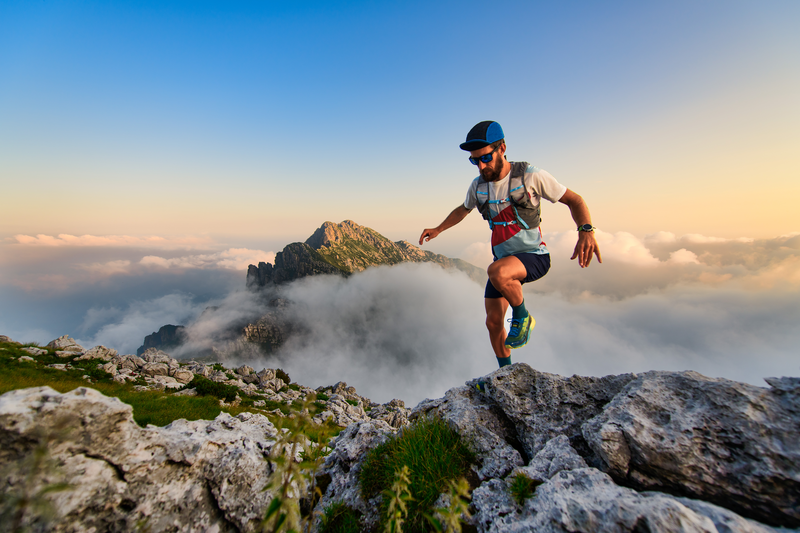
94% of researchers rate our articles as excellent or good
Learn more about the work of our research integrity team to safeguard the quality of each article we publish.
Find out more
BRIEF RESEARCH REPORT article
Front. Pharmacol. , 12 June 2020
Sec. Pharmacology of Ion Channels and Channelopathies
Volume 11 - 2020 | https://doi.org/10.3389/fphar.2020.00903
This article is part of the Research Topic Israel Ion Channel and Transporters Meeting 2019 View all 12 articles
Neuronal signals are processed along the nociceptive pathway to convey discriminative information, which would manifest in the produced pain sensation. The transient receptor potential vanilloid 1 (TRPV1), an important signaling complex in nociceptors termini, is activated by different noxious stimuli that underlie distinct pain sensations. For example, while endovanilloids are associated with inflammatory pain and hypersensitivity through TRPV1 activation, the exovanilloid toxin, capsaicin, evokes an acute pain by activating this channel. Differences in the TRPV1 activation profile evoked by exogenous and endogenous vanilloids were suggested to underlie this disparity in pain sensations. However, the cellular processes that lead to these differences in pain sensation mediated by the same channel are not fully understood. Here, we sought to describe the neuronal response of TRPV1-expressing nociceptors to exo-and endovanilloids. To this end, we performed current-clamp recordings in rat trigeminal neurons exposed to either capsaicin or intracellular endovanilloids produced downstream of the bradykinin receptor BK2. Our results show that lipoxygenase metabolites generate persistent TRPV1-dependent action potential firing while capsaicin evokes robust depolarization and high-frequency firing that is quickly terminated by depolarization block. Additionally, we found that a weak TRPV1 activation prolongs action potential firing. Overall, our results indicate different firing patterns evoked by inflammatory mediators and capsaicin via TRPV1 that correlate with the respective subsequent pain sensation. These findings also suggest that differences in neuronal activation stem from the variable degree of TRPV1 activation they produce.
TRPV1 has been identified as an essential component of the nociceptive mechanisms underlying different pain conditions and pain sensations (Davis et al., 2000; Patapoutian et al., 2009; Mishra et al., 2011; Fattori et al., 2016). This non-selective cation channel, which is mainly found in nociceptors is a polymodal receptor that detects a wide array of noxious stimuli such as heat (>42°C), protons (low pH), plant, and animal toxins, as well as bioactive lipids (Julius, 2013). TRPV1 activators engage diverse binding domains producing a modulatory effect on this multi-steric channel (Winter et al., 2013). One main binding domain is the intracellular vanilloid binding site (VBS), located between S3 and S4, with which both plant toxins and bioactive lipids interact (Jordt and Julius, 2002). Vanilloid plant toxins, such as the prototypical capsaicin (found in chili peppers), activating TRPV1, and produce acute pain (Yang et al., 2015). In contrast, TRPV1 bioactive lipids are endogenous vanilloids mainly produced during inflammation (Levine and Alessandri-Haber, 2007; Julius, 2013). This TRPV1 activation following injury was shown to be essential for heat hyperalgesia and pain hypersensitivity (Caterina et al., 2000; Shin et al., 2002). Thus, TRPV1 mediates both the acute burning pain following the ingestion of hot peppers and the dull and prolonged pain as well as hypersensitivity that often accompany inflammation.
The endovanilloid lipids, such as 12-Hydroeicosatetraenoic acid (12-HETE), are products of arachidonic acid (AA) metabolism by lipoxygenases (LOX), downstream of the Gq/GPCR pathway (Hwang et al., 2000; Starowicz et al., 2007; Veldhuis et al., 2015; Kumar et al., 2017). Thus, different components of the inflammatory soup such as bradykinin and histamine indirectly activate TRPV1 by inducing the Gq signaling cascade through their respective receptors co-expressed with TRPV1 in nociceptors (Shin et al., 2002; Shim et al., 2007). PKC is also activated in this signaling pathway and is essential for endovanilloid activity as it phosphorylates and sensitizes TRPV1 (Premkumar and Ahern, 2000; Kumar et al., 2017). Also, LOX metabolites are highly labile as they are quickly reduced to products with reduced potency (Hwang et al., 2000; Starowicz et al., 2007). Thus, endovanilloids are components of a highly regulated machinery essential for the initial recognition and subsequent healing of tissue injury, in contrast to exovanilloid toxins that provide the evolutionary advantage of evoking a deterring intense pain.
We previously showed that endovanilloids produced through Gq signaling evoke reduced open probability in TRPV1 and thus a weak channel activation in comparison to capsaicin (Kumar et al., 2017). The different properties of TRPV1 activation by exogenous and endogenous vanilloids were suggested to underlie the distinct pain sensations experienced during acute and inflammatory settings (Kumar et al., 2017). Indeed, it was suggested that while endovanilloids evoke prolonged action potential firing in nociceptors, exovanilloids produce a short burst of action potentials followed by a depolarization block, a cessation of firing despite suprathreshold membrane potential due to the inactivation of voltage-gated sodium (NaV) channels (Liu et al., 2001; Shin et al., 2002; Blair and Bean, 2003). However, these observations were obtained using different experimental settings for endo- and exovanilloids. Moreover, in both cases, recordings were performed in ruptured cells where intracellular signaling (i.e., Gq signaling) is disrupted. Thus, exactly how these two types of vanilloids exert a different effect in neurons remains to be elucidated.
