- 1Department of Pharmacology, College of Medicine and Health Sciences, UAE University, Al Ain, United Arab Emirates
- 2Department of Pharmacology, Dubai Medical College, Dubai Medical University, Dubai, United Arab Emirates
- 3MolOptima Biotech Inc., Santa Margarita, CA, United States
- 4Department of Anatomy and Cellular Biology, Khalifa University, Abu Dhabi, United Arab Emirates
- 5Department of Cellular Biology and Pharmacology, Herbert Wertheim College of Medicine, Florida International University, Miami, FL, United States
- 6Department of Biological Sciences, Schmid College of Science and Technology, Chapman University, Orange, CA, United States
- 7Department of Physiology, College of Medicine and Health Sciences, UAE University, Al Ain, United Arab Emirates
- 8Department of Pharmacology and Therapeutics, Faculty of Pharmacy, Kuwait University, Safat, Kuwait
In this study, effects of capsaicin, an active ingredient of the capsicum plant, were investigated on human 5-hydroxytryptamine type 3 (5-HT3) receptors. Capsaicin reversibly inhibited serotonin (5-HT)-induced currents recorded by two-electrode voltage clamp method in Xenopus oocytes. The inhibition was time- and concentration-dependent with an IC50 = 62 μM. The effect of capsaicin was not altered in the presence of capsazepine, and by intracellular BAPTA injections or trans-membrane potential changes. In radio-ligand binding studies, capsaicin did not change the specific binding of the 5-HT3 antagonist [3H]GR65630, indicating that it is a noncompetitive inhibitor of 5-HT3 receptor. In HEK-293 cells, capsaicin inhibited 5-HT3 receptor induced aequorin luminescence with an IC50 of 54 µM and inhibition was not reversed by increasing concentrations of 5-HT. In conclusion, the results indicate that capsaicin acts as a negative allosteric modulator of human 5-HT3 receptors.
Introduction
Capsaicin, a unique alkaloid extracted from Chili peppers of Capsicum family, is responsible for the hot pungent taste of this plant. Capsaicin together with dihydrocapsaicin constitute nearly 90% of the capsaicinoid alkaloids found in chili pepper (O’Neill et al., 2012). In recent years, therapeutic effects of capsaicin have been gaining increasing interest in various fields of medicine ranging from analgesia, anti-inflammation, and obesity to treatment of cancer (Sharma et al., 2013; Srinivasan, 2016; Patowary et al., 2017; Zhang et al., 2020).
In earlier studies, it has been well established that capsaicin causes its pain-relieving effect by activating and desensitizing the capsaicin receptor, which is known as “Transient receptor potential cation channel, subfamily V, member 1” (TRPV1). TRPV1 is a non-selective, Ca2+ permeable cation channel activated by protons, noxious heat, endogenous lipids, and exogenous ligands, such as resiniferatoxin and capsaicin (Lumpkin and Caterina, 2007; O’Neill et al., 2012). Although, the activation of TRPV1 is considered to be an important mechanism, the exact nature of the widely ranging biological actions of capsaicin is currently unknown.
The serotonin type three (5-HT3) receptor is a member of the cys-loop family of ligand-gated ion channels. Fast depolarizing synaptic actions of 5-HT are mediated by 5-HT3 receptors in the central and peripheral nervous systems (Thompson and Lummis, 2006). Currently, 5-HT3 receptor antagonists are used in clinics for the treatment of chemotherapy-induced nausea, vomiting, and irritable bowel syndrome (Thompson and Lummis, 2007; Binienda et al., 2018). In recent years, there has been renewed interest in exploring the therapeutical potential of 5-HT3 receptor modulators in various neuropsychiatric disorders such as schizophrenia, depression, anxiety, and drug abuse (Fakhfouri et al., 2019; Juza et al., 2020). In the present study, using electrophysiological and biochemical methods, we have investigated the effect of capsaicin on the functional properties of human 5-HT3 receptors expressed in Xenopus oocytes and HEK-219 cells.
Materials and Methods
Mature female Xenopus laevis frogs were obtained from Xenopus leavis I, Ann Arbor, MI, USA. Experiments and methods used in this study were in accordance with the Guide for the Care and Use of Laboratory Animals of the National Institutes of Health (Bethesda, MD, USA) and our protocol (A9/08) was approved by the Institutional Animal Care and Use Committee at the College of Medicine and Health Sciences, United Arab Emirates University. Clusters of oocytes were removed surgically under benzocaine (0.03% w/v; Sigma, St.Louis, MO) anesthesia. Individually dissected oocytes were stored for 2 to 8 days in modified Barth’s solution (MBS) containing (in mM): NaCl 88; KCl 1; NaHCO3 2.4; CaCl2; 2; MgSO4 0.8; HEPES 10 (pH 7.5), supplemented with sodium pyruvate 2 mM, penicillin 10,000 IU/L, streptomycin 10 mg/L, gentamicin 50 mg/L, and theophylline 0.5 mM. Human 5-HT3A receptor cRNA (3 ng in 50 nl) was injected into each oocyte as described before (Ashoor et al., 2013). In co-expression of subunit combinations, cDNAs for 5-HT3A and 5-HT3B subunits, were mixed in ratios of 1:1 (or 1:2), respectively. Following day, oocytes were placed in a 0.2 ml recording chamber and superfused at a constant rate of 3 to 5 ml/min. The bathing solution consisted of: 95 mM NaCl; 2 mM KCl; 2 mM CaCl2; and 5 mM HEPES 5 (pH 7.5). The oocytes were impaled with two standard glass microelectrodes filled with a 3 M KCl (1–3 MΩ) and voltage clamped at a holding potential of −70 mV using GeneClamp-500B amplifier (Axon Instruments Inc., Burlingame, CA). Current responses were digitized by A/D converter and analyzed using pClamp 10.4 (Molecular Devices-Axon Instruments, San Jose, CA USA) or Origin™ (Originlab Corp. Northampton, MA, USA), run on an IBM/PC. Compounds were applied by addition to the superfusate. Capsaicin ((E)-N-[(4-Hydroxy-3-methoxyphenyl)methyl]-8-methyl-6-nonenamide, ≥98%; Cat. No. 0462), capsazepine (≥99%; Cat. No. 0464), BAPTA (≥95%; Cat. No. 2786/100), 5-HT, 2-methyl-5HT, and MDL72222 (Tropanyl 3, 5-dichlorobenzoate; ≥99; Cat. No. 0640) were purchased from Tocris Cookson (St. Louis, MO). Dihydrocapsaicin (98%; Cat. No. 03813), vanillin (99% Cat. No. V1104), and all chemicals used in preparing the solutions were provided by Sigma-Aldrich (St. Louis, MO, USA). Procedures for the injections of BAPTA (50 nl, 100 mM) were performed as described previously (Oz et al., 1998). Injections were performed 10 min prior to recordings using oil-driven ultra microsyringe pumps (Micro4; WPI, Inc. Sarasota, FL, USA). Stock solutions of capsaicin were prepared in DMSO. Vehicle (DMSO) alone did not affect 5-HT3 receptor function when added at concentrations as high as 0.3% (v/v), a concentration twice above the most concentrated application of the agents used.
Synthesis of cRNA
The cDNA clones of the human 5-HT3A and 5-HT3B subunits were provided by OriGen Inc. (Rockville, MD). Complementary RNAs (cRNAs) were synthesized in vitro using a mMessage mMachine RNA transcription kit (Ambion Inc., Austin, TX). The quality and size of synthesized cRNAs were confirmed by denatured RNA agarose gels.
Radioligand Binding Studies
Oocytes were injected with 10 ng human 5-HT3 cRNA, and functional expression of the receptors was assessed by electrophysiology on day three. Isolation of oocyte membranes was carried out by modification of a method described earlier (Oz et al., 2004). Briefly, oocytes were suspended (20 μl/oocyte) in a homogenization buffer (HB) containing HEPES 10 mM, EDTA 1 mM, 0.02% NaN3, 50 μg/mL bacitracin, and 0.1 mM PMSF (pH 7.4) at 4°C on ice and homogenized using a motorized Teflon homogenizer (six strokes, 15 s each at high speed). The homogenate was centrifuged for 10 min at 800 g. The supernatant was collected, and the pellet was resuspended in HB and re-centrifuged at 800g for 10 min. Supernatants were then combined and centrifuged for 1 h at 36,000g. The membrane pellet was resuspended in HB at the final protein concentration of 0.5 to 0.7 mg/ml and used for the binding studies.
Binding assays were performed in 500 μl of 10 mM HEPES (pH 7.4) containing 50 μl of oocyte preparation and 1 nM [3H]GR65630 (Perkin-Elmer, Inc. Waltham, MA, USA). Nonspecific binding was determined using 100 μM MDL72222. Oocyte membranes were incubated with [3H]GR65630 in the absence and presence of capsaicin at 4°C for 1 h before the bound radioligand was separated by rapid filtration onto GF/B filters pre-soaked in 0.3% polyethylenemine. Filters were then washed with two 5-mL washes of ice-cold HEPES buffer and left in 3 mL of scintillation fluid for at least 4 h before scintillation counting was conducted to determine amounts of membrane-bound radioligand.
