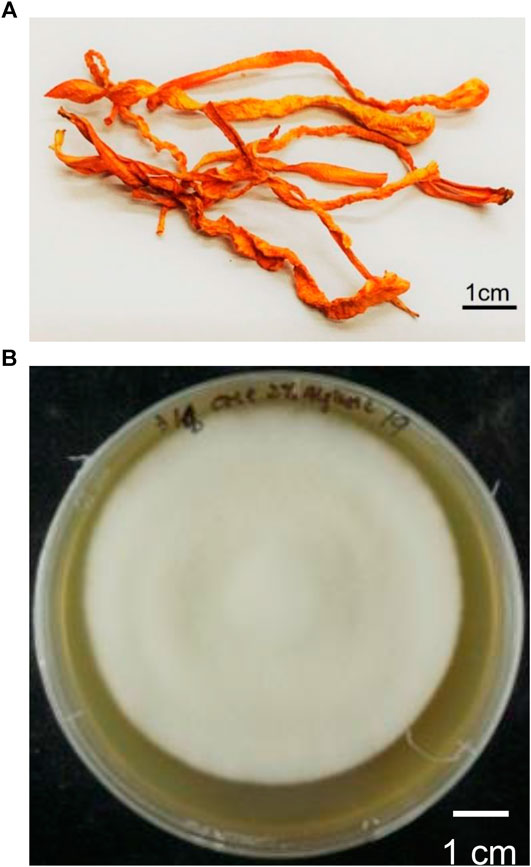
FIGURE 1. (A) The dried fruiting body products of C. militaris(B) The cultured mycelia of C. militaris.
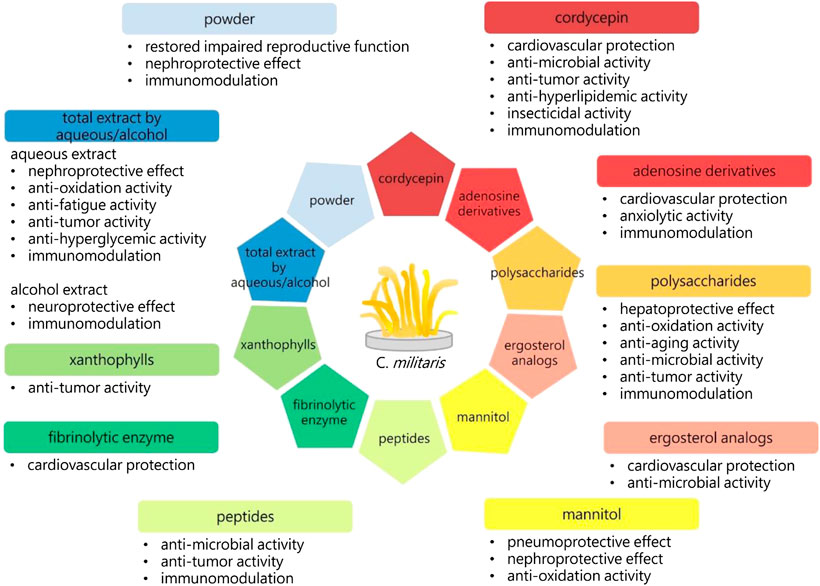
FIGURE 2. The biological activities of C. militaris. 3′-deoxyadenosine (cordycepin), adenosine derivatives, polysaccharides, ergosterol analogs, mannitol, peptides, fibrinolytic enzyme, xanthophylls, aqueous or alcohol extracts, and the powders of C. militaris have exhibited specific biological activities. The immunoregulation is one of the important functions of C. militaris. Immune response has been divided into type 1 and type 2, the balance between these two types of immunity is crucial to our health.
However, few review papers have focused on the relationships between the bioactive compounds of C. militaris and immunomodulation, despite the importance of this topic (Zhang et al., 2020b). The balanced interplay between type 1 and type 2 immunity is crucial to health (Bieber, 2008; Kay, 2001a, Kay, 2001b; Lloyd and Snelgrove, 2018, McInnes and Schett, 2011; Moutsopoulos et al., 2017). For example, autoimmune diseases (e.g., rheumatoid arthritis) are related to stronger type 1 immunity, and atopic diseases (e.g., asthma, atopic dermatitis, and allergic rhinitis) are associated with stronger type 2 immunity. This two fold immunity classification can be considered analogous to the “Yin–Yang” in traditional Chinese medicine (Figure 3). Generally, immune response can be divided into type 1 and type 2 immunity (i1–i2 axis). The i1–i2 axis in helper (Th) T cells represents Th1 and Th2, while the i1–i2 axis in macrophages represents M1 and M2 (Lozupone, 2018; Shapouri-Moghaddam et al., 2018). Type 1 immunity is related to cytotoxic functions including the activities of natural killer (NK) cells, Th1, and CD8+ T cells, and its major function in hosts is to eradicate tumor cells or cells with intracellular pathogens. By contrast, type 2 immunity is related to immunosuppressive functions—TGF-β and interleukin (IL)-10 are common markers—and the inflammatory functions of cytokines IL-4, IL-5, and IL-13; the major function of type 2 immunity is antiparasitic activity, such as the activation of eosinophils and mast cells as well as the secretion of IgE by B cells (Yamaguchi et al., 2015). Type 1 immunity can cause much greater tissue damage than type 2 immunity, and many researchers have claimed that type 2 immunity is crucial in tissue repair (Gause et al., 2013). However, in some cases, type 2 immunity may also cause tissue damage, such as tissue fibrosis (Gieseck et al., 2018).
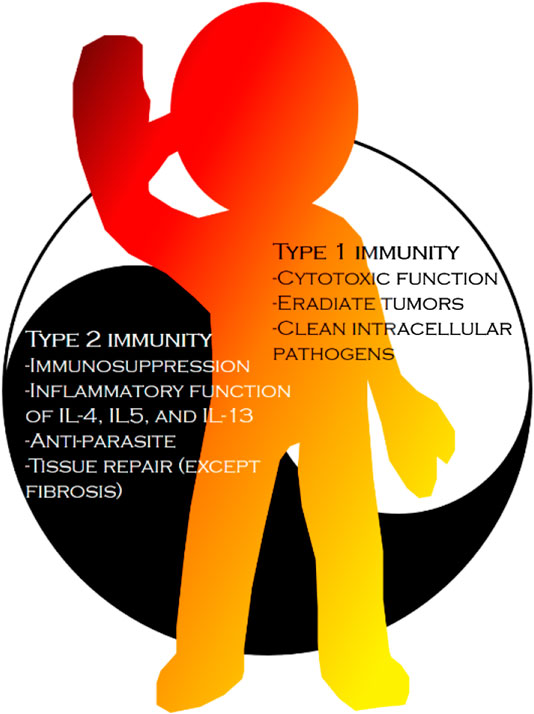
FIGURE 3. The difference between type 1 immunity and type 2 immunity. Type 1 immunity is related to cytotoxic functions and type 2 immunity is related to immunosuppressive function or inflammatory functions of cytokine IL-4, IL-5 and IL-13. In nowadays, type 1 immunity is associated with tumor and intracellular pathogens killing and type 2 immunity is associated with extracellular parasite eradiation and tissue repair, except fibrosis.
In the current review, a survey was conducted to update on research activities related to immunology exhibited by C. militaris which is divided into three sections, each of which addresses a bioactive form of C. militaris: total extracts, polysaccharides and 3′-deoxyadenosine (cordycepin). Each bioactive compound of C. militaris was classified into either the type 1 or type 2 immune response category. This article is the first to classify the immunomodulatory effects of different extracts of C. militaris. In addition, this review can provide the readers a comprehensive understanding on the immunomodulatory effects of the precious folk medicine and guidance on having it for both health and immune deficient people.
Immunomodulatory Functions of C. militaris
Total Extracts
To understand the pharmacological effects of C. militaris on immune regulation, researchers have used various extraction methods to test its biological functions (Lu et al., 2014; Wang and Wang, 2017), and total extracts are one commonly used method (Chu et al., 2011). Some findings of related studies are summarized in Table 2. For example, the anti-inflmmatory activity of the extracts from fruiting body and cultured mycelia of C. militaris was compared by Won and Park (2005) (Won and Park, 2005). Two different parts of the fungus were extracted using 70% ethanol. Results indicated that the extracts from both the fruiting body and cultured mycelia could reduce ear edema in an animal study. The inhibition percentages of fruiting body extracts, cultured mycelia extracts, and indomethacin were 58.7, 51.8, and 61.6%, respectively. The two extracts were then further used to examine the inducible nitric oxide synthase (iNOS) expression, which is highly expressed in inflammatory diseases and leads to cellular injury. The results revealed that fruiting body extracts and cultured mycelia extracts had similar antiinflammatory effects. Further investigation indicated that fruiting body extract and cultured mycelia extract could substantially reduce vascular development and reduce angiogenesis, with stronger antiangiogenesis effects observed in the cultured mycelia extracts.
Water extract from the fruiting body of C. militaris was prepared and orally administered to mice at a dose of 20 mg/kg (Kim et al., 2008). Kim et al. (2008) reported that the extracts could increase the level of interferon (IFN)-γ secretion by macrophages through IL-18. Therefore, they proposed that C. militaris could be used as an immune activator or antitumor agent.
Water extracts were obtained from fruiting body of C. militaris by Hsu et al. (2008) and found to have potential therapeutic effects on asthma in an ovalbumin (OVA)-induced asthma mice model (Hsu et al., 2008). Briefly, the mice were given 100 µg of OVA through intraperitoneal (IP) injection on day 0, and subsequently, 50 µg of OVA was administered intranasally on days 14, 25, 26, and 27 to induce asthma. A total of 4 g/kg/day of C. militaris extract was administered orally to treat the mice on days 15–27. Results revealed that the serum immunoglobulin E (IgE) level decreased, but not significantly, in the group treated with C. militaris extract compared with nontreated control, montelukast (leukotriene receptor antagonist) and prednisolone sodium phosphate solution (steroid) groups. The serum IgE levels in the nontreated control, steroid-treated, montelukast-treated and C. militaris extract-treated mice were 1833.52 ± 402.03, 574.03 ± 120.71, 284.46 ± 45.41 and 1,591.35 ± 350.68 ng/ml, respectively. In addition, the researchers observed fewer infiltrating cells in the airway in C. militaris extract-treated group than in the nontreated control, montelukast and steroid groups. However, the difference was not significant. Infiltrating cell counts in the nontreated control, steroid-treated, montelukast-treated and C. militaris extract-treated groups were 11.83 ± 2.2 × 105/ml, 3.83 ± 0.6 ×105/ml, 4.33 ± 0.85 × 105/ml, and 8.5 ± 1.71 × 105/ml, respectively. Finally, histology was used to evaluate airway inflammatory changes. The results indicated that C. militaris extract treatment only partially attenuated the inflammatory cells recruited in the airway and the smooth muscle layer thickened in the OVA-treated group. The researchers concluded that C. militaris extract could modulate airway inflammation in asthma but was only suitable for use as an auxiliary agent in asthma control because its effect was not as strong as those of montelukast and steroids.
