- 1Department of Emergency Medicine, Faculty of Medicine, University of Debrecen, Debrecen, Hungary
- 2MTA-DE Momentum Genome Architecture and Recombination Research Group, Department of Biochemistry and Molecular Biology, Faculty of Medicine, University of Debrecen, Doctoral School of Molecular Cell and Immune Biology, Debrecen, Hungary
- 3Faculty of Pharmacy, University of Debrecen, Debrecen, Hungary
A serious adverse effect of cancer therapies is cardiovascular toxicity, which significantly limits the widespread use of antineoplastic agents. The promising new field of cardio-oncology offers the identification of potent anti-cancer therapeutics that effectively inhibit cancer cell proliferation without causing cardiotoxicity. Future introduction of recently identified cardio-safe compounds into clinical practice (including ERK dimerization inhibitors or BAX allosteric inhibitors) is expected to help oncologists avoid unwanted cardiological complications associated with therapeutic interventions.
1 Introduction
One of the most devastating adverse effects of anticancer treatments is cardiovascular toxicity, which significantly restricts the effective use of conventional and targeted tumor therapeutics (Cameron and Chen, 2018; Herrmann, 2020). Therapy-related cardiovascular diseases involve a wide range of disorders from thromboembolism, stroke, systemic and pulmonary hypertension, myocarditis, cardiac arrhythmias to even sudden cardiac death, which collectively contribute to an increased morbidity and mortality of cancer patients. In addition to cardiovascular damage, a significant fraction of cancer patients suffers from acute or chronic kidney disease, requiring renal replacement therapies (Czifra et al., 2013; Barta et al., 2014), resulting in an increased risk of atrial and ventricular arrhythmias, heart failure and other adverse effects (Szabó et al., 2020). Nevertheless, increased prevalence of cancer among patients with heart failure indicates common molecular triggers and risk factors that contribute to both diseases (de Boer et al., 2019; Meijers and De Boer, 2019). Regardless of hemodynamic impairment, heart failure stimulates tumor development by secreting various circulating factors (Meijers et al., 2018; Richards, 2018), suggesting a causal relationship between heart failure and tumorigenesis. These associations encourage the development of new cardio-safe strategies to increase the effectiveness of cancer therapies (Hetey et al., 2017; Boros-oláh et al., 2019).
The MAPK/ERK pathway is one of the major targets of anti-tumor therapies in several malignancies as gain-of-function mutations of this pathway are collectively associated with more than 90% of cancers (Nissan et al., 2013; Smorodinsky-Atias et al., 2020). The cascade involves the sequential activation of receptor tyrosine kinases (RTK), RAS GTPases, RAF kinases (MAP3K), MEK (MAP2K), which ultimately phosphorylates ERK (MAPK) (Lee et al., 2020). Activated ERK (extracellular signal-regulated kinase) is located at the bottom of the cascade phosphorylating hundreds of cytosolic and nuclear targets (Plotnikov et al., 2015) that mediate opposing signaling pathways leading to cell survival, apoptosis, cancer growth or cardiac disfunction (Figure 1).