Aequorin Luminescence Assay
Luminescence experiments were performed according to methods and protocols described earlier (Walstab et al., 2007), with some modifications. Human embryonic kidney (HEK 293) cells stably expressing apoaequorin (HEK293-AEQ17 cells; Button and Brownstein, 1993) were cultured as described previously for HEK-293/EM4 cells (Oz et al., 2010). Cells were seeded in 25-cm2 cell culture flasks in Dulbecco’s modified Eagle’s medium (DMEM)/Ham’s F12 (1:1) + 10% fetal bovine serum to obtain a cell density of 50% to 70%, and the following day, transiently transfected with cDNA encoding human 5-HT3A receptor (3 µg) using Lipofectamine 2000 reagent (Thermo Fisher Scientific-Invitrogen, Waltham, MA) according to the manufacturer’s instructions. Two days after transfection, cells were harvested by centrifugation and resuspended in 0.5 ml (25-cm2 flask) DMEM/Ham’s F12 (1:1) + 0.1% bovine serum albumin. The cell suspension was incubated with 10 μM Coelenterazine h (Thermo Fisher Scientific-Invitrogen, Waltham, MA, USA) for three hours at room temperature in the dark. After loading, cells were harvested by centrifugation and resuspended in assay buffer containing 150 mM NaCl, 1.8 mM CaCl2, 5.4 mM KCl, 10 mM Hepes, and 20 mM D-glucose at pH 7.4 at the approximate cell density of 3 to 5 × 106 cells/ml. Cell suspension (60 μl) was preincubated with 20 µl capsaicin in a 96-well plate for 10 min. at room temperature and activated by 20 μl of 10 μM 5-HT injection. Luminescence was measured using a Luminoskan (Thermo Fisher Scientific, Waltham, MA, USA) equipped with an injector and recorded at a sampling rate of 2 Hz for up to 60 s. At the end of the experiments, cells were lysed with Triton X-100 0.1% (v/v) and CaCl2 50 mM, and aequorin luminescence was recorded to obtain the maximum Ca2+ response. Each capsaicin concentration was measured in quadruplicates in two experiments. Data were exported to software Origin™ 8.5 (Originlab Corp. Northampton, MA, USA). Peak values in relative light unit (RLU) for 5-HT responses were obtained by subtraction of baseline luminescence from the agonist-induced peak luminescence and normalizing to maximal Ca2+ response.
Data Analysis
For the nonlinear curve fitting and regression fits of the dose-response curves and radio-ligand binding data, the computer software Origin™ 8.5 (Originlab Corp. Northampton, MA, USA) was used. In functional assays, average values were calculated as mean ± standard error means (S.E.M.). Statistical significance was analyzed using ANOVA or Student’s t test and post hoc Bonferroni test was used following ANOVA. Concentration-response curves were obtained by fitting the data to the logistic equation,
where x and y are concentration and response, respectively, Emax is the maximal response, Emin is the minimal response, EC50 is the half-maximal concentration, and n is the slope factor.
Docking Studies
Docking calculations were performed on 5HT3 receptor (Protein Data Bank ID code 4PIR (Hassaine et al., 2014)). Docking of compounds capsaicin, dihydrocapsaicin, vanillin, and capsazepine to structural model was made by Autodock Vina program (Trott and Olson, 2010), results were verified using Gold docking software, which is part of CSD Discovery suite from Cambridge Crystallographic Data Center (Groom et al., 2016). Ligand files were downloaded from PubChem structural database (Kim et al., 2018). Ligand and receptor files were prepared using m Autodock Tools (ADT) (Morris et al., 2009). Polar hydrogens, united atoms Kollman charges and solvation parameters were identified, files were saved in PDBQT format. Affinity grid maps of 30 Å × 30 Å × 30 Å with spacing 0.375 Å were added. Grid center was designated x, y, z dimensions: 139.00, 219.00 and 273.00. These coordinates correspond to allosteric binding site of 5HT3 receptor for ginger compounds identified in an earlier study (Lohning et al., 2016) on human 5HT3 receptor. Docking calculations were performed using the Lamarckian genetic algorithm (LGA) (Morris et al., 1998). During the docking procedure, both the protein and ligands were considered as rigid. The poses with lowest binding free energy were aligned with receptor for further analysis of interactions. Binding poses were verified by Gold docking program. In GOLD docking was prepared using the Hermes program and wizard for docking with default parameters such as population size (100); selection- pressure (1.1); number of operations (10,000); number of islands (1); niche size (2); and operator weights for migrate (0), mutate (100), and crossover (100). The active site with a 10 Å radius sphere was defined by selecting an active site residue of protein. Default Genetic Algorithm settings were used for all calculations and a set of 10 solutions were saved for each ligand. GOLD was used by a GoldScore fitness function.
Results
In initial experiments, fast inward currents activated by 5-HT (1 μM) or 2-methyl-5-HT (10 μM) were completely inhibited by 0.5 µM MDL72222, a specific 5-HT3 receptor antagonist, indicating that functional 5-HT3 receptors are expressed in Xenopus oocytes (n = 7). Capsaicin (100 µM for 1 min) alone did not induce current responses in oocytes expressing 5-HT3 receptors in the absence (n = 5) and presence of 0.5 µM MDL72222 (n = 5).
Figure 1A shows the recordings of currents activated by 5-HT (1 μM) in control (on the left), after 10 min capsaicin (100 µM) application (in the middle), and after 20 min of washout (on the right). Figure 1B presents the time course of the capsaicin effect on the maximal amplitudes of currents (n = 6–8 oocytes). Amplitudes of currents remained unchanged and stable during the course of experiments in the presence of vehicle (0.3% v/v DMSO; n = 5). However, current amplitudes decreased gradually during the application of 100 µM capsaicin and completely recovered after 15 to 20 min of washout period (Figure 1B). Inhibition of 5-HT3 receptor-induced currents by capsaicin was concentration-dependent with an IC50 of 62 ± 5 µM and a slope of 1.4 (Figure 1C).
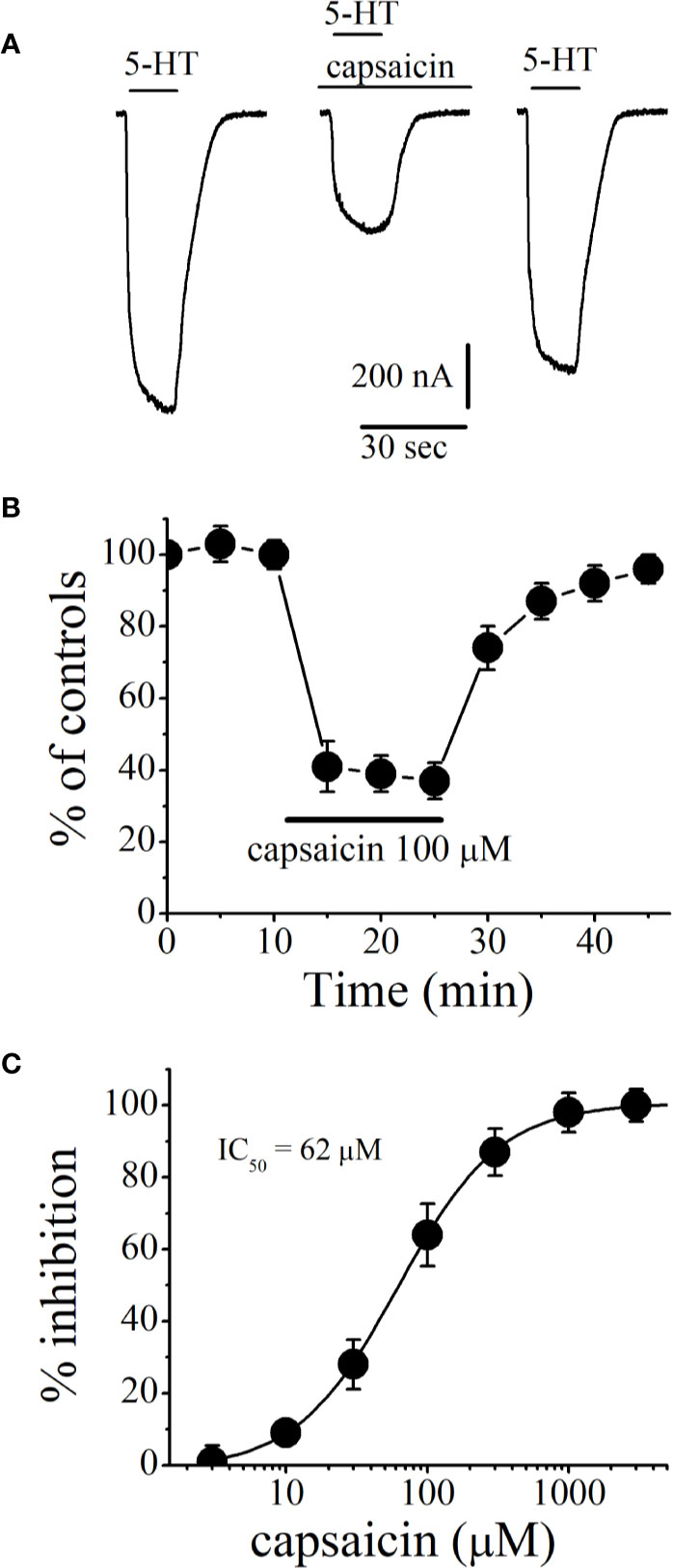
Figure 1 Effects of capsaicin on the function of human 5-HT3 receptors expressed in Xenopus oocytes. (A) Representative traces of currents activated by 5-HT (1 µM; on the left), coapplication of 5-HT and 100 µM capsaicin after 10 min capsaicin pre-application (middle), 20 min wash-out (right). (B) Effect of capsaicin application on the normalized amplitudes of currents activated by 5-HT (1 µM) at 5 min intervals. Current amplitudes were normalized to first agonist application in each experiment. Solid bar represents application time for capsaicin (100 µM). Data points represent means ± S.E.M. of 7–8 cells. (C) Capsaicin inhibits the function of 5-HT3 receptor in concentration-dependent manner. For all concentrations used, capsaicin was applied for 10 min. Data points represent mean ± S.E.M. (n = 6–8).
