- Department of Medicine, the Second Affiliated Hospital and Yuying Children’s Hospital of Wenzhou Medical University, Wenzhou, China
Astragaloside IV (AS-IV) has a variety of biological activities and is widely used to treat kidney diseases. We conducted a systematic review of 24 animal studies including 424 animals to evaluate the efficacy of AS-IV for diabetic nephropathy (DN); all current possible mechanisms were summarized. A search strategy was applied to eight databases from inception to June 2020. The CAMARADES 10-item quality checklist and Rev-Man 5.3 software were used to analyze the risks of bias of each study and data regarding outcome measures, respectively. The mean study quality score was 5.4 points (range 3–8 points). Meta-analyses data and comparisons between groups showed that AS-IV significantly slowed the progression of pathological signs in the kidney including glomeruli and tubules, increasing creatinine clearance rate, decreasing blood urea nitrogen, serum creatinine, 24-h urinary neutrophil gelatinase-associated lipocalin and N-acetyl-β-D-glucosaminidase, 24-h urinary albumin, 24-h urinary microalbumin and HbA1c. There were no significant differences between experimental and control groups with respect to mortality or levels of alanine aminotransferase and aspartate aminotransferase. In terms of the possible mechanisms of treatment of DN, AS-IV acts through antifibrotic, antioxidant, and antiapoptotic mechanisms, thereby alleviating endoplasmic reticulum stress, inhibiting mitochondrial fission, and increasing autophagic activity. Taken together, our findings suggest that AS-IV is a multifaceted renoprotective candidate drug for DN.
Introduction
Diabetic nephropathy (DN) is defined as impairment of renal function arising from chronic hyperglycemia. It is among the most severe and common microvascular complications of diabetes mellitus (Saran et al., 2015; Flyvbjerg, 2017). It is characterized by proteinuria, hypertension, and progressive renal insufficiency resulting from compromise of the glomerular filtration barrier, eventually resulting in end-stage renal disease (ESRD) in 30–40% of patients (Bjornstad et al., 2014; Saran et al., 2015; Wang et al., 2016; Du et al., 2017). Management of hypertension and hyperglycemia is the most commonly used approach for treatment of DN. These have been shown to reduce the proportion of patients reaching ESRD (National Kidney Foundation, 2012), although with limited effects (Sharma et al., 2017). Unfortunately, no new therapies that specifically improve progression of DN have been translated into clinical use successfully (Fernandez-Fernandez et al., 2014). For this reason, new therapies are needed urgently to prevent progressive renal failure in these patients.
Astragalus membranaceus (Fisch.) Bunge is a perennial herbal plant of the Leguminous family that first appeared in the earliest complete Pharmacopoeia of China (Sheng Nong’s herbal classic work). It was thought to promote Qi according to the theory of traditional Chinese medicine and therefore was widely used for various kidney diseases for thousands of years. Astragaloside IV (AS-IV, C41H68O14, molecular weight = 784, Figure 1) is a small molecular saponin that is the active ingredient in A. membranaceus (Fisch.) Bunge. Recent studies demonstrated that AS-IV has several pharmacological activities in vivo and in vitro, including anti-inflammatory, antioxidative, antiapoptotic, antifibrotic, and immunoregulatory functions. AS-IV has also been reported to improve the prognosis of DN in streptozotocin-induced (STZ) diabetic rats via inhibition of renal inflammation (Gui et al., 2013a), inhibition of renal oxidative stress (Gui et al., 2013b), attenuation of podocyte apoptosis (Guo et al., 2016), and subsequent delay of DN progression (Sun et al., 2016). Nevertheless, the efficacy of AS-IV for DN has not been evaluated systematically, and the mechanisms have not been summarized comprehensively. Therefore, the aim of the present study was to evaluate systematically research reports on the subject in order to summarize the significant outcomes on efficacy and mechanisms.
Methods
Data Sources and Search Strategies
Animal experimental studies of AS-IV for DN were identified using a computerized literature search of Chinese Science and Technology Journal Database, WanFang, China National Knowledge Infrastructure, Chinese Biomedical Database, EMBASE, Cochrane library, PubMed, and Web of Science. All search strategies were performed from inception to June 2020. The following search terms were used in PubMed and were modified to suit other databases: “Astragaloside IV OR AS-IV OR Astragalus membranaceus” AND “Diabetes OR diabetic nephropathy”. The reference lists of all the eligible studies were searched carefully to obtain additional studies.
Eligibility Criteria
The abstracts and titles of studies were screened and the full-text articles were subsequently reviewed for inclusion and exclusion by two authors (Hong Wang and Zhuang Zhuang) independently. Inclusion criteria were as follows (1) AS-IV (as monotherapy in any dose) to treat animal models of DN established in various ways; (2) nonfunctional and equal volumes of liquid (normal saline) or no treatment adopted for the control group; and (3) primary outcome measures were renal pathology, creatinine clearance rate (CCr), 24-h urinary albumin or microalbumin, 24-h urinary neutrophil gelatinase-associated lipocalin (NGAL) or N-acetyl-β-D-glucosaminidase (NAG), serum creatinine (SCr), blood urea nitrogen (BUN), HbA1c, or indicators of adverse reactions. The renoprotective mechanisms of AS-IV for DN were selected as secondary outcome measures. Exclusion criteria were as follows: (1) not in vivo studies (in vitro studies, clinical trials, review articles, case reports, comments, editorials, and abstracts); (2) treatment with AS-IV-based prescriptions or combinations with other drugs; (3) comparisons with other drugs with unclear efficacy; (4) no predetermined outcome index or available data; (5) not a DN model; (6) duplicate publication; and (7) no control group.
Data Extraction
Two authors (HW and Y-YH) independently evaluated all the eligible articles for data extraction in terms of: (1) the publication year of the study and the name of the first author; (2) details regarding the animals; (3) the methods to establish animal models, the criteria for modeling successfully and the use of anesthetics in the course of experiment; (4) the therapeutic regimen and the control group; and (5) primary, secondary outcomes, and intergroup differences. If the outcomes were displayed through gradient doses of drug therapy or multiple time points, only the data of the highest dose group and peak time point group were included. The authors were contacted for specific data when the results were only rendered graphically. If a response was not received, the graph data were measured using Photoshop.
Risk of Bias in Individual Studies
For each included study, quality assessment was carried out by two authors (ZZ and Y-YH) independently using the CAMARADES 10-item quality checklist (Macleod et al., 2004) with minor modifications (modified sections: D: blinded induction of model [group randomly after modeling, or transgenic mice, or knockout mice]; F: use of anesthetic without significant renal protective activity or nephrotoxicity). Disagreements in selecting studies, extracting data, or assessing the quality of studies were resolved by consensus or arbitration by the correspondence authors (QZ and Y-LW).
Statistical Analysis
RevMan 5.3 software downloaded on the website (https://www.cochrane.org/) was used for data analysis where possible. When meta-analysis failed to run, comparisons between groups were performed for individual studies. The combined overall effect sizes of outcome measures were estimated by utilizing standard mean difference (SMD) with 95% confidence interval (CI). Heterogeneity was determined using the Cochrane Q-statistic test and the I2-statistic test (random effects model [I2 greater than 50%] or a fixed effects model [I2 less than or equal to 50%]). Sensitivity analyses were conducted when individual results deviated substantially. Bar graphs were drawn using Prism 6. When the probability value was less than 0.05, the difference was considered statistically significant.