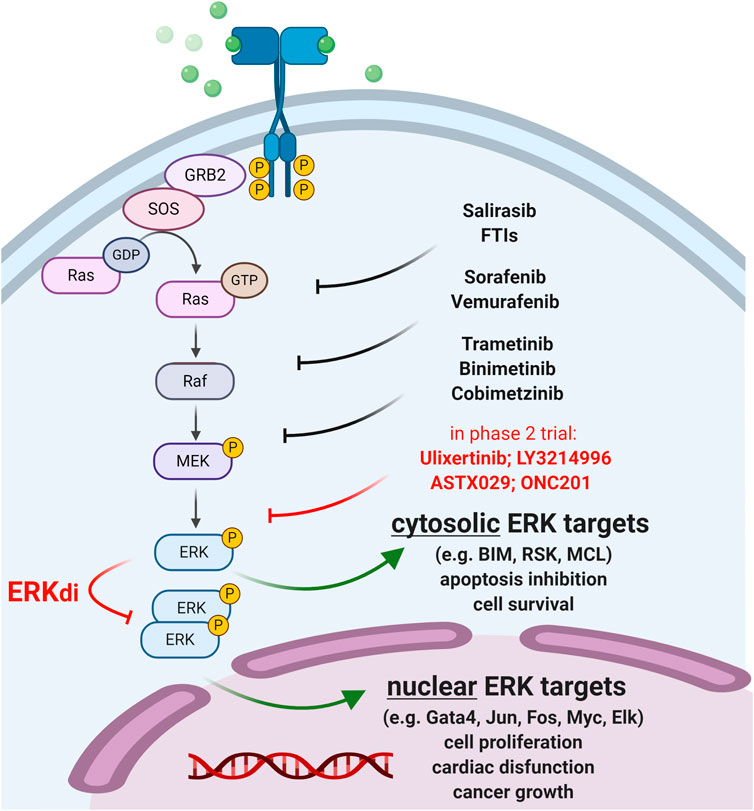
FIGURE 1. Therapeutic targeting of the MAPK/ERK pathway. Approved anti-cancer compounds targeting RAS/RAF/MEK are indicated in black. ERK inhibitors (ERKi) in phase 2 clinical trials are shown in red. Newly developed ERK dimerization inhibitors (ERKdi) are currently in pre-clinical phases. ERKdi compounds prevent nuclear translocation of ERK, thereby inhibiting the activation of transcription factors that cause tumor cell proliferation and mediate cardiac disfunction in normal cardiac tissue.
Therapeutic targeting of ERK is of key interest since ERK inhibitors (ERKi) have the potential to overcome common drug resistance to “upstream” MEK/RAF/RAS inhibitors (Ryan and Corcoran, 2018; Smorodinsky-Atias et al., 2020). Indeed, ERKi therapies are expected to show greater antitumor efficacy in advanced metastatic and multidrug-resistant tumors with reactivated MAPK signaling, however, their broad clinical use is limited (Lee et al., 2020). This is because ERK is associated with many diseases, such as heart failure, and thus ERKi therapy may cause severe cardiotoxic side effects. Multiple ERK inhibitors are currently in clinical trials for the treatment of RAS/RAF-mutated and BRAFi/MEKi-resistant tumors (Supplementary Table S1); however, new cardio-safe strategies need to be developed to overcome acquired resistance to MAPK inhibitor therapies.
In this respect, recently developed ERK-dimerization inhibitors (ERKdi) show potential to prevent tumorigenesis in preclinical models, without causing severe cardiotoxicity (Herrero et al., 2015; Tomasovic, 2020). The latest compound (Tomasovic, 2020) specifically blocks ERK1/2 dimer formation and consequent Thr188 phosphorylation (that is a prerequisite for ERK nuclear translocation, oncogenic activation and cardiotoxic side effects (Tomasovic, 2020)), preventing heart failure while preserving ERK1/2 catalytic activity and cytosolic survival signaling. This dual consequence of ERKdi treatment is in sharp contrast with the adverse effect of “upstream” MAPK/RAS inhibitors (e.g., MEKi, RAFi), which severely compromise cardiomyocyte mitochondria function and often lead to cardiac injury. The newly developed ERKdi drug may also be relevant in other diseases that rely on intensive ERK signaling, such as secondary cardiac injuries (e.g., due to hypertension, oxidative damage, ischemia), which are expected to extend therapeutic modalities to tumors resistant to upstream MAPK inhibitors.
In addition to MAPK inhibitors, doxorubicin (dox) is one of the most common anthracycline-based chemotherapeutics due to its broad spectrum and high efficacy; however, its applicability is highly limited by its severe cardiotoxic side-effects (Wallace et al., 2020). A recent study identified a new Sirt6–Tip60–Gata4 molecular axis that includes a histone deacetylase (Sirt6), a histone acetylase (Tip60), and a transcription factor (Gata4) that regulates an anti-apoptotic pathway and thus prevents dox-induced cardiotoxicity (Peng et al., 2020). Importantly, Gata4 is activated directly by ERK (via phosphorylation on serine 105 (Liang et al., 2001)) and thus inhibiting the deacetylase activity of Sirt6, which in turn disrupts the equilibrium in local histone acetylation. The resulting open chromatin allows the expression of cardiac-expressed genes involved in cardiac development and hypertrophy, as well as Bcl2 survival factors that suppress apoptosis and dox cardiotoxicity. Therefore, the Sirt6-Tip60-Gata4 axis represents a new therapeutic target to enhance the safety and efficacy of dox chemotherapy.