An open-channel blocker would access its binding site during the channel opening time and the extent of drug inhibition would be independent of its pre-incubation time. However, close examination of the time course of capsaicin inhibition showed fast and slow phases with the respective time constants of τ1/2fast = 6 s. and τ1/2slow = 0.8 min, arguing against open channel blockade (Figure 2A). Without preincubation, co-application of capsaicin (100 µM) and 5-HT (1 µM) induced a 46 ± 5% inhibition of controls (n = 4). TRPV1 receptors are endogenously expressed and activated by capsaicin in Xenopus tropicalis frogs (Ohkita et al., 2012). We have tested the effect of capsazepine (10 µM), a competitive antagonist of TRPV1 receptors (Figure 2B) on capsaicin inhibition of 5-HT3 receptors. The extent of capsaicin inhibition is not altered in the presence of capsazepine (ANOVA, n = 5–7, P>0.05). Capsazepine (10 µM) alone did not cause any significant change in the amplitudes of 5-HT3 receptor-mediated currents (ANOVA, n = 4, P>0.05). Capsaicin has been shown to release Ca2+ and interact directly with second messenger pathways (Savitha et al., 1990; Kim et al., 2005; Xu et al., 2012; Chien et al., 2013; Kida et al., 2018). Considering the time course of capsaicin effect, it was possible that capsaicin acts by modulating the effects Ca2+ activated kinases on 5-HT3 receptor (Zhang et al., 1995; Jones and Yakel, 2003; Hu et al., 2004). Therefore, we tested the effect of the Ca2+ chelator BAPTA on capsaicin inhibition of 5-HT3 receptors (Figure 2C). In oocytes injected with BAPTA, extent of capsaicin (100 µM) inhibition was not significantly different from controls (controls injected with 50 nl distilled water, ANOVA, n = 5–6, P > 0.05), indicating that the effect of capsaicin is not mediated by changes in intracellular Ca2+ levels.
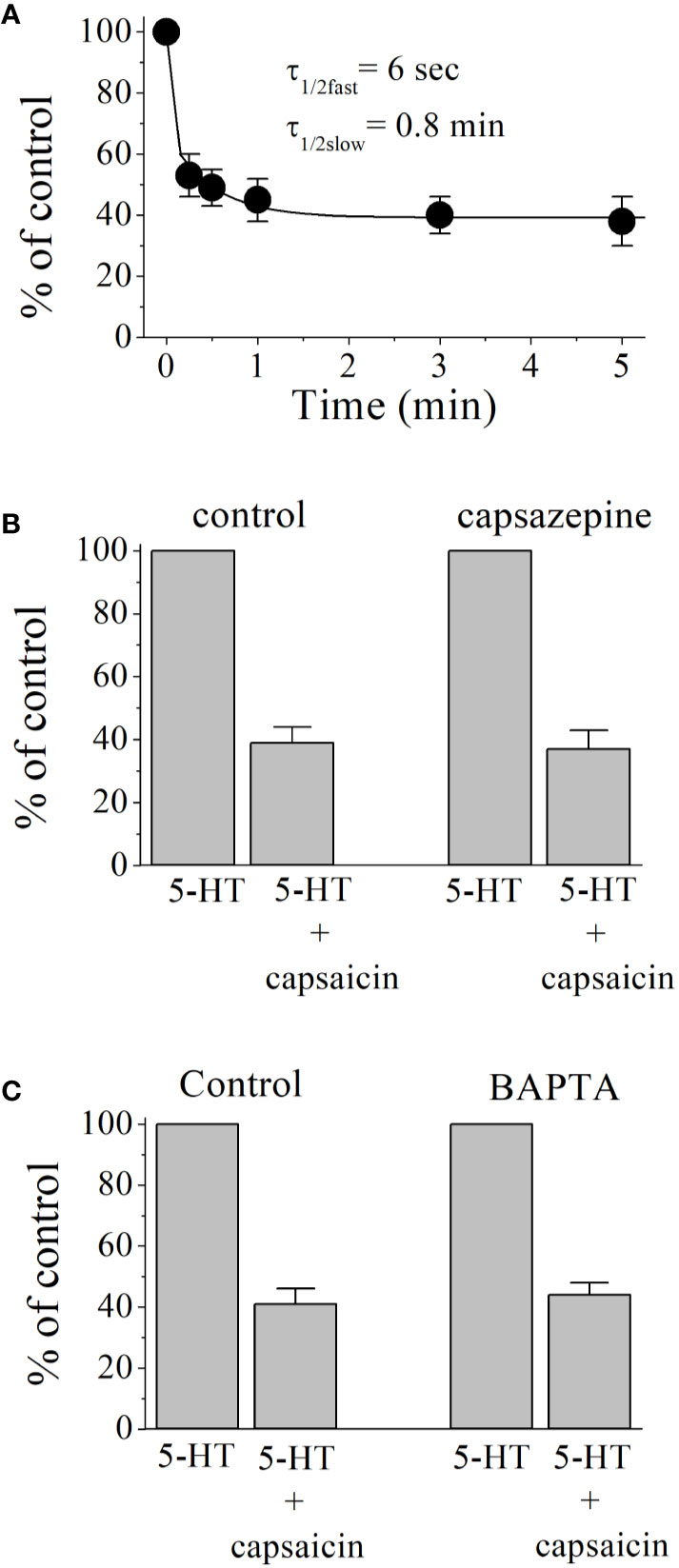
Figure 2 Inhibitory effect of capsaicin on 5-HT3 receptor increases with pre-application times and independent of TRPV1 receptors and intracellular Ca2+ levels. (A) Capsaicin inhibition of 5-HT3 receptor as a function of pre-incubation time. Exponential decay curve with two time constants τfast and τslow, shows the best fit for data point in the figure. Each data point represents the means ± SEM from 7 to 8 oocytes. (B) Effects of capsazepine (10 µM) on 5-HT (1 µM) induced currents (n = 5–7). Bars represent the means ± S.E.M. (C) Effect of BAPTA injection on the capsaicin inhibition of 5-HT-induced currents. 5-HT (1 μM)-induced currents were recorded before and after 10 min capsaicin (100 μM) application in oocytes injected with 50 nl distilled-water (controls, n = 5) or 50 nl of BAPTA (200 mM, n = 6). Bars represent the means ± S.E.M.
Earlier electrophysiological studies reported that capsaicin inhibits the function of voltage-gated Na+ channels (Lundbaek et al., 2005; Wang et al., 2007) and K+ channels (Kuenzi and Dale, 1996), and Ca2+ channels (Hagenacker et al., 2005) in a voltage-dependent manner. We plotted the current-voltage (I-V) relationships of 5-HT3 receptor-induced currents before and after 15 min capsaicin (100 µM) application (Figure 3A). Extent of capsaicin inhibition was not altered by changing membrane potentials (Figure 3B). Subunit combination of 5-HT3 receptors has been shown to alter effects of various drugs (Thompson and Lummis, 2007; Barnes et al., 2009). We compared the effect of capsaicin (100 μM) between 5-HT3A and 5-HT3AB subunits. Results indicated that the extent of capsaicin inhibition was not statistically different among 5-HT3A, 5-HT3AB (injected with cRNA ratio of 5-HT3A and 5-HT3B subunits, respectively), and 5-HT3AB (ratio of 1:2) receptors (n = 5–7, ANOVA, P>0.05; Figure 3C).