Water extracts from the fruiting body of C. militaris were demonstrated by Kim’s group (2010) that could modulate macrophage activity (Shin et al., 2010). They used 12.5, 25, 50, 100, or 200 μg/ml extracts to treat RAW 264.7 macrophages or primary culture peritoneal macrophages. They observed that expression of nitrogen oxide; proinflammatory cytokines, such as IL-1β, IL-6, tumor necrosis factor-α (TNF-α), and prostaglandin E2 (PGE2); and iNOS and cycloxygenase-2 (COX-2) were significantly elevated in a dose-dependent manner compared with nontreated controls. In addition, the expression of costimulatory molecules such as B7-1 [e.g., cluster of differentiation (CD)80] protein, B7-2 (e.g., CD86) protein, and intracellular adhesion molecules-1 (ICAM-1) were also enhanced. The researchers reported that C. militaris extracts could activate macrophage activity through activation of nuclear factor kappa-light-chain-enhancer of activated B cells (NF-κB). In the same year, Kim’s group also reported that the water extracts from the fruiting body of C. militaris could modulate dendritic cell function (Shin et al., 2010). They used 12.5, 25, 50, 100, or 200 μg/ml extracts to treat both dendritic cells DC2.4 and primary bone marrow-derived dendritic cells. They found that C. militaris extracts could increase the expression of the H-2Kb gene [major histocompatibility complex (MHC) class I] and I-Ab gene (MHC class II) but did not alter the phagocytotic activities of dendritic cells. Results further demonstrated that these extracts could enhance the presentation of both MHC class I and MHC class II in dendritic cells, which could successfully increase CD4 T cell and CD8 T cell activation. In addition, the researchers speculated that C. militaris extracts could increase inflammatory cytokine expression (such as IL-1β, IL-6, and TNF-α), and expression of cytokine-related enzymes (such as iNOS and COX-2) and that the activation of dendritic cells caused by C. militaris extract might be result from NF-κB upregulation.
Han et al. (2011) found that the water extract of mycelium of C. militaris attenuated dextran sodium sulfate–induced acute colitis in mice (Han et al., 2011). They used 500 mg/kg of the extract to treat mice with acute colitis and observed that it could reduce body weight loss, diarrhea, and major bleeding. In addition, C. militaris extract could prevent the shortening of the colon length and crypt length and suppress epithelial damage, loss of goblet cells, loss of crypts, and infiltration of inflammatory cells. The researchers revealed that the extract could inhibit iNOS and TNF-α expression in the colon of mice with acute colitis and in lipopolysaccharide (LPS)-induced RAW 264.7 macrophages. Degranulation of mast cells in colon tissue and antigen-stimulated mast cells could also be suppressed after extract treatment. They exhibited that C. militaris extract could be a potential reagent for the prevention or treatment of inflammatory bowel diseases.
Ethyl acetate extract of C. militaris reported to inhibit allergy reaction by Oh et al. (2011) (Oh et al., 2011). They used 10, 30, 100, or 300 μg/ml extracts to examine the antiallergic response in RBL-2H3 mast cells and found that ethyl acetate extracts could inhibit antigen-induced degranulation with half maximal inhibitory concentration (IC50) = 28.5 μg/ml in RBL-2H3 cells. The inhibitory effect of the extract on antigen-induced passive cutaneous anaphylaxis was evaluated in a mice model. Results indicated that the ethyl acetate extract could reduce the passive cutaneous anaphylaxis reaction in a dose-dependent manner with median effective dose (ED50) = 665 mg/kg. Furthermore, the extract could reduce TNF-α and IL-4 expression in antigen-stimulated mast cells. This reaction might be associated with lowering the expression levels of the phosphorylation tyrosine-protein kinase (SYK), extracellular-signal-regulated kinase (ERK), p38 mitogen-activated protein kinases (p38), and c-Jun N-terminal kinase (JNK). Results also revealed that the ethyl acetate extract could directly mediate mast cell immune response.
Zhu et al. (2013) compared the immunomodulation effects of fresh and dried C. militaris extracts (Zhu et al., 2013). Briefly, 50, 100, or 200 mg/kg of fresh or dried extracts were used to treat cyclophosphamide (cy)-induced immunosuppression in mice. Analysis results indicated that the amount of adenosine and cordycepin in fresh and dried C. militaris was almost identical. By contrast, polysaccharides, total polyphenol, and total flavonoids were more abundant in fresh than in dried extracts. The researchers observed that fresh or dried C. militaris could reverse the inhibition of spleen and thymus index (spleen or thymus weight/body weight) in cy-induced mice in a dose-dependent manner. In addition, both fresh and dried C. militaris could recover the phagocytotic effect in cy-treated mice, and the fresh extract had a stronger effect than did the dried extract. Both fresh and dried C. militaris could considerably increase IL-2 and IFN-γ secretion levels in cy-induced mice or cy-treated T cells. The researchers concluded that fresh C. militaris is superior to dried C. militaris in terms of immune modulation.
C. militaris extract was reported by Lee et al. (2014) to have some protective effects in influenza A virus-infected mice (Lee et al., 2014). After 7 days of oral administration of 30, 100, or 300 mg/kg/day C militaris extracts, mice were then infected with influenza A/NWS/33 (H1N1) virus. The researchers found that IL-12 was elevated and TNF-α was reduced. They argued that the protective effect of the extract in H1N1-infected mice might be related to the elevation of IL-12, increase of natural killer (NK) cells, and decrease of TNF-α level.
An ethanol extract of C. militaris was exhibited by Kang et al. (2015) to enhance cell-mediated immunity in healthy Korean men (Kang et al., 2015). A total of 1.5 g/day of C. militaris was orally delivered to healthy volunteers. Results revealed that the extract did not cause any adverse reaction. The researchers found that the IL-2 and the IFN-γ were significantly increased after the 4 weeks treatment (N = 39) compared with the placebo group (N = 40). In addition, they observed that C. militaris could increase NK cell activity and T cell proliferation. They posited that the extract could serve as a safe immunomodulator to increase cell-mediated immunity.
C. militaris grown on germinated soybean extract (GSC) could inhibit 2,4-dinitro-1-fluorobenzene (DNFB)-induced contact dermatitis (Park, 2015). 300 mg/kg of GSC was used to treat DNFB-induced contact dermatitis and it was found that GSC mitigated ear swelling compared with results in the nontreated control. Furthermore, immunohistochemical analysis of the infiltrated T cells in the ear tissue of the mice illustrated that GSC could significantly reduce CD4 and CD8 T cell infiltration in the ear of DNFB-induced contact dermatitis mice. In addition, they found that GSC could inhibit concanavalin A–induced T cell proliferation. They suggested that GSC could act as a reagent to alleviate allergic contact dermatitis.
Extracts from fruiting body of C. militaris exhibited an immunomodulatory and antioxidative activity (Liu et al., 2016a; Liu et al., 2016b). Three dosages of extracts (50, 100, or 200 mg/kg/day) were fed to healthy Kunming mice. The researchers demonstrated that thymic and splenic indices were significantly increased in mice treated with C. militaris polysaccharides in a dose-dependent manner. Total white blood cell count was increased in extract-treated mice in a dose-dependent manner. In the white blood cell population, lymphocytes and monocytes were increased, neutrophils were decreased, and eosinophils and basophils seemed to display no overt changes. In addition, the IgG content increased in the mice treated with C. militaris polysaccharides. TNF-α and IL-1β expressions were also increased in the spleen after treatment with the extract. Superoxidase dismutase, glutathione peroxidase, and total antioxidant capacity activity were increased in liver, kidney, and heart tissue; the end product of lipid peroxidation, the malondialdehyde levels, was decreased in the liver, kidney, heart, and serum samples. They concluded that C. militaris extracts could modulate the immune system and antioxidative enzymes in heathy organisms.
The results presented in this section lead to some conclusions. First, nearly no difference exists between the extracts of fruiting body and those cultured mycelia in terms of immunomodulatory effect. However, cultured mycelia extract exhibited a more potent effect in antiangiogenesis than did the fruiting body extract. Second, in comparison with dry C. militaris, the fresh extract had a stronger immunoregulation effect, which could be attributed to the higher levels of polysaccharides, total polyphenol, and total flavonoids in fresh C. militaris (Zhu et al., 2013). Finally, the total extracts of C. militaris using water or 50% ethanol are likely to activate macrophage and dendritic cells while reducing allergy effects. Furthermore, C. militaris extracts using 70–80% ethanol seems to have anti-inflammatory effects.
Polysaccharides
Polysaccharides are crucial bioactive components in C. militaris (Beltrame et al., 2019; Yang et al., 2019; Barcelos et al., 2020; Mohan et al., 2020; Wu et al., 2020). Polysaccharides are known to have antiviral, antioxidative, antiinflammatory, antitumor, neuroprotective, antihypertensive, and immunomodulatory biological effects (Sims et al., 2011; Lee et al., 2017; Rodrigues et al., 2020). Some research findings related to polysaccharides are summarized in Table 3.
CPS-1 extracted from C. militaris acts as an immunomodulator (Yu et al., 2004). CPS-1 is a polysaccharide with a molecular weight (MW) of 2.3 × 104 Da and is composed of rhamnose (Rha), xylose (Xyl), d-mannose (D-Man), d-glucose (D-Glc), and d-galactose (D-Gal). CPS-1 might contain rhamnose bonded with galactose by a (1→2) or (1→3) linkage, xylose bonded by a (1→4) linkage and mannose bonded by a (1→2) linkage. First, the researchers found that an IP injection of 50, 100, or 200 mg/kg CPS-1 in croton oil–induced mice ear edema could significantly reduce inflammation (the inhibition percentage was 48.3, 61.5, and 71.7%, respectively) in a dose-dependent manner. Second, they injected mice with 25, 50, or 100 mg/kg CPS-1 subcutaneously and found that CPS-1 decreases vascular permeability in the acetic acid–induced model compared with normal saline treatment. Third, the researchers used sheep red blood cells and observed reduced serum hemolysin formation in mice that received IP injections of 15 and 30 mg/kg CPS-1. Finally, CPS-1 was used to evaluate the phagocytotic function and delayed-type hypersensitivity in vivo; no differences were observed between the CPS-1-treated group and the control group. According to the aforementioned results, the researchers suggested that CPS-1 can reduce inflammation and humoral immunity but reported no significant change in the phagocytotic function of innate immunity and cellular immunity.