Results
Study Selection
The search strategy yielded 1,053 potentially relevant studies from the eight databases, of which 878 were duplicated or irrelevant studies. We excluded 91 non-animal studies after checking the titles and abstracts. Detailed inspection was performed for remaining 61 full-text studies; 37 of these were not considered because they presented at least one of the excluding criteria. Finally, 24 eligible studies were identified: 13 in English (Gui et al., 2012; Chen et al., 2014; Lu et al., 2015; Wang et al., 2015; Guo et al., 2016; Sun et al., 2016; Guo et al., 2017; Liu et al., 2017; Du et al., 2018; Lei et al., 2018a; Lei et al., 2018b; Fan et al., 2019; Ju et al., 2019) and 11 in Chinese (Chen and Chen, 2016; Huang et al., 2016; Li and Zhang, 2016; Ran and Ma, 2016; Wang et al., 2017; Han et al., 2018; He et al., 2018; Li, 2018; Liu et al., 2019; Ma et al., 2019; Song et al., 2020) (Figure 2).
Characteristics of Included Studies
Twenty-four studies with 424 animals were included. The sample size ranged from 12 to 28 animals in each study. Sprague Dawley (SD) male rats were used in 16 studies; male Wistar rats in two studies, male C57BL/6 mice in two studies, male db/db mice in three studies, and male KKAy mice in one study. The weight of SD or Wistar rats varied between 170 and 260 g, and the weight of mice varied between 16 and 24 g. Eighteen studies established the DN model by intraperitoneal injection of streptozotocin (STZ); two studies used feeding high-fat diet for several weeks and intraperitoneal injection of STZ; four studies used mutant or transgenic mice with spontaneous diabetes (four studies used db/db mice and one study used KKAy mice) that exhibits clinical and histological features of DN resembling those found in human DN (Sharma et al., 2003; Tesch and Lim, 2010). To induce anesthesia, anesthetics were unreported in 11 studies, pentobarbital sodium was reported in seven studies, chloral hydrate was used in four studies, urethane was used in one study, and ether was used in one study. Detailed information of AS-IV in each study is displayed in Table 1. Twenty-two studies implemented a dose gradient of AS-IV ranging from 3 to 1.08 g•kg−1•d−1 using oral or intragastric administration. Rats received 5 mmol•d−1 AS-IV by oral gavage in one study and normal standard diet supplemented with AS-IV (AS-IV: feed = 5 g:1 kg) was administered to rats by oral gavage in one study. In terms of outcome measures, renal pathology was utilized as primary outcome measure in 19 studies, BUN in 13 studies, SCr in 13 studies, 24-h urinary protein in 12 studies, 24-h urinary microalbumin in six studies, 24-h urinary NAG in three studies, 24-h urinary NGAL in two studies, blood glucose (BG) in 17 studies, glycated hemoglobin (HbA1c) in four studies; CCr in one study; alanine aminotransferase (ALT) in three studies, and aspartate aminotransferase (AST) in two studies. Secondary outcomes were as follows: superoxide dismutase (SOD) was reported in three studies; catalase (CAT) in two studies; malondialdehyde (MDA) in four studies; glutathione peroxidase (GSH-PX) in three studies; tumor necrosis factor-α (TNF-α) in one study; monocyte chemotactic protein-1 (MCP-1) in one study; caspase-3 in two studies; caspase-12 in one study; Bax and Bcl-2 in two studies; transforming growth factor-β1 (TGF-β1) in five studies; Smad2/3 in two studies; Smad7 in two studies; phosphothreonine kinase (p-Akt) in four studies; phosphorylated phosphatidylinositol-3-kinase (p-PI3K) in two studies; PKR-like eukaryotic initiation factor 2A kinase 1/2 (pERK-1/2) in three studies; PTEN-induced putative kinase (PINK) in one study; Jun N-terminal kinases (JUN) in two studies; forkhead box O1 (Fox O1) in one study; mammalian target of rapamycin (mTOR) in two studies; nuclear factor kappa B (NF-κB) in 2 studies; glucose-regulated protein 78 (GRP78) in three studies; sarco-endoplasmic reticulum calcium adenosine triphosphatase (SERCA) in two studies. The detailed characteristics of the included studies are displayed in Table 2.
Study Quality
The CAMARADES 10-item quality checklist was adopted to judge the risk of bias of each study and the number of criteria met varied from 3/10 to 8/10 with the average of 5.4. The review authors’ judgments about each risk of bias item for each included study are summarized in Table 3.
Effectiveness
Renal Pathology
Compared with the control group, AS-IV treatment inhibited podocyte apoptosis and ameliorate podocyte foot process effacement significantly in nine studies as assessed using electron or light microscopy. Treatment alleviated mesangial cell proliferation and the broadening of mesangial matrix significantly in 14 studies, but not to a significant degree in one study. There was inhibition of basement membrane thickening in four studies, narrowing the enlarged glomerular volume and alleviated the level of glomerular fibrosis in six studies, and alleviation of wall edema in proximal tubules and reduction of apoptosis of renal tubular epithelial cells in four studies.
CCr, BUN, and SCr
We found that CCr was reduced significantly by AS-IV compared with the control group (P < 0.05). Meta-analysis of 13 studies indicated BUN was reduced significantly by AS-IV compared with the control group (Figure 3). The publication bias funnel indicated that there is no substantial publication bias (Figure 4). Meta-analysis of 13 studies indicated SCr was decreased significantly by AS-IV (Figure 5). Sensitivity analyses of indicators of BUN and SCr were carried out, and these indicated that heterogeneity did not decline significantly after eliminating any study.
Levels of 24-h Urinary Albumin, Microalbumin, NAG, and NGAL
Meta-analysis of 12 and six studies reported that 24-h urinary albumin (Figure 6) and 24-h urinary microalbumin, respectively, were reduced significantly by AS-IV. After sensitivity analyses, one study was removed because the DN experimental model in that study was established using intraperitoneal injection of STZ with 125 vs ≤65 mg/kg in other studies. Subsequently, heterogeneity of this indicator decreased substantially (Figure 7). Meta-analysis of three and two studies indicated 24-h urinary NAG (Figure 8) and 24-h urinary NGAL (Figure 8), respectively, were reduced significantly by AS-IV.