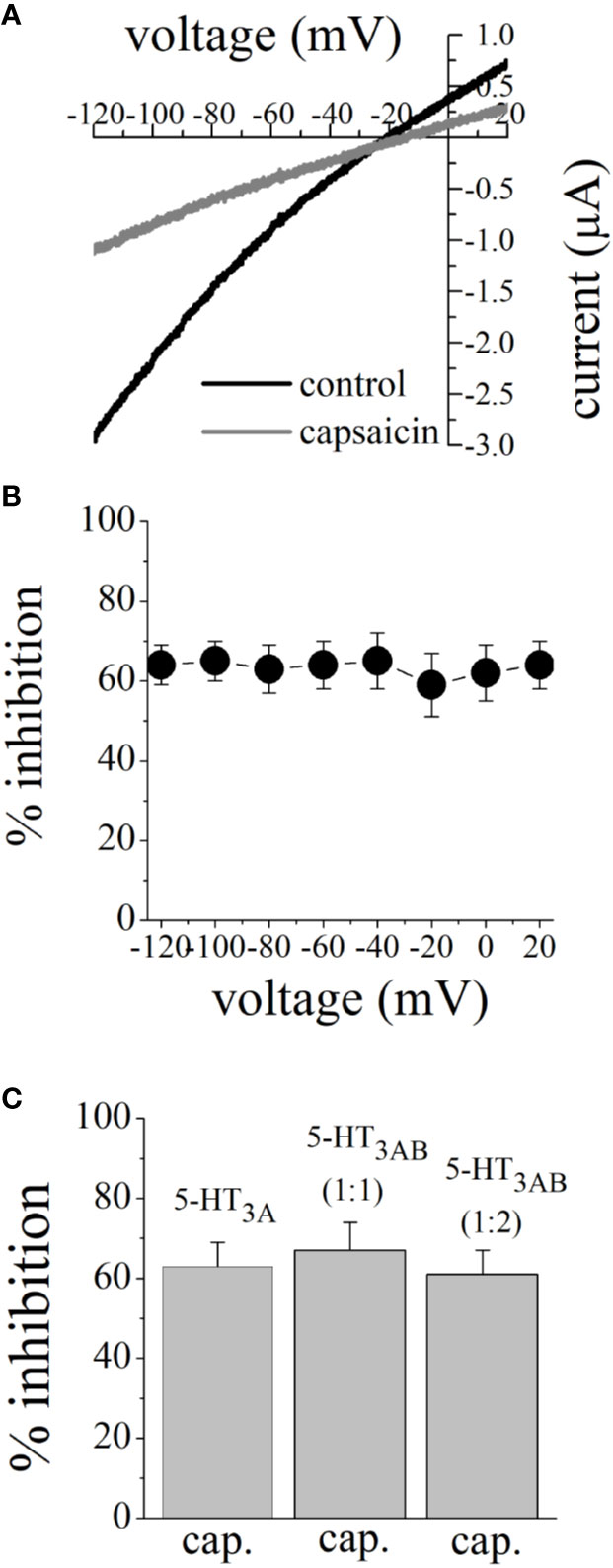
Figure 3 Effects of membrane potential and subunit combination on capsaicin inhibition of 5-HT-activated currents. (A) Current-voltage relationships of 5-HT (1 μM)-activated currents before and after 10 min pre-application of 100 µM capsaicin. Data points are the means ± SEM (n = 5) measured from 2-s voltage ramps. (B) Inhibition of 5-HT-activated current by 100 µM capsaicin at different membrane potentials. Capsaicin inhibition of 5-HT-activated currents did not change significantly at different membrane potentials (P>0.05, ANOVA; n = 5). (C) The effect of 100 μM capsaicin on human 5-HT3A, 5-HT3A and 5-HT3B receptors co-expressed in subunit ratios 1:1 and 1:2. Currents were activated by 3 µM and 30 µM 5-HT for 5-HT3A and 5-HT3AB receptor combinations, respectively. The bar graph shows mean ± SEM from 5 to 7 oocytes.
Capsaicin may inhibit 5-HT3 receptor by competing with the binding of 5-HT to the receptor. For this reason, we examined 5-HT concentration-responses in the absence and presence of 100 µM capsaicin (Figure 4A). Capsaicin inhibited maximal 5-HT responses without causing a significant change in EC50 values (in the absence and presence of capsaicin were 1.4 ± 0.3 and 1.9. ± 0.4 μM, respectively; n = 6–8), suggesting noncompetitive inhibition. In radioligand binding experiments, specific binding of [3H]GR65630 was inhibited by increasing concentrations of 5-HT in oocyte membranes containing 5-HT3 receptor (Figure 4B). The IC50 values for 5-HT inhibition of [3H]GR65630 binding were not significantly altered by 100 μM capsaicin (in the absence and presence of capsaicin were 591 ± 154 and 612 ± 141 nM, respectively; ANOVA, n = 8–11; P>0.05). Similarly, increasing capsaicin concentrations did not change the specific binding of [3H]GR65630 (Figure 4C).
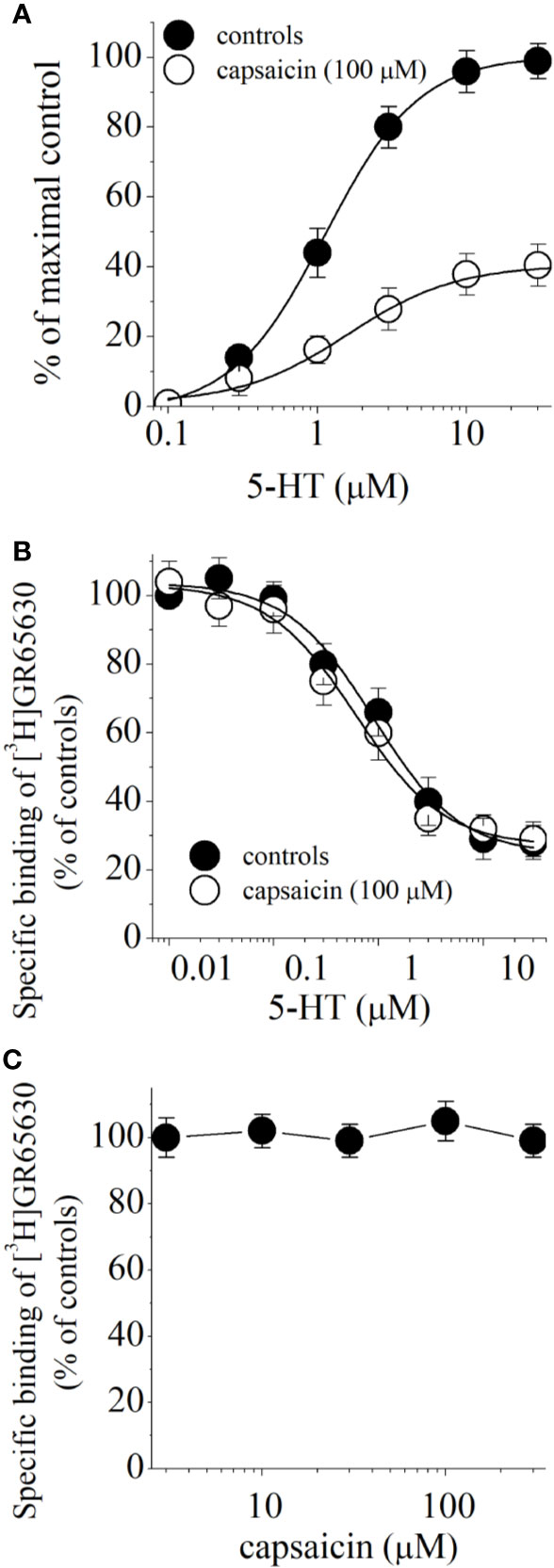
Figure 4 Effect of capsaicin on 5-HT concentration-response relationship and binding of [3H]GR65630 to 5-HT3 receptor expressed in Xenopus oocytes. (A) Concentration-response curves for 5-HT-activated currents in the absence and presence of 100 µM capsaicin. Data points represent the mean ± S.E.M. (n = 6–8). The curves depict the best fit of the data to the logistic equation described in the methods. The concentration-response for capsaicin is normalized to maximal control response. (B) Effects of capsaicin on the displacement of specific [3H]GR65630 binding by nonlabeled 5-HT in oocyte membranes. Membrane preparations were pre-incubated 100 μM capsaicin for 1 hour. The concentration of [3H]GR65630 was 1 nM. Data points indicate means ± SEM from 8 to 11 measurements from 3 experiments. (C) Effects of increasing concentrations of capsaicin on the specific binding of [3H]GR65630 (1 nM). Data points indicate means ± S.E.M from 7 to 10 measurements.
We also investigated whether the vanillyl group in capsaicin is involved in the inhibition of 5-HT3 receptors. Application of vanillin (100 µM, for 15 min), which has only a vanillyl group, did not affect the 5-HT3 receptor (ANOVA, n = 8, P>0.05). In contrast, the application of 100 µM dihydrocapsaicin, which contains a vanillyl residue and an acyl chain, inhibited 5-HT3 receptors to 62% ± 6% (ANOVA, n = 6–9, P<0.05) suggesting that the inhibition of 5-HT3 receptors requires the acyl chain, which causes the compound to be lipophilic.
In HEK-293-AEQ17 cells transfected with human 5-HT3 receptor, application of 5-HT induced concentration-dependent increases in aequorin luminescence with an EC50 value of 2.3 μM and slope of 2.7 (n = 4–5 for each concentration point). Aequorin response to 5-HT (10 μM) was completely inhibited by 0.5 μM granisetron (n = 4). In coelenterazine h-loaded HEK-293-AEQ17 cells not transfected with 5-HT3, injection of 30 μM 5-HT did not cause luminescence activation (n = 4). Application of capsaicin (100 μM) alone did not cause a significant change in baseline aequorin luminescence (n = 4). Figure 5A shows the capsaicin inhibition of 5-HT3 receptor mediated aequorin responses. Capsaicin inhibited in concentration-dependent manner with an IC50 value of 54 μM. Figure 5B represents the extent of capsaicin (50 μM) inhibition on aequorin luminescence induced by 3, 10, and 30 μM 5-HT in HEK-293-AEQ cells transfected with human 5-HT3 receptor. There was no statistically significant difference in the extent of capsaicin inhibition at increasing 5-HT concentrations (ANOVA, n = 8–11; P>0.05).