An acid polysaccharide (APS) isolated from C. militaris was revealed to have antiinfluenza virus activity by Ohta et al. (2007) (Ohta et al., 2007). APS, with an MW of 5.76 × 105 Da, consists of D-Gal, arabinose (Ara), Xyl, Rha, and galacturonic acid. After mice infected with H1N1 were treated with 0.1 mg of APS, the researchers found that APS could reduce mortality in comparison with controls (mortality of APS:mortality of control = 18%:70%). In addition, the researchers observed that APS might modulate macrophage activity to protect against influenza virus A infection. Results indicated that APS could increase TNF-α and IFN-γ expression in influenza A virus–infected mice. These results were also confirmed using RAW 264.7 macrophage cells: iNOS, IL-1β, IL-6, and IL-10 expression levels were elevated after RAW 264.7 macrophage cells were treated with APS. Results indicated that APS could modulate not only inflammatory cytokine (IL-1β, IL-6, TNF-α, and IFN-γ) and iNOS expression, which could increase antivirus activity, but also anti-inflammatory cytokine (IL-10) expression, which could prevent excessive macrophage activation and reduce deaths caused by influenza A virus in vivo (Ohta et al., 2007).
Cysinocan, a polysaccharide extracted from C. militaris and with a MW of 8.2 × 104 Da, is composed of D-Glc, D-Man, and D-Gal (Cheung et al., 2009). Cheung et al. (2009) found that cordysinocan modulated both T lymphocyte and macrophage activities. The researchers observed that cordysinocan induced T cell proliferation [half maximal effective concentration (EC50) = 6 μg/ml] and IL-2 secretion (EC50 = 8.5 μg/ml). Moreover, IL-2, IL-6, IL-8, and IL-10 were markedly overexpressed. The study also investigated whether the cordysinocan-induced T cell proliferation occurred through the ERK pathway. The researchers revealed that cordysinocan could act as an immunomodulator on T lymphocytes and macrophages.
Cordlan polysachharide was found to induce dendritic cell maturation (Kim et al., 2010). The authors found that 10, 30, or 100 μg/ml of cordlan could help immature dendritic cells to mature. Cordlan enhanced allogenic T cell stimulation, decreased endocytosis, and elevates the phenotypic markers of mature dendritic cells, such as CD40, CD8 and CD86, the main function of MHC class I; and functional markers of MHC class II molecules and mature dendritic cell, such as IL-12, IL-1β, TNF-α, and IFN-αβ. The researchers revealed that cordlan induced maturation in immature dendritic cells by failing to induce toll-like receptor (TLR)4 knock out from immature dendritic cells into mature dendritic cells, possibly through the TLR4 pathway.
CPSN Fr II and CPMN Fr III were isolated by Hong’s group (2010). CPSN Fr II, with an MW 3.6 × 104 Da was discovered to consist of D-Man (65.12%), D-Gal (28.72%) and D-Glc (6.12%). CPMN Fr III with a MW of 2.1 × 105 Da consisted of D-Man (72.22%), D-Gal (18.61%) and D-Glc (9.17%) (Lee et al., 2010; Lee et al., 2010). The researchers indicated that CPSN Fr II or CPMN Fr III could both induce macrophages to secrete proinflammatory cytokines (TNF-α and IL-1β) and produce nitric oxide. They evaluated the structure of CPSN Fr II and found that this polysaccharide had a backbone of (1→2)-linked d-mannopyranosyl and (1→6)-linked d-mannopyranosyl residues, which occasionally branches at O-6. The branches were mainly composed of (1→4)-linked d-galactopyranosyl residues and terminated with d-galactopyranosyl residues, with a degree of branching of 0.2. The researchers also examined the structure of CPMN Fr III and found that this polysaccharide had a backbone of (1→6)-linked d-mannopyranosyl and (1→6)-linked d-glucopyranosyl residue. The branches were mainly composed of (1→4)-linked d-mannopyranosyl residue and terminated with d-galactopyranosyl residues and d-mannopyranosyl residues, with a degree of branching of 0.33. In addition, Hong’s group revealed that polysaccharides isolated from C. militaris–stimulated macrophage activity might be associated with the mitogen-activated protein kinase (MAPK) and NF-κB pathway (Lee et al., 2015). Furthermore, the researchers observed that anti-TLR2, anti-TLR4, and antidectin-1 antibodies could abolish the activation effect on polysaccharide-induced macrophages. According to these findings, the authors suggested that the polysaccharides activated the macrophages, possibly through the MAPK and NF-κB pathway using TLR2, TLR4, and dectin-1. Hong’s group reported that polysaccharides might have immunomodulatory and antitumor effects (Lee and Hong, 2011). Polysaccharide could regulate peritoneal macrophages and RAW 264.7 cells to increase the expression of reactive oxygen species (ROS), nitric oxide, TNF-α, and phagocytic uptake in a dose-dependent manner. They posited that the polysaccharide-induced immune response might be associated with NF-κB and all three MAPK pathways through TLR2 and dectin-1. They also evaluated the antitumor effects on polysaccharide-treated B16-F10 melanoma tumor–bearing mice and found that either IP injection or oral administration could reduce the tumor growth rate. Furthermore, they used 500 μg/ml of polysaccharide to directly treat B16-F10 melanoma and found that the extract did not cause B16-F10 melanoma death. Therefore, the results indicated that polysaccharides exert antitumor effects through immunomodulation but do not induce tumor cells death directly.
Polysaccharides could abolish cy-induced immunosuppression in a mice model (Wang et al., 2012). They used 80 mg/kg/day cy through IP injection from day 1 to day 3, and 17.5, 35, or 70 mg/kg of polysaccharide was administrated orally from day 4 to day 18. Subsequently, the spleen and thymus indices, lymphocyte proliferation, phagocytic index, and biochemical parameters were measured. Results revealed that the spleen and thymus indices were reduced in the cy-injected group in comparison with those of the nontreated controls. Moreover, in 17.5, 35, or 70 mg/kg polysaccharide-treated cy-injected mice, the spleen and thymus indices were reversed in a dose-dependent manner. The researchers also found that the lymphocyte proliferation rate in polysaccharide-treated cy-injected mice was significantly increased relative to that of cy-injected mice. Furthermore, the phagocytotic index in cy-treated mice was reduced, but it recovered after polysaccharide treatment. The authors demonstrated that polysaccharide could improve the immune and antioxidation function in cy-induced immunosuppressive mice.
Two polysaccharides (CMP-W1 and CMP-S1) were extracted by Luo et al. (2017) (Luo et al., 2017). CMP-W1 and CMP-S1—with MW of 3.66 × 105 Da and 4.6 × 105 Da, respectively—are both heteropolysaccharides that are composed of D-Man, D-Glc, and D-Gal. The researchers found that these polysaccharides could induce splenocyte proliferation in mice, and also had synergistic effects on concanavalin A–induced T cells and LPS-induced B cells, respectively. They suggested that CMP-W1 and CMP-S1 could act as potential immunomodulatory reagents.
A novel homogeneous polysaccharide named CMP-III was discovered by Lin’s group (2020); it was composed of D-Glc, D-Man and D-Gal with an MW of 4.796 × 107 Da. The main linkage types of CMP-Ⅲ consisted of (1→4)-α-D-Glc (70.08%) (1→4,6)-α-D-Man (9.59%) (1→)-α-D-Man (10.79%) and (1→2,6)-α-D-Gal (3.93%). They found that CMP-III enhanced macrophage phagocytosis ability and increased TNF-α, IL-6 and NO secretion (He et al., 2020).
Discovered by Da Yu et al. (2020), a polysaccharide called CMPB90–1 with an MW of 5.8 × 103 Da suggested to be capable of converting M2 macrophage into M1-like macrophage and eradicating tumor. In addition, they also found that CMPB90–1 could increase T cell activity by inhibiting the programmed death ligand-1 (PD-L1)/programmed death -1 (PD-1) axis between tumor associated macrophage and T cells. CMPB90–1 is composed of (1→6)-α-d-glucopyranosyl and (1→3)-α-d-glucopyranosyl residues (with branching at O-6) which consists of (1→4)-β-d-mannopyranosyl and (1→6)-α-d-glucopyranosyl residues, respectively. These results suggested that CMPB-90–1 might have potential as an immune regulator to aid tumor eradication (Bi et al., 2020).
Pattern recognition receptors are critical in innate immunity (Kawai and Akira, 2010; Fitzgerald and Kagan, 2020). Polysaccharides are one of the suitable candidates to bind pattern recognition receptors and may be able to alter immunity (Schnaar, 2016). Here, we found that the immunomodulatory effects of C. militaris polysaccharides might be associated with binding TLR2, TLR4, and dectin-1 on immune cells. Notably, APS, a polysaccharide derived from C. militaris, was demonstrated to have perform two types of functions, namely immune stimulation and anti-inflammation, in an influenza virus A–infection mice model. This crucial finding suggests that APS can be used for the treatment of microbe-induced cytokine storms, a life-threatening condition caused by excessive immune response (D'Elia et al., 2013).
Cordycepin
Cordycepin is a valuable bioactive compound produced by C. militaris that was discovered in the early 1950s (Cunningham et al., 1950; Ruthes, 2016; Qin et al., 2019). Many researchers have demonstrated the strong immunomodulatory effects of cordycepin (Kitamura et al., 2011; Wang et al., 2019). The findings of its immunomodulatory effects are summarized in Table 4.
Cordycepin could inhibit iNOS expression in LPS-induced RAW 264.7 macrophage cells (Kim et al., 2006). The researchers treated LPS-induced RAW 264.7 macrophage cells with 5, 10, 15, or 30 μg/ml of cordycepin, which reduced the production of nitric oxide in a dose-dependent manner in comparison with nontreated group. In addition, the authors detected iNOS, COX-2, and TNF-α expression in these cells and found that cordycepin could significantly reduce their expression. According to their findings, the inhibition of iNOS, COX-2, and TNF-α expression might be due to the suppression of NF-κB through Akt and p38 inhibition.