In another recent attempt, dox-based tumor therapy was made cardio-safe in combination with a specific Bax inhibitor (Amgalan et al., 2020). When dox was administered with a newly developed Bax allosteric inhibitor that modulate Bax function by conformational changes (BAI1 (Garner et al., 2019), Figure 2), cardiomyopathy was completely prevented without compromising the tumor-killing efficacy of dox treatment (Amgalan et al., 2020). The differential effect of Bax inhibition on cardiomyocytes vs. cancerous cells was attributed to the ubiquitously high Bax expression of cancer cells relative to the heart tissue (see e.g., https://www.proteinatlas.org/ENSG00000087088-BAX/pathology; (Thul et al., 2017)). High Bax levels in tumors may help suspend the inhibitory effect of the drug, promoting the apoptosis of cancer cells. These associations designate Bax as a potent tumor-selective drug target to prevent dox-induced cardiotoxicity. While it remains to be tested, the compound may also be used to prevent cardiomyopathies in other diseases in which Bax-mediated apoptotic and necrotic pathways are involved (e.g., infarction, stroke).
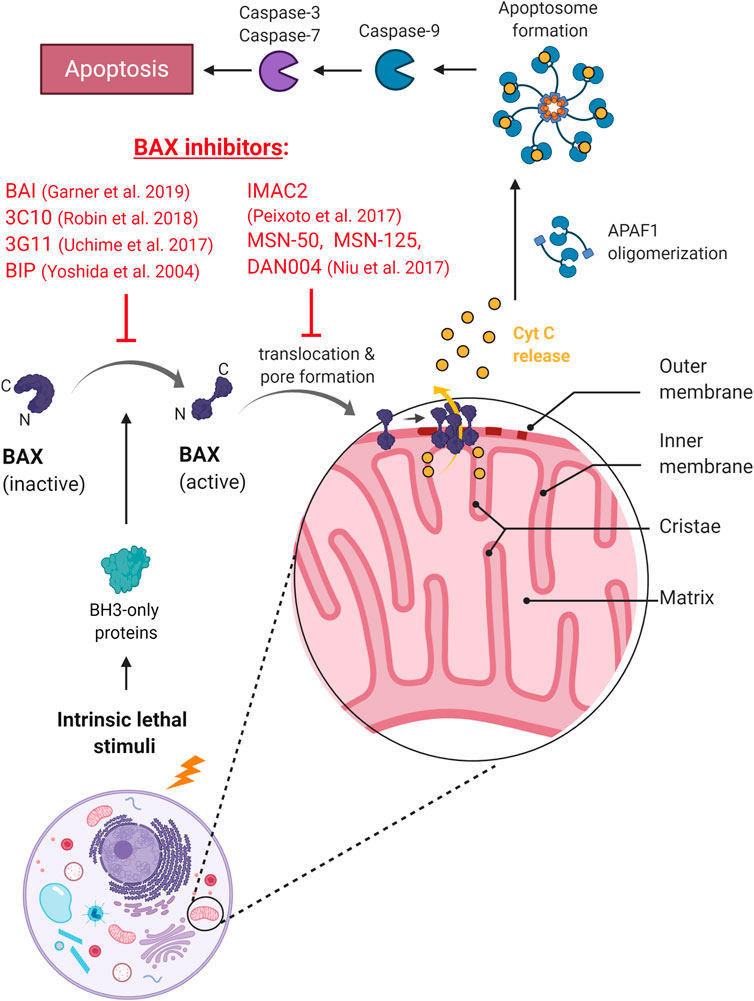
FIGURE 2. The mechanism of action of BAX inhibitors. BAXi prevents conformational change and activation of BAX, or inhibits mitochondrial translocation and pore formation, preventing the release of cytochrome C and the onset of apoptosis.