Here, we sought to further describe the neuronal response of TRPV1 expressing nociceptors to exo- and endovanilloids. To this end, we performed electrophysiological recordings in perforated trigeminal neurons in which the intracellular signaling apparatus is preserved. We show that endovanilloids produced downstream to bradykinin receptor activation evoke prolonged action potentials firing, which is TRPV1 dependent, while capsaicin-evoked firing is short-lived. Firing evoked by bradykinin requires the generation of endovanilloids catalyzed by PLC and LOX in the Gq signaling pathway. Also, we found that the lower depolarization induced by endovanilliods could underlie the prolonged firing as lower capsaicin concentrations partially emulate this firing pattern. Overall, our results indicate that the highly regulated, reduced activation of TRPV1 by endovanilloids produced by LOX enables prolonged firing in nociceptors in contrast to the robust firing quickly followed by depolarization block obtained following the application of the highly efficacious capsaicin. Thus, these findings demonstrate that different neuronal activation patterns could be evoked through the same binding site in TRPV1, potentially encoding different pain sensations.
The Institutional Animal Care Committee of The Hebrew University approved all procedures that involved the use of animals. Acute culture of rat TG neurons (n = 42) was performed as previously described (Bohlen et al., 2010). In brief, dissected ganglia from two to four rat pups (P0–3) were collected in ice-cold DPBS (with Ca2+ and Mg2+) (Sigma-Aldrich, St. Louis, MO, USA), followed by digestion with 0.025% collagenase type IV (Worthington Biochemical Corp., Lakewood, NJ, USA) and then 0.25% Trypsin (Sigma-Aldrich, St. Louis, MO, USA). After enzymatic digestion, ganglia were triturated with fire-polished Pasteur glass pipettes of decreasing tip diameter. Cells were re-suspended in Dulbecco's modified Eagle's medium (DMEM, Gibco, Waltham, MA, USA) supplemented with 10% fetal bovine serum (FBS, Gibco), 1% penicillin-streptomycin (Gibco), and 25 mM 4-(2-hydroxyethyl)-1-piperazineethanesulfonic acid (HEPES; pH 7.3; Gibco, Waltham, MA, USA) (hereafter: complete DMEM) and plated on Poly-D-Lysine (PDL; Sigma, St. Louis, MO, USA) coated glass coverslips. Cells were allowed to recover for 3 h at 37°C in a 5% CO2 atmosphere before patch-clamp experiments. Dissociated rat TG neurons (n = 4) were infected with 0.5 ng/ml adeno-associated virus vector, serotype 2 (rAAV2) containing hSyn-HA-hM3D(Gq)-IRES-mCitrine or hSyn-EGFP (University of North Carolina Vector Core, Chapel Hill, NC, USA), in a complete media supplemented with 50 ng/ml nerve growth factor (NGF) (Alomone Labs, Jerusalem, Israel) and 1 ng/ml glial cell-derived neurotrophic factor (GDNF) (Alomone Labs, Jerusalem, Israel) for one week before electrophysiological analysis. Infected neurons were selected based on their fluorophore expression.
Membrane voltages were recorded under the current-clamp mode (I = 0) using an Axopatch 200B patch-clamp amplifier (Molecular Devices, Sunnyvale, CA, USA). Membrane voltages were digitized using a Digidata 1440A interface board and pCLAMP 10.6 software (Molecular Devices, Sunnyvale, CA, USA) at room temperature. Sampling frequency was set to 20 kHz and low-pass filtered at 5 kHz. Patch electrodes were fabricated from borosilicate glass using the P1000 Micropipette Puller (Sutter Instrument) and fire-polished using the Microforge MF-900 (Narishige, East Meadow, NY, USA) to a resistance of 2 to 4 MΩ for whole-cell and perforated patch recordings. Perforated patch or standard whole-cell configurations of the patch-clamp technique were obtained in voltage-clamp mode before proceeding to current-clamp recording mode. The perforated patch configuration was carried out as previously described (Priel and Silberberg, 2004). Briefly, the pipette solution constituted (mM): 75 K2SO4, 55 KCl, 5 MgSO4, 10 HEPES, adjusted to pH 7.2 with KOH. Nystatin (Sigma, St. Louis, MO, USA) was used for patch perforation at a working concentration of 180 µM. To this end, it was dissolved in Dimethyl Sulfoxide (DMSO; Sigma, St. Louis, MO, USA) to obtain a 55 mM stock solution, which following 1 min ultra-sonication was diluted in pipette solution to obtain a working solution of 180 µM. Nystatin solutions were freshly prepared in the dark every 2 h. Only cells with a series resistance of ≤15 MΩ were used for analysis. The extracellular solution contained (mM): 140 NaCl, 2.8 KCl, 2 MgSO4, 1.8 CaCl2, 10 HEPES, 10 D-glucose, adjusted to pH 7.4 with NaOH (Ringer solution). Once the perforated-patch or the standard whole-cell configuration was established, cells were continuously superfused with extracellular solutions via the ValveBank perfusion system (AutoMate Scientific, Berkeley, CA, USA). Cells were incubated with U73122 for 5 minutes before recording, whereas other drugs and chemicals were applied as indicated in the figures. For the standard whole-cell configuration pipette solution contained (mM): 130 potassium-gluconate (KC6H11O7), 2 MgSO4, 6 KCl, 10 NaCl, 10 HEPES, 5 MgATP, 0.5 Li2GTP, adjusted to pH 7.4 with KOH. Small TG neurons, (~25 μm), shown to be nociceptors based on their capsaicin-sensitivity (Cardenas et al., 1995; Le Pichon and Chesler, 2014) were selected for recording. Small TG neurons with stable (<10% variation) resting membrane potentials (≤−50 mV), action potentials with overshoots >40 mV, and amplitudes >85 mV were analyzed (Blair and Bean, 2003; Cummins et al., 2009; Gudes et al., 2015). Only neurons with a threshold of −35 ± 3 mV, determined according to the first action potential elicited under the fast current-clamp mode of a single ramp of depolarizing current injection (500 ms; 0–150 pA) (Gudes et al., 2015), were selected for analysis. In perforated current-clamp recordings, junction potential was calculated to be 9.3 mV (using Clampfit 10.6). The membrane potential was corrected accordingly. The phase plots of dV/dt vs. membrane voltage were formed by plotting the rate of change of membrane potential to time (dV/dt), following the application of bradykinin or capsaicin, as a function of membrane potential. The first two evoked APs in each cell were analyzed. Maximum upstroke velocity was obtained from (dV/dt) Max.