Kim’s group (2009) found that cordycepin could regulate type II diabetes (Shin, Lee, et al., 2009). The researchers used 5, 10, 20, or 40 μg/ml cordycepin to treat LPS-induced RAW 264.7 macrophage cells and found that TNF-α, IL-6, IL-1β, iNOS, and COX-2 expression decreased significantly in cordycepin-treated LPS-induced RAW 264.7 macrophage cells compared with that in the nontreated controls in a dose-dependent manner. They tested the type II diabetes–related genes in LPS-induced RAW 264.7 cells and found that LPS could greatly increase the levels of RANTES, 11β-HSD1, and PPARγ genes; however, type II diabetes–related genes decreased signifcantly in the cordycepin-treated group. In addition, cordycepin could decrease the expression of costimulatory molecules, such as B7-1/-2 and ICAM-1, in LPS-induced RAW 264.7 cells. The researchers also observed that cordycepin could decrease the expression of NF-κB p65 in LPS-exposed RAW 264.7 macrophage cells. In summary, this study demonstrated that cordycepin has antiinflammatory and antidiabetic effects on macrophages, and that these effects might be related to the NF-κB pathway. In the same year, Kim’s group demonstrated that cordycepin could switch the phenotype of macrophages to M2 macrophage (Shin et al., 2009). They used 5, 10, 20, or 40 μg/ml cordycepin to treat LPS-induced RAW 264.7 cells to analyze the phenotypic switching in macrophage cells. The researchers found that cordycepin reduced the secretion of M1 cytokines, such as IL-1β and TNF-α, and the expression of M1 chemokine and its receptors, such as CX3CR1, CX3CL1, and RANTES, in LPS-induced RAW 264.7 macrophage cells. M2 cytokines such as IL-1ra, IL-10, and TGF-β were upregulated in cordycepin-treated LPS-induced RAW 264.7 macrophages. The authors suggested that cordycepin could induce macrophages to differentiate into M2 macrophages.
Microglia, a type of macrophage present in brain tissue, is instrumental in the host defense and tissue repair in the central nervous system. An abnormal immune response might cause severe neurodegenerative diseases, such as Alzheimer disease, Parkinson disease, multiple sclerosis, trauma, and cerebral ischemia (Sack et al., 2018). Jeong et al. (2010) demonstrated that cordycepin could regulate the immune response of BV2 microglia cells and might, consequently, be a potential therapeutic reagent for neurodegenerative disease (Jeong et al., 2010). When treating LPS-induced BV2 microglia with 1, 2.5, 5, or 7.5 μg/ml cordycepin, nitric oxide and PGE2 expression were reduced. In addition, the iNOS, COX-2, TNF-α, and IL-1β expression levels also decreased in cordycepin-treated LPS-induced BV2 microglia. The researchers also examined whether the inhibition of immune response might be associated with the suppression of the NF-κB, Akt, and MAPK signaling pathways. They argued that cordycepin could modulate microglia cells and could be used to treat neurodegenerative disease. The mechanism by which cordycepin regulated the NF-κB pathway was investigated by Ren et al. (2012) using TNF-α-activated transfected NF-κB Luc and an empty pcDNA3.1 vector HEK-293 T (Ren et al., 2012). They found that cordycepin did not block the nuclear translocation of the p65 subunit. Cordycepin inhibited the nuclear factor of the kappa light polypeptide gene enhancer in B cells and alpha (IκBα) phosphorylation, reducing IκBα degradation. This mechanism might be associated with cordycepin inhibiting of IκB kinase gamma [IKKγ, also known as NF-κB essential modifier (NEMO)] degradation and IKKγ binding to IKKα/β to reduce their ability to phosphorylate IκBα. The researchers proposed that cordycepin'|’s regulation of the NF-κB pathway might be related to the decreased IKKγ degradation, which results in decreasing IKKα/β activity to phosphorylate IκBα.
Cordycepin could supress T cell activation (Xiong et al., 2013). Cordycepin in dosages of 10, 20, or 40 μg/ml could decrease the proliferation of concanavalin A–induced splenocytes in a dose-dependent manner. Results exhibited that cordycepin could reduce both Th1 (IL-2 and IFN-γ) and Th2 cytokine (IL-4 and IL-6) secretion. The researchers proposed that cordycepin’s suppression of concanavalin A–induced T cell activation might be attributed to its reduction of phosphorylated IκB levels and nuclear factor of activated T cells 2 (NFAT2). In addition, the DNFB-induced delayed-type hypersensitivity reaction could be reduced in cordycepin-treated mice. They concluded that cordycepin’s suppression of T cell activation in vitro and in vivo might be associated with the NFAT2 signaling pathway.
The inhibitory effects of cordycepin-treated LPS-induced macrophage activation might be associated with the TLR4-mediated MAPK and NF-κB pathways (Choi et al., 2014). Cordycepin at dosages of 10, 20, or 30 μg/ml were used to treat LPS-induced RAW 264.7 cells, which led to reduced expression levels of iNOS and COX-2. The expression of IL-1β and TNF-α were also reduced after LPS-induced macrophages were treated with cordycepin. Results indicated that p65 expression decreased in the nucleus and IκB expression increased in the cytosol after pretreating LPS-induced RAW 264.7 cells with cordycepin. The researchers found that MAPK activity could be suppressed in cordycepin-treated LPS-induced RAW 264.7 cells. Furthermore, LPS-induced TLR4 and myeloid differentiation primary response 88 (MYD88) expression was abolished by cordycepin treatment. The authors illustrated that the inhibitory effects of cordycepin on LPS-stimulated RAW 264.7 cells are related to the suppression of the MAPK pathway and the activation of NF-κB by inhibition of the TLR4 signaling pathway.
Cordycepin could alleviate asthma in a mice model through the inhibition of the Th2-type immune response (Yang et al., 2015). In OVA-induced asthma, cordycepin treatment led to a reduction in serum IgE in a dose-dependent manner in comparison with that in the nontreated controls. The researchers observed that eotaxin, ICAM-1, and Th2 cytokines (IL-4, IL-5, and IL-13) in the bronchoalveolar lavage fluid (BALF) were significantly reduced in cordycepin-treated mice. In addition, they found that cordycepin could reduce the total infiltrated cells in the BALF—including eosinophils, neutrophils, macrophages, and lymphocytes. The authors also evaluated the preventive effects of cordycepin on airway inflammation and mucus hypersecretion in a pathological study. The study demonstrated that the aforementioned phenomenon is potentially associated with the blockage of the p38-MAPK and NF-κB signaling pathways.
Cordycepin was reported by Yuan et al. (2016) to protect the integrity of the blood-brain barrier (BBB) in a traumatic brain injury rat model (Yuan et al., 2016). They intravenously injected 1, 5, 10, or 20 mg/kg cordycepin for 30 min to treat traumatic brain injury in rats. The results revealed that the neurological severity score, brain infarction volume, and brain water content were considerably reduced in the cordycepin-treated group in a dose-dependent manner compared with saline-treated rats. The researchers also found that the two major proteins for BBB integrity, ZO-1 and occludin, were restored in rats treated with 20 mg/kg of cordycepin. Furthermore, the study revealed that levels of IL-1β, iNOS, myeloperoxidase, matrix metalloproteinases-9, and nicotinamide adenine dinucleotide phosphate oxidase-1 were reduced and those of arginase and IL-10 were increased in cordycepin-treated rats compared with those in the nontreated controls. This research exhibited that cordycepin could serve as a reagent to protect BBB integrity.
Cordycepin was found by Yoou et al. (2017) to be capable of regulate mast cell proliferation (Yoou et al., 2017). Thymic stromal lymphopoietin (TSLP) is a cytokine that is associated with atopic dermatitis. The researchers used 0.1, 1, or 10 µM of cordycepin to treat TSLP-induced human mast cells (HMC-1) and found that cordycepin could reduce IL-13 secretion in a dose-dependent manner. Phosphorylation signal transducer and activator of transcription (pSTAT6) is a well-known protein that is associated with TSLP-induced mast cell proliferation. Results of this study indicated that TSLP-induced HMC-1 cell expression of pSTAT6 could be abolished in the cordycepin-treated group. Furthermore, the researchers indicated that cordycepin could downregulate murine double minute 2 (MDM2) and Bcl-2 expression and upregulate p53, caspase 3, and cleaved poly ADP-ribose polymerase (PARP) levels in TSLP-induced HMC-1 cells. They also revealed that TSLP-induced IL-6, TNF-α, and IL-1β were reduced in cordycepin-treated TSLP-induced HMC-1 cells. This study successfully demonstrated that cordycepin could reduce IL-13 and MDM2 expression in TSLP-induced HMC-1 cells.
Cordycepin was reported by Fei et al. (2017) to be capable of inhibiting airway remodeling in a chronic asthma rat model (Fei et al., 2017). A dosage of 50 mg/kg of cordycepin with or without budesonide was used to treat rats with OVA-induced asthma to investigate potential underlying molecular mechanisms. The results indicated that cotreatment with cordycepin and budesonide synergistically reduced the total cells, eosinophils, and basophils in the BALF of rats with OVA-induced asthma. In addition, cordycepin with budesonide could reduce the cytokine expression levels of IL-5, IL-13, and TNF-α and the IgE level more than cordycepin without budesonide. The authors also found that cordycepin with budesonide more greatly reduced the thickness of the bronchial wall than cordycepin without budesonide. Notably, they observed that cordycepin with or without budesonide could markedly inhibit the p38-MAPK pathway and upregulate the adenosine A2A receptor. However, budesonide alone did not have the same effect. The researchers revealed that the synergistic effects of cordycepin with budesonide on cytokine expression, IgE secretion, and airway remodeling might be due to the p38-MAPK pathway and A2A receptor.
Cordycepin was discovered to regulate neuroimmune response by Du’s group (2019). They demonstrated that the administration of cordycepin through IP injection in mice model could reduce anxious behavior. The authors further evaluated the possible mechanism and found that cordycepin could increase IL-4 and IL-10 expression and decrease TNF-α and IL-1β expression in prefrontal cortex. IL-4 expression has now been demonstrated to be very strongly positively correlated with reduced anxiety (Gao et al., 2019).