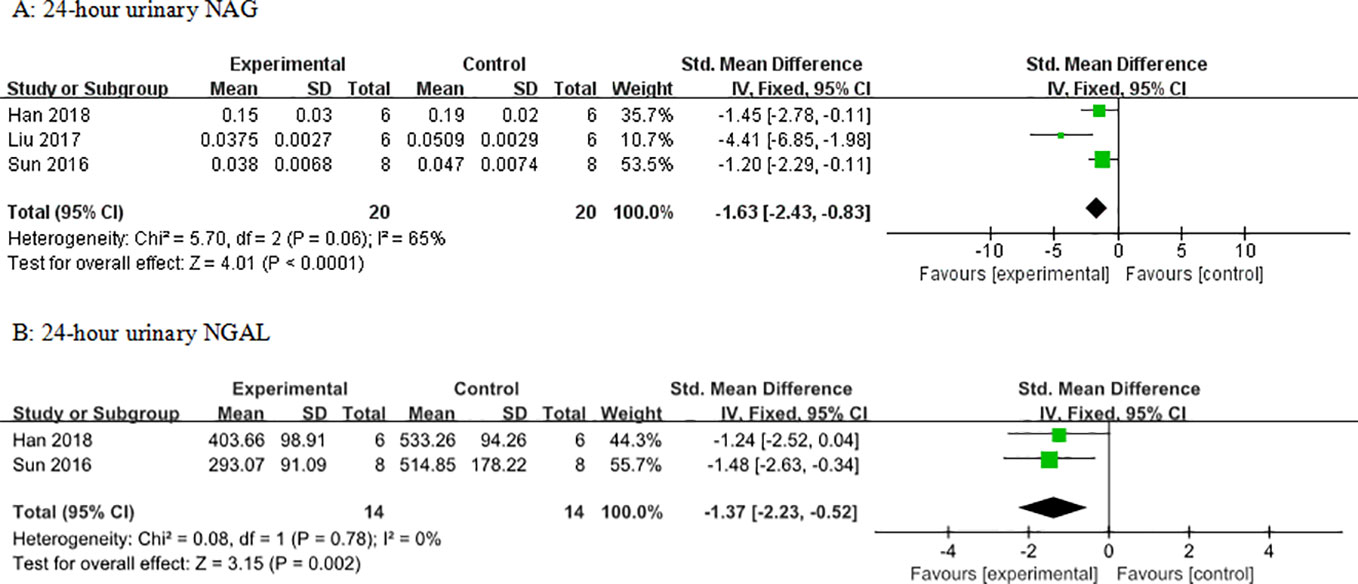
Figure 8 Effect of astragaloside IV on (A) 24-h urinary N-acetyl-β-D-glucosaminidase (NAG) and (B) neutrophil gelatinase-associated lipocalin (NGAL) vs control.
BG and HbA1c
Seventeen studies used BG as an outcome measure, and all of these indicated that BG was decreased by AS-IV compared with the control group (P < 0.05). HbA1c in four studies failed to pool in the analysis because the ways of calculating HbA1c varied. Meta-analysis of the remaining three studies indicated that HbA1c level was significantly decreased by AS-IV (Figure 9).
Adverse Reactions
Three studies utilized ALT and three studies utilized AST as outcome measure to assess the adverse effects of AS-IV on the liver. Meta-analysis of these studies showed no significant effect of AS-IV on ALT (Figure 10) or AST (Figure 10). Two studies reported mortality as an outcome measure, and meta-analysis of both studies showed that there was no significant difference in mortality between the AS-IV group and the control group (Figure 11). Two studies reported external manifestations in animals (including, activity, and glossiness of fur) with the AS-IV + STZ group showing better results than the STZ group.
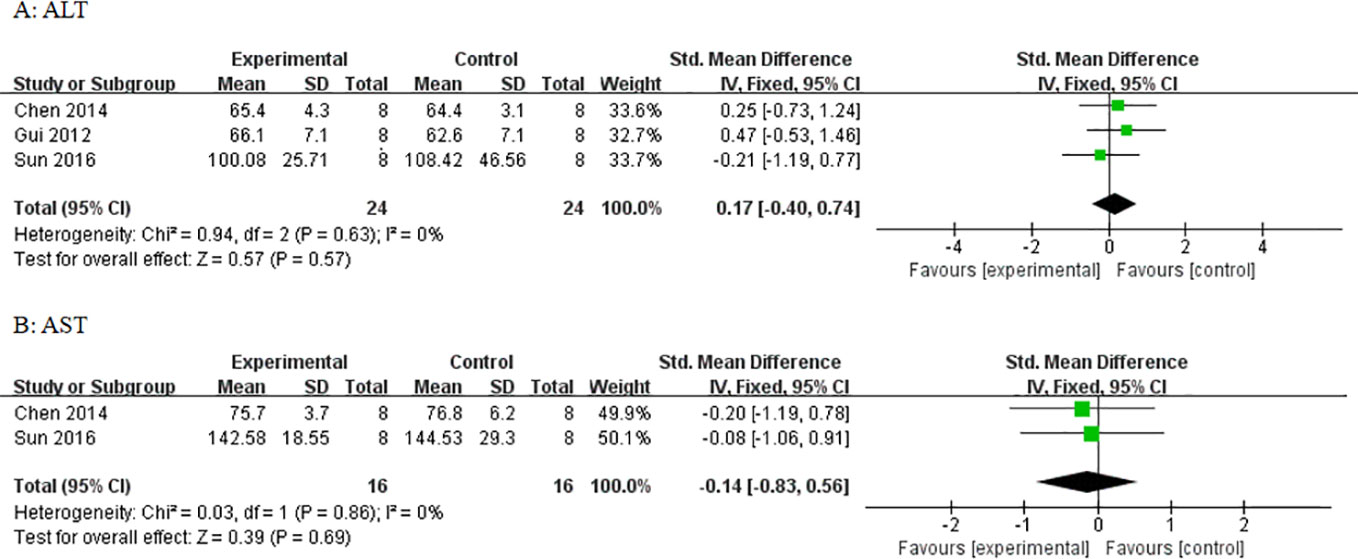
Figure 10 Effect of astragaloside IV on (A) alanine aminotransferase (ALT) and (B) aspartate aminotransferase (AST) vs control.
Renoprotective Mechanisms
Compared with control groups, meta-analysis of three studies and two studies showed that AS-IV increased SOD (Figure 12) and CAT (Figure 12), respectively. In three studies, there was increased GSH-px (P < 0.05). In four studies, there were decreased levels of MDA (P < 0.05). In one study, there were decreased levels of TNF-α and MCP-1. In two studies, there were decreased levels of caspase-3. In one study, there were decreased levels of caspase-12. In two studies, there were decreased levels of Bax and increased levels of Bcl-2. In five studies, there were decreased levels of TGF-β1. In two studies, there were decreased levels of Smad2/3. In two studies, there were increased levels of Smad7. In two studies, there were decreased levels of NF-κB. In three studies, there were decreased levels of GRP78.
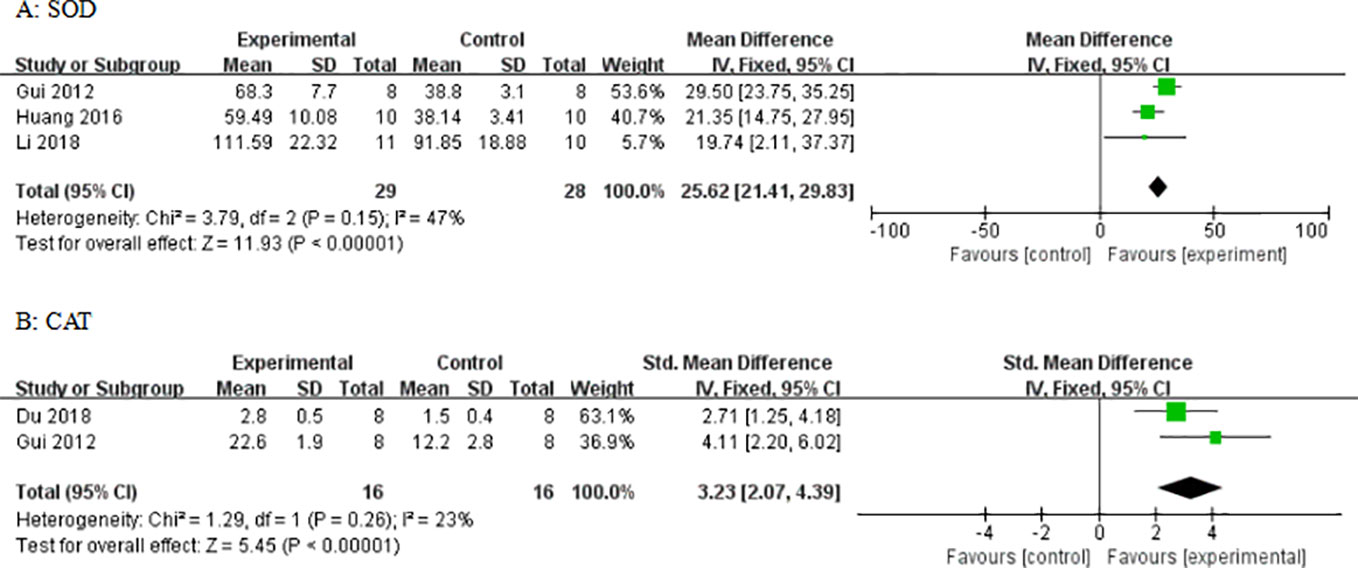
Figure 12 Effect of astragaloside IV on (A) superoxide dismutase (SOD) and (B) catalase (CAT) vs control.