2 Conclusion
The above cardio-safe drugs (including ERK dimerization inhibitors or BAX allosteric inhibitors) are just the tip of the iceberg: superimposed on them, further research into new molecular pathways and anticancer therapeutics is needed to prevent tumorigenesis without instigating severe cardiotoxicity. BAXi data are still in the preclinical phase and ERKi human clinical trials are still preliminary. Classical ERK inhibitors have some common side effects that can be mild (e.g., diarrhea, nausea, fatigue, and rash) and severe, including neurotoxicity (CC-90003, NCT02313012) and myocardial infarction (Merchant et al., 2014; Sullivan et al., 2018; Chin et al., 2019; Weekes et al., 2020). In addition, tumors treated with ERKi often exhibit resistance to ERK inhibitors, just like upstream Ras/Raf/Mek kinases (Goetz et al., 2014; Jha et al., 2016; Jaiswal et al., 2018), which limits therapeutic efficacy. Whether the new generational ERK dimerization inhibitors (ERKdi) are better tolerable in combination with other cytostatic agents remains to be demonstrated. We also note that the ERK target GATA-4 is regulated by other post-translational modifications in myocardial cells involving e.g. p38 mitogen-activated protein kinase (p38 MAPK), small GTPase RhoA-associated coil-forming kinase (ROCK), glycogen synthase kinase-3beta (GSK-3beta) and cAMP-responsive element-binding protein binding protein (CBP) (Pikkarainen et al., 2004), which greatly increase the complexity of the system in terms of therapeutic options. This is a promising new field of cardio-oncology which is expected to improve the effectiveness of cancer treatments and help physicians avoid unexpected secondary effects related to therapeutic intervention.
Author Contributions
Conceptualization: LS, ZS manuscript writing, LS, ZS, MM, and LH; supervision, LS; funding acquisition, LS. All authors have read and agreed to the published version of the manuscript.
Funding
This work was funded by NKFIH-NNE-130913, GINOP-2.3.2-15-2016-00024, and the Thematic Excellence Programme (TKP2020-IKA-04) of the Ministry for Innovation and Technology in Hungary. LS was supported by the Bolyai Janos fellowship of the Hungarian Academy of Sciences and the UNKP-20–5-DE-47 new national excellence program of the Ministry For Innovation and Technology from the source of the National Research, Development and Innovation Fund.
Conflict of Interest
The authors declare that the research was conducted in the absence of any commercial or financial relationships that could be construed as a potential conflict of interest.
Supplementary Material
The Supplementary Material for this article can be found online at: https://www.frontiersin.org/articles/10.3389/fphar.2020.569955/full#supplementary-material.
References
Amgalan, D., Garner, T. P., Pekson, R., Jia, X. F., Yanamandala, M., Paulino, V., et al. (2020). A small-molecule allosteric inhibitor of BAX protects against doxorubicin-induced cardiomyopathy. Nat. Can. 1, 315–328. doi:10.1038/s43018-020-0039-1
Barta, K., Czifra, Á., Kun, C., Páll, A., Kulcsár, J., Paragh, G., et al. (2014). Hemodiafiltration beneficially affects QT interval duration and dispersion compared to hemodialysis. Clin. Exp. Nephrol. 18, 952–959. doi:10.1007/s10157-014-0950-9
Boros-oláh, B., Dobos, N., Hornyák, L., Szabó, Z., Karányi, Z., Halmos, G., et al. (2019). Drugging the R-loop interactome : RNA-DNA hybrid binding proteins as targets for cancer therapy. DNA Repair 84, 102642. doi:10.1016/j.dnarep.2019.102642