Cordycepin was shown to suppress T cell activity by Yang’s group (2020) (Wang et al., 2020). Mice received IP injections of different concentrations of cordycepin daily for 2 weeks, followed by an intradermal injection of Complete Freund’s Adjuvant into their hind paw to test for an anti-inflammation effect. They found that cordycepin could reduce inflammation phenomenon including the thymus inhibition, spleen enlargement, and the reduction of edema and T cell infiltration in mice paws. In addition, they showed that cordycepin could reduce T cell proliferation and IL-2 expression. They further investigated the possible mechanism and found that cordycepin could inhibit the T cell receptor signaling pathway by reducing TAP-70 and Erk phosphorylation.
In this section, we found that cordycepin is capable of suppressing macrophage activity by, for example, reducing the expression of iNOS, COX-2, and TNF-α, which might relieve some disease symptoms—such as those of type II diabetes and traumatic brain injury. Researchers have also indicated that cordycepin can directly inhibit T cell activation and proliferation after stimulation through injection with Complete Freund'|’s Adjuvant, a widely accepted Th1-response dominant type of stimulation. Cordycepin can act as an anxiolytic reagent because it upregulates IL-4 expression in prefrontal cortex, which is negatively correlated with the frequency of anxious behavior. In addition, some researchers have indicated that cordycepin has some beneficial effects in OVA-induced asthma and DNFB-induced delayed-type hypersensitivity reaction.
Trace Elements and Powders
Trace elements are essential to immune regulation and infection prevention (Li et al., 2019). The action of C. militaris trace elements and their ability to counteract the influenza virus were analyzed by Wang and Hou (2011) (Wang and Hou, 2011). They orally administered 4 mg/kg/day of C. militaris extracts to treat H1N1-infected mice and monitored mortality and levels of Cu, Zn, Mg, Mn, Se, and Fe in the serum. The researchers illustrated that H1N1-infected mice treated with the extract had lower mortality than did the nontreated controls (survival rates of all control and C. militaris treatment groups were 25 and 50%, respectively). The concentrations of Cu, Zn, Mg, Mn, Se, and Fe in the serum (μg/ml) were 1.11 ± 0.51, 0.69 ± 0.53, 15.68 ± 1.53, 0.008 ± 0.53, 0.26 ± 0.53, and 0.16 ± 0.03, respectively. Zn, Mg, and Se were significantly increased in mice treated with C. militaris extracts compared with corresponding levels in the controls. The researchers posited that Zn, Mg, and Se might play direct or indirect roles in counteracting the influenza virus through stimulating the host immune response.
The effect of C. militaris powder on the immunoregulation function of healthy volunteers was investigated by Sun et al. (Sun et al., 2014). The researchers treated 12 volunteers with 0.5, 1.5, or 3 g of C. militaris powder for one day and then analyzed and compared their blood samples before and after treatment. In the low-dosage group, the authors observed that eotaxin, fibroblast growth factor-2, growth regulated oncogene, and monocyte chemoattractant protein-1 were downregulated after treatment with the extract. In the medium-dosage group, the expression of growth regulated oncogene, sCD40L, and TNF-α were downregulated, and in the high-dosage group, IL-12, IFN-γ, inducible protein 10, monocyte chemoattractant protein-1, and macrophage inflammatory protein-1β were reduced. The researchers suggested that C. militaris powder, by virtue of its ability to reduce the excessive immune response, may play an inhibitory role in tumorigenesis and serve as an immunomodulator to treat some diet- and autoimmune-related diseases.
The available data are limited, therefore the immunoregulation trends of trace elements and powders of C. militaris remain unclear.
Discussion
Novel researches on the immunomodulatory effects of C. militaris are summarized in Figure 4 according to its form: total extract, polysaccharides and cordycepin. Notably, we found that the investigators that used total extracts by water or 50% ethanol and the polysaccharides of C. militaris were likely to result in an immune response to type 1 immunity, such as increased TNF-α secretion in macrophages, decreased OVA-induced asthma in a mice model, or enhanced NK cells activities. In contrast, those using 70–80% ethanol extracts and cordycepin of C. militaris were more likely to obtain an immune response to type 2 immunity. However, some studies have indicated that cordycepin might also reduce OVA-induced asthma.
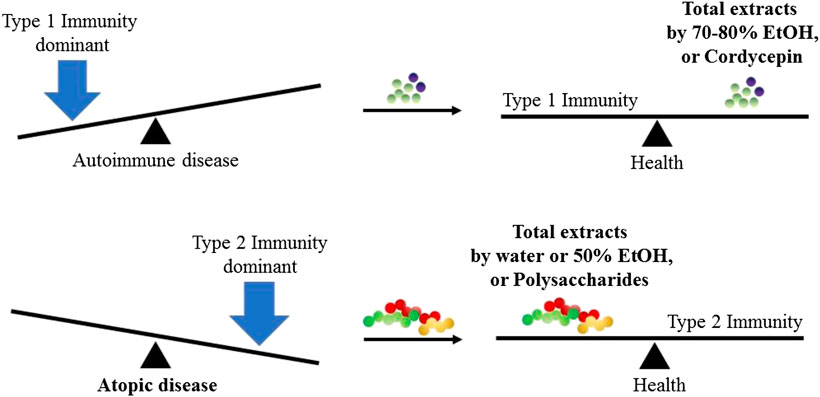
FIGURE 4. Immunomodulatory effects from different ingredients of C. militaris. In normal condition, host immune system always reach the balance of type 1 immunity and type 2 immunity. When the balance is broken, the host might suffer from the disease.
The immunomodulatory mechanism of total extract obtained using water or different concentration of ethanol is unclear. However, some studies have reported that the activation of macrophages and dendritic cells might be related with activating IL-18 or NF-κB pathway (Kim et al., 2008; Shin, Kwon, et al., 2010; Shin, Park, et al., 2010) and the effect of antiallergic effects might be associated with lowering the expression level of the phosphorylation Syk, ERK, p38 and JNK (Oh et al., 2011). In addition, we suspect that the different immunomodulatory effects of C. militaris extracts obtained using different solvents may be associated with the polarity of the final product. Water extract with a low concentration of ethanol extract might produce high polarity product which prone to drive our immune system to type1 immunity; while a high concentration of ethanol extract might result in low polarity product that might drive our immune system to type2 immunity. The detail mechanisms of total extract by different solution should be further investigated.
The immunomodulatory mechanism of polysaccharide in Dendrobium (reported by the first author of this article in 2017) may be associated with TLR4. However, the polysaccharide in C. militaris appeared to be related not only to TLR4 but also to TLR2 and dectin-1 (Lee et al., 2017). In future research, we might compare the polysaccharide structures of these two commonly used herbs in traditional Chinese medicine to clarify their immunomodulatory effects. The immunomodulatory mechanism of cordycepin in different immune cells is related to different pathways. In macrophages, cordycepin reduces the activation of NF-κB pathway; while in T-lymphocytes, cordycepin can suppress this activity and may be associated with the NFAT2 signaling pathway; finally, in mast cells, cordycepin can reduce the IL-13 and MDM2 expression in TSLP-induced HMC-1 cells.
The treatment of an excessive immune response caused by microbe infection, also known as a cytokine storm, is a critical field of research because it cytokine storms cause severe tissue damage, poor outcomes, and even death (Tisoncik et al., 2012; D'Elia et al., 2013; Guo and Thomas, 2017; Srikiatkhachorn et al., 2017). Here, we found that cordyceps extracts, namely a polysaccharide called APS, and trace elements from C. militaris might have beneficial effects in an influenza-induced cytokine storm and could reduce mortality in infected mice. Under normal conditions, a host immune system always reaches a balance between type 1 and type 2 immunity. When the balance is broken, the host might become ill. In this review, we found that total extracts using 70–80% ethyl alcohol and cordycepin of C. militaris were likely to result in type 2 host immunity, whereas polysaccharides and total extract by water or 50% ethyl alcohol of C. militaris were likely to result in type 1 immunity. This conclusion might serve as a principle to adjust our immune system and improve our health.
Conclusion
We found that different C. militaris extracts have different immunomodulatory functions. Total extracts using water or 50% ethanol and polysaccharides from C. militaris could be used to treat tumors, allergies, and viral infections because these ingredients tend to drive the immune response toward type 1 immunity. Total extracts using 70–80% ethanol and cordycepin from C. militaris could be used as immunosuppressive agents for delayed-type hypersensitivity and tend to drive the immune response toward type 2 immunity. Thus, this review elucidated the bioactive compounds of C. militaris and their immunomodulatory effects.
Author Contributions
CTL, KSH, JFS, JRC, WSK, GS, AMG, AHH and CHY organized the main text. CTL, KSH, YTW, JSW, YPH, YML, HHH, and CHY prepared the Figure and Tables.
Funding
This work was financially supported by grants from I-Shou University and the Ministry of Science and Technology, Taiwan.
Conflict of Interest
The authors declare that the research was conducted in the absence of any commercial or financial relationships that could be construed as a potential conflict of interest.
Glossary
Ara arabinose
BBB blood-brain barrier
BALF bronchoalveolar lavage fluid
JNK c-Jun N-terminal kinase
CD cluster of differentiation
C. militaris Cordyceps militaris
cy cyclophosphamide
COX-2 cycloxygenase-2
DNFB 2,4-dinitro-1-fluorobenzene
ERK extracellular-signal-regulated kinase
Gal galactose
Glc glucose
EC50 half maximal effective concentration
IC50 half maximal inhibitory concentration
Th helper T
IgE immunoglobulin E
iNOS inducible nitric oxide synthase
IFN interferon
IL interleukin
ICAM-1 intracellular adhesion molecules-1
IP intraperitoneal
LPS lipopolysaccharides
IKKγ IκB kinase gamma
MHC major histocompatibility complex
Man mannose
MAPK mitogen-activated protein kinase
MW molecular weight
MYD88 myeloid differentiation primary response 88
NK natural killer
NEMO NF-κB essential modifier
NFAT2 nuclear factor of activated T cells 2
NF-κB nuclear factor kappa-light-chain-enhancer of activated B cells
IκBα nuclear factor of kappa-light polypeptide gene enhancer in B-cells inhibitor, alpha
OVA ovalbumin
p38 p38 mitogen-activated protein kinases
poly ADP-ribose polymerase (PARP
PGE2 prostaglandin E2
ROS reactive oxygen specie
Rha rhamnose
TSLP thymic stromal lymphopoietin
TLR toll-like receptor
TNF-α tumor necrosis factor-α
Syk tyrosine-protein kinase
Xyl xylose
References
Barcelos, M. C. S., Vespermann, K. A. C., Pelissari, F. M., and Molina, G. (2020). Current status of biotechnological production and applications of microbial exopolysaccharides. Crit. Rev. Food Sci. Nutr. 60 (9), 1475–1495. doi:10.1080/10408398.2019.1575791.