Subgroup Analysis
Potential confounding factors (including various methods of modeling, different animal species, varying doses of AS-IV and duration of treatment) that may have increased the heterogeneity of outcome measure were explored using stratified analysis of the BUN and SCr. The subgroup analysis of Scr showed basically the same result as that of BUN (Figures 13 and 14). In the subgroup analysis of the various modeling methods, the effect size of the model induced by mutant mice showed a different result than that of the models induced by administration of STZ; the method of low dose STZ injection combined with high-fat diet (SMD −4.24 vs. SMD −1.89 vs. SMD −1.42 P < 0.05, Figure 13; SMD −0.1 vs. SMD −2.09 vs. SMD −3.04 P < 0.05, Figure 14), and the heterogeneity of the three groups decreased substantially. The group in which DN was induced in C57BL/6J mice showed greater effect size than in rats (SMD −3.62 vs. SMD −1.64, P < 0.05, Figure 13; SMD −6.73 vs. SMD −1.88, P < 0.05, Figure 14), and the heterogeneity of two groups decreased substantially. No difference was seen between the high-dose AS-IV group (>18 mg/kg, QD) and the low-dose group (≤18 mg/kg, QD) (SMD −1.96 vs. SMD −2.04, P > 0.05, Figure 13; SMD −3.17 vs. SMD −1.58, P > 0.05, Figure 14). Notably, the longer period of AS-IV treatment showed a smaller effect size than the shorter treatment lasting 8 weeks or less with a small drop in the heterogeneity of the two groups (SMD −1.23 vs. SMD −2.26, P < 0.05, Figure 13; SMD −0.92 vs. SMD −2.04, P < 0.05, Figure 14).
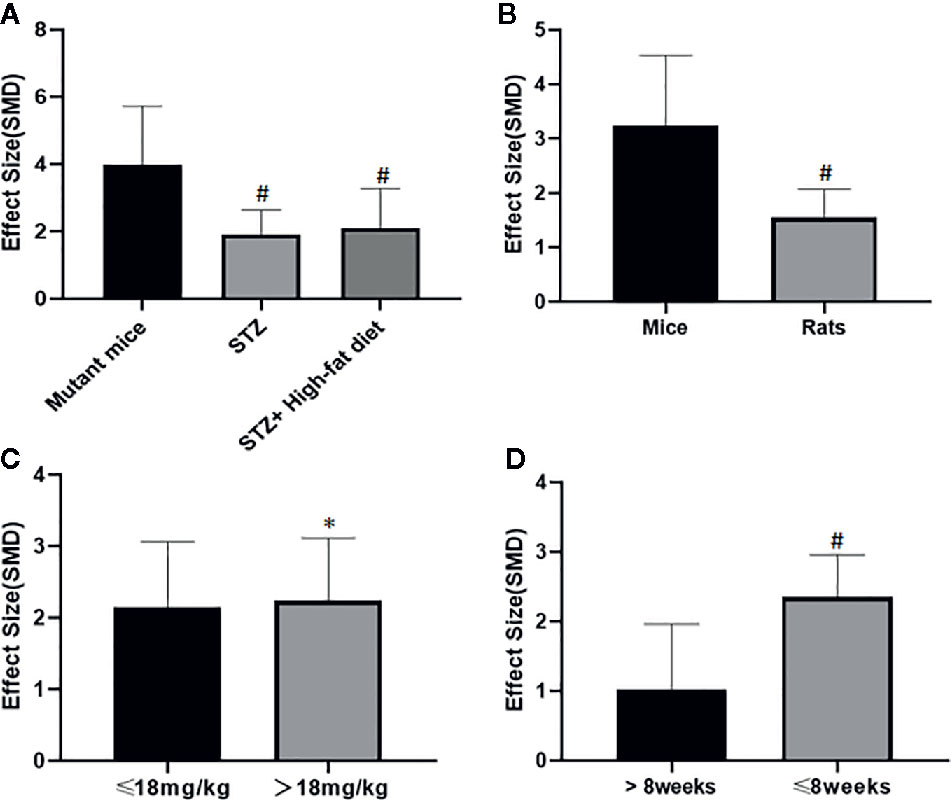
Figure 13 Effect of astragaloside IV on blood urea nitrogen in subgroups. (A) Induction type; (B) Species; (C) AS-IV dose; (D) Duration of treatment. #P < 0.05 vs control; *P > 0.05 vs control.
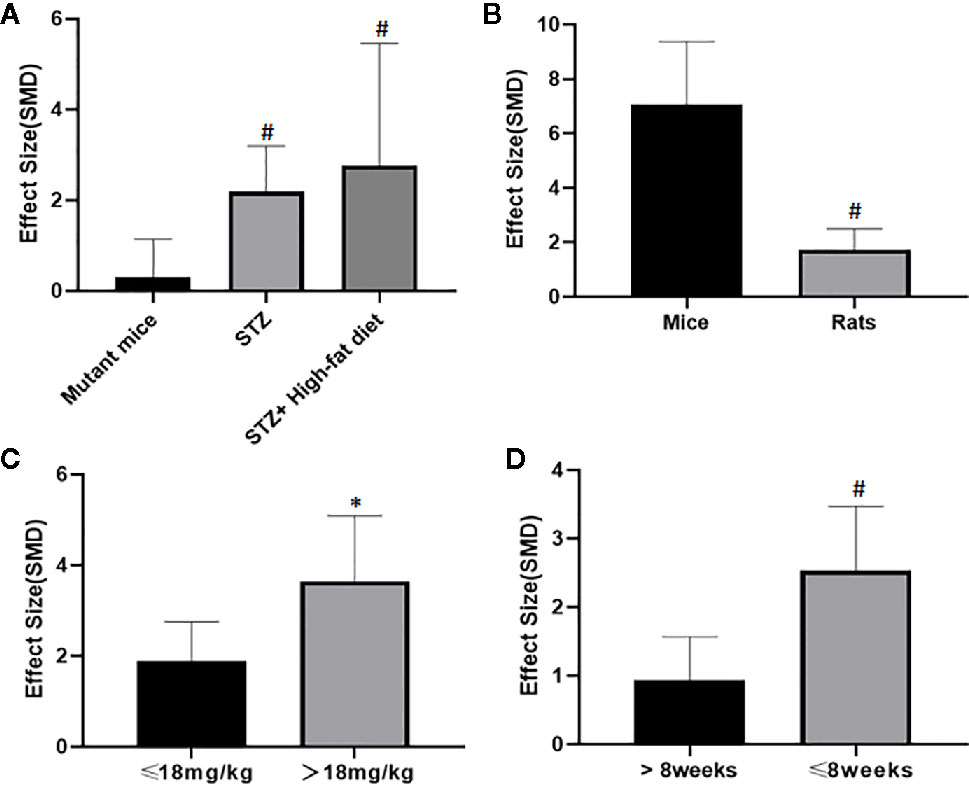
Figure 14 Effect of astragaloside IV on serum creatinine in subgroups. (A) Induction type; (B) Species; (C) AS-IV dose; (D) Duration of treatment. #P < 0.05 vs control; * P > 0.05 vs control.