Cameron, S. J., and Chen, H. (2018). Cardiotoxicity of anticancer therapeutics. Front. Cardiovasc. Med. 5, 9. doi:10.3389/fcvm.2018.00009
Chin, H., Lai, D., and Falchook, G. (2019). Extracellular signal-regulated kinase (ERK) inhibitors in oncology clinical trials. J. Immunother. Precis. Oncol. 2, 10. doi:10.4103/jipo.jipo_17_18
Czifra, Á., Páll, A., Kulcsár, J., Barta, K., Kertész, A., Paragh, G., et al. (2013). Hemodialysis and hemodiafiltration differently modulate left ventricular diastolic function. BMC Nephrol. 14, 74. doi:10.1186/1471-2369-14-76
de Boer, R. A., Meijers, W. C., van der Meer, P., and van Veldhuisen, D. J. (2019). Cancer and heart disease: associations and relations. Eur. J. Heart Fail. 21, 1515–1525. doi:10.1002/ejhf.1539
Garner, T. P., Amgalan, D., Reyna, D. E., Li, S., Kitsis, R. N., and Gavathiotis, E. (2019). Small-molecule allosteric inhibitors of BAX. Nat. Chem. Biol. 15, 322–330. doi:10.1038/s41589-018-0223-0
Goetz, E. M., Ghandi, M., Treacy, D. J., Wagle, N., and Garraway, L. A. (2014). ERK mutations confer resistance to mitogen-activated protein kinase pathway inhibitors. Canc. Res. 74, 7079–7089. doi:10.1158/0008-5472.CAN-14-2073
Herrero, A., Pinto, A., Colon-Bolea, P., Casar, B., Jones, M., Agudo-Ibanez, L., et al. (2015). Small molecule inhibition of ERK dimerization prevents tumorigenesis by RAS-ERK pathway oncogenes. Canc. Cell 28, 170–182. doi:10.1016/j.ccell.2015.07.001
Herrmann, J. (2020). Adverse cardiac effects of cancer therapies: cardiotoxicity and arrhythmia. Nat. Rev. Cardiol. 17 (8), 474–502. doi:10.1038/s41569-020-0348-1
Hetey, S., Boros-Oláh, B., Kuik-rózsa, T., Li, Q., Karányi, Z., Szabó, Z., et al. (2017). Biophysical characterization of histone H3.3 K27 M point mutation. Biochem. Biophys. Res. Commun. 490, 868–875. doi:10.1016/j.bbrc.2017.06.133
Jaiswal, B. S., Durinck, S., Stawiski, E. W., Yin, J., Wang, W., Lin, E., et al. (2018). ERK mutations and amplification confer resistance to ERK-inhibitor therapy. Clin. Canc. Res. 24, 4044–4055. doi:10.1158/1078-0432.CCR-17-3674
Jha, S., Morris, E. J., Hruza, A., Mansueto, M. S., Schroeder, G. K., Arbanas, J., et al. (2016). Dissecting therapeutic resistance to ERK inhibition. Mol. Canc. Therapeut. 15, 548–559. doi:10.1158/1535-7163.MCT-15-0172
Lee, S., Rauch, J., and Kolch, W. (2020). Targeting MAPK signaling in cancer: mechanisms of drug resistance and sensitivity. Int. J. Mol. Sci. 21, 1–29. doi:10.3390/ijms21031102
Liang, Q., Wiese, R. J., Bueno, O. F., Dai, Y.-S., Markham, B. E., and Molkentin, J. D. (2001). The transcription factor GATA4 is activated by extracellular signal-regulated kinase 1- and 2-mediated phosphorylation of serine 105 in cardiomyocytes. Mol. Cell Biol. 21, 7460–7469. doi:10.1128/mcb.21.21.7460-7469.2001
Meijers, W. C., and De Boer, R. A. (2019). Common risk factors for heart failure and cancer. Cardiovasc. Res. 115, 844–853. doi:10.1093/cvr/cvz035
Meijers, Wouter. C., Maglione, M., Bakker, S. J. L., Oberhuber, R., Kieneker, L. M., Jong, S. de., et al. (2018). Heart failure stimulates tumor growth by circulating factors. Circulation 138, 678–691. doi:10.1161/CIRCULATIONAHA.117.030816
Merchant, M., Chan, J., Orr, C., Cheng, J., Wang, X., Hunsaker, T., et al. (2014). 387 Combination of the ERK inhibitor GDC-0994 with the MEK inhibitor cobimetinib significantly enhances anti-tumor activity in KRAS and BRAF mutant tumor models. Eur. J. Canc. 50, 124. doi:10.1016/s0959-8049(14)70513-1