Recordings from TG neurons were performed as previously described (Kumar et al., 2016). Briefly, membrane currents were recorded under the voltage-clamp using an Axopatch 200B patch-clamp amplifier (Molecular Devices, Sunnyvale, CA, USA). Membrane currents were digitized using a Digidata 1440A interface board and pCLAMP 10.6 software (Molecular Devices, Sunnyvale, CA, USA) with sampling frequency set to 10 kHz and were low-pass filtered at 5 kHz. The holding voltage was −60 mV. All experiments were carried out at room temperature.
The electrophysiological analysis was performed using pCLAMP 10.6 software (Molecular Devices, Sunnyvale, CA, USA). All statistical data were calculated using SigmaPlot 11 (Systat Software, San Jose, CA, USA) and Prism 7 (Graphpad Software, La Jolla, CA, USA) software. Student's t-test and ANOVA were used to determine statistical significance.
All salts and buffers were purchased from Sigma-Aldrich (St. Louis, MO, USA). Capsaicin (Cap), Capsazepine (CPZ), U73122, and Bisindolylmaleimide I (BIM I; GF 109203X) were purchased from Tocris Bioscience (Bristol, UK). Bradykinin (Bk), Histamine dihydrochloride (His), Nordihydroguaiaretic acid (NDGA), Bupivacaine hydrochloride (bupivacaine), and Clozapine N-oxide (CNO) were purchased from Sigma-Aldrich (St. Louis, MO, USA). All drugs were dissolved according to manufacturer protocol.
Endo- and exovanilloids produce different activation profiles of TRPV1 expressed in a heterologous expression system (Kumar et al., 2017). To characterize the neuronal response to endogenous and exogenous vanilloids (i.e., capsaicin), we performed recordings in acutely dissociated trigeminal neurons from neonatal rats. In contrast to exovanilloids, endovanilloids are highly lipophilic, and thus their ability to cross the plasma membrane and reach the intracellular VBS in the TRPV1 channel when applied extracellularly is reduced as they can be trapped in the lipid bilayer (Hwang et al., 2000; Starowicz et al., 2007; Ursu et al., 2010). Under physiological conditions, endovanilloids were suggested to be LOX metabolites, produced downstream of Gq/GPCR (Hwang et al., 2000; Veldhuis et al., 2015). This pathway can be activated through receptors such as the bradykinin receptor BK2 and the histamine receptor H1 that were shown to co-express with TRPV1 (Carr et al., 2002; Shin et al., 2002; Pethő and Reeh, 2012). Thus, throughout this study, we sought to induce cell-autonomous endovanilloid production by activating the Gq/GPCR pathway using the inflammatory mediators bradykinin and histamine. Accordingly, we employed the perforated whole-cell configuration of the patch-clamp technique, which in contrast to the standard whole-cell configuration, preserves the cellular milieu and allows signaling events downstream of GPCR activation (Knauer and Yoshida, 2019). Indeed, in perforated patch recordings, 18 out of 27 TRPV1-expressing nociceptors responded to bradykinin, whereas only 1 out of 6 were bradykinin- sensitive in whole-cell configuration recordings (Figure 1A). Of note, six out of the 33 small diameter neurons recorded under the perforated configuration were sensitive to bradykinin but not to capsaicin. Also, just four of 36 capsaicin-responsive nociceptors responded to histamine in the perforated configuration. This low rate of co-expression is expected as H1R expression in TRPV1+ neurons is scarce, whereas TRPV1 is expressed in most H1R+ neurons (Roberson et al., 2013). As shown in Figures 1B, C, while exposure to bradykinin or histamine evoked a long train of action potentials, exposure to capsaicin (0.2 µM) resulted in a robust depolarization with few action potentials that were followed by an immediate depolarization block. While the persistent neuronal activation by bradykinin allowed a higher number of action potentials firing, capsaicin evoked a higher firing frequency (Figures 1D, E). Therefore, our analysis indicates that inflammatory mediators and capsaicin (0.2 µM) produce distinctive neuronal firing patterns as both bradykinin and histamine evoke a more prolonged neuronal activation, albeit with reduced firing rate in TRPV1-positive nociceptors as compared to capsaicin. In order to further analyze the TRPV1-induced firing patterns, we compared the rate of change of membrane voltage as a function of membrane voltage, dV/dt vs. V, between the first and second APs. As shown in Figure 2, we found that sequential capsaicin-evoked APs present a significant decrease in maximal upstroke velocity [(dV/dt)max]. As (dV/dt)max is determined by the number of available NaV channels (i.e., non-inactivated), this indicates that the fast and robust depolarization evoked by capsaicin causes the gradual inactivation of these channels until depolarization block is reached (Gudes et al., 2015). In contrast, bradykinin-evoked APs are mostly unchanged, suggesting the full recovery of NaV channels between APs.
Figure 1 Endo- and exovanilloids evoke distinct patterns of neuronal activation. (A) Bar diagram showing the average (± SEM) percentage of bradykinin (“Bk”; 0.5 µM) sensitive nociceptors (n = 6–27) at different configurations of the patch-clamp technique, normalized to the percentage of capsaicin (“Cap”; 0.2 µM) -sensitive cells. Sensitive cells were defined by the detection of agonist-evoked action potentials. Statistical significance between perforated whole-cell (“Perforated”; n = 27) and the whole-cell (“WC”; n = 6) configurations were determined using ANOVA with multiple comparisons, where *** represents p ≤ 0.001. (B) Representative current-clamp recording (I = 0) from rat TG neurons using the perforated whole-cell configuration in response to capsaicin (“Cap”; red bar) and bradykinin (“Bk”; cyan bar) (n = 12). Bradykinin (0.5 µM) was applied for 15 s followed by capsaicin (0.2 µM) application for 5 s. (C) Representative current-clamp recording (I = 0) from rat TG neurons using the perforated whole-cell configuration in response to capsaicin (“Cap”; red bar) and histamine (“His”; dark blue bar) (n = 4). Histamine (20 µM) was applied for 15 s followed by capsaicin (0.2 µM) application for 5 s. (D) Box and whiskers plot shows the number of evoked action potentials (APs (#)) in response to bradykinin (0.5 µM) or capsaicin (0.2 µM) (n = 12). ***p ≤ 0.001, paired Student t-test. (E) Box and whiskers plot shows the firing rate (Frequency) in response to bradykinin (0.5 µM) or capsaicin (0.2 µM) (n = 12). **p ≤ 0.01 paired Student's t-test.