Beltrame, G., Trygg, J., Rahkila, J., Leino, R., and Yang, B. (2019). Structural investigation of cell wall polysaccharides extracted from wild Finnish mushroom Craterellus tubaeformis (Funnel Chanterelle). Food Chem. 301, 125255. doi:10.1016/j.foodchem.2019.125255.
Bi, S., Huang, W., Chen, S., Huang, C., Li, C., Guo, Z., et al. 2020). Cordyceps militaris polysaccharide converts immunosuppressive macrophages into M1-like phenotype and activates T lymphocytes by inhibiting the PD-L1/PD-1 axis between TAMs and T lymphocytes. Int. J. Biol. Macromol. 150, 261–280. doi:10.1016/j.ijbiomac.2020.02.050.
Bieber, T. (2008). Atopic dermatitis. N. Engl. J. Med. 358 (14), 1483–1494. doi:10.1056/nejmra074081.
Cheung, J. K. H., Li, J., Cheung, A. W. H., Zhu, Y., Zheng, K. Y. Z., Bi, C. W. C., et al. 2009). Cordysinocan, a polysaccharide isolated from cultured Cordyceps, activates immune responses in cultured T-lymphocytes and macrophages: signaling cascade and induction of cytokines. J. Ethnopharmacol. 124 (1), 61–68. doi:10.1016/j.jep.2009.04.010.
Choi, Y. H., Kim, G.-Y., and Lee, H. H. (2014). Anti-inflammatory effects of cordycepin in lipopolysaccharide-stimulated RAW 264.7 macrophages through Toll-like receptor 4-mediated suppression of mitogen-activated protein kinases and NF-κB signaling pathways. Dddt 8, 1941–1953. doi:10.2147/dddt.s71957.
Chu, H.-L., Chien, J.-C., and Duh, P.-D. (2011). Protective effect of Cordyceps militaris against high glucose-induced oxidative stress in human umbilical vein endothelial cells. Food Chem. 129 (3), 871–876. doi:10.1016/j.foodchem.2011.05.037.
Cui, J. D. (2015). Biotechnological production and applications ofCordyceps militaris, a valued traditional Chinese medicine. Crit. Rev. Biotechnol. 35 (4), 475–484. doi:10.3109/07388551.2014.900604.
Cunningham, K. G., Manson, W., Spring, F. S., and Hutchinson, S. A. (1950). Cordycepin, a metabolic product isolated from cultures of Cordyceps militaris (Linn.) Link. Nature 166 (4231), 949. doi:10.1038/166949a0.
D'Elia, R. V., Harrison, K., Oyston, P. C., Lukaszewski, R. A., and Clark, G. C. (2013). Targeting the “cytokine storm” for therapeutic benefit. Clin. Vaccine Immunol. 20 (3), 319–327. doi:10.1128/cvi.00636-12.
Das, S. K., Masuda, M., Sakurai, A., and Sakakibara, M. (2010). Medicinal uses of the mushroom Cordyceps militaris: current state and prospects. Fitoterapia 81 (8), 961–968. doi:10.1016/j.fitote.2010.07.010.
Dong, J. Z., Ding, J., Yu, P. Z., Lei, C., Zheng, X. J., and Wang, Y. (2013). Composition and distribution of the main active components in selenium-enriched fruit bodies of Cordyceps militaris link. Food Chem. 137 (1–4), 164–167. doi:10.1016/j.foodchem.2012.10.021.
Fei, X., Zhang, X., Zhang, G.-q., Bao, W.-p., Zhang, Y.-y., Zhang, M., et al. (2017). Cordycepin inhibits airway remodeling in a rat model of chronic asthma. Biomed. Pharmacother. 88, 335–341. doi:10.1016/j.biopha.2017.01.025.
Fitzgerald, K. A., and Kagan, J. C. (2020). Toll-like receptors and the control of immunity. Cell 180 (6), 1044–1066. doi:10.1016/j.cell.2020.02.041.
Gao, T., Li, B., Hou, Y., Luo, S., Feng, L., Nie, J., et al. (2019). Interleukin-4 signalling pathway underlies the anxiolytic effect induced by 3-deoxyadenosine. Psychopharmacology 236 (10), 2959–2973. doi:10.1007/s00213-019-5186-7.
Gause, W. C., Wynn, T. A., and Allen, J. E. (2013). Type 2 immunity and wound healing: evolutionary refinement of adaptive immunity by helminths. Nat. Rev. Immunol. 13 (8), 607–614. doi:10.1038/nri3476.
Gieseck, R. L., Wilson, M. S., and Wynn, T. A. (2018). Type 2 immunity in tissue repair and fibrosis. Nat. Rev. Immunol. 18 (1), 62–76. doi:10.1038/nri.2017.90.
Guo, X.-z. J., and Thomas, P. G. (2017). New fronts emerge in the influenza cytokine storm. Semin. Immunopathol. 39 (5), 541–550. doi:10.1007/s00281-017-0636-y.
Guo, Z., Chen, W., Dai, G., and Huang, Y. (2020). Cordycepin suppresses the migration and invasion of human liver cancer cells by downregulating the expression of CXCR4. Int. J. Mol. Med. 45 (1), 141–150. doi:10.3892/ijmm.2019.4391.
Han, E. S., Oh, J. Y., and Park, H.-J. (2011). Cordyceps militaris extract suppresses dextran sodium sulfate-induced acute colitis in mice and production of inflammatory mediators from macrophages and mast cells. J. Ethnopharmacol. 134 (3), 703–710. doi:10.1016/j.jep.2011.01.022.
He, B.-L., Zheng, Q.-W., Guo, L.-Q., Huang, J.-Y., Yun, F., Huang, S.-S., et al. (2020). Structural characterization and immune-enhancing activity of a novel high-molecular-weight polysaccharide from Cordyceps militaris. Int. J. Biol. Macromol. 145, 11–20. doi:10.1016/j.ijbiomac.2019.12.115.
He, X., Fang, J., Guo, Q., Wang, M., Li, Y., Meng, Y., et al. (2020). Advances in antiviral polysaccharides derived from edible and medicinal plants and mushrooms. Carbohydr. Polym. 229, 115548. doi:10.1016/j.carbpol.2019.115548.
Hsu, C.-H., Sun, H.-L., Sheu, J.-N., Ku, M.-S., Hu, C.-M., Chan, Y., et al. (2008). Effects of the immunomodulatory agent Cordyceps militaris on airway inflammation in a mouse asthma model. Pediatrics & Neonatology 49 (5), 171–178. doi:10.1016/s1875-9572(09)60004-8.
Jeong, J.-W., Jin, C.-Y., Kim, G.-Y., Lee, J.-D., Park, C., Kim, G.-D., et al. (2010). Anti-inflammatory effects of cordycepin via suppression of inflammatory mediators in BV2 microglial cells. Int. Immunopharm. 10 (12), 1580–1586. doi:10.1016/j.intimp.2010.09.011.
Kang, H. J., Baik, H. W., Kim, S. J., Lee, S. G., Ahn, H. Y., Park, J. S., et al. (2015). Cordyceps militarisEnhances cell-mediated immunity in healthy Korean men. J. Med. Food 18 (10), 1164–1172. doi:10.1089/jmf.2014.3350.
Kawai, T., and Akira, S. (2010). The role of pattern-recognition receptors in innate immunity: update on toll-like receptors. Nat. Immunol. 11 (5), 373–384. doi:10.1038/ni.1863.
Kay, A. B. (2001a). Allergy and allergic diseases. N. Engl. J. Med. 344 (1), 30–37. doi:10.1056/nejm200101043440106.
Kay, A. B. (2001b). Allergy and allergic diseases. N. Engl. J. Med. 344 (2), 109–113. doi:10.1056/nejm200101113440206.
Khan, M. A., and Tania, M. (2020). Cordycepin in anticancer research: molecular mechanism of therapeutic effects. Cmc 27 (6), 983–996. doi:10.2174/0929867325666181001105749.
Kim, C. S., Lee, S.-Y., Cho, S.-H., Ko, Y.-M., Kim, B.-H., Kim, H.-J., et al. (2008). Cordyceps militaris induces the IL-18 expression via its promoter activation for IFN-γ production. J. Ethnopharmacol. 120 (3), 366–371. doi:10.1016/j.jep.2008.09.010.
Kim, H. G., Shrestha, B., Lim, S. Y., Yoon, D. H., Chang, W. C., Shin, D. J., et al. (2006). Cordycepin inhibits lipopolysaccharide-induced inflammation by the suppression of NF-kappaB through Akt and p38 inhibition in RAW 264.7 macrophage cells. Eur. J. Pharmacol. 545 (2–3), 192–199. doi:10.1016/j.ejphar.2006.06.047.
Kim, H. S., Kim, J. Y., Kang, J. S., Kim, H. M., Kim, Y. O., Hong, I. P., et al. (2010). Cordlan polysaccharide isolated from mushroom Cordyceps militaris induces dendritic cell maturation through toll-like receptor 4 signalings. Food Chem. Toxicol. 48 (7), 1926–1933. doi:10.1016/j.fct.2010.04.036.
Kitamura, M., Kato, H., Saito, Y., Nakajima, S., Takahashi, S., Johno, H., et al. (2011). Aberrant, differential and bidirectional regulation of the unfolded protein response towards cell survival by 3′-deoxyadenosine. Cell Death Differ. 18 (12), 1876–1888. doi:10.1038/cdd.2011.63.
Lee, C. T., Kuo, H. C., Chen, Y. H., and Tsai, M. Y. (2017). Current advances in the biological activity of polysaccharides in dendrobium with intriguing therapeutic potential. Curr. Med. Chem. 25 (14), 1663–1681.
Lee, H. H., Park, H., Sung, G.-H., Lee, K., Lee, T., Lee, I., et al. (2014). Anti-influenza effect of Cordyceps militaris through immunomodulation in a DBA/2 mouse model. J. Microbiol. 52 (8), 696–701. doi:10.1007/s12275-014-4300-0.