Nissan, M. H., Rosen, N., and Solit, D. B. (2013). ERK pathway Inhibitors: how low should we go? Canc. Discov. 3, 719–721. doi:10.1158/2159-8290.CD-13-0245
Peng, L., Qian, M., Liu, Z., Tang, X., Sun, J., Jiang, Y., et al. (2020). Deacetylase-independent function of SIRT6 couples GATA4 transcription factor and epigenetic activation against cardiomyocyte apoptosis. Nucl. Acids Res. 48, 4992–5005. doi:10.1093/nar/gkaa214
Pikkarainen, S., Tokola, H., Kerkelä, R., and Ruskoaho, H. (2004). GATA transcription factors in the developing and adult heart. Cardiovasc. Res. 63, 196–207. doi:10.1016/j.cardiores.2004.03.025
Plotnikov, A., Flores, K., Maik-rachline, G., Zehorai, E., Kapri-pardes, E., Berti, D. A., et al. (2015). The nuclear translocation of ERK1/2 as an anticancer target. Nat. Commun. 6, 6685. doi:10.1038/ncomms7685
Richards, A. M. (2018). Can heart failure cause cancer? Nat. Rev. Cardiol. 16, 7–8. doi:10.1038/s41569-018-0105-x
Ryan, M. B., and Corcoran, R. B. (2018). Therapeutic strategies to target RAS-mutant cancers. Nat. Rev. Clin. Oncol. 15, 709–720. doi:10.1038/s41571-018-0105-0
Smorodinsky-Atias, K., Soudah, N., and Engelberg, D. (2020). Mutations that confer drug-resistance, oncogenicity and intrinsic activity on the ERK MAP kinases–current state of the art. Cells 9, 129. doi:10.3390/cells9010129
Sullivan, R. J., Infante, J. R., Janku, F., Lee Wong, D. J., Sosman, J. A., Keedy, V., et al. (2018). First-in-class ERK1/2 inhibitor ulixertinib (BVD-523) in patients with MAPK mutant advanced solid tumors: results of a phase I dose-escalation and expansion study. Canc. Discov. 8, 184–195. doi:10.1158/2159-8290.CD-17-1119
Szabó, Z., Ujvárosy, D., Ötvös, T., Sebestyén, V., and Nánási, P. P. (2020). Handling of ventricular fibrillation in the emergency setting. Front. Pharmacol. 10, 1–18. doi:10.3389/fphar.2019.01640
Thul, P. J., Akesson, L., Wiking, M., Mahdessian, D., Geladaki, A., Ait Blal, H., et al. (2017). A subcellular map of the human proteome. Science 80–356, aal3321. doi:10.1126/science.aal3321
Tomasovic, A. (2020). Interference with ERK-dimerization at the nucleocytosolic interface targets pathological ERK1/2 signaling without cardiotoxic side-effects. Natutre Commun 11, 1733. doi:10.1038/s41467-020-15505-4
Wallace, K. B., Sardão, V. A., and Oliveira, P. J. (2020). Mitochondrial determinants of doxorubicin- induced cardiomyopathy. Circ. Res. 126, 926–941. doi:10.1161/CIRCRESAHA.119.314681
Keywords: MAPK/ERK pathway, bax, cancer therapy, clinical trial, cardiotoxicity
Citation: Szabó Z, Hornyák L, Miskei M and Székvölgyi L (2021) Two Targets, One Hit: new Anticancer Therapeutics to Prevent Tumorigenesis Without Cardiotoxicity. Front. Pharmacol. 11:569955. doi: 10.3389/fphar.2020.569955
Received: 05 June 2020; Accepted: 08 December 2020;
Published: 10 February 2021.
Edited by:
Jonathan Satin, University of Kentucky, United StatesReviewed by:
Xingrong Du, St. Jude Children's Research Hospital, United StatesMichele Russo, University of Turin, Italy
Copyright © 2021 Szabó, Hornyák, Miskei and Székvölgyi. This is an open-access article distributed under the terms of the Creative Commons Attribution License (CC BY). The use, distribution or reproduction in other forums is permitted, provided the original author(s) and the copyright owner(s) are credited and that the original publication in this journal is cited, in accordance with accepted academic practice. No use, distribution or reproduction is permitted which does not comply with these terms.
*Correspondence: Lóránt Székvölgyi, bG9yYW50c3pAbWVkLnVuaWRlYi5odQ==