Figure 2 Bradykinin-evoked activation of TRPV1 produces repetitive AP firing. (A) Top: Current-clamp recording (I = 0) from small-diameter dissociated rat trigeminal ganglion (TG) TRPV1+ neurons (P0) using the perforated whole-cell configuration of the patch-clamp technique. Representative trace of the first three APs following the application of bradykinin (“Bk”; cyan bar; 0.5 µM) is shown. Bottom: Phase plots of the rate of change of the membrane potential (dV/dt) during the first (black trace) and second (cyan trace) APs vs. membrane potential (V). (B) Top: Current-clamp recording (I = 0) from small-diameter dissociated rat trigeminal ganglion (TG) TRPV1+ neurons (P0) using the perforated whole-cell configuration of the patch-clamp technique. Representative trace of the first three APs following the application of capsaicin (“Cap”; red bar; 3 µM) is shown. Bottom: Phase plots of the rate of change of the membrane potential (dV/dt) during the first (black trace) and second (red trace) APs vs. membrane potential (V). (C) Mean/scatter-dot plot representing the difference in the maximal velocity (dV/dt) of the upstroke of the AP between the second and first APs evoked by either bradykinin (“Bk”; 0.5 µM; cyan circles) or capsaicin (“Cap”; red circles; 3 µM) (n = 5). ****p ≤ 0.0001, unpaired Student's t-test.
The bradykinin receptor, BK2, was previously shown to cause membrane depolarization by activating ion channels other than TRPV1 (Katanosaka et al., 2008). This includes the activation of ANO1, sensitization of TRPA1, and inhibition of Kv7 channels (Liu et al., 2010). However, as was previously shown by others (Shin et al., 2002), the application of the competitive TRPV1 antagonist, capsazepine, completely abolished action potential firing evoked by bradykinin as well as capsaicin (Figure 3A). Like other GPCRs, BK2 can activate various G protein signaling pathways (i.e., Gi, and Gq) (Miyamoto et al., 1997; Sandhu et al., 2019). In contrast, the engineered Gq-DREADD (hM3D(Gq)) receptor exclusively interacts with Gq proteins when it is bound to its selective activator, clozapine-N-oxide (CNO) (Roth, 2016). Nonetheless, in dissociated TRPV1-positive TG neurons infected with adeno-associated virus-carrying Gq-DREADD (hM3D(Gq)), CNO evoked a comparable response to that evoked by bradykinin in EGFP-infected neurons (Figure S1). Thus, activation of the promiscuous BK2 mimics that of the Gq-DREADD that specifically induces Gq signaling, suggesting that other pathways have a minor effect on BK-evoked nociceptive firing. Inhibiting PLC, which initiates the signaling cascade downstream Gq, by incubating neurons with U73122, produced an attenuated response to bradykinin while the capsaicin response remained intact (Figure 3B). This further demonstrates that the neuronal response to bradykinin is Gq-dependent. To confirm the role of endovanilloids, produced by LOX in the neuronal response to bradykinin, neurons were incubated with the broad LOX inhibitor, nordihydroguaiaretic acid (NDGA). Similarly to PLC inhibition, neurons in which LOX was inhibited presented a diminished response to bradykinin, whereas the response to capsaicin was unaffected (Figure 3C). Thus, neuronal activation by bradykinin is mediated by endovanilloids, which are LOX metabolites. Sensitization of TRPV1 by PKC phosphorylation was shown to be essential to the activation of the channel by endovanilloids (Bhave et al., 2003; Kumar et al., 2017). Indeed, as shown in Figure 3D, inhibition of PKC by bisindoylmaleimide I (BIM) resulted in a reduced action potential firing evoked by bradykinin, thus demonstrating that PKC-mediated sensitization potentiates the neuronal response to endovanilloids. Together, these results confirm that the bradykinin-evoked prolonged firing in these neurons occurs through the activation of TRPV1 by endovanilloids produced downstream of the Gq signaling pathway (Figure 3E).
Figure 3 Endovanilloids produced by LOX evoke TRPV1-dependent nociceptor firing. Current-clamp recordings (I = 0) from small-diameter dissociated rat trigeminal ganglion (TG) neurons (P0) using the perforated whole-cell configuration of the patch-clamp technique. (A) Representative recording in which bradykinin (0.5 µM) was applied for 15 s followed by capsaicin (1 µM) application for 20 s, in the continuous presence of capsazepine (“CPZ”; 40 µM; grey bar) (n = 6). (B) Representative recording in which bradykinin (0.5 µM) was applied for 15 s followed by capsaicin (0.2 µM) application for 5 s, in the continuous presence of U73122 (3 µM; bar) (n = 6). (C) Representative recording in which bradykinin (0.5 µM) was applied for 10 s followed by capsaicin (0.2 µM) application for 5 s, in the continuous presence of nordihydroguaiaretic acid (“NDGA”; 10 µM; bar) (n = 7). (D) Representative recording in which bradykinin (0.5 µM) was applied for 10 s followed by capsaicin (0.2 µM) application for 5 s, in the continuous presence of bisindolylmaleimide I (“BIM I”; 10 µM; grey bar) (n = 6). (E) Mean/scatter-dot plot representing the number of action potentials (APs) evoked by bradykinin while PLC (“PLCi”; dark grey circles), LOX (“LOXi”; light grey squares) or PKC (“PKCi”; grey triangles) are inhibited (n = 6–7). The dashed line indicates the average number of action potentials evoked by bradykinin in the absence of inhibitors.