Discussion
Summary of Evidence
This first-ever preclinical systematic review including 24 studies with 424 animals measured the efficacy of AS-IV for treatment of DN. The quality of included original studies was moderate. Our findings suggest that AS-IV is a multifaceted renoprotective candidate drug for treatment of DN.
Limitations
There are some limitations of this meta-analysis and systematic review: (1) though blinding induction of model was reported in 10 studies, most of the studies had flaws with respect to blinding assessment of outcome and sample size calculation (Moher et al., 2015); (2) selection bias was unavoidable because only eight frequently-used databases were searched for English and Chinese language articles; (3) the absence of negative studies might have led to the true effect of AS-IV being overestimated (Franco et al., 2014); (4) though animal welfare regulations were observed in 14 studies, no study reported disinfection when the experimenter performed intrusive procedures such as intraperitoneal injection, subcutaneous injection, and blood glucose measurement, all of which are regarded as crucial steps in animal models, especially in diabetic models; and (5) no study utilized animals with relevant comorbidities.
Implications
It is commendable that the blinding protocols were reported in ten studies; nevertheless, the average score of the included studies was moderate. According to the ARRIVE guidelines (Kilkenny et al., 2010), lack of crucial standards in study design (Moher et al., 2015) such as sample size estimation and blinded assessment of outcome is the primary defect. In future animal studies, the ARRIVE guidelines should be followed; sample size estimations and blinded assessments of outcome should be emphasized. Specific methods of these two points could be referred to in the following two studies (Hayes and Bennett, 1999; Hooijmans et al., 2014). Use of experimental animals with comorbidities such as advanced age, obesity, hypertension, hyperlipidemia, or other risk factors may be more in line with the physiology of diabetic patients and may be helpful for the clinical translation of experimental results (Brosius et al., 2009).
Different animals display varying sensitivities to STZ. C57BL/6 and CD-1 mice are reliably sensitive to STZ (Like and Rossini, 1976; Rossini et al., 1977) as are Wistar and SD rats. Indeed, rats rather than mice tend to be selected to establish diabetic models because their greater size is conducive to monitoring of renal physiology, access to sufficient renal tissue, and repeated blood sampling for analysis. It is difficult but necessary to strictly control the dosage of STZ to avoid problems of high mortality and low modeling rate due to the high sensitivity of rats to STZ. The subgroup analysis indicated that the group in which DN model was induced in the C57BL/6J mice showed greater effect size than that of rats though the dosage of STZ in the mice group (100 mg/kg, IP, QD for 2 d), much larger than that of the rat group (≤65 mg/kg), suggesting that high doses of STZ may cause less β-cell damage in mice. Although high-proficiency operational skills and high-quality nursing are required, replacing rats with mice less sensitive to STZ may be beneficial to increase the tolerance to STZ and to reduce the mortality of experimental animals. Furthermore, the included studies used three methods to imitate the characteristics of type 1 diabetes or type 2 diabetes to establish DN model according to varying purposes and laboratory conditions: 18 studies established the DN model using STZ; two used low-dose STZ injection combined with high-fat diet; and four studies used mutant or transgenic mice. In our subgroup analysis, the effect size of AS-IV for decreasing BUN and SCr in various models induced by different methods showed significant differences, suggesting that this factor may be the source of the high degree heterogeneity.
Although no difference was seen between the high- and low-dose AS-IV treatment groups, this subgroup analysis was carried out to determine the sources of high heterogeneity rather than its dose–effect relationship given that it is a comparison under different experimental conditions. We perused all included studies that were designed to study the effects of different doses of AS-IV in DN in the same experiments. Of these, two studies reported that the levels of BUN were markedly greater in the DN-vehicle group than in the control group, both of which were significantly reduced by AS-IV treatment in a dose-dependent manner (3–12 mg/kg). Six studies reported that varying doses of AS-IV (0.75–80mg/kg) decreased the levels of BUN, though not significantly (P > 0.05). Such a large oral dose range yields similar results suggesting that there may be problems in AS-IV absorption or reaction to receptor binding. Actually, previous studies have reported that the high molecular weight and low lipophilicity of AS-IV may limit its passive transport in the intestine which directly results in low permeability and low bioavailability of AS-IV (the absolute bioavailability in rat: 2.2% only) (Gu et al., 2004; Huang et al., 2006). Up to now, various strategies have also been studied to solve this problem. Absorption enhancers of AS-IV that can open the tight junction are one of the major directions to explore the absorption enhance strategy of AS-IV (Gu et al., 2004). Special solvent such as self-microemulsifying drug delivery system was explored to enhance absorption of AS-IV (Zhang et al., 2019). In addition, the development and application of astragaloside injection may effectively solve the intestinal absorption problem of AS-IV (Chen et al., 2019). In the present study, it still stays at the stage to explore whether the drug is effective, and its mechanisms and the results show that AS-IV has renoprotective functions in DN. The relationship between dose and efficacy remains uncertain probably due to low bioavailability of AS-IV at present. Thus, we suggested that the methods of appeal to promote the bioavailability of AS-IV should be applied more in future experiments to explore the optimal dose range of AS-IV in DN. Furthermore, the longer period of AS-IV treatment suggested poorer efficacy than the shorter treatment lasting 8 weeks or less, suggesting that the duration of treatment may be a source of the high degree of heterogeneity. We attribute this to the fact that DN is a progressive and irreversible disease, and extended treatment time of AS-IV merely delayed the progression of DN rather than reversing it.