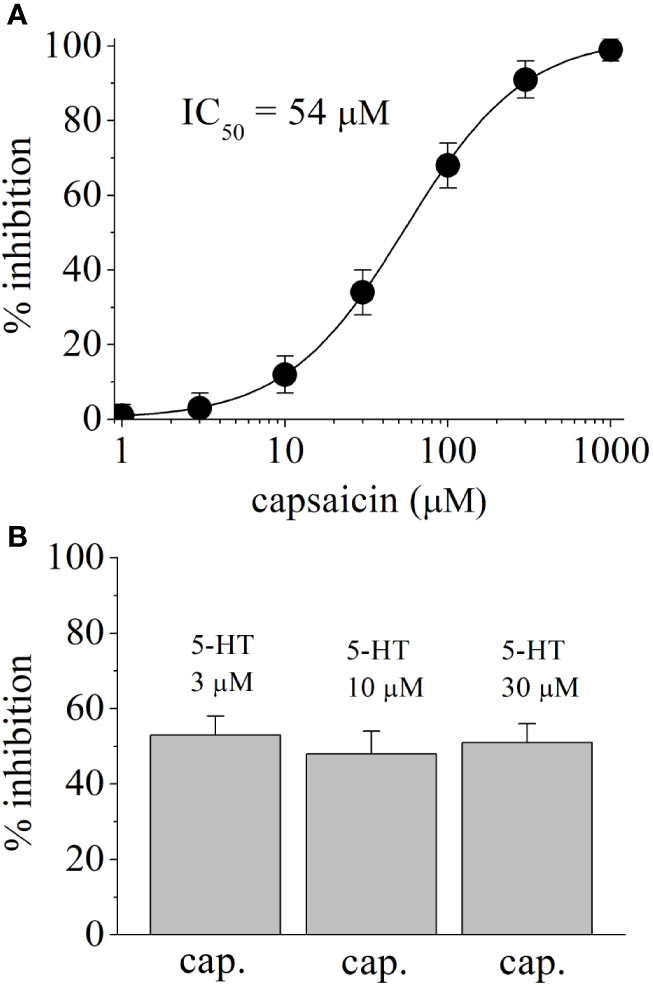
Figure 5 Effects of capsaicin on 5-HT-induced Ca2+ influx through human 5-HT3 receptors. (A) Concentration-dependent inhibition of 5-HT3 receptors by capsaicin. Aequorin luminescence induced by 3 μM 5-HT was recorded as a measure of an increased cytosolic Ca2+ concentration in coelenterazine h-loaded HEK-293-AEQ17 cells heterologously expressing human 5-HT3 receptors. Capsaicin was present 10 min before and during 5-HT application. Data are expressed as percentages of the response to 5-HT in the absence of capsaicin (means ± SEM; n = 5). (B) The effects of capsaicin (50 µM) on aequorin luminescence activated by 3 μM, 10 µM, and 30 μM 5-HT. Bars represent means ± SEM; n = 16.
The results of docking calculations are presented in Figure 6. All binding poses of capsazepine are located at the interface between transmembrane domain (TMD) and extra cellular domain. This binding site is situated in similar position with allosteric binding site predicted by (Lohning et al., 2016). Free energy of binding predicted by Autodock Vina for most favorable docking pose is −7.8 kcal/mol. Gold has predicted similar binding poses for capsazepine. Inside of the capsazepine binding pocket Gln56 and Pro274 form hydrogen bonds with the hydroxyl on the benzazepine group of capsazepine, while Gln184 makes hydrogen bond with amide group of the capsazepine. Phe222 as well as backbone part of the Glu53 and Lys54 interact with the benzazepine moiety of the capsazepine.
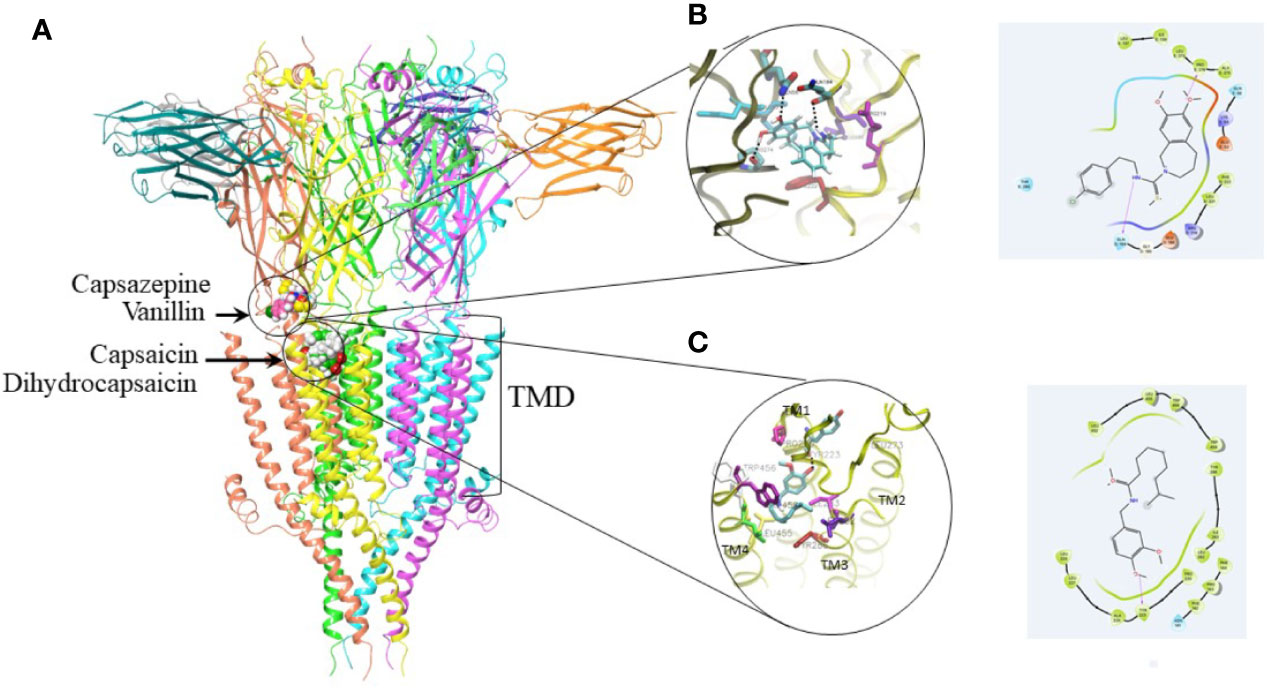
Figure 6 Docking studies on capsaicin, dihydrocapsaicin, capsazepine, and vanillin. (A) 5HT3 receptor with best ranking poses of the docked capsaicin, dihydrocapsaicin, capsazepine, and vanillin. Proposed binding site for capsazepine and vanillin is located at interface with transmembrane domain (TMD). Proposed binding site for capsaicin and dihydrocapsaicin is located in the TMD. (B) 3D and 2D binding interactions within capsazepine binding pocket showing potential key residues. Residues that form hydrogen bond with capsazepine are shown in CPK color. (C) 3D and 2D binding interactions within capsaicin binding pocket showing potential key residues. Residue that forms hydrogen bond with capsaicin is shown in CPK color.
The preferable positions of capsaicin and dihydrocapsaicin are located in upper part of the TMD between TM4, TM3 and TM1. Free energy of binding predicted by Vina for best ranking pose of capsaicin is −7.8 kcal/mol. Dihydrocapsaicin has comparable binding free energies. Gold docking program has predicted similar binding poses. Capsaicin alkyl chain makes hydrophobic interaction with the amino acids residues located on TM3 helix such as Ile283, Leu282 as well as with the backbone of Tyr286. This alkyl chain also makes hydrophobic contact with the amino acids residues located on TM4 helix such as Trp454, Trp456, and Leu455. Methoxyphenyl group of capsaicin makes hydrogen bond with the backbone of the Tyr223 located on the TM1 helix. The interaction of the capsaicin that includes three helices belonging to the one subunit of the TMD may interfere with the pore opening that includes rearrangement of transmembrane helices. It can explain experimental funding presented in this paper that capsaicin works as effective negative allosteric modulator. The results of the docking calculations for vanillin show that it can bind to both binding pockets with similar predicted binding free energy −4.8 kcal/mol. In the transmembrane binding pocket, vanillin is situated between TM4 and TM3 helices. Aldehyde group of vanillin interacts with the Tyr286 on TM3 helix, hydroxyl group makes hydrogen bond with Asn141. Phenol makes hydrophobic contact with Trp459 on TM4.
Discussion
Results indicate that capsaicin inhibits the function of human 5-HT3 receptors. Inhibition by capsaicin is time and concentration dependent with IC50 values of 62 and 54 µM in Xenopus oocytes and HEK-293 cells, respectively. The results of functional and radio-ligand binding studies indicate that capsaicin does not share the same binding site with 5-HT and act as a negative allosteric modulator of 5-HT3 receptor.