The properties of membrane depolarization affect the activity of ion channels that participate in the generation of action potentials (Bean, 2007). To examine the possibility that the different patterns of vanilloids-mediated neuronal activation result from differences in membrane depolarization, we measured voltage changes in TG neurons exposed to bradykinin or capsaicin (Figure 4A). To prevent the generation of action potentials and depolarization evoked by voltage-gated sodium channels, nociceptors were treated with bupivacaine throughout the analysis (Scholz et al., 1998). As summarized in Figure 4B, while capsaicin evoked a robust membrane depolarization (ΔVcapsaicin = 52 ± 7 mV), similarly to previous reports (Liu et al., 2001; Blair and Bean, 2003), exposure to bradykinin resulted in a moderate response (ΔV bradykinin = 17 ± 5 mV). Therefore, our analysis indicates that endovanilloids evoke close-to-threshold neuronal depolarization, which is in contrast to the robust neuronal depolarization evoked by capsaicin. Thus, our findings indicate that endo- and exovanilloids induce different levels of neuronal membrane depolarization. The observed membrane depolarizations suggest the induction of different current amplitudes by endo- and exovanilloids. To test this possibility, we measured currents in TG neurons under voltage clamp and found that while capsaicin evoked a typical robust current, endovanilloids downstream of bradykinin evoke lower current amplitude (Figure 4C). To test whether lowering capsaicin concentration could mimic the endovanilloids -evoked response, we measured neuronal firing in response to saturating (2 µM), EC50 (0.2 µM), and sub-EC50 (0.02 µM) capsaicin concentrations (Hazan et al., 2015; Kumar et al., 2016). Of note, we previously showed that 0.02 µM is at the lower limit of capsaicin that evokes TRPV1 activation in rat TRPV1 (rTRPV1) (Figure S2) (Hazan et al., 2015). As shown in Figures 4D, E, lowering capsaicin concentration allowed the development of more action potentials. However, bradykinin application evoked ~3-fold more action potentials as compared to capsaicin, even when the latter was applied at a sub-EC50 concentration (compare Figure 1B to 4D and 1D to 4E). Importantly, for all analyzed capsaicin concentrations, depolarization block was reached. Therefore, our findings indicate that the different properties of endo- and exovanilloids, as well as their concentration, dictate the different neuronal response. Nonetheless, most exovanilloids produce depolarization block in neurons, while endovanilloids do not.
Figure 4 Endo- and exovanilloids evoke distinct neuronal membrane depolarization. (A) Representative current-clamp recording (I = 0) from rat TG neurons using the perforated whole-cell configuration in response to bradykinin (“Bk”; 1 µM; cyan bar and circles) that was applied for 15 s followed by capsaicin (“Cap”; 3 µM; red bar and squares) application for 15 s in the continuous presence of bupivacaine (300 µM; light grey bar) (n = 7). (B) Mean/scatter-dot plot representing the evoked membrane depolarization (“Mem. depo.”; ΔmV) in the presence of bupivacaine, as shown in A (n = 7). ***p ≤ 0.001, paired Student's t-test. (C) Representative perforated whole-cell recordings from a TG neuron at a holding potential of −60 mV. Cells were exposed to bradykinin (“Bk”; 0.5 µM; cyan bar) followed by applications of capsaicin (“Cap”; 1 µM; red bar) (n = 5). (D) Representative traces (n = 11–18) in which capsaicin (“Cap”) was applied for 20 s in sub-EC50 (0.02 µM; yellow; top trace), EC50 (0.2 µM; orange; middle trace) and saturating (2 µM; red; bottom trace) concentrations. (E) Box and whiskers plots are showing the mean number of evoked action potentials (“APs (#)”; upper) and membrane potential at the end of agonist application (as indicated by an arrow in D; bottom) in response to the different capsaicin concentrations (n = 11–18). **p ≤ 0.01 and ***p ≤ 0.001 and ns- no statistical significance, ANOVA with multiple comparisons.
Endo- and exovanilloids are associated with distinct pain sensations. Here, we show that these vanilloids promote different neuronal responses. Our findings indicate that while exovanilloids produce robust but acute neuronal firing, endovanilloids–evoked TRPV1 activation supports a prolonged neuronal response (Figure 1B). In this work, we applied the inflammatory mediator, bradykinin, to rat TG neurons in order to induce the biosynthesis of endovanilloids intracellularly. Also, electrophysiological recordings were performed in perforated neurons in which the intracellular environment required for the production of these TRPV1 activators is intact. This experimental design better simulates the physiological conditions in which endovanilloids activate TRPV1 in comparison to either the direct application of these bioactive lipids or recordings using configurations that cause the dilution of the intracellular milieu. In our experimental settings, endovanilloids evoke lower neuronal membrane depolarization, which is sufficient to evoke firing yet does not develop into the depolarization block associated with the potent exovanilloids (Figures 1B, 2, and 4A). The weaker depolarization evoked by endovanilloids reflects their reduced activation of TRPV1 in comparison to capsaicin (Figure 4C) (Kumar et al., 2017). Also, we found that the reduced TRPV1 activation evoked by low capsaicin concentrations produces longer firing reminiscent of the effect of endovanilloids. However, even in sub-EC50 capsaicin concentrations, the neuronal firing is eventually terminated by a depolarization block, suggesting that additional molecular mechanisms may underlie TRPV1-induced neuronal firing (Figure 4C). Thus, we propose that the different profiles of TRPV1 activation by endo- and exovanilloids and subsequent membrane depolarization may determine the duration, and potentially the intensity, of the evoked pain response.
Our results demonstrate that individual TG neurons can produce diverse firing patterns in response to different stimuli. Indeed, the different patterns of pain sensation evoked by endo- and exo-vanilloids may reflect their contradictory functions. The prototypical, exovanilloids, capsaicin, and resiniferatoxin (RTX), are phytotoxins believed to have evolved as plants' defense mechanism as they induce a robust response by harnessing central functional protein sites (such as the TRPV1 VBS) (Cromer and McIntyre, 2008; Bohlen et al., 2010; Cao et al., 2013). As such, exposure to these toxins produces acute and intense pain. In contrast, endovanilloids are essential components in the process through which tissue injury and inflammation are detected and resolved, which requires tight regulation of their concentration and activity (Hwang et al., 2000; Hylands-White et al., 2017; Kumar et al., 2017). Thus, we propose that while exo- and endovanilloids act through the same binding site in TRPV1, they produce distinct neuronal activations that underlie their different painful effects.