Systematic reviews of preclinical studies are thought to be valuable tools to determine mechanisms and to provide important insights into the designed animal studies (Van et al., 2013). The possible mechanisms of AS-IV that mediated kidney protection in the included studies are summed up as follows: (1) alleviating renal fibrosis by inhibiting the expression of TGF-β1 in renal tissues, further decreasing Smad2/3 and enhancing Smad7 levels to reduce the expression of α-SMA; (2) antioxidant action by increasing GSH, SOD, and CAT to reduce the release of MDA and enhancing Nrf2 to upregulate the expression of NQO1 and HO-1; (3) inhibiting apoptosis by downregulating the PERK-ATF4-CHOP pathway, Bax/Bcl-2 and the expression of caspase-3; in addition, inhibiting apoptosis in podocytes specifically by downregulating ILK and by upregulating integrins α3 and β1; by enhancing TUG1 and miR-378 and downregulating the expression of TRAF5; and by up-regulating APN; (4) reducing the proliferation of mesangial cells by regulating the Akt/mTOR pathways; (5) alleviating endoplasmic reticulum stress by enhancing the activity of SERCA and the expression of SERCA2, and reducing the activation of the PERK/eIF2α and IRE1/JNK pathways; (6) reducing the damage to mesangial cells by downregulating the Akt/NF-κB pathway; (7) inhibiting mitophagy by downregulating the PINK1/Parkin pathway; (8) inhibiting mitochondrial fission by reducing the expression of renal dynamin-related protein 1 (Drp-1), mitochondrial fission protein 1 (Fis-1), and mitochondrial fission factor (MFF); (9) inhibiting fusion of podocyte processes by downregulating p-130Cas; and (10) increasing autophagic activity of renal tissue cells by downregulating the PI3K/Akt/FoxO1 pathway (Figure 15).
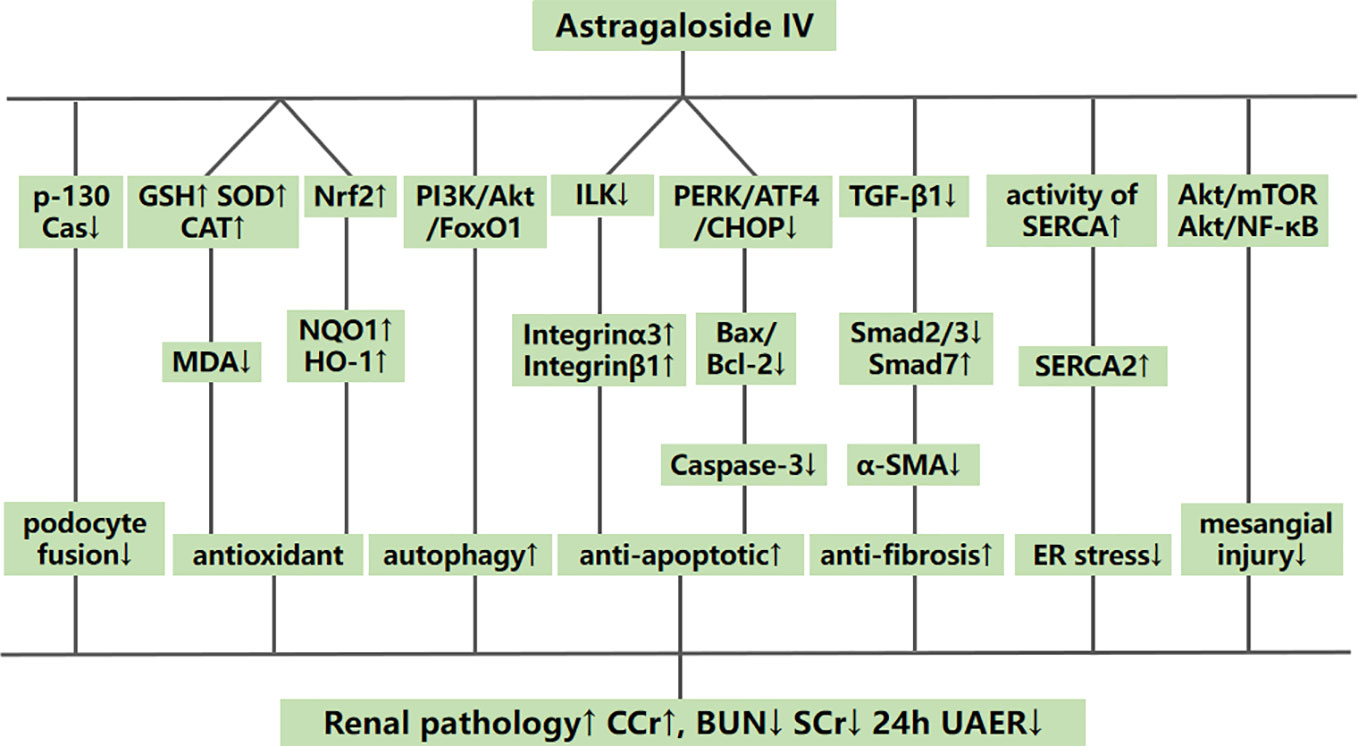
Figure 15 A schematic representation of possible renoprotective mechanisms of astragaloside IV for diabetic nephropathy.
Conclusion
Preclinical in vivo evidence suggests that AS-IV has renoprotective functions in DN, probably via antifibrotic, antioxidant, and antiapoptotic actions, resulting in alleviation of endoplasmic reticulum stress, inhibition of mitochondrial fission, and increased autophagic activity. Taken together, the findings suggest that AS-IV is a renoprotective candidate drug for treatment of DN.
Author Contributions
HW, ZZ, and Y-YH contributed equally to this work. QZ, HW, and Y-LW designed the study; HW, YJ, X-JL, and Z-ZZ collected the data; HW, Y-YH, and ZZ performed all analyses. All authors contributed to the article and approved the submitted version.
Funding
This work was supported by the Clinical Research Foundation of the 2nd Affiliated Hospital of Wenzhou Medical University (SAHoWMU-CR2018-01-105), Lin He’s New Medicine and Clinical Translation Academician Workstation Research Fund (17331208), Wenzhou Science and Technology Bureau Programs (H2015006 and Y20170322), and the Programs of Administration of Traditional Chinese Medicine in Zhejiang (2015ZB077 and 2018ZB080).
Conflict of Interest
The authors declare that the research was conducted in the absence of any commercial or financial relationships that could be construed as a potential conflict of interest.
References
Bjornstad, P., Cherney, D., Maahs, D. M. (2014). Early diabetic nephropathy in type 1 diabetes: new insights. Curr. Opin. Endocrinol. Diabetes Obes. 21 (4), 279–286. doi: 10.1097/MED.0000000000000074.
Brosius, F. C., Alpers, C. E., Bottinger, E. P., Breyer, M. D., Coffman, T. M., Gurley, S. B., et al. (2009). Mouse models of diabetic nephropathy. J. Am. Soc. Nephrol. 20, 2503–2512. doi: 10.1681/ASN2009070721
Chen, B. N., Chen, G. Y. (2016). A Preliminary Study on the Protection Mechanism of Astragaloside for Diabetic Nephropathy Rats. Yunnan J. Tradit. Chin. Med. Mater. Med. 37 (2), 58–60. doi: 10.16254/j.cnki.53-1120/r.2016.02.027.
Chen, J., Chen, Y., Luo, Y., Gui, D., Huang, J., He, D. (2014). Astragaloside IV ameliorates diabetic nephropathy involving protection of podocytes in streptozotocin induced diabetic rats. Eur. J. Pharmacol. 736, 86–94. doi: 10.1016/j.ejphar.2014.04.037
Chen, X., Wu, H., Chen, H., Wang, Q., Xie, X. J., Shen, J. (2019). Astragaloside VI Promotes Neural Stem Cell Proliferation and Enhances Neurological Function Recovery in Transient Cerebral Ischemic Injury via Activating EGFR/MAPK Signaling Cascades. Mol. Neurobiol. 56 (4), 3053–3067. doi: 10.1007/s12035-018-1294-3.