Capsaicin has been shown to release Ca2+ from intracellular stores, modulate store-operated Ca2+ channels, and interact with various Ca2+ sensitive kinases in a TRPV1 receptor-independent manner (Savitha et al., 1990; Kim et al., 2005; Xu et al., 2012; Chien et al., 2013; Kida et al., 2018). Considering the time-course of capsaicin effect, it was possible that Ca2+ activated kinases may be involved. However, capsaicin inhibition of 5-HT3 receptor remained unaltered in oocytes injected with BAPTA. Furthermore, capsaicin alone did not cause changes in holding current, which is moderately sensitive to Ca2+ due to the presence of Ca2+-activated Cl− channels in Xenopus oocytes (Dascal, 1987). Similarly, application of capsaicin alone did not activate aequorin luminescence in HEK-293 cells, suggesting that intracellular Ca2+ is not involved in observed effects of capsaicin.
Capsaicin in the concentration ranges used in this study has been shown to act directly on various ion channels in a TRPV1 independent manner. Capsaicin directly modulates the functions of voltage-gated Na+ channels (Bielefeldt, 2000, IC50 = 40 µM; Duan et al., 2007, IC50 = 39 µM; Wang et al., 2007, IC50 = 76 µM; Tomohiro et al., 2013, IC50 = 100 µM), K+ channels (Grissmer et al., 1994, IC50 = 158 µM; Kuenzi and Dale, 1996, IC50 = 21 µM; Wu et al., 2011, IC50 = 103 µM; Aréchiga-Figueroa et al., 2017, IC50 = 10 µM), and Ca2+ channels (Kuenzi and Dale, 1996, IC50 = 44 µM; Castillo et al., 2007, IC50 = 38 µM). In the present study, capsaicin inhibited 5-HT3 receptors with IC50 values of 54 and 62 µM which are comparable to values obtained in other studies on the direct effects of capsaicin.
In various dermatological disorders, topical application of capsaicin has been widely used for analgesia and shown to provide adequate absorption from the skin and good bioavailability (Rollyson et al., 2014). In topically applied preparations, the concentration of capsaicin ranges between 3 and 260 mM (0.1–8%) (Bley, 2013). Assuming that 2% of topically applied capsaicin is absorbed into the skin (Lee et al., 1997; Wohlrab et al., 2015), it is likely that the concentration of capsaicin in the dermis ranges between 60 and 5.2 mM for 0.1% and 8% cutaneous applications, respectively. Importantly, membrane concentration of capsaicin is expected to be greatly higher than that in extracellular compartments due to its high lipophilic structure with a LogP (octanol–water partition coefficient) value of 3.8 (Rollyson et al., 2014; Swain and Kumar Mishra, 2015). Following subcutaneous or intravenous administration in animals, the concentrations of capsaicin in the brain and spinal cord were approximately 5-fold higher than that in blood (O’Neill et al., 2012). Thus, modulation of 5-HT3 receptors demonstrated in this study may be of pharmacological relevance.
In electrophysiological studies, capsaicin inhibited the maximum 5-HT responses without altering EC50 of the 5-HT, indicating that capsaicin does not compete with the 5-HT binding site of the receptor. In addition, in radio-ligand binding studies, binding of competitive 5-HT3 receptor antagonist [3H]GR65630 was not significantly affected by capsaicin, further suggesting that capsaicin does not interact with the 5-HT binding site. Furthermore, aequorin luminescence studies in HEK-293-AEQ17 cells indicated that the extent of capsaicin inhibition of aequorin responses was not changed significantly by increasing 5-HT concentrations. Thus, the results of electrophysiological, luminescence, and radioligand binding experiments indicate that capsaicin acts as an allosteric inhibitor of 5-HT3 receptor. Importantly, in a recent in silico docking study, a high scoring allosteric and hydrophobic capsaicin binding site located at the interface between the extracellular and transmembrane domain of 5-HT3A receptor subunit has been identified (Lohning et al., 2016). Our results are also in agreement with an earlier study investigating the effects of more than 200 odorous compounds, terpenes, alcohols, and pungent substances (Ziemba et al., 2015), reporting that various gingerol derivatives, capsaicin and polygodial, inhibit 5-HT3 receptors. Furthermore, our results indicated that dihydrocapsaicin, but not vanillin, inhibited 5-HT3 receptor, suggesting that the lipophilicity is an important property for capsaicin effect on this receptor.
As a highly lipophilic agent, capsaicin has been shown to alter physicochemical properties of cell membranes, perturb the bilayer structure, and inhibit the functions of various ion channels (Lundbaek et al., 2005; Lundbaek et al., 2010; Ingólfsson et al., 2014). Thus, it is likely that capsaicin first dissolves into the lipid membrane, changes the physicochemical properties of the cell membrane and, subsequently or simultaneously, diffuses and reaches to binding site(s) located on the transmembrane domains of the 5-HT3 receptor. Consistent with this assumption, direct effects of capsaicin on several ion channels including the 5-HT3 receptor usually require several minutes to reach steady-state maximal levels. Similarly, several minutes of application times (5–15 min) are prerequisite for actions of several lipophilic and allosteric modulators such as steroids, endocannabinoids, and cannabinoids (Oz et al., 2002a; Oz et al., 2002b; Yang et al., 2010a; Yang et al., 2010b) on 5-HT3 receptors (for reviews, Oz, 2006; Oz et al., 2015; Al Kury et al., 2018), suggesting that the binding site(s) for these allosteric modulators is located inside the lipid membrane. Notably, these results also indicate that drug exposure time rather than channel opening is important for the effects of these lipophilic modulators, suggesting that they can interact with the channel during the closed state.
Computational results suggest that capsaicin and dihydrocapsaicin bind to allosteric transmembrane binding site situated between transmembrane (TM), TM1, TM2, TM3, and TM4 in close proximity to extracellular domain. Capsaicin and dihydrocapsaicin make hydrophobic interactions with TM4, TM3 and hydrogen bond with TM1, which may stabilize 5HT3 in closed conformation. Capsaicin and dihydrocapsaicin have bended conformation inside of the binding pocket where flexible alkyl tail is situated between TM4 and TM3 making hydrophobic contact with them. According to the docking calculations, capsazepine has preferable binding position between extracellular and transmembrane domain making hydrogen bonds inside of the binding site. Although capsazepine is structural analog of capsaicin it is less flexible.Vanillin binds to both allosteric binding sites with similar probability while in the transmembrane binding site it makes interactions with the amino acids located on TM3 and TM4 helices.
Recently, capsaicin has been shown to inhibit glycine (Thakre and Bellingham, 2017; Thakre and Bellingham, 2019) and α7-nicotinic acetylcholine receptors (Alzaabi et al., 2019) indicating that, in addition to 5-HT3 receptors, other members of ligand-gated ion channel family are also targets mediating wide range of pharmacological actions of capsaicin. In conclusion, our results indicate that capsaicin acts as a negative allosteric modulator of not only homomerically, but also heteromerically (5-HT3AB with 1:1 and 1:2 ratio) expressed human 5-HT3 receptor.
Data Availability Statement
The raw data supporting the conclusions of this article will be made available by the authors, without undue reservation, to any qualified researcher.
Ethics Statement
The animal study was reviewed and approved by the Institutional Animal Care and Use Committee at the College of Medicine and Health Sciences, United Arab Emirates University (Protocol A9/08).
Author Contributions
EEN, TP, LH, and AHA conducted experiments and analyzed the data. TP, DEL, K-HSY, and FCH assisted on data analysis and writing the manuscript. MO planned and organized the study. All authors contributed to the article and approved the submitted version.
Funding
The research in this study was supported by grants from CMHS, UAE University and Kuwait University-The Kuwait Foundation for the Advancement of Sciences (KFAS).
Conflict of Interest
The authors declare that the research was conducted in the absence of any commercial or financial relationships that could be construed as a potential conflict of interest.