It is noteworthy that not all exovanilloids produce robust TRPV1 activation and pungency. Namely, capsaicin analogs were found to cause desensitization of sensory neurons without evoking a pungent sensation (Jancsó-Gábor et al., 1970). In addition, the synthetic exovanilloids, olvanil, arvanil, and SDZ249-665, were shown to evoke little or no pungency when applied to the eye in guinea pigs (Ursu et al., 2010). While the exact mechanism of this lack of pungency remains unclear, it was shown that non-pungent exovanilloids evoke slower kinetics of TRPV1 activation and depolarization, resulting in lower probability for AP generation (Ursu et al., 2010; Viana, 2011). The reduced rate of TRPV1 activation was suggested to stem from slow penetration through the membrane, further supporting our experimental design of inducing endovanilloid synthesis intracellularly (Ursu et al., 2010). Nonetheless, we show here that slowly developing depolarization induced by TRPV1 activation allows for the recovery of NaV channels, resulting in prolonged AP firing (Figures 2 and 4). Regardless, these findings demonstrate that the kinetics of the activation profile of TRPV1 is a significant factor affecting neuronal firing and consequent response.
We have previously shown that the submaximal activation of TRPV1 by endovanilloids stems from a low open probability of the channel compared to capsaicin–evoked activation (Kumar et al., 2017). Another TRPV1 activator which elicits reduced TRPV1 activation is the peptide spider toxin, double-knot toxin (DkTx), as it locks the channel in a sub-conducting state (Geron et al., 2018). The injection of DkTx-containing venom following this spider's bite produces prolonged pain that was attributed to the persistent activation of TRPV1 by this toxin (Liang, 2004; Bohlen et al., 2010). It is also possible that the lower TRPV1 activation by this toxin evokes weaker depolarization and avoidance of depolarization block that facilitates a continuous pain sensation. However, this spider's venom was also reported to produce an immediate intense pain similarly to capsaicin (Liang, 2004). While effects of the isolated DkTx on the neuronal and behavioral levels were not yet thoroughly tested, this toxin further demonstrates the ability of different TRPV1 activators to produce various activation profiles which may underlie distinct pain sensations.
Bradykinin affects ion channels other than TRPV1, such as ANO1 and Kv7 channels, to produce excitation in nociceptors (Liu et al., 2010). Nonetheless, as demonstrated in Figure 3A, the TRPV1 inhibitor, capsazepine, completely inhibits AP firing. Thus, at least in TRPV1+ neurons, TRPV1 activation by endovanilloids is necessary for the generation of AP firing. This does not preclude other effects induced by BK2 that may cause other changes in the firing properties of these neurons (Choi and Hwang, 2018). These effects produced by the bradykinin receptor are mainly Gq dependent, a signaling pathway that is also activated by other inflammatory mediators and is required for the endovanilloid activation of TRPV1 (Choi and Hwang, 2018). Thus, the effects of bradykinin on other ion channels are physiologically relevant and are part of TRPV1 activation by endovanilloids during inflammation.
Overall, our results suggest that the regulated activity of TRPV1 by endovanilloids allows a prolonged, low-frequency neuronal response. When toxins (such as capsaicin) bypass this regulation, neuronal membrane depolarization block is achieved, and the response is rapidly terminated. Thus, although capsaicin is a powerful tool to study this pivotal pain receptor, its activity may not reproduce the endogenous receptor response. The hydrophobicity of endovanilloids and the transient nature of their synthesis make them challenging to investigate (Starowicz et al., 2007). Nevertheless, a full understanding of TRPV1's physiological role necessitates studying its response to multiple endogenous stimuli. Elucidating the mechanisms through which inflammatory pain is generated may facilitate the identification of new targets as well as the development of new tools for pain management.
The datasets generated for this study are available on request to the corresponding author.
The animal study was reviewed and approved by Ethics Committee of the Hebrew University.
AP designed research. RK, MG, and AH performed research. RK, MG, AH, and AP analyzed data. MG and AP wrote the paper.
This work was supported by the Israel Science Foundation (Grant 1444/16 to AP), the Brettler Center and David R. Bloom Center, School of Pharmacy (The Hebrew University of Jerusalem; to AP), a Jerusalem Brain Committee Postdoctoral Fellowship (to RK) and the Paula Goldberg fellowship (to MG).
The authors declare that the research was conducted in the absence of any commercial or financial relationships that could be construed as a potential conflict of interest.
We thank the AP laboratory members for the insightful comments on the manuscript.
The Supplementary Material for this article can be found online at: https://www.frontiersin.org/articles/10.3389/fphar.2020.00903/full#supplementary-material
Bean, B. P. (2007). The action potential in mammalian central neurons. Nat. Rev. Neurosci. 8, 451–465. doi: 10.1038/nrn2148
Bhave, G., Hu, H.-J., Glauner, K. S., Zhu, W., Wang, H., Brasier, D. J., et al. (2003). Protein kinase C phosphorylation sensitizes but does not activate the capsaicin receptor transient receptor potential vanilloid 1 (TRPV1). Proc. Natl. Acad. Sci. U. S. A. 100, 12480–12485. doi: 10.1073/pnas.2032100100