Du, N., Liu, S., Cui, C., Zhang, M., Jia, J., Cao, X. (2017). DMP-1 attenuates oxidative stress and inhibits TGF-β activation in rats with diabetic kidney disease. Ren. Fail. 39 (1), 229–235. doi: 10.1080/0886022X.2016.1256319.
Du, N., Xu, Z., Gao, M., Liu, P., Sun, B., Cao, X. (2018). Combination of Ginsenoside Rg1 and Astragaloside IV reduces oxidative stress and inhibits TGF-β1/Smads signaling cascade on renal fibrosis in rats with diabetic nephropathy. Drug Des. Devel. Ther. 12, 3517–3524. doi: 10.2147/DDDT.S171286.
Fan, Y., Fan, H., Zhu, B., Zhou, Y., Liu, Q., Li, P. (2019). Astragaloside IV protects against diabetic nephropathy via activating eNOS in streptozotocin diabetes-induced rats. BMC Complement. Altern. Med. 19, 355. doi: 10.1186/s12906-019-2728-9
Fernandez-Fernandez, B., Ortiz, A., Gomez-Guerrero, C. (2014). Therapeutic approaches to diabetic nephropathy-beyond the RAS. Nat. Rev. Nephrol. 10 (6), 325–346. doi: 10.1038/nrneph.2014.74.
Flyvbjerg, A. (2017). The role of the complement system in diabetic nephropathy. Nat. Rev. Nephrol. 13 (5), 311–318. doi: 10.1038/nrneph.2017.31.
Franco, A., Malhotra, N., Simonovits, G. (2014). Social science. Publication bias in the social sciences: unlocking the file drawer. Science 345 (6203), 1502–1505. doi: 10.1126/science.1255484.
Gu, Y., Wang, G., Pan, G. Y. (2004). Transport and bioavailability studies of astragaloside IV, an active ingredient in Radix astragali. Basic Clin. Pharmacol. Toxicol. 95, 295–298. doi: 10.1111/j.1742-7843.2004.t01-1-pto950508.x
Gui, D., Guo, Y., Wang, F., Liu, W., Chen, J., Chen, Y., et al. (2012). Astragaloside IV, a novel antioxidant, prevents glucose-induced podocyte apoptosis in vitro and in vivo. PloS One 7 (6), e39824. doi: 10.1371/journal.pone.0039824.
Gui, D., Huang, J., Guo, Y., Chen, J., Chen, Y., Xiao, W., et al. (2013a). Astragaloside IV ameliorates renal injury in streptozotocin-induced diabetic rats through inhibiting NF-κB-mediated inflammatory genes expression. Cytokine 61 (3), 970–977. doi: 10.1016/j.cyto.2013.01.008
Gui, D., Huang, J., Liu, W., Guo, Y., Xiao, W., Wang, N. (2013b). Astragaloside IV prevents acute kidney injury in two rodent models by inhibiting oxidative stress and apoptosis pathways. Apoptosis 18 (4), 409–422. doi: 10.1007/s10495-013-0801-2
Guo, H., Cao, A., Chu, S., Wang, Y., Zang, Y., Mao, X., et al. (2016). Astragaloside IV Attenuates Podocyte Apoptosis Mediated by Endoplasmic Reticulum Stress through Upregulating Sarco/Endoplasmic Reticulum Ca(2+)-ATPase 2 Expression in Diabetic Nephropathy. Front. Pharmacol. 21 (7), 500. doi: 10.3389/fphar.2016.00500.
Guo, H., Wang, Y., Zhang, X., Zang, Y., Zhang, Y., Wang, L., et al. (2017). Astragaloside IV protects against podocyte injury via SERCA2-dependent ER stress reduction and AMPKα-regulated autophagy induction in streptozotocin-induced diabetic nephropathy. Sci. Rep. 7 (1), 6852. doi: 10.1038/s41598-017-07061-7
Han, P. X., Song, G. F., Sun, H. L., Wang, W. J., Shao, M. M., Yu, X. W., et al. (2018). Astragaloside IV suppresses the renalase/Akt/mTOR signal pathway in streptozotocin-induced type 1 diabetic mice. Chongqing Med. 39 (22), 3299–3304. doi: 10.13820/j.cnki.gdyx.20181206.003.
Hayes, R. J., Bennett, S. (1999). Simple sample size calculation for cluster-randomized trials. Int. J. Epidemiol. 28 (2), 319–326. doi: 10.1093/ije/28.2.319
He, D. Y., He, J. G., Chen, Y. F., Zheng, Z. G. (2018). Astragaloside IV ameliorates diabetic nephropathy by inhibiting p-130Cas expression in podocytes. Zhejiang Med. J. 40 (14), 1532–1539. doi: 10.12056/j.issn.1006-2785.2018.40.14.2018-653
Hooijmans, C. R., Rovers, M. M., de Vries, R. B., Leenaars, M., Ritskes-Hoitinga, M., Langendam, M. W. (2014). SYRCLE’s risk of bias tool for animal studies. BMC Med. Res. Methodol. 14, 43. doi: 10.1186/1471-2288-14-43.
Huang, C. R., Wang, G. J., Wu, X. L., Li, H., Xie, H. T., Lv, H., et al. (2006). Absorption enhancement study of astragaloside IV based on its transport mechanism in caco-2 cells. Eur. J. Drug Metab. Pharmacokinet. 31, 5–10. doi: 10.1007/BF03190635
Huang, X. D., Shen, N., Lu, Q., Liu, W., Chang, Y., Fan, H. Y., et al. (2016). Effect of astragaloside on oxygen radical metabolism and expression of TGF-β1 mRNA in diabetic nephropathic rats. J. JiLin Univ. 42 (1), 48–53. doi: 10.13481/J.1671-587X.20160110.
Ju, Y., Su, Y., Chen, Q., Ma, K., Ji, T., Wang, Z., et al. (2019). Protective effects of Astragaloside IV on endoplasmic reticulum stress-induced renal tubular epithelial cells apoptosis in type 2 diabetic nephropathy rats. BioMed. Pharmacother. 109:, 84–92. doi: 10.1016/j.biopha.2018.10.041.
Kilkenny, C., Browne, W. J., Cuthill, I. C., Emerson, M., Altman, D. G. (2010). Improving bioscience research reporting: the ARRIVE guidelines for reporting animal research. J. Pharmacol. Pharmacother. 1, 94–99. doi: 10.4103/0976-500X.72351
Lei, X., Zhang, B. D., Ren, J. G., Luo, F. L. (2018a). Astragaloside suppresses apoptosis of the podocytes in rats with diabetic nephropathy via miR-378/TRAF5 signaling pathway. Life Sci. 206:, 77–83. doi: 10.1016/j.lfs.2018.905.037.
Lei, X., Zhang, L., Li, Z., Ren, J. (2018b). Astragaloside IV/lncRNA-TUG1/TRAF5 signaling pathway participates in podocyte apoptosis of diabetic nephropathy rats. Drug Des. Devel. Ther. 12, 2785–2793. doi: 10.2147/DDDT.S166525.
Li, Z., Zhang, P. H. (2016). Effect of Astragaloside IV on Kidney of Diabetic Nephropathy Rats via TGF-β/Smad Signaling Pathway. Guangdong Med. J. 37 (11), 1623–1628. doi: 10.13820/j.cnki.gdyx.20160613.004.