References
Al Kury, L. T., Mahgoub, M., Howarth, F. C., Oz, M. (2018). Natural Negative Allosteric Modulators of 5-HT3 Receptors. Mol. (Basel Switzerland) 23, 3186. doi: 10.3390/molecules23123186
Alzaabi, A. H., Howarth, L., El Nebrisi, E., Syed, N., Susan Yang, K. H., Howarth, F. C., et al. (2019). Capsaicin inhibits the function of α7-nicotinic acetylcholine receptors expressed in Xenopus oocytes and rat hippocampal neurons. Eur. J. Pharmacol. 857, 172411. doi: 10.1016/j.ejphar.2019.172411
Aréchiga-Figueroa, I. A., Morán-Zendejas, R., Delgado-Ramírez, M., Rodríguez-Menchaca, A. A. (2017). Phytochemicals genistein and capsaicin modulate Kv2.1 channel gating. Pharmacol. Rep. 2017 (69), 1145–1153. doi: 10.1016/j.pharep.2017.05.018
Ashoor, A., Nordman, J. C., Veltri, D., Yang, K. H., Shuba, Y., Al Kury, L., et al. (2013). Menthol inhibits 5-HT3 receptor-mediated currents. J. Pharmacol. Exp. Ther. 347, 398–409. doi: 10.1124/jpet.113.203976
Barnes, N. M., Hales, T. G., Lummis, S. C., Peters, J. A. (2009). The 5-HT3 receptor–the relationship between structure and function. Neuropharmacology 56, 273–284. doi: 10.1016/j.neuropharm.2008.08.003
Bielefeldt, K. (2000). Differential effects of capsaicin on rat visceral sensory neurons. Neuroscience 101, 727–736. doi: 10.1016/S0306-4522(00)00375-4
Binienda, A., Storr, M., Fichna, J., Salaga, M. (2018). Efficacy and Safety of Serotonin Receptor Ligands in the Treatment of Irritable Bowel Syndrome: A Review. Curr. Drug Targets 19, 1774–1781. doi: 10.2174/1389450119666171227225408
Bley, K. (2013). Effects of Topical Capsaicin on Cutaneous Innervation: Implications for Pain Management. Open Pain J. 6, 81–94. doi: 10.2174/1876386301306010081
Button, D., Brownstein, M. (1993). Aequorin-expressing mammalian cell lines used to report Ca2+ mobilization. Cell Calcium 14, 663–671. doi: 10.1016/0143-4160(93)90091-J
Castillo, E., Lopez-Gonzalez, I., De Regil-Hernandez, R., Reyes-Duarte, D., Sanchez-Herrera, D., Lopez-Munguia, A., et al. (2007). Enzymatic synthesis of capsaicin analogs and their effect on the T-type Ca2+ channels. Biochem. Biophys. Res. Commun. 356, 424–430. doi: 10.1016/j.bbrc.2007.02.144
Chien, C. S., Ma, K. H., Lee, H. S., Liu, P. S., Li, Y. H., Huang, Y. S., et al. (2013). Dual effect of capsaicin on cell death in human osteosarcoma G292 cells. Eur. J. Pharmacol. 718, 350–360. doi: 10.1016/j.ejphar.2013.08.011
Dascal, N. (1987). The use of Xenopus oocytes for the study of ion channels. C.R.C. Crit. Rev. Biochem. 22, 317–387. doi: 10.3109/10409238709086960
Duan, Y., Zheng, J., Nicholson, R. A. (2007). Vanilloid (subtype 1) receptor-modulatory drugs inhibit [3H]batrachotoxinin-A 20-alpha-benzoate binding to Na+ channels. Basic Clin. Pharmacol. Toxicol. 100, 91–95. doi: 10.1111/j.1742-7843.2006.00010.x
Fakhfouri, G., Rahimian, R., Dyhrfjeld-Johnsen, J., Zirak, M. R., Beaulieu, J. M. (2019). 5-HT3 Receptor Antagonists in Neurologic and Neuropsychiatric Disorders: The Iceberg Still Lies beneath the Surface. Pharmacol. Rev. 71, 383–412. doi: 10.1124/pr.118.015487
Grissmer, S., Nguyen, A. N., Aiyar, J., Hanson, D. C., Mather, R. J., Gutman, G. A., et al. (1994). Pharmacological characterization of five cloned voltage-gated K+ channels, types Kv1.1, 1.2, 1.3, 1.5, and 3.1, stably expressed in mammalian cell lines. Mol. Pharmacol. 45, 1227–1234.
Groom, C. R., Bruno, I. J., Lightfoot, M. P., Ward, S. C. (2016). The Cambridge Structural Database. Acta Crystallogr. Section B. Struct. Sci. Crystal Eng. Mater. 72, 171–179. doi: 10.1107/S2052520616003954
Hagenacker, T., Splettstoesser, F., Greffrath, W., Treede, R. D., Büsselberg, D. (2005). Capsaicin differentially modulates voltage-activated calcium channel currents in dorsal root ganglion neurones of rats. Brain Res. 1062, 74–85. doi: 10.1016/j.brainres.2005.09.033
Hassaine, G., Deluz, C., Grasso, L., Wyss, R., Tol, M. B., Hovius, R., et al. (2014). X-ray structure of the mouse serotonin 5-HT3 receptor. Nature 512, 276–281. doi: 10.1038/nature13552
Hu, W. P., You, X. H., Guan, B. C., Ru, L. Q., Chen, J. G., Li, Z. W. (2004). Substance P potentiates 5-HT3 receptor-mediated current in rat trigeminal ganglion neurons. Neurosci. Lett. 365, 147–152. doi: 10.1016/j.neulet.2004.04.072
Ingólfsson, H. I., Thakur, P., Herold, K. F., Hobart, E. A., Ramsey, N. B., Periole, X., et al. (2014). Phytochemicals perturb membranes and promiscuously alter protein function. A.C.S. Chem. Biol. 9, 1788–1798. doi: 10.1021/cb500086e
Jones, S., Yakel, J. L. (2003). Casein kinase ii (protein kinase ck2) regulates serotonin 5-ht(3) receptor channel function in ng108-15 cells. Neuroscience 119, 629–634. doi: 10.1016/S0306-4522(03)00202-1
Juza, R., Vlcek, P., Mezeiova, E., Musilek, K., Soukup, O., Korabecny, J. (2020). Recent advances with 5-HT3 modulators for neuropsychiatric and gastrointestinal disorders. Med. Res. Rev. 40, 1593–1678. doi: 10.1002/med.21666
Kida, R., Noguchi, T., Murakami, M., Hashimoto, O., Kawada, T., Matsui, T., et al. (2018). Supra-pharmacological concentration of capsaicin stimulates brown adipogenesis through induction of endoplasmic reticulum stress. Sci. Rep. 8, 845. doi: 10.1038/s41598-018-19223-2
Kim, J. A., Kang, Y. S., Lee, Y. S. (2005). A phospholipase C-dependent intracellular Ca2+ release pathway mediates the capsaicin-induced apoptosis in HepG2 human hepatoma cells. Arch. Pharm. Res. 28, 73–80. doi: 10.1007/BF02975139
Kim, S., Chen, J., Cheng, T., Gindulyte, A., He, J., He, S., et al. (2018). PubChem 2019 update: improved access to chemical data. Nucleic Acids Res. 47, D1102–D1109. doi: 10.1093/nar/gky1033
Kuenzi, F. M., Dale, N. (1996). Effect of capsaicin and analogues on potassium and calcium currents and vanilloid receptors in Xenopus embryo spinal neurones. Br. J. Pharmacol. 119, 81–90. doi: 10.1111/j.1476-5381.1996.tb15680.x
Lee, A. J., King, J. R., Barrett, D. A. (1997). Percutaneous absorption: a multiple pathway model. J. Controlled Release 45, 141–151. doi: 10.1016/S0168-3659(96)01567-2
Lohning, A. E., Marx, W., Isenring, L. (2016). In silico investigation into the interactions between murine 5-HT3 receptor and the principle active compounds of ginger (Zingiber officinale). J. Mol. Graph. Model 70, 315–327. doi: 10.1016/j.jmgm.2016.10.008
Lumpkin, E. A., Caterina, M. J. (2007). Mechanisms of sensory transduction in the skin. Nature 445, 858–865. doi: 10.1038/nature05662
Lundbaek, J. A., Birn, P., Tape, S. E., Toombes, G. E., Sogaard, R., Koeppe, R. E., et al. (2005). Capsaicin regulates voltage-dependent sodium channels by altering lipid bilayer elasticity. Mol. Pharmacol. 68, 680–689. doi: 10.1124/mol.105.013573
Lundbaek, J. A., Koeppe, R. E. 2nd., Andersen, O. S. (2010). Amphiphile regulation of ion channel function by changes in the bilayer spring constant. Proc. Natl. Acad. Sci. U. S. A. 107, 15427–15430. doi: 10.1073/pnas.1007455107
Morris, G. M., Goodsell, D. S., Halliday, R. S., Huey, R., Hart, W. E., Belew, R. K., et al. (1998). Automated docking using a Lamarckian genetic algorithm and an empirical binding free energy function. J. Comput. Chem. 19, 1639–1662. doi: 10.1002/(SICI)1096-987X(19981115)19:14<1639::AID-JCC10>3.0.CO;2-B
Morris, G. M., Huey, R., Lindstrom, W., Sanner, M. F., Belew, R. K., Goodsell, D. S., et al. (2009). AutoDock4 and AutoDockTools4: Automated docking with selective receptor flexibility. J. Comput. Chem. 30, 2785–2791. doi: 10.1002/jcc.21256
Ohkita, M., Saito, S., Imagawa, T., Takahashi, K., Tominaga, M., Ohta, T. (2012). Molecular cloning and functional characterization of Xenopus tropicalis frog transient receptor potential vanilloid 1 reveal its functional evolution for heat, acid, and capsaicin sensitivities in terrestrial vertebrates. J. Biol. Chem. 287, 2388–2397. doi: 10.1074/jbc.M111.305698