Lee, J. S., and Hong, E. K. (2011). Immunostimulating activity of the polysaccharides isolated from Cordyceps militaris. Int. Immunopharm. 11 (9), 1226–1233. doi:10.1016/j.intimp.2011.04.001.
Lee, J. S., Kwon, D. S., Lee, K. R., Park, J. M., Ha, S.-J., and Hong, E. K. (2015). Mechanism of macrophage activation induced by polysaccharide from Cordyceps militaris culture broth. Carbohydr. Polym. 120, 29–37. doi:10.1016/j.carbpol.2014.11.059.
Lee, J. S., Kwon, J. S., Won, D. P., Lee, K. E., Shin, W. C., and Hong, E. K. (2010). Study on macrophage activation and structural characteristics of purified polysaccharide from the liquid culture broth of Cordyceps militaris. Carbohydr. Polym. 82, 982–988. doi:10.1016/j.carbpol.2010.06.025.
Lee, J. S., Kwon, J. S., Yun, J. S., Pahk, J. W., Shin, W. C., Lee, S. Y., et al. (2010). Structural characterization of immunostimulating polysaccharide from cultured mycelia of Cordyceps militaris. Carbohydr. Polym. 80, 1011–1017. doi:10.1016/j.carbpol.2010.01.017.
Li, J., Shen, B., Nie, S., Duan, Z., and Chen, K. (2019). A combination of selenium and polysaccharides: promising therapeutic potential. Carbohydr. Polym. 206, 163–173. doi:10.1016/j.carbpol.2018.10.088.
Lin, B., and Li, S. (2011). “Cordyceps as an herbal drug,” in Herbal medicine: biomolecular and clinical aspects. Editors I. F. F., Benzie, and S., Wachtel-Galor (Boca Raton, FL).
Lin, Y.-S., Yang, C.-H., Lu, K., Huang, K.-S., and Zheng, Y.-Z. (2011). Synthesis of agar microparticles using temperature-controlled microfluidic devices for Cordyceps militaris cultivation. Electrophoresis 32 (22), 3157–3163. doi:10.1002/elps.201100343.
Liu, J.-y., Feng, C.-p., Li, X., Chang, M.-c., Meng, J.-l., and Xu, L.-j. (2016a). Immunomodulatory and antioxidative activity of Cordyceps militaris polysaccharides in mice. Int. J. Biol. Macromol. 86, 594–598. doi:10.1016/j.ijbiomac.2016.02.009.
Liu, X.-C., Zhu, Z.-Y., Tang, Y.-L., Wang, M.-f., Wang, Z., Liu, A.-J., et al. (2016b). Structural properties of polysaccharides from cultivated fruit bodies and mycelium of Cordyceps militaris. Carbohydr. Polym. 142, 63–72. doi:10.1016/j.carbpol.2016.01.040.
Lloyd, C. M., and Snelgrove, R. J. (2018). Type 2 immunity: expanding our view. Sci. Immunol. 3 (25), eaat1604. doi:10.1126/sciimmunol.aat1604.
Lozupone, C. A. (2018). Unraveling interactions between the microbiome and the host immune system to decipher mechanisms of disease. mSystems 3 (2), e00183–00117. doi:10.1128/msystems.00183-17.
Lu, R.-L., Bao, G.-H., Hu, F.-L., Huang, B., Li, C.-R., and Li, Z.-Z. (2014). Comparison of cytotoxic extracts from fruiting bodies, infected insects and cultured mycelia of Cordyceps formosana. Food Chem. 145, 1066–1071. doi:10.1016/j.foodchem.2013.09.001.
Luo, X., Duan, Y., Yang, W., Zhang, H., Li, C., and Zhang, J. (2017). Structural elucidation and immunostimulatory activity of polysaccharide isolated by subcritical water extraction from Cordyceps militaris. Carbohydr. Polym. 157, 794–802. doi:10.1016/j.carbpol.2016.10.066.
McInnes, I. B., and Schett, G. (2011). The pathogenesis of rheumatoid arthritis. N. Engl. J. Med. 365 (23), 2205–2219. doi:10.1056/nejmra1004965.
Meng, X., Li, Y., Li, S., Gan, R.-Y., and Li, H.-B. (2018). Natural products for prevention and treatment of chemical-induced liver injuries. Compr. Rev. Food Sci. Food Saf. 17 (2), 472–495. doi:10.1111/1541-4337.12335.
Mohan, K., Muralisankar, T., Uthayakumar, V., Chandirasekar, R., Revathi, N., Ramu Ganesan, A., et al. 2020). Trends in the extraction, purification, characterisation and biological activities of polysaccharides from tropical and sub-tropical fruits - a comprehensive review. Carbohydr. Polym. 238, 116185. doi:10.1016/j.carbpol.2020.116185.
Moutsopoulos, N. M., Zerbe, C. S., Wild, T., Dutzan, N., Brenchley, L., DiPasquale, G., et al. 2017). Interleukin-12 and interleukin-23 blockade in leukocyte adhesion deficiency type 1. N. Engl. J. Med. 376 (12), 1141–1146. doi:10.1056/nejmoa1612197.
Ng, T. B.,, and Wang, H. X. (2005). Pharmacological actions of Cordyceps, a prized folk medicine. J Pharm Pharmacol., 57 (12), 1509–1519. doi:10.1211/jpp.57.12.0001.
Oh, J. Y., Choi, W.-S., Lee, C. H., and Park, H.-J. (2011). The ethyl acetate extract of Cordyceps militaris inhibits IgE-mediated allergic responses in mast cells and passive cutaneous anaphylaxis reaction in mice. J. Ethnopharmacol. 135 (2), 422–429. doi:10.1016/j.jep.2011.03.030.
Ohta, Y., Lee, J.-B., Hayashi, K., Fujita, A., Park, D. K., and Hayashi, T. (2007). In vivo anti-influenza virus activity of an immunomodulatory acidic polysaccharide isolated from Cordyceps militaris grown on germinated soybeans. J. Agric. Food Chem. 55 (25), 10194–10199. doi:10.1021/jf0721287.
Park, H.-J. (2015). Ethanol extract of Cordyceps militaris grown on germinated soybeans inhibits 2, 4-dinitrophenolfluorobenzene-induced allergic contact dermatitis. Journal of Functional Foods 17, 938–947. doi:10.1016/j.jff.2015.06.046.
Qin, P., Li, X., Yang, H., Wang, Z.-Y., and Lu, D. (2019). Therapeutic potential and biological applications of Cordycepin and metabolic mechanisms in Cordycepin-producing fungi. Molecules 24 (12), 2231. doi:10.3390/molecules24122231.
Ren, Z., Cui, J., Huo, Z., Xue, J., Cui, H., Luo, B., et al. (2012). Cordycepin suppresses TNF-α-induced NF-κB activation by reducing p65 transcriptional activity, inhibiting IκBα phosphorylation, and blocking IKKγ ubiquitination. Int. Immunopharm. 14 (4), 698–703. doi:10.1016/j.intimp.2012.10.008.
Rodrigues, C., Sousa, C., Lopes, J. A., Novais, Â., and Peixe, L. (2020). A front line on klebsiella pneumoniae capsular polysaccharide knowledge: fourier transform infrared spectroscopy as an accurate and fast typing tool. mSystems 5 (2), e00386–00319. doi:10.1128/msystems.00386-19.
Ruthes, A. C., Smiderle, F. R., and Iacomini, M. (2016). Mushroom heteropolysaccharides: a review on their sources, structure and biological effects. Carbohydr. Polym. 136, 358–375. doi:10.1016/j.carbpol.2015.08.061.
Sacks, D., Sacks, D., Baxter, B., Campbell, B. C. V., Carpenter, J. S., Cognard, C., et al. 2018). Multisociety consensus quality improvement revised consensus statement for endovascular therapy of acute ischemic stroke. Int. J. Stroke 13 (6), 612–632. doi:10.1177/1747493018778713.
Schnaar, R. L. (2016). Glycobiology simplified: diverse roles of glycan recognition in inflammation. J. Leukoc. Biol. 99 (6), 825–838. doi:10.1189/jlb.3ri0116-021r.
Shapouri-Moghaddam, A., Mohammadian, S., Vazini, H., Taghadosi, M., Esmaeili, S.-A., Mardani, F., et al. (2018). Macrophage plasticity, polarization, and function in health and disease. J. Cell. Physiol. 233 (9), 6425–6440. doi:10.1002/jcp.26429.
Shen, H.-S., Shao, S., Chen, J.-C., and Zhou, T. (2017). Antimicrobials from mushrooms for assuring food safety. Compr. Rev. Food Sci. Food Saf. 16 (2), 316–329. doi:10.1111/1541-4337.12255.
Shin, S., Kwon, J., Lee, S., Kong, H., Lee, S., Lee, C.-K., et al. (2010). Immunostimulatory effects ofCordyceps militarison macrophages through the enhanced production of cytokines via the activation of NF-κB. Immune Netw 10 (2), 55–63. doi:10.4110/in.2010.10.2.55.
Shin, S., Lee, S., Kwon, J., Moon, S., Lee, S., Lee, C.-K., et al. (2009). Cordycepin suppresses expression of diabetes regulating genes by inhibition of lipopolysaccharide-induced inflammation in macrophages. Immune Netw 9 (3), 98–105. doi:10.4110/in.2009.9.3.98.
Shin, S., Moon, S., Park, Y., Kwon, J., Lee, S., Lee, C.-K., et al. (2009). Role of cordycepin and adenosine on the phenotypic switch of macrophages via induced anti-inflammatory cytokines. Immune Netw 9 (6), 255–264. doi:10.4110/in.2009.9.6.255.
Shin, S., Park, Y., Kim, S., Oh, H.-E., Ko, Y.-W., Han, S., et al. (2010). Cordyceps militarisEnhances MHC-restricted antigen presentation via the induced expression of MHC molecules and production of cytokines. Immune Netw 10 (4), 135–143. doi:10.4110/in.2010.10.4.135.
Sims, I. M., Frese, S. A., Walter, J., Loach, D., Wilson, M., Appleyard, K., et al. 2011). Structure and functions of exopolysaccharide produced by gut commensal Lactobacillus reuteri 100-23. ISME J. 5 (7), 1115–1124. doi:10.1038/ismej.2010.201.