Li, Z. Q. (2018). Protective effect of astragaloside IV on early renal injury in diabetic rats and its mechanism, Anhui Medical University. Master’s thesis.
Like, A. A., Rossini, A. A. (1976). Streptozotocin- induced pancreatic insulitis: New model of diabetes mellitus. Science 193, 415–417. doi: 10.1126/science.180605
Liu, X., Wang, W., Song, G., Wei, X., Zeng, Y., Han, P., et al. (2017). Astragaloside IV ameliorates diabetic nephropathy by modulating the mitochondrial quality control network. PloS One 12 (8), e0182558. doi: 10.1371/journal.pone.0182558
Liu, C., Huang, S., Jiang, D. (2019). Effect of astragaloside combined with losartan on PI3K/Akt/NF-κB pathway in diabetic rat kidney. Cent. South Pharm. 17, 10. doi: 10.7539/j.issn.1672-2981.2019.10.008.
Lu, W. S., Li, S., Guo, W. W., Chen, L. L., Li, Y. S. (2015). Effects of Astragaloside IV on diabetic nephropathy in rats. Genet. Mol. Res. 14 (2), 5427–5434. doi: 10.4238/2015
Ma, K. K., Ju, Y. H., Chen, Q. Q., Li, W. Z., Li, W. P. (2019). Effect of Astragaloside IV on Regulation of PI3K/Akt/FoxO1 Signal in Kidney of Type 2 Diabetic Nephropathy Rats. Chin. J. Exp. Tradit. Med. Formulae 25 (2), 74–81. doi: 10.13422/j.cnki.syfjx.20190227.
Macleod, M. R., O’Collins, T., Howells, D. W., Donnan, G. A. (2004). Pooling of animal experimental data reveals influence of study design and publication bias. Stroke 35, 1203–1208. doi: 10.1161/01.STR.0000125719.25853.20.
Moher, D., Avey, M., Antes, G., Altman, D. G. (2015). Erratum: the national institutes of health and guidance for reporting preclinical research. BMC Med. 13, 1741–7015. doi: 10.1186/s12916-015-0321-8
National Kidney Foundation (2012). KDOQI Clinical Practice Guideline for Diabetes and CKD: 2012 Update. Am. J. Kidney Dis. 60 (5), 850–886. doi: 10.1053/j.ajkd.2012.07.005
Ran, L. H., Ma, H. Z. (2016). Protective effect of astragaloside IV on kidney of diabetic nephropathy rats. J. Shandong Univ. Tradit. Chin. Med. 17 (2), 22–24. doi: 10.3969/j.issn.1000-7369.2016.02.009
Rossini, A. A., Appel, M. C., Williams, R. M., Like, A. A. (1977). Genetic influence of the streptozotocin-induced insulitis and hyperglycemia. Diabetes 26, 916–920. doi: 10.2337/diab.26.10.916.
Saran, R., Li, Y., Robinson, B., Ayanian, J., Balkrishnan, R., Bragg-Gresham, J., et al. (2015). US renal data system 2014 annual data report: epidemiology of kidney disease in the united states. Am. J. Kidney Dis. 66 (3), 545. doi: 10.1053/j.ajkd.2015.05.001.
Sharma, K., McCue, P., Dunn, S. R. (2003). Diabetic kidney disease in the db/db mouse. Am. J. Physiol. Renal Physiol. 284 (6), F1138–F1144. doi: 10.1152/ajprenal.00315.2002
Sharma, D., Bhattacharya, P., Kalia, K., Tiwari, V. (2017). Diabetic nephropathy: New insights into established therapeutic paradigms and novel molecular targets. Diabetes Res. Clin. Pract. 128, 91–108. doi: 10.1016/j.diabres.2017.04.010
Song, Z., Rao, Y., Liu, Y., Xia, R., Xu, S., Yang, L. (2020). Protective Effects of Astragaloside IV on Podocyte Slit Diaphragm of the Rats with Diabetic Nephropathy. Western J. Tradit. Chin. Med. 33, 1. doi: 10.12174/j.issn.1004-6852.2020.01.04.
Sun, H., Wang, W., Han, P., Shao, M., Song, G., Du, H., et al. (2016). Astragaloside IV ameliorates renal injury in db/db mice. Sci. Rep. 6, 32545. doi: 10.1038/srep32545
Tesch, G. H., Lim, A. K. (2010). Recent insights into diabetic renal injury from the db/db mouse model of type 2 diabetic nephropathy. Am. J. Physiol. Renal Physiol. 300 (2), F301–F310. doi: 10.1152/ajprenal.00607.2010.
Van, L. J., Leenaars, M., Hooijmans, C., Wever, K., de, V. R., Ritskes-Hoitinga, M. (2013). Towards evidence-based translational research: the pros and cons of conducting systematic reviews of animal studies. ALTEX 30 (2), 256–257. doi: 10.14573/altex.2013.2.256.
Wang, Z. S., Xiong, F., Xie, X. H., Chen, D., Pan, J. H., Cheng, L. (2015). Astragaloside IV attenuates proteinuria in streptozotocin-induced diabetic nephropathy via the inhibition of endoplasmic reticulum stress. BMC Nephrol. 16, 44. doi: 10.1186/s12882-015-0031-7
Wang, X., Li, W., Kong, D. (2016). Cyclocarya paliurus extract alleviates diabetic nephropathy by inhibiting oxidative stress and aldose reductase. Ren. Fail. 38 (5), 678–685. doi: 10.3109/0886022X.2016.1155394
Wang, Y. N., Liu, Y. Q., Gao, J. X., Lin, C., Liu, N. G. (2017). Effects of Astragaloside IV on the Expression of TGF-β1, SMAD2/3 and α-SMA in Kidney Tissues of Diabetic KKAy Mice. Chongqing Med. 46 (5), 596–599. doi: 10.3969/J.ISSN.1671-8348.2017.05.007.
Keywords: astragaloside IV, rodent models, diabetic nephropathy, meta-analysis, systematic review
Citation: Wang H, Zhuang Z, Huang Y-Y, Zhuang Z-Z, Jin Y, Ye H-Y, Lin X-J, Zheng Q and Wang Y-L (2020) Protective Effect and Possible Mechanisms of Astragaloside IV in Animal Models of Diabetic Nephropathy: A Preclinical Systematic Review and Meta-Analysis. Front. Pharmacol. 11:988. doi: 10.3389/fphar.2020.00988
Received: 15 September 2019; Accepted: 18 June 2020;
Published: 30 June 2020.
Edited by:
Heike Wulff, University of California, Davis, United StatesReviewed by:
Jianping Chen, Guangzhou University of Chinese Medicine, ChinaRene Cardenas, National Autonomous University of Mexico, Mexico
Copyright © 2020 Wang, Zhuang, Huang, Zhuang, Jin, Ye, Lin, Zheng and Wang. This is an open-access article distributed under the terms of the Creative Commons Attribution License (CC BY). The use, distribution or reproduction in other forums is permitted, provided the original author(s) and the copyright owner(s) are credited and that the original publication in this journal is cited, in accordance with accepted academic practice. No use, distribution or reproduction is permitted which does not comply with these terms.
*Correspondence: Qun Zheng, MzQ0NDU3NTEyQHFxLmNvbQ==; Yi-Luan Wang, MjAyMDE2QHd6aGVhbHRoLmNvbQ==