O’Neill, J., Brock, C., Olesen, A. E., Andresen, T., Nilsson, M., Dickenson, A. H. (2012). Unravelling the mystery of capsaicin: a tool to understand and treat pain. Pharmacol. Rev. 64, 939–971. doi: 10.1124/pr.112.006163
Oz, M., Soldatov, N. M., Melia, M. T., Abernethy, D. R., Morad, M. (1998). Functional coupling of human L-type Ca2+ channel and angiotensin AT1A receptor coexpressed in Xenopus oocytes. Mol. Pharmacol. 54, 1106–1112. doi: 10.1124/mol.54.6.1106
Oz, M., Zhang, L., Morales, M. (2002a). Endogenous cannabinoid, anandamide, acts as a noncompetitive inhibitor on 5-HT3 receptor-mediated responses in Xenopus oocytes. Synapse 46, 150–156. doi: 10.1002/syn.10121
Oz, M., Zhang, L., Spivak, C. E. (2002b). Direct noncompetitive inhibition of 5-HT(3) receptor-mediated responses by forskolin and steroids. Arch. Biochem. Biophys. 404, 293–301. doi: 10.1016/S0003-9861(02)00279-5
Oz, M., Zakharova, I., Dinc, M., Shippenberg, T. (2004). Cocaine inhibits cromakalim-activated K+ currents in follicle-enclosed Xenopus oocytes. Naunyn Schmiedebergs Arch. Pharmacol. 369, 252–259. doi: 10.1007/s00210-003-0838-9
Oz, M., Jaligam, V., Galadari, S., Petroianu, G., Shuba, Y. M., Shippenberg, T. S. (2010). The endogenous cannabinoid, anandamide, inhibits dopamine transporter function by a receptor-independent mechanism. J. Neurochem. 112, 1454–1464. doi: 10.1111/j.1471-4159.2009.06557.x
Oz, M., Lozon, Y., Sultan, A., Yang, K. H., Galadari, S. (2015). Effects of monoterpenes on ion channels of excitable cells. Pharmacol. Ther. 152, 83–97. doi: 10.1016/j.pharmthera.2015.05.006
Oz, M. (2006). Receptor-independent actions of cannabinoids on cell membranes: focus on endocannabinoids. Pharmacol. Ther. 111, 114–144. doi: 10.1016/j.pharmthera.2005.09.009
Patowary, P., Pathak, M. P., Zaman, K., Raju, P. S., Chattopadhyay, P. (2017). Research progress of capsaicin responses to various pharmacological challenges. Biomed. Pharmacother. 96, 1501–1512. doi: 10.1016/j.biopha.2017.11.124
Rollyson, W. D., Stover, C. A., Brown, K. C., Perry, H. E., Stevenson, C. D., McNees, C. A., et al. (2014). Bioavailability of capsaicin and its implications for drug delivery. J. Control. Release 196, 96–105. doi: 10.1016/j.jconrel.2014.09.027
Savitha, G., Panchanathan, S., Salimath, B. P. (1990). Capsaicin inhibits calmodulin-mediated oxidative burst in rat macrophages. Cell. Signal. 6, 577–585. doi: 10.1016/0898-6568(90)90080-T
Sharma, S. K., Vij, A. S., Sharma, M. (2013). Mechanisms and clinical uses of capsaicin. Eur. J. Pharmacol. 720, 55–62. doi: 10.1016/j.ejphar.2013.10.053
Srinivasan, K. (2016). Biological Activities of Red Pepper (Capsicum annuum) and Its Pungent Principle Capsaicin: A Review. Crit. Rev. Food. Sci. Nutr. 56, 1488–1500. doi: 10.1080/10408398.2013.772090
Swain, J., Kumar Mishra, A. (2015). Location, Partitioning Behavior, and Interaction of Capsaicin with Lipid Bilayer Membrane: Study Using Its Intrinsic Fluorescence. J. Phys. Chem. B. 119, 12086–12093. doi: 10.1021/acs.jpcb.5b05351
Thakre, P. P., Bellingham, M. C. (2017). Capsaicin Enhances Glutamatergic Synaptic Transmission to Neonatal Rat Hypoglossal Motor Neurons via a TRPV1-Independent Mechanism. Front. Cell. Neurosci. 11, 383. doi: 10.3389/fncel.2017.00383
Thakre, P. P., Bellingham, M. C. (2019). Capsaicin causes robust reduction in glycinergic transmission to rat hypoglossal motor neurons via a TRPV1-independent mechanism. J. Neurophysiol. 121, 1535–1542. doi: 10.1152/jn.00059.2019
Thompson, A. J., Lummis, S. C. (2006). 5-HT3 receptors. Curr. Pharm. Des. 12, 3615–3630. doi: 10.2174/138161206778522029
Thompson, A. J., Lummis, S. C. (2007). The 5-HT3 receptor as a therapeutic target. Expert. Opin. Ther. Targets 11, 527–540. doi: 10.1517/14728222.11.4.527
Tomohiro, D., Mizuta, K., Fujita, T., Nishikubo, Y., Kumamoto, E. (2013). Inhibition by capsaicin and its related vanilloids of compound action potentials in frog sciatic nerves. Life Sci. 92, 368–378. doi: 10.1016/j.lfs.2013.01.011
Trott, O., Olson, A. J. (2010). AutoDock Vina: improving the speed and accuracy of docking with a new scoring function, efficient optimization and multithreading. J. Comput. Chem. 31, 455–461. doi: 10.1002/jcc.21334
Walstab, J., Combrink, S., Brüss, M., Göthert, M., Niesler, B., Bönisch, H. (2007). Aequorin luminescence-based assay for 5-hydroxytryptamine (serotonin) type 3 receptor characterization. Anal. Biochem. 368, 185–192. doi: 10.1016/j.ab.2007.06.007
Wang, S. Y., Mitchell, J., Wang, G. K. (2007). Preferential block of inactivation-deficient Na+ currents by capsaicin reveals a non-TRPV1 receptor within the Na+ channel. Pain 127, 73–83. doi: 10.1016/j.pain.2006.08.002
Wohlrab, J., Neubert, R. H., Heskamp, M. L., Michael, J. (2015). Cutaneous drug delivery of capsaicin after in vitro administration of the 8% capsaicin dermal patch system. Skin Pharmacol. Physiol. 28, 65–74. doi: 10.1159/000362740
Wu, T., Song, L., Shi, X., Jiang, Z., Santos-Sacchi, J., Nuttall, A. L. (2011). Effect of capsaicin on potassium conductance and electromotility of the guinea pig outer hair cell. Hear Res. 272, 117–124. doi: 10.1016/j.heares.2010.10.010
Xu, Y. P., Zhang, J. W., Li, L., Ye, Z. Y., Zhang, Y., Gao, X., et al. (2012). Complex regulation of capsaicin on intracellular second messengers by calcium dependent and independent mechanisms in primary sensory neurons. Neurosci. Lett. 517, 30–35. doi: 10.1016/j.neulet.2012.04.011
Yang, K. H., Galadari, S., Isaev, D., Petroianu, G., Shippenberg, T. S., Oz, M. (2010a). The nonpsychoactive cannabinoid cannabidiol inhibits 5-hydroxytryptamine3A receptor-mediated currents in Xenopus laevis oocytes. J. Pharmacol. Exp. Ther. 333, 547–554. doi: 10.1124/jpet.109.162594
Yang, K. H., Isaev, D., Morales, M., Petroianu, G., Galadari, S., Oz, M. (2010b). The effect of Δ9-tetrahydrocannabinol on 5-HT3 receptors depends on the current density. Neuroscience 171, 40–9. doi: 10.1016/j.neuroscience.2010.08.044
Zhang, L., Oz, M., Weight, F. F. (1995). Potentiation of 5-HT3 receptor-mediated responses by protein kinase C activation. Neuroreport 6, 1464–1468. doi: 10.1097/00001756-199507100-00025
Zhang, S., Wang, D., Huang, J., Hu, Y., Xu, Y. (2020). Application of capsaicin as a potential new therapeutic drug in human cancers. J. Clin. Pharm. Ther. 45, 16–28. doi: 10.1111/jcpt.13039
Keywords: capsaicin, 5-HT3 receptor, Xenopus oocytes, HEK-293 cells, serotonin, allosteric modulator, docking
Citation: Nebrisi EE, Prytkova T, Lorke DE, Howarth L, Alzaabi AH, Yang K-HS, Howarth FC and Oz M (2020) Capsaicin Is a Negative Allosteric Modulator of the 5-HT3 Receptor. Front. Pharmacol. 11:1274. doi: 10.3389/fphar.2020.01274
Received: 26 May 2020; Accepted: 31 July 2020;
Published: 31 August 2020.
Edited by:
Antonio Ferrer-Montiel, Miguel Hernández University of Elche, SpainReviewed by:
Marco Caprini, University of Bologna, ItalyOscar Moran, Italian National Research Council, Italy
Copyright © 2020 Nebrisi, Prytkova, Lorke, Howarth, Alzaabi, Yang, Howarth and Oz. This is an open-access article distributed under the terms of the Creative Commons Attribution License (CC BY). The use, distribution or reproduction in other forums is permitted, provided the original author(s) and the copyright owner(s) are credited and that the original publication in this journal is cited, in accordance with accepted academic practice. No use, distribution or reproduction is permitted which does not comply with these terms.
*Correspondence: Murat Oz, murat.oz@hsc.edu.kw; ahmet.oz@ku.edu.kw