Blair, N. T., Bean, B. P. (2003). Role of tetrodotoxin-resistant Na+ current slow inactivation in adaptation of action potential firing in small-diameter dorsal root ganglion neurons. J. Neurosci. 23, 10338–10350. doi: 10.1523/JNEUROSCI.23-32-10338.2003
Bohlen, C. J., Priel, A., Zhou, S., King, D., Siemens, J., Julius, D. (2010). A bivalent tarantula toxin activates the capsaicin receptor, TRPV1, by targeting the outer pore domain. Cell 141, 834–845. doi: 10.1016/j.cell.2010.03.052
Cao, E., Liao, M., Cheng, Y., Julius, D. (2013). TRPV1 structures in distinct conformations reveal activation mechanisms. Nature 504, 113–118. doi: 10.1038/nature12823
Cardenas, C. G., Mar, L. P. D. E. L., Scroggs, R. S., Del Mar, L. P. (1995). Variation in serotonergic inhibition of calcium channel currents in four types of rat sensory neurons differentiated by membrane properties. J. Neurophysiol. 74, 1870–1879. doi: 10.1152/jn.1995.74.5.1870
Carr, M. J., Kollarik, M., Meeker, S. N., Undem, B. J. (2002). A Role for TRPV1 in Bradykinin-Induced Excitation of Vagal Airway Afferent Nerve Terminals. J. Pharmacol. Exp. Ther. 304, 1275–1279. doi: 10.1124/jpet.102.043422
Caterina, M. J., Leffler, A., Malmberg, A. B., Martin, W. J., Trafton, J., Petersen-Zeitz, K. R., et al. (2000). Impaired nociception and pain sensation in mice lacking the capsaicin receptor. Science 288, 306–313. doi: 10.1126/SCIENCE.288.5464.306
Choi, S.-I., Hwang, S. W. (2018). Depolarizing Effectors of Bradykinin Signaling in Nociceptor Excitation in Pain Perception. Biomol. Ther. (Seoul) 26, 255–267. doi: 10.4062/biomolther.2017.127
Cromer, B., McIntyre, P. (2008). Painful toxins acting at TRPV1. Toxicon 51, 163–173. doi: 10.1016/j.toxicon.2007.10.012
Cummins, T. R., Rush, A. M., Estacion, M., Dib-Hajj, S. D., Waxman, S. G. (2009). Voltage-clamp and current-clamp recordings from mammalian DRG neurons. Nat. Protoc. 4, 1103–1112. doi: 10.1038/nprot.2009.91
Davis, J. B., Gray, J., Gunthorpe, M. J., Hatcher, J. P., Davey, P. T., Overend, P., et al. (2000). Vanilloid receptor-1 is essential for inflammatory thermal hyperalgesia. Nature 405, 183–187. doi: 10.1038/35012076
Fattori, V., Hohmann, M., Rossaneis, A., Pinho-Ribeiro, F., Verri, W. (2016). Capsaicin: Current Understanding of Its Mechanisms and Therapy of Pain and Other Pre-Clinical and Clinical Uses. Molecules 21, 844. doi: 10.3390/molecules21070844
Geron, M., Kumar, R., Zhou, W., Faraldo-Gómez, J. D., Vásquez, V., Priel, A. (2018). TRPV1 pore turret dictates distinct DkTx and capsaicin gating. Proc. Natl. Acad. Sci. 115, 201809662. doi: 10.1073/PNAS.1809662115
Gudes, S., Barkai, O., Caspi, Y., Katz, B., Lev, S., Binshtok, A. M. (2015). The role of slow and persistent TTX-resistant sodium currents in acute tumor necrosis factor-α-mediated increase in nociceptors excitability. J. Neurophysiol. 113, 601–619. doi: 10.1152/jn.00652.2014
Hazan, A., Kumar, R., Matzner, H., Priel, A. (2015). The pain receptor TRPV1 displays agonist-dependent activation stoichiometry. Sci. Rep. 5, 12278. doi: 10.1038/srep12278
Hwang, S. W., Cho, H., Kwak, J., Lee, S. Y., Kang, C. J., Jung, J., et al. (2000). Direct activation of capsaicin receptors by products of lipoxygenases: endogenous capsaicin-like substances. Proc. Natl. Acad. Sci. U. S. A. 97, 6155–6160. doi: 10.1073/pnas.97.11.6155
Hylands-White, N., Duarte, R. V., Raphael, J. H. (2017). An overview of treatment approaches for chronic pain management. Rheumatol. Int. 37, 29–42. doi: 10.1007/s00296-016-3481-8
Jancsó-Gábor, A., Szolcsányi, J., Jancsó, N. (1970). Irreversible impairment of thermoregulation induced by capsaicin and similar pungent substances in rats and guinea-pigs. J. Physiol. 206, 495–507. doi: 10.1113/jphysiol.1970.sp009027
Jordt, S.-E., Julius, D. (2002). Molecular Basis for Species-Specific Sensitivity to “Hot” Chili Peppers. Cell 108, 421–430. doi: 10.1016/S0092-8674(02)00637-2
Julius, D. (2013). TRP channels and pain. Annu. Rev. Cell Dev. Biol. doi: 10.1146/annurev-cellbio-101011-155833
Katanosaka, K., Banik, R. K., Giron, R., Higashi, T., Tominaga, M., Mizumura, K. (2008). Contribution of TRPV1 to the bradykinin-evoked nociceptive behavior and excitation of cutaneous sensory neurons. Neurosci. Res. 62, 168–175. doi: 10.1016/J.NEURES.2008.08.004
Knauer, B., Yoshida, M. (2019). Switching between persistent firing and depolarization block in individual rat CA1 pyramidal neurons. Hippocampus 29, 817–835. doi: 10.1002/hipo.23078
Kumar, R., Hazan, A., Basu, A., Zalcman, N., Matzner, H., Priel, A. (2016). Tyrosine Residue in TRPV1 Vanilloid Binding Pocket Regulates Deactivation Kinetics. J. Biol. Chem. 1997, 13855–13863. doi: 10.1074/jbc.M116.726372
Kumar, R., Hazan, A., Geron, M., Steinberg, R., Livni, L., Matzner, H., et al. (2017). Activation of transient receptor potential vanilloid 1 by lipoxygenase metabolites depends on PKC phosphorylation. FASEB J. 31, 1238–1247. doi: 10.1096/fj.201601132R
Le Pichon, C. E., Chesler, A. T. (2014). The functional and anatomical dissection of somatosensory subpopulations using mouse genetics. Front. Neuroanat. 8, 21. doi: 10.3389/fnana.2014.00021
Levine, J. D., Alessandri-Haber, N. (2007). TRP channels: Targets for the relief of pain. Biochim. Biophys. Acta - Mol. Basis Dis. 1772, 989–1003. doi: 10.1016/j.bbadis.2007.01.008
Liang, S. (2004). An overview of peptide toxins from the venom of the Chinese bird spider Selenocosmia huwena Wang [=Ornithoctonus huwena (Wang)]. Toxicon 43, 575–585. doi: 10.1016/j.toxicon.2004.02.005
Liu, L., Oortgiesen, M., Li, L., Simon, S. A. (2001). Capsaicin inhibits activation of voltage-gated sodium currents in capsaicin-sensitive trigeminal ganglion neurons. J. Neurophysiol. 85, 745–758. doi: 10.1152/jn.2001.85.2.745