Srikiatkhachorn, A., Mathew, A., and Rothman, A. L. (2017). Immune-mediated cytokine storm and its role in severe dengue. Semin. Immunopathol. 39 (5), 563–574. doi:10.1007/s00281-017-0625-1.
Sun, T., Dong, W., Jiang, G., Yang, J., Liu, J., Zhao, L., et al. (2019). Cordyceps militaris improves chronic kidney disease by affecting TLR4/NF-kappaB redox signaling pathway. Oxidative Medicine and Cellular Longevity 2019, 7850863. doi:10.1155/2019/7850863.
Sun, Y., Shao, Y., Zhang, Z., Wang, L., Mariga, A. M., Pang, G., et al. (2014). Regulation of human cytokines by Cordyceps militaris. J. Food Drug Anal. 22 (4), 463–467.doi:10.1016/j.jfda.2014.01.025.
Sun, Y., Zhang, M., and Fang, Z. (2019). Efficient physical extraction of active constituents from edible fungi and their potential bioactivities: a review. Trends in food science and technology 105, 468–482. doi:10.1016/j.tifs.2019.02.02
Tisoncik, J. R., Korth, M. J., Simmons, C. P., Farrar, J., Martin, T. R., and Katze, M. G. (2012). Into the eye of the cytokine storm. Microbiol. Mol. Biol. Rev. 76 (1), 16–32. doi:10.1128/mmbr.05015-11.
Wang, C., and Wang, S. (2017). Insect pathogenic fungi: genomics, molecular interactions, and genetic improvements. Annu. Rev. Entomol. 62, 73–90. doi:10.1146/annurev-ento-031616-035509.
Wang, L., and Hou, Y. (2011). Determination of trace elements in anti-influenza virus mushrooms. Biol. Trace Elem. Res. 143 (3), 1799–1807. doi:10.1007/s12011-011-8986-0.
Wang, M., Meng, X. Y., Yang, R. L., Qin, T., Wang, X. Y., Zhang, K. Y., et al. 2012). Cordyceps militaris polysaccharides can enhance the immunity and antioxidation activity in immunosuppressed mice. Carbohydr. Polym. 89 (2), 461–466. doi:10.1016/j.carbpol.2012.03.029.
Wang, X., Xi, D., Mo, J., Wang, K., Luo, Y., Xia, E., et al. (2020). Cordycepin exhibits a suppressive effect on T cells through inhibiting TCR signaling cascade in CFA-induced inflammation mice model. Immunopharmacol. Immunotoxicol. 42 (2), 119–127. doi:10.1080/08923973.2020.1728310.
Wang, Y., Stata, M., Wang, W., Stajich, J. E., White, M. M., and Moncalvo, J.-M. (2018). Comparative genomics reveals the core gene toolbox for the fungus-insect symbiosis. mBio 9 (3), e00636–18. doi:10.1128/mbio.00636-18.
Wang, Z., Chen, Z., Jiang, Z., Luo, P., Liu, L., Huang, Y., et al. (2019). Cordycepin prevents radiation ulcer by inhibiting cell senescence via NRF2 and AMPK in rodents. Nat. Commun. 10 (1), 2538. doi:10.1038/s41467-019-10386-8.
Won, S.-Y., and Park, E.-H. (2005). Anti-inflammatory and related pharmacological activities of cultured mycelia and fruiting bodies of Cordyceps militaris. J. Ethnopharmacol. 96 (3), 555–561. doi:10.1016/j.jep.2004.10.009.
Wu, L., Sun, H., Hao, Y., Zheng, X., Song, Q., Dai, S., et al. (2020). Chemical structure and inhibition on α-glucosidase of the polysaccharides from Cordyceps militaris with different developmental stages. Int. J. Biol. Macromol. 148, 722–736. doi:10.1016/j.ijbiomac.2020.01.178.
Xiang, R. K., Zhen, Y. Z., Xiao, J. Z., and Yong, M. Z. (2020). Effects of cordyceps polysaccharides on pasting properties and in vitro starch digestibility of wheat starch. Food Hydrocolloids 102, 1–8. doi:10.1016/j.foodhyd.2019.105604
Xiong, Y., Zhang, S., Xu, L., Song, B., Huang, G., Lu, J., et al. (2013). Suppression of T-cell activation in vitro and in vivo by cordycepin from Cordyceps militaris. J. Surg. Res. 185 (2), 912–922. doi:10.1016/j.jss.2013.06.057.
Yamaguchi, T., Takizawa, F., Fischer, U., and Dijkstra, J. (2015). Along the axis between Type 1 and Type 2 immunity; principles conserved in evolution from fish to mammals. Biology 4 (4), 814–859. doi:10.3390/biology4040814.
Yang, C.-H., Kao, Y.-H., Huang, K.-S., Wang, C.-Y., and Lin, L.-W. (2012). Cordyceps militaris and mycelial fermentation induced apoptosis and autophagy of human glioblastoma cells. Cell Death Dis. 3, e431. doi:10.1038/cddis.2012.172.
Yang, M., Belwal, T., Devkota, H. P., Li, L., and Luo, Z. (2019). Trends of utilizing mushroom polysaccharides (MPs) as potent nutraceutical components in food and medicine: a comprehensive review. Trends Food Sci. Technol. 92, 94–110. doi:10.1016/j.tifs.2019.08.009
Yang, X., Li, Y., He, Y., Li, T., Wang, W., Zhang, J., et al. (2015). Cordycepin alleviates airway hyperreactivity in a murine model of asthma by attenuating the inflammatory process. Int. Immunopharm. 26 (2), 401–408. doi:10.1016/j.intimp.2015.04.017.
Yoon, S., Park, S., and Park, Y. (2018). The anticancer properties of cordycepin and their underlying mechanisms. Ijms 19 (10), 3027. doi:10.3390/ijms19103027.
Yoou, M.-s., Yoon, K. W., Choi, Y., Kim, H.-M., and Jeong, H.-J. (2017). Cordycepin diminishes thymic stromal lymphopoietin-induced interleukin-13 production. Eur. J. Pharmacol. 802, 1–6. doi:10.1016/j.ejphar.2017.02.033.
Yu, R., Song, L., Zhao, Y., Bin, W., Wang, L., Zhang, H., et al. (2004). Isolation and biological properties of polysaccharide CPS-1 from cultured Cordyceps militaris. Fitoterapia 75 (5), 465–472. doi:10.1016/j.fitote.2004.04.003.
Yuan, J., Wang, A., He, Y., Si, Z., Xu, S., Zhang, S., et al. (2016). Cordycepin attenuates traumatic brain injury-induced impairments of blood-brain barrier integrity in rats. Brain Res. Bull. 127, 171–176. doi:10.1016/j.brainresbull.2016.09.010.
Yue, K., Ye, M., Zhou, Z., Sun, W., and Lin, X. (2013). The genusCordyceps: a chemical and pharmacological review. J. Pharm. Pharmacol. 65 (4), 474–493. doi:10.1111/j.2042-7158.2012.01601.x.
Zhang, A.-X., Mouhoumed, A.-Z., Tong, S.-M., Ying, S.-H., and Feng, M.-G. (2019). BrlA and AbaA govern virulence-required dimorphic switch, conidiation, and pathogenicity in a fungal insect pathogen. mSystems 4 (4), e00140. doi:10.1128/msystems.00140-19.
Zhang, F. L., Yang, X. F., Wang, D., Lei, S. R., Guo, L. A., Liu, W. J., et al. (2020a). A simple and effective method to discern the true commercial Chinese cordyceps from counterfeits. Sci. Rep. 10 (1), 2974. doi:10.1038/s41598-020-59900-9.
Zhang, J., Wen, C., Duan, Y., Zhang, H., and Ma, H. (2019). Advance in Cordyceps militaris (Linn) link polysaccharides: isolation, structure, and bioactivities: a review. Int. J. Biol. Macromol. 132, 906–914. doi:10.1016/j.ijbiomac.2019.04.020.
Zhang, J., Wen, C., Zhang, H., Duan, Y., and Ma, H. (2020b). Recent advances in the extraction of bioactive compounds with subcritical water: a review. Trends Food Sci. Technol. 95, 183–195. doi:10.1016/j.tifs.2019.11.018.
Zhang, Y., Zeng, Y., Cui, Y., Liu, H., Dong, C., and Sun, Y. (2020c). Structural characterization, antioxidant and immunomodulatory activities of a neutral polysaccharide from Cordyceps militaris cultivated on hull-less barley. Carbohydr. Polym. 235, 115969. doi:10.1016/j.carbpol.2020.115969.
Zheng, L.-Y., Guo, X.-S., He, B., Sun, L.-J., Peng, Y., Dong, S.-S., et al. (2011). Genome-wide patterns of genetic variation in sweet and grain sorghum (Sorghum bicolor). Genome Biol. 12 (11), R114. doi:10.1186/gb-2011-12-11-r114.
Keywords: Cordyceps militaris, immunomodulation, polysaccharides, cordycepin, type 1 immunity, type 2 immunity
Citation: Lee C-T, Huang K-S, Shaw J-F, Chen J-R, Kuo W-S, Shen G, Grumezescu AM, Holban AM, Wang Y-T, Wang J-S, Hsiang Y-P, Lin Y-M, Hsu H-H and Yang C-H (2020) Trends in the Immunomodulatory Effects of Cordyceps militaris: Total Extracts, Polysaccharides and Cordycepin. Front. Pharmacol. 11:575704. doi: 10.3389/fphar.2020.575704
Received: 29 June 2020; Accepted: 14 October 2020;
Published: 30 November 2020.
Edited by:
Cheorl-Ho Kim, Sungkyunkwan University, South KoreaReviewed by:
Akihiko Sakurai, University of Fukui, JapanJavad Sharifi-Rad, Shahid Beheshti University of Medical Sciences, Iran
Shashidhar Ghatnur, Other, Rajasthan, India
Copyright © 2020 Lee, Huang, Shaw, Chen, Kuo, Shen, Grumezescu, Holban, Wang, Wang, Hsiang, Lin, Hsu and Yang. This is an open-access article distributed under the terms of the Creative Commons Attribution License (CC BY). The use, distribution or reproduction in other forums is permitted, provided the original author(s) and the copyright owner(s) are credited and that the original publication in this journal is cited, in accordance with accepted academic practice. No use, distribution or reproduction is permitted which does not comply with these terms.
*Correspondence: Chih-Hui Yang, Y2h5YW5nQGlzdS5lZHUudHc=
†These authors have contributed equally to this work and share senior authorship