Liu, B., Linley, J. E., Du, X., Zhang, X., Ooi, L., Zhang, H., et al. (2010). The acute nociceptive signals induced by bradykinin in rat sensory neurons are mediated by inhibition of M-type K+ channels and activation of Ca2+-activated Cl– channels. J. Clin. Invest. 120, 1240–1252. doi: 10.1172/JCI41084
Mishra, S. K., Tisel, S. M., Orestes, P., Bhangoo, S. K., Hoon, M. A. (2011). TRPV1-lineage neurons are required for thermal sensation. EMBO J. 30, 582–593. doi: 10.1038/emboj.2010.325
Miyamoto, A., Laufs, U., Pardo, C., Liao, J. K. (1997). Modulation of bradykinin receptor ligand binding affinity and its coupled G-proteins by nitric oxide. J. Biol. Chem. 272, 19601–19608. doi: 10.1074/jbc.272.31.19601
Patapoutian, A., Tate, S., Woolf, C. J. (2009). Transient receptor potential channels: targeting pain at the source. Nat. Rev. Drug Discovery 8, 55–68. doi: 10.1038/nrd2757
Pethő, G., Reeh, P. W. (2012). Sensory and Signaling Mechanisms of Bradykinin, Eicosanoids, Platelet-Activating Factor, and Nitric Oxide in Peripheral Nociceptors. Physiol. Rev. 92, 1699–1775. doi: 10.1152/physrev.00048.2010
Premkumar, L. S., Ahern, G. P. (2000). Induction of vanilloid receptor channel activity by protein kinase C. Nature 408, 985–990. doi: 10.1038/35050121
Priel, A., Silberberg, S. D. (2004). Mechanism of ivermectin facilitation of human P2X4 receptor channels. J. Gen. Physiol. 123, 281–293. doi: 10.1085/jgp.200308986
Roberson, D. P., Gudes, S., Sprague, J. M., Patoski, H. A. W., Robson, V. K., Blasl, F., et al. (2013). Activity-dependent silencing reveals functionally distinct itch-generating sensory neurons. Nat. Neurosci. 16, 910–918. doi: 10.1038/nn.3404
Roth, B. L. (2016). DREADDs for Neuroscientists. Neuron. 89 (4), 683–694. doi: 10.1016/j.neuron.2016.01.040
Sandhu, M., Touma, A. M., Dysthe, M., Sadler, F., Sivaramakrishnan, S., Vaidehi, N. (2019). Conformational plasticity of the intracellular cavity of GPCR-G-protein complexes leads to G-protein promiscuity and selectivity. Proc. Natl. Acad. Sci. U. S. A. 116, 11956–11965. doi: 10.1073/pnas.1820944116
Scholz, A., Kuboyama, N., Hempelmann, G., Vogel, W. (1998). Complex Blockade of TTX-Resistant Na + Currents by Lidocaine and Bupivacaine Reduce Firing Frequency in DRG Neurons. J. Neurophysiol. 79, 1746–1754. doi: 10.1152/jn.1998.79.4.1746
Shim, W.-S., Tak, M.-H., Lee, M.-H., Kim, M., Kim, M., Koo, J.-Y., et al. (2007). TRPV1 mediates histamine-induced itching via the activation of phospholipase A2 and 12-lipoxygenase. J. Neurosci. 27, 2331–2337. doi: 10.1523/JNEUROSCI.4643-06.2007
Shin, J., Cho, H., Hwang, S. W., Jung, J., Shin, C. Y., Lee, S.-Y., et al. (2002). Bradykinin-12-lipoxygenase-VR1 signaling pathway for inflammatory hyperalgesia. Proc. Natl. Acad. Sci. U. S. A. 99, 10150–10155. doi: 10.1073/pnas.152002699
Starowicz, K., Nigam, S., Di Marzo, V. (2007). Biochemistry and pharmacology of endovanilloids. Pharmacol. Ther. 114, 13–33. doi: 10.1016/j.pharmthera.2007.01.005
Ursu, D., Knopp, K., Beattie, R. E., Liu, B., Sher, E. (2010). Pungency of TRPV1 agonists is directly correlated with kinetics of receptor activation and lipophilicity. Eur. J. Pharmacol. 641, 114–122. doi: 10.1016/J.EJPHAR.2010.05.029
Veldhuis, N. A., Poole, D. P., Grace, M., McIntyre, P., Bunnett, N. W. (2015). The G protein-coupled receptor-transient receptor potential channel axis: molecular insights for targeting disorders of sensation and inflammation. Pharmacol. Rev. 67, 36–73. doi: 10.1124/pr.114.009555
Viana, F. (2011). Chemosensory properties of the trigeminal system. ACS Chem. Neurosci. 2, 38–50. doi: 10.1021/cn100102c
Winter, Z., Buhala, A., Otvös, F., Jósvay, K., Vizler, C., Dombi, G., et al. (2013). Functionally important amino acid residues in the transient receptor potential vanilloid 1 (TRPV1) ion channel – an overview of the current mutational data. Mol. Pain 9, 30. doi: 10.1186/1744-8069-9-30
Keywords: TRPV1, endovanilloids, exovanilloids, capsaicin, bradykinin, Gq- GPCR, nociceptors, inflammatory pain
Citation: Kumar R, Geron M, Hazan A and Priel A (2020) Endogenous and Exogenous Vanilloids Evoke Disparate TRPV1 Activation to Produce Distinct Neuronal Responses. Front. Pharmacol. 11:903. doi: 10.3389/fphar.2020.00903
Received: 13 February 2020; Accepted: 02 June 2020;
Published: 12 June 2020.
Edited by:
Antonio Ferrer-Montiel, Universidad Miguel Hernández de Elche, SpainReviewed by:
Felix Viana, Institute of Neurosciences of Alicante (IN), SpainCopyright © 2020 Kumar, Geron, Hazan and Priel. This is an open-access article distributed under the terms of the Creative Commons Attribution License (CC BY). The use, distribution or reproduction in other forums is permitted, provided the original author(s) and the copyright owner(s) are credited and that the original publication in this journal is cited, in accordance with accepted academic practice. No use, distribution or reproduction is permitted which does not comply with these terms.
*Correspondence: Avi Priel, YXZpcEBla21kLmh1amkuYWMuaWw=
†Present address: Rakesh Kumar, Zoology Department, Ravenshaw University, Cuttack, India
‡These authors have contributed equally to this work
Disclaimer: All claims expressed in this article are solely those of the authors and do not necessarily represent those of their affiliated organizations, or those of the publisher, the editors and the reviewers. Any product that may be evaluated in this article or claim that may be made by its manufacturer is not guaranteed or endorsed by the publisher.
Research integrity at Frontiers
Learn more about the work of our research integrity team to safeguard the quality of each article we publish.